- 1Department of Neurology, Samsung Medical Center, Sungkyunkwan University School of Medicine, Seoul, South Korea
- 2Department of Health Sciences and Technology, Samsung Advanced Institute for Health Sciences and Technology, Sungkyunkwan University, Seoul, South Korea
- 3Stroke Center and Korean Brain MRI Data Center, Dongguk University Ilsan Hospital, Goyang, South Korea
- 4Samsung Medical Center, Clinical Research Institute, Seoul, South Korea
Revascularization therapies have been established as the treatment mainstay for acute ischemic stroke. However, a substantial number of patients are either ineligible for revascularization therapy, or the treatment fails or is futile. At present, non-contrast computed tomography is the first-line neuroimaging modality for patients with acute stroke. The use of magnetic resonance imaging (MRI) to predict the response to early revascularization therapy and to identify patients for delayed treatment is desirable. MRI could provide information on stroke pathophysiologies, including the ischemic core, perfusion, collaterals, clot, and blood–brain barrier status. During the past 20 years, there have been significant advances in neuroimaging as well as in revascularization strategies for treating patients with acute ischemic stroke. In this review, we discuss the role of MRI and post-processing, including machine-learning techniques, and recent advances in MRI-based triage for revascularization therapies in acute ischemic stroke.
Introduction
Revascularization therapies, including rt-PA and EVT, have been established as the mainstay of treatment for acute ischemic stroke. It has become clear that consideration of heterogeneity among stroke patients is of importance in these therapies. Neuroimaging has been used as a triage tool for revascularization therapy in patients with acute stroke. The use of magnetic resonance imaging (MRI) for predicting the response to early revascularization therapy and for identifying patients in whom delayed treatment is appropriate is desirable.
During the past 20 years, there have been significant advances in both neuroimaging as well as in revascularization strategies for treating patients with acute ischemic stroke. In this review, the role of MRI discussed and the recent advances in MRI-based triage for revascularization therapies and in post-processing, including machine learning techniques, in these patients.
Neuroimaging Studies in the Acute Stroke Intervention Field: Results From Randomized Controlled Trials
Previous intravenous rt-PA trials [the NINDS rt-PA (1) and ECASS-III (2) trials] have used non-contrast computed tomography (NCCT) images. Randomized controlled trials (RCTs) of EVT have implemented MRI or computed tomography perfusion/angiography (CTP/CTA) techniques, in addition to the NCCT Alberta Stroke Program Early CT Score (ASPECTS). However, the results of RCTs and the rapid evolution of neuroimaging techniques have led to significant changes in the international guidelines for neuroimaging in acute ischemic stroke over time (Figure 1).
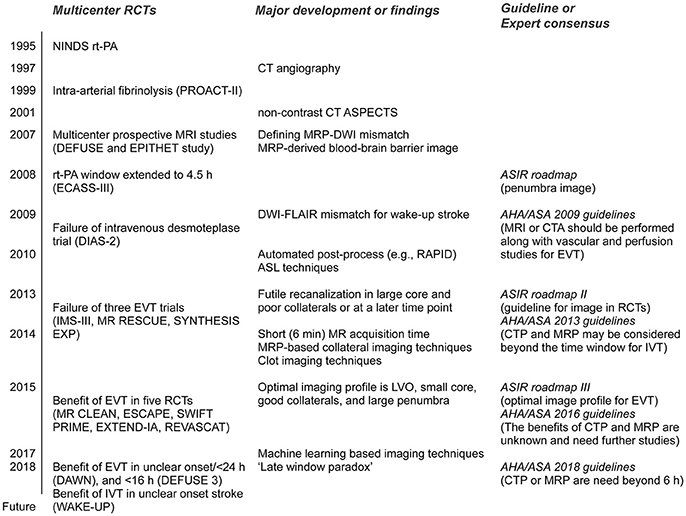
Figure 1. Major randomized controlled trials (RCTs) and corresponding guidelines in the field of acute stroke therapy. rt-PA, recombinant tissue plasminogen activator; ASPECTS, Alberta Stroke Program Early CT Score; MRP, magnetic resonance perfusion; DWI, diffusion-weighted image; FLAIR, fluid attenuation inversion recovery; ASIR, Acute Stroke Imaging Research; AHA/ASA, American Heart Association/American Stroke Association; DIAS-2, Desmoteplase in acute ischemic stroke-2; RAPID, RApid postprocess for PerfusIon and Diffusion; ASL, arterial spin labeling; LVO, large vessel occlusion; EVT, endovascular therapy. Please see Glossary for other abbreviations.
Multicenter prospective MRI studies, including the DEFUSE and EPITHET trials for intravenous rt-PA given more than 3 h post-stroke, reported a significant association between recanalization and reduced infarct growth in patients with an MR perfusion–diffusion mismatch (3, 4). Expert consensus on the Acute Stroke Imaging Research (ASIR) Roadmap reported on the methodological issues in perfusion and penumbral imaging (5). The 2009 American Heart Association/American Stroke Association (AHA/ASA) guidelines recommended that MRI or CTA should be performed in conjunction with vascular and perfusion studies for EVT (6).
The IMS III (7), MR RESCUE (8), and SYNTHESIS expansion (9) trials were multicenter, prospective RCTs that failed to show a benefit from EVT for acute ischemic stroke. The potential reasons for this failure include time delays to angiographic reperfusion and the inclusion of patients with large core or no large vessel occlusion. In addition, the MR RESCUE trial used an algorithm that implements multiple diffusion-weighted imaging (DWI) and MR perfusion (MRP) parameters, but failed to show that patients with a favorable penumbral pattern on neuroimaging benefitted from EVT (8). Given the lessons from these aforementioned three RCTs, published 2013, the ASIR Roadmap II provided guidelines for the use of imaging in stroke clinical trials (10). In the AHA/ASA 2013 guidelines, the recommendations were changed to “CTP and MRP may be considered” (11).
Further phase III RCTs were conducted in 2015; these included the MR CLEAN (12), ESCAPE (13), EXTEND-IA (14), SWIFT PRIME (15) and REVASCAT (16) trials. The findings of these RCTs demonstrated overwhelming evidence of the benefit of EVT for treatment of acute ischemic stroke with a small core (as measured by the ASPECTS) and large vessel occlusion. The ASIR Roadmap III proposed the optimal imaging profile for EVT, based on the results of these recent positive RCTs: the presence of large vessel occlusion, a smaller core, good collaterals, and a large penumbra (17). After the success of the ASPECTS-based RCTs of EVT in 2015, the recommendations were again changed to “the benefits of CTP and MRP are unknown and need further studies” (18). For patients eligible for EVT, the 2016 AHA/ASA guidelines require the absence of bleeding and an ASPECTS of 6 points or more in NCCT, as well as the presence of causative occlusion of the internal carotid artery or proximal middle cerebral artery (18).
Very recently, the results of the phase III RCTs of EVT in an extended time-window showed a significant and remarkable functional recovery with EVT vs. that with medical treatment in carefully selected patients (19, 20). EVT was initiated between 6 and 16 h after onset in patients with a target mismatch in the DEFUSE 3 trial (20), and 6–24 h after onset in patients with mismatch between clinical presentation and DWI/CTP in the DAWN trial (19). In these trials, the benefits of EVT persisted [or even increased, i.e., “late-window paradox” (21)] across the period when patients had a small core and large salvageable tissues. Based on these trials, the new 2018 guidelines recommended that CTP or DWI/MRP scans be obtained if the patient presents more than 6 h after his/her last known normal status and has large vessel occlusion (LVO), and to perform EVT when eligibility criteria from these trials were met (22).
Implications of MRI-Based Triage on the Number of Patients Receiving EVT
The beneficial effect of EVT has been confirmed in selected patients with acute ischemic stroke. However, a substantial proportion of patients are EVT ineligible (only 7–13% of acute ischemic stroke patients are eligible for EVT) (23), have failure of reperfusion (TICI 0–2a in 14–41% in five recent phase III RCTs) (12–16), or have futile reperfusion (26–49% showed a poor outcome, despite successful recanalization) (24). These findings indicate that imaging-guided tailored treatment may be beneficial in acute ischemic stroke. Although there have been significant advances in CT techniques, the advantages of MRI techniques make MRI more desirable for use (25, 26). The advantages and limitations of CT-based triage for EVT as well as the recent advances in MRI techniques are summarized in Table 1.
In EVT-eligible patients, MRI-based triage may increase the efficacy of EVT, at the expense of decreasing the number of patients receiving EVT by excluding patients with large lesions on DWI (27) (DWI is superior to any CT techniques in imaging the infarct core) (25). In contrast, MRI-based triage can also increase EVT use in patients considered ineligible under the current guidelines, as follows.
First, wake-up stroke occurs in one-fifth of patients with stroke; it was estimated that 58,000 patients with wake-up strokes presented to an emergency department in the U.S. in 2005 (3 million wake-up stroke cases worldwide) (28). In these cases, less time might have elapsed from the onset of stroke because circadian variation for stroke is well-known, with most cerebrovascular events known to occur during the morning (29). When also considering cases with an unknown time of symptom onset, for example, non-witnessed stroke with aphasia or disturbance of consciousness, it appears that the time of symptom onset is unknown in 14–35% of patients with acute stroke (28, 30–32). It is possible that some of these patients could benefit from revascularization therapies. A population-based study has shown that more than one-third of wake-up strokes would have been eligible for thrombolysis if arrival time were not a factor (28). MRI features combining fluid attenuation inversion recovery (FLAIR) sequences with DWI have been investigated as a surrogate marker for lesion age and a DWI-positive/FLAIR-negative mismatch pattern was identified in patients within 4.5 h of stroke onset in the middle cerebral artery territory, with high predictive values (33, 34). A recent RCT (WAKE-UP) showed that in patients with acute ischemic stroke with an unknown time of onset, intravenous rt-PA guided by a mismatch between DWI and FLAIR in the region of ischemia resulted in a significantly better functional outcome than the control group (35).
Second, patients could receive EVT if they have ASPECTS of ≥6 on NCCT and LVO on CTA, and if treatment can be initiated within 6 h of symptom onset (18). However, a significant proportion of patients arrived late at a comprehensive stroke center, where EVT can be performed, and some patients may require a longer procedural time. Data from US academic medical centers have shown that one-third of patients arrived more than 6 h after symptom onset (36). The ESCAPE trial showed that EVT improved functional outcomes and reduced mortality in patients with a small infarct core and moderate-to-good collateral circulation, up to 12 h after symptom onset (13). The HERMES investigators performed a meta-analysis of individual patient data from five recent RCTs of EVT, to test whether EVT is efficacious across a diverse population (i.e., a lower ASPECTS or longer onset-to-groin puncture time, etc.) (37). Patients with small cores (high ASPECTS) had a slower decline in benefit with longer symptom onset-to-reperfusion, than patients with larger infarct cores (38). In addition, reperfusion is related to a positive clinical outcome only if adequate collateralization can prevent infarction until the vessel can be recanalized. A good collateral status could thus feasibly extend the time-window for EVT (39–41). Patients with good collaterals as assessed by MRI showed a favorable outcome in terms of infarct growth at day 7 and modified Rankin score at day 90 (42, 43). Therefore, inclusion of patients with good collaterals, but not in those with larger cores, the time-window for EVT may be extended. Indeed, the results of the DAWN and DEFUSE 3 trials have extended the time-window in these patients to 16–24 h (19, 20).
Lastly, EVT is not recommended in patients with ASPECTS of <6 points on NCCT, according to the current guidelines (18). Data from the ECASS II study, in which 800 patients were randomized to rt-PA or placebo within 6 h of symptom onset, showed that the median ASPECTS value was 9, and that about one in seven patients showed ASPECTS of <6 on NCCT (44). Interestingly, the effect of rt-PA on functional outcome was not influenced by baseline ASPECTS, although patients with low ASPECTS have a substantially increased risk of thrombolysis-related parenchymal hemorrhage (44). In the DEFUSE 3 trial, there was no difference in the effect of EVT according to the ASPECTS score (<8 vs. ≥8) (20), which suggests that advanced image-guided selection could be considered in patients who have a low ASPECTS.
Advances in MRI Techniques for Image Processing and Individual Stroke Pathophysiology
There have been significant advances in MRI techniques in terms of availability, acquisition (scanning and post-processing) time, direct visualization of cardinal features (the 4 Cs; i.e., [tissue]-clock, clot, collaterals, and core), and machine learning-based algorithm implementation.
Availability
An NCCT scan is usually one of the first tests done in the evaluation of acute stroke. MRI takes longer and is often not available under emergency conditions, while NCCT has advantages in terms of fast acquisition time, widespread availability, and ease of interpretation in an emergency setting. However, MRI is available in all comprehensive stroke centers, where EVT can be performed. One single-center study showed that MRI-based triage for EVT is feasible in terms of the scan-to-groin puncture time, with acceptable rates of poor outcome and symptomatic hemorrhage (45). A recent randomized trial (General or Local Anesthesia in Intra Arterial Therapy, GOLIATH) showed that MRI selection for endovascular therapy can be accomplished rapidly and within a similar time frame as computed tomography-based selection (46). The door-to-MRI time can be reduced by a quality improvement process (47). Moreover, although a comprehensive MRI protocol can be implemented in ~20 min, a fast MRI protocol can be implemented in about 6 min, rivaling the time of any comprehensive acute stroke CT protocol (48). This fast MRI protocol includes DWI, FLAIR, gradient echo, and MR angiography, and MRP. The CTP image requires additional imaging time (2–3 min) and post-processing time (5–15 min) (49).
For clinical use, automated software that allows fast post-processing is mandatory, and is increasingly being used in clinical trials. For example, the RApid postprocess for PerfusIon and Diffusion (RAPID; Rapid Software Corporation, Grapevine, TX, USA), an automated software package for performing quantitative evaluation of the apparent diffusion coefficient to estimate the ischemic core and an MRP threshold of Tmax > 6 s for defining critical hypoperfusion (50), has been used in several RCTs. Similarly, automated software for collateral assessment, the Fast Analysis SysTem for COLLaterals (FAST-COLL) (42), has been developed; it requires <5 min, and allows a clinical decision to be made at the workstation or bedside, based on the collateral grade.
Visualization of Individual Stroke Pathophysiology
Aside from demonstrating a perfusion-diffusion mismatch and delineating penumbral and irreversibly infarcted regions, MRI could provide additional information on the blood–brain barrier (BBB), collaterals, and clot. MRI techniques for determining the age of the infarct was mentioned above. Representative cases are presented in Figures 2, 3.
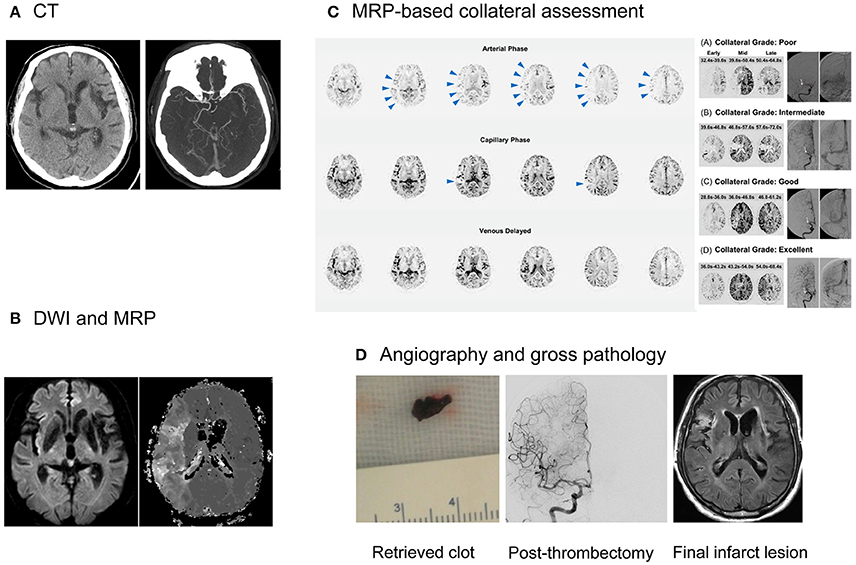
Figure 2. Collateral imaging-based delayed endovascular treatment. A 79-year-old female, with history of left MCA infarction and atrial fibrillation, presented with left-sided weakness and neglect symptoms at 940 min after symptom onset. (A) CT imaging documented right MCA occlusion, with an ASPECTS of 8. (B) MRI showed a significant mismatch, with a small core and large hypoperfusion regions. (C) MRP-based collateral imaging showed an excellent collateral flow, i.e., complete and rapid collateral flow to the vascular bed in the occluded MCA territory. Contrast staining in the lesional hemisphere was absent in the arterial phase (arrowheads), but evident in the capillary and venous phase. (D) Successful recanalization (mTICI grade 2b) was achieved and red clots were retrieved at the first passage of a stentriever at 1,090 min after the onset of symptoms. She was functionally independent and her modified Rankin score at the 90th day was 1. MCA, middle cerebral artery; CT, computed tomography; ASPECT, Alberta stroke program early CT Score; MRI, magnetic resonance imaging; DWI, diffusion-weighted imaging; MRP, MR perfusion; mTICI, modified treatment in cerebral ischemia.
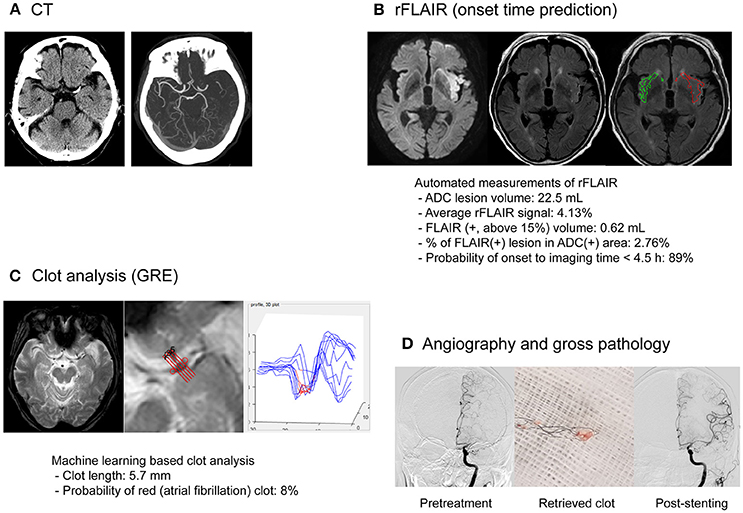
Figure 3. MRI-based endovascular treatment of a stroke patient with unclear symptom onset time and atherosclerotic occlusion of the middle cerebral artery. An 81-year-old male presented with right-sided weakness and global aphasia, with an unclear onset time. (A) On the CT image, left MCA occlusion was documented with an ASPECTS of 8. The patient underwent multimodal MRI using a fast (9 min) MRI protocol. (B) Minimal signal change was observed on FLAIR imaging and the probability of stroke onset within 4.5 h, based on relative FLAIR signal analysis, was high. (C) Clot analysis using GRE imaging and machine-learning techniques showed a low possibility of atrial fibrillation as cause of the clot. (D) Whitish clots were retrieved during endovascular treatment. Successful recanalization (mTICI grade 2b) was achieved with rescue stenting after repetitive reocclusion with a stentriever. His modified Rankin score at the 90th day was 1. CT, computed tomography; MCA, middle cerebral artery, ASPECTS, Alberta Stroke Program Early CT Score; ADC, apparent diffusion coefficient; FLAIR, fluid attenuation inversion recovery; GRE, gradient echo; mTICI, modified treatment in cerebral ischemia.
Collateral Flow
Better collaterals are associated with improved clinical and radiological outcomes, while poor collaterals are linked to hemorrhagic complications and poor recanalization rates after revascularization therapy for acute ischemic stroke (51). A collateral flow map derived from MRP source data can be generated by automatic post-processing (42). This study showed good correlation between MRI-based collateral grade and conventional angiography-based collateral grade, indicating that pretreatment MRI-based collateral evaluation could replace conventional angiographic evaluation in the angio-suite, which may require >20 min before EVT can be initiated. The role of MRI-based collateral imaging (FAST-COLL) is currently being tested in a prospective observational study (Clinicaltrials.identifier NCT02668627), to evaluate whether MRI-based collateral imaging is feasible and can predict the response to EVT in a wide range of patients with acute ischemic stroke. This study is evaluating the early infarct grow rate and eligibility according to the DAWN and DEFUSE 3 criteria, depending on the pretreatment MRI-based collateral grades. It is interesting that a significant proportion of patients who are eligible according to the DEFUSE 3 criteria did not meet the DAWN criteria for eligibility (20, 21).
Clot Treatment
Successful reperfusion may be associated with the histopathology of occlusive thrombi, including the existence of atheromatous gruel and the proportion of erythrocyte components (52). Intracranial atherosclerosis is particularly prevalent in Asians, and is associated with frequent EVT failure. In this condition, adjuvant therapy, such as the use of a GP IIb/IIIa inhibitor or permanent stent placement, may be needed (53). Although previous studies have attempted to predict the response to revascularization therapy using CT imaging of the clot, a recent study showed a lack of association between CT-based clot images and the histopathology of thrombi, and stroke etiology (54). MRI can identify clots with high specificity and can measure the clot burden more accurately than CT images. Blooming artifacts caused by paramagnetic materials in GRE or susceptible weighted images have been associated with cardioembolic stroke (55, 56).
BBB Derangement
GRE images are as accurate as CT at detecting acute hemorrhage in patients with acute stroke. BBB permeability dysfunction often precedes hemorrhagic transformation (57). Gadolinium contrast agents are routinely used to detect BBB disruptions in patients with strokes or tumors. Using a simple post-processing algorithm that employs pretreatment MRP source data, MRI permeability images can visualize BBB dysfunction and identify patients at risk of hemorrhagic transformation, with high specificity (57). A multicenter study tested various MRP-derived permeability measures in acute stroke patients and showed that MRI permeability images may be used in clinical practice (58). The multicenter DEFUSE 2 and MR RESCUE trials showed that the amount of BBB disruption seen on pretreatment MRI is associated with the severity of intracranial bleeding after EVT (59, 60).
Machine Learning
Machine learning is an approach used to achieve artificial intelligence goals. The field of artificial intelligence has evolved significantly with the introduction of a number of sophisticated algorithms, some of which are capable of self-learning. Application of artificial intelligence in the stroke field is increasing, and is used in the prediction of stroke [e.g., risk factors (61) and fine particulate matter (PM2.5) (62)] and pervasive health monitoring, by using smart monitoring devices embedded in the living environment (e.g., real-time monitoring via smartphone for adherence to oral anticoagulant treatment) (63). It can be particularly helpful in decision-making in every step of EVT for acute ischemic stroke: in clinical and imaging recognition of acute ischemic stroke in the ambulance or emergency room (64), and in predicting the outcome after EVT (65).
Machine learning-based assessment has advantages over simple visual estimation as follows. First, the application of deep learning to create an algorithm for automated detection of abnormal neuroimaging findings can improve inter-rater correlation. For example, the NCCT ASPECTS is widely used worldwide and there have been efforts to increase the inter-rater reliability, including implementation of a teaching program. However, inter-rater reliability has been reported to be low, particularly in a hyperacute setting. One recent systematic review showed that, in patients considered for EVT, there may be insufficient agreement between clinicians for the ASPECTS to be used reliably as a criterion for treatment decisions (66). Although the agreement could be higher among stroke experts than stroke trainees, it may still be lower than that achieved with a machine learning-based decision algorithm developed using various neuroimaging data. A computer algorithm that would automatically read a brain CT scan and automatically generate an ASPECTS (e-ASPECTS) may improve reliability in terms of calculating the score. A multicenter trial has shown that e-ASPECTS was non-inferior to neuroradiologists in determining the ASPECTS score using NCCT images obtained from acute stroke patients (67).
Second, machine-learning techniques facilitate the merging of information from various MR sequences. Integration of information from several MRI sequences could improve the role of MRI-based triage for EVT. For example, although combining DWI and FLAIR data showed high predictive values for identifying patients within 4.5 h of symptom onset, adding information on collaterals or perfusion improved the accuracy of predicting the time from symptom onset within 4.5 h (68, 69). Combining data from quantitative image analyses with other types of data (e.g., clinical or laboratory) can provide models that are helpful in the choice of work up, prediction of outcome or response to treatment.
Finally, because deep learning uses numerous imaging features that are most predictive for certain types of stroke pathophysiology, rather than explicitly detecting clinical features with which stroke physicians are familiar (e.g., conventional DWI), both physicians and patients have to trust a “black box” to determine a disease state. With increased numbers of MR sequences, many variables may influence how a machine defines referable stroke pathophysiologies. These include heterogeneous populations comprising different races (with different stroke subtypes, along with normal variations), heterogeneous prestroke conditions (e.g., preexisting atherosclerotic changes, white matter changes and other age-related changes) and novel features of specific sequences. Because the software does not detect the implication of the algorithm generated by the machine learning process, the stroke neurologist and radiologist need to provide the background and interpretation.
Conclusions and Perspectives
CT has been the standard neuroimaging modality for determining whether EVT should be applied due to the limited availability and standardization and time required for acquisition or post-processing of MRI data. However, more information may be available within the permitted time-windows if rapid acquisition of MRI data, automated fast post-processing and machine learning-based decision algorithms can be implemented. The role of imaging in EVT may shift from “go/no go” to “how to go.” With the advances in transformative technologies (such as machine learning and artificial intelligence) along with a better understanding of stroke pathophysiology and MRI physics, it is highly likely that multimodal MRI information could guide treatment strategies for patients with acute ischemic stroke.
As technology becomes more complex, selection of the technology used becomes more important. Stroke physicians will need to understand the evolution of such technology. Appropriate RCTs are required to verify the usefulness of imaging-based algorithms for the selection of EVT before these techniques can be incorporated into routine clinical practice.
Author Contributions
OB, the corresponding author, established the study idea, wrote the manuscript, and made critical revisions to the manuscript with substantive intellectual content. J-WC, JS, and Y-CK established the study idea, analyzed the data, and wrote the manuscript. W-SR, D-EK, W-KS, and G-MK established the database and made critical revisions to the manuscript with substantive intellectual content.
Conflict of Interest Statement
The authors declare that the research was conducted in the absence of any commercial or financial relationships that could be construed as a potential conflict of interest.
Acknowledgments
This research was supported by grants from the Global Research Lab (GRL) program (NRF-2015K1A1A2028228) and the Bio-SPC program (NRF-2018M3A9G1024808) of the National Research Foundation, funded by the Korean government, Republic of Korea.
References
1. National Institute of Neurological Disorders and Stroke rt-PA Stroke Study Group. Tissue plasminogen activator for acute ischemic stroke. N Engl J Med. (1995) 333:1581–7. doi: 10.1056/NEJM199512143332401
2. Hacke W, Kaste M, Bluhmki E, Brozman M, Davalos A, Guidetti D, et al. Thrombolysis with alteplase 3 to 4.5 hours after acute ischemic stroke. N Engl J Med. (2008) 359:1317–29. doi: 10.1056/NEJMoa0804656
3. Albers GW, Thijs VN, Wechsler L, Kemp S, Schlaug G, Skalabrin E, et al. Magnetic resonance imaging profiles predict clinical response to early reperfusion: the diffusion and perfusion imaging evaluation for understanding stroke evolution (DEFUSE) study. Ann Neurol. (2006) 60:508–17. doi: 10.1002/ana.20976
4. Davis SM, Donnan GA, Parsons MW, Levi C, Butcher KS, Peeters A, et al. Effects of alteplase beyond 3 h after stroke in the echoplanar imaging thrombolytic evaluation trial (EPITHET): a placebo-controlled randomised trial. Lancet Neurol. (2008) 7:299–309. doi: 10.1016/S1474-4422(08)70044-9
5. Wintermark M, Albers GW, Alexandrov AV, Alger JR, Bammer R, Baron JC, et al. Acute stroke imaging research roadmap. Stroke (2008) 39:1621–8. doi: 10.1161/STROKEAHA.107.512319
6. Latchaw RE, Alberts MJ, Lev MH, Connors JJ, Harbaugh RE, Higashida RT, et al. Recommendations for imaging of acute ischemic stroke: a scientific statement from the American Heart Association. Stroke (2009) 40:3646–78. doi: 10.1161/STROKEAHA.108.192616
7. Broderick JP, Palesch YY, Demchuk AM, Yeatts SD, Khatri P, Hill MD, et al. Endovascular therapy after intravenous t-PA versus t-PA alone for stroke. N Engl J Med. (2013) 368:893–903. doi: 10.1056/NEJMoa1214300
8. Kidwell CS, Jahan R, Gornbein J, Alger JR, Nenov V, Ajani Z, et al. A trial of imaging selection and endovascular treatment for ischemic stroke. N Engl J Med. (2013) 368:914–23. doi: 10.1056/NEJMoa1212793
9. Ciccone A, Valvassori L, Nichelatti M, Sgoifo A, Ponzio M, Sterzi R, et al. Endovascular treatment for acute ischemic stroke. N Engl J Med. (2013) 368:904–13. doi: 10.1056/NEJMoa1213701
10. Wintermark M, Albers GW, Broderick JP, Demchuk AM, Fiebach JB, Fiehler J, et al. Acute stroke imaging research roadmap II. Stroke (2013) 44:2628–39. doi: 10.1161/STROKEAHA.113.002015
11. Jauch EC, Saver JL, Adams HP Jr., Bruno A, Connors JJ, Demaerschalk BM, et al. Guidelines for the early management of patients with acute ischemic stroke: a guideline for healthcare professionals from the American Heart Association/American Stroke Association. Stroke (2013) 44:870–947. doi: 10.1161/STR.0b013e318284056a
12. Berkhemer OA, Fransen PS, Beumer D, van den Berg LA, Lingsma HF, Yoo AJ, et al. A randomized trial of intraarterial treatment for acute ischemic stroke. N Engl J Med. (2015) 372:11–20. doi: 10.1056/NEJMoa1411587
13. Goyal M, Demchuk AM, Menon BK, Eesa M, Rempel JL, Thornton J, et al. Randomized assessment of rapid endovascular treatment of ischemic stroke. N Engl J Med. (2015) 372:1019–30. doi: 10.1056/NEJMoa1414905
14. Campbell BC, Mitchell PJ, Kleinig TJ, Dewey HM, Churilov L, Yassi N, et al. Endovascular therapy for ischemic stroke with perfusion-imaging selection. N Engl J Med. (2015) 372:1009–18. doi: 10.1056/NEJMoa1414792
15. Saver JL, Goyal M, Bonafe A, Diener HC, Levy EI, Pereira VM, et al. Stent-retriever thrombectomy after intravenous t-PA vs. t-PA alone in stroke. N Engl J Med. (2015) 372:2285–95. doi: 10.1056/NEJMoa1415061
16. Jovin TG, Chamorro A, Cobo E, de Miquel MA, Molina CA, Rovira A, et al. Thrombectomy within 8 hours after symptom onset in ischemic stroke. N Engl J Med. (2015) 372:2296–306. doi: 10.1056/NEJMoa1503780.
17. Warach SJ, Luby M, Albers GW, Bammer R, Bivard A, Campbell BC, et al. Acute stroke imaging research roadmap III Imaging selection and outcomes in acute stroke reperfusion clinical trials: consensus recommendations and further research priorities. Stroke (2016) 47:1389–98. doi: 10.1161/STROKEAHA.115.012364
18. Powers WJ, Derdeyn CP, Biller J, Coffey CS, Hoh BL, Jauch EC, et al. American Heart Association/American Stroke Association focused update of the 2013 guidelines for the early management of patients with acute ischemic stroke regarding endovascular treatment: a guideline for healthcare professionals from the American Heart Association/American Stroke Association. Stroke (2015) 46:3020–35. doi: 10.1161/STR.0000000000000074
19. Nogueira RG, Jadhav AP, Haussen DC, Bonafe A, Budzik RF, Bhuva P, et al. Thrombectomy 6 to 24 hours after stroke with a mismatch between deficit and infarct. N Engl J Med. (2018) 378:11–21. doi: 10.1056/NEJMoa1706442
20. Albers GW, Marks MP, Kemp S, Christensen S, Tsai JP, Ortega-Gutierrez S, et al. Thrombectomy for stroke at 6 to 16 hours with selection by perfusion imaging. N Engl J Med. (2018) 378:708–18. doi: 10.1056/NEJMoa1713973
22. Powers WJ, Rabinstein AA, Ackerson T, Adeoye OM, Bambakidis NC, Becker K, et al. 2018 guidelines for the early management of patients with acute ischemic stroke: a guideline for healthcare professionals from the American Heart Association/American Stroke Association. Stroke (2018) 49:e46–110. doi: 10.1161/STR.0000000000000158
23. Chia NH, Leyden JM, Newbury J, Jannes J, Kleinig TJ. Determining the number of ischemic strokes potentially eligible for endovascular thrombectomy: a population-based study. Stroke (2016) 47:1377–80. doi: 10.1161/STROKEAHA.116.013165
24. Molina CA. Futile recanalization in mechanical embolectomy trials: a call to improve selection of patients for revascularization. Stroke (2010) 41:842–3. doi: 10.1161/STROKEAHA.110.580266
25. Kohrmann M, Schellinger PD. Acute stroke triage to intravenous thrombolysis and other therapies with advanced CT or MR imaging: pro MR imaging. Radiology (2009) 251:627–33. doi: 10.1148/radiol.2513081074
26. Leslie-Mazwi TM, Hirsch JA, Falcone GJ, Schaefer PW, Lev MH, Rabinov JD, et al. Endovascular stroke treatment outcomes after patient selection based on magnetic resonance imaging and clinical criteria. JAMA Neurol. (2016) 73:43–9. doi: 10.1001/jamaneurol.2015.3000
27. Wisco D, Uchino K, Saqqur M, Gebel JM, Aoki J, Alam S, et al. Addition of hyperacute MRI AIDS in patient selection, decreasing the use of endovascular stroke therapy. Stroke (2014) 45:467–72. doi: 10.1161/STROKEAHA.113.003880
28. Mackey J, Kleindorfer D, Sucharew H, Moomaw CJ, Kissela BM, Alwell K, et al. Population-based study of wake-up strokes. Neurology (2011) 76:1662–7. doi: 10.1212/WNL.0b013e318219fb30
29. Elliott WJ. Circadian variation in the timing of stroke onset: a meta-analysis. Stroke (1998) 29:992–6.
30. Serena J, Davalos A, Segura T, Mostacero E, Castillo J. Stroke on awakening: looking for a more rational management. Cerebrovasc Dis. (2003) 16:128–33. doi: 10.1159/000070592
31. Moradiya Y, Janjua N. Presentation and outcomes of “wake-up strokes” in a large randomized stroke trial: analysis of data from the International Stroke Trial. J Stroke Cerebrovasc Dis. (2013) 22:e286–92. doi: 10.1016/j.jstrokecerebrovasdis.2012.07.016
32. Reid JM, Dai D, Cheripelli B, Christian C, Reidy Y, Gubitz GJ, et al. Differences in wake-up and unknown onset stroke examined in a stroke registry. Int J Stroke. (2015) 10:331–5. doi: 10.1111/ijs.12388
33. Thomalla G, Rossbach P, Rosenkranz M, Siemonsen S, Krutzelmann A, Fiehler J, et al. Negative fluid-attenuated inversion recovery imaging identifies acute ischemic stroke at 3 hours or less. Ann Neurol. (2009) 65:724–32. doi: 10.1002/ana.21651
34. Thomalla G, Cheng B, Ebinger M, Hao Q, Tourdias T, Wu O, et al. DWI-FLAIR mismatch for the identification of patients with acute ischaemic stroke within 4.5 h of symptom onset (PRE-FLAIR): a multicentre observational study. Lancet Neurol. (2011) 10:978–86. doi: 10.1016/S1474-4422(11)70192-2
35. Thomalla G, Simonsen CZ, Boutitie F, Andersen G, Berthezene Y, Cheng B, et al. MRI-guided thrombolysis for stroke with unknown time of onset. N Engl J Med. (2018). doi: 10.1056/NEJMoa1804355. [Epub ahead of print].
36. Lichtman JH, Watanabe E, Allen NB, Jones SB, Dostal J, Goldstein LB. Hospital arrival time and intravenous t-PA use in US Academic Medical Centers, 2001–2004. Stroke (2009) 40:3845–50. doi: 10.1161/STROKEAHA.109.562660
37. Goyal M, Menon BK, van Zwam WH, Dippel DW, Mitchell PJ, Demchuk AM, et al. Endovascular thrombectomy after large-vessel ischaemic stroke: a meta-analysis of individual patient data from five randomised trials. Lancet (2016) 387:1723–31. doi: 10.1016/S0140-6736(16)00163-X
38. Saver JL, Goyal M, van der Lugt A, Menon BK, Majoie CB, Dippel DW, et al. Time to treatment with endovascular thrombectomy and outcomes from ischemic stroke: a meta-analysis. JAMA (2016) 316:1279–88. doi: 10.1001/jama.2016.13647
39. Galimanis A, Jung S, Mono ML, Fischer U, Findling O, Weck A, et al. Endovascular therapy of 623 patients with anterior circulation stroke. Stroke (2012) 43:1052–7. doi: 10.1161/STROKEAHA.111.639112
40. Ribo M, Flores A, Rubiera M, Pagola J, Sargento-Freitas J, Rodriguez-Luna D, et al. Extending the time window for endovascular procedures according to collateral pial circulation. Stroke (2011) 42:3465–9. doi: 10.1161/STROKEAHA.111.623827
41. Hwang YH, Kang DH, Kim YW, Kim YS, Park SP, Liebeskind DS. Impact of time-to-reperfusion on outcome in patients with poor collaterals. Am J Neuroradiol. (2015) 36:495–500. doi: 10.3174/ajnr.A4151
42. Kim SJ, Son JP, Ryoo S, Lee MJ, Cha J, Kim KH, et al. A novel magnetic resonance imaging approach to collateral flow imaging in ischemic stroke. Ann Neurol. (2014) 76:356–69. doi: 10.1002/ana.24211
43. Son JP, Lee MJ, Kim SJ, Chung JW, Cha J, Kim GM, et al. Impact of slow blood filling via collaterals on infarct growth: comparison of mismatch and collateral status. J Stroke. (2017) 19:88–96. doi: 10.5853/jos.2016.00955
44. Dzialowski I, Hill MD, Coutts SB, Demchuk AM, Kent DM, Wunderlich O, et al. Extent of early ischemic changes on computed tomography (CT) before thrombolysis: prognostic value of the Alberta Stroke Program Early CT Score in ECASS II. Stroke (2006) 37:973–8. doi: 10.1161/01.STR.0000206215.62441.56
45. Simonsen CZ, Sorensen LH, Karabegovic S, Mikkelsen IK, Schmitz ML, Juul N, et al. MRI before intraarterial therapy in ischemic stroke: feasibility, impact, and safety. J Cereb Blood Flow Metab. (2014) 34:1076–81. doi: 10.1038/jcbfm.2014.57
46. Simonsen CZ, Yoo AJ, Rasmussen M, Sorensen KE, Leslie-Mazwi T, Andersen G, et al. Magnetic resonance imaging selection for endovascular stroke therapy: workflow in the GOLIATH trial. Stroke (2018) 49:1402–6. doi: 10.1161/STROKEAHA.118.021038
47. Shah S, Luby M, Poole K, Morella T, Keller E, Benson RT, et al. Screening with MRI for accurate and rapid stroke treatment: SMART. Neurology (2015) 84:2438–44. doi: 10.1212/WNL.0000000000001678
48. Nael K, Khan R, Choudhary G, Meshksar A, Villablanca P, Tay J, et al. Six-minute magnetic resonance imaging protocol for evaluation of acute ischemic stroke: pushing the boundaries. Stroke (2014) 45:1985–91. doi: 10.1161/STROKEAHA.114.005305
49. Lee JS, Demchuk AM. Choosing a hyperacute stroke imaging protocol for proper patient selection and time efficient endovascular treatment: lessons from recent trials. J Stroke. (2015) 17:221–8. doi: 10.5853/jos.2015.17.3.221
50. Straka M, Albers GW, Bammer R. Real-time diffusion-perfusion mismatch analysis in acute stroke. J Magn Reson Imaging. (2010) 32:1024–37. doi: 10.1002/jmri.22338
51. Bang OY, Goyal M, Liebeskind DS. Collateral circulation in ischemic stroke: assessment tools and therapeutic strategies. Stroke (2015) 46:3302–9. doi: 10.1161/STROKEAHA.115.010508
52. Hashimoto T, Hayakawa M, Funatsu N, Yamagami H, Satow T, Takahashi JC, et al. Histopathologic analysis of retrieved thrombi associated with successful reperfusion after acute stroke thrombectomy. Stroke (2016) 47:3035–7. doi: 10.1161/STROKEAHA.116.015228
53. Baek JH, Kim BM, Kim DJ, Heo JH, Nam HS, Song D, et al. Importance of truncal-type occlusion in stentriever-based thrombectomy for acute stroke. Neurology (2016) 87:1542–50. doi: 10.1212/WNL.0000000000003202
54. Brinjikji W, Duffy S, Burrows A, Hacke W, Liebeskind D, Majoie CB, et al. Correlation of imaging and histopathology of thrombi in acute ischemic stroke with etiology and outcome: a systematic review. J Neurointerv Surg. (2016) 9:529–34. doi: 10.1136/neurintsurg-2016-012391
55. Kang DW, Jeong HG, Kim DY, Yang W, Lee SH. Prediction of stroke subtype and recanalization using susceptibility vessel sign on susceptibility-weighted magnetic resonance imaging. Stroke (2017) 48:1554–9. doi: 10.1161/STROKEAHA.116.016217
56. Zhang R, Zhou Y, Liu C, Zhang M, Yan S, Liebeskind DS, et al. Overestimation of susceptibility vessel sign: a predictive marker of stroke cause. Stroke (2017) 48:1993–6. doi: 10.1161/STROKEAHA.117.016727
57. Bang OY, Buck BH, Saver JL, Alger JR, Yoon SR, Starkman S, et al. Prediction of hemorrhagic transformation after recanalization therapy using T2*-permeability magnetic resonance imaging. Ann Neurol. (2007) 62:170–6. doi: 10.1002/ana.21174
58. Scalzo F, Alger JR, Hu X, Saver JL, Dani KA, Muir KW, et al. Multi-center prediction of hemorrhagic transformation in acute ischemic stroke using permeability imaging features. Magn Reson Imaging (2013) 31:961–9. doi: 10.1016/j.mri.2013.03.013
59. Leigh R, Christensen S, Campbell BC, Marks MP, Albers GW, Lansberg MG, et al. Pretreatment blood-brain barrier disruption and post-endovascular intracranial hemorrhage. Neurology (2016) 87:263–9. doi: 10.1212/WNL.0000000000002862
60. Nael K, Knitter JR, Jahan R, Gornbein J, Ajani Z, Feng L, et al. Multiparametric magnetic resonance imaging for prediction of parenchymal hemorrhage in acute ischemic stroke after reperfusion therapy. Stroke (2017) 48:664–70. doi: 10.1161/STROKEAHA.116.014343
61. Weng SF, Reps J, Kai J, Garibaldi JM, Qureshi N. Can machine-learning improve cardiovascular risk prediction using routine clinical data? PLoS ONE (2017) 12:e0174944. doi: 10.1371/journal.pone.0174944
62. Ong BT, Sugiura K, Zettsu K. Dynamically pre-trained deep recurrent neural networks using environmental monitoring data for predicting PM2.5. Neural Comput Appl. (2016) 27:1553–66. doi: 10.1007/s00521-015-1955-3
63. Labovitz DL, Shafner L, Reyes Gil M, Virmani D, Hanina A. Using artificial intelligence to reduce the risk of nonadherence in patients on anticoagulation therapy. Stroke (2017) 48:1416–9. doi: 10.1161/STROKEAHA.116.016281
64. Abedi V, Goyal N, Tsivgoulis G, Hosseinichimeh N, Hontecillas R, Bassaganya-Riera J, et al. Novel screening tool for stroke using artificial neural network. Stroke (2017) 48:1678–81. doi: 10.1161/STROKEAHA.117.017033
65. Asadi H, Dowling R, Yan B, Mitchell P. Machine learning for outcome prediction of acute ischemic stroke post intra-arterial therapy. PLoS ONE (2014) 9:e88225. doi: 10.1371/journal.pone.0088225
66. Farzin B, Fahed R, Guilbert F, Poppe AY, Daneault N, Durocher AP, et al. Early CT changes in patients admitted for thrombectomy: intrarater and interrater agreement. Neurology (2016) 87:249–56. doi: 10.1212/WNL.0000000000002860
67. Nagel S, Sinha D, Day D, Reith W, Chapot R, Papanagiotou P, et al. e-ASPECTS software is non-inferior to neuroradiologists in applying the ASPECT score to computed tomography scans of acute ischemic stroke patients. Int J Stroke (2017) 12:615–22. doi: 10.1177/1747493016681020
68. Wouters A, Dupont P, Christensen S, Norrving B, Laage R, Thomalla G, et al. Association between time from stroke onset and fluid-attenuated inversion recovery lesion intensity is modified by status of collateral circulation. Stroke (2016) 47:1018–22. doi: 10.1161/STROKEAHA.115.012010
69. Wouters A, Dupont P, Norrving B, Laage R, Thomalla G, Albers GW, et al. Prediction of stroke onset is improved by relative fluid-attenuated inversion recovery and perfusion imaging compared to the visual diffusion-weighted imaging/fluid-attenuated inversion recovery mismatch. Stroke (2016) 47:2559–64. doi: 10.1161/STROKEAHA.116.013903
Keywords: stroke, MRI, endovascular treatment, machine learning, triage
Citation: Bang OY, Chung J-W, Son JP, Ryu W-S, Kim D-E, Seo W-K, Kim G-M and Kim Y-C (2018) Multimodal MRI-Based Triage for Acute Stroke Therapy: Challenges and Progress. Front. Neurol. 9:586. doi: 10.3389/fneur.2018.00586
Received: 15 May 2018; Accepted: 29 June 2018;
Published: 24 July 2018.
Edited by:
David S. Liebeskind, University of California, Los Angeles, United StatesReviewed by:
Claus Ziegler Simonsen, Aarhus University Hospital, DenmarkMaurizio Acampa, Azienda Ospedaliera Universitaria Senese, Italy
Copyright © 2018 Bang, Chung, Son, Ryu, Kim, Seo, Kim and Kim. This is an open-access article distributed under the terms of the Creative Commons Attribution License (CC BY). The use, distribution or reproduction in other forums is permitted, provided the original author(s) and the copyright owner(s) are credited and that the original publication in this journal is cited, in accordance with accepted academic practice. No use, distribution or reproduction is permitted which does not comply with these terms.
*Correspondence: Oh Young Bang, b2h5b3VuZy5iYW5nQHNhbXN1bmcuY29t