- 1Department of Neuroscience, Erasmus MC Rotterdam, Rotterdam, Netherlands
- 2Princeton Neuroscience Institute, Princeton, NJ, United States
- 3Neuroscience Program, University of Illinois at Urbana-Champaign, Champaign, IL, United States
- 4Department of Animal Sciences, University of Illinois at Urbana-Champaign, Champaign, IL, United States
- 5Division of Nutritional Science, University of Illinois at Urbana-Champaign, Champaign, IL, United States
Introduction: Pigs have been an increasingly popular preclinical model in nutritional neuroscience, as their anatomy, physiology, and nutrition requirements are highly comparable to those of humans. Eyeblink conditioning is one of the most well-validated behavioral paradigms in neuroscience to study underlying mechanisms of learning and memory formation in the cerebellum. Eyeblink conditioning has been performed in many species but has never been done on young pigs. Therefore, our aim here was to develop and validate an eyeblink conditioning paradigm in young pigs.
Method: Eighteen intact male pigs were artificially reared from postnatal day 2–30. The eyeblink conditioning setup consisted of a sound-damping box with a hammock that pigs were placed in, which allowed the pig to remain comfortable yet maintain a typical range of head motion. In a delay conditioning paradigm, the conditional stimulus (CS) was a 550 ms blue light-emitting diode (LED), the unconditional stimulus (US) was a 50 ms eye air-puff, the CS-US interval was 500 ms. Starting at postnatal day 14, pigs were habituated for 5 days to the eyeblink conditioning setup, followed by 5 daily sessions of acquisition training (40 paired CS-US trials each day).
Results: The group-averaged amplitude of conditioned eyelid responses gradually increased over the course of the 5 days of training, indicating that pigs learned to make the association between the LED light CS and the air-puff US. A similar increase was found for the conditioned response (CR) probability: the group-averaged CR probability on session 1 was about 12% and reached a CR probability of 55% on day 5. The latency to CR peak time lacked a temporal preference in the first session but clearly showed preference from the moment that animals started to show more CRs in session 2 and onwards whereby the eyelid was maximally closed exactly at the moment that the US would be delivered.
Conclusion: We concluded that 3-week-old pigs have the capability of performing in a cerebellar classical conditioning task, demonstrating for the first time that eyeblink conditioning in young pigs has the potential to be a valuable behavioral tool to measure neurodevelopment.
Introduction
The use of pigs as an experimental animal model has been increasing in various fields, including neuroscience (Lind et al., 2007; Gieling et al., 2011; Kornum and Knudsen, 2011) and pediatric nutrition (Rytych et al., 2012; Liu et al., 2014; Fleming et al., 2019), for its several major advantages. First, pigs share gross neuroanatomical similarities to humans (Dickerson and Dobbing, 1967). Second, pigs are precocial in nature, which allows them to be weaned at birth, raised in controlled environments, and trained on behavioral paradigms early in life to assess various cognitive capacities, such as sensory discrimination, spatial learning and memory, and recognition memory (Friess et al., 2007; Wang et al., 2007; Fleming and Dilger, 2017). Third, young pigs have similar gastrointestinal anatomy, physiology, and nutrient requirements to infants, which makes them a great preclinical model for pediatric nutrition (Odle et al., 2014). For these reasons, the use of young pigs as a translational model in nutritional and developmental neuroscience is increasing (Mudd and Dilger, 2017).
In the field of nutritional and developmental neuroscience, pigs have been tested in a variety of behavioral tasks, including T- maze, radial arm maze, and novel object recognition (Bolhuis et al., 2004; Dilger and Johnson, 2010; Fleming and Dilger, 2017). Most of these tasks focus on the function of the hippocampus and/or the neocortex. However, pigs have not been extensively investigated in a task that focuses specifically on the cerebellar motor response in eyeblink conditioning. Therefore, our aim was to establish procedures to conduct Pavlovian eyeblink conditioning in pigs, which is a cerebellar-dependent learning task. During eyeblink conditioning, subjects typically hear a short beep or see a light flash (conditional stimulus, CS), followed several hundred milliseconds later by an air-puff on the eye (unconditional stimulus, US). In a cerebellar-dependent “delay paradigm”, the CS and US have different onset delays but co-terminate (Figures 1A,B). As a result of repeated CS-US pairings, subjects eventually associate the CS with US, and in anticipation of the US learn to close their eyes in response to the CS. This anticipatory behavior to close the eye after the CS but before the US is called the conditioned response (CR; Freeman and Steinmetz, 2011; Heck et al., 2013; ten Brinke et al., 2015). Eyeblink conditioning became a popular learning model because it is simple in its form but is discrete in that it specifically measures associative and sensory-motor learning (Heiney et al., 2014b). As a result, the neural circuits and plasticity mechanisms involved in eyeblink conditioning have been studied in very high detail (Figure 1C). Work done in many species, including humans (Cason, 1922; Oristaglio et al., 2013; Thürling et al., 2015), cats (Woody and Brozek, 1969), ferrets (Svensson et al., 1997), rabbits (for instance: Gormezano et al., 1962; McCormick et al., 1982), rodents (for instance: Boele et al., 2010; Heiney et al., 2014a, b; Albergaria et al., 2018), and sheep (Johnson et al., 2008), has pointed towards a crucial role for cerebellum during eyeblink conditioning. Purkinje cells in well-defined areas of the cerebellar cortex receive converging inputs from the mossy fiber–parallel fiber system, which conveys sensory CS signals, and input from a single climbing fiber, which transmits the instructive US signal (Yeo et al., 1985a,b,c; Yeo and Hesslow, 1998; Jirenhed et al., 2007; Mostofi et al., 2010; Heiney et al., 2014a; Steinmetz and Freeman, 2014; Halverson et al., 2015; Ohmae and Medina, 2015; ten Brinke et al., 2015; Thürling et al., 2015). During the conditioning process, various forms of synaptic and non-synaptic plasticity contribute to the acquisition of a well-timed suppression of Purkinje cell simple spike firing in response to the CS (Jirenhed et al., 2007; Gao et al., 2012; Halverson et al., 2015; Johansson et al., 2015; Ohmae and Medina, 2015; ten Brinke et al., 2015; Boele et al., 2016, 2018; Grasselli et al., 2020), herewith temporarily disinhibiting the cerebellar nuclei, which drive the overt eyeblink CR.
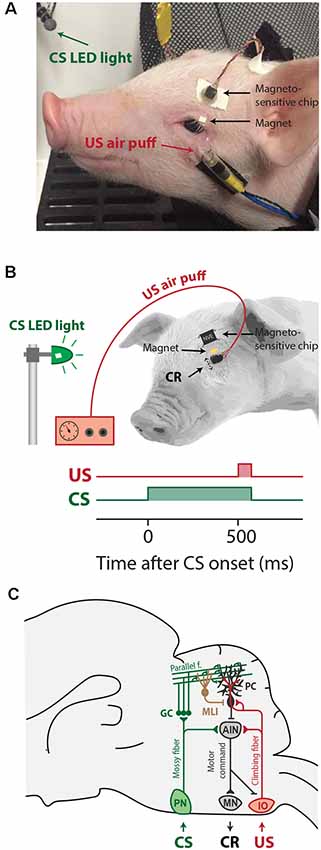
Figure 1. Overview of eyeblink conditioning apparatus, trial parameters, and neural circuitries involved in Pavlovian eyeblink conditioning (A) Apparatus in place for eyeblink conditioning trials. The CS was a blue light-emitting diode (LED) light and the US was a mild air-puff applied to the cornea. Since pigs tend to move their heads a lot during the experiment, the equipment needed to deliver the air-puff US and record the eyelid position was attached to the pig’s face, while the pig remained comfortably in a hammock. (B) Simplified illustration of the apparatus attachments and trial parameters of CS (green) and US (red). We used a delay eyeblink conditioning paradigm, whereby the start of the CS preceded the onset of the US by 500 ms, and both CS and US co-terminated at 550 ms after CS onset. (C) Simplified illustration of the neural circuits underlying delay eyeblink conditioning. The CS is transmitted via the mossy fiber–parallel fibers system (green) to Purkinje cells in well-defined microzones in the cerebellar cortex. The same Purkinje cells receive input from the climbing fibers transmitting the US signal (red). Memory formation takes place at this point of convergence of the CS and US in the cerebellar cortex. CRs are driven by the cerebellar output to brainstem motor neurons that innervate the eyelid musculature. Abbreviations: AIN, anterior interposed nucleus; CR, conditioned response; CS, conditional stimulus; IO, inferior olive; MLI, molecular layer interneuron; MN, brainstem motor neurons innervating the eyelid musculature (N. III, N. VI, N. VII); PN, pontine nuclei; UR, unconditioned response; US, unconditional stimulus.
To our knowledge, eyeblink conditioning has never been performed on young pigs. Still, eyeblink conditioning may be a suitable behavioral paradigm for the pig because it can accurately measure sensitive changes in behavioral, cognitive, and cerebellar development in early life, when other behavioral paradigms may not be applicable yet (Reeb-Sutherland and Fox, 2013). It can also be utilized as a valuable tool for studying neurodevelopmental disorders or nutritional challenges during this critical time-period.
Materials and Methods
Animal Housing and Care
All animal care and experimental procedures were in compliance with the National Research Council Guide for the Care and Use of Laboratory Animal Care and Use Committee and approved by the University of Illinois Urbana-Champaign Institutional Animal Care and Use Committee. Beginning on postnatal day (PND) 2, 18 naturally farrowed intact male pigs (n = 18) that were the offspring of Line 2 boars and Line 3 sows (A Large White and Landrace cross, also known as “Camborough”. Pig Improvement Company, Henderson, TN) were artificially reared to PND 30 and provided a nutritionally complete milk replacer formula (Purina ProNurse, Land O’Lakes Inc., Arden Hills, MN, USA). Pigs were individually housed in a custom artificial rearing system, which allowed the pigs to see, hear, and smell, but not touch the nearby pigs to closely control the individual cage environment (Mudd et al., 2016a). Pigs were fed ad libitum using an automated milk replacer delivery system that dispensed milk from 10:00 h to 06:00 h the next day. Lights were automatically turned on at 08:00 h and turned off at 20:00 h. Daily observations and pig body weights were recorded to track clinical indicators (e.g., diarrhea, lethargy, weight loss, or vomiting).
Eyeblink Conditioning System
Each eyeblink conditioning experimental setup consisted of a solid, sound-damping box with its inner dimension being (76.2 cm × 76.2 cm × 76.2 cm; length × width × height), and a custom-designed hammock securely attached approximately 30 cm above the bottom of the chamber, in which the pig rested during the experiment. A 20 mA blue light-emitting diode (LED) was attached inside the eyeblink chamber, approximately 10 cm from the anticipated location of the pig’s head. The blue hue was chosen because pigs are known to be capable of distinguishing blue (Tanida et al., 1993). This blue LED light was used as a conditional stimulus (CS). The eyeblink chamber was semi-dimmed, to ensure that pigs were able to see the light clearly. We chose a LED light as CS, since an auditory CS is known to elicit more alpha or eyelid startle responses in other species, including human infants (Boele et al., 2010; Goodman et al., 2018). Additionally, an airline connected to a regulator was attached to the pig’s head, about 3–5 cm below the left eye of each pig. The air puff was delivered at approximately 10 psi, and it was used as an unconditional stimulus (US). These pieces were attached using medical-grade surgical tape and glue (Figure 1A). Individual blinks were determined by measuring the distance between a giant magnetoresistance (GMR) magnetometer (NVE, Eden Prairie, MN) adhered to the pig’s forehead and a small magnet adhered to the pig’s eyelid (Koekkoek et al., 2002). The magnetic distance measurement technique (MDMT) was a good option for detecting the eyelid position in pigs. MDMT has the advantage that it provides a direct position or amplitude signal of the eyelid, and not, like EMG, muscular activity. MDMT was able to detect eyelid movements at high spatiotemporal resolution while putting minimal restraint on the pigs by allowing relatively free head movement. The instrumentation is also very small and lightweight and does not disturb the animal. We have explored utilizing infrared detectors; however, this signal was disturbed by reflections from the piglet’s pale skin and the long white eyelashes. Also, the amplitude of the signal was dependent upon the position of the animals relative to the LED light that was used as a CS. We have also used high-speed video recordings of the piglet’s face using Basler cameras. However, in order to do this reliably, one needs to install at least two cameras in the eyeblink chamber, since the pigs tend to move their head during the experiment, and good computer vision algorithms that are able to extract the eyelid position (similar to what we have developed for human EBC previously, see Bakker et al., 2020). One possibility, that we have not tried yet, are new ultrasmall high-speed video cameras, installed on the pig’s head (although these are still larger than the MDMT instrumentation). National Instruments (NI-PXI; Austin, TX) equipment was used to control experimental parameters and to acquire the eyelid position signal.
Trial Parameters
The overall behavioral experiment consisted of two phases: habituation and acquisition. The habituation phase started at PND 14 and lasted for five consecutive days. During the habituation phase, the pigs acclimated to resting in the hammock and the testing environment without receiving any stimuli for 15 min each day. Following the habituation phase, the acquisition phase started at PND 19 as lasted for five consecutive days. Knowing that different species show different learning speeds (Rasmussen, 2019), we expected pigs to reach asymptotic levels of conditioned responses in 150–200 trials within 4–5 days. During the acquisition phase, the pigs were subjected to 40 trials per day for 5 consecutive days. There were a total of five blocks of trials per day, and each block contained 1 US-only trial, six paired trials, and one CS-only trial. The first 500 ms of each trial was a baseline period, followed by the onset of CS (Figure 1B). The onset of US was 1,000 ms after the beginning of the trial with 500 ms inter-stimulus interval (ISI), and US and CS co-terminated at 1,050 ms after 50 ms of temporal overlap of CS and US. The inter-trial interval was determined by the following criteria: (1) a random duration between 8 and 12 sat the minimum must pass; (2) the eye must at least be half-open [i.e., fraction eyelid closure (FEC) must be equal or larger than 0.5]; and (3) the eyelid must be stable for at least 2 s A trained observer carefully monitored the pig and experimental parameters and adjusted equipment if necessary. The overall behavioral experiment started around the same time of the day, and a single handler stayed consistent for the entire experiment to minimize human handling bias.
Analysis of Eyeblink Conditioning Data
Individual eyeblink traces were analyzed with custom computer software and R (v. 4.0.4, R Core Team, Austria). For each type of trial (i.e., CS-only, US-only, CS-US paired), a single snippet was taken from the MDMT eyelid position signal. Each snippet, hereafter called an “eyeblink trace”, had a duration of 2,000 ms. Eyeblink traces were filtered in forward and reverse direction with a low-pass Butterworth filter using a cutoff frequency at 50 Hz. Trials with significant activity in the 500 ms pre-CS period [>7-times the interquartile range (IQR)] were regarded as invalid and disregarded for further analysis. Trials were normalized by aligning the 500 ms pre-CS baselines and normalizing the signal so that the size of a full blink was 1 FEC. This normalization was achieved by using the reflexive blinks to the air-puff (unconditioned responses, UR) as a reference.
For each session, we calculated the maximum value in the median eyelid trace plus one IQR, and data were normalized by dividing each trace by this value. As a consequence, in the normalized traces, an FEC of 1 corresponded with the eye being fully closed, an FEC of 0 corresponded with the eye being fully open. In valid normalized CS-US paired trials, all eyelid movements larger than 0.1 and with a latency to CR onset between 50 and 500 ms and a latency to CR peak between 150 and 500 ms (both relative to CS onset) were considered a CR (Figure 3B). For valid normalized CS-only trials we used the same criteria, except that the latency to CR peak had to range between 150–1,000 ms after CS onset (Figure 3C). Responses with earlier latencies have been suggested as alpha or “startle responses” and should not be considered as CR (Boele et al., 2010; Goodman et al., 2018), although it appeared in the end that pigs did not startle to the visual CS in our experiments. Additionally, we determined for each individual trial the following parameters: (1) FEC full interval: the maximum eyelid closure (= fraction eyelid closure) in the CS-US interval calculated over all valid trials wherein a CS was presented (CS-only and CS-US paired trials); (2) FEC at 500 ms: the maximum eyelid closure (= fraction eyelid closure) at the moment that the US is delivered (i.e., 500 ms after CS onset), calculated over all valid trials wherein a CS was presented (CS-only and CS-US paired trials); (3) FEC CR trials: the maximum eyelid closure (= fraction eyelid closure) in the CS-US interval calculated over the trials wherein a CR was present; (4) the latency to CR onset in trials wherein a CR was present; (5) the latency to CR peak in trials wherein a CR was present (Figure 3).
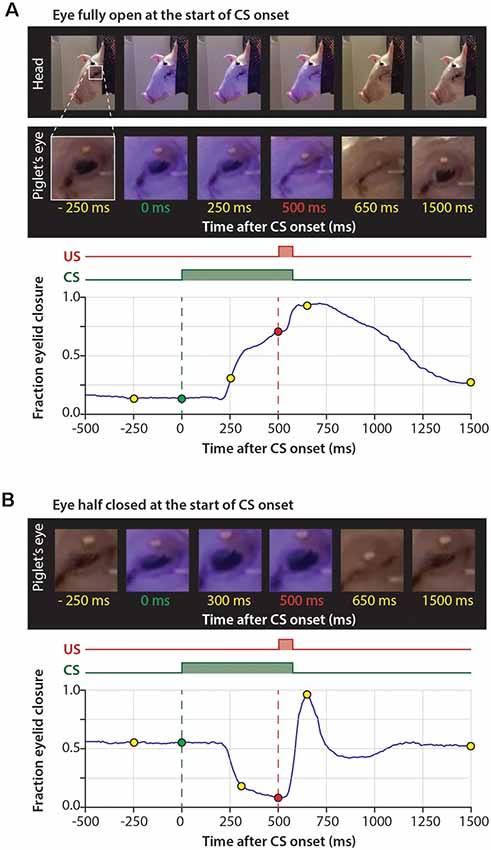
Figure 2. Series of pictures during a trial and corresponding time-points in the eyelid trace. (A) The eye is fully open at the onset of the CS and there is no reaction to the CS. (B) The eye is half-closed at the onset of the CS and the eye opens in response to the CS. The blue LED used as a CS is reflected on the skin of the pig. Abbreviations: CS, conditional stimulus; US, unconditional stimulus.
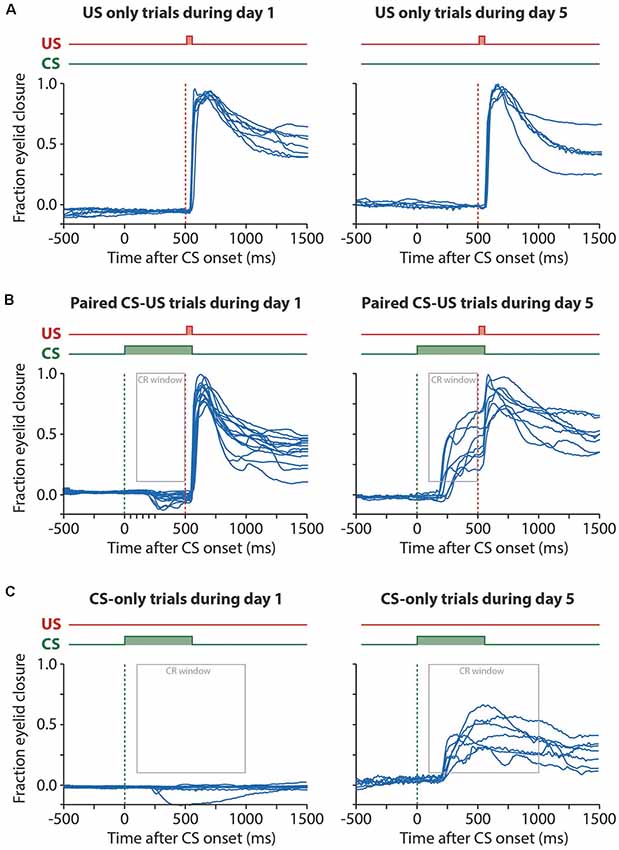
Figure 3. Example raw eyelid responses in US-only, paired CS-US, and CS-only trials during session 1 and day 5. For all panels, the onset of the CS is marked with a green dashed line and the onset of the US with a red dashed line. (A) US only trials showing similar reflexive eyeblink, or “unconditional responses (UR)”, to the air puff during session 1 and session 5. (B) Paired CS-US trials showing no CRs during session 1 and large CRs during session 5. Note that the US still leads to a UR at the end of the training, masking the full kinetic profile of the CRs. Also, note that at day 1 there is a significant further opening of the eyelid in response to the CS (see Figure 2). The gray rectangle indicates the window that was used as a CR criterion. (C) CS only trials showing no CRs during session 1 and large CRs during session 5. Note that in CS only trials the full kinetic profile of the eyeblink CR is visible. Abbreviations: CS, conditional stimulus; US, unconditional stimulus.
Because pigs responded with a partial eye-opening to the CS at the start of training, we quantified for the amplitude (or strength) of the eyelid closures in response to the CS using three different outcome measures: (1) maximum amplitude of the eyelid closure in 150–500 ms interval after CS onset calculated over all trials (FEC150–500); (2) amplitude of the eyelid closure at 500 ms after CS onset calculated over all trials (FEC500); and (3) maximum amplitude of the eyelid closure in the 150–500 ms interval after the onset of the CS calculated over only the trials wherein a CR was present (CRamplitude150–500).
Statistical analysis was done using multilevel linear mixed-effects (LME) models in R Studio (code available upon request). The LME has several major advantages over standard parametric and non-parametric tests (Aarts et al., 2014; Schielzeth et al., 2020), as they are more robust to violations of normality assumptions, which is often the case in biological data samples. Moreover, the use of LME models is able to accommodate the nested structure of our data (i.e., trial nested within session, session nested within animal, animal nested within group). Finally, LME models are objectively better at handling missing data points than repeated measures analysis of variance (ANOVA) models and do not require homoscedasticity as an inherent assumption. In our LME, we used the session as a fixed effect, and pig as a random effect (R code: lme (outcome ~ session_nr), control = ctrl, data = df1, random = ~ session_nr | pig_id, method = “REML”, na.action = na.exclude), Covariance structure: unstructured). The goodness of fit model comparison was determined by evaluating log likelihood ratio, BIC, and AIC values. The distribution of residuals was inspected visually by plotting the quantiles of standard normal vs. standardized residuals (i.e., Q-Q plots). Data were considered statistically significant if the p-value was smaller than 0.05.
Results
All pigs were trained for five consecutive days in the delay Pavlovian eyeblink conditioning test. The training protocol used the association of a 550-ms LED light flash as the CS, ending with a 50-ms air-puff delivered to the pig’s cornea as the US, which triggered the involuntary eyeblink response (i.e., UR).
Eye-Openings in Response to the Novel LED Serving as CS
When inspecting the raw eyeblink traces, we noticed that pigs often responded with a further opening of the eye (represented by a decrease below zero in fraction eyelid closure) in response to the CS during the first two training sessions (Figures 2A,B, 3, 5A). Examination of the MDMT signal and videos during the eyeblink conditioning test suggested that pigs often had the upper eyelid partly closed, whereby the upper eyelid was not naturally in the fully opened position. This partial eye closure was the neutral position of the pig’s eye and we confirmed that it was not artificially produced by instrumentation around the eye. As a consequence, the averaged eyeblink traces (Figure 5A) for testing days 1 and 2 show a clear eye-opening in response to the CS (see “Discussion” section).
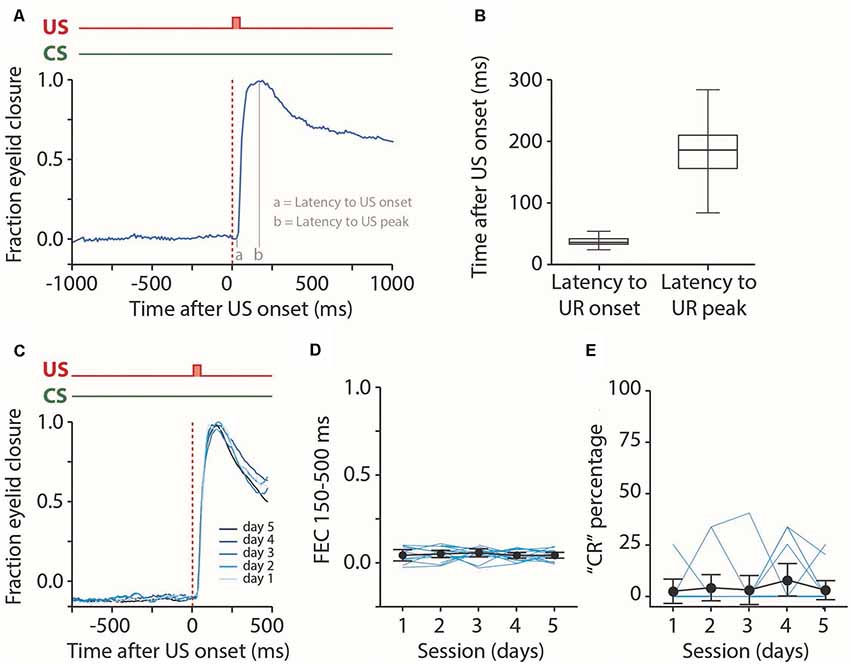
Figure 4. Analysis of reflexive blinks. (A) Raw eyeblink trace showing a reflexive blink, or unconditioned response (UR), to the puff of air applied to the pig’s eye. We analyzed the latency to UR onset and latency to UR peak. (B) Median latency to UR onset and latency to UR peak for all five sessions combined. No effect was found for the session (see Table 2). (C) Averaged eyeblink traces for each training session. A fraction eyelid closure (FEC) of 0 corresponds with full eye-opening, while an FEC of 1 corresponds with a full eyelid closure. (D,E) Individual pig learning curves (thin light blue lines) and group-averaged learning curve (thick black line) showing the FEC150–500 (D) and CR probability (E) as a function training session in US only trials. We used these values as a proxy for spontaneous blinks that could occur in the CS-US interval. No statistically significant effect of the session was found for FEC150–500 nor CR probability. Error bars indicate the 95% confidence interval (CI).
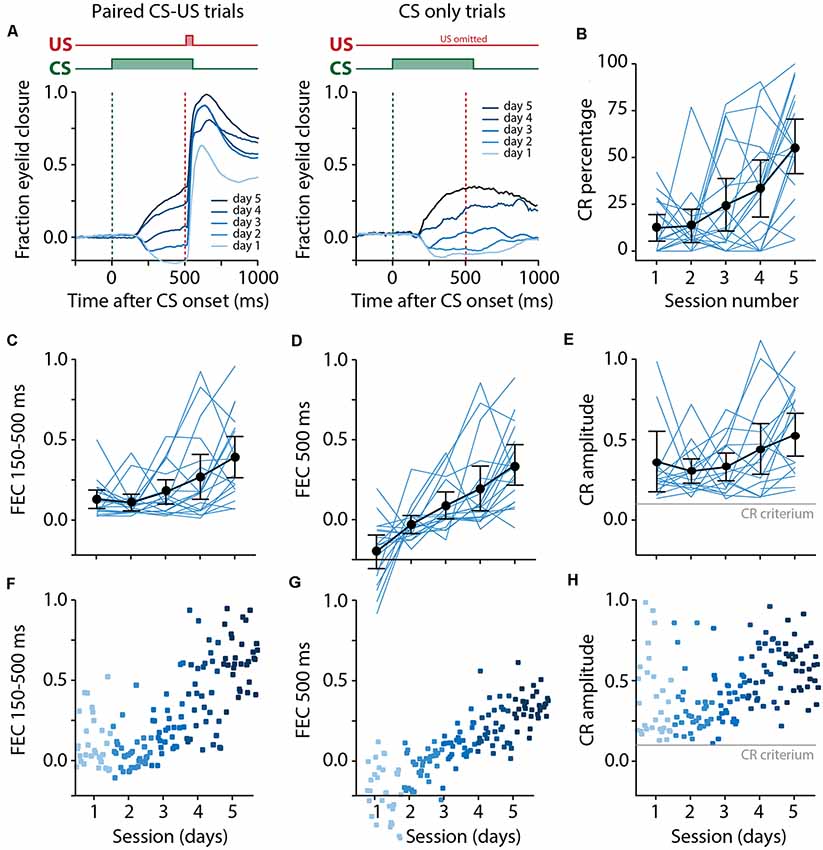
Figure 5. Pigs learn the eyeblink conditioning task, shown by a gradual increase of the CR probability and amplitude of eyelid responses to the CS. (A) Averaged eyeblink traces for each training session for CS-US trials (left) and CS only trials (right). A FEC of 0 corresponds with full eye-opening, while an FEC of 1 corresponds with a full eyelid closure. During the first and second training sessions, the averaged eyeblink traces exhibit a prominent eye-opening in response to the CS. Prolonged training (sessions 3–5) resulted in eyelid closures in response to the CS, which are considered as conditioned responses (CR). (B) Individual pig learning curves (thin light blue lines) and group-averaged learning curve (thick black line) showing the CR probability as a function training session. Error bars indicate the 95% confidence interval. A statistically significant effect of the session was found for CR probability. (C–E) Individual pig learning curves (thin light blue lines) and group-averaged learning curve (thick black line) as a function of session. Error bars indicate the 95% confidence interval. (F–H) Trial-by-trial values whereby each dot represents the average for all values for that trial. The colors (ranging from light blue to dark blue) correspond with the colors of the averaged eyeblink traces in panel (A). (C,F) The maximum amplitude of the eyelid closure in 150–500 ms interval after CS onset calculated over all trials (FEC150–500). A statistically significant effect of the session was found for FEC150–500. (D,G) The amplitude of the eyelid closure at 500 ms after CS onset calculated over all trials (FEC500). A statistically significant effect of the session was found for FEC500. (E,H) The maximum amplitude of the eyelid closure in 150–500 ms interval after the onset of the CS calculated over only the trials wherein a CR was present (CRamplitude150–500). A statistically significant effect of the session was found for CRamplitude150–500. For all statistical effects, please refer to Table 1. Abbreviations: CR, conditioned response; CS, conditional stimulus; FEC, fraction eyelid closure.
Reflex Blinks to the Eye Puff: Unconditioned Responses
First, we looked at the properties of the pig’s reflexive blinks to the air puff US (Figure 4A). We found that pigs had a median latency to UR onset of 30 ms after puff onset and a median latency to UR peak of 1,153 ms (Figure 4B). We found no significant main effect of session on latency to UR onset (F(4, 56) = 1.2, p = 0.34) nor latency to UR peak (F(4, 56) = 0.22, p = 0.92, ANOVA on LME, Table 2). Next, we looked at the occurrence of spontaneous, non-associative, eyeblink responses in the CS-US interval. For this, we used the exact same CR criteria that were used for paired CS-US trials. We found no significant main effect of session on CR percentage (F(4, 48) = 0.55, p = 0.69) nor with a FEC150–500 (F(4, 48) = 1.16, p = 0.34, ANOVA on LME, Figures 4C–E, Table 2). Thus, we conclude that the air puff reliably elicits unconditioned responses in pigs, that these URs stayed stable over time, and that the occurrence of spontaneous blinks was very low and did not change over time.
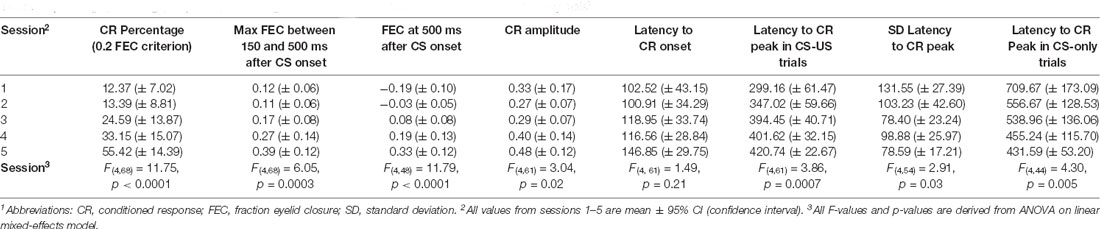
Table 1. Average percentage, amplitude, and timing of conditioned responses in paired CS-US and CS only trials1.
CR Probability and the Amplitude of Eyelid Responses to the CS
We found a significant main effect of day on CR probability (F(4, 68) = 11.75, p < 0.0001, ANOVA on LME; Figure 5B, Table 1). A CR was defined as an FEC larger than 0.1 (full closure is 1, full opening is 0) in the interval of 150–500 ms after CS onset. On average, pigs started with a probability of 12.37 [±7.02 95% confidence interval (CI)] on session 1 and reached a CR probability of 55.42 (±14.39 95% CI) on day 5 (Figure 5B, Table 1).
The averaged FEC150–500 showed a statistically significant effect of the session (F(4, 68) = 6.05, p = 0.0003, ANOVA on LME). On average, pigs started with a FEC150–500 of 0.12 (±0.06 95% CI) on session 1 and reached a value of 0.39 (±0.12 95% CI) on day 5 (Figures 5C–F, Table 1). Similarly, for FEC500 there was a main effect of the day (F (4, 48) = 11.79, p < 0.0001, ANOVA on LME). On average, pigs started with a FEC500 ms of −0.19 (±0.10 95% CI) on day 1 and reached a value of 0.33 (±0.12 95% CI) at the end of training (Figures 5D–G, Table 1). Note the negative value on day 1 for FEC500, reflecting the partial eyelid opening, that is not masked when only looking at the FEC150–500. Finally, we also found a small but significant main effect of the session for CRamplitude150–500 (F(4, 61) = 3.04, p = 0.02). On average, pigs started with a CRamplitude150–500 of 0.33 (±0.17 95% CI) on day 1 (although there were only a handful of CRs on day 1, most of them probably being spontaneous blinks that were indistinguishable from learned CRs) and ended with an CRamplitude150–500 of 0.48 (±0.12 95% CI; Figures 5E,H, Table 1).
Adaptive Timing of the Conditioned Responses
Next, we examined more closely the adaptive timing of the eyeblink CR over the course of training. For this, we looked at paired CS-US trials but also at CS-only trials since CS-only trials provide the full kinetic profile of the eyeblink CR. For CR timing, we quantified three different outcomes only in trials wherein a CR was present: (1) latency in milliseconds to the onset of eyelid CR in the interval between 150 and 500 ms after CS onset; (2) latency in milliseconds to the maximum peak of the CR in the interval between 150 and 500 ms after CS onset. Note that the onset of the air-puff US is at 500 ms after CS onset. In addition, we looked at the variability of the latencies to CR peak, since we observed in the raw traces that over the course of training the timing of these CR peaks became more precise, i.e., became more centered around the onset of the air-puff US.
The latency to CR peak time lacked a temporal preference in the first session, but clearly showed preference from the moment that animals started to show CRs more reliably in session 2 and onwards. In CS-only trials, the averaged latency to CR peak showed a statistically significant effect of the session (F(4, 44) = 4.30, p = 0.005, ANOVA on LME). On average, pigs started with a latency to CR peak of 709.67 (±173.09 95% CI) ms on session 1 and a value of 431.59 (±53.20 95% CI) ms on the last day of training (Figures 6A–C, Table 1), and herewith the eyelid was maximally closed exactly at the moment that the US would be delivered. In paired CS-US trials, we observed a similar phenomenon, whereby the averaged latency to CR peak showed a statistically significant effect of the session (F(4, 61) = 3.86, p = 0.007, ANOVA on LME). On average, pigs started with a latency to CR peak of 299.16 (±61.47 95% CI) ms on session 1 and a value of 420.74 (±22.67 95% CI) ms on the last day of training (Figures 6D–F, Table 1), and herewith the eyelid was maximally closed exactly at the moment that the US would be delivered. Note that the CR window for CS-only trials extended the duration of the CS-US interval, whereas the window for paired CS-US trials stopped at the US onset. Because we noted that variability became smaller over time, we also quantified the standard deviation of latency to CR peak for each session and each pig. The averaged standard deviation of CR peak time latencies showed a statistically significant effect of the session (F(4, 54) = 2.91, p = 0.03, ANOVA on LME). On day 1 we calculated an average standard deviation of 131.55 (±27.39 95% CI) milliseconds, and this value got gradually smaller, reaching a minimum value of 78.59 (±17.21 95% CI) on day 5 (Figure 4G, Table 1). The averaged latency to CR onset showed no statistically significant effect of the session (F(4, 61) = 1.49, p = 0.21, ANOVA on LME). On average, pigs started with a latency to CR onset of 102.52 ms (±43.15 95% CI) on session 1 and this value stayed relatively stable with a value of 146.85 (±29.75 95% CI) ms on session 5 (Figures 4H,I, Table 1). Based on these timing parameters of the eyeblink CR, we conclude that pigs were able to adaptively time their eyeblink CR.
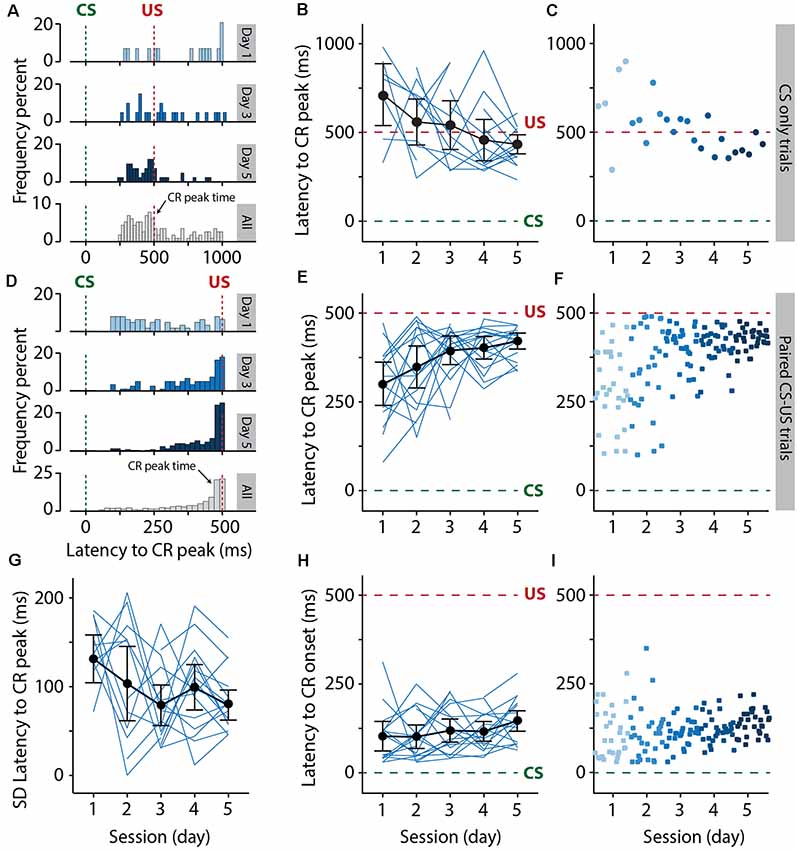
Figure 6. Pigs learn the eyeblink conditioning task, as shown by the adaptive timing of the conditioned eyelid responses. (A–C) Development of the distribution of the latency to CR peak from day 1 to day 5 in CS only trials. (D–F) Similar to (A–C) but now for paired CS-US trials. The green dashed line indicates CS onset and the red dashed line indicates US onset. (A–C) Histograms showing the distribution of latency to CR peak for day 1, 3, 5, and all days combined. (B–E) Individual pig learning curves (thin light blue lines) and group-averaged learning curve (thick black line) as a function of session. Error bars indicate the 95% confidence interval. (C–F) Trial-by-trial values whereby each dot represents the average of all animals for all values for that trial. The colors (ranging from light blue to dark blue) correspond with the colors of the averaged eyeblink traces in panel (A). Green dashed line indicates CS onset, red dashed line indicates US onset. The latency to CR peak time lacked a temporal preference in the first session, but clearly showed preference from the moment that animals started to show CR more reliably in session 2 and onwards. Note how the CR peaks concentrate around the onset of the US. A statistically significant effect of session was found for the latency to CR peak. (G) Standard deviation of the latency to CR peak as a function of session. Thin light blue lines represent individual pig curves and the thick black line is the group-averaged curve. Error bars indicate the 95% confidence interval. A statistically significant effect of session was found for the SD of the latency to CR peak, indicating that the variability in the CR peak times get smaller over time. (H,I) The latency in milliseconds to the onset of eyelid CR in the interval between 150 and 500 ms after CS onset. The latency to CR onset remained stable over time. No statistically significant effect of session was found for the latency to CR onset. For all statistical effects, please refer to Table 1. Abbreviations: CR, conditioned response; CS, conditional stimulus; SD, standard deviation, US, unconditional stimulus.
Discussion
The main purpose of the present study was to develop and validate the eyeblink conditioning paradigm in young pigs. We found that pigs were indeed able to learn the eyeblink conditioning task: both CR probability and the CR amplitude showed a gradual increase over the course of 5 days of training. Moreover, the eyeblink CRs were properly timed, in the sense that the eyelid was maximally closed exactly around the onset of the air-puff US, herewith providing the optimal protection against the aversive air-puff while perturbing the pig’s vision for the shortest amount of time. Thus, the eyeblink conditioning paradigm in young pigs can serve as a new neurobehavioral task to study the effect of nutrition on cerebellar development. In this study, we did not do an unpaired control condition. Thus, although theoretically speaking the increase in eyeblink responses to the CS can still be a non-associative process (pseudo-conditioning or sensitization to the tone), we believe that this is unlikely given the adaptive timing of eyeblink CRs (Figure 6) and absence of any increase in spontaneous blinks frequency before the US onset (Figures 4C–E).
Learning Rates and CS-US Interval
It is known that different species learn at different rates. Humans often need only a single session of 50 paired CS-US trials to learn the task (Knickmeyer et al., 2008; Thürling et al., 2015), for rabbits it often takes about 5–6 days of about 50 paired CS-US trials (Gormezano et al., 1962; Welsh and Harvey, 1989), and mice and rats are closer to 8–9 days of 100 paired CS-US trials a day to reach asymptotic levels of conditioning (Albergaria et al., 2018). Based on these findings, we estimated that it would require a pig to reach asymptotic levels of conditioning in 4–5 days. However, our data shows that 5 days of conditioning with 40 paired CS-US trials per day was not sufficient to reach these asymptotic levels. Future studies on pig eyeblink conditioning should consider using either more trials per day or more days of training, to reach higher values for CR probability and CR amplitude.
The duration of the CS-US interval has an effect on learning speed. Mice learn the delay eyeblink task the quickest at intervals close to 200 ms (Chettih et al., 2011; Heiney et al., 2014b). For humans and rabbits, intervals around 500 ms are commonly used and induce reliable conditioning. For that reason, we also chose an interval of 500 ms between CS and US onset for the pigs, and it appeared that pigs learned reasonably well in this interval, but it would be worth investigating other (i.e., shorter) CS-US intervals. It fell beyond the scope of this study to extensively investigate what the optimal interval is for pigs to learn the eyeblink conditioning task, so further investigation in this area is warranted.
Eye-Openings in Response to the Novel CS
As mentioned above, we observed that pigs often responded with a further opening of the eye in response to the CS during the first two training sessions. Examination of the MDMT signal and videos during the eyeblink conditioning test taught us that pigs often had the eyelid partly closed, whereby the upper eyelid was dropped down a bit. This partial eye closure was the neutral position of the pig’s eye and was not due to any instrumentation around the eye, since we observed the same eyelid position during the habituation sessions when there was no equipment attached to the pig’s face and even when the animals were just in their home cage. As a consequence, the averaged eyeblink traces for days 1 and 2 show a clear eye-opening in response to the CS. These eye-openings were not considered a CR, since they were even present from the start of training and even during the habituation sessions before any CS-US pairings had occurred. Whereas the language may be construed as speculative, we considered this phenomenon as a sign of the pig’s curiosity to the novel stimulus. A similar, but much more subtle, response has been reported in mice (Grasselli et al., 2020). Prolonged training of the pigs led to eyelid closures instead of openings in response to the CS, which reinforces the plastic nature of the response. These eyeblink closures, as seen on sessions 3, 4, and 5, were considered as true eyeblink CR.
Pigs as an Appropriate Model for Studies on Nutrition and Brain Development
Translating infancy to young pigs, 1 month in a pig’s life equates to roughly 1 year in a human’s life in terms of their total brain volume growth (Thibault and Margulies, 1998). Thus, our 4-to-5-week-old pigs can be considered as 4-to-5-month-old infants. Interestingly, infants aged 4–5 months, or even younger, have shown their capability of performing delay eyeblink conditioning (Lintz et al., 1967; Ivkovich et al., 1999; Herbert et al., 2003). During this critical period, delay eyeblink conditioning can serve as a sensitive behavioral paradigm to study cerebellar development.
As discussed above, the pigs have similar nutrient requirements and intestinal anatomy and physiology to those of humans (Odle et al., 2014), and these similarities allow the pigs to be utilized as an established preclinical and translational model, especially in nutritional neuroscience studies. Nutrition in neonatal pigs has profound and wide–ranging effects on neurodevelopment. Regarding cerebellar development, it has been demonstrated using Magnetic resonance imaging that an iron deficiency in pigs results in a decrease in relative cerebellum volume as they aged from PND 32 to 61, suggesting iron deficiency results in neurodevelopmental alterations of the cerebellum (Mudd et al., 2018).
While eyeblink conditioning has been suggested as a valuable biomarker for defining several neurological disorders such as fetal alcohol syndrome and autism spectrum disorder (Reeb-Sutherland and Fox, 2013), eyeblink conditioning has not been extensively utilized in nutritional developmental neuroscience. In this regard, we consider studies involving the direct effects of caffeine or alcohol on eyeblink conditioning performance as separate from nutritional intervention, and thus, believe they are not particularly useful for nutritional and developmental neuroscience. Only one study involving the effects of perinatal iron deficiency in rats employed eyeblink conditioning, where the nutrient deficiency elicited mild to severe impairments in eyeblink conditioning performance (McEchron et al., 2008). Thus, as nutritional deficiency and supplementation during early life can influence brain development and cognitive functions (Liu et al., 2014; Mudd et al., 2016b; Fleming et al., 2019), sensitive behavioral paradigms such as eyeblink conditioning in pigs can serve as valuable methodological tools to investigate how these dietary changes can influence cognitive and behavioral development.
Quiet Wakefulness
One of the noteworthy technical challenges with eyeblink conditioning in young pigs is controlling their alertness level. We observed pigs sometimes displayed squinting behaviors during the inter-trial intervals, and some pigs were even falling asleep. Incidents of a loss of alertness and falling asleep especially occurred in the latter part of the training (sessions 3, 4, 5). This is rather challenging to work with, as it may result in reduced reactions to the CS and US, resulting in decreased CR performance levels; therefore, the level of alertness can greatly influence the performance in eyeblink conditioning. This behavior is not exclusively present in young pigs, but it is a technical challenge that must be considered. It closely resembles the state of quiet wakefulness that has been reported for mice when the eyeblink conditioned task was performed in the home cage of the animal (Boele et al., 2010). The state of quiet wakefulness in mice is described as “the animal sitting quietly in the corner of its cage with its eyes partially closed”, and it is not thought to be an anxiety-related freezing behavior (Boele et al., 2010). In mice, it appeared that making the eyeblink task more engaging, by letting the animal walk on a treadmill system, for instance, was more successful than modifications such as mild food restrictions or delivery of short auditory cues to startle the animals. Similarly, in pigs, one might consider ways to make the eyeblink task more engaging, for instance by introducing a screen to watch a movie, similar to what is often done in human eyeblink conditioning (Manns et al., 2000a, b; Rasmussen and Jirenhed, 2017), or the placement of new objects for the animal to explore during the task.
Conclusion
As the use of the pig as a preclinical and translational model in nutritional neuroscience research has been gaining popularity, the development of sensitive behavioral paradigm for pigs has been critical to accurately measure changes in cognitive functions that may have been resulted from nutritional changes. Pigs that are 3 weeks of age are capable of performing eyeblink conditioning, as demonstrated by the CR percentage, the amplitude of the eyelid responses to the CS, and the improvement in the timing of their CR across sessions. However, 5 days of training with 40 paired trials per session does not appear to be sufficient to observe asymptotic learning. Overall, the current experiment was the first study to demonstrate that eyeblink conditioning in 3-week-old pigs may serve as a sensitive and valuable behavioral paradigm to measure cognitive development.
Data Availability Statement
The raw data supporting the conclusions of this article will be made available by the authors, without undue reservation.
Ethics Statement
The animal study was reviewed and approved by University of Illinois Urbana-Champaign Institutional Animal Care and Use Committee.
Author Contributions
Conceptualization: H-JB, SJ, AM, SF, SK, and RD. Methodology: H-JB, AM, SF, SK, JF, and RD. Investigation: H-JB, SJ, AM, SF, SK, and JF. Analysis: H-JB and AM. Visualization: H-JB. Funding acquisition: RD. Project administration: H-JB, SJ, and RD. Supervision: SK and RD. Writing—original draft: H-JB and SJ. Writing—review and editing: all authors. All authors contributed to the article and approved the submitted version.
Funding
This work was supported by Netherlands Organization for Scientific Research—Veni ZonMW, 91618112 (H-JB) and Erasmus MC Fellowship 106958 (H-JB). This work was partially supported by the United States Department of Agriculture National Institute of Food and Agriculture, Hatch project 1009051 (RD). The USDA funders had no role in study design, data collection and analysis, decision to publish, or preparation of the manuscript.
Conflict of Interest
The authors declare that the research was conducted in the absence of any commercial or financial relationships that could be construed as a potential conflict of interest.
Acknowledgments
We would like to thank Kristen Karkiewicz and Adam Jones for daily animal care.
Abbreviations
CS, Conditional stimulus; US, unconditional stimulus; CR, conditioned response; PND, postnatal day; MDMT, magnetic distance measurement technique; ISI, inter-stimulus interval; FEC, fraction eyelid closure; UR, unconditioned response; LME, linear mixed-effects models.
References
Aarts, E., Verhage, M., Veenvliet, J. V., Dolan, C. V., and Van Der Sluis, S. (2014). A solution to dependency: using multilevel analysis to accommodate nested data. Nat. Neurosci. 17, 491–496. doi: 10.1038/nn.3648
Albergaria, C., Silva, N. T., Pritchett, D. L., and Carey, M. R. (2018). Locomotor activity modulates associative learning in mouse cerebellum. Nat. Neurosci. 21, 725–735. doi: 10.1038/s41593-018-0129-x
Bakker, P., Boele, H.-J., Al-Ars, Z., and Strydis, C. (2020). Real-time face and landmark localization for eyeblink detection. arXiv [Preprint]. Available online at: https://arxiv.org/abs/2006.00816.
Boele, H. J., ten Brinke, M. M., and De Zeeuw, C. I. (2016). “Chapter 3 - Classical conditioning of timed motor responses: neural coding in cerebellar cortex and cerebellar nuclei,” in The Neuronal Codes of the Cerebellum ed Detlef H. Heck (Elsevier), 53–96.
Boele, H-J, Koekkoek, S. K. E., and De Zeeuw, C. I. (2010). Cerebellar and extracerebellar involvement in mouse eyeblink conditioning: the ACDC model. Front. Cell Neurosci. 3:19. doi: 10.3389/neuro.03.019.2009
Boele, Henk Jan, Peter, S., Ten Brinke, M. M., Verdonschot, L., Ijpelaar, A. C. H., Rizopoulos, D., Gao, Z., et al. (2018). Impact of parallel fiber to Purkinje cell long-term depression is unmasked in absence of inhibitory input. Sci. Adv. 4: eaas9426. doi: 10.1126/sciadv.aas9426
Bolhuis, J. E., Schouten, W. G. P., de Leeuw, J. A., Schrama, J. W., and Wiegant, V. M. (2004). Individual coping characteristics, rearing conditions and behavioural flexibility in pigs. Behav. Brain Res. 152, 351–360. doi: 10.1016/j.bbr.2003.10.024
Cason, H. (1922). The conditioned eyelid reaction. J. Exp. Psychol. 5, 153–196. doi: 10.1037/h0074822
Chettih, S. N., Mcdougle, S. D., Ruffolo, L. I., and Medina, J. F. (2011). Adaptive timing of motor output in the mouse: the role of movement oscillations in eyelid conditioning. Front. Integr. Neurosci. 5:72. doi: 10.3389/fnint.2011.00072
Dickerson, J. W. T., and Dobbing, J. (1967). Prenatal and postnatal growth and development of the central nervous system of the pig. Proc. R Soc. Lond. B Biol. Sci. 166, 384–395. doi: 10.1098/rspb.1967.0002
Dilger, R. N., and Johnson, R. W. (2010). Behavioral assessment of cognitive function using a translational neonatal piglet model. Brain Behav. Immun. 24, 1156–1165. doi: 10.1016/j.bbi.2010.05.008
Fleming, S. A., and Dilger, R. N. (2017). Young pigs exhibit differential exploratory behavior during novelty preference tasks in response to age, sex and delay. Behav. Brain Res. 321, 50–60. doi: 10.1016/j.bbr.2016.12.027
Fleming, S. A., Monaikul, S., Patsavas, A. J., Waworuntu, R. V., Berg, B. M., and Dilger, R. N. (2019). Dietary polydextrose and galactooligosaccharide increase exploratory behavior, improve recognition memory and alter neurochemistry in the young pig. Nutr. Neurosci. 22, 499–512. doi: 10.1080/1028415X.2017.1415280
Freeman, J. H., and Steinmetz, A. B. (2011). Neural circuitry and plasticity mechanisms underlying delay eyeblink conditioning. Learn. Mem. 18, 666–677. doi: 10.1101/lm.2023011
Friess, S. H., Ichord, R. N., Owens, K., Ralston, J., Rizol, R., Overall, K. L., et al. (2007). Neurobehavioral functional deficits following closed head injury in the neonatal pig. Exp. Neurol. 204, 234–243. doi: 10.1016/j.expneurol.2006.10.010
Gao, Z., Van Beugen, B. J., and De Zeeuw, C. I. (2012). Distributed synergistic plasticity and cerebellar learning. Nat. Rev. Neurosci. 13, 619–635. doi: 10.1038/nrn3312
Gieling, E. T., Nordquist, R. E., and van der Staay, F. J. (2011). Assessing learning and memory in pigs. Anim. Cogn. 14, 151–173. doi: 10.1007/s10071-010-0364-3
Goodman, L. K., Anstice, N. S., Stevens, S., Thompson, B., and Wouldes, T. A. (2018). Classical short-delay eyeblink conditioning in one-year-old children. J. Vis. Exp. 2018:58037. doi: 10.3791/58037
Gormezano, I., Schneiderman, N., Deaux, E., and Fuentes, I. (1962). Nictitating membrane: classical conditioning and extinction in the albino rabbit. Science 138, 33–34. doi: 10.1126/science.138.3536.33
Grasselli, G., Boele, H. J., Titley, H. K., Bradford, N., van Beers, L., Jay, L., et al. (2020). SK2 channels in cerebellar Purkinje cells contribute to excitability modulation in motor-learning-specific memory traces. PLoS Biol. 18:e3000596. doi: 10.1371/journal.pbio.3000596
Halverson, H. E., Khilkevich, A., and Mauk, M. D. (2015). Relating cerebellar Purkinje cell activity to the timing and amplitude of conditioned eyelid responses. J. Neurosci. 35, 7813–7832. doi: 10.1523/JNEUROSCI.3663-14.2015
Heck, D. H., De Zeeuw, C. I., Jaeger, D., Khodakhah, K., and Person, A. L. (2013). The neuronal code(s) of the cerebellum. J. Neurosci. 33, 17603–17609. doi: 10.1523/JNEUROSCI.2759-13.2013
Heiney, S. A., Kim, J., Augustine, G. J., and Medina, J. F. (2014a). Precise control of movement kinematics by optogenetic inhibition of Purkinje cell activity. J. Neurosci. 34, 2321–2330. doi: 10.1523/JNEUROSCI.4547-13.2014
Heiney, S. A., Wohl, M. P., Chettih, S. N., Ruffolo, L. I., and Medina, J. F. (2014b). Cerebellar-dependent expression of motor learning during eyeblink conditioning in head-fixed mice. J. Neurosci. 34, 14845–14853. doi: 10.1523/JNEUROSCI.2820-14.2014
Herbert, J. S., Eckerman, C. O., and Stanton, M. E. (2003). The ontogeny of human learning in delay, long-delay and trace eyeblink conditioning. Behav. Neurosci. 117, 1196–1210. doi: 10.1037/0735-7044.117.6.1196
Ivkovich, D., Collins, K. L., Eckerman, C. O., Krasnegor, N. A., and Stanton, M. E. (1999). Classical delay eyeblink conditioning in 4- and 5-month-old human infants. Psychol. Sci. 10, 4–8. doi: 10.1111/1467-9280.00097
Jirenhed, D. A., Bengtsson, F., and Hesslow, G. (2007). Acquisition, extinction and reacquisition of a cerebellar cortical memory trace. J. Neurosci. 27, 2493–2502. doi: 10.1523/JNEUROSCI.4202-06.2007
Johansson, F., Carlsson, H. A. E., Rasmussen, A., Yeo, C. H., and Hesslow, G. (2015). Activation of a temporal memory in Purkinje cells by the mGluR7 receptor. Cell Rep. 13, 1741–1746. doi: 10.1016/j.celrep.2015.10.047
Johnson, T. B., Stanton, M. E., Goodlett, C. R., and Cudd, T. A. (2008). Eyeblink classical conditioning in the preweanling lamb. Behav. Neurosci. 122, 722–729. doi: 10.1037/0735-7044.122.3.722
Knickmeyer, R. C., Gouttard, S., Kang, C., Evans, D., Wilber, K., Smith, J. K., et al. (2008). A structural MRI study of human brain development from birth to 2 years. J. Neurosci. 28, 12176–12182. doi: 10.1523/JNEUROSCI.3479-08.2008
Koekkoek, S. K. E., Den Ouden, W. L., Perry, G., Highstein, S. M., and De Zeeuw, C. I. (2002). Monitoring kinetic and frequency-domain properties of eyelid responses in mice with magnetic distance measurement technique. J. Neurophysiol. 88, 2124–2133. doi: 10.1152/jn.2002.88.4.2124
Kornum, B. R., and Knudsen, G. M. (2011). Cognitive testing of pigs (Sus scrofa) in translational biobehavioral research. Neurosci. Biobehav. Rev. 35, 437–451. doi: 10.1016/j.neubiorev.2010.05.004
Lind, N. M., Moustgaard, A., Jelsing, J., Vajta, G., Cumming, P., and Hansen, A. K. (2007). The use of pigs in neuroscience: modeling brain disorders. Neurosci. Biobehav. Rev. 31, 728–751. doi: 10.1016/j.neubiorev.2007.02.003
Lintz, L. M., Fitzgerald, H. E., and Brackbill, Y. (1967). Conditioning the eyeblink response to sound in infants. Psychon. Sci. 7, 405–406.
Liu, H., Radlowski, E. C., Conrad, M. S., Li, Y., Dilger, R. N., and Johnson, R. W. (2014). Early supplementation of phospholipids and gangliosides affects brain and cognitive development in neonatal piglets. J. Nutr. 144, 1903–1909. doi: 10.3945/jn.114.199828
Manns, J. R., Clark, R. E., and Squire, L. R. (2000a). Parallel acquisition of awareness and trace eyeblink classical conditioning. Learn. Mem. 7, 267–272. doi: 10.1101/lm.33400
Manns, J. R., Clark, R. E., and Squire, L. R. (2000b). Awareness predicts the magnitude of single-cue trace eyeblink conditioning. Hippocampus 10, 181–186. doi: 10.1002/(SICI)1098-1063(2000)10:2<181::AID-HIPO7>3.0.CO;2-V
McCormick, D. A., Clark, G. A., Lavond, D. G., and Thompson, R. F. (1982). Initial localization of the memory trace for a basic form of learning. Proc. Natl. Acad. Sci. U S A 79, 2731–2735. doi: 10.1073/pnas.79.8.2731
McEchron, M. D., Alexander, D. N., Gilmartin, M. R., and Paronish, M. D. (2008). Perinatal nutritional iron deficiency impairs hippocampus-dependent trace eyeblink conditioning in rats. Dev. Neurosci. 30, 243–254. doi: 10.1159/000110502
Mostofi, A., Holtzman, T., Grout, A. S., Yeo, C. H., and Edgley, S. A. (2010). Electrophysiological localization of eyeblink-related microzones in rabbit cerebellar cortex. J. Neurosci. 30, 8920–8934. doi: 10.1523/JNEUROSCI.6117-09.2010
Mudd, A. T., and Dilger, R. N. (2017). Early-life nutrition and neurodevelopment: use of the piglet as a translational model. Adv. Nutr. 8, 92–104. doi: 10.3945/an.116.013243
Mudd, A. T., Alexander, L. S., Berding, K., Waworuntu, R. V., Berg, B. M., Donovan, S. M., et al. (2016a). Dietary prebiotics, milk fat globule membrane and lactoferrin affects structural neurodevelopment in the young piglet. Front. Pediatr. 4:4. doi: 10.3389/fped.2016.00004
Mudd, A. T., Getty, C. M., Sutton, B. P., and Dilger, R. N. (2016b). Perinatal choline deficiency delays brain development and alters metabolite concentrations in the young pig. Nutr. Neurosci. 19, 425–433. doi: 10.1179/1476830515Y.0000000031
Mudd, A., Fil, J., Knight, L., Lam, F., Liang, Z.-P., and Dilger, R. (2018). Early-life iron deficiency reduces brain iron content and alters brain tissue composition despite iron repletion: a neuroimaging assessment. Nutrients 10:135. doi: 10.3390/nu10020135
Odle, J., Lin, X., Jacobi, S. K., Kim, S. W., and Stahl, C. H. (2014). The suckling piglet as an agrimedical model for the study of pediatric nutrition and metabolism. Annu. Rev. Anim. Biosci. 2, 419–444. doi: 10.1146/annurev-animal-022513-114158
Ohmae, S., and Medina, J. F. (2015). Climbing fibers encode a temporal-difference prediction error during cerebellar learning in mice. Nat. Neurosci. 18, 1798–1803. doi: 10.1038/nn.4167
Oristaglio, J., Hyman West, S., Ghaffari, M., Lech, M. S., Verma, B. R., Harvey, J. A., et al. (2013). Children with autism spectrum disorders show abnormal conditioned response timing on delay, but not trace, eyeblink conditioning. Neuroscience 248, 708–718. doi: 10.1016/j.neuroscience.2013.06.007
Rasmussen, A. (2019). “Why do species learn at different rates,” in Cerebellar Gordon Research Conference, July 14–19 (Les Diablerets, Switzerland). Poster Presentation.
Rasmussen, A., and Jirenhed, D. A. (2017). Learning and timing of voluntary blink responses match eyeblink conditioning. Sci. Rep. 7:3404. doi: 10.1038/s41598-017-03343-2
Reeb-Sutherland, B. C., and Fox, N. A. (2013). Eyeblink conditioning: a non-invasive biomarker for neurodevelopmental disorders. J. Autism Dev. Dis. 45, 376–394. doi: 10.1007/s10803-013-1905-9
Rytych, J. L., Elmore, M. R. P., Burton, M. D., Conrad, M. S., Donovan, S. M., Dilger, R. N., et al. (2012). Early life iron deficiency impairs spatial cognition in neonatal piglets. J. Nutr. 142, 2050–2056. doi: 10.3945/jn.112.165522
Schielzeth, H., Dingemanse, N. J., Nakagawa, S., Westneat, D. F., Allegue, H., Teplitsky, C., et al. (2020). Robustness of linear mixed-effects models to violations of distributional assumptions. Methods Ecol. Evol. 11, 1141–1152. doi: 10.1111/2041-210x.13434
Steinmetz, A. B., and Freeman, J. H. (2014). Localization of the cerebellar cortical zone mediating acquisition of eyeblink conditioning in rats. Neurobiol. Learn. Mem. 114, 148–154. doi: 10.1016/j.nlm.2014.06.003
Svensson, P., Ivarsson, M., and Hesslow, G. (1997). Effect of varying the intensity and train frequency of forelimb and cerebellar mossy fiber conditioned stimuli on the latency of conditioned eye-blink responses in decerebrate ferrets. Learn. Mem. 4, 105–115. doi: 10.1101/lm.4.1.105
Tanida, H., Senda, K., Suzuki, S., and Tanaka, T. (1993). Color discrimination in weanling pigs. Animal Sci. Technol. 62, 1029–1034.
ten Brinke, M. M., Boele, H. J., Spanke, J. K., Potters, J. W., Kornysheva, K., Wulff, P., et al. (2015). Evolving models of pavlovian conditioning: cerebellar cortical dynamics in awake behaving mice. Cell Rep. 13, 1977–1988. doi: 10.1016/j.celrep.2015.10.057
Thibault, K. L., and Margulies, S. S. (1998). Age-dependent material properties of the porcine cerebrum: effect on pediatric inertial head injury criteria. J. Biomech. 31, 1119–1126. doi: 10.1016/s0021-9290(98)00122-5
Thürling, M., Kahl, F., Maderwald, S., Stefanescu, R. M., Schlamann, M., Boele, H. J., et al. (2015). Cerebellar cortex and cerebellar nuclei are concomitantly activated during eyeblink conditioning: a 7T fMRI study in humans. J. Neurosci. 35, 1228–1239. doi: 10.24875/RIC.21000152
Wang, B., Yu, B., Karim, M., Hu, H., Sun, Y., Mcgreevy, P., et al. (2007). Dietary sialic acid supplementation improves learning and memory in piglets 1-3. Am. J. Clin. Nutr. 85, 561–569. doi: 10.1093/ajcn/85.2.561
Welsh, J. P., and Harvey, J. A. (1989). Cerebellar lesions and the nictitating membrane reflex: performance deficits of the conditioned and unconditioned response. J. Neurosci. 9, 299–311. doi: 10.1523/JNEUROSCI.09-01-00299.1989
Woody, C. D., and Brozek, G. (1969). Changes in evoked responses from facial nucleus of cat, with conditioning and extinction of an eve blink. J. Neurophysiol. 32, 717–726. doi: 10.1152/jn.1969.32.5.717
Yeo, C. H., Hardiman, M. J., and Glickstein, M. (1985a). Classical conditioning of the nictitating membrane response of the rabbit - II. lesions of the cerebellar cortex. Exp. Brain Res. 60, 99–113. doi: 10.1007/BF00237023
Yeo, C. H., Hardiman, M. J., and Glickstein, M. (1985b). Classical conditioning of the nictitating membrane response of the rabbit - III. connections of cerebellar lobule HVI. Exp. Brain Res. 60, 114–126. doi: 10.1007/BF00237024
Yeo, C. H., Hardiman, M. J., and Glickstein, M. (1985c). Classical conditioning of the nictitating membrane response of the rabbit - I. lesions of the cerebellar nuclei. Exp. Brain Res. 60, 87–98. doi: 10.1007/BF00237022
Keywords: pig, eyeblink conditioning, cerebellar learning, associative learning, nutrition
Citation: Boele H-J, Joung S, Fil JE, Mudd AT, Fleming SA, Koekkoek SKE and Dilger RN (2021) Young Domestic Pigs (Sus scrofa) Can Perform Pavlovian Eyeblink Conditioning. Front. Behav. Neurosci. 15:690019. doi: 10.3389/fnbeh.2021.690019
Received: 01 April 2021; Accepted: 04 June 2021;
Published: 29 June 2021.
Edited by:
Kevin D. Beck, Rutgers, The State University of New Jersey, United StatesReviewed by:
Michael Todd Allen, University of Northern Colorado, United StatesJohn Green, University of Vermont, United States
Copyright © 2021 Boele, Joung, Fil, Mudd, Fleming, Koekkoek and Dilger. This is an open-access article distributed under the terms of the Creative Commons Attribution License (CC BY). The use, distribution or reproduction in other forums is permitted, provided the original author(s) and the copyright owner(s) are credited and that the original publication in this journal is cited, in accordance with accepted academic practice. No use, distribution or reproduction is permitted which does not comply with these terms.
*Correspondence: Ryan N. Dilger,cmRpbGdlcjJAaWxsaW5vaXMuZWR1