- 1School of Psychology, Keele University, Newcastle-under-Lyme, United Kingdom
- 2Department of Neurosciences, Biomedicine and Movement Sciences, University of Verona, Verona, Italy
Background: In Parkinson's disease (PD), impulse control disorders (ICDs) develop as side-effect of dopaminergic replacement therapy (DRT). Cognitive, affective, and motivational correlates of ICD in medicated PD patients are debated. Here, we systematically reviewed and meta-analyzed the evidence for an association between ICD in PD and cognitive, affective, and motivational abnormalities.
Methods: A systematic review and meta-analysis was performed on PubMed, Science Direct, ISI Web of Science, Cochrane, EBSCO for studies published between 1-1-2000 and 8-3-2017 comparing cognitive, affective, and motivational measures in PD patients with ICD (ICD+) vs. those without ICD (ICD–). Exclusion criteria were conditions other than PD, substance and/or alcohol abuse, dementia, drug naïve patients, cognition assessed by self-report tools. Standardized mean difference (SMD) was used, and random-effect model applied.
Results: 10,200 studies were screened (title, abstract), 79 full-texts were assessed, and 25 were included (ICD+: 625 patients; ICD–: 938). Compared to ICD–, ICD+ showed worse performance reward-related decision-making (0.42 [0.02, 0.82], p = 0.04) and set-shifting tasks (SMD = −0.49 [95% CI −0.78, −0.21], p = 0.0008). ICD in PD was also related to higher self-reported rate of depression (0.35 [0.16, 0.54], p = 0.0004), anxiety (0.43 [0.18, 0.68], p = 0.0007), anhedonia (0.26 [0.01, 0.50], p = 0.04), and impulsivity (0.79 [0.50, 1.09], p < 0.00001). Heterogeneity was low to moderate, except for depression (I2 = 61%) and anxiety (I2 = 58%).
Conclusions: ICD in PD is associated with worse set-shifting and reward-related decision-making, and increased depression, anxiety, anhedonia, and impulsivity. This is an important area for further studies as ICDs have negative impact on the quality of life of patients and their caregivers.
Introduction
Impulse control disorders (ICDs), such as pathological gambling, hypersexuality, binge-eating, and compulsive shopping, can occur in over 13% of medicated Parkinson's disease (PD) patients (1). Although ICDs are recognized as side-effect of dopamine replacement therapy (DRT), mainly D2 dopamine agonists and levodopa, their pathophysiology is unclear.
It has been hypothesized that, in vulnerable individuals, DRT used to restore dopamine levels in nigrostriatal circuitry may overstimulate the less severely affected mesocorticolimbic circuitry (2). Mesocorticolimbic overstimulation may disrupt prefrontal-dependent executive function, affect and motivation and thus increase vulnerability to ICD. According to this view, in medicated PD patients, we should expect a correlation between ICD and cognitive, affective and motivational factors. However, data in the literature are inconclusive.
Studies on cognition, affective processing and motivation conducted in small cohorts of PD patients with and without ICD (i.e., n: 17–155 patients) yielded inconsistent findings with respect to frontal cognitive abilities in PD patients with ICD. Some studies reported worse performance in executive function, including set-shifting (3–7), working memory (8), concept formation and reasoning (5, 7), and reward-related decision-making (9–15) in PD with ICD (ICD+) compared to PD without ICD (ICD-). Conversely, other studies found similar performances for inhibition (9, 16–18), set-shifting (19, 20), working memory (3, 11, 17, 21, 22), and reward-related decision-making (16, 17, 20, 23). Finally, a single study reported better executive functions in ICD+ (24). Reports on affective factors are also inconclusive, as self-reported depression and anxiety were sometimes found to be associated with ICD (18, 20, 21, 25–28), and sometimes not (3–6, 17, 19, 22, 29–31). However, motivational factors such as self-reported apathy (11, 21, 27, 28), anhedonia (27, 32), and impulsivity (17, 20–22, 32) appeared to be elevated in ICD+ vs. ICD–.
A recent meta-analysis identified several cognitive subdomains (i.e., concept formation, set-shifting, reward-related decision-making, and visuospatial abilities) to be worse in ICD+ vs. ICD– (33), but it included a mixed sample of medicated and drug naïve patients that did not allow to explore the relationship between cognitive disturbances, DRT and ICD.
Moreover, it included patients with comorbidities for substance abuse and/or dementia, two factors that could be independently associated with cognitive changes. Finally, the relationship between cognition-emotion and cognition-motivation, critical to understanding the broader context in which ICDs develop, was not explored in the previous meta-analysis (34).
To reconcile discordant findings in the literature about cognitive, affective and motivational correlates of ICD in medicated PD patients, a systematic review and meta-analysis was conducted. Moreover, this work is meant to address the issues of a previous meta-analysis and to offer new information on this topic. To this aim, we applied stricter inclusion and exclusion criteria, by including only studies on PD patients under DRT at the time of assessment and free from co-morbid substance abuse and/or dementia. Moreover, we included studies with affective and motivational measures, so that any cognitive change could be interpreted within the broader context of cognition-emotion and cognition-motivation relationships (34). A clear understanding of cognitive, affective and motivational changes in ICD may indirectly increase our understanding of ICD pathophysiology and in turn its management.
Methods
Study Design, Participants, and Comparators
A systematic review and meta-analysis were performed to identify cognitive, affective and motivational factors associated with ICD in PD under DRT (ICD+). The comparator group was patients with PD but no ICD (ICD–).
Search Strategy and Selection Criteria
On June 26th 2016, PubMed, Science Direct, ISI Web of Science, Cochrane, EBSCO were searched for peer-reviewed papers in English, Italian and Spanish published since January 2000, when the first report of ICD development after dopaminergic medication initiation was reported (35). The systematic review was further updated on March 8th 2017.
Studies were identified using the following string (36) in PubMed: “(Parkinson's disease) AND (impulse control disorders OR impulsivity OR cognition OR decision-making).” The search strategy for the other databases included (Parkinson's disease) AND (impulse control disorders), then (Parkinson's disease) AND (impulsivity), then (Parkinson's disease) AND (cognition), and (Parkinson's disease) AND (decision-making). A total of 40,672 papers were identified. After exclusion of duplicates, 10,200 papers were title and abstract screened.
Studies were included if: (a) PD patients were under DRT; (b) ICD assessment was performed in a reliable manner with the Questionnaire for Impulsive-Compulsive Disorders in Parkinson's Disease (QUIP), the QUIP rating scale (QUIP-rs), the Minnesota Impulse Disorders Interview, clinical interview based on diagnostic criteria, or a combination of these; (c) performances of PD patients with ICD (ICD+) were compared with those with PD but no history of ICD (ICD–); (d) cognitive, affective, and/or motivational measures were reported. A further inclusion criterion was independence of samples. Only baseline data for prospective studies and the study with the largest sample for multiple studies published by the same author(s) were included.
We excluded reviews, case studies, commentaries, letters, abstracts and dissertations, and postal surveys. Studies including drug naïve PD patients were also excluded since we were interested in ICD developed as a DRT side-effect. Studies in which PD patients underwent non-pharmacological treatments such as deep brain stimulation (DBS) were excluded. This criterion was based on controversial reports of either ICD amelioration or ICD appearance after DBS (37), and the notion that DBS may worsen some cognitive outcomes (38). Studies including participants with dementia and drug/alcohol abuse were excluded, as these conditions might be independently associated with cognitive and neuropsychiatric changes. Other exclusion criteria were: cognition assessed by self-report measures or by general screening tools (e.g., Mini-Mental State Examination) because of their limited specificity and sensitivity (39). Studies focusing on dopamine dysregulation syndrome and/or punding only were not included since these conditions are considered different from ICD, as they are more common in patients with advanced PD, cognitive impairment and dementia (40). However, screening questionnaires (e.g., QUIP, QUIP-rs) include dopamine dysregulation syndrome and punding, and some ICD+ patients we included may have had these conditions too, in addition to ICD. Finally, to ensure that the ICD- group included patients without any type of ICD, studies not assessing all ICD types (e.g., using only the South Oaks Gambling Screen) were excluded.
Data Extraction
Following exclusion of duplicate and irrelevant articles through title and abstract screening, 79 papers were included for full-text evaluation. Reference lists of these studies were manually searched to identify additional relevant articles, and two papers were included at this stage.
Two reviewers (AM, DDL) independently screened titles and abstracts using Rayyan software (41), and three reviewers (AM, DDL, ST) independently evaluated papers selected for full-text examination. Disagreements were resolved through discussions. Disagreement concerned one paper (42) over the 75 selected for full-text examination (inter-rater agreement: 98.67%). Twenty-five articles were included for quantitative analysis (Figure 1).
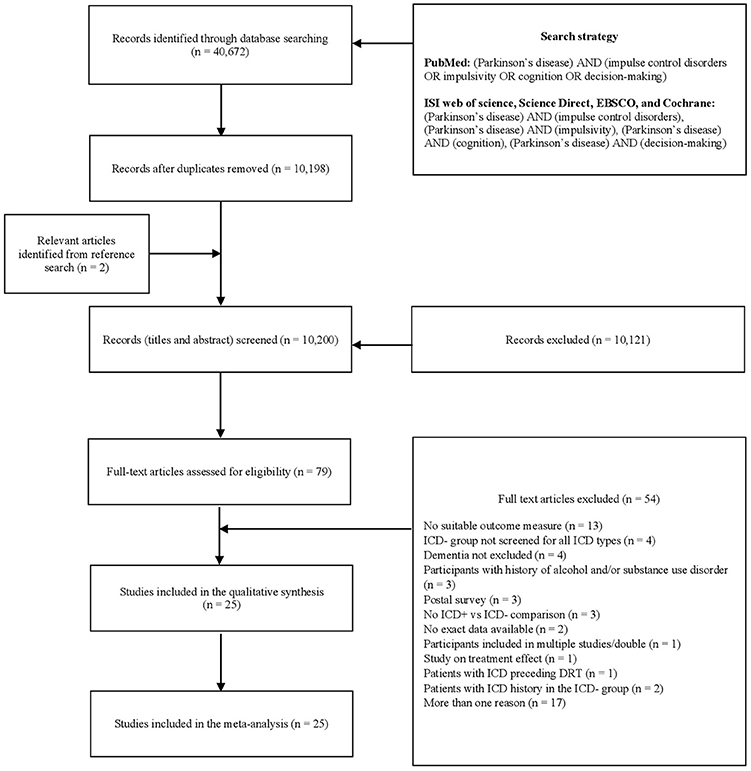
Figure 1. PRISMA diagram of the study (www.prisma-statement.org). DRT, dopaminergic replacement treatment; ICD, impulse control disorder; ICD+, PD patients with ICD; ICD–, PD patients without ICD; PD, Parkinson's disease.
Corresponding authors of five studies were contacted for exact data. Means and standard deviations were obtained for two studies, which reported median and interquartile ranges (20, 25), according to a proposed formula (43). Two reviewers (AM, DDL) independently extracted the following data: sample size, age at evaluation, age at PD onset, PD duration, education (years), Hoehn and Yahr (H and Y) stage, Unified Parkinson's Disease Rating Scale motor section (UPDRS-III) ON-medication, depression, antidepressants use, antipsychotics use, total levodopa equivalent daily dose (LEDD, mg), levodopa LEDD, dopamine agonist LEDD, outcomes, ICD screening tool, ICD type, and statistics.
Primary outcomes were cognitive, affective, and motivational scores. Cognitive tests were categorized on the basis of the main cognitive process involved (44). The categories were “memory”(short-term verbal and visuospatial memory, long-term verbal and visuospatial memory); “working memory”; “attention”; “executive function” (concept formation and reasoning, concept formation sort and shift, set-shifting, inhibition, cognitive flexibility, reward-related decision-making); “visuospatial abilities”; “language”; “apraxia”; “novelty seeking”; “incentive salience” and “data gathering.” Concept formation and reasoning relates to the development of ideas based on the common properties of objects, events, or qualities using abstraction and generalization processes whilst concept formation sort and shift requires to form a sorting principles and apply it (sort), and then abandon it and switch to a different principle (shift) (44).
Affective and motivational measures were categorized as depression, anxiety, anhedonia, apathy, and impulsivity.
Cognitive processes assessed in a single study (i.e., novelty seeking, incentive salience, data gathering, apraxia) were not included in the meta-analysis. When a study reported multiple measures for the same outcome, the most relevant one was chosen by two reviewers with expertise on neuropsychological assessment (AM, DDL).
Data Analysis
Data were analyzed using ReviewManager v5.3 (45). Effect size was estimated as standardized mean difference (SMD), which is comparable to Hedges' adjusted g value. Effect sizes of 0.2, 0.5, and 0.8 or more are considered as small, moderate and large, respectively (46). Cochran's Q (χ2) was used to test heterogeneity between studies. The degree of heterogeneity was quantified by I2, which values range between 0 and 100%. I2 percentages of 25, 50, 75 are considered as low, moderate and high, respectively (47). Random-effect model was applied, as patients differ in clinical (e.g., UPDRS-III ON medication range: 10.9–36.7) and demographic characteristics (e.g., age range: 54.6–71.4), therefore the true effect may vary from study to study. In contrast to fixed-effect models, random-effect models consider both within and between study variances. As heterogeneity was moderate to high for some outcomes (i.e., working memory, depression, anxiety, and apathy), the consequences of applying a fixed-effect model, which does not consider between studies variance, may result in type I error rate inflation (48). Conversely, if random-effect models are applied with effect sizes that vary only due to sampling error as when heterogeneity is low (i.e., short-term visuospatial memory, attention, concept formation reasoning, anhedonia), the consequences are less dramatic (e.g., using Hedges' method, the additional between-study effect size variance used in the random effect method becomes zero when sample effect sizes are homogeneous, yielding the same result as the fixed effect method) (48). Moreover, following this approach, studies were not excluded because of their small sample size, because in random-effect models effect sizes are weighed by their variance, which is higher in smaller studies.
Two authors independently explored funnel plots for publication bias (AM, DDL), and incongruences were resolved by discussion with two other authors (ST, JAG). Funnel plots of outcomes with less than ten studies were not inspected since the power is too low to discriminate publication bias's asymmetry from chance (49). Blinding of assessors (performance bias) and incomplete data outcome (attrition bias) were independently assessed for each study as “low risk,” “high risk,” or “unclear” by two reviewers (AM, DDL) following Cochrane Collaboration recommendations. Sensitivity analysis was performed by excluding one study at time and verifying its impact on the overall effect size. Sensitivity analysis was not performed for outcomes with two studies. Moderator analysis via meta-regression was performed using SPSS version 21.0 (50). We tested the hypothesis that variation among studies in effect size was associated with differences in age, years of education, disease duration, UPDRS-III score, H and Y score, total LEDD, levodopa LEDD, and dopamine agonist LEDD. As suggested by Borenstein (51), moderator analysis was conducted only for outcomes in which there were at least 10 studies to one covariate.
Results
After removal of duplicates, 10,200 records were screened by title and abstract, 79 full-text articles were assessed for eligibility, and 54 were excluded (Figure 1). Twenty-five studies were included in the meta-analysis (Table 1).
Four studies investigated cognitive performance without affective and motivational outcomes (8, 9, 16, 23), 17 studies included both cognitive, affective and motivational outcomes (3–6); (10, 11, 17–22); (27, 30–32, 50), and four studies included affective and motivational data only (25, 26, 28, 29). Three studies divided ICD+ in two groups: PD patients with pathological gambling and those with ICD other than pathological gambling (16, 27, 32), and one study divided the ICD+ in multiple and single ICD groups (26). As the comparison between ICD subtypes was not relevant in our meta-analysis, sub-groups were merged by calculating the pooled means and standard deviations. In one study (6) ICD+ group was divided in pathological gambling, binge-eating, hypersexuality and multiple ICD sub-groups. Since seven PD patients belonging to either the pathological gambling or the binge-eating sub-groups developed ICD before DRT initiation, only data from hypersexuality and multiple ICD sub-groups were extracted and merged as described above. Six studies focused on neuroimaging outcomes but also provided affective (26) and cognitive measures (3–5); (23, 30). One study retrospectively investigated persistent, remitting, and new-onset ICD before and after subthalamic nucleus DBS (STN-DBS) (42). For this study, only pre-STN-DBS data of persistent and never experienced ICD were included in the meta-analysis. Despite the fact that dementia was not explicitly excluded (42), data were included because STN-DBS is performed in non-demented patients only.
The meta-analysis includes 1,563 subjects. The ICD+ group was composed of 625 patients (mean age range: 54.6–68.7 years; mean PD duration: 2.4–14.3 years; mean H and Y: 1.3–2.8; mean UPDRS-III score ON medication: 10.9–36.7). The ICD– group included 938 patients (mean age: 55–71.4 years; mean PD duration: 2.3–13.1 years; mean H and Y stage: 1.4–2.5; mean UPDRS-III score ON medication: 11.7–32.3).
Fourteen meta-analyses were performed to compare cognitive outcomes and five to compare affective and motivational measures in ICD+ compared to ICD– groups.
The following cognitive outcomes were explored: short-term verbal and visuospatial memory, long-term verbal and visuospatial memory, working memory, attention, set-shifting, concept formation (reasoning, sort and shift), inhibition, cognitive flexibility, reward-related decision-making, visuospatial abilities, and language (Table 2).
ICD+ showed worse performance in set-shifting (SMD = −0.49; 95% CI: −0.78, −0.21; Z = 3.37; p = 0.0008) and reward-related decision-making (SMD = 0.42; 95% CI: 0.02, 0.82; Z = 2.05; p = 0.04). The heterogeneity was low-to-moderate for set-shifting (χ2 = 9.32, p = 0.16, I2 = 36%) and moderate for reward-related decision-making (χ2 = 15.50, p = 0.03, I2 = 55%). Effect sizes for the other cognitive outcomes did not differ significantly between groups. Heterogeneity was low for short-term visuospatial memory, attention, concept formation (reasoning), moderate for cognitive flexibility, concept formation (sort and shift), and language, high for short-term verbal memory, long-term verbal memory, long-term visuospatial memory, visuospatial abilities, and inhibition, moderate-to-high for working memory (Figures 2–6).
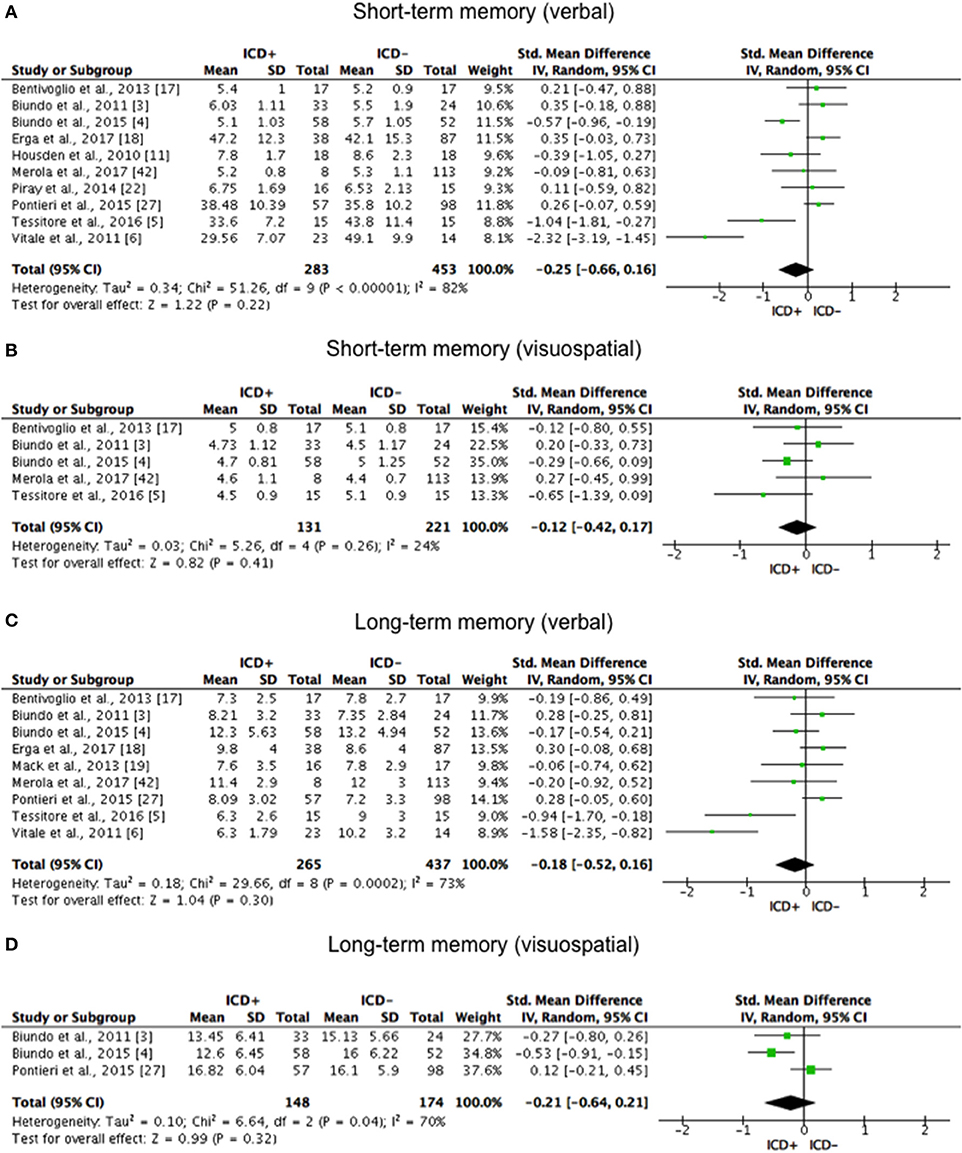
Figure 2. Forest plots for memory. Here are reported forest plots for short-term (verbal, (A) visuospatial, (B) and long-term (verbal, (C); visuospatial, (D) memory outcomes. Standardized mean difference represents Hedges's g effect size. The size of the square indicates the weight of the study. The horizontal line represents the 95% confidence interval. The diamond represents the pooled effect size. Negative effect sizes indicate worse performance in PD patients with ICD (ICD+) in comparison to those without ICD (ICD–). ICD, impulse control disorder; PD, Parkinson's disease.
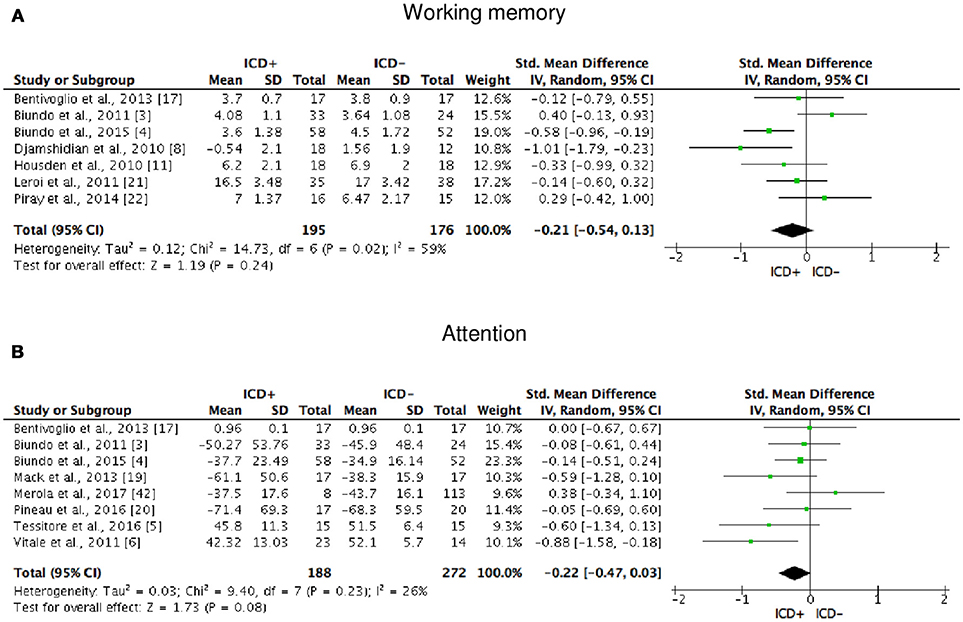
Figure 3. Forest plots for working memory and attention. Here are reported forest plots for working memory (A) and attention (B). Standardized mean difference represents Hedges's g effect size. The size of the square indicates the weight of the study. The horizontal line represents the 95% confidence interval. The diamond represents the pooled effect size. Negative effect sizes indicate worse performance in PD patients with ICD (ICD+) in comparison to those without ICD (ICD−). ICD, impulse control disorder; PD, Parkinson's disease.
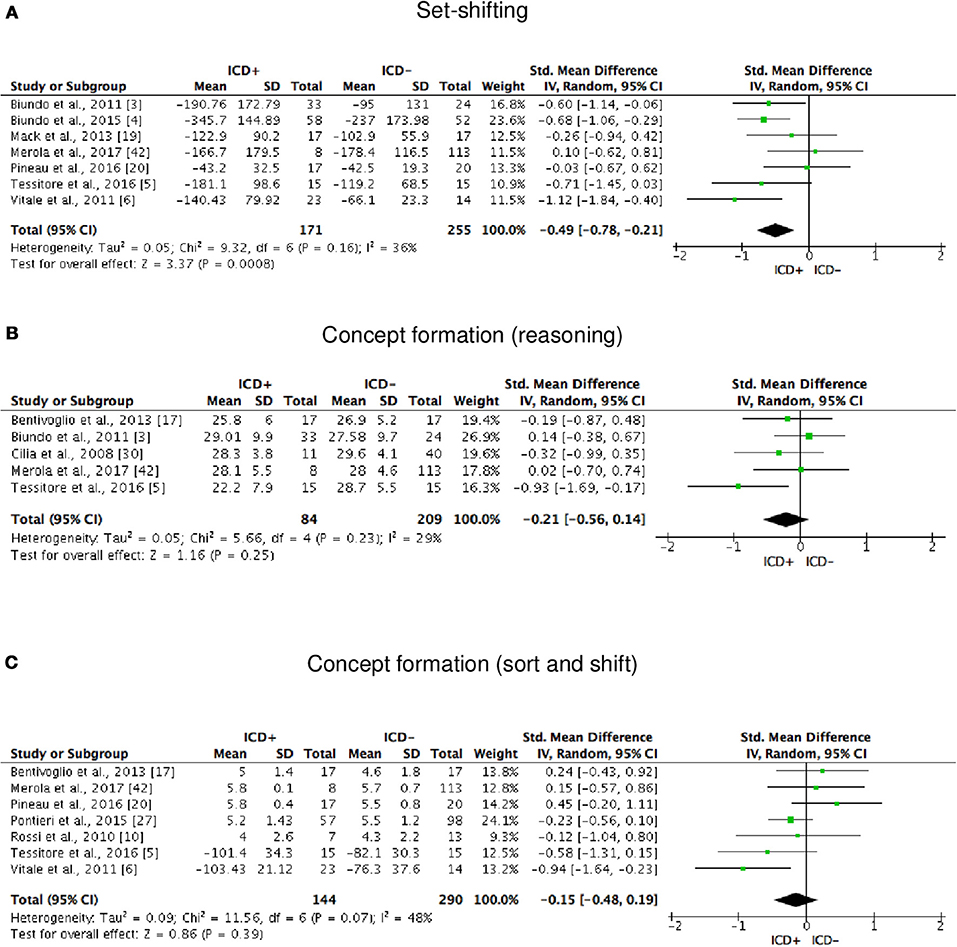
Figure 4. Forest plots for executive functions set-shifting and concept formation. Here are reported forest plots for set-shifting (A), and concept formation (reasoning, B; sort and shift, C).
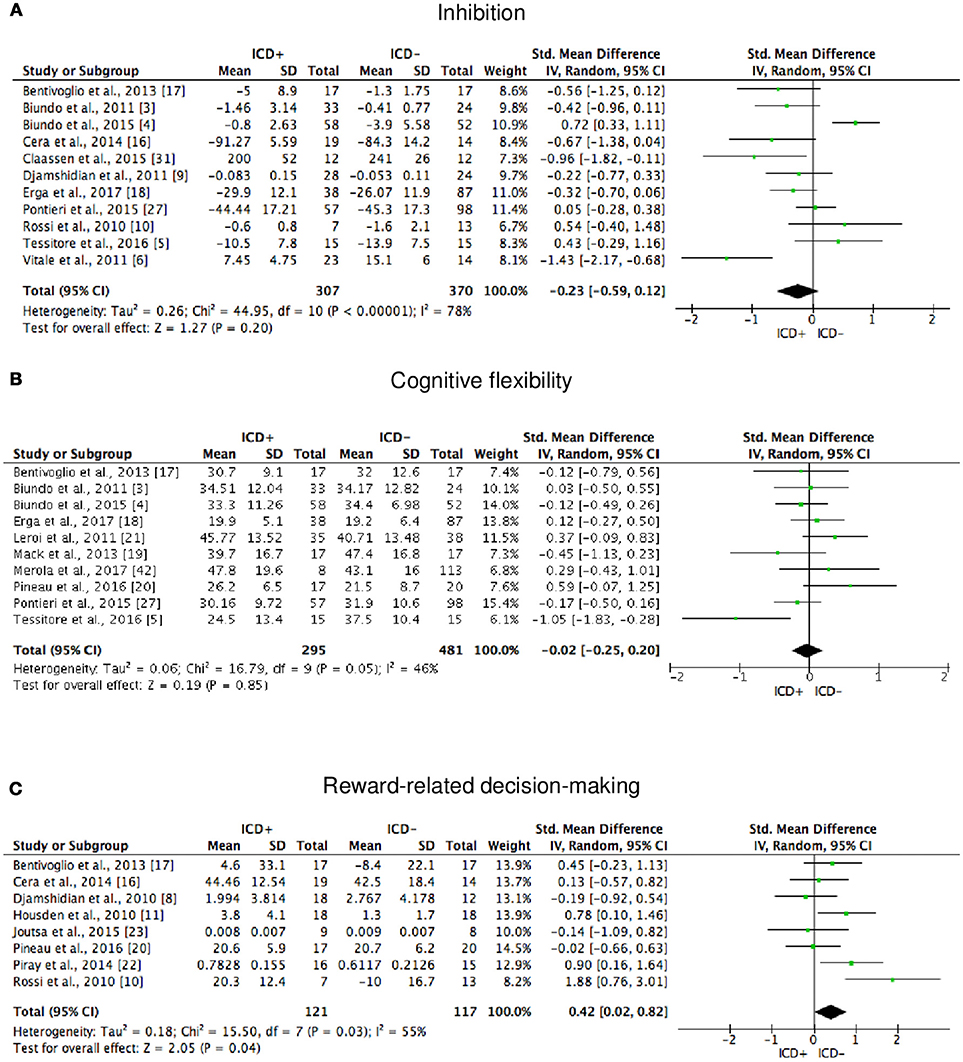
Figure 5. Forest plots for executive functions inhibition, cognitive flexibility, and reward-related decision-making. Here are reported forest plots for inhibition (A), cognitive flexibility (B), and reward-related decision-making (C). Standardized mean difference represents Hedges's g effect size. The size of the square indicates the weight of the study. The horizontal line represents the 95% confidence interval. The diamond represents the pooled effect size. Negative effect sizes indicate worse performance in PD patients with ICD (ICD+) in comparison to those without ICD (ICD–). ICD, impulse control disorder; PD, Parkinson's disease.
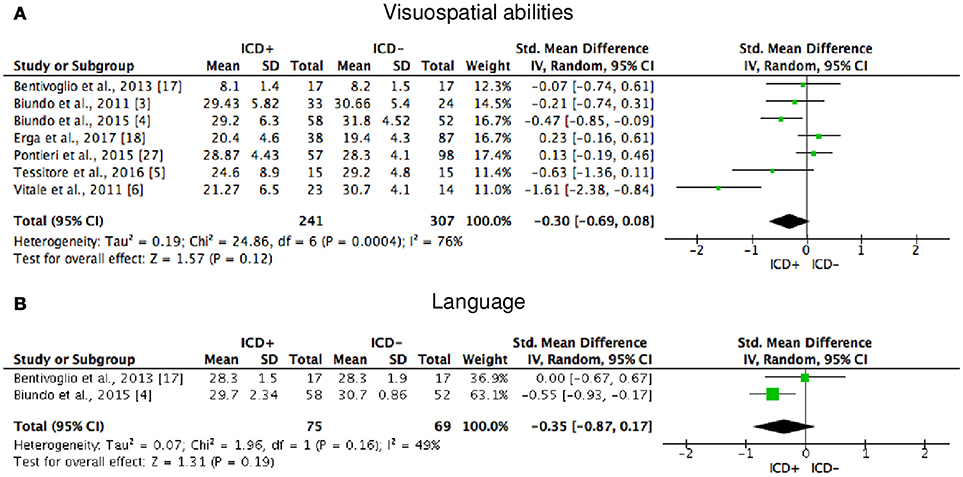
Figure 6. Forest plots for visuospatial abilities and language. Here are reported forest plots for visuospatial abilities (A) and language (B). Standardized mean difference represents Hedges's g effect size. The size of the square indicates the weight of the study. The horizontal line represents the 95% confidence interval. The diamond represents the pooled effect size. Negative effect sizes indicate worse performance in PD patients with ICD (ICD+) in comparison to those without ICD (ICD−). ICD, impulse control disorder; PD, Parkinson's disease.
The following self-reported affective and behavior outcomes were explored: depression, anxiety, anhedonia, apathy, and impulsivity. ICD+ showed increased depression (SMD = 0.35; 95% CI: 0.16, 0.54; Z = 3.54; p = 0.0004), anxiety (SMD = 0.43; 95% CI: 0.18, 0.68; Z = 3.39; p = 0.0007), anhedonia (SMD = 0.26; 95% CI: 0.01, 0.50; Z = 2.01; p = 0.04), and impulsivity (SMD = 0.79; 95% CI: 0.50, 1.09; Z = 5.26; p < 0.00001), but comparable apathy symptoms (Figure 7). Heterogeneity was low for anhedonia (χ2 = 0.01, p = 0.94, I2 = 0%), moderate for impulsivity (χ2 = 8.89, p = 0.11, I2 = 44%), and moderate-to-high for depression (χ2 = 51.42, p = 0.0001, I2 = 61%), anxiety (χ2 = 21.27, p = 0.01, I2 = 58%), and apathy (χ2 = 9.09, p = 0.03, I2 = 67%; Figure 7). Results of the meta-analyses are summarized in Table 3.
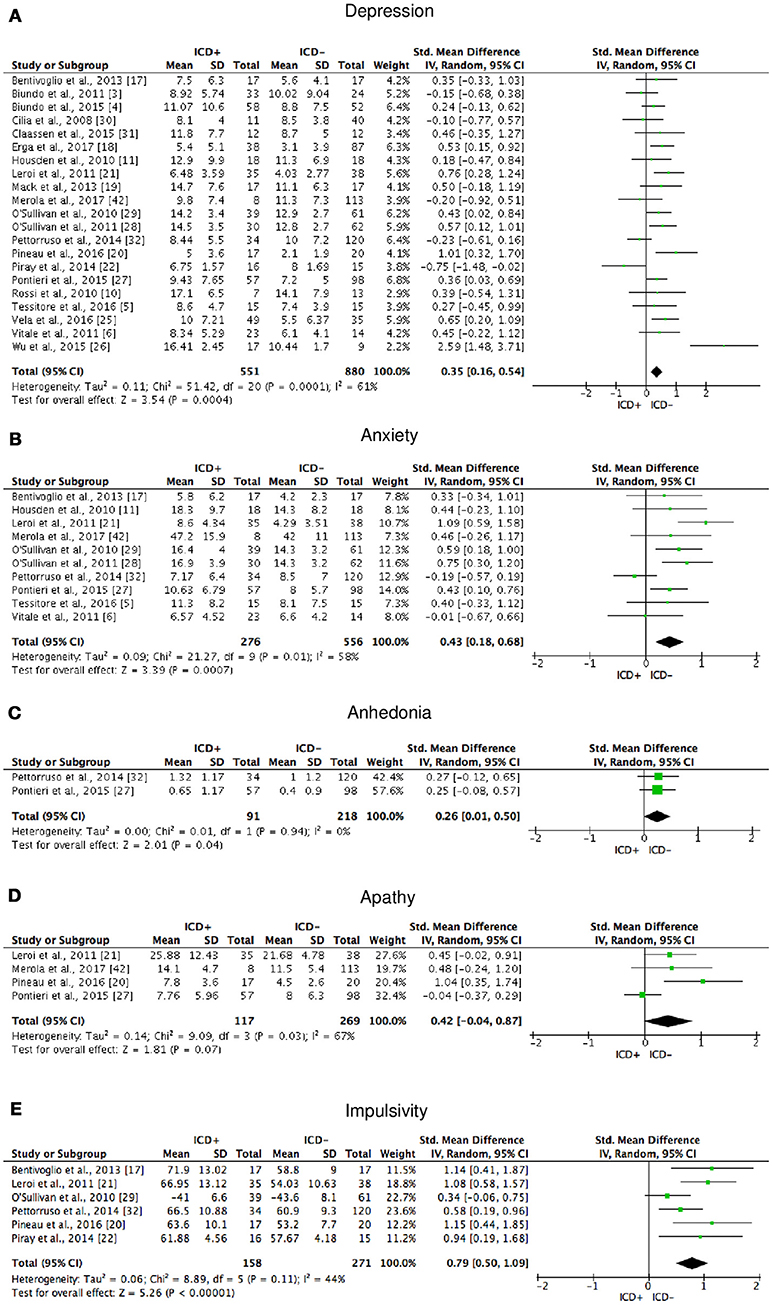
Figure 7. Forest plots for affective and motivational outcomes. Here are reported forest plots for depression (A), anxiety (B), anhedonia (C), apathy (D), and impulsivity (E). Standardized mean difference represents Hedges's g effect size. The size of the square indicates the weight of the study. The horizontal line represents the 95% confidence interval. The diamond represents the pooled effect size. Negative effect sizes indicate worse performance in PD patients with ICD (ICD+) in comparison to those without ICD (ICD−). ICD, impulse control disorder; PD, Parkinson's disease.
Risk of Bias
Visual exploration of funnel plots did not suggest possible publication bias for short-term verbal memory, inhibition, cognitive flexibility, depression, and anxiety that were the only outcomes with at least 10 studies (Figure 8).
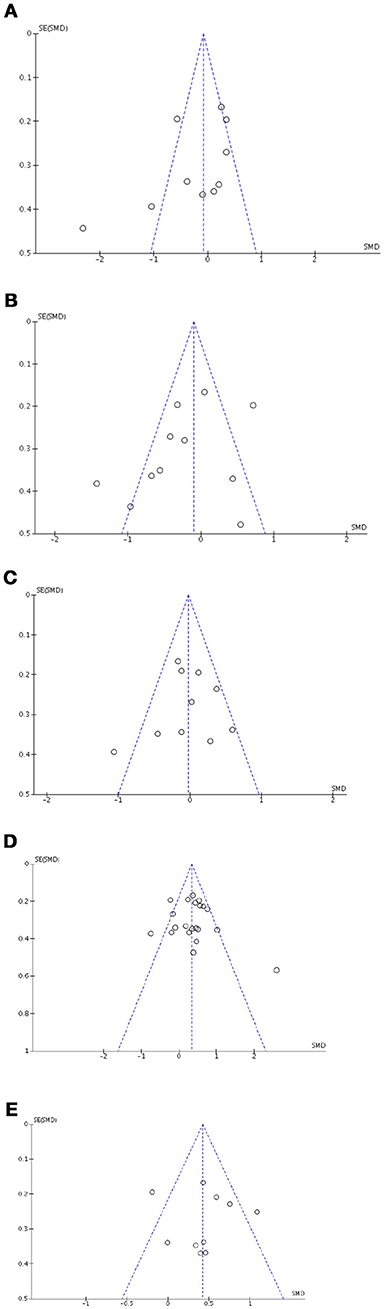
Figure 8. Funnel plots for cognitive, affective and motivational outcomes. Here are reported funnel plots for short-term verbal memory (A), inhibition (B), phonological fluency (C), depression (D), and anxiety (E). There is no evidence to suggest publication bias.
Risk of performance bias was unclear with only 2/25 studies indicating assessors blinding procedures.
Attrition bias was low, with 4/25 studies with missing data.
Sensitivity Analysis and Moderator Analysis
Sensitivity analysis showed that after removing Pontieri et al. (27), the overall effect size of long-term visuospatial memory became significant (SMD = −0.44; 95% CI: −0.75, −0.13; Z = 2.81; p = 0.005) and the heterogeneity changed from high (χ2 = 6.64, p = 0.04, I2 = 70%) to low (χ2 = 0.62, p = 0.43, I2 = 0%). After removing Biundo et al. (3), the overall effect size of working memory became significant (SMD = −0.32; 95% CI: −0.63, −0.01; Z = 2.05; p = 0.04) and the heterogeneity changed from high (χ2 = 14.73, p = 0.02, I2 = 59%) to moderate (χ2 = 8.41, p = 0.13, I2 = 41%). The overall effect size of attention became significant after removing Merola et al. (42) (SMD = −0.27; 95% CI: −0.50, −0.04; Z = 2.29; p = 0.02), but heterogeneity remained low. The overall effect size of inhibition became significant after removing Biundo et al. (4) (SMD = −0.34; 95% CI: −0.65, −0.03; Z = 2.18; p = 0.03) and heterogeneity changed from high to moderate-to-high (χ2 = 24.18, p = 0.004, I2 = 63%). The overall effect size of reward-related decision-making lost significance after removing Bentivoglio et al. (17) (SMD = 0.42; 95% CI: −0.05, 0.89; Z = 1.75; p = 0.08), Housden et al. (11) (SMD = 0.36; 95% CI: −0.08, 0.81; Z = 1.59; p = 0.11), Piray et al. (22) (SMD = 0.35; 95% CI: −0.08, 0.78; Z = 1.58; p = 0.11), and Rossi et al. (10) (SMD = 0.29; 95% CI: −0.03, 0.61; Z = 1.78; p = 0.07). After removing Rossi et al. (10), heterogeneity changed from moderate (χ2 = 15.50, p = 0.03, I2 = 55%) to low (χ2 = 8.27, p = 0.22, I2 = 27%). Including or excluding the other studies did not change heterogeneity. The overall effect size of apathy became significant after removing Pontieri et al. (27) (SMD = 0.60; 95% CI: 0.25, 0.95; Z = 3.38; p = 0.0007) and heterogeneity changed from high (χ2 = 9.09, p = 0.03, I2 = 67%) to low (χ2 = 2.07, p = 0.35, I2 = 4%). Moderator analysis was performed for short-term verbal memory, inhibition, cognitive flexibility, and depression, which were the only outcomes that included at least 10 studies each (51). Anxiety did not undergo moderator analysis, because none of the covariates of interest were assessed in at least 10 studies. Moderator analysis showed no effect of age, education, PD duration, H and Y, UPDRS-III, and total LEDD, levodopa LEDD, dopamine agonist LEDD on short-term verbal memory, inhibition, cognitive flexibility, and depression (Table 4).
Discussion
The primary aim of this meta-analysis of 25 studies was to describe the pattern of cognitive function in DRT-medicated ICD+ compared to ICD–. A stricter set of inclusion criteria was applied than used previously (33), to achieve a more homogenous ICD+ group, and a better understanding of the relationship between ICD and cognition in medicated PD. A secondary aim was to examine affective and motivational correlates of ICD, as emotion-cognition and motivation-cognition relationships are receiving increasing attention to understand psychopathology and improve pharmacological and psychological treatments (34).
Our findings suggest ICD to be associated with worse performance on a set of executive function measures assessing set-shifting (Trail Making Test part B, and B-A) and reward-related decision-making (Iowa Gambling Task, Monetary Risk Task, Kirby Delay Discounting Questionnaire), with relative sparing of other executive tasks that assess concept formation and reasoning (Raven's progressive matrices standard and colored versions), concept formation sort and shift (Wisconsin card sorting test standard and modified versions), inhibition (Stroop, Stop Signal Task, Go/no-Go), and cognitive flexibility (phonological fluency), as well as memory, working memory, attention, visuospatial abilities, and language.
Set-shifting and reward-related decision-making abilities are important determinants of advantageous behavior, serving to translate goals into action planning, as well as monitoring response and errors (52).
Structural and functional neuroimaging outcomes were not included in this meta-analysis, but neuroanatomical findings in patients with abnormalities in set-shifting and reward-related decision-making may help speculate on brain areas that may undergo DRT overdose in PD. Lesion-symptom mapping studies suggest reward-related decision-making to rely upon an anatomical network composed of the ventromedial, orbitofrontal and frontopolar cortices. Set-shifting, which is one of the processes underlying cognitive control, depends on rostral anterior cingulate cortex functioning (52). These brain areas form part of the mesocorticolimbic system that, in the early stages of PD, undergo less dopaminergic damage than the dorsal striatal pathways.
According to the “overdose hypothesis,” the DRT amount required to control motor symptoms in PD has the potential to move the same patient away from the optimum for certain cognitive functions (53). The relationship between the efficiency of neuronal activity and the state of dopaminergic modulation is represented by a Yerkes-Dodson inverted U-shaped curve with cognitive functions declining with deviation away from optimum dopamine levels, indicated by the center of the curve (2). Extrapolating this model to set-shifting and reward-related decision-making implies that DRT has the capacity to both improve and impair these executive functions depending on baseline dopamine levels in the underlying neural circuitry. For patients with low baseline dopamine levels in the mesocorticolimbic system, DRT may optimize activity as supported by improved set-shifting and reward-related decision-making when assessed in an optimally medicated state compared to the same patients assessed following DRT withdrawal (54, 55). By the same token, if patients start out with higher mesocorticolimbic baseline levels of dopamine, DRT causes dopamine over-activity in the mesocorticolimbic system. This view is consistent with evidence that dopamine agonists increase frontal cortex blood flow (56), and enhance reward-related risk-taking behavior in ICD+ compared to ICD– (57).
A recent meta-analysis of case-control studies on the prevalence of ICD in PD provides indirect evidence of dopaminergic over-activity, as being medicated for PD and disease duration were both factors that increased the risk of ICD (58). As disease duration advances, the dopaminergic degeneration spread to brain areas that were spared in the early stages of the disease, such as prefrontal cortex (59). The progressive involvement of brain areas during PD progression may have two consequences. The first is a dysregulation of brain regions involved in the top-down mechanisms of cognitive control of behavior (60). The second is the need to increase DRT dosage to compensate motor symptoms and the consequent overstimulation of less damaged brain areas. However, the relationship between ICD and DRT dosage is not well-established; some studies report no difference between DRT doses and ICD (18, 25, 61, 62), with others reporting an association between ICD and dopamine agonists doses (63–68). In this meta-analysis we lacked the power for conducting moderator analysis for disease duration, total LEDD, LD LEDD, and DA LEDD in reward-related decision-making and set-shifting leaving this question unanswered.
Our data may help reconcile the debate whether ICD in PD is associated with frontal lobe dysfunction (69–72). The discrepancy between previous reports is likely due to differences in the tasks and the underlying executive function subdomains investigated. Our data indicate that some frontal tasks and related subdomains may not be affected by ICD. Therefore, neuropsychological evaluation of ICD+ patients should include a broad range of executive function tasks, encompassing both reward-related decision-making and set-shifting, and not be limited to a general frontal screening test, such as the Frontal Assessment Battery, which does not include those subdomains.
The profile of executive dysfunction we found confirms the conclusions of a previous meta-analysis (33) that also reported reduced abstraction/concept formation and visuospatial abilities in ICD+. The discrepancy between the two meta-analyses can be ascribed to our inclusion of two reports (18, 50) not available at the time of the former one, and by our stricter exclusion criteria. We excluded four studies included by Santangelo et al. (7, 14, 58, 59), because of (a) patients with hypersexuality and compulsive shopping included the ICD– group (7), (b) dementia not excluded (14), and (c) patients screened for pathological gambling (73) or punding (74) only, thereby the presence of other ICDs in the ICD– group could not be ruled out.
Our secondary aim was to explore affective and motivational outcomes associated with ICD, as evidence indicates a role for dopamine dysregulation in the pathophysiology of impulsivity, apathy, and anhedonia in pathological gambling, drug addiction, and ICD+ (75–77). We found increased rates of self-reported depression, anxiety, anhedonia, and impulsivity, but not apathy in ICD+ compared to ICD–.
Impulsivity and apathy have been suggested to represent opposite ends of a dopaminergic continuum, where the former and the latter are associated with hyper and hypodopaminergic state, respectively (75). According to this view, DRT mesocorticolimbic overstimulation increases impulsivity that, in turn, may enhance reward-related behavior that, over time, may become addictive in nature (78). The association between ICD+ and impulsivity but not apathy in our meta-analysis is consistent with this model and the evidence that the D2 dopamine agonist pramipexole improves apathy in PD patients without ICD (79) but also increases impulsivity (1).
Anhedonia is defined as the decreased ability to experience pleasure from positive stimuli (80). Pramipexole may reduce anhedonia in ICD–, suggesting its hypodopaminergic nature (81).
The co-occurrence of hypodopaminergic anhedonia with hyperdopaminergic ICD is surprising. One possible explanation is that ICD+ patients may have decreased ability to experience pleasure when not engaged in ICD. This hypothesis is supported by the evidence that people addicted to alcohol or drugs experience anhedonia during withdrawal syndrome, a feature that may facilitate relapse (82). However, the relationship between anhedonia and dopaminergic states is not so straightforward and anhedonia is also recognized as one of the overlapping symptoms between apathy and depression (83). The association with anhedonia may be confounded by the presence of depression, which in some cases might be serotoninergically mediated (84). However, there are only two studies and further investigation is needed.
The pathophysiology of depression and anxiety in PD is likely to be multifactorial including reaction to disease diagnosis and anxiety about its future course. Depression and anxiety are present in the premorbid PD stage (85), therefore suggesting they may represent a core feature of PD. In our meta-analysis depression and anxiety levels were higher in ICD+ compared to ICD–. ICD may have a negative impact on the quality of life (21, 25), and in turn increase depression and anxiety levels. Also, as the mesocorticolimbic pathways dysfunction may be involved in depression, anxiety and ICD, they might co-occur as epiphenomena of shared neural correlates (40).
The main limitation of this meta-analysis is the small number of studies, most of which with small samples that might have contributed to high heterogeneity for some of the outcomes explored. This consideration could be reflected in the sensitivity analysis data for long-term visuospatial memory, working memory, attention, inhibition, reward-related decision-making, apathy, and it suggests caution in the interpretation of the results for these outcomes. Moreover, the inclusion in the same domains of tasks that might involve different cognitive processes could have contributed to the high heterogeneity and the low stability of some results. However, considering the single cognitive task would have resulted in a reduction of the power, because of the low number of studies using the same tasks. Unfortunately, we were not able to perform separate analyses for dopamine agonists and levodopa, as the majority of the studies included patients who were under both types of DRT. Due to the small number of studies, moderator analysis for levodopa and dopamine agonist LEDD was performed for depression only, which showed no effect. This is not surprising, as in the larger study published so far, ICDs were found to be associated either with dopamine agonists or, to a lesser extent, with levodopa (1). These data are in keeping with the notion that both levodopa and dopamine agonists can interfere with the phasic and tonic activity of dopaminergic neurons (86) that, by facilitating neuroadaptive changes in dopaminergic system functioning, may predispose to ICD.
Another limitation is the inclusion of cross-sectional studies that impede the exploration of the direction of the cause-effect relationship between cognitive, affective and motivational outcomes and ICD; therefore multi-center and longitudinal studies are needed. Moreover, even if we excluded studies focusing on punding and dopamine dysregulation syndrome only, these conditions were present in many studies, and probably contributed to high heterogeneity for some outcomes. Furthermore, 23/25 studies did not mention assessors to be blind to the ICD status and this might have affected tools administration and scoring. Future studies should be conducted following blinding procedures. Finally, QUIP, a validated screening instrument with high sensitivity (94%) but low specificity (72%) to ICD in PD (87) was used in two studies (18, 25), possibly leading to false positive and/or subclinical ICD inclusion. Still unanswered questions include whether set-shifting and reward-related decision-making abnormalities in PD patients with ICD reflect structural and functional mesocorticolimbic changes due to acute or chronic DRT effects, or whether they can revert following ICD treatment and remission. Future studies should address these points, since better understanding ICD pathophysiology may help tailoring treatment of ICD+.
Author Contributions
The study has been designed by AM, DD, NE, and ST. Data have been gathered and analyzed by AM and DD under the supervision of JG. The manuscript has been drafted by AM, NE, and ST. AM, DD, NE, JG, and ST revised the manuscript.
Conflict of Interest Statement
The authors declare that the research was conducted in the absence of any commercial or financial relationships that could be construed as a potential conflict of interest.
Acknowledgments
The work has been supported by a PhD scholarship from Keele University.
Abbreviations
DBS, deep brain stimulation; DRT, dopamine replacement treatment; H and Y, Hoehn and Yahr scale; ICD, impulse control disorder; LEDD, levodopa equivalent daily dose; PD, Parkinson's disease; QUIP, Questionnaire for Impulsive-Compulsive Disorders in Parkinson's Disease; SDM, standardized mean difference; STN-DBS, sub thalamic nucleus deep brain stimulation; UPRDS, Unified Parkinson's Disease Rating Scale.
References
1. Weintraub D, Koester J, Potenza MN, Siderowf AD, Stacy M, Voon V, et al. Impulse control disorders in parkinson disease: a cross-sectional study of 3090 patients. Arch Neurol. (2010) 67:589–95. doi: 10.1001/archneurol.2010.65
2. Cools R, Robbins TW. Chemistry of the adaptive mind. Philos Trans A Math Phys Eng Sci. (2004) 362:2871–88. doi: 10.1098/rsta.2004.1468
3. Biundo R, Formento-Dojot P, Facchini S, Vallelunga A, Ghezzo L, Foscolo L, et al. Brain volume changes in Parkinson's disease and their relationship with cognitive and behavioural abnormalities. J Neurol Sci. (2011) 310:64–9. doi: 10.1016/j.jns.2011.08.001
4. Biundo R, Weis L, Facchini S, Formento-Dojot P, Vallelunga A, Pilleri M, et al. Patterns of cortical thickness associated with impulse control disorders in Parkinson's disease. Mov Disord. (2015) 30:688–95. doi: 10.1002/mds.26154
5. Tessitore A, Santangelo G, De Micco R, Vitale C, Giordano A, Raimo S, et al. Cortical thickness changes in patients with Parkinson's disease and impulse control disorders. Parkinsonism Relat Disord. (2016) 24:119–25. doi: 10.1016/j.parkreldis.2015.10.013
6. Vitale C, Santangelo G, Trojano L, Verde F, Rocco M, Grossi D, et al. Comparative neuropsychological profile of pathological gambling, hypersexuality, and compulsive eating in Parkinson's disease. Mov Disord. (2011) 26:830–6. doi: 10.1002/mds.23567
7. Santangelo G, Vitale C, Trojano L, Verde F, Grossi D, Barone P. Cognitive dysfunctions and Pathological gambling in patients with Parkinson's disease. Mov Disord. (2009) 24:899–905. doi: 10.1002/mds.22472
8. Djamshidian A, Jha A, O'Sullivan SS, Silveira-Moriyama L, Jacobson C, Brown P, et al. Risk and learning in impulsive and non-impulsive patients with Parkinson's disease. Mov Disord. (2010) 25:2203–10. doi: 10.1002/mds.23247
9. Djamshidian A, O'Sullivan SS, Lees A, Averbeck BB. Stroop test performance in impulsive and non impulsive patients with Parkinson's disease. Parkinsonism Relat Disord. (2011) 17:212–4. doi: 10.1016/j.parkreldis.2010.12.014
10. Rossi M, Gerschcovich ER, De Achaval D, Perez-Lloret S, Cerquetti D, Cammarota A, et al. Decision-making in Parkinson's disease patients with and without pathological gambling. Eur J Neurol. (2010) 17:97–102. doi: 10.1111/j.1468-1331.2009.02792.x
11. Housden CR, O'Sullivan SS, Joyce EM, Lees AJ, Roiser JP. Intact reward learning but elevated delay discounting in Parkinson's disease patients with impulsive-compulsive spectrum behaviors. Neuropsychopharmacology (2010) 35:2155–64. doi: 10.1038/npp.2010.84
12. Voon V, Gao J, Brezing C, Symmonds M, Ekanayake V, Fernandez H, et al. Dopamine agonists and risk: Impulse control disorders in Parkinson's Disease. Brain (2011) 134:1438–46. doi: 10.1093/brain/awr080
13. Voon V, Reynolds B, Brezing C, Gallea C, Skaljic M, Ekanayake V, et al. Impulsive choice and response in dopamine agonist-related impulse control behaviors. Psychopharmacology (2010) 207:645–59. doi: 10.1007/s00213-009-1697-y
14. Leroi I, Barraclough M, McKie S, Hinvest N, Evans J, Elliott R, et al. Dopaminergic influences on executive function and impulsive behaviour in impulse control disorders in Parkinson's disease. J Neuropsychol. (2013); 7:306–25. doi: 10.1111/jnp.12026
15. Martini A, Ellis SJ, Grange JA, Tamburin S, Dal Lago D, Vianello G, et al. Risky decision-making and affective features of impulse control disorders in Parkinson's disease. J Neural Transm. (2018) 125:131–43. doi: 10.1007/s00702-017-1807-7
16. Cera N, Bifolchetti S, Martinotti G, Gambi F, Sepede G, Onofrj M, et al. Amantadine and cognitive flexibility: decision making in Parkinson's patients with severe pathological gambling and other impulse control disorders. Neuropsychiatr Dis Treat. (2014) 10:1093–101. doi: 10.2147/NDT.S54423
17. Bentivoglio AR, Baldonero E, Ricciardi L, De Nigris F, Daniele A. Neuropsychological features of patients with Parkinson's disease and impulse control disorders. Neurol Sci. (2013) 34:1207–13. doi: 10.1007/s10072-012-1224-5
18. Erga AH, Alves G, Larsen JP, Tysnes OB, Pedersen KF. Impulsive and compulsive behaviors in Parkinson disease: the Norwegian ParkWest study. J Parkinsons Dis. (2017) 7:183–91. doi: 10.3233/JPD-160977
19. Mack J, Okai D, Brown RG, Askey-Jones S, Chaudhuri KR, Martin A, et al. The role of self-awareness and cognitive dysfunction in Parkinson's disease with and without impulse-control disorder. J Neuropsychiatry Clin Neurosci. (2013) 25:141–9. doi: 10.1176/appi.neuropsych.12030076
20. Pineau F, Roze E, Lacomblez L, Bonnet AM, Vidailhet M, Czernecki V, et al. Executive functioning and risk-taking behavior in Parkinson's disease patients with impulse control disorders. J Neural Transm. (2016) 123:573–81. doi: 10.1007/s00702-016-1549-y
21. Leroi I, Ahearn DJ, Andrews M, McDonald KR, Byrne EJ, Burns A. Behavioural disorders, disability and quality of life in Parkinson's disease. Age Ageing (2011) 40:614–621. doi: 10.1093/ageing/afr078
22. Piray P, Zeighami Y, Bahrami F, Eissa AM, Hewedi DH, Moustafa AA. Impulse control disorders in Parkinson's disease are associated with dysfunction in stimulus valuation but not action valuation. J Neurosci. (2014) 34:7814–24. doi: 10.1523/JNEUROSCI.4063-13.2014
23. Joutsa J, Voon V, Johansson J, Niemelä S, Bergman J, Kaasinen V, et al. Dopaminergic function and intertemporal choice. Transl Psychiatry (2015) 5:e491. doi: 10.1038/tp.2014.133
24. Siri C, Cilia R, Gaspari D, Canesi M, Meucci N, Zecchinelli AL, et al. Cognitive status of patients with Parkinson's disease and pathological gambling. J Neurol. (2010) 257:247–52. doi: 10.1007/s00415-009-5301-5
25. Vela L, Martínez Castrillo JC, García Ruiz P, Gasca-Salas C, Macías Macías Y, Pérez Fernández E, et al. The high prevalence of impulse control behaviors in patients with early-onset Parkinson's disease: A cross-sectional multicenter study. J Neurol Sci. (2016) 368:150–4. doi: 10.1016/j.jns.2016.07.003
26. Wu K, Politis M, O'Sullivan SS, Lawrence AD, Warsi S, Bose S, et al. Single versus multiple impulse control disorders in Parkinson's disease: an (11)C-raclopride positron emission tomography study of reward cue-evoked striatal dopamine release. J Neurol. (2015) 262:1504–14. doi: 10.1007/s00415-015-7722-7
27. Pontieri FE, Assogna F, Pellicano C, Cacciari C, Pannunzi S, Morrone A, et al. Sociodemographic, neuropsychiatric and cognitive characteristics of pathological gambling and impulse control disorders NOS in Parkinson's disease. Eur Neuropsychopharmacol. (2015) 25:69–76. doi: 10.1016/j.euroneuro.2014.11.006
28. O'Sullivan S, Loane CM, Lawrence AD, Evans AH, Piccini P, Lees AJ, et al. Sleep disturbance and impulsive-compulsive behaviours in Parkinson's disease. J Neurol Neurosurg Psychiatry (2011) 82:620–2. doi: 10.1136/jnnp.2009.186874
29. O'Sullivan, Djamshidian A, Evans AH, Loane CM, Lees AJ, Lawrence AD. Excessive hoarding in Parkinson's disease. Mov Disord. (2010) 25:1026–33. doi: 10.1002/mds.23016
30. Cilia R, Siri C, Marotta G, Isaias IU, De Gaspari D, Canesi M, et al. Functional abnormalities underlying pathological gambling in Parkinson disease. Arch Neurol. (2008) 65:1604–11. doi: 10.1001/archneur.65.12.1604
31. Claassen DO, van den Wildenberg WP, Harrison MB, van Wouwe NC, Kanoff K, Neimat JS, et al. Proficient motor impulse control in Parkinson disease patients with impulsive and compulsive behaviors. Pharmacol Biochem Behav. (2015) 129:19–25. doi: 10.1016/j.pbb.2014.11.017
32. Pettorruso M, Martinotti G, Fasano A, Loria G, Di Nicola M, De Risio L, et al. Anhedonia in Parkinson's disease patients with and without pathological gambling: a case-control study. Psychiatry Res. (2014) 215:448–52. doi: 10.1016/j.psychres.2013.12.013
33. Santangelo G, Raimo S, Barone P. The relationship between Impulse Control Disorders and cognitive dysfunctions in Parkinson's Disease: a meta-analysis. Neurosci Biobehav Rev. (2017) 77:129–47. doi: 10.1016/j.neubiorev.2017.02.018
34. Crocker LD, Heller W, Warren SL, O'Hare AJ, Infantolino ZP, Miller GA, et al. Relationships among cognition, emotion, and motivation: implications for intervention and neuroplasticity in psychopathology. Front Hum Neurosci. (2013) 7:261. doi: 10.3389/fnhum.2013.00261
35. Seedat S, Kesler S, Niehaus DJ, Stein DJ. Pathological gambling behaviour: Emergence secondary to treatment of Parkinson's disease with dopaminergic agents. Depress Anxiety (2000) 11:185–6. doi: 10.1002/1520-6394(2000)11:4<185::AID-DA8>3.0.CO;2-H
36. Callesen MB, Scheel-Krüger J, Kringelbach ML, Møller A. A systematic review of impulse control disorders in Parkinson's disease. J Parkinsons Dis. (2013) 3:105–38. doi: 10.3233/JPD-120165
37. Samuel M, Rodriguez-Oroz M, Antonini A, Brotchie JM, Ray Chaudhuri K, Brown RG, et al. Management of impulse control disorders in Parkinson's disease: Controversies and future approaches. Mov Disord. (2015) 30:150–9. doi: 10.1002/mds.26099
38. Combs HL, Folley BS, Berry DT, Segerstrom SC, Han DY, Anderson-Mooney AJ, et al. Cognition and depression following deep brain stimulation of the subthalamic nucleus and globus pallidus pars internus in Parkinson's disease: a meta-analysis. Neuropsychol Rev. (2015) 25:439–54. doi: 10.1007/s11065-015-9302-0
39. Hoops S, Nazem S, Siderowf AD, Duda JE, Xie SX, Stern MB, et al. Validity of the MoCA and MMSE in the detection of MCI and dementia in Parkinson disease. Neurology (2009) 73:1738–45. doi: 10.1212/WNL.0b013e3181c34b47
40. Vriend C, Pattij T, van der Werf YD, Voorn P4, Booij J, Rutten S, et al. Depression and impulse control disorders in Parkinson's disease: two sides of the same coin? Neurosci Biobehav Rev. (2014) 38:60–71. doi: 10.1016/j.neubiorev.2013.11.001
41. Ouzzani M, Hammady H, Fedorowicz Z, Elmagarmid A. Rayyan—a web and mobile app for systematic reviews. Syst Rev. (2016) 5:210. doi: 10.1186/s13643-016-0384-4
42. Merola A, Romagnolo A, Rizzi L, Rizzone MG, Zibetti M2, Lanotte M, et al. Impulse control behaviors and subthalamic deep brain stimulation in Parkinson disease. J Neurol. (2017) 264:40–8. doi: 10.1007/s00415-016-8314-x
43. Hozo SP, Djulbegovic B, Hozo I. Estimating the mean and variance from the median, range, and the size of a sample. BMC Med Res Methodol. (2005) 5:13. doi: 10.1186/1471-2288-5-13
44. Lezak MD, Howieson DB, Bigler ED, Tranel D. Neuropsychological Assessment 5th ed. New York, NY: Oxford University Press (2012).
46. Cohen J. Statistical Power Analysis for the Behavioral Sciences 2nd ed. Hillsdale, NJ: Lawrence Erlbaum Associates (1977) 567.
47. Higgins JPT, Thompson SG, Deeks JJ, Altman DG. Measuring inconsistency in meta-analyses. Br Med J. (2003) 327:557–60. doi: 10.1136/bmj.327.7414.557
48. Field A, Gillet R. How to do a meta-analysis. Br J Math Stat Psychol. (2010) 63(Pt 3):665–94. doi: 10.1348/000711010X502733
49. Sterne JAC, Sutton AJ, Ioannidis JP, Terrin N, Jones DR, Lau J, et al. Recommendations for examining and interpreting funnel plot asymmetry in meta-analyses of randomised controlled trials. BMJ (2011) 343:d4002. doi: 10.1136/bmj.d4002
51. Borenstein M. Effect sizes for continuous data. In: Cooper H, Hedges LV, Valentine JC, editors. The Handbook of Research Synthesis and Meta-Analysis New York, NY: Russell Sage Foundation (2009). p. 221–35.
52. Gläscher J, Adolphs R, Damasio H, Bechara A, Rudrauf D, Calamia M, et al. Lesion mapping of cognitive control and value-based decision making in the prefrontal cortex. Proc Natl Acad Sci USA. (2012) 109:14681–6. doi: 10.1073/pnas.1206608109
53. Rowe JB, Hughes L, Ghosh BC, Eckstein D, Williams-Gray CH, Fallon S, et al. Parkinson's disease and dopaminergic therapy-differential effects on movement, reward and cognition. Brain (2008) 131:2094–105. doi: 10.1093/brain/awn112
54. Boller JK, Barbe MT, Pauls KA, Reck C, Brand M, Maier F, et al. Decision-making under risk is improved by both dopaminergic medication and subthalamic stimulation in Parkinson's disease. Exp Neurol. (2014) 254:70–7. doi: 10.1016/j.expneurol.2014.01.005
55. Cools R, Barker RA, Sahakian BJ, Robbins TW. Enhanced or imapired cognitive function in Parkinson's Disease as a function of dopaminergic medication and task demands. Cereb Cortex (2001) 11:1136–43. doi: 10.1093/cercor/11.12.1136
56. Claassen DO, Stark AJ, Spears CA, Petersen KJ, van Wouwe NC, Kessler RM, et al. Mesocorticolimbic hemodynamic response in Parkinson's disease patients with compulsive behaviors. Mov Disord. (2017) 32:1574–83. doi: 10.1002/mds.27047
57. Claassen DO, van den Wildenberg WP, Ridderinkhof KR, Jessup CK, Harrison MB, Wooten GF, et al. The risky business of dopamine agonists in Parkinson disease and impulse control disorders. Behav Neurosci. (2011) 125: 492–500. doi: 10.1037/a0023795
58. Molde H, Moussavi Y, Kopperud ST, Erga AH, Hansen AL, Pallesen S. Impulse-control disorders in Parkinson's disease: a meta- analysis and review of case – control studies. Front Neurol. (2018) 9:330. doi: 10.3389/fneur.2018.00330
59. Marinus J, Zhu K, Marras C, Aarsland D, van Hilten JJ. Risk factors for non-motor symptoms in Parkinson' s disease. Lancet Neurol. (2018) 17:559–68. doi: 10.1016/S1474-4422(18)30127-3
60. Cilia R, Cho SS, van Eimeren T, Marotta G, Siri C, Ko JH, et al. Pathological gambling in patients with Parkinson's disease is associated with fronto-striatal disconnection: a path modeling analysis. Mov Disord. (2011) 26:225–33. doi: 10.1002/mds.23480
61. Avanzi M, Baratti M, Cabrini S, Uber E, Brighetti G, Bonfà F. Prevalence of pathological gambling in patients with Parkinson's disease. Mov Disord. (2006) 21:2068–72. doi: 10.1002/mds.21072
62. Isaias IU, Siri C, Cilia R, De Gaspari D, Pezzoli G, Antonini A. The relationship between impulsivity and impulse control disorders in Parkinson's disease. Mov Disord. (2008) 23:411–5. doi: 10.1002/mds.21872
63. Perez-Lloret S, Rey MV, Fabre N, Ory F, Spampinato U, Brefel-Courbon C, et al. Prevalence and pharmacological factors associated with impulse-control disorder symptoms in patients with parkinson disease. Clin Neuropharmacol. (2012) 35:261–5. doi: 10.1097/WNF.0b013e31826e6e6d
64. Valença GT, Glass PG, Negreiros NN, Duarte MB, Ventura LM, Mueller M, et al. Past smoking and current dopamine agonist use show an independent and dose-dependent association with impulse control disorders in Parkinson's disease. Parkinsonism Relat Disord. (2013) 19:698–700. doi: 10.1016/j.parkreldis.2013.03.004
65. Biundo R, Weis L, Abbruzzese G, Calandra-Buonaura G, Cortelli P, Jori MC, et al. Impulse control disorders in advanced Parkinson's disease with dyskinesia: The ALTHEA study. Mov Disord. (2017) 32:1557–65. doi: 10.1002/mds.27181
66. Joutsa J, Martikainen K, Vahlberg T, Kaasinen V. Effects of dopamine agonist dose and gender on the prognosis of impulse control disorders in Parkinson's disease. Parkinsonism Relat Disord. (2012) 18:1079–83. doi: 10.1016/j.parkreldis.2012.06.005
67. Corvol J-C, Artaud F, Cormier-Dequaire F, Rascol O, Durif F, Derkinderen P, et al. Longitudinal analysis of impulse control disorders in Parkinson disease. Neurology (2018) 91:e189–201. doi: 10.1212/WNL.0000000000005816
68. Zhang Y, He A qi, Li L, Chen W, Liu ZG. Clinical characteristics of impulse control and related disorders in Chinese Parkinson's disease patients. BMC Neurol. (2017) 17:98. doi: 10.1186/s12883-017-0874-6
69. Djamshidian A, O'Sullivan SS, Lawrence AD, Foltynie T, Aviles-Olmos I, Magdalinou N, et al. Perceptual decision-making in patients with Parkinson's disease. J Psychopharmacol. (2014) 28:1149–54. doi: 10.1177/0269881114548437
70. Siri C, Cilia R, Reali E, Pozzi B, Cereda E, Colombo A, et al. Long-term cognitive follow-up of Parkinson's disease patients with impulse control disorders. Mov Disord. (2015) 30:696–704. doi: 10.1002/mds.26160
71. Steeves TD, Miyasaki J, Zurowski M, Lang AE, Pellecchia G, Van Eimeren T, et al. Increased striatal dopamine release in Parkinsonian patients with pathological gambling: a [11C] raclopride PET study. Brain (2009) 132:1376–85. doi: 10.1093/brain/awp054
72. Van Eimeren T, Pellecchia G, Cilia R, Ballanger B, Steeves TD, Houle S, et al. Drug-induced deactivation of inhibitory networks predicts pathological gambling in PD. Neurology (2010) 75:1711–6. doi: 10.1212/WNL.0b013e3181fc27fa
73. Cerasa A, Salsone M, Nigro S, Chiriaco C, Donzuso G, Bosco D, et al. Cortical volume and folding abnormalities in Parkinson's disease patients with pathological gambling. Park Relat Disord. (2014) 20:1209–14. doi: 10.1016/j.parkreldis.2014.09.001
74. Yoo HS, Yun HJ, Chung SJ, Sunwoo MK, Lee JM, Sohn YH, et al. Patterns of neuropsychological profile and cortical thinning in Parkinson's disease with punding. PLoS ONE (2015) 10:e0134468. doi: 10.1371/journal.pone.0134468
75. Sinha N, Manohar S, Husain M. Impulsivity and apathy in Parkinson's disease. J Neuropsychol. (2013) 7:255–83. doi: 10.1111/jnp.12013
76. Clark L, Stokes PR, Wu K, Michalczuk R, Benecke A, Watson BJ, et al. Striatal dopamine D2/D3receptor binding in pathological gambling is correlated with mood-related impulsivity. Neuroimage (2012) 63:40–6. doi: 10.1016/j.neuroimage.2012.06.067
77. Bloomfield MA, Morgan CJ, Kapur S, Curran HV, Howes OD. The link between dopamine function and apathy in cannabis users: an [18F]-DOPA PET imaging study. Psychopharmacology (2014) 231:2251–9. doi: 10.1007/s00213-014-3523-4
78. Antonini A, Cilia R. Behavioural adverse effects of dopaminergic treatments in Parkinson ' s disease and prevention. Drug Saf. (2009) 32:475–88. doi: 10.2165/00002018-200932060-00004
79. Leentjens AFG, Koester J, Fruh B, Shephard DT, Barone P, Houben JJ. The effect of Pramipexole on mood and motivational symptoms in Parkinson's disease: a meta-analysis of placebo-controlled studies. Clin Ther. (2009) 31:89–98. doi: 10.1016/j.clinthera.2009.01.012
80. American Psychiatric Association. Diagnostic and Statistical Manual of Mental Disorders (DSM-5). Arlington, VA (2013).
81. Lemke MR, Brecht HM, Koester J, Kraus PH, Reichmann H. Anhedonia, depression, and motor functioning in Parkinson's disease during treatment with pramipexole. J Neuropsychiatry Clin Neurosci. (2005) 17:214–20. doi: 10.1176/jnp.17.2.214
82. Hatzigiakoumis DS, Martinotti G, Di Giannantonio M, Janiri L. Anhedonia and substance dependence: clinical correlates and treatment options. Front Psychiatry (2011) 2:10. doi: 10.3389/fpsyt.2011.00010
83. Pagonabarraga J, Kulisevsky J, Strafella AP, Krack P. Apathy in Parkinson's disease: clinical features, neural substrates, diagnosis, and treatment. Lancet Neurol. (2015) 14:518–31. doi: 10.1016/S1474-4422(15)00019-8
84. Boileau I, Warsh JJ, Guttman M, Saint-Cyr JA, McCluskey T, Rusjan P, et al. Elevated serotonin transporter binding in depressed patients with Parkinson's disease: a preliminary PET study with [11C]DASB. Mov Disord. (2008) 23:1776–80. doi: 10.1002/mds.22212
85. Ishihara L, Brayne C. A systematic review of depression and mental illness preceding Parkinson's disease. Acta Neurol Scand. (2006) 113:211–20. doi: 10.1111/j.1600-0404.2006.00579.x
86. Voon V, Napier TC, Frank MJ, Sgambato-Faure V, Grace AA, Rodriguez-Oroz M, et al. Impulse control disorders and levodopa-induced dyskinesias in Parkinson's disease: an update. Lancet Neurol. (2017) 16:238–50. doi: 10.1016/S1474-4422(17)30004-2
Keywords: Parkinson's disease, impulse control disorder, cognition, affective factors, motivation, impulsivity, meta-analysis, depression
Citation: Martini A, Dal Lago D, Edelstyn NMJ, Grange JA and Tamburin S (2018) Impulse Control Disorder in Parkinson's Disease: A Meta-Analysis of Cognitive, Affective, and Motivational Correlates. Front. Neurol. 9:654. doi: 10.3389/fneur.2018.00654
Received: 21 April 2018; Accepted: 23 July 2018;
Published: 28 August 2018.
Edited by:
Mayela Rodríguez-Violante, Instituto Nacional de Neurología y Neurocirugía (INNN), MexicoReviewed by:
Ruey-Meei Wu, National Taiwan University, TaiwanRou-Shayn Chen, Chang Gung Memorial Hospital, Taiwan
Copyright © 2018 Martini, Dal Lago, Edelstyn, Grange and Tamburin. This is an open-access article distributed under the terms of the Creative Commons Attribution License (CC BY). The use, distribution or reproduction in other forums is permitted, provided the original author(s) and the copyright owner(s) are credited and that the original publication in this journal is cited, in accordance with accepted academic practice. No use, distribution or reproduction is permitted which does not comply with these terms.
*Correspondence: Alice Martini, YS5tYXJ0aW5pQGtlZWxlLmFjLnVr