- 1Institute of Sports Science, Leibniz University Hanover, Hanover, Germany
- 2Victor Chang Cardiac Research Institute, Sydney, NSW, Australia
Rhythmic auditory cueing has been shown to enhance gait performance in several movement disorders. The “entrainment effect” generated by the stimulations can enhance auditory motor coupling and instigate plasticity. However, a consensus as to its influence over gait training among patients with multiple sclerosis is still warranted. A systematic review and meta-analysis was carried out to analyze the effects of rhythmic auditory cueing in studies gait performance in patients with multiple sclerosis. This systematic identification of published literature was performed according to PRISMA guidelines, from inception until Dec 2017, on online databases: Web of science, PEDro, EBSCO, MEDLINE, Cochrane, EMBASE, and PROQUEST. Studies were critically appraised using PEDro scale. Of 602 records, five studies (PEDro score: 5.7 ± 1.3) involving 188 participants (144 females/40 males) met our inclusion criteria. The meta-analysis revealed enhancements in spatiotemporal parameters of gait i.e., velocity (Hedge's g: 0.67), stride length (0.70), and cadence (1.0), and reduction in timed 25 feet walking test (−0.17). Underlying neurophysiological mechanisms, and clinical implications are discussed. This present review bridges the gaps in literature by suggesting application of rhythmic auditory cueing in conventional rehabilitation approaches to enhance gait performance in the multiple sclerosis community.
Introduction
Multiple sclerosis is a prevalent, progressive demyelinating disease of the central nervous system (1). It is one of the most common causes of non-traumatic progressive disability in younger population groups (2, 3), but is also not uncommon in aged population (4). The main pathological characteristics of multiple sclerosis include progressive demyelination, and disruption of blood brain barrier due to inflammatory changes (5). This eventually affects the functioning of relevant axonal tracts, thereby causing widespread neurological symptoms (1, 6). The clinical manifestations in patients with multiple sclerosis include disruptions in sensory, motor and cognitive functioning. For instance, paresthesia, sensory loss, progressive hemiparesis, ataxia, fatigue, and depression have been widely reported (7, 8).
Gait and postural dysfunctions are also common in patients with multiple sclerosis especially due to the involvement of pyramidal track, cerebellar and spinal cord dysfunctions (9–11). Prosperini et al. (2) for instance, reported lesions primarily in cerebellar, supratentorial associative bundles to affect the static and dynamic stability in patients with multiple sclerosis. Likewise, pathological involvement of leukocortical, intracortical, and subpial regions have also been reported (7, 12). Together, these sensory, motor and cognitive dysfunctions affect motor control and coordination (13, 14), eventually promoting falls (15), and affecting the quality of life (16). Typical gait characteristics exhibited by patients with multiple sclerosis include reduced gait velocity, stride length, cadence, and increased step width, asymmetric gait, double limb support duration (17, 18) [for a detailed review see (16, 19)]. Kinematic analysis of gait further reports larger range of motion at hip joint (20), increased knee flexion, reducing in ankle plantarflexion (21), and higher pelvic obliquity (22). Furthermore, electromyographic studies report abnormal musculoskeletal co-activation pattern especially at the ankle joint (23). These adjustments in gait kinematics and muscular co-contractions have been affirmed as cautionary measures adopted by patients for promoting stability during gait (24). These gait modifications although are intended to safeguard oneself from falling. Retrospectively, these modifications promote a rather slow, uneconomical, fatigue promoting, and highly fall prone gait pattern (25–28).
Common treatment strategies to curb motor dysfunctions in multiple sclerosis include physical exercise (29, 30), training with virtual-reality (31), physical/occupational therapy (32), hydrotherapy (33), electrical stimulations (16), martial arts (34), dual-task training (28), and external sensory cueing (35, 36). Studies report that sensory dysfunctions in patients with multiple sclerosis primarily play a key role in disrupting motor control and coordination (37). Disruptions in the perception of visual (38), and proprioceptive (39, 40), systems have been well-documented. Therefore, providing additional sensory cueing to support movement execution might serve as a viable option to overcome this loss. Only a handful of studies have analyzed the effects of external sensory stimulations (auditory, visual) on motor performance in patients with multiple sclerosis (35, 36, 41, 42). Nevertheless, the predominant role of auditory cueing as compared to its visual counterpart has been emphasized in literature (43, 44). Predominantly auditory cortex has been reported to perceive rhythmic stimuli by as short as 20–30 ms, which is considerably shorter as compared to visual and tactile thresholds (45–47). Moreover, it utilizes the rich interconnectivity of the auditory cortex to motor centers from spinal cord extending from the brainstem, cortical and subcortical structures (48–50). This also enables the auditory system to operate in a quite fast, precise, and efficient manner (51, 52). Several types of rehabilitation approaches have been reported in the literature for delivering external auditory stimulations, such as rhythmic auditory cueing (50), patterned sensory enhancement (53, 54), and real-time auditory feedback (55, 56). However, rhythmic auditory cueing is the most widely studied treatment strategy with respect to healthy population groups (28), population groups, and patients affected from movement disorders such as parkinsonism (47), stroke (57), and cerebral palsy (58). This type of stimulation can allow enhancements in motor execution in a multifaceted manner (47, 52). For instance, the sensory cueing can enhance biological motion perception (55, 59), promote audio-motor imagery (60, 61), reducing shape variability in muscle co-activation (62), mediate cortical reorganization, neural-plasticity (63), reduce cognitive overload (64), and more (45).
Moreover, recent research suggests increased financial burden on patients with multiple sclerosis (65, 66), especially because of the disease's progressive and relapsing nature (67). Therefore, development of affordable, and convenient rehabilitation strategies must be emphasized. Rhythmic auditory cueing is an effective strategy in these terms as it is viable, cheap, and can also be effectively applied as a home-based intervention (26–28). Therefore, we attempted to develop a state of knowledge by conducting a systematic review and meta-analyses to determine the effects of rhythmic auditory cueing on gait performance in patients with multiple sclerosis.
Methods
This review was conducted according to the guidelines outlined in Preferred Reporting Items for Systematic Reviews and Meta-analysis: The PRISMA statement (68).
Data Sources and Search Strategy
Academic databases such as Web of science, PEDro, EBSCO, MEDLINE, Cochrane, EMBASE, and PROQUEST were searched from inception until December 2017. A sample search strategy has been provided in (Table 1).
Data Extraction
Upon selection for review, the following data were extracted from each article i.e., author, date of publication, selection criteria, sample size, sample description (gender, age, health status), intervention, characteristics of auditory cueing, outcome measures, results, and conclusions. The data were then summarized and tabulated (Table 2).
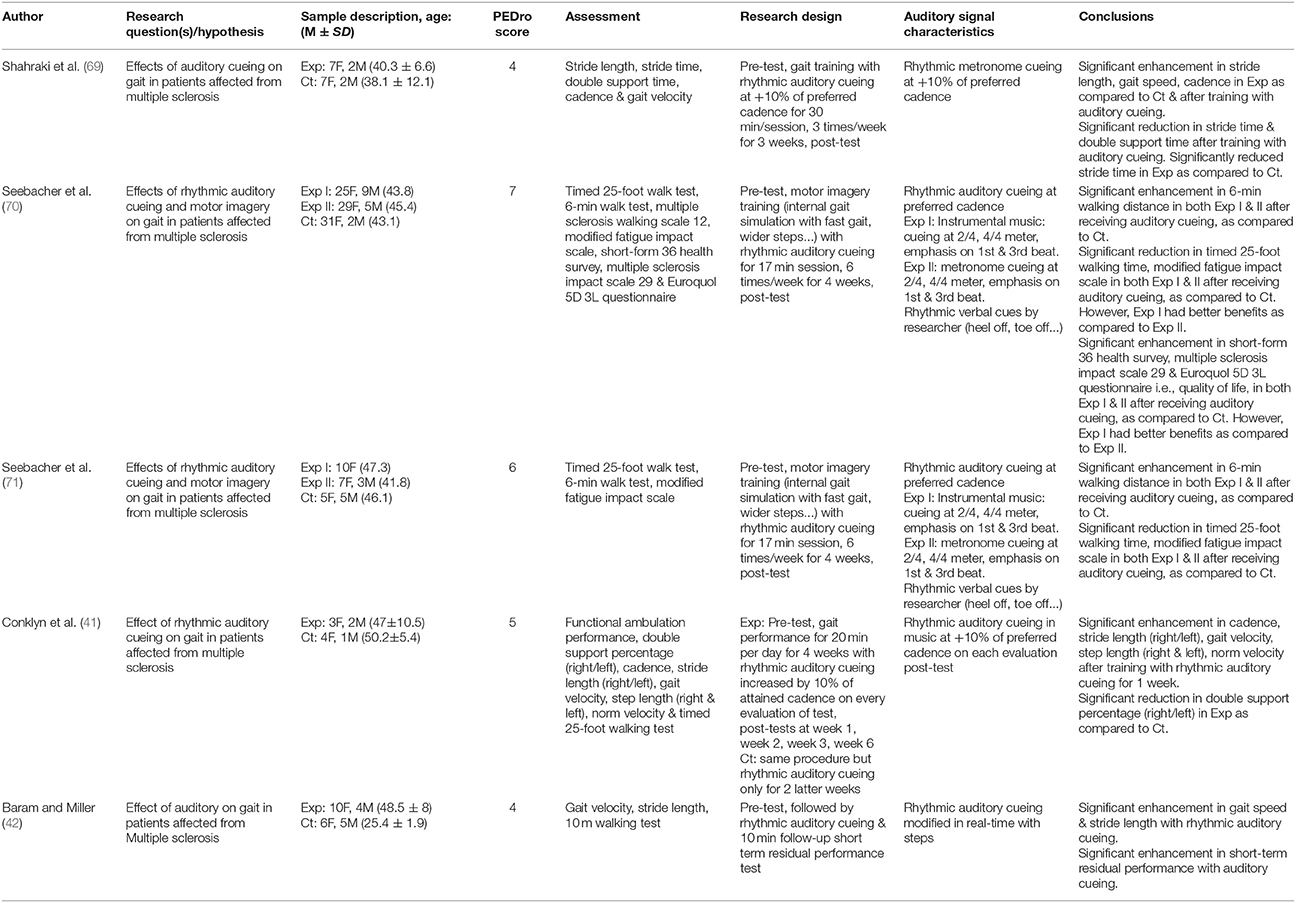
Table 2. Studies analyzing the effects of rhythmic auditory cueing on gait in patients with multiple sclerosis.
The inclusion criteria for the studies was (i) Performed studies were either randomized controlled trials, cluster randomized controlled trials, or controlled clinical trials; (ii) Studies reporting reliable and valid spatiotemporal gait parameters (iii) Studies including dynamic aspects of gait stability (iv) Studies qualified PEDro methodological quality scale (≥4 score); (v) Experiments conducted on human participants; (vi) Published in a peer-reviewed academic journals; (vii) Articles published in English, German and Korean languages.
Quality and Risk of Bias Assessment
The quality of the studies was assessed using the PEDro methodological quality scale (72). The scale consists of 11 items addressing external validity, internal validity, and interpretability and can detect potential bias with fair to good reliability (73), and validity (72). A blinded rating of the methodological quality of the studies was carried out by the primary reviewer. Ambiguous issues were discussed between the 1st (SG) and the 2nd (IG) reviewer and consensus were reached. Included studies were rated, and interpreted according to scoring of 9–10, 6–8, and 4–5 considered of “excellent,” “good,” and “fair” quality (74), respectively. Inadequate randomization, non-blinding of assessors, no intention to treat analysis and no measurement of compliance were considered as major threats to biasing (75).
Data Analysis
This systematic review also included a meta-analysis approach even with a few number of studies (76), with an aim to develop a better understanding of the incorporated interventions (77). The presence and lack of heterogeneity asserted the use of either random or fixed effect meta-analysis (78). A narrative synthesis of the findings structured around the intervention, population characteristics, methodological quality (Table 2) and the type of outcome are also provided. Likewise, summaries of intervention effects for each study were provided in a tabular form (Table 2). A meta-analysis was conducted between pooled studies using CMA (Comprehensive meta-analysis V 2.0, USA). Heterogeneity between the studies was assessed using I2 statistics. The data in this review was systematically distributed and for each available variable pooled, dichotomous data was analyzed and forest plots with 95% confidence intervals are reported. The effect sizes were adjusted and reported as Hedge's g (79). Thresholds for interpretation of effect sizes were as follows: a standard mean effect size of 0 means no change, mean effect size of 0.2 is considered as a small effect, 0.5 is considered as a medium effect and 0.8 as a large effect (80). Interpretation of heterogeneity via I2 statistics was that values from 0-0, 25, 75% were viewed to sustain negligible, moderate, and substantial heterogeneity, respectively. A significance level of 0.05 was adopted.
Results
Characteristics of Included Studies
Our initial search yielded a total of 602 studies, which on implementing our inclusion/exclusion criteria, were reduced to five (Figure 1). Data from the included studies have been summarized in (Table 2). Of the five included studies, one was a randomized controlled trial, whereas four were controlled clinical trials.
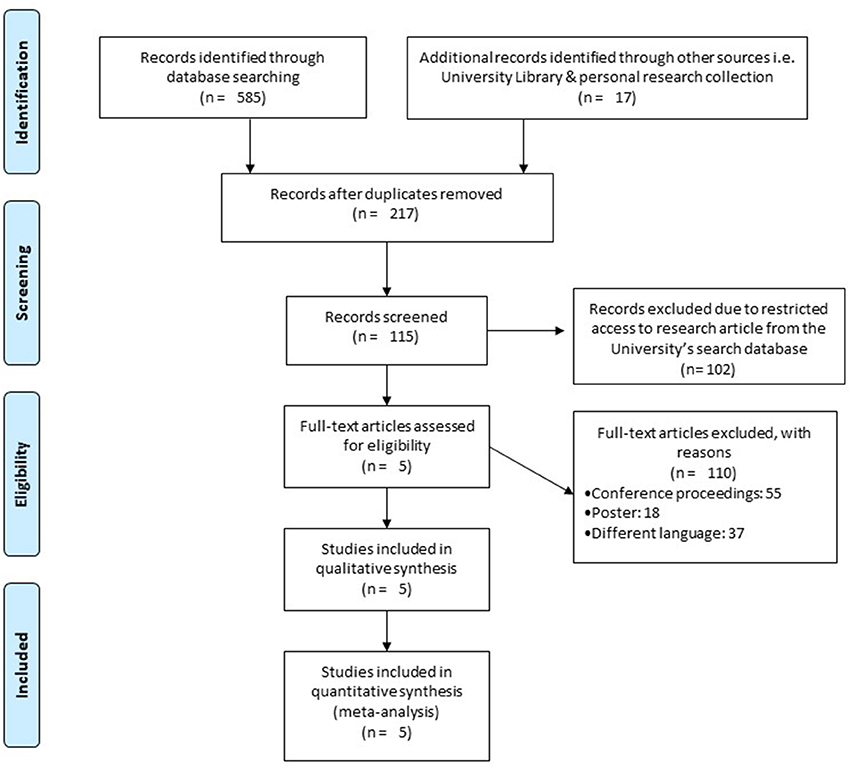
Figure 1. PRISMA flow chart for the inclusion of studies (68).
Participants
A total of 188 participants were analyzed in the incorporated studies (144 females/40 males). All the studies evaluated a mixed gender sample size.
Risk of Bias
To reduce the risks of bias, studies scoring ≥4 on PEDro were included in the review. Moreover, the limitation of research protocols to be included in the review were limited to gold standard randomized controlled trials, cluster randomized controlled trials and controlled clinical trials. The individual scores attained by the studies using the PEDro scale have been reported (Tables 2, 3). The average PEDro score for the five included studies were computed to be 5.2 out of 11, indicating fair-quality of the overall studies. One study scored 7, one scored 6, one scored 5, and two studies scored 4. Publication bias was analyzed by plotting a Hedge's g against standard error (Figure 2). Asymmetries concerning mean in the funnel plot might suggest bias (either positive or negative), in which case results are published. Risk of bias across the studies has been demonstrated in Figure 3.
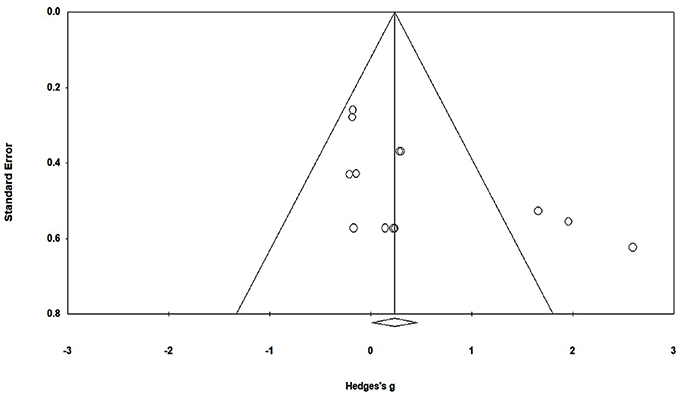
Figure 2. Funnel plot for Hedge's g & standardized effect for each effect in the meta-analysis. Each of the effect is represented in the plot as a circle. Funnel boundaries represent area where 95% of the effects are expected to abstain if there were no publication bias. The vertical line represents mean standardized effect of zero. Absence of publication bias is represented when the effects should be equally dispersed on either side of the line.
Meta-Analysis
Outcomes
The results suggest evidence for a positive impact of rhythmic auditory cueing on spatiotemporal gait parameters patients affected from multiple sclerosis. In the five included studies, all the studies reported significant enhancements in gait parameters with application of rhythmic auditory cueing.
Meta-Analyses
The evaluation of research studies via meta-analysis requires a strict inclusion criteria to efficiently limit the heterogeneity (81). However, among the pooled group of studies post a strict inclusion criterion, some amount of unexplained heterogeneity was still observed. Here, the few number of studies included in the meta-analysis limited our capability to perform additional sub-group analysis. The evaluated parameters were the spatiotemporal gait parameters such as gait velocity, cadence, stride length, and Timed-25 feet walking test.
Gait Velocity (Meter Per Second)
The meta-analysis on gait velocity for patients with multiple sclerosis revealed (Figure 4) a medium effect size in positive domain with moderate heterogeneity (Hedge's g: 0.67, 95% CI: 0.14 to 1.20, I2: 71.6%, p = 0.02).
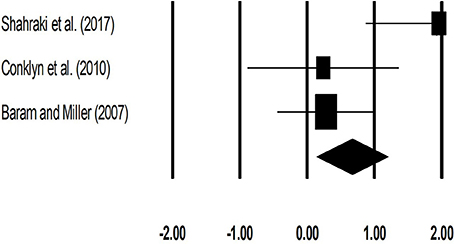
Figure 4. Forest plot illustrating individual studies evaluating the effects of rhythmic auditory cueing, on gait velocity (meter per second) for patients with multiple sclerosis. Weighted effect sizes; Hedge's g (boxes) and 95% C.I (whiskers) are presented, demonstrating repositioning errors for individual studies. The (Diamond) represents pooled effect sizes and 95% CI.
Stride Length (Meters)
The meta-analysis on stride length for patients with multiple sclerosis revealed (Figure 5) a medium effect size in positive domain with substantial heterogeneity (Hedge's g: 0.71, 95% CI: 0.17 to 1.26, I2: 82.3%, p = 0.03).
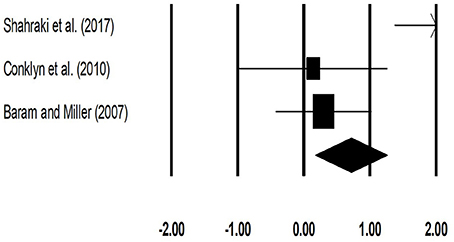
Figure 5. Forest plot illustrating individual studies evaluating the effects of rhythmic auditory cueing, on stride length (meters) for patients with multiple sclerosis. Weighted effect sizes; Hedge's g (boxes) and 95% C.I (whiskers) are presented, demonstrating repositioning errors for individual studies. The (Diamond) represents pooled effect sizes and 95% CI.
Cadence (Number of Steps Per Minute)
The meta-analysis on cadence for patients with multiple sclerosis revealed (Figure 6) a large effect size in positive domain with substantial heterogeneity (Hedge's g: 1.00, 95% CI: 0.24 to 1.76, I2: 70.3%, p = 0.06).
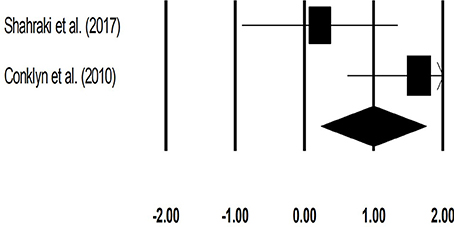
Figure 6. Forest plot illustrating individual studies evaluating the effects of rhythmic auditory cueing, on cadence (number of steps per minute) for patients with multiple sclerosis. Weighted effect sizes; Hedge's g (boxes) and 95% C.I (whiskers) are presented, demonstrating repositioning errors for individual studies. The (Diamond) represents pooled effect sizes and 95% CI.
Timed 25 Feet Walking Test (Seconds)
The meta-analysis for timed-25 feet walking test for patients with multiple sclerosis revealed (Figure 7) a small effect size in negative domain with substantial heterogeneity (Hedge's g: −0.17, 95% CI: −0.48 to 0.12, I2: 0%, p > 0.05).
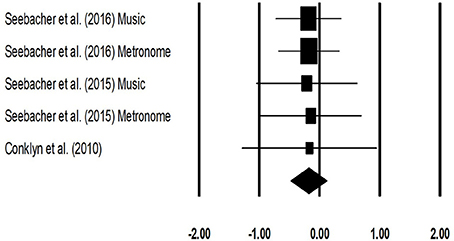
Figure 7. Forest plot illustrating individual studies evaluating the effects of rhythmic auditory cueing, on Timed 25 feet walking (seconds) test for patients with multiple sclerosis. Weighted effect sizes; Hedge's g (boxes) and 95% C.I (whiskers) are presented, demonstrating repositioning errors for individual studies. The (Diamond) represents pooled effect sizes and 95% CI.
Discussion
The primary objective of this present systematic review and meta-analysis was to develop a current state of knowledge for the effects of rhythmic auditory cueing on gait performance in patients with multiple sclerosis. All the included studies reported significant enhancements in gait performance post training with auditory cueing. The meta-analysis revealed significant small-to-large standardized effects for the beneficial influence of rhythmic auditory cueing on spatiotemporal gait parameters. Previous studies have reported a detrimental effect of multiple sclerosis on spatiotemporal gait parameters (16). For instance, Muratori et al. (82) has conclusively reported that a decrease in gait velocity, cadence, and stride length are important predictors for decreased quality of life, and increased fall related morbidity/mortality. Authors reported that gait velocity had a strong correlation with disease severity i.e., Expanded Disability Status scale and Multiple Sclerosis quality of life-54 scale. Likewise, Community Balance and Mobility scale has a strong relationship with step length and cadence (82). The current systematic review and meta-analysis reveals that training with rhythmic auditory cueing enhances gait velocity (Hedge's g: 0.67), stride length (0.70), cadence (1.0). Similarly, timed 25-foot walk test has been characterized as an important predictor to determine quality of life by focusing on functional independence and its impact on occupation, and social life (83–85). Here as well, a decrease in Timed 25-feet walking test (−0.17) was also reported in the analysis. This therefore suggests potential benefits of rhythmic auditory cueing for directly enhancing the quality of life and reducing morbidity/mortality ratios in patients with multiple sclerosis.
Neurophysiological mechanisms due to which auditory cueing enhances gait performance in patients with multiple sclerosis are not well-understood (16, 36, 42). In multiple sclerosis the onset of movement disorders is usually due to dysfunctions in white matter regions (16, 36, 86). Here inference can be drawn for the beneficial effects of auditory cueing, from a few studies analyzing the effects of auditory-sensorimotor training on white matter plasticity in musicians (87, 88). Bengtsson et al. (87) reported that auditory-sensorimotor training can increase myelination due to increased neural activity in the fiber tracts during training. The authors reported enhanced Fractional Anisotropy [usually reduced in multiple sclerosis (89, 90)] in corpus callosum, cortico-spinal, cortico-cortical tracts, and the posterior limb of the internal capsule. These neural structures are of critical importance when considering fine motor performance, bimanual coordination, auditory processing and motor learning (91, 92). Therefore, we hypothesize that training with auditory cueing could have enhanced the gait performance by facilitating the deficit white matter regions and/or mediating re-myelination. However, no research till date has analyzed the influence of auditory cueing on white matter plasticity in patients with multiple sclerosis. We strongly recommend future research to analyze the effects of auditory-motor entrainment on white matter plasticity in patients with multiple sclerosis.
Additionally, research in the past decades, for instance by Grimaud et al. (86) has reported that involvement of deep gray matter regions such as basal ganglia is unusual in patients with multiple sclerosis. However, recent evidence suggests that focal lesions and diffused neurodegeneration in deep gray matter regions such as basal ganglia, thalamus are an important precursors for contributing in development of neurological disabilities (1, 93–99), cognitive dysfunctions (97, 100), and the onset of fatigue (101, 102). Interestingly, research has also revealed a strong correlation between the quantitative susceptibility mapping of putamen and caudate nucleus with the severity of disease (97). Thereby suggesting greater involvement of gray matter structures with disease progression. This therefore again in our opinion might offer an additional explanation that application of rhythmic auditory cueing could have targeted the deficit basal ganglia circuitry similarly as in patients with Parkinson's disease to enhance gait performance, reduce the level of depression, anxiety, and fatigue in patients with multiple sclerosis [for a detailed mechanism see (47) and (27)]. Additionally, deficits in cerebellum [both gray and white matter regions (103)] have also been widely reported in patients with multiple sclerosis (104, 105). Here, findings of Molinari et al. (106) can justify the enhancements in gait performance with the application of auditory cueing. Molinari et al. (106) suggests that cerebellar dysfunctions such as in multiple sclerosis might impair the capability to consciously detect rhythmic variations for stabilizing motor response. However, the authors suggest that unconscious effects to entrain movements with external auditory cues might still be preserved in such patients. The authors suggest that in such cases the motor entrainment to auditory cueing might be induced unconsciously, independent of cerebellar processing at either the spinal or the cortical level. The authors proposed that computing of the timing information in such cases can be achieved peripherally i.e., directly in the auditory nerve by neural excitation patterns generated by precise physiological coding. This information can then be transferred directly into adjacent motor structures, which entrain with the neural motor codes and allow enhanced synchronization between the auditory stimuli and motor response (106, 107).
Furthermore, research suggests that application of auditory cueing can facilitate cortical reorganization in patients with multiple sclerosis (50). Till date only one research has analyzed the influence of rhythmic auditory cueing on cortical activation in patients with multiple sclerosis (108). The authors reported enhanced activation in left superior frontal gyrus, left anterior cingulate, and left superior temporal gyrus after gait training with rhythmic auditory cueing (36, 108). The increased activation in these neural centers has been associated with enhancements in executive functioning, auditory-motor entrainment, attention and motivation (50, 53). Similarly, enhanced activations in inferior colliculi (109), cerebellum, brainstem (110, 111), sensorimotor cortex (112, 113), premotor areas (114) have been reported post application of rhythmic auditory cueing in other movement disorders such as stroke and parkinsonism. Furthermore, modulation of neuromagnetic β oscillations (representing functional coordination between auditory-motor systems) with application of auditory cueing has been reported in auditory cortex, inferior frontal gyrus, somatosensory area, sensorimotor cortex and cerebellum (115). This ability of auditory cues has been recently demonstrated by Ross et al. (116) to facilitate immediate neural plasticity by facilitating feedforward mechanisms. Studies also suggest that training with rhythmic auditory cueing might offer reorganization of cortical and cerebellar circuits (63). Schaefer (117) for instance, suggested that auditory cueing infused with regularity and repetition of movement can result in an accelerated learning and neuroplasticity. Patients with multiple sclerosis have been reported to possess similar rapid-onset motor plasticity levels than that of healthy controls (118). Taken together, this evidence suggests strong therapeutic potential of external auditory stimulations to enhance gait performance in patients with multiple sclerosis. However, lack of conclusive evidence limits our interpretations, therefore we recommend future studies to analyse these components in neuroimaging studies.
Furthermore, extending beyond the neurophysiological effects of auditory stimulations Shahraki et al. (69) suggested that external auditory stimulations could also enhanced stability by facilitating the vestibular system via the medial-medial geniculate nuclei and organ of Corti (119). The authors demonstrated enhancements in spatiotemporal gait parameters with the application of rhythmic auditory cueing as compared to conventional physiotherapeutic gait training interventions in patients with multiple sclerosis. Likewise, Baram and Miller (42) too reported the beneficial aspects of external auditory cueing as compared to visual cueing. The authors reported higher gait velocity due to auditory cueing as compared to visual cueing, because of reduced reaction time facilitated by auditory stimulations during voluntary movements. The authors reported significant enhancements in gait velocity (Experimental: 12.8% vs. control: −3.0%) and stride length (8.3 vs. 0.3%) with the application of online rhythmic auditory cueing. Moreover, the authors demonstrated enhanced learning during residual performance (without auditory cueing) for both gait velocity (18.7 vs. 2.4%), and stride length (9.9 vs. 4.0%).
Moreover, we believe that the external auditory cueing could have also guided the gait of the patients' by explicitly synchronizing their ground contact and lift-off times (120). The cueing could have allowed the patients to effectively plan their movements before executing them (121). Likewise, enhanced kinematic efficiency and reduced variability in musculoskeletal activation patterns have been reported post training with rhythmic auditory cueing (26). Moreover, change in tempo of the auditory stimulation could have also played a major role in mediating gait performance. In the current review, only one study (69), trained their participants with a higher tempo (+10%) of rhythmic auditory cueing as compared to their preferred cadence. This “change in tempo” characteristic although not evaluated in the meta-analysis due to lack of data can serve as a crucial factor in rehabilitation of gait. For example, change in tempo has been associated with various neurophysiological changes such as increased neuronal activation in frontal-occipital cortical networks (122), and increased excitability of spinal motor neurons through the reticulospinal pathways (integral for reducing the response time in a motor task). Moreover, it has been reported that prolonged training with a constant pattern of rhythm can decrease fractal scaling of stride times from healthy 1/f structure (123–125). Here, we hypothesize that changing the tempo regularly during training can promote the development of a stable, and adaptable gait pattern. In rehabilitation this might serve as a measure to teach patients on how to regulate gait when passing through different fall prone environments.
Another crucial aspect analyzed in the current review is the effects of auditory cueing induced mental imagery in patients multiple sclerosis (71, 70). Labriffe et al. (126) reported higher activations in primary sensorimotor cortex and secondary somatosensory cortex bilaterally during the imagination of gait. The authors further reported correlated activations in bilateral somatosensory area and right pre-somatosensory area during mental imagery of gait. This training regime seems plausible in patients with multiple sclerosis where physical fatigue is a major concern for medical practitioners (127). Seebacher et al. (70) in their randomized controlled trial, asked the patients to kinaesthetically imagine gait from the first-person perspective with music and metronome induced rhythmic auditory cueing (71). The authors reported that mental imagery, which is usually diminished in patients with multiple sclerosis can be facilitated with rhythmic auditory cueing. Further, their study revealed significant enhancements spatiotemporal gait parameters such as timed 25-foot walking test, and 6-min walking test with the application of metronome/music-cued motor imagery groups. Here, comparable enhancements during 6-min walking test in music-cued (512.6 m), and metronome-cued (533.9 m) groups as compared to control group (471.2 m) clearly demonstrates beneficial effects of training with auditory cueing for enhancing physiological performance i.e., reduced fatigue. Likewise, improvements in multiple sclerosis related quality of life, pain, physical and mental health related quality of life were larger both music/metronome-cued groups as compared to control group. We would like to suggest that the beneficial effects of mental imagery here can also be effectively incorporated in home-based interventions. For instance, physiological fatigue might force the patient to train less at home. However, in such cases the patients can be taught to imagine themselves performing gait, while also imagining auditory cues. Previous studies suggest that the retention of enhancements in rehabilitation is dependent on how much the patient follows the treatment protocol at home (27, 28, 128). Therefore, developing interventions which can be easily followed by patients at home are desired. One of the included studies incorporated a home-based training intervention with external auditory cueing (41). Conklyn et al. (41) utilized a simple mp3 player to deliver rhythmic auditory cueing for practizing gait as a home-based intervention. The authors reported enhancements in spatiotemporal gait parameters and found increased patient adherence to the treatment. This type of home-based intervention could possibly be beneficial for people lacking proper exposure to medical interventions in developing countries (129). For instance, patients lacking effective medical resources can utilize smartphone devices with metronome applications for example Walkmate (124), Listenmee (130), or imagine gait with external stimulations or even imagine gait with auditory stimulations (joint audio-motor imagery).
Finally, a quantitative assessment for analyzing specific training dosage could not be performed in this study because of the limited amount of data and substantial heterogeneity in between the studies. Nevertheless, four of the included studies used a training regime that lasted for more than 17 min per session and was performed for at least three times a week for more than 3 weeks (41, 69–71). Likewise, based on the current evidence of training dosage for other movement disorders this dosage seems viable., for instance suggested a dosage of 25–40 min/session, for 3–5 sessions per week for patients with Parkinson's disease. Moreover, according to the findings of Bangert and Altenmüller (131) this training dosage seems plausible. The authors investigated cortical activation patterns during an audio-motor task and reported auditory-sensorimotor EEG co-activity after at least 20 min of training. Bangert and Altenmüller (131) speculate that this time frame is crucial for sensitive auditory monitoring, forming associations with the auditory target image in the working memory, during motor execution. Therefore, we suggest future studies to design training regimes with external auditory stimulations with at least 20 min training sessions. A limitation of the present review is that a meta-analysis was performed on a limited number of studies. Although, the main aim for conducting a meta-analysis was to allow a better understanding of the effects of auditory cueing over different spatiotemporal gait parameters for medical practitioners, patients and future researchers. This, however, does not rule out the possibility of incurring a type II error. We strongly suggest the reader to carefully interpret the results, while also considering the qualitative description of include studies provided in this review.
This review for the first time synthesized the evidence for effects of training with rhythmic auditory on gait in patients with multiple sclerosis. Our results are consistent with the findings of review studies suggesting the beneficial effects of rhythmic auditory cueing in healthy population (28), and population groups with movement disorders such as parkinsonism (47), stroke (57), and cerebral palsy (58). In conclusion, this review and meta-analysis suggests the incorporation of rhythmic auditory cueing for enhancing gait performance in patients with multiple sclerosis.
Future Directions
Extending beyond the beneficial effects of conventional isosynchronous auditory cueing, we recommend future studies to analyse the effects of biologically variable auditory stimulations on gait performance in patients with multiple sclerosis. Due to excessive sensory loss higher than normal threshold for action relevant acoustic input might be beneficial for patients with multiple sclerosis (132). Therefore, using ecologically valid action related sounds (walking on gravel, snow) conveying spatio-temporal information can possibly enhance saliency of sensory information for patients with multiple sclerosis (133–136). Similarly, analyzing the effects of methods providing real-time auditory information could possess considerable benefits for enhancing gait performance as well. This type of feedback allows converting the movement parameters in real-time to sound (mapping with pitch, amplitude). Here, the aim is to enhance motor perception and performance by targeting areas associated with biological motion perception (59, 137, 55). have shown that the synchronization of cyclic movement patterns with real-time auditory feedback can reduces variability and increases consistency of movements when compared with isosynchoronous rhythmic stimulations (56). According to this feedback can enable the patients to identify their own movement amplitudes and compare their produced sound patterns with the sound of an auditory movement model, thereby creating a new auditory reference framework. This then can possibly allow a better comparison between instructed and intended movement while simultaneously amplifying the internal representation of movements (138). In summary, we recommend future studies to focus on mediating auditory signal characteristics (ecologically valid, online feedback) for developing an efficient auditory stimulation, which can allow widespread benefits for patients with multiple sclerosis in both psychophysiological domains.
We also suggest future research to analyse the combined effects of external auditory stimulations with music therapy, as it might yield additional benefits to curb deficits in cognitive and physiological domain. For instance, Thaut et al. (139) demonstrated that musical mnemonics can facilitate a stronger oscillatory network synchronization in prefrontal regions during a word learning task in patients with multiple sclerosis. The authors suggested that musical stimuli might allow a “deep encoding” during a learning task and might also sharpen the timings of neural dynamics in brain which are normally degraded by the demyelination process. The authors also reported that this enhancement in cognitive performance was correlated with higher EDSS scores (139). Thereby, indicating that patients in more severe disease stages also benefited from the music facilitated “deep learning” strategies (139, 140). Likewise, enhanced cortical reorganization and regeneration in areas associated with cognition have been reported post music therapy (141, 142). We strongly recommend future research to analyse these effects in patients with multiple sclerosis. Furthermore, beneficial effects of music therapy in patients with multiple sclerosis has also been reported on respiratory musculature (143, 144). Future studies can focus on developing experimental protocols that use rhythmic cueing during music to facilitate breathing while performing gait. This approach might allow simultaneous strengthening of respiratory musculature while performing physical activities. Finally, it is important to consider the important psychological support that music therapy can offer to the patients with multiple sclerosis by reducing anxiety, depression, improving mood, self-acceptance and motivation (145–147). Future studies can also focus on analyzing these psychological aspects during the training regimes as this might allow in development of a multifaceted rehabilitation approach focusing on psychophysiological recovery of patients with multiple sclerosis.
Author Contributions
SG conceptualized the study, carried out the systematic-review, statistical analysis, and wrote the paper. IG assisted in the systematic-review process and reviewed the manuscript.
Conflict of Interest Statement
The authors declare that the research was conducted in the absence of any commercial or financial relationships that could be construed as a potential conflict of interest.
Acknowledgments
The publication of this article was funded by the Open Access fund of Leibniz Universität Hanover.
References
1. Prins M, Schul E, Geurts JP, van der Valk, Drukarch B, van Dam AM. Pathological differences between white and grey matter multiple sclerosis lesions. Ann NY Acad Sci. (2015) 1351:99–113. doi: 10.1111/nyas.12841
2. Prosperini L, Sbardella E, Raz E, Cercignani M, Tona F, Bozzali M, et al. Multiple sclerosis: white and gray matter damage associated with balance deficit detected at static posturography. Radiology (2013) 26:8181–9. doi: 10.1148/radiol.13121695
3. Haselkorn JK, Hughes C, Rae-Grant A, Henson LJ, Bever CT, Lo AC, et al. Summary of comprehensive systematic review: rehabilitation in multiple sclerosis. report of the guideline development, dissemination, and implementation subcommittee of the American Academy of Neurology. Neurology (2015) 85:1896–903. doi: 10.1212/WNL.0000000000002146
4. Solaro C, Ponzio M, Moran E, Tanganelli P, Pizio R, Ribizzi G, et al. The changing face of multiple sclerosis: prevalence and incidence in an aging population. Mult Scler. (2015) 21:1244–50. doi: 10.1177/1352458514561904
5. Lassmann H. Review: the architecture of inflammatory demyelinating lesions: implications for studies on pathogenesis. Neuropathol Appl Neurobiol. (2011) 37:698–710. doi: 10.1111/j.1365-2990.2011.01189.x
6. Tallantyre EC, Bo L, Al-Rawashdeh O, Owens T, Polman CH, Lowe JS, et al. Clinico-pathological evidence that axonal loss underlies disability in progressive multiple sclerosis. Mult Scler. (2010) 16:406–11. doi: 10.1177/1352458510364992
7. Ontaneda D, Thompson AJ, Fox RJ, Cohen JA. Progressive multiple sclerosis: prospects for disease therapy, repair, and restoration of function. Lancet (2017) 389:1357–66. doi: 10.1016/S0140-6736(16)31320-4
8. Zuvich RL, McCauley JL, Pericak-Vance MA, Haines JL. Genetics and pathogenesis of multiple sclerosis. Semin Immunol. (2009) 21:328–33. doi: 10.1016/j.smim.2009.08.003
9. Calabrese M, Poretto V, Favaretto A, Alessio S, Bernardi V, Romualdi C, et al. Cortical lesion load associates with progression of disability in multiple sclerosis. Brain (2012) 135:2952–61. doi: 10.1093/brain/aws246
10. Motl RW, Goldman MD, Benedict RH. Walking impairment in patients with multiple sclerosis: exercise training as a treatment option. Neuropsychiatr Dis Treat. (2010) 6:767. doi: 10.2147/NDT.S10480
11. Kutzelnigg A, Lassmann H. Cortical lesions and brain atrophy in MS. J Neurol Sci. (2005) 233:55–9. doi: 10.1016/j.jns.2005.03.027
12. Peterson JW, Bö, L, Mörk S, Chang A, Trapp BD. Transected neurites, apoptotic neurons, and reduced inflammation in cortical multiple sclerosis lesions. Ann Neurol. (2001) 50:389–400. doi: 10.1002/ana.1123
13. Thoumie P, Lamotte D, Cantalloube S, Faucher M, Amarenco G. Motor determinants of gait in 100 ambulatory patients with multiple sclerosis. Mult Scler. (2005) 11:485–91. doi: 10.1191/1352458505ms1176oa
14. Ghai S, Driller MW, Masters RSW. The influence of below-knee compression garments on knee-joint proprioception. Gait. Posture. (2018) 60:258–61. doi: 10.1016/j.gaitpost.2016.08.008
15. Matsuda PN, Shumway-Cook A, Bamer AM, Johnson SL, Amtmann D, Kraft GH. Falls in multiple sclerosis. Phys Med Rehabil. (2011) 3:624–32; quiz: 632. doi: 10.1016/j.pmrj.2011.04.015
16. Bethoux F. Gait disorders in multiple sclerosis. Continuum (2013) 19:1007–22. doi: 10.1212/01.CON.0000433286.92596.d5
17. Preiningerova JL, Novotna K, Rusz J, Sucha L, Ruzicka E, Havrdova E. Spatial and temporal characteristics of gait as outcome measures in multiple sclerosis (EDSS 0 to 6.5). J Neuroeng Rehabil. (2015) 12:14. doi: 10.1186/s12984-015-0001-0
18. McLoughlin JV, Barr CJ, Patritti B, Crotty M, Lord SR, Sturnieks DL. Fatigue induced changes to kinematic and kinetic gait parameters following six minutes of walking in people with multiple sclerosis. Disabil Rehabil. (2016) 38:535–43. doi: 10.3109/09638288.2015.1047969
19. Comber L, Galvin R, Coote S. Gait deficits in people with multiple sclerosis: a systematic review and meta-analysis. Gait Posture (2017) 51:25–35. doi: 10.1016/j.gaitpost.2016.09.026
20. Benedetti M, Piperno R, Simoncini L, Bonato P, Tonini A, Giannini S. Gait abnormalities in minimally impaired multiple sclerosis patients. Multiple Sclerosis J. (1999) 5:363–8. doi: 10.1177/135245859900500510
21. Nogueira LA, Teixeira L, Sabino P, Filho HA, Alvarenga RM, Thuler LC. Gait characteristics of multiple sclerosis patients in the absence of clinical disability. Disabil Rehabil. (2013) 35:1472–78. doi: 10.3109/09638288.2012.738760
22. van der Linden ML, Scott SM, Hooper JE, Cowan P, Mercer TH. Gait kinematics of people with multiple sclerosis and the acute application of functional electrical stimulation. Gait Posture (2014) 39:1092–6. doi: 10.1016/j.gaitpost.2014.01.016
23. Kelleher KJ, Spence W, Solomonidis S, Apatsidis D. The characterisation of gait patterns of people with multiple sclerosis. Disabil Rehabil. (2010) 32:1242–50. doi: 10.3109/09638280903464497
24. Wurdeman SR, Huisinga JM, Filipi M, Stergiou N. Multiple sclerosis alters the mechanical work performed on the body's center of mass during gait. J Appl Biomech. (2013) 29:435–42. doi: 10.1123/jab.29.4.435
25. Cofré, Lizama LE, Khan F, Lee PV, Galea MP. The use of laboratory gait analysis for understanding gait deterioration in people with multiple sclerosis. Mult Scler. (2016) 22:1768–76. doi: 10.1177/1352458516658137
26. Ghai S, Ghai I, Effenberg AO. Effect of rhythmic auditory cueing on gait in cerebral palsy: a systematic review and meta-analysis. Neuropsychiatr Dis Treat. (2018) 14:43–59. doi: 10.2147/NDT.S148053
27. Ghai S, Ghai I, Schmitz G, Effenberg AO. Effect of rhythmic auditory cueing on parkinsonian gait: a systematic review and meta-analysis. Sci Rep. (2018) 8:506. doi: 10.1038/s41598-017-16232-5
28. Ghai S, Ghai I, Effenberg AO. Effect of rhythmic auditory cueing on aging gait: a systematic review and meta-analysis. Aging Dis. (2017) 131–200. doi: 10.14336/AD.2017.1031
29. Motl RW, Pilutti LA. Is physical exercise a multiple sclerosis disease modifying treatment? Expert Rev Neurother. (2016) 16:951–60. doi: 10.1080/14737175.2016.1193008
30. Klaren RE, Sebastiao E, Chiu CY, Kinnett-Hopkins D, McAuley E, Motl RW. Levels and rates of physical activity in older adults with multiple sclerosis. Aging Dis. (2016) 7:278-84.
31. Massetti T, Trevizan IL, Arab C, Favero FM, Ribeiro-Papa DC, de Mello Monteiro CB. Virtual reality in multiple sclerosis - a systematic review. Mult Scler Relat Disord. (2016) 8:107–12. doi: 10.1016/j.msard.2016.05.014
32. Amatya B, Khan F, Ng L, Galea M. Rehabilitation for people with multiple sclerosis: an overview of Cochrane systematic reviews. Cochrane Database Syst Rev. (2017) CD012732. doi: 10.1002/14651858.CD012732
33. Castro-Sánchez AM, Matarán-Peñarrocha GA, Lara-Palomo I, Saavedra-Hernández M, Arroyo-Morales M, Moreno-Lorenzo C. Hydrotherapy for the treatment of pain in people with multiple sclerosis: a randomized controlled trial. Evid Based Complement Altern Med. (2012) 2012:473963. doi: 10.1155/2012/473963
34. Burschka JM, Keune PM, Oy UH, Oschmann P, Kuhn P. Mindfulness-based interventions in multiple sclerosis: beneficial effects of Tai Chi on balance, coordination, fatigue and depression. BMC Neurol. (2014) 14:165. doi: 10.1186/s12883-014-0165-4
35. Baram Y, Miller A. Glide-symmetric locomotion reinforcement in patients with multiple sclerosis by visual feedback. Disabil Rehabil Assist Technol. (2010) 5:323–6. doi: 10.3109/17483101003671717
36. Bethoux F. Functionality, music and multiple sclerosis. Altern Complement Ther. (2017) 23:125–8. doi: 10.1089/act.2017.29120.fbe
37. Rolak LA. Multiple sclerosis: it's not the disease you thought it was. Clin Med Res. (2003) 1:57–60. doi: 10.3121/cmr.1.1.57
38. Sakai RE, Feller DJ, Galetta KM, Galetta SL, Balcer LJ. Vision in multiple sclerosis (MS): the story, structure-function correlations, models for neuroprotection. J Neuroophthalmol. (2011) 31:362–73. doi: 10.1097/WNO.0b013e318238937
39. Rougier P, Faucher M, Cantalloube S, Lamotte D, Vinti M, Thoumie P. How proprioceptive impairments affect quiet standing in patients with multiple sclerosis. Somatosens Motor Res. (2007) 24:41–51. doi: 10.1080/08990220701318148
40. Ghai S, Driller M, Ghai I. Effects of joint stabilizers on proprioception and stability: a systematic review and meta-analysis. Phys Ther Sport. (2017) 25:65–75. doi: 10.1016/j.ptsp.2016.05.006
41. Conklyn D, Stough D, Novak E, Paczak S, Chemali K, Bethoux F. A home-based walking program using rhythmic auditory stimulation improves gait performance in patients with multiple sclerosis: a pilot study. Neurorehabil Neural Repair (2010) 24:835–42. doi: 10.1177/1545968310372139
42. Baram Y, Miller A. Auditory feedback control for improvement of gait in patients with Multiple Sclerosis. J Neurol Sci. (2007) 254:90–4. doi: 10.1016/j.jns.2007.01.003
43. Spaulding SJ, Barber B, Colby M, Cormack B, Mick T, Jenkins ME. Cueing and gait improvement among people with Parkinson's disease: a meta-analysis. Arch Phys Med Rehabil. (2013) 94:562–70. doi: 10.1016/j.apmr.2012.10.026
44. Thaut MH, Abiru M. Rhythmic auditory stimulation in rehabilitation of movement disorders: a review of current research. Music Percept Interdiscipl J. (2010) 27:263–9. doi: 10.1525/mp.2010.27.4.263
45. Raglio A. Music therapy interventions in Parkinson's disease: the state-of-the-art. Front Neurol. (2015) 6:185. doi: 10.3389/fneur.2015.00185
46. Shelton J, Kumar GP. Comparison between auditory and visual simple reaction times. Neurosci Med. (2010) 1:30. doi: 10.4236/nm.2010.11004
47. Nombela C, Hughes LE, Owen AM, Grahn JA. Into the groove: can rhythm influence Parkinson's disease? Neurosci Biobehav. Rev. (2013) 37:2564–70. doi: 10.1016/j.neubiorev.2013.08.003
48. Ermolaeva VY, Borgest A. Intercortical connections of the auditory areas with the motor area. Neurosci Behav Physiol. (1980) 10:210–5. doi: 10.1007/BF01182212
49. Felix RA, Fridberger A, Leijon S, Berrebi AS, Magnusson AK. Sound rhythms are encoded by postinhibitory rebound spiking in the superior paraolivary nucleus. J Neurosci. (2011) 31:12566–78. doi: 10.1523/JNEUROSCI.2450-11.2011
50. Thaut MH, McIntosh GC, Hoemberg V. Neurobiological foundations of neurologic music therapy: rhythmic entrainment and the motor system. Front Psychol. (2014) 5:1185. doi: 10.3389/fpsyg.2014.01185
52. Thaut MH. Neural basis of rhythmic timing networks in the human brain. Ann NY Acad Sci. (2003) 999:364–73. doi: 10.1196/annals.1284.044
53. Thaut MH. Rhythm, Music, and the Brain: Scientific Foundations and Clinical Applications. New York, NY: Routledge (2005).
54. Bukowska AA, Krezałek P, Mirek E, Bujas P, Marchewka A. Neurologic music therapy training for mobility and stability rehabilitation with Parkinson's disease–A pilot study. Front Hum Neurosci. (2015) 9:710. doi: 10.3389/fnhum.2015.00710
55. Effenberg AO, Fehse U, Schmitz G, Krueger B, Mechling H. Movement sonification: Effects on motor learning beyond rhythmic adjustments. Front Neurosci. (2016) 10:219. doi: 10.3389/fnins.2016.00219
56. Ghai S, Schmitz G, Hwang TH, Effenberg AO. Auditory proprioceptive integration: effects of real-time kinematic auditory feedback on knee proprioception. Front Neurosci. (2018) 12:142. doi: 10.3389/fnins.2018.00142
57. Yoo GE, Kim SJ. Rhythmic auditory cueing in motor rehabilitation for stroke patients: systematic review and meta-analysis. J Music Ther. (2016) 53:149–77. doi: 10.1093/jmt/thw003
58. Kim SJ, Kwak EE, Park ES, Lee DS, Kim KJ, Song JE, et al. Changes in gait patterns with rhythmic auditory stimulation in adults with cerebral palsy. Neurorehabilitation (2011) 29:233–41. doi: 10.3233/NRE-2011-0698
59. Schmitz G, Mohammadi B, Hammer A, Heldmann M, Samii A, Münte TF, et al. Observation of sonified movements engages a basal ganglia frontocortical network. BMC Neurosci. (2013) 14:1. doi: 10.1186/1471-2202-14-32
60. Heremans E, Nieuwboer A, Spildooren J, De Bondt S, D'hooge AM, Helsen W, et al. Cued motor imagery in patients with multiple sclerosis. Neuroscience (2012) 206:115–21. doi: 10.1016/j.neuroscience.2011.12.060
61. Heremans E, Nieuwboer A, Feys P, Vercruysse S, Vandenberghe W, Sharma N, et al. External cueing improves motor imagery quality in patients with Parkinson disease. Neurorehabil Neural Repair (2012) 26:27–35. doi: 10.1177/1545968311411055
62. Miller RA, Thaut MH, McIntosh GC, Rice RR. Components of EMG symmetry and variability in parkinsonian and healthy elderly gait. Electroencephalogr Clin Neurophysiol. (1996) 101:1–7. doi: 10.1016/0013-4694(95)00209-X
63. Luft AR, McCombe-Waller S, Whitall J, Forrester LW, Macko R, Sorkin JD, et al. Repetitive bilateral arm training and motor cortex activation in chronic stroke: a randomized controlled trial. J Am Med Assoc. (2004) 292:1853–61. doi: 10.1001/jama.292.15.1853
64. Rochester L, Baker K, Nieuwboer A, Burn D. Targeting dopa-sensitive and dopa-resistant gait dysfunction in Parkinson's disease: selective responses to internal and external cues. Mov Disord. (2011) 26:430–5. doi: 10.1002/mds.23450
65. Flachenecker P, Kobelt G, Berg J, Capsa D, Gannedahl M. New insights into the burden and costs of multiple sclerosis in Europe: results for Germany. Mult Scler. (2017) 23:78–90. doi: 10.1177/1352458517708141
66. Ernstsson O, Gyllensten H, Alexanderson K, Tinghög P, Friberg E, Norlund A. Cost of illness of multiple sclerosis - a systematic review. PLoS ONE (2016) 11:e0159129. doi: 10.1371/journal.pone.0159129
67. Naci H, Fleurence R, Birt J, Duhig A. Economic burden of multiple sclerosis: a systematic review of the literature. Pharmacoeconomics (2010) 28:363–79. doi: 10.2165/11532230-000000000-00000
68. Liberati A, Altman DG, Tetzlaff J, Mulrow C, Gøtzsche PC, Ioannidis JP, et al. The PRISMA statement for reporting systematic reviews and meta-analyses of studies that evaluate health care interventions: explanation and elaboration. Ann Intern Med. (2009) 151:W65–94. doi: 10.7326/0003-4819-151-4-200908180-00136
69. Shahraki M, Sohrabi M, Torbati HT, Nikkhah K, NaeimiKia M. Effect of rhythmic auditory stimulation on gait kinematic parameters of patients with multiple sclerosis. J Med Life (2017) 10:33.
70. Seebacher B, Kuisma R, Glynn A, Berger T. The effect of rhythmic-cued motor imagery on walking, fatigue and quality of life in people with multiple sclerosis: a randomised controlled trial. Mult. Scler (2016) 23:286–96. doi: 10.1177/1352458516644058
71. Seebacher B, Kuisma R, Glynn A, Berger T. Rhythmic cued motor imagery and walking in people with multiple sclerosis: a randomised controlled feasibility study. Pilot Feasibil Stud. (2015) 1:25. doi: 10.1186/s40814-015-0021-3
72. de Morton NA. The PEDro scale is a valid measure of the methodological quality of clinical trials: a demographic study. Austral J Physiother. (2009) 55:129–33. doi: 10.1016/S0004-9514(09)70043-1
73. Maher CG, Sherrington C, Herbert RD, Moseley AM, Elkins M. Reliability of the PEDro scale for rating quality of randomized controlled trials. Phys Ther. (2003) 83:713–21. doi: 10.1093/ptj/83.8.713
74. Teasell R, Foley N, Salter K, Bhogal S, Jutai J. Evidence-based review of stroke rehabilitation: executive summary, 12th edn. Top Stroke Rehabil. (2009) 16:463–88. doi: 10.1310/tsr1606-463
75. Ramsey L, Winder RJ, McVeigh JG. The effectiveness of working wrist splints in adults with rheumatoid arthritis: a mixed methods systematic review. J Rehabil Med. (2014) 46:481–92. doi: 10.2340/16501977-1804
76. Longford NT. Estimation of the effect size in meta-analysis with few studies. Stat Med. (2010) 29:421–30. doi: 10.1002/sim.3814
77. Borenstein M, Hedges LV, Higgins J, Rothstein HR. A basic introduction to fixed-effect and random-effects models for meta-analysis. Res Syn Methods (2010) 1:97–111. doi: 10.1002/jrsm.12
78. Higgins JPT, Green S. (editors). Cochrane Handbook for Systematic Reviews of Interventions Version 5.1.0 [updated March 2011]. The Cochrane Collaboration (2011). Available online at: http://handbook.cochrane.org
79. Cumming G. Understanding the New Statistics: Effect Sizes, Confidence Intervals, and Meta-Analysis. New York, NY: Routledge (2013).
80. Cohen J. Statistical Power Analysis for the Behavioral Sciences. Hillsdale, NJ: Lawrence Erlbaum Associates, Inc. (1988).
81. Bolier L, Haverman M, Westerhof GJ, Riper H, Smit F, Bohlmeijer E. Positive psychology interventions: a meta-analysis of randomized controlled studies. BMC Public Health (2013) 13:119. doi: 10.1186/1471-2458-13-119
82. Muratori L, Martin E, Fafard L, Bumstead B, Zarif M, Gudesblatt M. Multiple sclerosis, EDSS and gait: putting legs that work on a walking scale (P2.126). Neurology (2016) 86. Available online at: http://n.neurology.org/content/86/16_Supplement/P2.126
83. Goldman MD, Motl RW, Scagnelli J, Pula JH, Sosnoff JJ, Cadavid D. Clinically meaningful performance benchmarks in MS: Timed 25-Foot walk and the real world. Neurology (2013) 811:856–1863. doi: 10.1212/01.wnl.0000436065.97642.d2
84. Motl RW, Cohen JA, Benedict R, Phillips G, LaRocca N, Hudson LD, et al. Validity of the timed 25-foot walk as an ambulatory performance outcome measure for multiple sclerosis. Mult Scler. (2017) 23:704–10. doi: 10.1177/1352458517690823
85. Hakim EA, Bakheit AM, Bryant TN, Roberts MW, McIntosh-Michaelis SA, Spackman AJ, et al. The social impact of multiple sclerosis–a study of 305 patients and their relatives. Disabil Rehabil. (2000) 22:288–93. doi: 10.1080/096382800296755
86. Grimaud J, Millar J, Thorpe JW, Moseley IF, McDonald WI, Miller DH. Signal intensity on MRI of basal ganglia in multiple sclerosis. J Neurol Neurosurg Psychiatry (1995) 59:306–8. doi: 10.1136/jnnp.59.3.306
87. Bengtsson SL, Nagy Z, Skare S, Forsman L, Forssberg H, Ullén F. Extensive piano practicing has regionally specific effects on white matter development. Nat Neurosci. (2005) 8:1148. doi: 10.1038/nn1516
88. Imfeld A, Oechslin MS, Meyer M, Loenneker T, Jancke L. White matter plasticity in the corticospinal tract of musicians: a diffusion tensor imaging study. Neuroimage (2009) 46:600–7. doi: 10.1016/j.neuroimage.2009.02.025
89. Elshafey R, Hassanien O, Khalil M. Diffusion tensor imaging for characterizing white matter changes in multiple sclerosis. Egypt J Radiol Nuclear Med. (2014) 45:881–8. doi: 10.1016/j.ejrnm.2014.04.006
90. Inglese M, Bester M. Diffusion imaging in multiple sclerosis: research and clinical implications. NMR Biomed. (2010) 23:865–72. doi: 10.1002/nbm.1515
91. Swinnen SP, Wenderoth N. Two hands, one brain: cognitive neuroscience of bimanual skill. Trends Cogn Sci. (2004) 8:18–25. doi: 10.1016/j.tics.2003.10.017
92. Tanji J. Sequential organization of multiple movements: involvement of cortical motor areas. Ann Rev Neurosci. (2001) 24:631–51. doi: 10.1146/annurev.neuro.24.1.631
93. Minagar A, Barnett MH, Benedict RH, Pelletier D, Pirko I, Sahraian MA, et al. The thalamus and multiple sclerosis: modern views on pathologic, imaging, and clinical aspects. Neurology (2013) 80:210–9. doi: 10.1212/WNL.0b013e31827b910b
94. Pirko I, Lucchinetti CF, Sriram S, Bakshi R. Gray matter involvement in multiple sclerosis. Neurology (2007) 68:634–42. doi: 10.1212/01.wnl.0000250267.85698.7a
95. Haider L, Simeonidou C, Steinberger G, Hametner S, Grigoriadis N, Deretzi G, et al. Multiple sclerosis deep grey matter: the relation between demyelination, neurodegeneration, inflammation and iron. J Neurol Neurosurg Psychiatry (2014) 85:1386–95. doi: 10.1136/jnnp-2014-307712
96. Krutenkova E, Aitmagambetova G, Khodanovich M, Bowen J, Gangadharan B, Henson L, et al. Demyelination of subcortical nuclei in multiple sclerosis. J Phys Conf Ser. (2016) 677:012006. doi: 10.1088/1742-6596/677/1/012006
97. Schmalbrock P, Prakash RS, Schirda B, Janssen A, Yang GK, Russell M, et al. Basal ganglia iron in patients with multiple sclerosis measured with 7T quantitative susceptibility mapping correlates with inhibitory control. Am J Neuroradiol. (2016) 37:439–46. doi: 10.3174/ajnr.A4599
98. Hasan KM, Walimuni IS, Abid H, Frye RE, Ewing-Cobbs L, Wolinsky JS, et al. Multimodal quantitative magnetic resonance imaging of thalamic development and aging across the human lifespan: implications to neurodegeneration in multiple sclerosis. J Neurosci. (2011) 31:16826–32. doi: 10.1523/JNEUROSCI.4184-11.2011
99. Cifelli A, Arridge M, Jezzard P, Esiri MM, Palace J, Matthews PM. Thalamic neurodegeneration in multiple sclerosis. Ann Neurol. (2002) 52:650–3. doi: 10.1002/ana.10326
100. Cui F, Zhou L, Wang Z, Lang C, Park J, Tan Z, et al. Altered functional connectivity of striatal subregions in patients with multiple sclerosis. Front Neurol. (2017) 8:129. doi: 10.3389/fneur.2017.00129
101. Finke C, Schlichting J, Papazoglou S, Scheel M, Freing A, et al. Altered basal ganglia functional connectivity in multiple sclerosis patients with fatigue. Mult Scler. (2014) 21:925–34. doi: 10.1177/1352458514555784
102. DeLuca J, Genova HM, Capili EJ, Wylie GR. Functional neuroimaging of fatigue. Phys Med Rehabil Clin N Am. (2009) 20:325–37. doi: 10.1016/j.pmr.2008.12.007
103. Koppelmans V, Hirsiger S, Merillat S, Jancke L, Seidler RD. Cerebellar gray and white matter volume and their relation with age and manual motor performance in healthy older adults. Hum Brain Mapp. (2015) 36:2352–63. doi: 10.1002/hbm.22775
104. Wilkins A. Cerebellar dysfunction in multiple sclerosis. Front Neurol. (2017) 8:312. doi: 10.3389/fneur.2017.00312
105. Weier K, Banwell B, Cerasa A, Collins DL, Dogonowski AM, Lassmann H, et al. The role of the cerebellum in multiple sclerosis. Cerebellum (2015) 14:364–74. doi: 10.1007/s12311-014-0634-8
106. Molinari M, Leggio MG, De Martin M, Cerasa, A, Thaut M. Neurobiology of rhythmic motor entrainment. Ann NY Acad Sci. (2003) 999:313–21. doi: 10.1196/annals.1284.042
107. Ghai S, Ghai I, Effenberg AO. “Low road” to rehabilitation: a perspective on subliminal sensory neuroprosthetics. Neuropsychiatr Dis Treat. (2018) 14:301. doi: 10.2147/NDT.S153392
108. Bethoux F, Lowe M, Koenig K, Stough D, Gallagher L, Conklyn D. Effects of Rhythmic Auditory Stimulation on Gait on Cortical Activation with Mental Imagery of Walking in Patients with MS, CMSC ACTRIMS Cooperative Meeting. Dallas, TX (2014). Available online at: https://cmsc.confex.com/cmsc/2014/webprogram/Paper2764.html
109. Tierney A, Kraus N. The ability to move to a beat is linked to the consistency of neural responses to sound. J Neurosci. (2013) 33:14981–8. doi: 10.1523/JNEUROSCI.0612-13.2013
110. Debaere F, Wenderoth N, Sunaert S, Van Hecke P, Swinnen SP. Internal vs. external generation of movements: differential neural pathways involved in bimanual coordination performed in the presence or absence of augmented visual feedback. Neuroimage (2003) 19:764–76. doi: 10.1016/S1053-8119(03)00148-4
111. Hausdorff JM, Lowenthal J, Herman T, Gruendlinger L, Peretz C, Giladi N. Rhythmic auditory stimulation modulates gait variability in Parkinson's disease. Eur J Neurosci. (2007) 26:2369–75. doi: 10.1111/j.1460-9568.2007.05810.x
112. Asanuma H, Keller A. Neuronal mechanisms of motor learning in mammals. Neuroreport (1991) 2:217–24. doi: 10.1097/00001756-199105000-00001
113. Suh JH, Han SJ, Jeon SY, Kim HJ, Lee JE, Yoon TS, et al. Effect of rhythmic auditory stimulation on gait and balance in hemiplegic stroke patients. Neurorehabilitation (2014) 34:193–9. doi: 10.3233/NRE-131008
114. Grahn JA, Brett M. Rhythm and beat perception in motor areas of the brain. J Cogn Neurosci. (2007) 19:893–906. doi: 10.1162/jocn.2007.19.5.893
115. Fujioka T, Trainor LJ, Large EW, Ross B. Internalized timing of isochronous sounds is represented in neuromagnetic beta oscillations. J Neurosci. (2012) 32:1791–802. doi: 10.1523/JNEUROSCI.4107-11.2012
116. Ross B, Barat M, Fujioka T. Sound-making actions lead to immediate plastic changes of neuromagnetic evoked responses and induced beta-band oscillations during perception. J Neurosci. (2017) 37:5948–59. doi: 10.1523/JNEUROSCI.3613-16.2017
117. Schaefer RS. Auditory rhythmic cueing in movement rehabilitation: findings and possible mechanisms. Philos Trans R Soc B Biol Sci. (2014) 369:20130402. doi: 10.1098/rstb.2013.0402
118. Zeller D, aufm Kampe K, Biller A, Stefan K, Gentner R, Schutz A, et al. Rapid-onset central motor plasticity in multiple sclerosis. Neurology (2010) 74:728–35. doi: 10.1212/WNL.0b013e3181d31dcf
119. Horak FB. Clinical measurement of postural control in adults. Phys Ther. (1987) 67:1881–5. doi: 10.1093/ptj/67.12.1881
120. Ford MP, Malone LA, Nyikos I, Yelisetty R, Bickel CS. Gait training with progressive external auditory cueing in persons with Parkinson's disease. Arch Phys Med Rehabil. (2010) 91:1255–61. doi: 10.1016/j.apmr.2010.04.012
121. Thaut MH, Leins AK, Rice RR, Argstatter H, Kenyon GP, McIntosh GC, et al. Rhythmic auditory stimulation improves gait more than NDT/Bobath training in near-ambulatory patients early poststroke: a single-blind, randomized trial. Neurorehabil Neural Repair (2007) 21:455–9. doi: 10.1177/1545968307300523
122. Thaut MH, Gardiner JC, Holmberg D, Horwitz J, Kent L, Andrews G, et al. Neurologic music therapy improves executive function and emotional adjustment in traumatic brain injury rehabilitation. Ann NY Acad Sci. (2009) 1169:406–16. doi: 10.1111/j.1749-6632.2009.04585.x
123. Delignières D, Torre K. Fractal dynamics of human gait: a reassessment of the 1996 data of Hausdorff et al. J Appl Physiol. (2009) 106:1272–79. doi: 10.1152/japplphysiol.90757.2008
124. Hove MJ, Suzuki K, Uchitomi H, Orimo S, Miyake Y. Interactive rhythmic auditory stimulation reinstates natural 1/f timing in gait of Parkinson's patients. PLoS ONE (2012) 7:e32600. doi: 10.1371/journal.pone.0032600
125. Hausdorff JM, Purdon PL, Peng C, Ladin Z, Wei JY, Goldberger AL. Fractal dynamics of human gait: stability of long-range correlations in stride interval fluctuations. J Appl Physiol. (1996) 80:1448–57. doi: 10.1152/jappl.1996.80.5.1448
126. Labriffe M, Annweiler C, Amirova LE, Gauquelin-Koch G, Ter, Minassian A, Leiber, LM, et al. Brain activity during mental imagery of gait versus gait-like plantar stimulation: a novel combined functional mri paradigm to better understand cerebral gait control. Front Hum Neurosci. (2017) 11:106. doi: 10.3389/fnhum.2017.00106
127. Demougeot L, Papaxanthis C. Muscle fatigue affects mental simulation of action. J Neurosci. (2011) 31:10712–20. doi: 10.1523/JNEUROSCI.6032-10.2011
128. Ghai S, Ghai I, Effenberg AO. Effects of dual tasks and dual-task training on postural stability: a systematic review and meta-analysis. Clin Interv Aging (2017) 12:557–77. doi: 10.2147/CIA.S125201
129. Browne P, Chandraratna D, Angood C, Tremlett H, Baker C, Taylor BV, et al. Atlas of multiple sclerosis 2013: a growing global problem with widespread inequity. Neurology (2014) 83:1022–4. doi: 10.1212/WNL.0000000000000768
130. Lopez WO, Higuera CA, Fonoff ET, Souza Cde O, Albicker U, Martinez JA. Listenmee and Listenmee smartphone application: synchronizing walking to rhythmic auditory cues to improve gait in Parkinson's disease. Hum Mov Sci. (2014) 37:147–56. doi: 10.1016/j.humov.2014.08.001
131. Bangert M, Altenmüller EO. Mapping perception to action in piano practice: a longitudinal DC-EEG study. BMC Neurosci. (2003) 4:26. doi: 10.1186/1471-2202-4-26
132. Furst M, Levine RA. Hearing disorders in multiple sclerosis. Handb Clin Neurol. (2015) 129:649–65. doi: 10.1016/B978-0-444-62630-1.00036-6
133. Gaver WW. How do we hear in the world? Explorations in ecological acoustics. Ecol Psychol. (1993) 5:285–313. doi: 10.1207/s15326969eco0504_2
134. Young WR, Rodger MW, Craig CM. Auditory observation of stepping actions can cue both spatial and temporal components of gait in Parkinson? s disease patients. Neuropsychologia (2014) 57:140–53. doi: 10.1016/j.neuropsychologia.2014.03.009
135. Young W, Rodger M, Craig CM. Perceiving and reenacting spatiotemporal characteristics of walking sounds. J Exp Psychol Hum Percept Perform. (2013) 39:464. doi: 10.1037/a0029402
136. Dotov D, Bayard S, de Cock VC, Geny C, Driss V, Garrigue G et al. Biologically-variable rhythmic auditory cues are superior to isochronous cues in fostering natural gait variability in Parkinson's disease. Gait Posture (2017) 51:64–9. doi: 10.1016/j.gaitpost.2016.09.020
137. Effenberg AO. Sensory Systems: auditory, tactile, proprioceptive. In: Eklund RC, Tenenbaum G, editors. Encyclopedia of Sport and Exercise Psychology. Los Angeles, CA: SAGE Publications (2014). p. 663–7.
138. Tagliabue M, McIntyre J. A modular theory of multisensory integration for motor control. Front Comput Neurosci. (2014) 8:1. doi: 10.3389/fncom.2014.00001
139. Thaut MH, Peterson DA, McIntosh GC, Hoemberg V. Music mnemonics aid verbal memory and induce learning – related brain plasticity in multiple sclerosis. Front Hum Neurosci. (2014) 8:395. doi: 10.3389/fnhum.2014.00395
140. Hallam S, Cross I, Thaut M. Oxford Handbook of Music Psychology. Oxford: Oxford University Press (2011).
141. Särkämö T., Altenmüller E, Rodríguez-Fornells A, Peretz I. Editorial: music, brain, and rehabilitation: emerging therapeutic applications and potential neural mechanisms. Front Hum Neurosci. (2016) 10:103. doi: 10.3389/fnhum.2016.00103
142. Särkämö T, Ripollés P, Vepsäläinen H, Autti T, Silvennoinen HM, Salli E, et al. Structural changes induced by daily music listening in the recovering brain after middle cerebral artery stroke: a voxel-based morphometry study. Front Hum Neurosci. (2014) 8:245. doi: 10.3389/fnhum.2014.00245
143. Wiens ME, Reimer MA, Guyn HL. Music therapy as a treatment method for improving respiratory muscle strength in patients with advanced multiple sclerosis: a pilot study. Rehabil Nurs. (1999) 24:74–80. doi: 10.1002/j.2048-7940.1999.tb01840.x
144. Weller CM, Baker FA. The role of music therapy in physical rehabilitation: a systematic literature review. Nordic J Mus Ther. (2011) 20:43–61. doi: 10.1080/08098131.2010.485785
145. Aldridge D, Schmid W, Kaeder M, Schmidt C, Ostermann T. Functionality or aesthetics? A pilot study of music therapy in the treatment of multiple sclerosis patients. Complement Ther. Med. (2005) 13:25–33. doi: 10.1016/j.ctim.2005.01.004
146. Raglio A, Attardo L, Gontero G, Rollino S, Groppo E, Granieri E. Effects of music and music therapy on mood in neurological patients. World J Psychiatry (2015) 5:68–78. doi: 10.5498/wjp.v5.i1.68
Keywords: rhythm perception, gait, movement disorders, rehabilitation, falls, spasticity
Citation: Ghai S and Ghai I (2018) Effects of Rhythmic Auditory Cueing in Gait Rehabilitation for Multiple Sclerosis: A Mini Systematic Review and Meta-Analysis. Front. Neurol. 9:386. doi: 10.3389/fneur.2018.00386
Received: 05 March 2018; Accepted: 11 May 2018;
Published: 11 June 2018.
Edited by:
Robert Weissert, University of Regensburg, GermanyReviewed by:
Matthias Grothe, University of Greifswald, GermanyWalter Maetzler, Christian-Albrechts-Universität zu Kiel, Germany
Copyright © 2018 Ghai and Ghai. This is an open-access article distributed under the terms of the Creative Commons Attribution License (CC BY). The use, distribution or reproduction in other forums is permitted, provided the original author(s) and the copyright owner are credited and that the original publication in this journal is cited, in accordance with accepted academic practice. No use, distribution or reproduction is permitted which does not comply with these terms.
*Correspondence: Shashank Ghai, shashank.ghai@sportwiss.uni-hannover.de