- 1Department of Anesthesia and Perioperative Medicine, University of Manitoba, Winnipeg, MB, Canada
- 2University of Manitoba, Winnipeg, MB, Canada
- 3Health Sciences Centre, Winnipeg, MB, Canada
- 4Canada North Concussion Network, Winnipeg, MB, Canada
- 5Department of Surgery, University of Manitoba, Winnipeg, MB, Canada
- 6Department of Pediatrics and Child Health, University of Manitoba, Winnipeg, MB, Canada
- 7Section of Neurosurgery, University of Manitoba, Winnipeg, MB, Canada
- 8Pan Am Concussion Program, Winnipeg, MB, Canada
- 9Children’s Hospital Research Institute of Manitoba, University of Manitoba, Winnipeg, MB, Canada
- 10Pan Am Clinic Foundation, Winnipeg, MB, Canada
- 11Department of Radiology, University of Manitoba, Winnipeg, MB, Canada
- 12College of Medicine, University of Manitoba, Winnipeg, MB, Canada
- 13Department of Community Health Sciences, University of Manitoba, Winnipeg, MB, Canada
- 14Department of Medical Imaging, University of Toronto, Toronto, ON, Canada
- 15University Health Network Cerebrovascular Reactivity Research Group, Toronto, ON, Canada
- 16Department of Physiology, University of Toronto, Toronto, ON, Canada
- 17University of Toronto, Toronto, ON, Canada
- 18Department of Anesthesia, University of Toronto, Toronto, ON, Canada
Background: Advanced neuroimaging studies in concussion have been limited to detecting group differences between concussion patients and healthy controls. In this small pilot study, we used brain magnetic resonance imaging (MRI) CO2 stress testing to longitudinally assess cerebrovascular responsiveness (CVR) in individual sports-related concussion (SRC) patients.
Methods: Six SRC patients (three males and three females; mean age = 15.7, range = 15–17 years) underwent longitudinal brain MRI CO2 stress testing using blood oxygen level-dependent (BOLD) MRI and model-based prospective end-tidal CO2 targeting under isoxic conditions. First-level and second-level comparisons were undertaken using statistical parametric mapping (SPM) to score the scans and compare them to an atlas of 24 healthy control subjects.
Results: All tests were well tolerated and without any serious adverse events. Anatomical MRI was normal in all study participants. The CO2 stimulus was consistent between the SRC patients and control subjects and within SRC patients across the longitudinal study. Individual SRC patients demonstrated both quantitative and qualitative patient-specific alterations in CVR (p < 0.005) that correlated strongly with clinical findings, and that persisted beyond clinical recovery.
Conclusion: Standardized brain MRI CO2 stress testing is capable of providing a longitudinal assessment of CVR in individual SRC patients. Consequently, larger prospective studies are needed to examine the utility of brain MRI CO2 stress testing as a clinical tool to help guide the evaluation, classification, and longitudinal management of SRC patients.
Introduction
Concussion is a form of traumatic brain injury (TBI) that represents an important public health concern, especially in children and adolescents participating in sports. Although the vast majority will recover within 1–4 weeks of injury, an important proportion will develop prolonged symptoms or post-concussion syndrome (PCS) (1–6). This heterogeneous group of patients can be classified clinically into those with exercise intolerance, vestibulo-ocular dysfunction, cervical spine soft tissue injury, migraine headaches, and post-injury psychiatric disorders (7–12). In recent years, several advanced neuroimaging techniques have demonstrated significant differences in white matter anisotropy, brain activation patterns, and resting cerebral blood flow (CBF) between groups of concussion patients and healthy control subjects (13–23). Although these studies have advanced our understanding of the effects of concussion on brain structure and function, examination of group differences in neuroimaging biomarkers may not be an accurate reflection of the pathophysiological mechanisms underlying the symptoms and injury states in individual sports-related concussion (SRC) patients. Consequently, there remains a persistent need for neuroimaging assessment tools that can inform the evaluation and longitudinal management of individual concussion patients.
One of the metrics defining the health of the system responsible for regulating CBF during states of rest, activity, and disease is cerebrovascular reactivity/responsiveness (CVR), which is defined as the change in CBF in response to a vasodilatory stimulus (24, 25). Although previous studies have used techniques, such as transcranial Doppler ultrasonography to investigate changes in CVR following concussion (26–28), magnetic resonance imaging (MRI)-based assessment tools have several advantages, including superior spatial resolution and the ability to evaluate the entire brain (29–31). However, to provide accurate and longitudinal MRI-based assessment of CVR, a quantifiable, reliable, and reproducible vasoactive stimulus must be paired with an MRI sequence that can measure CBF or its surrogate (32, 33). Using model-based prospective targeting (MPET) of CO2 applied to blood oxygen level-dependent (BOLD) MRI, our group has introduced a brain MRI CO2 stress test that is capable of providing whole brain CVR mapping in individual concussion patients (34, 35). In a recent study, we demonstrated group and individual differences in CVR among adolescent PCS patients compared with healthy control subjects (35).
In this pilot study, we report results of longitudinal qualitative and quantitative brain MRI CO2 stress testing in individual adolescent SRC patients, comparing them to an atlas of healthy adolescent control subjects tested in the same way.
Materials and Methods
Research Design
This study was approved by the Biomedical Research Ethics Board at the University of Manitoba. We conducted a prospective case–control study of adolescent SRC patients and healthy control subjects. All adolescent SRC patients were recruited from the Pan Am Concussion Program in Winnipeg, MB, Canada. Patient inclusion criteria for this study included (1) diagnosis of SRC according to the definition set forth by the International Consensus on Concussion in Sport (36); (2) age 13–21 years. Healthy control subjects were recruited through word of mouth, including patient siblings and relatives. Control subject inclusion criteria included age 25 or younger. Control subject exclusion criteria were as follows: (1) the presence of a symptomatic concussion; (2) history of prior TBI or neurological condition resulting in structural brain abnormality on previous neuroimaging; (3) contra-indication to MRI; (4) presence of a neurological condition requiring prescription medication.
Clinical Assessment
All adolescent SRC patients underwent a clinical evaluation by a single neurosurgeon. All healthy control subjects underwent clinical interview to collect demographic data, past medical history, and past concussion history. In general, patients were deemed completely recovered when they were asymptomatic, tolerating full-time school, and neurological examination results were normal. In-season athletes were also required to successfully complete the International Consensus on Concussion in Sport return-to-play guidelines (36) to be deemed completely recovered. In some cases, hybrid neuropsychological testing and graded aerobic treadmill testing were used to confirm clinical recovery as indicated by the treating neurosurgeon. Patients were diagnosed with physiological post-concussion disorder if they had symptoms for 1 month or longer and demonstrated a symptom-limited threshold on graded aerobic treadmill testing (8, 11). Patients were diagnosed with vestibulo-ocular post-concussion disorder if they demonstrated subjective and objective evidence of vestibulo-ocular dysfunction symptoms for 1 month or longer and had no clinical evidence of exercise intolerance (9). Patients were diagnosed with a post-injury psychiatric disorder if they developed a novel psychiatric disorder following a SRC (10).
Neuroimaging Assessment
Following informed consent and clinical assessment, all subjects underwent neuroimaging assessment. SRC patients underwent initial neuroimaging assessment during the symptomatic phase of their injury and follow-up neuroimaging after documented clinical recovery. Follow-up neuroimaging assessment for SRC patients was scheduled based on patient and parent convenience rather than a pre-determined time point. MPET CO2 targeting was achieved by precise delivery of CO2 using a sequential gas delivery breathing circuit connected to a computerized gas-blender (RespirAct, Thornhill Research Inc., Toronto, ON, Canada). This device allows precise manipulation of PETCO2 levels under isoxic (target PETO2 = 115 mmHg) conditions. Hemodynamic monitoring during the study period included continuous heart rate and pulse oximetry and non-invasive blood pressure measurement (BP) at 3-min intervals.
All images were acquired using a Siemens Verio 3.0-T MR scanner with a 12-channel phased-array head coil. The MR-imaging protocol consisted of baseline anatomical imaging including sagittal 3D T1 MPRAGE (whole brain coverage; matrix: 256 × 256; slice thickness: 2.2 mm; no interslice gap; voxel size: 2 mm × 2 mm × 2 mm), axial fluid-attenuated inversion recovery (FLAIR), axial gradient recalled echo planar (GRE) sequences, and GRE B0-field mapping. CVR was assessed using continuous BOLD MRI during MPET CO2 targeting. The breathing sequence during BOLD imaging consisted of interval step-changes as follows: baseline ETCO2 (120 s), hypercapnia (5 mmHg above baseline for 120 s), baseline ETCO2 (30 s), hypercapnia (5 mmHg above baseline for 120 s), baseline ETCO2 (30 s), hypercapnia (5 mmHg above baseline for 120 s), baseline ETCO2 (120 s). BOLD MRI data were acquired with a T2*-weighted single-shot gradient echo pulse sequence with echoplanar readout (field of view: 24 cm × 24 cm; matrix: 64 × 64; TR: 2000 ms; TE: 30 ms; flip angle: 85°; slice thickness: 5.0 mm; interslice gap: 2.0 mm; voxel size: 3.75 mm × 3.75 mm × 6 mm; number of temporal frames = 330). Subject data were excluded if there was >3 mm of motion or inadequate end-tidal targeting.
Structural Neuroimaging
The structural neuroimaging component of each study was reviewed by a board-certified neuro-radiologist.
Preprocessing of MRI Sequences
Standard preprocessing of MRI EPI output was carried out for the BOLD sequences using SPM8 software and included batch processing by an SPM toolbox and custom written in-house MatLab scripts. The BOLD data were interpolated to the MPRAGE voxel dimensions. The fMRI model specification was a 2-pass process. We first modeled the data using the finite impulse response (FIR) package in SPM. The hypercapnic MPET model, as described earlier, using a triple stimulus block design for CO2 square wave response was assessed with a zero offset for this stimulus. The whole brain response to the CO2 stimulus was examined. An event-related response was calculated. The time to maximal response to hypercapnia was noted. This delay in response to the change in end-tidal CO2 was corrected based on a constructed series of time delay block stimulus files generated with delays from 0 to 30 s. This new fMRI model based on the time delay for brain activation by CO2 was now rerun as above.
We used the motion correction file generated with realignment as regressors in the model. First-level analysis results were based on this time-corrected analysis and the contrast images generated here were used in the second-level analysis. Masks were generated to correct for known inhomogeneous output of BOLD EPI signals. We made individualized masks based on GRE B0-field inhomogeneities greater than and less than 500 arbitrary units (AU). These binary images masked out voxels with inhomogeneities at the base of the brain, those adjacent to the petrous bone and those contiguous with the frontal sinuses. A dilated CSF mask with dilation × 2 (based on the Wake Forest University Pick Atlas) was generated to mask out the ventricular and periventricular inverse BOLD response in part related to choroid plexus response and interface changes at the ventricular edges (37). This combined binary image – labeled [(gm + wm) − (B0_inhomogeneity + dilated_ventricles)] was used in the second-level analysis as an inclusive mask to ensue that the abnormal voxels for an individual study were intraparenchymal. A representative mask is shown in the Supplementary Material. First-level analyses were undertaken with the author blinded to the subject’s group (healthy control versus SRC patient); however, second-level analyses were not blinded since they were based on the results of the first-level analysis.
Statistical Analysis
Cerebrovascular BOLD Responsiveness (First-Level Analysis)
First-level analyses were undertaken for each study participant. BOLD increases in response to the hypercapnic breathing stimulus and BOLD decreases in response to the stimulus were assessed at the p = 0.001 uncorrected level. The cluster size threshold was 10 voxels. The response to the applied CO2 stimulus and its inverse were expressed as voxel counts/whole-brain voxel count ratios.
Cerebrovascular BOLD Responsiveness (Second-Level Analysis)
A second-level analysis for the BOLD studies was completed based on comparison to an atlas of adolescent control subjects (n = 24), thereby allowing individual longitudinal analysis of the six SRC patients. Each SRC patient study underwent voxel-by-voxel comparisons for BOLD signals that were less than or greater than the mean control group response. This second-level analysis was conducted over a series of p-values (p = 0.001, 0.005, 0.01, and 0.05; we report our results at the p = 0.005 level; see below). For each individual second-level comparison, the images were masked using the combined mask outlined above for that individual versus the atlas output, and the voxel counts reported are corrected for the applied individual mask.
Results
Participants
In this study, longitudinal brain MRI CO2 stress testing was carried out in six SRC patients (three males and three females; mean age = 15.7, range = 15–17 years) whose test results were compared with those of a normal control atlas of 24 subjects (15 males and 9 females; mean age = 18.5, range = 13–25 years). Four SRC patients and 17 control subjects participated in a previous cross-sectional study (35). Three control subjects were excluded due to excessive motion during neuroimaging assessment. Among control subjects that made up the normal control atlas, their past medical history was significant for remote concussion in 7/24 (29.2%) subjects. One SRC patient was on medication for a post-injury anxiety and sleep disorder during both the initial and follow-up neuroimaging. Additional clinical and neuroimaging characteristics of the SRC patients are summarized in Table 1.
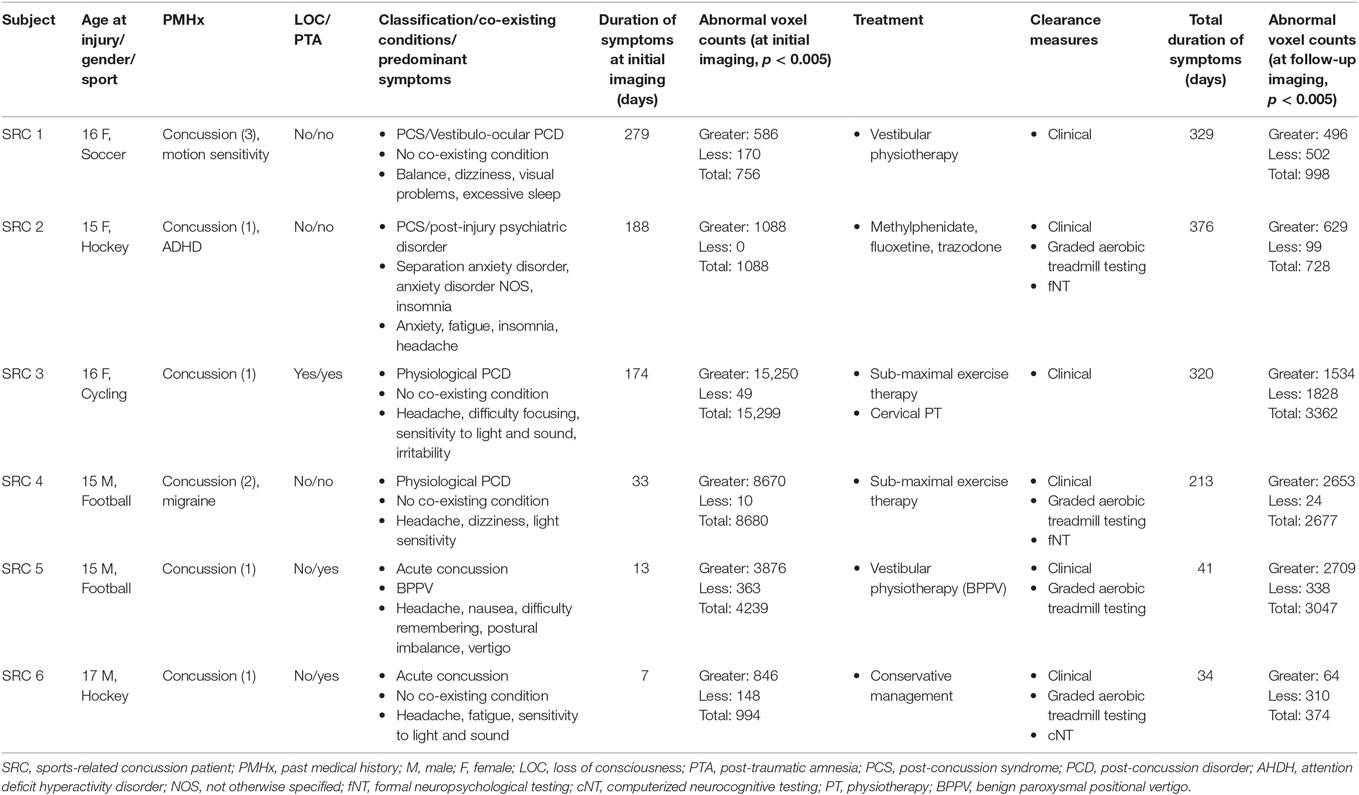
Table 1. Summary of demographic and clinical features of adolescent sports-related concussion patients.
Study Tolerability
All subjects successfully completed the full imaging study. One SRC patient reported transient and self-limiting headache and fatigue during initial neuroimaging assessment.
Structural Neuroimaging
Structural neuroimaging studies were normal in all subjects. No evidence of traumatic abnormalities was detected among SRC patients.
End-Tidal Gas Targeting and Hemodynamics
No significant differences in baseline PETCO2 (41.6 ± 3.8 mmHg – first imaging period and 41.3 ± 3.7 mmHg – second imaging period; p = 0.822 paired t-test), and the change in CO2 (ΔCO2) during CO2 targeting (4.3 ± 0.7 mmHg first imaging, 4.2 ± 1.1 mmHg second imaging; p = 0.874) were detected. Thus, the patients were exposed to the same CO2 stimulus during BOLD imaging on both occasions.
BOLD Cerebrovascular Responsiveness (First-Level Analysis)
There was no significant difference between the control subject atlas and SRC patient BOLD responses to CO2 (p = 0.001; 10 voxel cluster size). The mean BOLD response to the CO2 stimulus was 83 ± 12% in the SRC patients and 85 ± 6% in the control subjects (p = 0.668 between groups). The mean BOLD inverse response was 0.5 ± 0.4% in the SRC patients and 0.5 ± 0.4% in controls (p = 0.774 between groups). The number of voxels masked out by the inclusive mask [(gm + wm) − (B0_inhomogeneity + dilated_ventricles)] was 20,980 ± 3900 in the SRC group at imaging period 1 and 21,290 ± 4400 at imaging period 2, indicating no difference in number of voxels masked out between time periods (p = 0.919). The proportion of voxels masked out by the B0 field inhomogeneities represented 5.4 ± 1.7% of total voxel counts during imaging period 1 and 4.5 ± 1.7% in imaging period 2. Dilated ventricle masking represented 5.7 ± 3.2 and 6.9 ± 2.1%, respectively.
BOLD Cerebrovascular Responsiveness (Second-Level Analysis)
Second-level analysis comparing individual SRC patients to the healthy control atlas at their initial neuroimaging tests, during the symptomatic phase of injury, demonstrated patient-specific alterations in CVR in all patients with a predominant pattern of increased CVR. Follow-up neuroimaging, during the clinically recovered stage of injury, demonstrated relatively stable CVR in two patients, and improved but persistently abnormal CVR in four patients. Examples of longitudinal CVR assessment using brain MRI CO2 stress testing is shown in Figures 1 and 2. Images indicate voxel counts at the p = 0.005 level. The full complement of patient study images is available in Supplementary Material.
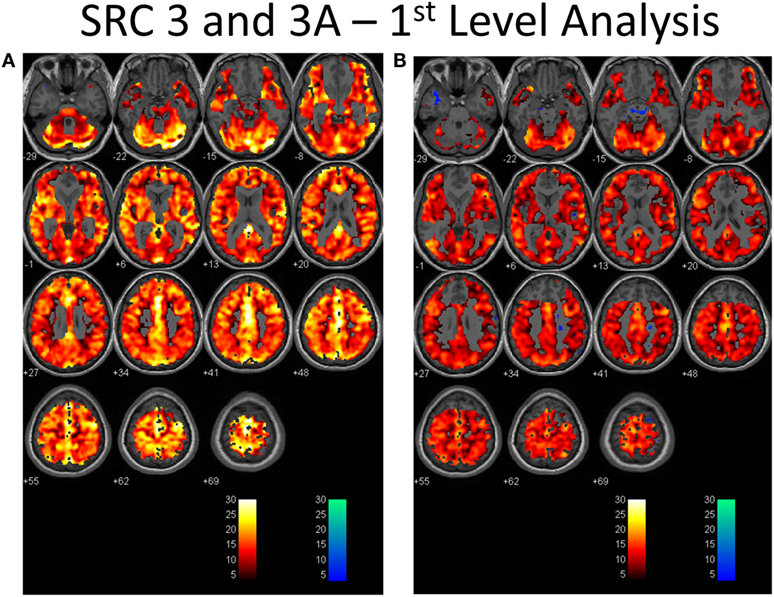
Figure 1. First-level analysis of longitudinal brain MRI CO2 stress testing in one physiological post-concussion disorder patient during the symptomatic (A) and clinically recovered stages of injury (B). In each case the hot voxels (orangish hues) indicate where voxels have responded (at the p = 0.001 level; t-statistic = 3.11) to the triple CO2 stimulus as delivered by the model-based prospective end-tidal targeting device as outlined in the methodology based on the general linear model constructed in the SPM analysis. A colored voxel indicates that the BOLD response increased when the CO2 stimulus increased and decreased as the CO2 stimulus decreased during the applied triple stimulus. The cold voxels (bluish hues) indicate where voxels have responded (at the p = 0.001 level; t-statistic = 3.11) in an inverse manner to the triple CO2 stimulus – that is the BOLD signal decreased when the CO2 signal increased and vice versa. Where the voxels remain gray the anatomic image template shows through indicating that the chosen level of statistical significance was not attained. In each study, an individualized inclusive mask was applied to eliminate artifactual voxels (see description in the methodology and a representative mask in Supplementary Material). The color bar numbers indicate the value of the t-statistic attained for the color displayed.
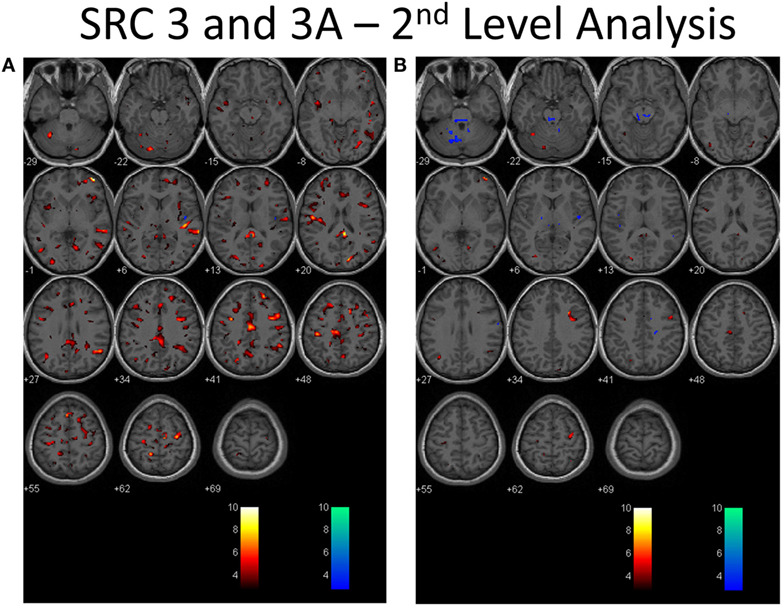
Figure 2. Second-level analysis of longitudinal brain MRI CO2 stress testing in the same physiological post-concussion disorder patient illustrated in Figure 1 during the symptomatic (A) and clinically recovered stages of injury (B) compared with the stand-alone control atlas. Results are reported at the p = 0.005 level for cut-off of significant voxels (t-statistic value = 2.82 in this case). Hot color voxels (orangish hues) indicate where BOLD CVR response is significantly greater than the mean value for the collated control group images and the cool color voxels (blue hues) indicate where the BOLD CVR response is significantly less when compared with the mean value for the control group. The scale indicates the color scheme as expressed in t-values. This approach permits quantitation of the longitudinal differences evident to the eye as seen in Figure 1 to be displayed.
Discussion
In this pilot study, when assessed for abnormality by comparison to a normal control atlas, patient-specific qualitative and quantitative alterations in CVR were detected in all SRC patients; with the highest abnormal voxel counts observed among patients with acute concussion and physiological post-concussion disorder. When assessed for changes longitudinally, two patients with physiological post-concussion disorder (SRC 3 and 4) demonstrated improvements in CVR that nevertheless remained abnormal. Two other PCS patients with more chronic symptoms, one with isolated vestibulo-ocular dysfunction (SRC 1) and one who developed a post-injury psychiatric disorder (SRC 2), demonstrated relatively stable CVR measures with little change over time. For the two acute SRC patients (SRC 5 and 6), both demonstrated improvements in CVR that nevertheless remained persistently abnormal despite clinical recovery.
The results of this pilot study, therefore, provide confirmatory evidence that SRC patients display both qualitative and quantitative patient-specific alterations in CVR that can be detected by brain MRI CO2 stress testing. Our findings also suggest that alterations in CVR may account for some of the clinical findings in patients with acute concussion and physiological post-concussion disorder, but that CVR changes may play a less prominent role in those with isolated vestibulo-ocular dysfunction and post-injury psychiatric disorders. Our results are in agreement with previous work that demonstrated alterations in mean resting CBF in groups of pediatric and collegiate SRC patients that persisted beyond clinical and neurocognitive recovery (18, 23). Whether alterations in resting CBF and CVR that persist beyond clinical recovery place these athletes at an elevated risk of future injury, or lead to long-term effects of cumulative brain injury, requires further study. It is possible that the vessels marked by abnormal CVR may, like other tissues, be more vulnerable to re-injury, or worsened physiological dysfunction, with repeated head trauma. The persistence of CVR abnormalities detected months after injury in many of these patients indicates that much longer evaluations are required to document the natural history of cerebrovascular dysfunction following concussion. Our preliminary experience with this population suggests that concussion may be characterized by a predominant pattern of increased CVR during the acute or sub-acute phase and a predominantly reduced pattern of CVR in the chronic phase. If CVR is an adequate biomarker for cerebrovascular dysfunction following concussion, additional study will be required to further refine the criteria for abnormal CVR and the adequacy of neurovascular recovery as well as take into account potential artifact related to data analysis and fluctuations in CVR over time among normal subjects.
Brain MRI CO2 stress testing meets several important requirements for a neuroimaging assessment tool that can provide longitudinal CVR assessments in concussion patients. First, as demonstrated here and in previous studies (34, 35) this test is safe and well tolerated among acute SRC and PCS patients and does not require exposure to radiation, provocative breathing maneuvers, or intravenous agents. Second, this technique delivers a precise, quantifiable, and reproducible CO2 stimulus under isoxic conditions, thereby allowing a consistent vasoactive stimulus to be applied during serial assessments without the confounding effects of alterations in PETO2 and individual variability in respiratory physiology (32, 38). Third, this technique generates qualitative and quantitative biomarkers that can be compared with other clinical outcomes in individual SRC patients (33). Although there were subtle 1–2 mmHg differences in the CO2 stimulus applied across subjects and time-points, this level of end-tidal gas control is similar to that achieved with mechanical ventilation in other CVR studies (39, 40), and represents a level of precision and reproducibility that is unachievable with other vasoactive stimuli including breath-holding, acetazolamide, and inhaled CO2 such as carbogen (32, 33).
This pilot study has several important limitations. First, the number of SRC patients who underwent longitudinal CVR assessment is small. Patients were recruited from a tertiary pediatric concussion program that may have selected for more severely injured SRC patients. Second, objective measures of clinical concussion recovery, including graded aerobic treadmill testing and formal neuropsychological testing, were not used to confirm physiological and neurocognitive recovery in all patients, and patients were not imaged at uniform and pre-selected time periods. Some patients had returned to contact and collision sports prior to follow-up neuroimaging assessment in which cases the effects of unreported concussions or sub-concussion injuries may have affected follow-up imaging results. Third, masking of the BOLD EPI for the second-level analysis removed from consideration some of the prefrontal and frontal cortex and periventricular white matter in each case that may have resulted in under-detection of abnormal voxels. Lastly, although previous work has demonstrated excellent within-day reliability of CVR assessment in pediatric subjects using this technique (41), longitudinal CVR assessments were not performed among the healthy control subjects. This limitation should be considered in future studies.
In conclusion, this study provides confirmatory empirical evidence that SRC is associated with patient-specific alterations in CVR. Larger prospective studies are needed to confirm these preliminary findings and examine the utility of brain MRI CO2 stress testing as a clinical tool to help guide the evaluation, classification, prognostication, and longitudinal management of SRC patients.
Author Contributions
WM – experimentation, data collation, analysis, interpretation, and writing. MJE – experimentation, data collation, analysis, interpretation, and writing. LR – experimentation, data collation, analysis, interpretation, and writing. MM – experimentation and data collation. PP – experimentation and data collation. BD – analysis and statistical consultation. ME – analysis and interpretation. DM – analysis, interpretation, and writing. JD – analysis, interpretation, and writing. JF – analysis, interpretation, and writing.
Conflict of Interest Statement
JF, JD, and DM are senior scientists at Thornhill Research Inc., (TRI), a company affiliated with the University Health Network that developed the RespirAct™, a non-commercial research tool assembled by TRI to enable cerebrovascular reactivity studies. The remaining authors declare that the research was conducted in the absence of any commercial or financial relationships that could be construed as a potential conflict of interest.
Funding
All phases of this study were supported by grants from the Health Sciences Center Foundation, the Manitoba Health Research Council and the Academic Oversight Committee, Winnipeg, MB, Canada.
Supplementary Material
The Supplementary Material for this article can be found online at http://journal.frontiersin.org/article/10.3389/fneur.2016.00107
References
1. Yeates KO, Luria J, Bartkowski H, Rusin J, Martin L, Bigler ED. Postconcussive symptoms in children with mild closed head injuries. J Head Trauma Rehabil (1999) 14:337–50. doi: 10.1097/00001199-199908000-00003
2. Barlow KM, Crawford S, Stevenson A, Sandhu SS, Belanger F, Dewey D. Epidemiology of postconcussion syndrome in pediatric mild traumatic brain injury. Pediatrics (2010) 126:e374–81. doi:10.1542/peds.2009-0925
3. Babcock L, Byczkowski T, Wade SL, Ho M, Mookerjee S, Bazarian JJ. Predicting postconcussion syndrome after mild traumatic brain injury in children and adolescents who present to the emergency department. JAMA Pediatr (2013) 167:156–61. doi:10.1001/jamapediatrics.2013.434
4. Brown NJ, Mannix RC, O’Brien MJ, Gostine D, Collins MW, Meehan WP III. Effect of cognitive activity level on duration of post-concussion symptoms. Pediatrics (2014) 133:e299–304. doi:10.1542/peds.2013-2125
5. Corwin DJ, Zonfrillo MR, Master CL, Arbogast KB, Grady MF, Robinson RL, et al. Characteristics of prolonged concussion recovery in a pediatric subspecialty referral population. J Pediatr (2014) 165:1207–15. doi:10.1016/j.jpeds.2014.08.034
6. Grubenhoff JA, Deakyne SJ, Brou L, Bajaj L, Comstock RD, Kirkwood MW. Acute concussion symptom severity and delayed symptom resolution. Pediatrics (2014) 134:54–62. doi:10.1542/peds.2013-2988
7. Leddy JJ, Willer B. Use of graded exercise testing in concussion and return-to-activity management. Curr Sports Med Rep (2013) 12:370–6. doi:10.1249/JSR.0000000000000008
8. Ellis MJ, Leddy JJ, Willer B. Physiological, vestibulo-ocular and cervicogenic post-concussion disorders: an evidence-based classification system with directions for treatment. Brain Inj (2014) 29(2):238–48. doi:10.3109/02699052.2014.965207
9. Ellis MJ, Cordingley D, Vis S, Reimer K, Leiter J, Russell K. Vestibulo-ocular dysfunction in pediatric sports-related concussion. J Neurosurg Pediatr (2015) 16:248–55. doi:10.3171/2015.1.PEDS14524
10. Ellis MJ, Ritchie LJ, Koltek M, Hosain S, Cordingley D, Chu S, et al. Psychiatric outcomes after pediatric sports-related concussion. J Neurosurg Pediatr (2015) 16(6):709–18. doi:10.3171/2015.5.PEDS15220
11. Leddy JJ, Baker JG, Merchant A, Picano J, Gaile D, Matuszak J, et al. Brain or strain? Symptoms alone do not distinguish physiologic concussion from cervical/vestibular injury. Clin J Sport Med (2015) 25:237–42. doi:10.1097/JSM.0000000000000128
12. Leddy JJ, Baker JG, Willer B. Active rehabilitation of concussion and post-concussion syndrome. Phys Med Rehabil Clin N Am (2016) 27:437–54. doi:10.1016/j.pmr.2015.12.003
13. Chen JK, Johnston KM, Frey S, Petrides M, Worsley K, Ptito A. Functional abnormalities in symptomatic concussed athletes: an fMRI study. Neuroimage (2004) 22:68–82. doi:10.1016/j.neuroimage.2003.12.032
14. Slobounov SM, Zhang K, Pennell D, Ray W, Johnson B, Sebastianelli W. Functional abnormalities in normally appearing athletes following mild traumatic brain injury: a functional MRI study. Exp Brain Res (2010) 202:341–54. doi:10.1007/s00221-009-2141-6
15. Cubon VA, Putukian M, Boyer C, Dettwiler A. A diffusion tensor imaging study on the white matter skeleton in individuals with sports-related concussion. J Neurotrauma (2011) 28:189–201. doi:10.1089/neu.2010.1430
16. Henry LC, Tremblay J, Tremblay S, Lee A, Brun C, Lepore N, et al. Acute and chronic changes in diffusivity measures after sports concussion. J Neurotrauma (2011) 28:2049–59. doi:10.1089/neu.2011.1836
17. Koerte IK, Kaufmann D, Hartl E, Bouix S, Pasternak O, Kubicki M, et al. A prospective study of physician-observed concussion during a varsity university hockey season: white matter integrity in ice hockey players. Part 3 of 4. Neurosurg Focus (2012) 33:E3, 1–7. doi:10.3171/2012.10.FOCUS12303
18. Maugans TA, Farley C, Altaye M, Leach J, Cecil KM. Pediatric sports-related concussion produces cerebral blood flow alterations. Pediatrics (2012) 129:28–37. doi:10.1542/peds.2011-2083
19. Kontos AP, Huppert TJ, Beluk NH, Elbin RJ, Henry LC, French J, et al. Brain activation during neurocognitive testing using functional near-infrared spectroscopy in patients following concussion compared to healthy controls. Brain Imaging Behav (2014) 8:621–34. doi:10.1007/s11682-014-9289-9
20. Pasternak O, Koerte IK, Bouix S, Fredman E, Sasaki T, Mayinger M, et al. Hockey concussion education project, part 2. Microstructural white matter alterations in acutely concussed ice hockey players: a longitudinal free-water MRI study. J Neurosurg (2014) 120:873–81. doi:10.3171/2013.12.JNS132090
21. Yuh EL, Hawryluk GW, Manley GT. Imaging concussion: a review. Neurosurgery (2014) 75(Suppl 4):S50–63. doi:10.1227/NEU.0000000000000491
22. Meier TB, Bellgowan PS, Singh R, Kuplicki R, Polanski DW, Mayer AR. Recovery of cerebral blood flow following sports-related concussion. JAMA Neurol (2015) 72(5):530–8. doi:10.1001/jamaneurol.2014.4778
23. Wang Y, Nelson LD, LaRoche AA, Pfaller AY, Nencka AS, Koch KM, et al. Cerebral blood flow alterations in acute sport-related concussion. J Neurotrauma (2016) 33(13):1227–36. doi:10.1089/neu.2015.4072
24. Bhogal AA, Philippens ME, Siero JC, Fisher JA, Petersen ET, Luijten PR, et al. Examining the regional and cerebral depth-dependent BOLD cerebrovascular reactivity response at 7T. Neuroimage (2015) 114:239–48. doi:10.1016/j.neuroimage.2015.04.014
25. Leung J, Kosinski PD, Croal PL, Kassner A. Developmental trajectories of cerebrovascular reactivity in healthy children and young adults assessed with magnetic resonance imaging. J Physiol (2016) 594:2681–9. doi:10.1113/JP271056
26. Len TK, Neary JP. Cerebrovascular pathophysiology following mild traumatic brain injury. Clin Physiol Funct Imaging (2011) 31:85–93. doi:10.1111/j.1475-097X.2010.00990.x
27. Len TK, Neary JP, Asmundson GJ, Goodman DG, Bjornson B, Bhambhani YN. Cerebrovascular reactivity impairment after sport-induced concussion. Med Sci Sports Exerc (2011) 43:2241–8. doi:10.1249/MSS.0b013e3182249539
28. Gardner AJ, Tan CO, Ainslie PN, van Donkelaar P, Stanwell P, Levi CR, et al. Cerebrovascular reactivity assessed by transcranial Doppler ultrasound in sport-related concussion: a systematic review. Br J Sports Med (2015) 49(16):1050–5. doi:10.1136/bjsports-2014-093901
29. Chan ST, Evans KC, Rosen BR, Song TY, Kwong KK. A case study of magnetic resonance imaging of cerebrovascular reactivity: a powerful imaging marker for mild traumatic brain injury. Brain Inj (2015) 29:403–7. doi:10.3109/02699052.2014.974209
30. Militana AR, Donahue MJ, Sills AK, Solomon GS, Gregory AJ, Strother MK, et al. Alterations in default-mode network connectivity may be influenced by cerebrovascular changes within 1 week of sports related concussion in college varsity athletes: a pilot study. Brain Imaging Behav (2016) 10(2):559–68. doi:10.1007/s11682-015-9407-3
31. da Costa L, Bas van Niftrik C, Crane D, Fierstra J, Bethune A. Temporal profile of cerebrovascular reactivity impairment, gray matter volumes, and persistent symptoms after mild traumatic brain injury. Front Neurol (2016) 7:70. doi:10.3389/fneur.2016.00070
32. Fierstra J, Sobczyk O, Battisti-Charbonney A, Mandell DM, Poublanc J, Crawley AP, et al. Measuring cerebrovascular reactivity: what stimulus to use? J Physiol (2013) 591(23):5809–21. doi:10.1113/jphysiol.2013.259150
33. Ellis MJ, Ryner L, Sobczyk O, Fierstra J, Mikulis D, Fisher JA, et al. Neuroimaging assessment of cerebrovascular reactivity in concussion: current concepts, methodological considerations and review of the literature. Front Neurol (2016) 7:61. doi:10.3389/fneur.2016.00061
34. Mutch WA, Ellis MJ, Graham MR, Wourms V, Raban R, Fisher JA, et al. Brain MRI CO2 stress testing: a pilot study in patients with concussion. PLoS One (2014) 9:e102181. doi:10.1371/journal.pone.0102181
35. Mutch WA, Ellis MJ, Ryner LN, Ruth Graham M, Dufault B, Gregson B, et al. Brain magnetic resonance imaging CO2 stress testing in adolescent postconcussion syndrome. J Neurosurg (2015) 18:1–13. doi:10.3171/2015.6.JNS15972
36. McCrory P, Meeuwisse WH, Aubry M, Cantu B, Dvorak J, Echemendia RJ, et al. Consensus statement on concussion in sport: the 4th international conference on concussion in sport held in Zurich, November 2012. Br J Sports Med (2013) 47:250–8. doi:10.1136/bjsports-2013-092313
37. Thomas BP, Liu P, Aslan S, King KS, van Osch MJ, Lu H. Physiologic underpinnings of negative BOLD cerebrovascular reactivity in brain ventricles. Neuroimage (2013) 83:505–12. doi:10.1016/j.neuroimage.2013.07.005
38. Sobczyk O, Crawley AP, Poublanc J, Sam K, Mandell DM, Mikulis DJ, et al. Identifying significant changes in cerebrovascular reactivity to carbon dioxide. AJNR Am J Neuroradiol (2016) 37(5):818–24. doi:10.3174/ajnr.A4679
39. Adelson PD, Clyde B, Kochanek PM, Wisniewski SR, Marion DW, Yonas H. Cerebrovascular response in infants and young children following severe traumatic brain injury: a preliminary report. Pediatr Neurosurg (1997) 26:200–7. doi:10.1159/000121192
40. Adelson PD, Srinivas R, Chang Y, Bell M, Kochanek PM. Cerebrovascular response in children following severe traumatic brain injury. Childs Nerv Syst (2011) 27:1465–76. doi:10.1007/s00381-011-1476-z
Keywords: sports-related concussion, adolescent, post-concussion syndrome, magnetic resonance imaging, blood oxygen level-dependent imaging, cerebrovascular reactivity, longitudinal
Citation: Mutch WAC, Ellis MJ, Ryner LN, Morissette MP, Pries PJ, Dufault B, Essig M, Mikulis DJ, Duffin J and Fisher JA (2016) Longitudinal Brain Magnetic Resonance Imaging CO2 Stress Testing in Individual Adolescent Sports-Related Concussion Patients: A Pilot Study. Front. Neurol. 7:107. doi: 10.3389/fneur.2016.00107
Received: 22 April 2016; Accepted: 21 June 2016;
Published: 08 July 2016
Edited by:
Niklas Marklund, University of Uppsala, SwedenReviewed by:
Eric Peter Thelin, Karolinska Institutet, SwedenRamon Diaz-Arrastia, Uniformed Services University of the Health Sciences, USA
Frederick Robert Carrick, Bedfordshire Centre for Mental Health Research in association with University of Cambridge, UK
Copyright: © 2016 Mutch, Ellis, Ryner, Morissette, Pries, Dufault, Essig, Mikulis, Duffin and Fisher. This is an open-access article distributed under the terms of the Creative Commons Attribution License (CC BY). The use, distribution or reproduction in other forums is permitted, provided the original author(s) or licensor are credited and that the original publication in this journal is cited, in accordance with accepted academic practice. No use, distribution or reproduction is permitted which does not comply with these terms.
*Correspondence: W. Alan C. Mutch, d2FjbXV0Y2hAc2hhdy5jYQ==