- 1The Solomon H. Snyder Department of Neuroscience, Johns Hopkins University School of Medicine, Baltimore, MD, United States
- 2Department of Psychiatry and Behavioral Sciences, Johns Hopkins University School of Medicine, Baltimore, MD, United States
- 3Department of Pharmacology and Molecular Sciences, Johns Hopkins University School of Medicine, Baltimore, MD, United States
Current medications for neurodegenerative and neuropsychiatric diseases such as Alzheimer's disease (AD), Huntington's disease (HD), Parkinson's disease (PD), and Schizophrenia mainly target disease symptoms. Thus, there is an urgent need to develop novel therapeutics that can delay, halt or reverse disease progression. AD, HD, PD, and schizophrenia are characterized by elevated oxidative and nitrosative stress, which play a central role in pathogenesis. Clinical trials utilizing antioxidants to counter disease progression have largely been unsuccessful. Most antioxidants are relatively non-specific and do not adequately target neuroprotective pathways. Accordingly, a search for agents that restore redox balance as well as halt or reverse neuronal loss is underway. The small molecules, cysteamine, the decarboxylated derivative of the amino acid cysteine, and cystamine, the oxidized form of cysteamine, respectively, mitigate oxidative stress and inflammation and upregulate neuroprotective pathways involving brain-derived neurotrophic factor (BDNF) and Nuclear factor erythroid 2-related factor 2 (Nrf2) signaling. Cysteamine can traverse the blood brain barrier, a desirable characteristic of drugs targeting neurodegeneration. This review addresses recent developments in the use of these aminothiols to counter neurodegeneration and neuropsychiatric deficits.
Introduction
Cysteamine, also known as 2-mercaptoethylamine or aminoethanethiol, is the decarboxylated derivative of the amino acid cysteine. It exerts radioprotective effects and is more effective than cysteine alone, although a combination of cysteamine and cysteine display synergistic effects (1, 2). Although cysteamine reduced mortality in irradiated Drosophila and mice, mutagenic effects of radiation were not prevented (3, 4). Cysteamine has been utilized for the treatment of cystinosis, a lysosomal disorder, and, more recently, has been evaluated for the treatment of neurodegenerative disorders. This review will summarize the current understanding of cysteamine and cystamine, its oxidized derivative.
In cells, the amino thiol is generated by the degradation of coenzyme A, which in turn, is generated from pantothenate (vitamin B5) and cysteine (Figure 1A) (5). Coenzyme A degradation yields pantetheine, which is hydrolyzed by pantetheinase or vanin, generating cysteamine and pantothenic acid. Cysteamine is then oxidized to hypotaurine by cysteamine dioxygenase (6). Hypotaurine can be converted into taurine by hypotaurine dehydrogenase. Taurine is eliminated in the form of bile salts such as taurocholate, either via the urine or feces (7). Levels of cysteamine has been reported to be in the low micromolar range in tissues such as the liver, kidney and brain, which were measured after treating lysates with DTT to liberate free cysteamine (6), indicating association with proteins via disulfide bonding. Similarly, another study measured cysteamine after reducing perchloric acid treated kidney and liver lysates with mercaptopropionic acid (8). The presence of disulfide-bonded cysteamine with proteins was subsequently shown by Duffel and associates (9), which could account for the effects of cysteamine and cystamine on the activity of several proteins.
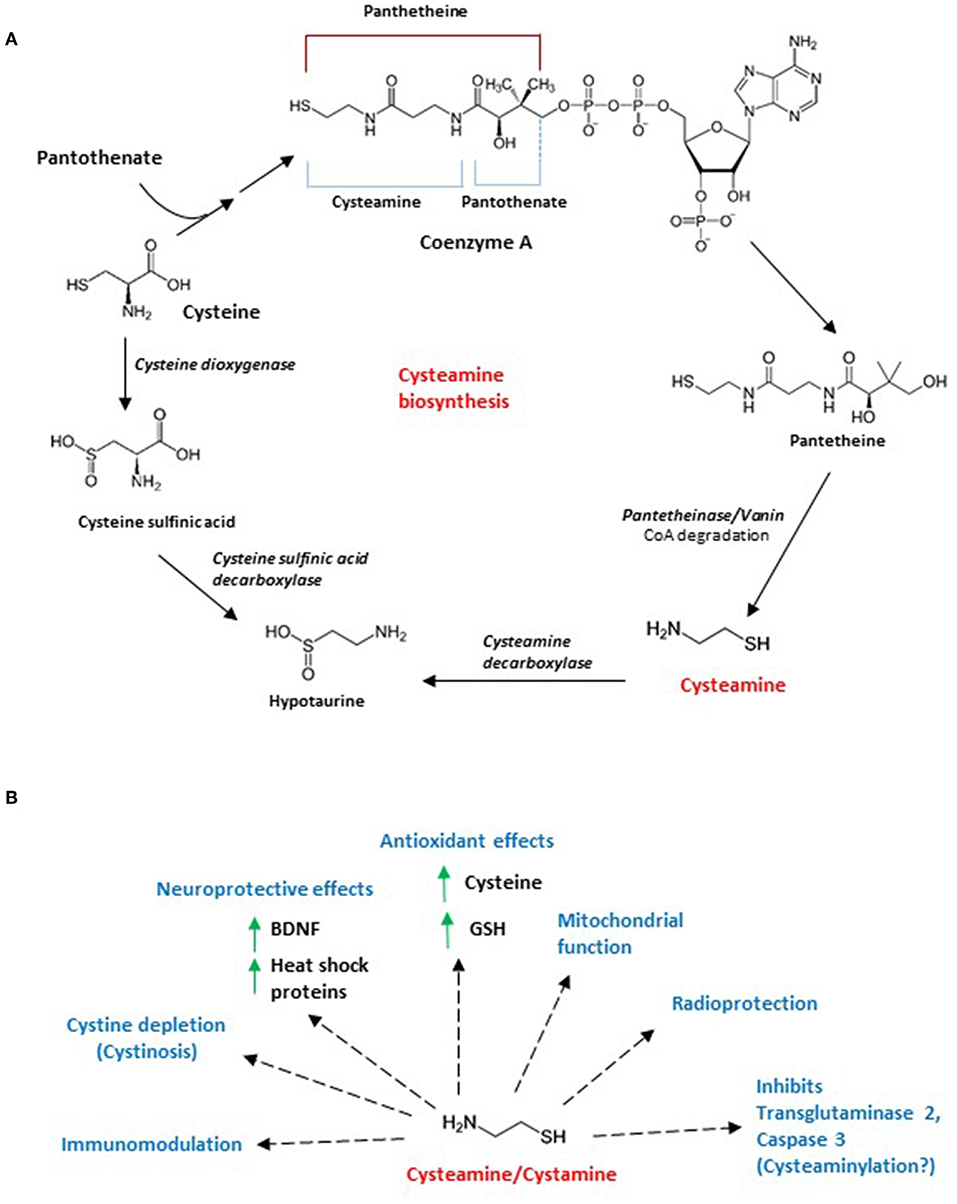
Figure 1. (A) Biosynthesis of cysteamine and intersection with cysteine catabolism. Cysteamine is generated in mammals by the degradation of coenzyme A, which is required for the metabolism of fatty acids, carbohydrates, amino acids and ketone bodies. When coenzyme A is cleaved (cleavage at the dotted line), pantetheine is generated, which is acted on by pantetheinase or vanin to form cysteamine. Cysteamine is converted to hypotaurine by cysteamine decarboxylase. Cysteine, a component of coenzyme A, is acted on by cysteine dioxygenase to form cysteine sulfonate which is decarboxylated by cysteine sulfonate decarboxylase to form hypotaurine. Hypotaurine generated is further metabolized to taurine by hypotaurine decarboxylase. (B) Effects of cysteamine/cystamine. Both cysteamine and its oxidized form cystamine have protective effects in cells and tissues. Originally identified as radioprotective molecules, subsequently these aminothiols have been reported to mitigate cystinosis, a condition characterized by accumulation of cystine crystals in the body. Cystamine and cysteamine have a variety of other effects which include antioxidant effects (by increasing cysteine and glutathione levels), inhibition of transglutaminase 2 and caspase 3 (possibly by modifying reactive cysteine residues or cysteaminylation), modulation of mitochondrial function, immunomodulation. These molecules have also been reported to increase levels of brain derived neurotrophic factor (BDNF) and heat shock proteins, which affords neuroprotective benefits.
The metabolism of cysteamine, cystamine and cysteine are linked in cells. Both cysteamine and cystamine increase cysteine levels intracellularly in a temporal and dose-dependent manner (10). As cysteine is a component of glutathione and a potent antioxidant itself, treatment of cells with these aminothiols can mitigate oxidative stress. Treatment of SN56 cholinergic cells causes an increase in cysteine levels in 30 min. Cystamine is first converted to cysteamine in the reducing atmosphere of cells, and treating cells with cystamine elicits an increase of cysteine in 3 h. N-acetylcysteine (NAC), 2-mercaptoethanesulfonic acid (MESNA) and mercaptopropionylglycine (MPG), on the other hand, elevate cysteine levels to a lesser extent (2-fold as compared to 6-fold in the case of cysteamine). The study also revealed the importance of these thiols in sequestering reactive aldehyde species in cells and bolstering the antioxidant capacity of cells. Thus, cystamine and cysteamine also act as antioxidants themselves. Consistent with these observations, cysteamine affords protection against acetaminophen- mediated liver damage, where the highly toxic unsaturated aldehyde acrolein, is produced (11, 12). Cysteamine has also been proposed to replace homocysteine as the substrate for cystathionine β-synthase (CBS) in a reaction with serine to generate thialysine or (S-(2-aminoethyl)-L-cysteine) (13). Consistent with these studies, thialysine levels increase in the brain after feeding cysteamine to rats (14).
Protective Effects of Cysteamine and Cystamine
Therapeutic Applications of Cysteamine and Cystamine in Peripheral Tissues
Both cysteamine and cystamine, have been used for the treatment of several conditions (Figure 1B). These compounds possess radioprotective properties and were initially used to treat radiation sickness that arises in cancer patients after radiotherapy, but subsequently discontinued after unsuccessful clinical trials (1, 15). One of the earliest uses of cysteamine in medicine, which is FDA-approved, is the treatment of cystinosis, an inherited autosomal recessive disorder in which the body accumulates cystine due a defect in the lysosomal cysteine transporter, cystinosin (16, 17). Cystine crystals build up in many tissues and damage organs such as the kidney and the eye. One of the initial manifestations of juvenile cystinosis is renal Fanconi syndrome which manifests as dysfunction of the renal proximal tubule leading to polyuria, phosphaturia, glycosuria, proteinuria, acidosis, growth retardation, and rickets (18). Cysteamine participates in disulfide exchange reactions to form cysteine and mixed disulfides of cysteine and cysteamine, which can then exit the lysosome.
Cysteamine also has anti-malarial effects preventing the replication of the parasite, Plasmodium falciparum in vivo and also potentiates the action of the anti-malarial artemisin (19, 20). Cysteamine has also been reported to have anti-HIV-1 effects (21, 22). Cysteamine elicits both beneficial and harmful effects, some of which included ulcer formation and anti-angiogenic effects (23). Cystamine, the oxidized form of cysteamine, inhibits erythrocyte sickling in sickle cell anemia (24). Incubating sickle cells with cystamine leads to the formation of an S-ethylamine derivative and a decrease in sickling under hypoxic conditions. Several other beneficial effects of the two cysteine derivatives are summarized in Table 1.
Therapeutic Applications of Cysteamine and Cystamine in Brain Diseases
Cysteamine and cystamine appear to be promising in the treatment of certain mouse models of neurodegenerative diseases, such as Parkinson's disease (PD) and Huntington's disease (HD) (47). Cysteamine can cross the blood-brain barrier, which makes it an attractive candidate for therapeutic applications (48).
Huntington's Disease
Huntington's disease is a neurodegenerative disorder caused by expansion of polyglutamine repeats in the protein huntingtin, Htt, which causes it to aggregate and cause widespread damage in almost all tissues expressing it (49). Initial studies on cystamine and its therapeutic effects on disease progression in HD focused on its inhibitory effects on the enzyme transglutaminase (37, 40). Transglutaminases catalyze the formation of ε-N-(γ-glutamyl)-lysyl crosslinks between proteins and were proposed to contribute to neuropathology of HD (50–52). However, later studies revealed that ablation of the transglutaminase gene did not prevent neurodegeneration in HD (53). Cystamine has also been beneficial in a fly model of HD, where photoreceptor degeneration was rescued in adult flies (54). Cystamine treatment in mouse models of HD lead to increased cysteine levels, which was proposed to be neuroprotective (35, 55). Cysteine is a potent antioxidant and dysregulated cysteine metabolism mediates neurodegeneration in HD (56–58). Cysteine is also the precursor of the gaseous signaling molecule, hydrogen sulfide, which participates in a myriad of physiological processes (59–61). Cystamine, in combination with mithramycin, was also shown to be protective in the R6/2 model of HD (62). The beneficial effects of cysteamine led to clinical trials in HD (63). In addition, cystamine can augment levels of brain derived neurotrophic factor, BDNF, in mouse models of HD (64). More recently cysteamine was shown to counteract toxicity mediated by mutant huntingtin in vitro in primary neuron and iPSC models of HD although the exact molecular mechanism by which cytoprotection is conferred is still unknown (65).
Alzheimer's Disease
Alzheimer's disease (AD) is the most prevalent neurodegenerative disorder and the most common form of dementia (66, 67). The molecular hallmarks of AD include increased load of amyloid plaques and neurofibrillary tangles, which affect multiple cellular processes. Numerous reports describe links between dementia and AD with amyloid deposits or tangles. Postmortem analysis of cognitively normal subjects have revealed increased amyloid plaques, a pathogenic signature of AD, but no dementia (68). Conversely, several diagnosed AD patients have no signs of neuritic plaques (69). Thus, the correlation between amyloid plaques and AD awaits further study (70). Regardless of these inconsistencies, it is clear that the brain has corrective mechanisms that delay cognitive decline and if harnessed, may stall neurodegeneration. The search for small molecules that stimulate neuroprotective signaling cascades may be beneficial. Cystamine and its derivatives are being evaluated as possible therapies for the disease. Chronic cysteamine treatment (daily injections for a period of 4 months) resulted in improvements in habituation and spatial learning deficits in the APP-Psen1 mouse model of AD (71). The APP-Psen1 model harbors the human transgenes for the Swedish mutation of the amyloid precursor protein (APP) and presenilin-1 (PSEN1) containing an L166P mutation, regulated by the Thy-1 promoter (72). AD patients have elevated transglutaminase levels, which colocalize with the amyloid plaques (34). Transglutaminases accelerate amyloid beta aggregation and toxicity. Accordingly, cystamine therapy is being considered for lowering the amyloid plaque burden in AD patients. In particular, Multi-Target Directed Ligands (MTDLs) or single compounds which may simultaneously act on different targets are being explored. Along these lines, a cysamine-tacrine dimer has been developed, which decreased acetylcholinesterase (AChE)-induced beta-amyloid aggregation (73).
Parkinson's Disease
Aggregation of alpha-synuclein, leading to the formation of Lewy bodies, is a hallmark of Parkinson's disease (PD), which affects the substantia nigra of the brain causing motor deficits and multiple abnormalities. Existing therapies for PD largely target symptoms and do not mitigate neuronal loss observed. Several lines of evidence suggest the therapeutic potential of the aminothiol in PD (71). Cystamine ameliorated mitochondrial dysfunction and oxidative stress associated with 6-hydroxydopamine and 1-methyl-4-phenyl-1,2,3,6-tetrahydropyridine (MPTP)-induced models of PD (74). In the MPTP-induced neurotoxicity model of PD in mice, independent studies revealed various effects of cystamine such as elevation in the levels of tyrosine hydroxylase and BDNF (30, 75). Similarly, cysteamine, the reduced form of cystamine, also afforded neuroprotection. Similar to AD, elevated transglutaminase activity caused an increase in the formation of cross-linked alpha-synuclein and insoluble aggregates, which could be abrogated by cystamine (36).
Amyotrophic Lateral Sclerosis
ALS, also known as Lou Gehrig's disease, is a neurodegenerative disease where selective degeneration of motor neurons in the brain and spinal cord occurs leading to paralysis of skeletal muscles and progressive weakness and atrophy of limbs (76). Difficulties in speech and movement follow and patients are typically wheelchair-bound. Causes of ALS can be either genetic or sporadic (refers to patients without a family history). Among the best studied genetic mutations in familial ALS include mutations in superoxide dismutase 1 (SOD1), which misfolds, aggregates, and elicit toxicity by multiple mechanisms (77, 78). Proposed reasons for SOD1 aggregation include crosslinking mediated by transglutaminase 2 (TG2). Studies with cell culture models of ALS reveal that cystamine prevents aggregation of SOD1 and improved cell survival (79). Furthermore, inhibiting spinal TG2 by cystamine reduces SOD1 oligomers, microglial activation and delayed progression in the G93A SOD1 mouse model of ALS (41). Thus, cystamine treatment may be beneficial in treating ALS.
Neurological Complications of Cystinosis
Although cystinosis was not considered to affect brain function, it is now known that cystinosis can result in neurocognitive deficits in adults as well as children. These include impaired visual spatial, visual memory, language problems, academic impairment, seizures, memory impairment, motor incoordination, and neuromuscular dysfunction and is often accompanied by structural abnormalities in the brain (80–82). Early treatment with cysteamine orally prevents several of these neurocognitive deficits. Patients with cystinosis treated at or after age 2 years (late-treatment group) score poorer than the early treatment group (before 2 years) on verbal, performance, and full-scale IQ tests and tests rating visual-spatial skills (83). Similarly, adults with cystinosis who receive consistent chronic treatment with cysteamine fare better on visual learning and memory skills (84).
Schizophrenia and Neuropsychiatric Diseases
Schizophrenia is a psychiatric disease, with complex genetic and neurological contributions of unclear origins, manifesting as a combination of symptoms which includes hallucinations, delusions, motivational and cognitive deficits (85). Although treatments for schizophrenia target psychotic symptoms, most existing drugs do not relieve social and cognitive deficits. The neurochemical changes in schizophrenia typically occur well before formal diagnosis, and, thus, preventive therapies could be beneficial. Schizophrenic patients have lower levels of BDNF so that schizophrenic patients might benefit from use of cysteamine due to its BDNF-enhancing properties and effects on the dopaminergic system (86, 87). In an amphetamine-induced psychosis model of schizophrenia, cysteamine prevents increased locomotor activity by decreasing dopamine release (88). Cysteamine counteracts the BDNF-lowering effects of haloperidol (89). The anti-depressant effect of cysteamine may also benefit other mental conditions (90). These studies are consistent with an earlier study which demonstrated that cysteamine blocked amphetamine-induced deficits in sensorimotor gating in male Sprague-Dawley rats (91). Similarly, cysteamine treatment increases BDNF levels in the frontal cortex and hippocampus and improved spatial memory in heterozygous reeler mice, which exhibit behavioral and neurochemical abnormalities similar to those in schizophrenia (92).
Similarly, cystamine and cysteamine may be beneficial in other conditions involving low neurotrophin levels, such as autism spectrum disorders (ASD). Analysis of postmortem human brain samples revealed increases in TG2 mRNA and protein levels in the middle frontal gyrus of subjects with autism spectrum disorder. Thus, cysteamine may alleviate symptoms of ASD by inhibiting TG2 and increasing BDNF levels (93). The same study demonstrated that ER stress induced TG2 expression and deficits in social behavior. Systemic administration of cysteamine attenuated these behavioral abnormalities. In mice lacking methyl-CpG binding protein 2 (MeCP2), a model of Rett syndrome, associated with decreased BDNF levels and obsessive compulsive phenotypes, cysteamine treatment improved lifespan, and improved motor function (94, 95). In a similar vein, cysteamine counteracted anxiety, and depression-like behaviors in a mouse model of anxiety/depression induced by chronic glucocorticoid exposure (96).
Potential Side-Effects of Cysteamine and Cystamine
Although cysteamine and cystamine have several desirable effects in cells and tissues, some studies have reported side-effects. For instance, in the treatment of HD patients using cysteamine (Cystagon) in the CYTE-I-HD clinical trials, rashes, nausea, and motor impairment along with bad breath were observed in a few patients (63). In phase II trials, asthenia or fatigue was more commonly observed (97). Despite these side-effects, cysteamine appeared to be well tolerated by almost all of the patients.
Concluding Remarks
Some therapies using antioxidants have not yielded satisfactory outcomes in clinical trials (98–101). Several reasons have been attributed to the failure of such trials. Certain antioxidants inhibit fundamental cellular processes such as autophagy, which is crucial to eliminate misfolded proteins and damaged organelles (102). Most antioxidants utilized only target specific free radicals and thus may counteract only selected types of free radicals. Most clinical trials were initiated relatively late in disease progression, when most of the oxidative damage has already accrued. Doses of antioxidants utilized have also not been adequately tested. Durations of several of these trials have also been short, and longer term uses of redox active molecules have not been studied in detail. Thus, development of antioxidant molecules that have multiple targets, while not inhibiting basic cellular processes such as autophagy, is crucial. Cysteamine normalizes the proteostasis machinery by restoring BECN1/Beclin 1-dependent autophagy in cystic fibrosis in mouse models of the disease and also in patients (103). Cysteamine dendrimers have been found to ameliorate autophagy deficits in cystic fibrosis (104). It is evident that signaling pathways modulated by cystamine and cysteamine are diverse (Figure 1B), and knowledge of these cascades will yield information that can be harnessed to tailor treatments for diverse diseases. The tissue-specific effects and optimal concentrations of the thiol redox couple that would be beneficial for specific diseases has still not been elucidated. Although these aminothiols possess beneficial disease-modifying effects in several conditions, it is still unclear whether these molecules or their metabolites mediate the cytoprotection observed in neurodegenerative diseases. However, increase in cysteine levels can promote neuroprotection, and some of the beneficial effects can be attributed to increases in cysteine to mitigate oxidative stress as has been observed in HD (56). Similarly, systematic studies measuring the concentration and metabolism of cysteamine and cystamine in pathological conditions have not been conducted and are areas of future investigation. Epigenetic effects of cystamine and cysteamine and cysteaminylation, the posttranslational modification mediated by cystamine and cysteamine await detailed investigation. The use of cystamine and cysteamine is another example of a repurposed drug, which has cytoprotective effects in the brain. Combination therapy of these aminothiols with other approved drugs offer attractive options to arrive at safe and effective drugs for these complex diseases.
Author Contributions
BP conceptualized the review. BP and SS wrote the review.
Funding
This work was supported by the US Public Health Service grants MH18501 and DA000266 to SS.
Conflict of Interest
The authors declare that the research was conducted in the absence of any commercial or financial relationships that could be construed as a potential conflict of interest.
Abbreviations
AChE, acetylcholinesterase; AD, Alzheimer's disease; APP, amyloid precursor protein; ALS, Amyotrophic lateral sclerosis; ASD, Autism spectrum disorders; BDNF, brain-derived neurotrophic factor; BECN1, beclin 1; CBS, cystathionine β-synthase; HD, Huntington's disease; MPTP, 1-methyl-4-phenyl-1,2,3,6-tetrahydropyridine; MESNA, 2-mercaptoethanesulfonic acid; MPG, mercaptopropionylglycine; MeCp2, methyl-CpG binding protein 2; MTDL, multi-target-directed ligand; PD, Parkinson's disease; Nrf2, Nuclear factor erythroid 2-related factor 2; PSEN1, presenilin 1; SOD1, superoxide dismutase 1; TG2, transglutaminase 2.
References
1. Bacq ZM, Dechamps G, Fischer P, Herve A, Le Bihan H, Lecomte J, et al. Protection against x-rays and therapy of radiation sickness with beta-mercaptoethylamine. Science. (1953) 117:633–6. doi: 10.1126/science.117.3049.633
2. Straube RL, Patt HM. Studies with cysteinamine and cysteine in x-irradiated animals. Proc Soc Exp Biol Med. (1953) 84:702–4. doi: 10.3181/00379727-84-20758
3. Kaplan WD, Lyon MF. Failure of mercaptoethylamine to protect against the mutagenic effects of radiation. I Experiments with Drosophila. Science. (1953) 118:776–7. doi: 10.1126/science.118.3078.776
4. Kaplan WD, Lyon MF. Failure of mercaptoethylamine to protect against the mutagenic effects of radiation. II Experiments with mice. Science. (1953) 118:777–8. doi: 10.1126/science.118.3078.777
5. Hoagland MB, Novelli GD. Biosynthesis of coenzyme A from phospho-pantetheine and of pantetheine from pantothenate. J Biol Chem. (1954) 207:767–73.
6. Coloso RM, Hirschberger LL, Dominy JE, Lee JI, Stipanuk MH. Cysteamine dioxygenase: evidence for the physiological conversion of cysteamine to hypotaurine in rat and mouse tissues. Adv Exp Med Biol. (2006) 583:25–36. doi: 10.1007/978-0-387-33504-9_3
7. Huxtable RJ. Physiological actions of taurine. Physiol Rev. (1992) 72:101–63. doi: 10.1152/physrev.1992.72.1.101
8. Pitari G, Malergue F, Martin F, Philippe JM, Massucci MT, Chabret C, et al. Pantetheinase activity of membrane-bound Vanin-1: lack of free cysteamine in tissues of Vanin-1 deficient mice. FEBS Lett. (2000) 483:149–54. doi: 10.1016/S0014-5793(00)02110-4
9. Duffel MW, Logan DJ, Ziegler DM. Cysteamine and cystamine. Methods Enzymol. (1987) 143:149–54. doi: 10.1016/0076-6879(87)43027-9
10. Wood PL, Khan MA, Moskal JR. Cellular thiol pools are responsible for sequestration of cytotoxic reactive aldehydes: central role of free cysteine and cysteamine. Brain Res. (2007) 1158:158–63. doi: 10.1016/j.brainres.2007.05.007
11. Peterson TC, Brown IR. Cysteamine in combination with N-acetylcysteine prevents acetaminophen-induced hepatotoxicity. Can J Physiol Pharmacol. (1992) 70:20–8. doi: 10.1139/y92-004
12. Arai T, Koyama R, Yuasa M, Kitamura D, Mizuta R. Acrolein, a highly toxic aldehyde generated under oxidative stress in vivo, aggravates the mouse liver damage after acetaminophen overdose. Biomed Res. (2014) 35:389–95. doi: 10.2220/biomedres.35.389
13. Shen W, McGath MK, Evande R, Berkowitz DB. A continuous spectrophotometric assay for human cystathionine beta-synthase. Anal Biochem. (2005) 342:103–10. doi: 10.1016/j.ab.2005.03.051
14. Pinto JT, Khomenko T, Szabo S, McLaren GD, Denton TT, Krasnikov BF, et al. Measurement of sulfur-containing compounds involved in the metabolism and transport of cysteamine and cystamine. Regional differences in cerebral metabolism. J Chromatogr B Analyt Technol Biomed Life Sci. (2009) 877:3434–41. doi: 10.1016/j.jchromb.2009.05.041
15. Healy JB. A trial of cystamine in radiation sickness. Br J Radiol. (1960) 33:512–4. doi: 10.1259/0007-1285-33-392-512
16. Town M, Jean G, Cherqui S, Attard M, Forestier L, Whitmore SA, et al. A novel gene encoding an integral membrane protein is mutated in nephropathic cystinosis. Nat Genet. (1998) 18:319–24. doi: 10.1038/ng0498-319
17. Gahl WA, Thoene JG, Schneider JA. Cystinosis. N Engl J Med. (2002) 347:111–21. doi: 10.1056/NEJMra020552
18. Cherqui S, Courtoy PJ. The renal Fanconi syndrome in cystinosis: pathogenic insights and therapeutic perspectives. Nat Rev Nephrol. (2017) 13:115–31. doi: 10.1038/nrneph.2016.182
19. Min-Oo G, Ayi K, Bongfen SE, Tam M, Radovanovic I, Gauthier S, et al. Cysteamine, the natural metabolite of pantetheinase, shows specific activity against Plasmodium. Exp Parasitol. (2010) 125:315–24. doi: 10.1016/j.exppara.2010.02.009
20. Min-Oo G, Fortin A, Poulin JF, Gros P. Cysteamine, the molecule used to treat cystinosis, potentiates the antimalarial efficacy of artemisinin. Antimicrob Agents Chemother. (2010) 54:3262–70. doi: 10.1128/AAC.01719-09
21. Ho WZ, Zhu XH, Song L, Lee HR, Cutilli JR, Douglas SD. Cystamine inhibits HIV type 1 replication in cells of monocyte/macrophage and T cell lineages. AIDS Res Hum Retroviruses. (1995) 11:451–9. doi: 10.1089/aid.1995.11.451
22. Bergamini A, Ventura L, Mancino G, Capozzi M, Placido R, Salanitro A, et al. In vitro inhibition of the replication of human immunodeficiency virus type 1 by beta-mercaptoethylamine (cysteamine). J Infect Dis. (1996) 174:214–8. doi: 10.1093/infdis/174.1.214
23. Szabo S, Deng X, Khomenko T, Chen L, Tolstanova G, Osapay K, et al. New molecular mechanisms of duodenal ulceration. Ann N Y Acad Sci. (2007) 1113:238–55. doi: 10.1196/annals.1391.033
24. Hassan W, Beuzard Y, Rosa J. Inhibition of erythrocyte sickling by cystamine, a thiol reagent. Proc Natl Acad Sci USA. (1976) 73:3288–92. doi: 10.1073/pnas.73.9.3288
25. Ientile R, Campisi A, Raciti G, Caccamo D, Curro M, Cannavo G, et al. Cystamine inhibits transglutaminase and caspase-3 cleavage in glutamate-exposed astroglial cells. J Neurosci Res. (2003) 74:52–9. doi: 10.1002/jnr.10702
26. Koyama R, Mizuta R. Acrolein scavengers, cysteamine and N-benzylhydroxylamine, reduces the mouse liver damage after acetaminophen overdose. J Vet Med Sci. (2017) 78:1903–5. doi: 10.1292/jvms.16-0325
27. Hsu TC, Chiu CC, Wang YW, Tzang BS. Effects of cystamine on antioxidant activities and regulatory T cells in lupus-prone mice. J Cell Mol Med. (2013) 17:1308–15. doi: 10.1111/jcmm.12107
28. Najafi A, Kia HD, Mohammadi H, Najafi MH, Zanganeh Z, Sharafi M, et al. Different concentrations of cysteamine and ergothioneine improve microscopic and oxidative parameters in ram semen frozen with a soybean lecithin extender. Cryobiology. (2014) 69:68–73. doi: 10.1016/j.cryobiol.2014.05.004
29. Li PC, Jiao Y, Ding J, Chen YC, Cui Y, Qian C, et al. Cystamine improves functional recovery via axon remodeling and neuroprotection after stroke in mice. CNS Neurosci Ther. (2015) 21:231–40. doi: 10.1111/cns.12343
30. Gibrat C, Bousquet M, Saint-Pierre M, Levesque D, Calon F, Rouillard C, et al. Cystamine prevents MPTP-induced toxicity in young adult mice via the up-regulation of the brain-derived neurotrophic factor. Prog Neuropsychopharmacol Biol Psychiatry. (2010) 34:193–203. doi: 10.1016/j.pnpbp.2009.11.005
31. Sun L, Xu S, Zhou M, Wang C, Wu Y, Chan P. Effects of cysteamine on MPTP-induced dopaminergic neurodegeneration in mice. Brain Res. (2010) 1335:74–82. doi: 10.1016/j.brainres.2010.03.079
32. Calkins MJ, Townsend JA, Johnson DA, Johnson JA. Cystamine protects from 3-nitropropionic acid lesioning via induction of nf-e2 related factor 2 mediated transcription. Exp Neurol. (2010) 224:307–17. doi: 10.1016/j.expneurol.2010.04.008
33. Zhang H, Zheng M, Wu M, Xu D, Nishimura T, Nishimura Y, et al. A pharmacogenetic discovery: cystamine protects against haloperidol-induced toxicity and ischemic brain injury. Genetics. (2016) 203:599–609. doi: 10.1534/genetics.115.184648
34. Kawabe K, Takano K, Moriyama M, Nakamura Y. Transglutaminases derived from astrocytes accelerate amyloid beta aggregation. Neurochem Res. (2017) 42:2384–91. doi: 10.1007/s11064-017-2258-0
35. Fox JH, Barber DS, Singh B, Zucker B, Swindell MK, Norflus F, et al. Cystamine increases L-cysteine levels in Huntington's disease transgenic mouse brain and in a PC12 model of polyglutamine aggregation. J Neurochem. (2004) 91:413–22. doi: 10.1111/j.1471-4159.2004.02726.x
36. Junn E, Ronchetti RD, Quezado MM, Kim SY, Mouradian MM. Tissue transglutaminase-induced aggregation of alpha-synuclein: implications for Lewy body formation in Parkinson's disease and dementia with Lewy bodies. Proc Natl Acad Sci USA. (2003) 100:2047–52. doi: 10.1073/pnas.0438021100
37. Dedeoglu A, Kubilus JK, Jeitner TM, Matson SA, Bogdanov M, Kowall NW, et al. Therapeutic effects of cystamine in a murine model of Huntington's disease. J Neurosci. (2002) 22:8942–50. doi: 10.1523/JNEUROSCI.22-20-08942.2002
38. Ribeiro M, Silva AC, Rodrigues J, Naia L, Rego AC. Oxidizing effects of exogenous stressors in Huntington's disease knock-in striatal cells–protective effect of cystamine and creatine. Toxicol Sci. (2013) 136:487–99. doi: 10.1093/toxsci/kft199
39. Mao Z, Choo YS, Lesort M. Cystamine and cysteamine prevent 3-NP-induced mitochondrial depolarization of Huntington's disease knock-in striatal cells. Eur J Neurosci. (2006) 23:1701–10. doi: 10.1111/j.1460-9568.2006.04686.x
40. Karpuj MV, Becher MW, Springer JE, Chabas D, Youssef S, Pedotti R, et al. Prolonged survival and decreased abnormal movements in transgenic model of Huntington disease, with administration of the transglutaminase inhibitor cystamine. Nat Med. (2002) 8:143–9. doi: 10.1038/nm0202-143
41. Oono M, Okado-Matsumoto A, Shodai A, Ido A, Ohta Y, Abe K, et al. Transglutaminase 2 accelerates neuroinflammation in amyotrophic lateral sclerosis through interaction with misfolded superoxide dismutase 1. J Neurochem. (2014) 128:403–18. doi: 10.1111/jnc.12441
42. Davies JE, Rose C, Sarkar S, Rubinsztein DC. Cystamine suppresses polyalanine toxicity in a mouse model of oculopharyngeal muscular dystrophy. Sci Transl Med. (2010) 2:34ra40. doi: 10.1126/scitranslmed.3000723
43. Engholm M, Eftekhari A, Chwatko G, Bald E, Mulvany MJ. Effect of cystamine on blood pressure and vascular characteristics in spontaneously hypertensive rats. J Vasc Res. (2011) 48:476–84. doi: 10.1159/000327773
44. Okamura DM, Bahrami NM, Ren S, Pasichnyk K, Williams JM, Gangoiti JA, et al. Cysteamine modulates oxidative stress and blocks myofibroblast activity in CKD. J Am Soc Nephrol. (2014) 25:43–54. doi: 10.1681/ASN.2012090962
45. Lee SM, Jeong EM, Jeong J, Shin DM, Lee HJ, Kim HJ, et al. Cysteamine prevents the development of lens opacity in a rat model of selenite-induced cataract. Invest Ophthalmol Vis Sci. (2012) 53:1452–9. doi: 10.1167/iovs.11-8636
46. Wang S, Li X, Li M, Jiang L, Yuan H, Han W, et al. Cystamine attenuated behavioral deficiency via increasing the expression of BDNF and activating PI3K/Akt signaling in 2,5-hexanedione intoxicated rats. Toxicol Res. (2017) 6:199–204. doi: 10.1039/C6TX00409A
47. Gibrat C, Cicchetti F. Potential of cystamine and cysteamine in the treatment of neurodegenerative diseases. Prog Neuropsychopharmacol Biol Psychiatry. (2011) 35:380–9. doi: 10.1016/j.pnpbp.2010.11.023
48. Bousquet M, Gibrat C, Ouellet M, Rouillard C, Calon F, Cicchetti F. Cystamine metabolism and brain transport properties: clinical implications for neurodegenerative diseases. J Neurochem. (2010) 114:1651–8. doi: 10.1111/j.1471-4159.2010.06874.x
49. McColgan P, Tabrizi SJ. Huntington's disease: a clinical review. Eur J Neurol. (2018) 25:24–34. doi: 10.1111/ene.13413
50. Kahlem P, Green H, Djian P. Transglutaminase action imitates Huntington's disease: selective polymerization of Huntingtin containing expanded polyglutamine. Mol Cell. (1998) 1:595–601. doi: 10.1016/S1097-2765(00)80059-3
51. Karpuj MV, Garren H, Slunt H, Price DL, Gusella J, Becher MW, et al. Transglutaminase aggregates huntingtin into nonamyloidogenic polymers, and its enzymatic activity increases in Huntington's disease brain nuclei. Proc Natl Acad Sci USA. (1999) 96:7388–93. doi: 10.1073/pnas.96.13.7388
52. Klock C, Khosla C. Regulation of the activities of the mammalian transglutaminase family of enzymes. Protein Sci. (2012) 21:1781–91. doi: 10.1002/pro.2162
53. Menalled LB, Kudwa AE, Oakeshott S, Farrar A, Paterson N, Filippov I, et al. Genetic deletion of transglutaminase 2 does not rescue the phenotypic deficits observed in R6/2 and zQ175 mouse models of Huntington's disease. PLoS ONE. (2014) 9:e99520. doi: 10.1371/journal.pone.0099520
54. Bortvedt SF, McLear JA, Messer A, Ahern-Rindell AJ, Wolfgang WJ. Cystamine and intrabody co-treatment confers additional benefits in a fly model of Huntington's disease. Neurobiol Dis. (2010) 40:130–4. doi: 10.1016/j.nbd.2010.04.007
55. Pinto JT, Van Raamsdonk JM, Leavitt BR, Hayden MR, Jeitner TM, Thaler HT, et al. Treatment of YAC128 mice and their wild-type littermates with cystamine does not lead to its accumulation in plasma or brain: implications for the treatment of Huntington disease. J Neurochem. (2005) 94:1087–101. doi: 10.1111/j.1471-4159.2005.03255.x
56. Paul BD, Sbodio JI, Xu R, Vandiver MS, Cha JY, Snowman AM, et al. Cystathionine gamma-lyase deficiency mediates neurodegeneration in Huntington's disease. Nature. (2014) 509:96–100. doi: 10.1038/nature13136
57. Sbodio JI, Snyder SH, Paul BD. Transcriptional control of amino acid homeostasis is disrupted in Huntington's disease. Proc Natl Acad Sci USA. (2016) 113:8843–8. doi: 10.1073/pnas.1608264113
58. Paul BD, Sbodio JI, Snyder SH. Cysteine metabolism in neuronal redox homeostasis. Trends Pharmacol Sci. (2018) 39:513–24. doi: 10.1016/j.tips.2018.02.007
59. Paul BD, Snyder SH. H(2)S signalling through protein sulfhydration and beyond. Nat Rev Mol Cell Biol. (2012) 13:499–507. doi: 10.1038/nrm3391
60. Paul BD, Snyder SH. Modes of physiologic H2S signaling in the brain and peripheral tissues. Antioxid Redox Signal. (2015) 22:411–23. doi: 10.1089/ars.2014.5917
61. Paul BD, Snyder SH. Gasotransmitter hydrogen sulfide signaling in neuronal health and disease. Biochem Pharmacol. (2018) 149:101–9. doi: 10.1016/j.bcp.2017.11.019
62. Ryu H, Lee J, Hagerty SW, Soh BY, McAlpin SE, Cormier KA, et al. ESET/SETDB1 gene expression and histone H3 (K9) trimethylation in Huntington's disease. Proc Natl Acad Sci USA. (2006) 103:19176–81. doi: 10.1073/pnas.0606373103
63. Dubinsky R, Gray C. CYTE-I-HD: phase I dose finding and tolerability study of cysteamine (Cystagon) in Huntington's disease. Mov Disord. (2006) 21:530–3. doi: 10.1002/mds.20756
64. Borrell-Pages M, Canals JM, Cordelieres FP, Parker JA, Pineda JR, Grange G, et al. Cystamine and cysteamine increase brain levels of BDNF in Huntington disease via HSJ1b and transglutaminase. J Clin Invest. (2006) 116:1410–24. doi: 10.1172/JCI27607
65. Arbez N, Roby E, Akimov S, Eddings C, Ren M, Wang X, et al. Cysteamine protects neurons from mutant huntingtin toxicity. J Huntingtons Dis. (2019) 8:129–43. doi: 10.3233/JHD-180312
66. Masters CL, Bateman R, Blennow K, Rowe CC, Sperling RA, Cummings JL. Alzheimer's disease. Nat Rev Dis Primers. (2015) 1:15056. doi: 10.1038/nrdp.2015.56
67. Lane CA, Hardy J, Schott JM. Alzheimer's disease. Eur J Neurol. (2018) 25:59–70. doi: 10.1111/ene.13439
68. Balasubramanian AB, Kawas CH, Peltz CB, Brookmeyer R, Corrada MM. Alzheimer disease pathology and longitudinal cognitive performance in the oldest-old with no dementia. Neurology. (2012) 79:915–21. doi: 10.1212/WNL.0b013e318266fc77
69. Serrano-Pozo A, Qian J, Monsell SE, Blacker D, Gomez-Isla T, Betensky RA, et al. Mild to moderate Alzheimer dementia with insufficient neuropathological changes. Ann Neurol. (2014) 75:597–601. doi: 10.1002/ana.24125
70. Beach TG, Monsell SE, Phillips LE, Kukull W. Accuracy of the clinical diagnosis of Alzheimer disease at National Institute on Aging Alzheimer Disease Centers, 2005-2010. J Neuropathol Exp Neurol. (2012) 71:266–73. doi: 10.1097/NEN.0b013e31824b211b
71. Cicchetti F, David LS, Siddu A, Denis HL. Cysteamine as a novel disease-modifying compound for Parkinson's disease: Over a decade of research supporting a clinical trial. Neurobiol Dis. (2019) 130:104530. doi: 10.1016/j.nbd.2019.104530
72. Radde R, Bolmont T, Kaeser SA, Coomaraswamy J, Lindau D, Stoltze L, et al. Abeta42-driven cerebral amyloidosis in transgenic mice reveals early and robust pathology. EMBO Rep. (2006) 7:940–6. doi: 10.1038/sj.embor.7400784
73. Minarini A, Milelli A, Tumiatti V, Rosini M, Simoni E, Bolognesi ML, et al. Cystamine-tacrine dimer: a new multi-target-directed ligand as potential therapeutic agent for Alzheimer's disease treatment. Neuropharmacology. (2012) 62:997–1003. doi: 10.1016/j.neuropharm.2011.10.007
74. Stack EC, Ferro JL, Kim J, Del Signore SJ, Goodrich S, Matson S, et al. Therapeutic attenuation of mitochondrial dysfunction and oxidative stress in neurotoxin models of Parkinson's disease. Biochim Biophys Acta. (2008) 1782:151–62. doi: 10.1016/j.bbadis.2007.12.006
75. Tremblay ME, Saint-Pierre M, Bourhis E, Levesque D, Rouillard C, Cicchetti F. Neuroprotective effects of cystamine in aged parkinsonian mice. Neurobiol Aging. (2006) 27:862–70. doi: 10.1016/j.neurobiolaging.2005.04.004
76. Taylor JP, Brown RHJr, Cleveland DW. Decoding ALS: from genes to mechanism. Nature. (2016) 539:197–206. doi: 10.1038/nature20413
77. Rosen DR, Siddique T, Patterson D, Figlewicz DA, Sapp P, Hentati A, et al. Mutations in Cu/Zn superoxide dismutase gene are associated with familial amyotrophic lateral sclerosis. Nature. (1993) 362:59–62. doi: 10.1038/362059a0
78. Gurney ME, Pu H, Chiu AY, Dal Canto MC, Polchow CY, Alexander DD, et al. Motor neuron degeneration in mice that express a human Cu,Zn superoxide dismutase mutation. Science. (1994) 264:1772–5. doi: 10.1126/science.8209258
79. Lazarev VF, Sverchinskyi DV, Ippolitova MV, Stepanova AV, Guzhova IV, Margulis BA. Factors affecting aggregate formation in cell models of huntington's disease and amyotrophic lateral sclerosis. Acta Nat. (2013) 5:81–9. doi: 10.32607/20758251-2013-5-2-81-89
80. Ballantyne AO, Trauner DA. Neurobehavioral consequences of a genetic metabolic disorder: visual processing deficits in infantile nephropathic cystinosis. Neuropsychiatry Neuropsychol Behav Neurol. (2000) 13:254–63.
81. Aly R, Makar S, El Bakri A, Soliman NA. Neurocognitive functions and behavioral profiles in children with nephropathic cystinosis. Saudi J Kidney Dis Transpl. (2014) 25:1224–31. doi: 10.4103/1319-2442.144256
82. Trauner D. Neurocognitive complications of cystinosis. J Pediatr. (2017) 183S:S15–8. doi: 10.1016/j.jpeds.2016.12.054
83. Viltz L, Trauner DA. Effect of age at treatment on cognitive performance in patients with cystinosis. J Pediatr. (2013) 163:489–92. doi: 10.1016/j.jpeds.2013.01.027
84. Frankel AM, Trauner DA. Visual and verbal learning and memory in cystinosis. Brain Cogn. (2019) 135:103578. doi: 10.1016/j.bandc.2019.103578
85. Kahn RS, Sommer IE, Murray RM, Meyer-Lindenberg A, Weinberger DR, Cannon TD, et al. Schizophrenia. Nat Rev Dis Primers. (2015) 1:15067. doi: 10.1038/nrdp.2015.67
86. Buckley PF, Miller BJ, Pillai A, Kirkpatrick B. Cysteamine, a pro-BDNF drug, as an adjunctive treatment for schizophrenia. Schizophr Res. (2014) 158:268–9. doi: 10.1016/j.schres.2014.06.030
87. Pae CU, Lee C, Paik IH. Therapeutic possibilities of cysteamine in the treatment of schizophrenia. Med Hypotheses. (2007) 69:199–202. doi: 10.1016/j.mehy.2006.10.045
88. Rakovska A, Javitt D, Petkova-Kirova P, Balla A, Ang R, Kalfin R. Neurochemical evidence that cysteamine modulates amphetamine-induced dopaminergic neuronal activity in striatum by decreasing dopamine release: an in vivo microdialysis study in freely moving rats. Brain Res Bull. (2019) 153:39–46. doi: 10.1016/j.brainresbull.2019.08.005
89. Pillai A, Veeranan-Karmegam R, Dhandapani KM, Mahadik SP. Cystamine prevents haloperidol-induced decrease of BDNF/TrkB signaling in mouse frontal cortex. J Neurochem. (2008) 107:941–51. doi: 10.1111/j.1471-4159.2008.05665.x
90. Shieh CH, Hong CJ, Huang YH, Tsai SJ. Potential antidepressant properties of cysteamine on hippocampal BDNF levels and behavioral despair in mice. Prog Neuropsychopharmacol Biol Psychiatry. (2008) 32:1590–4. doi: 10.1016/j.pnpbp.2008.06.003
91. Feifel D, Minor KL. Cysteamine blocks amphetamine-induced deficits in sensorimotor gating. Pharmacol Biochem Behav. (1997) 58:689–93. doi: 10.1016/S0091-3057(97)00020-8
92. Kutiyanawalla A, Promsote W, Terry A, Pillai A. Cysteamine treatment ameliorates alterations in GAD67 expression and spatial memory in heterozygous reeler mice. Int J Neuropsychopharmacol. (2012) 15:1073–86. doi: 10.1017/S1461145711001180
93. Crider A, Davis T, Ahmed AO, Mei L, Pillai A. Transglutaminase 2 induces deficits in social behavior in mice. Neural Plast. (2018) 2018:2019091. doi: 10.1155/2018/2019091
94. Chahrour M, Zoghbi HY. The story of Rett syndrome: from clinic to neurobiology. Neuron. (2007) 56:422–37. doi: 10.1016/j.neuron.2007.10.001
95. Roux JC, Zala D, Panayotis N, Borges-Correia A, Saudou F, Villard L. Modification of Mecp2 dosage alters axonal transport through the Huntingtin/Hap1 pathway. Neurobiol Dis. (2012) 45:786–95. doi: 10.1016/j.nbd.2011.11.002
96. Kutiyanawalla A, Terry AV Jr, Pillai A. Cysteamine attenuates the decreases in TrkB protein levels and the anxiety/depression-like behaviors in mice induced by corticosterone treatment. PLoS ONE. (2011) 6:e26153. doi: 10.1371/journal.pone.0026153
97. Prundean A, Youssov K, Humbert S, Bonneau D, Verny C. A phase II, open-label evaluation of cysteamine tolerability in patients with Huntington's disease. Mov Disord. (2015) 30:288–9. doi: 10.1002/mds.26101
98. Steinhubl SR. Why have antioxidants failed in clinical trials? Am J Cardiol. (2008) 101:14D−9D. doi: 10.1016/j.amjcard.2008.02.003
99. Persson T, Popescu BO, Cedazo-Minguez A. Oxidative stress in Alzheimer's disease: why did antioxidant therapy fail? Oxid Med Cell Longev. (2014) 2014:427318. doi: 10.1155/2014/427318
100. Kim GH, Kim JE, Rhie SJ, Yoon S. The role of oxidative stress in neurodegenerative diseases. Exp Neurobiol. (2015) 24:325–40. doi: 10.5607/en.2015.24.4.325
101. Ohlow MJ, Sohre S, Granold M, Schreckenberger M, Moosmann B. Why have clinical trials of antioxidants to prevent neurodegeneration failed? - A cellular investigation of novel phenothiazine-type antioxidants reveals competing objectives for pharmaceutical neuroprotection. Pharm Res. (2017) 34:378–93. doi: 10.1007/s11095-016-2068-0
102. Underwood BR, Imarisio S, Fleming A, Rose C, Krishna G, Heard P, et al. Antioxidants can inhibit basal autophagy and enhance neurodegeneration in models of polyglutamine disease. Hum Mol Genet. (2010) 19:3413–29. doi: 10.1093/hmg/ddq253
103. De Stefano D, Villella VR, Esposito S, Tosco A, Sepe A, De Gregorio F, et al. Restoration of CFTR function in patients with cystic fibrosis carrying the F508del-CFTR mutation. Autophagy. (2014) 10:2053–74. doi: 10.4161/15548627.2014.973737
Keywords: BDNF, brain, cystamine, cysteamine, cysteine, neurodegeneration, neuropsychiatric disorder, redox
Citation: Paul BD and Snyder SH (2019) Therapeutic Applications of Cysteamine and Cystamine in Neurodegenerative and Neuropsychiatric Diseases. Front. Neurol. 10:1315. doi: 10.3389/fneur.2019.01315
Received: 10 September 2019; Accepted: 27 November 2019;
Published: 12 December 2019.
Edited by:
Carlos Alberto Manssour Fraga, Federal University of Rio de Janeiro, BrazilReviewed by:
Marzia Perluigi, Sapienza University of Rome, ItalyWillayat Yousuf Wani, Northwestern University, United States
Copyright © 2019 Paul and Snyder. This is an open-access article distributed under the terms of the Creative Commons Attribution License (CC BY). The use, distribution or reproduction in other forums is permitted, provided the original author(s) and the copyright owner(s) are credited and that the original publication in this journal is cited, in accordance with accepted academic practice. No use, distribution or reproduction is permitted which does not comply with these terms.
*Correspondence: Bindu D. Paul, bpaul8@jhmi.edu