- 1Department of Neurology, University Medicine Rostock, Rostock, Germany
- 2Institute of Diagnostic and Interventional Radiology, University Medicine Rostock, Rostock, Germany
- 3DZNE, German Centre for Neurodegenerative Diseases, Rostock, Germany
Objective: Amyotrophic Lateral Sclerosis (ALS) is a neurodegenerative disorder predominantly affecting the motor system. In a number of patients, mirror movements (MMs) suggest involvement of transcallosal fiber tracts in conjunction with upper motor neuron involvement. The aim of the study was to elucidate functional and structural alterations of callosal integrity in ALS patients with MMs.
Methods: Nineteen patients with ALS displaying MMs and 20 controls underwent clinical assessment, transcranial magnetic stimulation (TMS), and diffusion tensor imaging (DTI). TBSS (tract based spatial statistics) was performed. We investigated ipsilateral silent period (iSP) as a measure of transcallosal inhibition, and diffusion changes in the corpus callosum and corticospinal tract (CST) as measure of structural integrity.
Results: In ALS patients TMS revealed a longer mean iSP latency than controls. Twelve ALS patients (63.2%) showed loss of iSP, but none of the controls. Using region of interest analysis, fractional anisotropy (FA) values of the CST were significantly lower in ALS patients compared with controls, but diffusion parameters of the corpus callosum did not differ between patients and controls. The lack of diffusion changes in the corpus callosum was confirmed in whole brain tract based statistics, assessing FA as well as mean, radial, and axial diffusivity. There was a significant negative correlation between resting motor threshold and FA values of the CST, but not between iSP and FA of the corpus callosum.
Conclusion: In conclusion the study failed to show microstructural changes in the corpus callosum in conjunction with MMs. One possible reason may be that functional disturbance of transcallosal pathways precede microstructural changes in the corpus callosum.
Introduction
Amyotrophic lateral sclerosis (ALS) is a progressive neurodegenerative disease mainly characterized by a motor syndrome with variable expression of lower (LMN) and upper (UMN) motor neuron dysfunction. El Escorial criteria (1) require UMN and LMN involvement in one or more body regions for making the diagnosis of definitive ALS. UMN signs may be spasticity, enhanced or preserved tendon reflexes and extensor plantar response. Mirror movements (MMs) have repeatedly been reported in ALS (2–4), but are still not part of the diagnostic criteria. MMs are involuntary movements contralaterally to an intended finger movement. MMs can be seen in normal children up to 10 years of age, but their prevalence and intensity declines after this age (5–7) most likely reflecting maturation of the corpus callosum (8). Persistence or novel manifestation of MMs in adults can arise from a variety of etiologies. Persistent congenital MMs have been described in different conditions ranging from the absence of other neurological abnormalities to severe congenital hemiparesis in cerebral palsy (9). MMs also have been reported in a variety of other acquired conditions such as Parkinson's disease (PD) (10, 11) or stroke (12, 13). Two main hypothesizes for the development of MMs were discussed: abnormal development of the primary motor system, involving the ipsilateral corticospinal tract, and lack of contralateral motor cortex inhibitory mechanisms, mainly through the corpus callosum (9, 14). Pathophysiological basis of MMs in all these acquired clinical conditions is thought to be the result of a predominant alteration of callosal projecting pathways. Nevertheless, an additional or pre-existing cortical or pyramidal malfunctioning appears to be necessary for the development of MMs (2). Moreover, MMs have been found to be associated with reduced transcallosal inhibition (TI) as measured by transcranial magnetic stimulation (TMS) in ALS, PD, and stroke (3, 4, 15–17). Regardless of the exact pathophysiological mechanism of MMs, the most important clinical aspect is that they are reflecting CNS involvement by the underlying pathological condition. Diffusion MRI techniques, such as diffusion tensor imaging (DTI) have been established to study integrity of neuronal tracts in vivo in the human brain. DTI studies found alterations of fiber tract integrity in neurodegenerative diseases like Alzheimer's disease and ALS (18–21), including callosal involvement (22–24). An observational study found a consistent reduction in fractional anisotropy in the corpus callosum of ALS patients, extending rostrally, and bilaterally to the region of the primary motor cortices (23). A more recent study using DTI and TMS for characterization of neurodegeneration in ALS and concluded a complementary role as diagnostic biomarkers of UMN dysfunction (25). Ellis and colleagues found a significant increase in the mean diffusivity and reduction of fractional anisotropy along the corticospinal tract in ALS patients with correlation to disease severity and UMN involvement (26). None of these studies focused on MMs or functional connectivity in ALS.
Therefore, we studied associations of functional TMS measures with diffusion markers of structural integrity of the corpus callosum and cerebrospinal tract in a prospective sample of ALS patients displaying MMs to elucidate the pathophysiological concepts of MMs in ALS. We hypothesized that ALS patients with MMs would show impaired functional integrity of the corpus callosum, associated with decline of structural integrity markers. Such findings would support the role of callosal dysfunction and structural impairment for MMs in ALS.
Patients and Methods
Subjects
Nineteen patients with ALS and 20 healthy control subjects underwent clinical assessment, and MRI examinations, including DTI. Detailed demographic date and clinical characteristic of the entire study cohort are shown in Table 1. All subjects were right-handed as assessed by the Edinburgh handedness inventory (27). At the time point of examination 4 patients showed definitive ALS, 8 probable ALS and 4 possible ALS according to the revised El Escorial criteria (1). Three patients displayed lower motor neuron variant. Mean disease duration was 34.8 ± 34.8 months. Clinical assessment consisted of neurological examination with special respect of handedness; MMs were evaluated by sequential finger tapping of one hand without optic control and observation of MMs according to the procedure of Woods and Teuber (28), and the evaluation of the revised ALS functional rating scale (-R) (29). Furthermore, to address the upper motor neuron (UMN) involvement an UMN “burden” (UMNB) was calculated by totalling the number of pathological UMN signs on examination (maximum score 16) (30). For cognitive screening we used the “Montreal Cognitive assessment” (MOCA) (31). The MOCA score ranged between 18 and 29 with an average of 24.5 ± 4 for the ALS patients, and between 27 and 29 with an average of 27.6 ± 0.7 for the controls (P = 0.006). Controls did not have cognitive complaints and scored within 1.5 standard deviations of the MOCA age and education adjusted norm value.
Patients and control individuals were only included in the study if written consent was given. The study was approved by the Institutional Review Board of the Medical Faculty, University of Rostock (A-2011-0026, A 2012-0083).
Transcranial Magnetic Stimulation
We performed TMS in all patients and in 10 of 20 healthy controls. Central motor conduction time (CMCT), motor evoked potentials (MEP) amplitudes, and contralateral silent period (cSP) were determined in all ALS patients and control subjects. MEPs were recorded from the first dorsal interosseus muscle (FDI) and from the anterior tibial muscle (TA) using a standard circular coil (outside diameter 9 cm) connected to a Magstim 200 stimulator (Magstim Co., Whitland, Dyfeld, UK). For data acquisition a commercially available MEP system was employed (Brain Quick System Plus, Inomed, Erlangen, Germany). Investigation of the ipsilateral silent period (iSP) was performed with a focal coil (external loop diameter 7 cm). The coil was oriented to induce a posterior-anteriorly directed current flow to the hand area of the motor cortex; the point of optimal excitability (POE) of the FDI muscle was determined over the contralateral motor cortex; TMS was applied at the POE with 1.5 times resting motor threshold (RMT) while subjects performed a maximum tonic activation of the ipsilateral FDI muscle and while they kept the contralateral FDI muscle relaxed as published in detail previously (3). For determination of iSP latency and duration 10 trials for each hemisphere were used. After offline rectification and averaging of EMG signals, iSP parameters were analyzed.
MRI Acquisition
MRI acquisitions of the brain were conducted using a 3-Tesla MRI scanner with a 32-channel phased-array head coil and parallel imaging capabilities (Magnetom Verio, Siemens, Erlangen, Germany, software syngo MR B17). Subjects were scanned in a single session without changing their position in the scanner. The following sequences were used: We acquired a sagittal high-resolutionT1-weighted magnetization prepared rapidly acquired gradient echo (MP-RAGE) 3D-sequence, matrix size of 256 × 256 × 192, isometric voxel size 1.0 mm3), TE/TI/TR of 4.82 ms/1,100 ms/2,500 ms, flip angle 7°. To identify white matter lesions a two-dimensional T2-weighted sequence was performed (fluid attenuation inversion recovery FLAIR, matrix size of 384 × 187, 24 slices with slice thickness of 5.0 mm, TE/TI/TR of 94 ms/2,500 ms/9,000 ms, flip angle 150°). Diffusion-weighted imaging was performed with an echo-planar-imaging sequence (TE/TR 81 ms/12,700 ms) Diffusion gradients were applied in 30 different spatial directions. The b values were 0 and 1,000 s/mm2. The images had a matrix size of 128 × 128 mm, slice thickness of 2 mm, the resulting voxel size was 2.0 × 2.0 × 2.0 mm2.
MRI Data Processing
DTI data were pre-processed using the DTI tool box of the FSL software (http://www.fmrib.ox.ac.uk/fsl/, FMRIB, Oxford, UK, Version 4.1) (32). We first applied corrections for eddy currents and head motion. The skull was stripped using Brain Extraction Tool for differentiation of brain tissue and non-brain tissue using a binary mask (threshold 0.15–0.3) and the diffusion tensors were fitted to the data with DTI fit (FMRIB Image Analysis Group, Oxford, UK). Fractional anisotropy (FA), and mean diffusivity (MD) maps as well as axial diffusivity (AD) and radial diffusivity (RD) maps were created.
TBSS analysis (tract based spatial statistics) were performed using FMRIB software (version 4.1 www.fmrib.ox.ac.uk/fsl) (33). TBSS allows spatial reorientation of FA maps into a standard space without systematic effects of spatial transformation on fiber tract directionality and without the need to select a spatial smoothing kernel that may impact upon the desired effects (22). Processing of diffusivity maps consisted of the following steps: FA maps were transformed to MNI (Montreal Neurologic Institute) space. Normalized FA maps of all subjects were averaged and skeletonized with a standardized threshold of 0.2. Based on the study specific with matter skeletons an individual skeleton for each subject was created.
For specific analysis of changes in diffusivity of individual fiber tracts we conducted a region of interest (ROI) analysis. The selection of ROIs from JHU was done as described before (34). Based on the JHU white matter atlas (35) we defined seven regions: corpus callosum with subregions genu, truncus, and splenium, corticospinal tract, and crus posterior of the internal capsula on both sides. Data processing was done by Matlab software 2013a (MathWorks Inc., MA, Natrick, USA) (Figure 1).
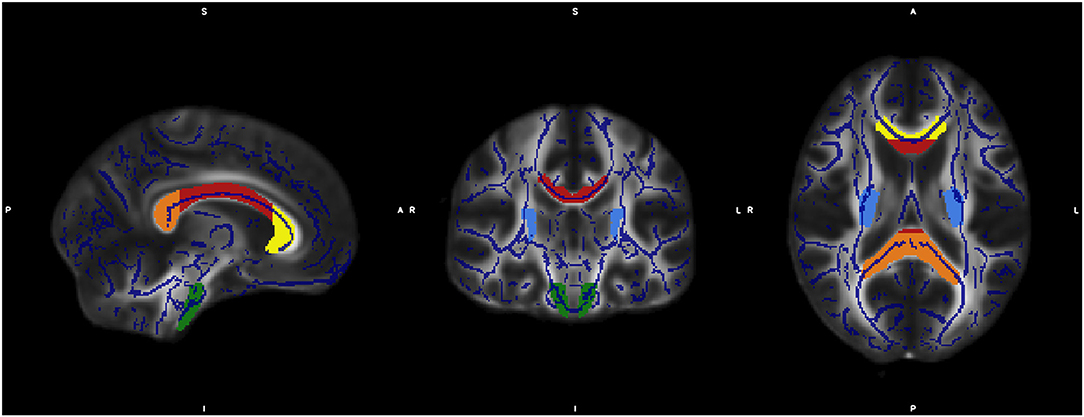
Figure 1. The figure shows the overlap of the mean FA skeleton map of our sample (blue) and the normalized MNI-FA template and the defined regions of interests based on JHU White matter atlas: genu (yellow), body (red), and Splenium (orange) of Corpus callosum, CST both sites (green) and crus posterior of the internal capsula on both sides (light blue).
For analysis of correlation between TMS values and DTI measures we performed a region of interest (ROI) analysis using MarsBaR (36) to extract mean values from corpus callosum and left and right corticospinal tract, respectively.
Statistical Analysis
Statistical analysis was conducted for the clinical scores, TMS measurements and ROI data in SPSS (version IBM SPSS statistics 20). For the whole brain analysis using the tool box “Randomize” under TBSS in FMRIB (version 4.1 www.fmrib.ox.ac.uk/fsl) was used. All clinical scores and TMS parameters showed a normal distribution, which was tested by the use of Kolmogorov Smirnov test (P > 0.1). Differences in TMS parameters were compared between groups using Student's t-test. The results of iSP measurements were dichotomized in pathological vs. non-pathological, and analyzed using Chi2 test.
For ROI analysis, group comparisons of FA of patients and controls were performed according to the findings of pathological iSP and non-pathological iSP in an univariate variance analysis and subsequent post-hoc tests. Furthermore, correlations between iSP findings and ALSFRS and FA parameters of all investigated ROIs was calculated. For correlation analysis Spearman-Rank-correlation were used and significance was set at P < 0.05.For whole brain analysis, we investigated group differences in fractional anisotropy and mean diffusivity using the general linear model on a voxel basis. Whole brain analyses were performed for all diffusion parameters. FA, MD, RD, and AD maps were compared by univariate variance analysis (factor: group, covariate: ALSFRS-R and UMNB). To avoid overestimation of significance, a test of multiple comparisons was applied (family wise error method) and significance was set at P < 0.05.
Results
TMS Results
TMS investigation in ALS patients revealed a mean iSP latency of 41.3 ± 5.4 ms/40.9 ± 5.7 ms (right/left), which was longer than that of the healthy controls 39.0 ± 4.6/37.7 ± 6.3 (right/left) (p = 0.006/0.012). No differences were found for the iSP duration between the ALS patients and control subjects. Twelve ALS patients (63.2%), but none of the controls showed loss of iSP. cSP was numerically shorter in ALS patients than in controls without reaching significance. ALS patients had a significantly lower mean RMT compared to controls (TMS results are shown in detail in Table 2 and Figure 2).
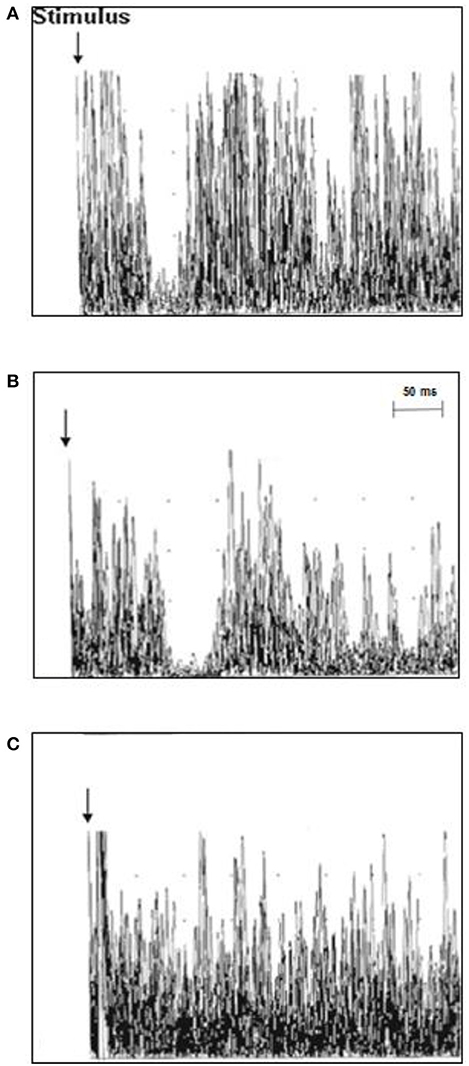
Figure 2. Ipsilateral muscle responses (10 rectified and superimposed EMG-traces) recorded from the first dorsal interosseous muscle (FDI) in individual ALS patients and controls. (A) The latency of the iSP was normal in control case 3 (37.8 ms), (B) the iSP latency was prolonged in ALS patient case 11 (51 ms), and (C) ALS patient case 18 displayed a loss of the iSP.
Group Differences in Fractional Anisotropy and Mean Diffusivity
Concerning the analyses of pre-specified ROIs FA values of the CST were significantly lower in ALS patients compared with controls. FA values of the different ROIs of the corpus callosum (genu, splenium, and body) did not differ between both groups (Table 3). AD and RD values showed similar findings. Whole brain analysis did not show any differences in FA, MD, RD, and AD between ALS patients and controls at an FWE corrected level of significance.
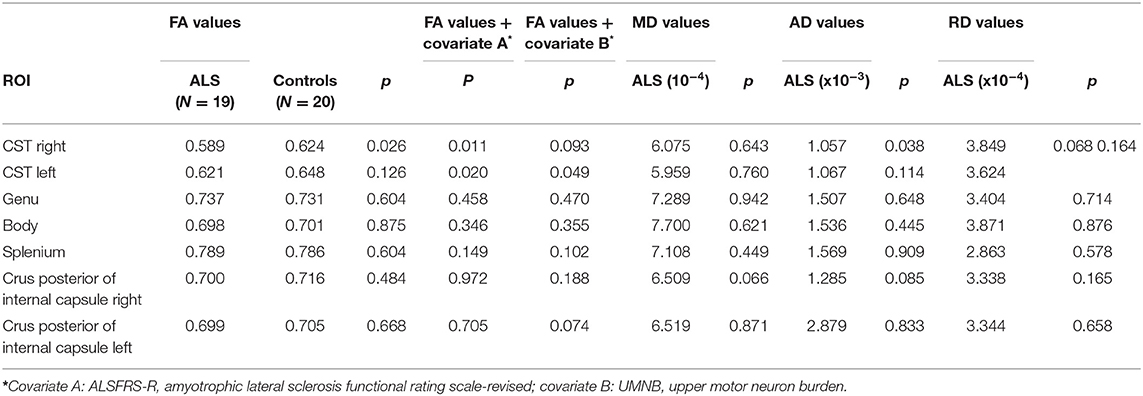
Table 3. DTI findings in ALS patients and controls [fractional anisotropy (FA), mean diffusivity (MD), axial diffusivity (AD), and radial diffusivity (RD)].
Correlation of Clinical Parameter, TMS Parameters, and DTI Measures
We found significant negative correlations of FA values of the CST with RMT values on both sides in ALS patients, but not in controls (Table 4). There was no significant correlation between FA values and iSP parameters (Table 4). In contrast, there was a significant correlation of ALS-FRS-R scores with FA values of the CST on both sides (r CST-right, ALS-FRS-R = 0.58, p = 0.009 and r CST-left, LS-FRS-R = 0.05, p = 0.02), but not with FA values of the corpus callosum.
Discussion
Lower and upper motor neuron dysfunction is a clinical hallmark of ALS. Besides involvement of primary motor areas, an early callosal dysfunction has been suggested in ALS as well (37). MMs may be a clinical sign of dysfunctional transcallosal pathways and could be observed in ~30% of ALS patients. Further evidence for the interpretation of MMs as markers of callosal dysfunction comes from a range of TMS studies showing callosal dysfunction as indicated by impaired transcallosal inhibition (2–4, 15) in conjunction with the presence of MMs in ALS and other conditions like Parkinson's disease (16). Furthermore, several DTI studies have shown microstructural changes not only in the pyramidal tract, but also in the corpus callosum in ALS (22, 23, 38). Callosal involvement has been demonstrated to be a relatively consistent feature of ALS, even without clinical UMN involvement, and may reflect interhemispheric spread of pathology and an impaired structural motor connectivity (23, 24).
In the current study we expected a significant correlation of DTI measures and TMS findings reflecting transcallosal inhibition in ALS patients with MMs. Consistent with our expectation, we found an impaired transcallosal inhibition in up to 68% of cases which is in line with previously published data from independent cohorts (3, 4). In contrast, however, ALS patients with MMs in the current sample did not show microstructural involvement of the corpus callosum, which is in contrast with some (22, 34), but not all previous studies (39). One interpretation of the lack of corpus callosum changes in our study may be that our patients exhibited an earlier functional disturbance potentially preceding the microstructural findings in transcallosal pathways. Thus, the two previous studies finding corpus callous diffusion changes (23, 41) included functionally more advanced cases (mean ALSFRS-R score 33.1 and 36.5, respectively, compared with 38.5 in the current study). A recent study demonstrated increased AD in the corpus callosum and reduction of FA in the right CST in ALS without considering MMs (25). The study of Geraldo et al. had no focus on electrophysiological changes in the transcallosally projecting pathways but cortical excitability and CST conduction properties were tested by investigation of the resting motor threshold (RMT) as measure of cortical excitability and the central motor conduction time (CMCT). Keeping in mind that Geraldo and co-workers used a different small hand muscle as target muscle (abductor digiti minimi vs. first dorsal interosseus muscle in our study) RMT was slightly lower in our study reflecting a greater cortical excitability in our cohort displaying MMs. CMCT measurements displayed comparable values to our study. Extending these previous findings to ALS patients with MMs, we could replicate FA reduction of the CST associated with TMS changes in ALS, without effects in the corpus callosum. Another explanation of the lack of significance of DTI findings in the corpus callosum in our sample might be the relative great heterogeneity of patients, which represents one limitation of our study. Another limitation is the low number of patients. A major reason for this circumstance might be the relative rarity of MMs, which could be observed in just approximately 30% of ALS patients. Because of limitations in available staff resource the study period could not be extended.
In conclusion, this is the first study investigating a cohort of ALS patients which all were displaying MMs. The present study replicates and extends previous findings on reduced fiber tract integrity in patients with ALS with MMs, but failed to show microstructural changes accompanying mirror movements and disturbed transcallosal inhibition. The affection of the pyramidal tract appears to be an important precondition for development of MMs. This study adds further evidence for the understanding of impaired connectivity in ALS patients. Therefore, further studies in bigger and more homogeneous cohorts are needed.
Data Availability Statement
Deidentified participant data will be shared, as well as the study protocol and statistical analyses, upon reasonable requests.
Ethics Statement
The studies involving human participant were reviewed and approved by the Institutional Review Board of the Medical Faculty, University of Rostock (A-2011-0026, A267 2012-0083). The patients/participants provided their written consent to participate in this study.
Author Contributions
MW: conception, design, drafting of manuscript, acquisition of data, and final approval of manuscript. NW, AG, and EK: acquisition of data, revision of manuscript, and final approval of manuscript. ST: concept, design, analysis, and interpretation of data, drafting of manuscript, revision of manuscript, and final approval of manuscript.
Conflict of Interest
ST has done the listed works below (all in Germany): MSD Sharp & Dohme GmbH, Lindenplatz 1, 85540 Haar
11.09.2018—Quality circle for physicians in Kühlungsborn, Talk: “Dementia and Diabetes—current report”
14.11.2018—MSD Expert-forum: NAB Alzheimer in Munich, participator as consultant
13.08.2019—Event “Diabetes and Dementia” in Rostock, Talk: “Dementia and Diabetes—current report” ROCHE Pharma AG, Emil-Barell-Str. 1, 79639 Grenzach-Wyhlen
12.09.2019-−3. National Advisory-Board in Frankfurt am Main, participation as consultant
27.09.2019—ROCHE Symposium at the DGN Congress in Stuttgart, Talk: “Amyloid as target for diagnosis and treatment in Alzheimer's disease.”
The remaining authors declare that the research was conducted in the absence of any commercial or financial relationships that could be construed as a potential conflict of interest.
References
1. Brooks BR, Miller RG, Swash M, Munsat TL. El escorial revisited: revised criteria for the diagnosis of amyotrophic lateral sclerosis. Amyotroph Lateral Scler Other Motor Neuron Disord. (2000) 1:293–9. doi: 10.1080/146608200300079536
2. Krampfl K, Mohammadi B, Komissarow L, Dengler R, Bufler J. Mirror movements and ipsilateral motor evoked potentials in ALS. Amyotroph Lateral Scler Other Motor Neuron Disord. (2004) 5:154–63. doi: 10.1080/14660820410019657
3. Wittstock M, Wolters A, Benecke R. Transcallohsal inhibition in amyotrophic lateral sclerosis. Clin. Neurophysiol. (2007) 118:301–7. doi: 10.1016/j.clinph.2006.09.026
4. Wittstock M, Meister S, Walter U, Benecke R, Wolters A. Mirror movements in amyotrophic lateral sclerosis. Amyotroph Lateral Scler. (2011) 6:393–7. doi: 10.3109/17482968.2011.577223
5. Reitz M, Müller K. Differences between 'congenital mirror movements' and 'associated movements' in normal children: a neurophysiological case study. Neurosci Lett. (1998) 256:69–72. doi: 10.1016/S0304-3940(98)00748-4
6. Mayston MJ, Harrison LM, Stephens JA. A neurophysiological study of mirror movements in adults and children. Ann Neurol. (1999) 45:583–94. doi: 10.1002/1531-8249(199905)45:5<583::AID-ANA6>3.0.CO;2-W
7. Koerte I, Eftimov L, Laubender RP, Esslinger O, Schroeder AS, Ertl-Wagner B, et al. Mirror movements in healthy humans across the lifespan: effects of development and ageing. Dev Med Child Neurol. (2010) 52:1106–12. doi: 10.1111/j.1469-8749.2010.03766.x
8. Müller K, Kass-Iliya F, Reitz M. Ontogeny of ipsilateral corticospinal projections: a developmental study with transcranial magnetic stimulation. Ann Neurol. (1997) 42:705–71. doi: 10.1002/ana.410420506
9. Cincotta M, Ziemann U. Neurophysiology of unimanual motor control and mirror movements. Clin Neurophysiol. (2008) 119:744–62. doi: 10.1016/j.clinph.2007.11.047
10. Van den Berg C, Beek PJ, Wagenaar RC, van Wieringen PC. Coordination disorders in patients with Parkinson's disease: a study of paced rhythmic forearm movements. Exp Brain Res. (2000) 134:174–86. doi: 10.1007/s002210000441
11. Espay AJ, Li JY, Johnston L, Chen R, Lang AE. Mirror movements in parkinsonism: evaluation of a new clinical sign. J Neurol Neurosurg Psychiatry. (2005) 76:1355–8. doi: 10.1136/jnnp.2005.062950
12. Weiller C, Ramsay SC, Wise RJ, Friston KJ, Frackowiak RS. Individual patterns of functional reorganization in the human cerebral cortex after capsular infarction. Ann Neurol. (1993) 33:181–9. doi: 10.1002/ana.410330208
13. Netz J, Lammers T, Hömberg V. Reorganization of motor output in the non-affected hemisphere after stroke. Brain. (1997) 120:1579–86. doi: 10.1093/brain/120.9.1579
14. Manara R, Salvalaggio A, Citton V, Palumbo V, D'Errico A, Elefante A, et al. Brain anatomical substrates of mirror movements in Kallmann syndrome. Neuroimage. (2015) 104:52–8. doi: 10.1016/j.neuroimage.2014.09.067
15. Karandreas N, Papadopoulou M, Kokotis P, Papapostolou A, Tsivgoulis G, Zambelis T. Impaired interhemispheric inhibition in amyotrophic lateral sclerosis. Amyotroph Lateral Scler. (2007) 8:112–8. doi: 10.1080/17482960601030113
16. Li JY, Espay AJ, Gunraj CA, Pal PK, Cunic DI, Lang AE, et al. Interhemispheric and ipsilateral connections in Parkinson's disease: relation to mirror movements. Mov Disord. (2007) 22:813–21. doi: 10.1002/mds.21386
17. Rocca MA, Mezzapesa DM, Comola M, Leocani L, Falini A, Gatti R, et al. Persistence of congenital mirror movements after hemiplegic stroke. AJNR Am J Neuroradiol. (2005) 26:831–4.
18. Teipel SJ, Wegrzyn M, Meindl T, Frisoni G, Bokde AL, Fellgiebel A, et al. EDSD study group. anatomical MRI and DTI in the diagnosis of Alzheimer's disease: a European multicenter study. J Alzheimers Dis. (2012) 31(Suppl. 3):S33–47. doi: 10.3233/JAD-2012-112118
19. Turner MR, Grosskreutz J, Kassubek J, Abrahams S, Agosta F, Benatar M, et al. First neuroimaging symosium in ALS (NISALS). Towards a neuroimaging biomarker for amyotrophic lateral sclerosis. Lancet Neurol. (2011) 10:400–3. doi: 10.1016/S1474-4422(11)70049-7
20. Müller HP, Turner MR, Grosskreutz J, Abrahams S, Bede P, Govind V, et al. Neuroimaging society in ALS (NiSALS) DTI study group. a large-scale multicentre cerebral diffusion tensor imaging study in amyotrophic lateral sclerosis. J Neurol Neurosurg Psychiatry. (2016) 87:570–9. doi: 10.1136/jnnp-2015-311952
21. Kassubek J, Müller HP, Del Tredici K, Lulé D, Gorges M, Braak H, et al. Imaging the pathoanatomy of amyotrophic lateral sclerosis in vivo: targeting a propagation-based biological marker. J Neurol Neurosurg Psychiatry. (2018) 89:374–81. doi: 10.1136/jnnp-2017-316365
22. Sach M, Winkler G, Glauche V, Liepert J, Heimbach B, Koch MA, et al. Diffusion tensor MRI of early upper motor neuron involvement in amyotrophic lateral sclerosis. Brain. (2004) 127:340–50. doi: 10.1093/brain/awh041
23. Filippini N, Douaud G, Mackay CE, Knight S, Talbot K, Turner MR. Corpus callosum involvement is a consistent feature of amyotrophic lateral sclerosis. Neurology. (2010) 75:1645–52. doi: 10.1212/WNL.0b013e3181fb84d1
24. Verstraete E, Veldink JH, Mandl RCW, van den Berg LH, van den Heuvel MP. Impaired structural motor connectome in amyotrophic lateral sclerosis. PLoS ONE. (2011) 6:e24239. doi: 10.1371/journal.pone.0024239
25. Geraldo AF, Pereira J, Nunes P, Reimão S, Sousa R, Castelo-Branco M, et al. Beyond fractional anisotropy in amyotrophic lateral sclerosis: the value of mean, axial, and radial diffusivity and its correlation with electrophysiological conductivity changes. Neuroradiology. (2018) 60:505–15. doi: 10.1007/s00234-018-2012-6
26. Ellis CM, Simmons A, Jones DK, Bland J, Dawson JM, Horsfield MA, et al. Diffusion tensor MRI assesses corticospinal tract damage in ALS. Neurology. (1999) 53:1051–8. doi: 10.1212/WNL.53.5.1051
27. Oldfield RC. The assessment and analysis of handedness: the Edinburgh inventory. Neuropsychologia. (1971) 9:97–113. doi: 10.1016/0028-3932(71)90067-4
28. Woods BT, Teuber HL. Mirror movements after childhood hemiparesis. Neurology. (1978) 28:1152–7. doi: 10.1212/WNL.28.11.1152
29. Cedarbaum JM, Stambler N, Malta E, Fuller C, Hilt D, Thurmond B, et al. The ALSFRS-R: a revised ALS functional rating scale that incorporates assessments of respiratory function. BDNF ALS study group (Phase III). J Neurol Sci. (1999) 169:13–21. doi: 10.1016/S0022-510X(99)00210-5
30. Turner MR, Cagnin A, Turkheimer FE, Miller CC, Shaw CE, Brooks DJ, et al. Evidence of widespread cerebral microglial activation in amyotrophic lateral sclerosis: an [11C](R)-PK11195 positron emission tomography study. Neurobiol Dis. (2004) 15:601–9. doi: 10.1016/j.nbd.2003.12.012@@uline@
31. Nasreddine ZS, Phillips NA, Bédirian V, Charbonneau S, Whitehead V, Collin I, et al. The Montreal Cognitive Assessment, MoCA: a brief screening tool for mild cognitive impairment. J Am. Geriatr Soc. (2005) 53:695–9. doi: 10.1111/j.1532-5415.2005.53221.x
32. Smith SM, Jenkinson M, Woolrich MW, Beckmann CF, Behrens TEJ, Johansen-Berg H, et al. Advances in functional and structural MR image analysis and implementation as FSL. NeuroImage. (2004) 23:208–19. doi: 10.1016/j.neuroimage.2004.07.051
33. Smith SM, Jenkinson M, Johansen-Berg H, Rueckert D, Nichols TE, Mackay CE, et al. Tract-based spatial statistics: voxelwise analysis of multi-subject diffusion data. Neuroimage. (2006) 31:1487–505. doi: 10.1016/j.neuroimage.2006.02.024
34. Teipel SJ, Kuper-Smith JO, Bartels C, Brosseron F, Buchmann M, Buerger K, et al. DELCODE study group. multicenter tract-based analysis of microstructural lesions within the Alzheimer's disease spectrum: association with amyloid pathology and diagnostic usefulness. J Alzheimers Dis. (2019) 72:455–65. doi: 10.3233/JAD-190446
35. Hua K, Zhang J, Wakana S, Jiang H, Li X, Reich DS, et al. Tract probability maps in stereotaxic spaces: analyses of white matter anatomy and tract-specific quantification. NeuroImage. (2008) 39:336–47. doi: 10.1016/j.neuroimage.2007.07.053
36. Brett M, Anton JL, Valabregue R, Poline JB. Region of interest analysis using SPM toolbox (abstract). Presented at the 8th international conference on functional mapping of the human brain, June 2-6, 2002, Sendai, Japan. NeuroImage. 16:253–62.
37. Caiazzo G, Corbo D, Trojsi F, Piccirillo G, Cirillo M, Monsurrò MR, et al. Distributed corpus callosum involvement in amyotrophic lateral sclerosis: a deterministic tractography study using q-ball imaging. J Neurol. (2014) 261:27–36. doi: 10.1007/s00415-013-7144-3
38. Bartels C, Mertens M, Hofer S, Merboldt KM, Dietrich J, Frahm J, et al. Callosal dysfunction in amyotrophic lateral sleosis correlates with diffusion tensor imaging of the central motor system. Neuromusc Disord. (2008) 18:398–407. doi: 10.1016/j.nmd.2008.02.005
Keywords: amyotrophic lateral sclerosis, diffusion tensor imaging, transcranial magnetic stimulation, mirror movements, corpus callosum
Citation: Wittstock M, Wilde N, Grossmann A, Kasper E and Teipel S (2020) Mirror Movements in Amyotrophic Lateral Sclerosis: A Combined Study Using Diffusion Tensor Imaging and Transcranial Magnetic Stimulation. Front. Neurol. 11:164. doi: 10.3389/fneur.2020.00164
Received: 26 November 2019; Accepted: 21 February 2020;
Published: 06 March 2020.
Edited by:
Carlos Gómez, University of Valladolid, SpainReviewed by:
Salem Hannoun, American University of Beirut, LebanonFrancesca Trojsi, University of Campania Luigi Vanvitelli, Italy
Copyright © 2020 Wittstock, Wilde, Grossmann, Kasper and Teipel. This is an open-access article distributed under the terms of the Creative Commons Attribution License (CC BY). The use, distribution or reproduction in other forums is permitted, provided the original author(s) and the copyright owner(s) are credited and that the original publication in this journal is cited, in accordance with accepted academic practice. No use, distribution or reproduction is permitted which does not comply with these terms.
*Correspondence: Matthias Wittstock, bWF0dGhpYXMud2l0dHN0b2NrQG1lZC51bmktcm9zdG9jay5kZQ==