- 1Department of Radiology, Brigham and Women's Hospital, Harvard Medical School, Boston, MA, United States
- 2Center for Clinical Spectroscopy, Brigham and Women's Hospital, Harvard Medical School, Boston, MA, United States
- 3Psychiatry Neuroimaging Laboratory, Brigham and Women's Hospital, Harvard Medical School, Boston, MA, United States
- 4Department of Neuroradiology, Klinikum rechts der Isar, Technische Universität München, Munich, Germany
- 5TUM-Neuroimaging Center, Klinikum rechts der Isar, Technische Universität München, Munich, Germany
- 6Department of Neurosurgery, Klinikum rechts der Isar, Technische Universität München, Munich, Germany
- 7Department of Psychiatry, Massachusetts General Hospital, Harvard Medical School, Boston, MA, United States
- 8Boston University Alzheimer's Disease and CTE Center, Boston University School of Medicine, Boston, MA, United States
- 9Department of Neurology, Boston University School of Medicine, Boston, MA, United States
- 10Department of Child and Adolescent Psychiatry, Psychosomatic and Psychotherapy, Ludwig-Maximilians-Universität, Munich, Germany
- 11Department of Neurology, Ludwig-Maximilians-Universität, Munich, Germany
- 123M Centre, The University of Western Ontario, London, ON, Canada
- 13School of Health Studies, The University of Western Ontario, London, ON, Canada
- 14Ruth Lilly Medical Library, Indiana University, Indianapolis, IN, United States
- 15VA Boston Healthcare System, Brockton, MA, United States
- 16Elliott Sports Medicine Clinic, Burlington, ON, Canada
Background: Previous research has shown evidence for transient neuronal loss after repetitive head impacts (RHI) as demonstrated by a decrease in N-acetylaspartate (NAA). However, few studies have investigated other neuro-metabolites that may be altered in the presence of RHI; furthermore, the relationship of neuro-metabolite changes to neurocognitive outcome and potential sex differences remain largely unknown.
Objective: The aim of this study was to identify alterations in brain metabolites and their potential association with neurocognitive performance over time as well as to characterize sex-specific differences in response to RHI.
Methods: 33 collegiate ice hockey players (17 males and 16 females) underwent 3T magnetic resonance spectroscopy (MRS) and neurocognitive evaluation before and after the Canadian Interuniversity Sports (CIS) ice hockey season 2011–2012. The MRS voxel was placed in the corpus callosum. Pre- and postseason neurocognitive performances were assessed using the Immediate Post-Concussion Assessment and Cognitive Test (ImPACT). Absolute neuro-metabolite concentrations were then compared between pre- and postseason MRS were (level of statistical significance after correction for multiple comparisons: p < 0.007) and correlated to ImPACT scores for both sexes.
Results: A significant decrease in NAA was observed from preseason to postseason (p = 0.001). Furthermore, a trend toward a decrease in total choline (Cho) was observed (p = 0.044). Although no overall effect was observed for glutamate (Glu) over the season, a difference was observed with females showing a decrease in Glu and males showing an increase in Glu, though this was not statistically significant (p = 0.039). In both males and females, a negative correlation was observed between changes in Glu and changes in verbal memory (p = 0.008).
Conclusion: The results of this study demonstrate changes in absolute concentrations of neuro-metabolites following exposure to RHI. Results suggest that changes in Glu are correlated with changes in verbal memory. Future studies need to investigate further the association between brain metabolites and clinical outcome as well as sex-specific differences in the brain's response to RHI.
Introduction
Concussions and head injuries in general are a frequent occurrence in contact sports, representing the most common injury in women's ice hockey and the second most common injury in men's ice hockey (1, 2). Further, ice hockey is a contact sport that predisposes players to concussive and subconcussive repetitive head impacts (RHI) based on the inherent nature of the sport. Though females make up nearly half of all collegiate student-athletes, females are an understudied population as few studies have included female athletes or focused on the differences in outcomes between males and females (3). Previous research nonetheless suggests that females generally have worse outcomes following concussion compared to males. More specifically, females demonstrate worse performance on neurocognitive evaluation, increased symptom severity, and have a longer recovery period compared to males (4, 5). However, the impact exposures in ice hockey in females are less frequent and of a lower magnitude compared to males (6). It is thus important to elucidate potential brain alterations in both sexes to understand how the brain responds to RHI in both sexes.
Magnetic resonance spectroscopy (MRS) has been repeatedly used to detect and to characterize changes in neuro-metabolites due to different degrees of brain injury, providing insight into the underlying mechanism of even subtle changes that can occur (7–14). MRS is a non-invasive technique that allows for the detection and quantification of neuro-metabolites in vivo. The primary neuro-metabolites typically assessed are N-acetylaspartate (NAA), a marker of neuronal and axonal integrity, creatine (Cr), often used as an internal reference for comparison to other metabolites, glutamate (Glu), a marker of excitatory neurotransmission, and myoinositol (mI), a marker for gliosis (15–17). Prior research suggests decreased levels of NAA following both acute and subacute phases of concussion (8, 14, 16). Furthermore, prior research suggests an increase in choline (Cho) and reductions in mI following brain injury (14, 16, 18, 19). More recently, changes in gamma-Aminobutyric acid (GABA) and glutathione (GSH) in response to concussion have also been reported (20, 21). In addition, and based on the literature, the corpus callosum is particularly vulnerable to the biomechanical forces suffered during RHI (22). There is therefore a need to determine the alteration of brain metabolites in regions vulnerable to RHI, such as the corpus callosum.
The aim of this study was to investigate the interactions between time and sex in male and female ice hockey players regarding changes in neuro-metabolites in the corpus callosum due to RHI during a season of collegiate ice hockey. This study evaluates the absolute concentrations of NAA, Glu, Cho, Cr, and mI at baseline and postseason. We hypothesized a decrease in NAA and an increase in total Cho (glycerophosphocholine and phosphocholine) from pre- to postseason based on previous research (7, 16, 17). Additionally, we correlated changes in neuro-metabolites due to RHI to neurocognitive performance, and hypothesized sex-specific differences in the aforementioned neuro-metabolites and correlations over time. To our knowledge, there is one study using MRS to detect sex-specific alterations in absolute neuro-metabolite concentrations due to RHI (23).
Materials and Methods
Participants and Study Methods
All study participants were part of the Hockey Concussion Education Project (HCEP), which was conducted during the U Sports (formerly Canadian Interuniversity Sports (CIS)) ice hockey season of 2011–2012. The HCEP used clinical examination, neurocognitive assessment via the Immediate Post-Concussion Assessment and Cognitive Test (ImPACT), and pre- and postseason magnetic resonance imaging (MRI) as well as sequential testing and imaging at three time points after any concussion among ice hockey players.
MRS data of the HCEP participants from the 2011–2012 season have previously been evaluated for a different purpose (7). In contrast to the present study, the previous publication (1) primarily focussed on concussive RHI, (2) used ratios instead of absolute neuro-metabolite concentrations, (3) only considered NAA, Glu, and mI (in relation to Cr) during data analysis, (4) did not apply partial-volume correction, and (5) did not correlate findings of neuro-metabolite changes with neurocognitive performance (7).
Written informed consent and release of medical information was obtained from all participants prior to investigations. The study was approved by a university research ethics board. The clinical data for this study are described by Echlin et al. (24). Individuals were excluded from participation in this study based on general MRI exclusion criteria (i.e., metallic implants), structural MRI abnormalities, previous eye surgery, severe cognitive impairment, and/or a history of any psychiatric or neurological diseases. Concussion was diagnosed by the team physician, using the Zürich consensus statement (25). For this study, participants that completed pre- and postseason MRS were considered.
In total, 45 ice hockey players (25 males and 20 females) were enrolled in the 2011–2012 HCEP. Among this cohort, eight males and four females were excluded in the present study for the following reasons: missing pre- or postseason MRS (six males, two females), poor scan quality in either pre- or postseason MRS (two males and one female) based on signal-to-noise ratio (SNR) less than six, linewidths greater than 0.08 ppm, and visual inspection for substational artifacts, and the incidental finding of a large arachnoid cyst (one female). Thus, 33 participants (17 males and 16 females) were included in the analyses (Table 1).
Neurocognitive Testing
Neurocognitive function was assessed using the ImPACT at two time points: before the season and after the hockey-playing season ends. The ImPACT is a computer-based assessment composed of a concussion symptom inventory as well as modules for assessment of neurocognitive function. Based on the results from the neurocognitive test modules, four composite scores were generated (verbal memory, visual memory, visual motor speed, and reaction time). ImPACT composite scores have been used in previous investigations among the 2011–2012 HCEP participants (26). The ImPACT results were independently evaluated by a neuropsychologist.
Acquisition of Magnetic Resonance Spectroscopy
Data acquisition was performed using a 3T MRI machine (Achieva 3T, Philips Medical Systems, The Netherlands B.V.) equipped with an eight-channel SENSE head coil array. Each player involved in this study received a baseline and postseason MRI evaluation. Athletes who sustained a concussion underwent additional imaging at 72 h, 2 weeks, and 2 months post-injury. This additional imaging data is not part of the current analysis.
The corpus callosum was chosen as the MRS region of interest because it forms the highest-density commissural white matter bundle in the brain. Additionally, it provides connections between hemispheres that project to the cerebrum. Furthermore, the corpus callosum is suspected to be vulnerable to damage from the biomechanical forces involved in concussion and is more predictive of outcomes compared to cortical regions (22, 27–30). The placement of the voxel within the corpus callosum also ensured an adequate distance from the ventricles, fatty tissue, and bone.
Each patient was placed in the MRI machine and underwent a standard localizer and SENSE calibration scan. Voxels were applied for the corpus callosum (10 × 20 × 30 mm). Spectroscopic examination was carried out using a Point RESolved Spectroscopy (PRESS) pulse sequence with the following settings: echo time (TE) = 35 ms, repetition time (TR) = 2,000 ms, 128 acquisitions, and 1,024 points. Each voxel underwent automated optimization including three-dimensional shimming, transmit gain, and water suppression. A representative spectrum and corresponding voxel location is shown in Figure 1.
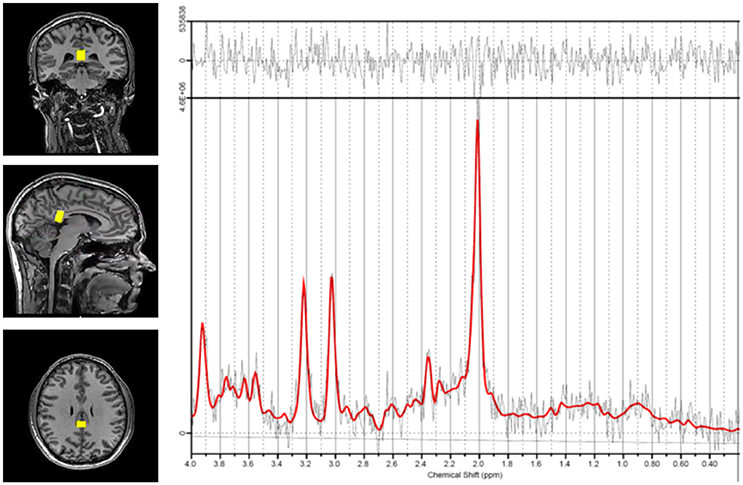
Figure 1. Representative spectrum and voxel location. Voxel positioning in the corpus callosum is shown on the left. The spectrum derived from measurements in this region is shown on the right. The red line indicates the basis set fit of the acquired data (black lines) and the bar at the top shows the residual difference between the fit and the spectrum.
Analysis of Magnetic Resonance Spectroscopy
Phase drift correction and frequency correction were performed prior to analysis with a linear combination model. A linear combination model was also used for metabolite quantification (31). The operator-independent spectral analysis software estimates metabolite concentrations using a set of basis reference spectra acquired from individual metabolites on the MRI instrument and using the water reference spectrum for quantitation. Concentrations are derived from the areas under the corresponding peaks. Cramer-Rao lower bounds (CRLB) were calculated for each neurochemical estimation. Only those metabolites with a CRLB less than 20% were used for data analysis. The following neurochemicals were quantified: NAA, Glu, Cho, Cr, and mI. It should be noted that while Glu CRLB was low, it cannot be ensured that glutamine did not contribute to some extent to the Glu resonance though its contribution is likely to be minimal. Concentrations were partial-volume corrected by segmenting gray matter, white matter, and cerebrospinal fluid (CSF) within the voxel by mapping the voxel onto the T1-weighted image and extracting their volumes. Water concentrations were corrected by multiplying by the following factor:
[(55,556/35,880) × (1.000 × partial volume fraction of CSF) + (0.779 × partial volume of gray matter) + (0.645 × partial volume of white matter)]
This equation corrects for the assumed LCModel water concentration (WCONC; 35,880 mM) with the actual water concentration (55,556 mM), and then corrects for partial volume of each compartment of water, where CSF is assumed 100% water content, gray matter is 77.9%, and white matter is 64.5%. T1 and T2 water relaxation times at 3T (1.0 for CSF, 0.779 for gray matter, and 0.645 for white matter) are incorporated into this equation to correct for the water attenuation in LCmodel (ATTH20) as described previously (32).
Statistical Analyses
All statistical analyses were performed using SPSS (version 24; IBM SPSS Statistics for Windows, IBM Corp., Armonk, NY, USA). The MRS metabolites were examined as absolute concentrations. Repeated measures analysis of covariance (rmANCOVA) was used to examine pre- to postseason changes in the mean concentrations of each MRS metabolite. Sex was included as a between-group factor, in order to examine the presence of a sex × time interaction effect. Any history of a concussion during the season of play was included as a covariate to determine whether season changes in the MRS metabolites were independent of a concussion and associated with subconcussive RHI. This rmANCOVA was conducted for each MRS metabolite and the significance level was adjusted for the family-wise error rate using a Bonferroni adjustment for multiple comparisons, resulting in a significance level set at p < 0.007. A series of bivariate correlations were then conducted to examine the associations among each of the MRS metabolites and the ImPACT composite scores (i.e., verbal memory, visual memory, visual motor speed, and reaction time), which were not separately corrected for multiple comparisons. Figures on results were created using GraphPad Prism (version 6.0; GraphPad Software Inc., La Jolla, CA, USA).
Results
Changes of Neuro-Metabolite Concentrations
Descriptive statistics for each metabolite at preseason and at postseason assessment are presented in Table 2. The average SNR was 8.69 for the preseason scans and 8.94 for the postseason scans. NAA was found to be significantly lower at the end of the season (postseason: 9.02 ± 0.55 mM) compared to the beginning in both males and females (preseason: 9.37 ± 0.59 mM; p = 0.001), regardless of whether the players had a concussion during the season (Figure 2A). Interestingly, when NAA/Cr ratio is used, there is no significant difference found (p = 0.228). No sex differences were observed in total change in NAA.
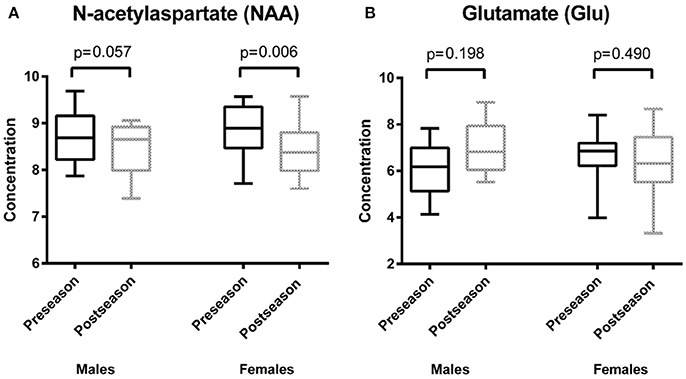
Figure 2. Results of repeated measures analysis of covariance (A) depicts box plots of average values of the concentration of N-acetylaspartate (NAA) at two time points: preseason and postseason. There was a statistically significant difference between pre-and postseason NAA concentrations (p = 0.001). In both males and females, NAA decreased when comparing the preseason to the postseason concentrations (males: p = 0.057, females: p = 0.006). (B) shows box plots of average values of the concentration of glutamate (Glu) at two time points: preseason and postseason. There was a trend regarding the difference between pre- and postseason Glu concentrations (p = 0.039). Females showed a decrease in Glu over time (p = 0.490), whereas males had an increase in Glu over time (p = 0.198).
A decrease in total Cho was observed, but this decrease was not statistically significant (preseason: 1.48 ± 0.16 mM, postseason: 1.41 ± 0.18 mM; p = 0.044). No sex differences were observed in change in total Cho. A sex effect, however, was observed for Glu, though the difference was only of trend level significance (males preseason: 6.57 ± 1.15 mM, postseason: 7.35 ± 1.16 mM; females preseason: 7.24 ± 1.11 mM, postseason: 6.76 ± 1.41 mM; p = 0.039), indicating that while males had an increase in Glu from pre- to postseason assessment, females had a decrease (Figure 2B). No time effect was observed from pre- to postseason MRS in regards to Glu. There was no statistically significant sex or time interactions with respect to concentrations of Cr or mI.
Correlation of Neuro-Metabolite Concentrations With ImPACT Scores
A negative correlation was found between change in Glu from preseason to postseason assessments and verbal memory (p = 0.008; Figure 3). There were no statistically significant neurocognitive differences between male and female participants except that females had a lower average visual memory score at preseason (p = 0.012; Table 1); however, there were no postseason differences.
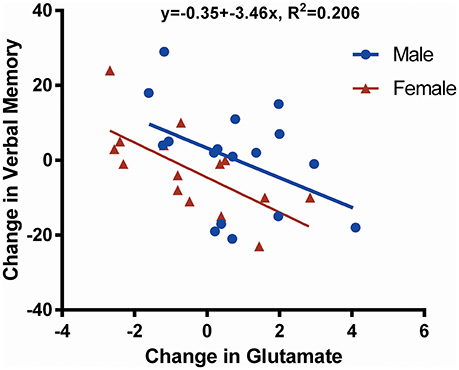
Figure 3. Scatter plot showing change in glutamate (Glu) against change in verbal memory. This figure illustrates the negative correlation (R2 = 0.206, p = 0.008) between the change in verbal memory and the change in Glu concentration over time (postseason minus preseason for males and females together). Data derived from male players are shown in blue, whereas data belonging to female players are shown in red. One female participant did not undergo neurocognitive assessment by the Immediate Post-Concussion Assessment and Cognitive Test (ImPACT); thus, only 32 data points are shown.
Discussion
This study found a significant decrease in NAA between pre- and postseason assessment in both male and female athletes (p = 0.001). A decrease in total Cho over the course of the season was observed but did not reach statistical significance when considering correction for multiple comparisons (p = 0.044). Females demonstrated a trend toward a decrease in Glu over the course of the play season while males demonstrated a trend toward an increase in Glu (p = 0.039). In both males and females, however, a negative correlation was observed between changes in Glu and changes in verbal memory (p = 0.008), as measured by the ImPACT.
Changes in N-Acetylaspartate
The significant decrease in NAA, a marker of viable neurons, from pre- to postseason was observed regardless of sex or whether the player had an in-season concussion. This result does not directly corroborate the findings of previous researchers, which have demonstrated a transient decrease in NAA after concussion, followed by a recovery in NAA (8, 33). The results of the present study suggest a persistent decrease in NAA that is still present at the end of the playing season, as opposed to a transient decrease followed by a recovery in NAA levels.
There are, however, several reasons why our findings differ from previous research. First, most previous studies investigated NAA levels in other cerebral areas, including the (dorsolateral) prefrontal cortex, posterior cingulate gyrus, or primary motor cortex (8–14). The present study investigated neuro-metabolic alterations in the corpus callosum. Therefore, it is possible that the recovery of NAA is specific to cortical areas, whereas white matter dense areas such as the corpus callosum may not be as amenable to recovery compared to cortical regions. In this context, long-coursing axons, as are present in the corpus callosum, may be more vulnerable to injury and evince less transient changes when compared to other regions (22, 30). Secondly, previous studies that demonstrated a recovery in NAA have studied concussed subjects, whereas the present study investigates both subjects with exposure to subconcussive and concussive RHI (8, 34). A previous study using participants also from the HCEP demonstrated that females who did not have an in-season concussion had a persistent decrease in NAA (i.e., at the postseason measurement), while males did not demonstrate any changes in NAA (7). One key difference between the (7) study and this study is that the NAA/Cr ratio was utilized whereas the present study used the absolute concentration of NAA (and absolute concentrations of other neuro-metabolites) (7). We chose to use absolute concentrations as opposed to the NAA/Cr ratio because it is known that Cr levels change in brain injury; therefore, we cannot distinctly assume Cr as a constant and thus should not use Cr as an internal reference standard for MRS (35, 36). As shown in the results, NAA/Cr did not show differences in this particular cohort whereas NAA concentration was significant.
The decrease in NAA levels from pre- to postseason, regardless of sex or the presence of an in-season concussion, further suggests that RHI contribute to diffuse axonal injury and neuronal loss in the corpus callosum. In this context, decreases in NAA, a marker of axonal function and integrity, are suggestive for axonal degeneration or loss (15–17, 37). Decreases in NAA are also observed in neuroinflammation and demyelination (38, 39). However, NAA alterations due to recent vigorous physical training cannot be excluded (40, 41). Thus, our results may reflect a mixed picture with the above-mentioned and other factors contributing to the observed NAA decline.
Changes in Glutamate
A trend of sex-specific difference was observed for Glu in that females had a decrease in Glu from pre- to postseason while males had an increase in Glu. This sex difference may be attributed to hormonal regulation of this excitatory neurotransmitter. The neuroprotective properties of estrogen and progesterone are well-known (42). Progesterone is thought to suppress the excitatory Glu response, while estrogen is thought to facilitate the effects of Glu transmission (43). This balance of ovarian hormones is essential for protecting the female brain from insults such as Glu excitotoxicity and oxidative stress (44, 45). Thus, the trend for a decrease in Glu in response to RHI observed in the present study may be attributed to hormonal regulation of Glu and neuroprotective properties of ovarian hormones in preventing Glu-mediated excitotoxicity.
Furthermore, previous research suggests Glu levels increase in response to injury in the male brain (11). Therefore, it is possible that the male brain does not have the hormonal neuroprotection against brain insults in the same way the female brain does. However, it is important to note that neuro-metabolite concentrations vary depending on which brain region is studied. From previous research, it is likely that Glu increases in response to brain injury, possibly potentiating aberrant neuronal signaling and propagating further signaling cascades in response to injury (11, 46). However, the regions assessed in previous research are gray matter regions; thus, the generalizability of these findings to the present study is limited. Other studies have demonstrated an increase in Glu in the splenium of the corpus callosum following mild traumatic brain injury, while a decrease in Glu in gray matter was observed (36, 47). This observation affirms the increased vulnerability of the corpus callosum to head injury, and possibly contributes to an increased likelihood of excitotoxicity in white matter regions compared to gray matter regions. However, sex differences were not investigated; thus, it is unclear if the same relationship was observed in both males and females. Furthermore, a recent study also including subjects of the HCEP demonstrated sex-dependent changes in white matter diffusivity following subconcussive head impacts, which could be due to differential hormonal regulation between males and females (48). Taken together, it is possible that hormonal regulation may play an essential role in differing levels of Glu in the corpus callosum. However, further studies are needed to elucidate the downstream consequences of increased Glu in white matter regions, and to clarify whether these downstream effects are sex-dependent.
The overall change in Glu was negatively correlated with changes in verbal memory. Previous research suggests Glu is increased in Alzheimer's disease (AD), playing a role in the pathophysiology of excitotoxicity due to an excess of N-methyl-D-aspartate (NMDA) receptor activation (49). The drug memantine is used for the treatment of cognitive impairment symptoms in AD and other dementias, and by acting as an NMDA receptor antagonist, it further supports the idea that Glu excitotoxicity may play a role in the cognitive symptomology of AD (50). To our knowledge, there are no studies linking an excess of Glu to a decline in verbal memory; however, it has been shown that Glu is necessary in order to perform verbal memory tasks (51, 52). Hence, an excess of Glu may be related to impaired verbal memory. This suggests an inverse relationship between excess of Glu and memory, which is in line with the findings of the present study.
Changes in Choline
A decrease, though not statistically significant, was seen in Cho, a marker of cell turnover, from pre- to postseason. Previous studies have demonstrated conflicting evidence for how Cho changes in brain injury. Many studies have reported an increase in Cho in response to brain injury, suggesting tissue breakdown and inflammation (11, 19, 53). On the other hand, another study did not find a change in Cho due to mild traumatic brain injury (54). There is modest evidence for a decrease in Cho in a cohort of retired rugby players (21). However, further studies need to be conducted in order to elucidate the complex relationship between Cho and RHI.
Limitations
There are several limitations to this study. First, the generalizability of our findings is limited by the small sample size and the lack of a control group which would have been helpful to determine if baseline metabolite levels differ between athletes and non-athletes and whether the NAA changes are chronic or if the changes observed may be the result of data acquisition or analysis. However, this study used a prospective study design including imaging and clinical assessment in which independent non-biased specialist physician investigators were present at each game. The study investigates a broad sample of neuro-metabolites that are not routinely used in MRS studies on brain injury, and it is one of the first studies to investigate sex-specific differences in neuro-metabolites over time.
Secondly, the ImPACT, primarily designed for the detection of concussion-related symptoms, may not be sufficiently sensitive for the detection of subtle neurocognitive alterations of subjects also exposed to subconcussive RHI. At the time of data acquisition, the ImPACT assessment represented one of the few widely accessible tools to measure specific changes in neurocognitive function; however, later studies investigated more sensitive methods to assess the effects of RHI (55–57). Future studies on RHI should utilize improved, valid, and more reliable neuropsychological measures.
Thirdly, the quality of the MRS data obtained was not optimal as each scan had a comparatively low SNR. Future studies should use a larger voxel size and increase the number of averages when acquiring MRS data to ensure high data quality. Another weakness is that by using a single voxel in the corpus callosum, we were not able to study other brain regions that may be vulnerable to RHI. Future studies need to use multiple voxel locations.
Lastly, we are not able to assess whether or not the concentrations of any of the neuro-metabolites changed following the end of the season since we do not have follow-up measurements after the postseason MRS assessments. Future longitudinal studies should include follow-up examinations at different time points after the end of a period of exposure to RHI to assess if neuro-metabolite levels remain persistently altered, or if it simply takes longer to recover after RHI. Prolonged recovery after NAA decrease due to RHI has been suggested previously (58). Furthermore, prospective studies should assess hormone levels and medications taken (particularly hormonal contraceptives) during the season to assess a possible relationship between hormonal changes and neuro-metabolite changes.
Conclusion
This study investigated differences in the change in absolute concentrations of a broad spectrum of neuro-metabolites in athletes exposed to concussive and subconcussive RHI while playing collegiate ice hockey, and, importantly, it also evaluated sex differences in these changes. Results of this study demonstrate a significant decrease in NAA, a marker of viable neurons, following exposure to RHI in both female and male athletes. Moreover, results suggest possible sex-specific differences in changes in Glu, though not statistically significant after correction for multiple comparisons. In both sexes, a negative correlation was observed between changes in Glu and changes in verbal memory. Future studies need to investigate further the association between neuro-metabolite concentration changes and clinical outcomes as well as the association of individual hormone levels and the differences in the brain's response to RHI between male and female athletes.
Availability of Data and Material
All data used for analysis are presented in the manuscript. The discussion and conclusions only rely on the data presented.
Ethics Statement
This study was carried out in accordance with the recommendations of the ethics committees at each CIS university with written informed consent from all subjects. All subjects gave written informed consent in accordance with the Declaration of Helsinki.
Author Contributions
PE is responsible for concept and design. HP, NS, OP, MA, PK DK, EH, LF, AJ, ES, MS, IK, PE, and AL were responsible for data acquisition and/or analyses, handled the acquired data, conducted statistical analysis, and performed literature research. HP, NS, IK, and AL drafted the manuscript. All authors reviewed and approved the final version of the manuscript.
Funding
This work was conducted with support from Harvard Catalyst | The Harvard Clinical and Translational Science Center (National Center for Research Resources and the National Center for Advancing Translational Sciences, National Institutes of Health Award UL1 TR001102) and financial contributions from Harvard University and its affiliated academic healthcare centers. Funding for this work was also provided to the HCEP and PE by the Ontario Trillium Foundation, The Dave Irwin Foundation for Brain Injury, the Ontario Neurotrauma Foundation, Air Canada, The Ontario Ministry of Health and Long Term Care, The Ontario Ministry of Tourism Culture and Sport and The Ontario Ministry of Education. The authors of this study were supported by the NIH (U01 NS 093334: IK, MS; R01 NS 100952: IK, AL), the Veterans Affairs (VA Merit Award I01 RX00928: MS), and the Department of Defense Congressionally Directed Medical Research Programs (W81XWH-08-2-0159: MS, WX81XWH-15-1-0412: AL). This research project was also partially supported by LMU Munich's Institutional Strategy LMUexcellent within the framework of the German Excellence Initiative (IK). The content is solely the responsibility of the authors and does not necessarily represent the official views of Harvard Catalyst, Harvard University and its affiliated academic healthcare centers, or the National Institutes of Health.
Conflict of Interest Statement
The authors declare that the research was conducted in the absence of any commercial or financial relationships that could be construed as a potential conflict of interest.
Acknowledgments
The authors acknowledge the players and staffs of two CIS varsity ice hockey teams for their participation in the HCEP, and the participating physicians, observers, and volunteers for their contributions to the HCEP. The authors would also like to acknowledge the contributions of the University of British Columbia MRI Center and all of the associated researchers and employees, especially Ms. Trudy Harris and Ms. Linda Chandler.
Abbreviations
AD, Alzheimer's disease; Cho, choline; CIS, Canadian Interuniversity Sports; Cr, creatine; CRLB, Cramer-Rao lower bounds; CSF, cerebrospinal fluid; GABA, gamma-aminobutyric acid; Glu, glutamate; GSH, glutathione; HCEP, Hockey Concussion Education Project; ImPACT, Immediate Post-Concussion Assessment and Cognitive Test; mI, myoinositol; MRI, magnetic resonance imaging; MRS, magnetic resonance spectroscopy; NAA, N-acetylaspartate; NMDA, N-methyl-D-aspartate; PRESS, Point RESolved Spectroscopy; RHI, repetitive head impacts; rmANCOVA, repeated measures analysis of covariance; SD, standard deviation; SNR, signal-to-noise ratio; TE, echo time; TR, repetition time.
References
1. Agel J, Dompier TP, Dick R, Marshall SW. Descriptive epidemiology of collegiate men's ice hockey injuries: National Collegiate Athletic Association Injury Surveillance System, 1988–1989 through 2003–2004. J Athl Train. (2007) 42:241–8.
2. Agel J, Dick R, Nelson B, Marshall SW, Dompier TP. Descriptive epidemiology of collegiate women's ice hockey injuries: National Collegiate Athletic Association Injury Surveillance System, 2000–2001 through 2003–2004. J Athl Train. (2007) 42:249–54.
3. Irick E. NCAA® Sports Sponsorship and Participation Rates Report. Indianapolis, IN: National Collegiate Athletic Association (2015).
4. Zuckerman SL, Apple RP, Odom MJ, Lee YM, Solomon GS, Sills AK Effect of sex on symptoms and return to baseline in sport-related concussion. J Neurosurg Pediatr. (2014) 13:72–81. doi: 10.3171/2013.9.PEDS13257
5. Baker JG, Leddy JJ, Darling SR, Shucard J, Makdissi M, Willer BS. Gender differences in recovery from sports-related concussion in adolescents. Clin Pediatr (Phila). (2016) 55:771–5. doi: 10.1177/0009922815606417.
6. Brainard LL, Beckwith JG, Chu JJ, Crisco JJ, McAllister TW, Duhaime AC, et al. Gender differences in head impacts sustained by collegiate ice hockey players. Med Sci Sports Exerc. (2012) 44:297–304. doi: 10.1249/MSS.0b013e31822b0ab4
7. Chamard E, Theoret H, Skopelja EN, Forwell LA, Johnson AM, Echlin PS. A prospective study of physician-observed concussion during a varsity university hockey season: metabolic changes in ice hockey players. Part 4 of 4. Neurosurg Focus. (2012) 33:E4:1–7. doi: 10.3171/2012.10.FOCUS12305
8. Vagnozzi R, Signoretti S, Tavazzi B, Floris R, Ludovici A, Marziali S, et al. Temporal window of metabolic brain vulnerability to concussion: a pilot 1H-magnetic resonance spectroscopic study in concussed athletes–part III. Neurosurgery (2008) 62:1286–95; discussion 95–6. doi: 10.1227/01.neu.0000333300.34189.74
9. Poole VN, Abbas K, Shenk TE, Breedlove EL, Breedlove KM, Robinson ME, et al. MR spectroscopic evidence of brain injury in the non-diagnosed collision sport athlete. Dev Neuropsychol. (2014) 39:459–73. doi: 10.1080/87565641.2014.940619
10. Tremblay S, De Beaumont L, Henry LC, Boulanger Y, Evans AC, Bourgouin P, et al. Sports concussions and aging: a neuroimaging investigation. Cereb Cortex (2013) 23:1159–66. doi: 10.1093/cercor/bhs102
11. Lin AP, Ramadan S, Stern RA, Box HC, Nowinski CJ, Ross BD, et al. Changes in the neurochemistry of athletes with repetitive brain trauma: preliminary results using localized correlated spectroscopy. Alzheimers Res Ther. (2015) 7:13. doi: 10.1186/s13195-015-0094-5
12. Poole VN, Breedlove EL, Shenk TE, Abbas K, Robinson ME, Leverenz LJ, et al. Sub-concussive hit characteristics predict deviant brain metabolism in football athletes. Dev Neuropsychol. (2015) 40:12–7. doi: 10.1080/87565641.2014.984810
13. Koerte IK, Lin AP, Muehlmann M, Merugumala S, Liao H, Starr T, et al. Altered neurochemistry in former professional Soccer players without a history of concussion. J Neurotrauma (2015) 32:1287–93. doi: 10.1089/neu.2014.3715
14. Henry LC, Tremblay S, Leclerc S, Khiat A, Boulanger Y, Ellemberg D, et al. Metabolic changes in concussed American football players during the acute and chronic post-injury phases. BMC Neurol. (2011) 11:105. doi: 10.1186/1471-2377-11-105
15. Lin A, Tran T, Bluml S, Merugumala S, Liao HJ, Ross BD. Guidelines for acquiring and reporting clinical neurospectroscopy. Semin Neurol. (2012) 32:432–53. doi: 10.1055/s-0032-1331814
16. Gardner A, Iverson GL, Stanwell P. A systematic review of proton magnetic resonance spectroscopy findings in sport-related concussion. J Neurotrauma (2014) 31:1–18. doi: 10.1089/neu.2013.3079
17. Koerte IK, Lin AP, Willems A, Muehlmann M, Hufschmidt J, Coleman MJ, et al. A review of neuroimaging findings in repetitive brain trauma. Brain Pathol. (2015) 25:318–49. doi: 10.1111/bpa.12249
18. Ross BD, Ernst T, Kreis R, Haseler LJ, Bayer S, Danielsen E, et al. 1H MRS in acute traumatic brain injury. J Magn Reson Imaging (1998) 8:829–40. doi: 10.1002/jmri.1880080412
19. Marino S, Zei E, Battaglini M, Vittori C, Buscalferri A, Bramanti P, et al. Acute metabolic brain changes following traumatic brain injury and their relevance to clinical severity and outcome. J Neurol Neurosurg Psychiatry (2007) 78:501–7. doi: 10.1136/jnnp.2006.099796
20. Friedman SD, Poliakov AV, Budech C, Shaw DWW, Breiger D, Jinguji T, et al. GABA alterations in pediatric sport concussion. Neurology (2017) 89:2151–6. doi: 10.1212/WNL.0000000000004666
21. Gardner AJ, Iverson GL, Wojtowicz M, Levi CR, Kay-Lambkin F, Schofield PW, et al. MR spectroscopy findings in retired professional Rugby league players. Int J Sports Med. (2017) 38:241–52. doi: 10.1055/s-0042-120843
22. Smits M, Houston GC, Dippel DW, Wielopolski PA, Vernooij MW, Koudstaal PJ, et al. Microstructural brain injury in post-concussion syndrome after minor head injury. Neuroradiology (2011) 53:553–63. doi: 10.1007/s00234-010-0774-6
23. Chamard E, Lassonde M, Henry L, Tremblay J, Boulanger Y, De Beaumont L, et al. Neurometabolic and microstructural alterations following a sports-related concussion in female athletes. Brain Inj. (2013) 27:1038–46. doi: 10.3109/02699052.2013.794968
24. Echlin PS. A prospective study of physician-observed concussion during a varsity university ice hockey season. Part 1 of 4. Neurosurg Focus (2012) 33:E1–7. doi: 10.3171/2012.9.FOCUS12287
25. McCrory P, Meeuwisse W, Aubry M, Cantu B, Dvorak J, Echemendia R, et al. Consensus statement on concussion in sport–the 4th international conference on concussion in sport held in Zurich, November 2012. Phys Ther Sport. (2013) 14:e1–13. doi: 10.1016/j.ptsp.2013.03.002
26. Echlin PS, Skopelja EN, Worsley R, Dadachanji SB, Lloyd-Smith DR, Taunton JA, et al. A prospective study of physician-observed concussion during a varsity university ice hockey season: incidence and neuropsychological changes. Part 2 of 4. Neurosurg Focus (2012) 33:E2: 1–11. doi: 10.3171/2012.10.FOCUS12286
27. Holshouser BA, Tong KA, Ashwal S, Oyoyo U, Ghamsary M, Saunders D, et al. Prospective longitudinal proton magnetic resonance spectroscopic imaging in adult traumatic brain injury. J Magn Reson Imaging (2006) 24:33–40. doi: 10.1002/jmri.20607
28. Johnson B, Gay M, Zhang K, Neuberger T, Horovitz SG, Hallett M, et al. The use of magnetic resonance spectroscopy in the subacute evaluation of athletes recovering from single and multiple mild traumatic brain injury. J Neurotrauma (2012) 29:2297–304. doi: 10.1089/neu.2011.2294
29. Johnson B, Zhang K, Gay M, Neuberger T, Horovitz S, Hallett M, et al. Metabolic alterations in corpus callosum may compromise brain functional connectivity in MTBI patients: an 1H-MRS study. Neurosci Lett. (2012) 509:5–8. doi: 10.1016/j.neulet.2011.11.013
30. Nishimoto T, Murakami S. Relation between diffuse axonal injury and internal head structures on blunt impact. J Biomech Eng. (1998) 120:140–7. doi: 10.1115/1.2834294
31. Provencher SW. Estimation of metabolite concentrations from localized in vivo proton NMR spectra. Magn Reson Med. (1993) 30:672–9.
32. Quadrelli S, Mountford C, Ramadan S. Hitchhiker's guide to voxel segmentation for partial volume correction of in vivo magnetic resonance spectroscopy. Magn Reson Insights (2016) 9:1–8. doi: 10.4137/MRI.S32903
33. Henry LC, Tremblay S, Boulanger Y, Ellemberg D, Lassonde M. Neurometabolic changes in the acute phase after sports concussions correlate with symptom severity. J Neurotrauma (2010) 27:65–76. doi: 10.1089/neu.2009.0962
34. Vagnozzi R, Signoretti S, Cristofori L, Alessandrini F, Floris R, Isgro E, et al. Assessment of metabolic brain damage and recovery following mild traumatic brain injury: a multicentre, proton magnetic resonance spectroscopic study in concussed patients. Brain (2010) 133:3232–42. doi: 10.1093/brain/awq200
35. Croall I, Smith FE, Blamire AM. Magnetic resonance spectroscopy for traumatic brain injury. Top Magn Reson Imaging (2015) 24:267–74. doi: 10.1097/RMR.0000000000000063
36. Yeo RA, Gasparovic C, Merideth F, Ruhl D, Doezema D, Mayer AR. A longitudinal proton magnetic resonance spectroscopy study of mild traumatic brain injury. J Neurotrauma (2011) 28:1–11. doi: 10.1089/neu.2010.1578
37. Rigotti DJ, Inglese M, Gonen O. Whole-brain N-acetylaspartate as a surrogate marker of neuronal damage in diffuse neurologic disorders. AJNR Am J Neuroradiol. (2007) 28:1843–9. doi: 10.3174/ajnr.A0774
38. Mader I, Rauer S, Gall P, Klose U. (1)H MR spectroscopy of inflammation, infection and ischemia of the brain. Eur J Radiol. (2008) 67:250–7. doi: 10.1016/j.ejrad.2008.02.033
39. Chang L, Munsaka SM, Kraft-Terry S, Ernst T. Magnetic resonance spectroscopy to assess neuroinflammation and neuropathic pain. J Neuroimmune Pharmacol. (2013) 8:576–93. doi: 10.1007/s11481-013-9460-x
40. Maddock RJ, Casazza GA, Fernandez DH, Maddock MI. Acute modulation of cortical glutamate and GABA content by physical activity. J Neurosci. (2016) 36:2449–57. doi: 10.1523/JNEUROSCI.3455-15.2016
41. Erickson KI, Weinstein AM, Sutton BP, Prakash RS, Voss MW, Chaddock L, et al. Beyond vascularization: aerobic fitness is associated with N-acetylaspartate and working memory. Brain Behav. (2012) 2:32–41. doi: 10.1002/brb3.30
42. Wei J, Xiao GM. The neuroprotective effects of progesterone on traumatic brain injury: current status and future prospects. Acta Pharmacol Sin. (2013) 34:1485–90. doi: 10.1038/aps.2013.160
43. Barth C, Villringer A, Sacher J. Sex hormones affect neurotransmitters and shape the adult female brain during hormonal transition periods. Front Neurosci. (2015) 9:37. doi: 10.3389/fnins.2015.00037
44. Mize AL, Shapiro RA, Dorsa DM. Estrogen receptor-mediated neuroprotection from oxidative stress requires activation of the mitogen-activated protein kinase pathway. Endocrinology (2003) 144:306–12. doi: 10.1210/en.2002-220698
45. Behl C, Widmann M, Trapp T, Holsboer F. 17-beta estradiol protects neurons from oxidative stress-induced cell death in vitro. Biochem Biophys Res Commun. (1995) 216:473–82. doi: 10.1006/bbrc.1995.2647
46. Hinzman JM, Thomas TC, Burmeister JJ, Quintero JE, Huettl P, Pomerleau F, et al. Diffuse brain injury elevates tonic glutamate levels and potassium-evoked glutamate release in discrete brain regions at two days post-injury: an enzyme-based microelectrode array study. J Neurotrauma (2010) 27:889–99. doi: 10.1089/neu.2009.1238
47. Gasparovic C, Yeo R, Mannell M, Ling J, Elgie R, Phillips J, et al. Neurometabolite concentrations in gray and white matter in mild traumatic brain injury: an 1H-magnetic resonance spectroscopy study. J Neurotrauma (2009) 26:1635–43. doi: 10.1089/neu.2009-0896
48. Sollmann N, Echlin PS, Schultz V, Viher PV, Lyall AE, Tripodis Y, et al. Sex differences in white matter alterations following repetitive subconcussive head impacts in collegiate ice hockey players. NeuroImage Clin. (2018) 17:642–9. doi: 10.1016/j.nicl.2017.11.020
49. Hynd MR, Scott HL, Dodd PR. Glutamate-mediated excitotoxicity and neurodegeneration in Alzheimer's disease. Neurochem Int. (2004) 45:583–95. doi: 10.1016/j.neuint.2004.03.007
50. Molinuevo JL, Llado A, Rami L. Memantine: targeting glutamate excitotoxicity in Alzheimer's disease and other dementias. Am J Alzheimers Dis Other Demen. (2005) 20:77–85. doi: 10.1177/153331750502000206
51. Wagner G, Gussew A, Kohler S, de la Cruz F, Smesny S, Reichenbach JR, et al. Resting state functional connectivity of the hippocampus along the anterior-posterior axis and its association with glutamatergic metabolism. Cortex (2016) 81:104–17. doi: 10.1016/j.cortex.2016.03.022
52. Nikolova S, Stark SM, Stark CEL. 3T hippocampal glutamate-glutamine complex reflects verbal memory decline in aging. Neurobiol Aging (2017) 54:103–11. doi: 10.1016/j.neurobiolaging.2017.01.026
53. Yoon SJ, Lee JH, Kim ST, Chun MH. Evaluation of traumatic brain injured patients in correlation with functional status by localized 1H-MR spectroscopy. Clin Rehabil. (2005) 19:209–15. doi: 10.1191/0269215505cr813oa
54. Kirov, II, Tal A, Babb JS, Lui YW, Grossman RI, Gonen O. Diffuse axonal injury in mild traumatic brain injury: a 3D multivoxel proton MR spectroscopy study. J Neurol. (2013) 260:242–52. doi: 10.1007/s00415-012-6626-z
55. Zhang MR, Red SD, Lin AH, Patel SS, Sereno AB. Evidence of cognitive dysfunction after soccer playing with ball heading using a novel tablet-based approach. PLoS ONE (2013) 8:e57364. doi: 10.1371/journal.pone.0057364
56. Koerte IK, Nichols E, Tripodis Y, Schultz V, Lehner S, Igbinoba R, et al. Impaired cognitive performance in youth athletes exposed to repetitive head impacts. J Neurotrauma (2017) 34:2389–95. doi: 10.1089/neu.2016.4960
57. Echemendia RJ, Bruce JM, Meeuwisse W, Comper P, Aubry M, Hutchison M. Long-term reliability of ImPACT in professional ice hockey. Clin Neuropsychol. (2016) 30:328–37. doi: 10.1080/13854046.2016.1158320
Keywords: magnetic resonance spectroscopy, ice hockey, sex difference, traumatic brain injury, repetitive head injury
Citation: Panchal H, Sollmann N, Pasternak O, Alosco ML, Kinzel P, Kaufmann D, Hartl E, Forwell LA, Johnson AM, Skopelja EN, Shenton ME, Koerte IK, Echlin PS and Lin AP (2018) Neuro-Metabolite Changes in a Single Season of University Ice Hockey Using Magnetic Resonance Spectroscopy. Front. Neurol. 9:616. doi: 10.3389/fneur.2018.00616
Received: 11 December 2017; Accepted: 09 July 2018;
Published: 20 August 2018.
Edited by:
Massimo S. Fiandaca, University of California, Irvine, United StatesReviewed by:
Rao P. Gullapalli, University of Maryland, United StatesJamie Near, McGill University, Canada
Copyright © 2018 Panchal, Sollmann, Pasternak, Alosco, Kinzel, Kaufmann, Hartl, Forwell, Johnson, Skopelja, Shenton, Koerte, Echlin and Lin. This is an open-access article distributed under the terms of the Creative Commons Attribution License (CC BY). The use, distribution or reproduction in other forums is permitted, provided the original author(s) and the copyright owner(s) are credited and that the original publication in this journal is cited, in accordance with accepted academic practice. No use, distribution or reproduction is permitted which does not comply with these terms.
*Correspondence: Alexander P. Lin, QXBsaW5AYndoLmhhcnZhcmQuZWR1
†These authors have contributed equally to this work