- The Affiliated Hospital of Qingdao University, Qingdao, China
Atherosclerosis is an inflammatory disease that can lead to cardiovascular disorders and stroke. In the atherosclerosis microenvironment, exosomes secreted from various cells, especially macrophage-derived exosomes, play an important role in cell–cell communication and cellular biological functions. In this article, we review previous studies on exosomal RNAs and discuss their potential value in atherosclerosis diagnosis and therapy. Based on our research, we concluded that macrophage exosomes have potential value in atherosclerosis diagnosis and therapy. However, there is a need for future studies to further investigate methods of exosome isolation and targeting.
Introduction
Atherosclerosis is a chronic inflammatory process that occurs in the arterial wall characterized by lipid accumulation and plaque formation (1). The pathogenesis of atherosclerosis can be divided into three stages: initiation, lesion formation, and thrombosis formation. The initial step is mediated by endothelial cells (ECs) (2). When ECs are subjected to various stimuli, such as changes in blood flow, a focal area of the endothelium will be activated, express adhesion molecules, and recruit leukocytes. ECs in high-lipid environments recruit and bind to monocytes instead of neutrophils; monocytes cross the endothelium after adhering to ECs (3). In response to increased endothelial permeability and changes in the extracellular matrix composition, low-density lipoproteins accumulate in the focal endothelium. After engulfing lipids, monocytes are transformed into foam cells and accumulate in the lesion area. Lesion progression is also associated with extracellular matrix deposition, migration, and proliferation of smooth muscle cells, and formation of a necrotic core (4). If the atherosclerotic plaque is large enough to restrict blood flow, clinical manifestations will occur. Plaque rupture can also lead to myocardial or ischemic stroke, which is associated with collagen-poor fibrous caps (5).
Exosomes are small extracellular vesicles (EVs) with diameters ranging from 40 to 200 nm. Recently, exosomes have become increasingly popular in the scientific community for their great potential for drug delivery. Exosomes are secreted by many cell types and exist in most bodily fluids (6). They play a vital role in cell–cell communication. The contents of exosomes include proteins, lipids, nucleic acids, and small non-coding RNAs such as microRNAs (miRNAs). Among the exosome contents, miRNAs have gained increased attention recently for their potential role in disease diagnosis and treatment. Previous studies suggest that miRNAs have a therapeutic effect in various diseases. The major challenge of using synthetic miRNAs for disease therapy is that they are easily degraded by ribonucleases in plasma. However, this instability can be overcome by inserting them into exosomes. Therefore, exosomes are attractive as a drug-delivery vehicle (7).
Exosomes can serve as carriers across different biological barriers, thereby transferring drugs or biological molecules to target cells. A study suggested that brain EC-derived exosomes can pass the blood-brain barrier (8). Circulating miRNAs have been used as diagnostic biomarkers in atherosclerosis (9). However, different cells might secrete the same miRNAs, which makes it quite difficult to identify their source. However, exosomes carry markers from their source cells. Thus, using these markers to identify the source cells of exosomes and analyzing changes in the content of exosomes can provide information about their parent cells, highlighting the events that occurred in the diseased or disease-causing cells (10). Nevertheless, several issues remain. In particular, exosome isolation is time-consuming and difficult to reproduce. This makes it difficult to compare results between laboratories, improve our knowledge of exosomal physiological functions, and correlate their features with pathological outcomes.
Considering the potential for exosomes to facilitate disease diagnosis and treatment, herein we review recent studies of miRNAs in atherosclerosis (AS)-associated cell-derived exosomes (such as those derived from ECs and macrophages) and in exosome-based therapy or diagnosis of atherosclerosis. This review summarizes our current understanding of the role of exosomal RNAs in atherosclerosis.
EV Families
Wolf first identified EVs in 1967. Initially, EVs were recognized as platelet-derived particles in plasma, so Wolf chose the name “platelet dust” (18, 19). In 1980, EVs were isolated from rectal adenoma microvillus cells (20) and were reported as “virus-like particles” in cell culture (21). These vesicles were also referred to as “prostasomes” (22). An ultrastructure study in 1983 suggested that these vesicles were released by the fusion of multi-vesicular bodies (MVBs) with the cell membrane in reticulocytes during the differentiation process (23, 24). In 1987, Johnstone's group (25) reported that these vesicles originated from reticulocytes and that “the externalization of these vesicles may be a mechanism for shedding of specific membrane functions, which are known to diminish during maturation of reticulocytes to erythrocytes.” In Johnstone's paper, these vesicles were termed “exosomes.”
According to their source cells and tissues, EVs can be called prostasomes (from the prostate), dexosomes (from dendritic cells), oncosomes (from tumor cells), synaptic vesicles (from nerve cells), or cardiosomes (from cardiac muscle cells). Based on their possible functions, they can be named matrix vesicles, argosomes, or tolerosomes (26, 27). According to their size, biological character, and biogenesis mechanism, there are three main types of EVs: apoptotic bodies, microvesicles (also known as ectosomes), and exosomes. Different kinds of EVs have different biogenesis and releasing mechanisms, markers, and contents.
Apoptotic bodies, ranging from 500 to 2,000 nm, are released when apoptosis occurs. Markers of apoptotic bodies contain phosphatidylserine and genomic DNA. Microvesicles are in the range of 200–1,000 nm, while exosomes are 40–200 nm. Microvesicles and exosomes serve as transporters of mRNA, miRNAs, and proteins to target cells. Microvesicle markers include integrins, selectins, and CD40, and markers of exosomes are tetraspanins, apoptosis-linked gene 2-interacting protein X, and tumor susceptibility gene 101 (28, 29). In general, the naming of EVs is very complex because there are no common markers to identify different kinds of EVs. Thus, the nomenclature of different EVs remains controversial (30). The International Society for Extracellular Vesicles advises the use of physicochemical properties, such as size, density, or biochemical components, to identify different EVs (31).
Exosome Biogenesis
Exosome biogenesis is mediated by the endosome system. There are three stages in exosome biogenesis. First, the plasma membrane invaginates, forming endocytic vesicles, and a number of endocytic vesicles fuse and produce early endosomes. Then, the early endosome membrane invaginates and forms many intraluminal vesicles (ILVs); in this stage, endosomes are also called MVBs. Finally, MVBs merge with the cell membrane, releasing ILVs into the extracellular space; these ILVs are called exosomes (32).
Various proteins are involved in the biogenesis process, such as the endosomal sorting complex required for transport, CD63, CD81, apoptosis-linked gene 2-interacting protein X, and tumor susceptibility gene 101. Exosome transport in cells relies on the cell skeleton system, and the SNARE protein complex and synaptotagmin family are involved in the exosome release process. The exosome membrane is rich in cholesterol, sphingomyelin, and ceramide. Ceramide plays an important role in the formation of ILVs. Exosomes originate from the cell membrane, and the exosome membrane also contains lipid bilayer structures. However, in the exosome membrane, lipids are located in the membrane randomly, while in the cell membrane, they are asymmetric (33–35) (Figure 1).
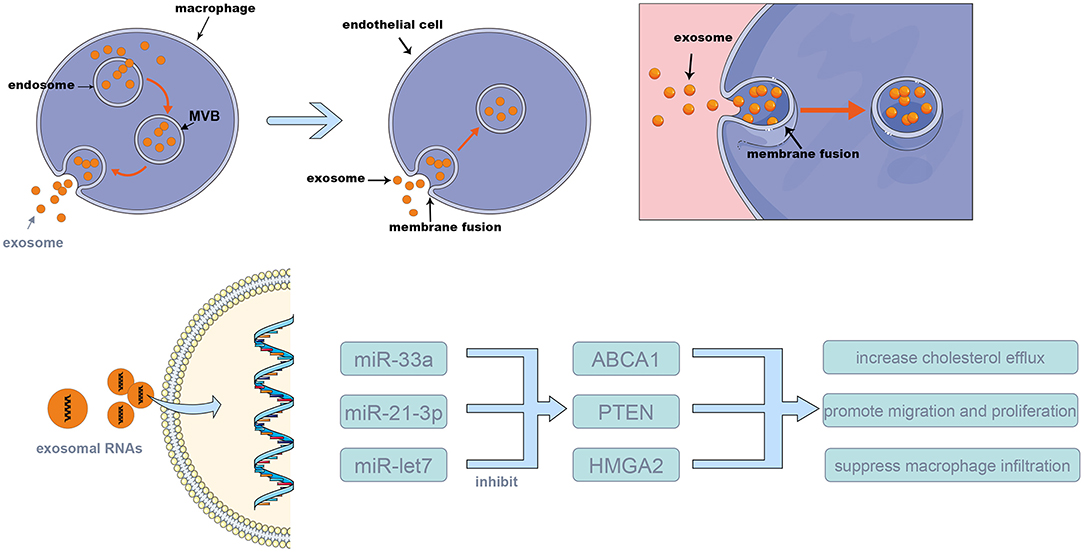
Figure 1. The mechanism of exosome biogenesis and releasing. The multi-vesicular bodies (MVBs) membrane invaginates and forms many exosomes. Then, MVBs merge with the cell membrane, releasing the exosomes into the extracellular space; these exosomes can merge with the membrane of target cells, and release their content to the target cells. The exosomes were released from source cells by MVBs and absorbed by target cells, via this procedure, and the exosomal RNAs such as miR-155, miR-33a, and miR-505 were transferred into target cells and take the biological effects (shown in Table 1).
Exosomal RNAs and ECs in Atherosclerosis
Injury to ECs is an initial factor of atherosclerosis. EC dysfunction, such as tight-junction disruption and release of inflammatory cytokines, leads to macrophage accumulation and inflammation at the site of injury. Various stimulations, such as changes in blood flow, can lead to EC dysfunction (2, 36). There is no clear conclusion that exosome-mediated cell–cell communication plays a good or bad role in the atherosclerosis process. Zheng et al. found that Krüppel-like factor 5-overexpressing vascular smooth muscle cells (VSMCs), which express a high level of miRNA-155, can secrete miRNA-155-containing exosomes, leading to the impairment of endothelial integrity and facilitating atherosclerosis plaque formation (11). VSMC-derived exosomes can be absorbed by ECs and can regulate EC function, suggesting that exosomes play an important role in communication between VSMCs and ECs. Gao et al. demonstrated that mature dendritic cells contribute to endothelial inflammation and increased atherosclerosis and that exosomes are involved in this process (37). They found that exosomes isolated from bone marrow dendritic cell culture medium increase the inflammation of ECs via the NF-κB pathway. They also found that exosomes injected intravenously can be absorbed by ECs in vivo and that tumor necrosis factor-α on the exosome membrane triggers NF-κB in ECs.
Cholesterol accumulation is also a significant cause of atherosclerosis. Stamatikos et al. suggested that EC-derived exosomal miRNA-33a increases macrophage and smooth muscle cell cholesterol efflux by silencing ABCA1. The researchers decreased miRNA-33a expression levels in EC-derived exosomes by constructing a helper-dependent adenovirus to transfect ECs and used these exosomes to treat macrophages and smooth muscle cells (12). Another in-vitro study confirmed that ABCA1 overexpression in ECs enhances ApoAI-mediated cholesterol efflux, the results showed that the ABCA1 protein in macrophages and smooth muscle cells was increased by 1.6–2.2-fold and that cholesterol efflux was increased by 1.4–1.6-fold (38). Besides, Stamatikos also reported that transduction of carotid artery ECs with a helper-dependent adenoviral (HDAd) vector expressing apo A-I reduced the atherosclerosis lesion growth (39). These researches demonstrated that modification of the exosome content to regulate the genes expression of target cells may be a new strategy for treating atherosclerosis.
Li et al. found that EC-derived exosomes can be internalized by VSMCs and can inhibit neointima formation and the phenotypic switch of VSMCs. However, activation of EC CD137 signaling attenuates the repressive effects of EC-derived exosomes (40). There are also studies suggesting that the content of EC-derived exosomes changes after treatment with oxidized low-density-lipoprotein (LDLs). For example, Chen found that exosome-encapsulated miR-505 from oxidized LDL-treated ECs induces neutrophil extracellular trap (NET) formation, which aggravates atherosclerosis (13). Mature dendritic cell-derived exosomes participate in the process of endothelial inflammation and atherosclerosis through the membrane tumor necrosis α-mediated NF-κB signaling pathway. Zhong et al. demonstrated that exosomes derived from mature dendritic cells increase adhesion molecule expression by activating the NF-κB pathway in ECs; however, the researchers also showed that ECs are resistant to a second stimulation by these exosomes. This suggests that a negative feedback loop of inflammation regulation occurs via dendritic cell-derived exosomes (41).
Exosomal long non-coding RNAs (lncRNAs) also play a role in atherosclerosis. Gao et al. found that human umbilical vein EC exosomal MALAT1 expression increases when the cells are treated with oxidized LDLs. Furthermore, oxidized LDL-treated human umbilical vein EC-derived exosomes were shown to aggravate atherosclerosis by promoting neutrophil NET formation, which is mediated by exosomal MALAT1 (14).
Exosomal RNAs and Macrophages in Atherosclerosis
When ECs are activated and express adhesion molecules, they recruit leukocytes, mainly monocytes. After the monocytes pass across the vascular wall, they transfer into macrophages, releasing cytokines that mediate focal inflammation. The communication between macrophages, ECs, and VSMCs is complicated, and evidence shows that exosomes are involved in this process. To investigate the treatment effect of exosomes in atherosclerosis, Pu et al. isolated exosomes from M2 macrophages and then electroporated the exosomes with hexyl 5-aminolevulinate hydrochloride. After systemic administration, these exosomes exhibit an anti-inflammatory effect via their surface-bonded chemokine receptors and the release of anti-inflammatory cytokines secreted from anti-inflammatory M2 macrophages. Furthermore, exosomal hexyl 5-aminolevulinate hydrochloride induces carbon monoxide and bilirubin, which further strengthens the anti-inflammatory effects and aggravates atherosclerosis (42).
According to Zhu et al., when macrophages are stimulated by nicotine, miRNA-21-3p expression in macrophage-derived exosomes increases, and these exosomes accelerate atherosclerosis by mediating VSMC migration and proliferation via the miRNA-21-3p/PTEN pathway (15). Zhang's study showed that miRNA-146 in macrophage-derived exosomes increase when macrophages are treated with oxidized LDLs, which promotes reactive oxygen species and NET release by targeting superoxide dismutase 2, finally aggravating atherosclerosis (43). Li et al. found that treatment with mesenchymal stem cell-derived exosomes decreases the atherosclerotic plaque size in ApoE−/− mice, inhibits the expression in macrophages of increased M2 markers and lipopolysaccharide-induced M1 markers, promotes M2 macrophage polarization through the miR-let7/HMGA2/NF-κB pathway, and suppresses macrophage infiltration via the miR-let7/IGF2BP1/PTEN pathway in plaques, which attenuates the progression of atherosclerosis (16). Oxidized-LDL-stimulated macrophages can attenuate the growth and tube formation of ECs (44). MiRNA-146a from atherogenic macrophage-derived exosomes can inhibit cell migration and promote macrophage adhesion in the vascular wall (17) and can accelerate the process of atherosclerosis.
Research has suggested that obesity is associated with atherosclerosis. Xie found that adipose tissue-derived exosomes from mice fed a high-fat diet regulates macrophage foam cell formation and polarization, suggesting that there is an association between adipose tissue and atherosclerosis in obesity (45). Chen et al. found that lncRNA growth arrest-specific 5 (GAS5) is increased in plaques of atherosclerosis patients and animals. After oxidized LDL stimulation, lncRNA GAS5 is also increased in THP-1 cell-derived exosomes. EC apoptosis was promoted by LncRNA GAS5-overexpressing THP-1 cell-derived exosomes but inhibited by lncRNA GAS5-knockdown THP-1 cell-derived exosomes (46). This suggests that atherosclerosis can be alleviated by regulating the apoptosis of macrophages and ECs via exosomes.
Potential Value of Macrophage Exosomal miRNAs in Atherosclerosis
Macrophages play an important role in the mechanism of atherosclerosis. Macrophages are attracted to the injured endothelium and are the main cells that secrete various inflammatory factors to regulate EC functions. The inflammatory environment could alter microRNA profile in exosomes secreted from macrophages, which could give us a better understanding of the mechanism of disease, the altered microRNAs could be a biomarker for diagnosis (47). These exosomes serve as the carrier of miRNAs among macrophages and between macrophages and ECs. The studies previously discussed suggest that macrophage-derived exosomes regulate EC functions via absorption by ECs. Importantly, various miRNAs can regulate the atherosclerosis process, such as miR-21. MiR-21 is the most abundant miRNA in macrophages and can regulate macrophage expression of inflammatory cytokines, such as IL-10 (48–50). Thus, modifying miR-21 in macrophage-derived exosomes has the potential to exert a therapeutic effect in patients with atherosclerosis. In addition, macrophage-derived exosomes can regulate VSMC functions, such as proliferation, providing another strategy for treating atherosclerosis. Compared with serum RNAs, exosomal miRNAs and lncRNAs are more stable, and changing their contents in exosomes may be more valuable for treating atherosclerosis.
Conclusion
In summary, we believe that macrophage exosomes have potential value in atherosclerosis diagnosis and therapy. However, there are still some issues that need to be resolved, and future studies should further investigate methods of exosome isolation and targeting.
Author Contributions
WY drafted the manuscript. AM and XP contributed to revise the manuscript. All authors contributed to the article and approved the submitted version.
Funding
This work was supported by National Natural Science Foundation (81971111).
Conflict of Interest
The authors declare that the research was conducted in the absence of any commercial or financial relationships that could be construed as a potential conflict of interest.
Acknowledgments
We gratefully acknowledge the support of many individuals who made this study possible and the contributions of plasma donors.
References
2. Gimbrone MA. Vascular endothelium, hemodynamic forces, and atherogenesis. Am J Pathol. (1999) 155:1–5. doi: 10.1016/S0002-9440(10)65090-0
4. Tabas I, Williams KJ, Boren J. Subendothelial lipoprotein retention as the initiating process in atherosclerosis - update and therapeutic implications. Circulation. (2007) 116:1832–44. doi: 10.1161/CIRCULATIONAHA.106.676890
5. Libby P, Ridker PM, Hansson GK. Progress and challenges in translating the biology of atherosclerosis. Nature. (2011) 473:317–25. doi: 10.1038/nature10146
6. Urbanelli L, Buratta S, Sagini K, Ferrara G, Lanni M, Emiliani C. Exosome-based strategies for diagnosis and therapy. Recent Patents CNS Drug Discov. (2015) 10:10–27. doi: 10.2174/1574889810666150702124059
7. Mitchell PS, Parkin RK, Kroh EM, Fritz BR, Wyman SK, Pogosova-Agadjanyan EL, et al. Circulating microRNAs as stable blood-based markers for cancer detection. Proc Natl Acad Sci USA. (2008) 105:10513–8. doi: 10.1073/pnas.0804549105
8. Haqqani AS, Delaney CE, Tremblay TL, Sodja C, Sandhu JK, Stanimirovic DB. Method for isolation and molecular characterization of extracellular microvesicles released from brain endothelial cells. Fluids Barriers CNS. (2013) 10:4. doi: 10.1186/2045-8118-10-4
9. Fichtlscherer S, De Rosa S, Fox H, Schwietz T, Fischer A, Liebetrau C, et al. Circulating microRNAs in patients with coronary artery disease. Circ Res. (2010) 107:677–84. doi: 10.1161/CIRCRESAHA.109.215566
10. Sahoo S, Klychko E, Thorne T, Misener S, Schultz KM, Millay M, et al. Exosomes from human CD34+ stem cells mediate their proangiogenic paracrine activity. Circ Res. (2011) 109:724–35. doi: 10.1161/CIRCRESAHA.111.253286
11. Zheng B, Yin WN, Suzuki T, Zhang XH, Zhang Y, Song LL, et al. Exosome-Mediated miR-155 transfer from smooth muscle cells to endothelial cells induces endothelial injury and promotes atherosclerosis. Mol Ther. (2017) 25:1279–94. doi: 10.1016/j.ymthe.2017.03.031
12. Stamatikos A, Knight E, Vojtech L, Bi L, Wacker B, Tang C, et al. Exosome-mediated transfer of anti-miR-33a-5p from transduced endothelial cells enhances macrophage and vascular smooth muscle cell cholesterol efflux. Hum Gene Ther. (2019) 31:219–32. doi: 10.1089/hum.2019.245
13. Chen L, Hu L, Li Q, Ma J, Li H. Exosome-encapsulated miR-505 from ox-LDL-treated vascular endothelial cells aggravates atherosclerosis by inducing NET formation. Acta Biochim Biophys Sin. (2019) 51:1233–41. doi: 10.1093/abbs/gmz123
14. Gao H, Wang X, Lin C, An Z, Yu J, Cao H, et al. Exosomal MALAT1 derived from ox-LDL-treated endothelial cells induce neutrophil extracellular traps to aggravate atherosclerosis. Biol Chem. (2019) 401:367–76. doi: 10.1515/hsz-2019-0219
15. Zhu J, Liu B, Wang Z, Wang D, Ni H, Zhang L, et al. Exosomes from nicotine-stimulated macrophages accelerate atherosclerosis through miR-21-3p/PTEN-mediated VSMC migration and proliferation. Theranostics. (2019) 9:6901–19. doi: 10.7150/thno.37357
16. Li J, Xue H, Li T, Chu X, Xin D, Xiong Y, et al. Exosomes derived from mesenchymal stem cells attenuate the progression of atherosclerosis in ApoE(-/-) mice via miR-let7 mediated infiltration and polarization of M2 macrophage. Biochem Biophys Res Commun. (2019) 510:565–72. doi: 10.1016/j.bbrc.2019.02.005
17. Nguyen MA, Karunakaran D, Geoffrion M, Cheng HS, Tandoc K, Perisic Matic L, et al. Extracellular vesicles secreted by atherogenic macrophages transfer microRNA to inhibit cell migration. Arteriosc Thromb Vasc Biol. (2018) 38:49–63. doi: 10.1161/ATVBAHA.117.309795
18. Chargaff E, West R. The biological significance of the thromboplastic protein of blood. J Biol Chem. (1946) 166:189–97.
19. Wolf P. The nature and significance of platelet products in human plasma. Br J Haematol. (1967) 13:269–88. doi: 10.1111/j.1365-2141.1967.tb08741.x
20. De Broe M, Wieme R, Roels F. Letter: membrane fragments with koinozymic properties released from villous adenoma of the rectum. Lancet. (1975) 2:1214–5.
21. Benz EW Jr, Moses HL. Small, virus-like particles detected in bovine sera by electron microscopy. J Natl Cancer Inst. (1974) 52:1931–4. doi: 10.1093/jnci/52.6.1931
22. Stegmayr B, Ronquist G. Promotive effect on human sperm progressive motility by prostasomes. Urol Res. (1982) 10:253–7. doi: 10.1007/BF00255932
23. Harding C, Heuser J, Stahl P. Endocytosis and intracellular processing of transferrin and colloidal gold-transferrin in rat reticulocytes: demonstration of a pathway for receptor shedding. Eur J Cell Biol. (1984) 35:256–63.
24. Pan BT, Johnstone RM. Fate of the transferrin receptor during maturation of sheep reticulocytes in vitro: selective externalization of the receptor. Cell. (1983) 33:967–78. doi: 10.1016/0092-8674(83)90040-5
25. Johnstone RM, Adam M, Hammond JR, Orr L, Turbide C. Vesicle formation during reticulocyte maturation. Association of plasma membrane activities with released vesicles (exosomes). J Biol Chem. (1987) 262:9412–20.
26. Colombo M, Raposo G, Thery C. Biogenesis, secretion, and intercellular interactions of exosomes and other extracellular vesicles. Ann Rev Cell Dev Biol. (2014) 30:255–89. doi: 10.1146/annurev-cellbio-101512-122326
27. S ELA, Mager I, Breakefield XO, Wood MJ. Extracellular vesicles: biology and emerging therapeutic opportunities. Nat Rev Drug Discov. (2013) 12:347–57. doi: 10.1038/nrd3978
28. Shao H, Im H, Castro CM, Breakefield X, Weissleder R, Lee H. New technologies for analysis of extracellular vesicles. Chem Rev. (2018) 118:1917–50. doi: 10.1021/acs.chemrev.7b00534
29. Gurunathan S, Kang MH, Jeyaraj M, Qasim M, Kim JH. Review of the isolation, characterization, biological function, and multifarious therapeutic approaches of exosomes. Cells. (2019) 8:307. doi: 10.3390/cells8040307
30. Echevarria J, Royo F, Pazos R, Salazar L, Falcon-Perez JM, Reichardt NC. Microarray-based identification of lectins for the purification of human urinary extracellular vesicles directly from urine samples. Chembiochem. (2014) 15:1621–6. doi: 10.1002/cbic.201402058
31. Thery C, Witwer KW, Aikawa E, Alcaraz MJ, Anderson JD, Andriantsitohaina R, et al. Minimal information for studies of extracellular vesicles 2018 (MISEV2018): a position statement of the international society for extracellular vesicles and update of the MISEV2014 guidelines. J Extracell Vesicles. (2018) 7:1535750. doi: 10.1080/20013078.2018.1461450
32. Lee Y, El Andaloussi S, Wood MJ. Exosomes and microvesicles: extracellular vesicles for genetic information transfer and gene therapy. Hum Mol Genet. (2012) 21:R125–34. doi: 10.1093/hmg/dds317
33. Raposo G, Stoorvogel W. Extracellular vesicles: exosomes, microvesicles, and friends. J Cell Biol. (2013) 200:373–83. doi: 10.1083/jcb.201211138
34. Trajkovic K, Hsu C, Chiantia S, Rajendran L, Wenzel D, Wieland F, et al. Ceramide triggers budding of exosome vesicles into multivesicular endosomes. Science. (2008) 319:1244–7. doi: 10.1126/science.1153124
35. Subra C, Laulagnier K, Perret B, Record M. Exosome lipidomics unravels lipid sorting at the level of multivesicular bodies. Biochimie. (2007) 89:205–12. doi: 10.1016/j.biochi.2006.10.014
36. Resnick N, Gimbrone MA Jr. Hemodynamic forces are complex regulators of endothelial gene expression. FASEB J. (1995). 9:874–82. doi: 10.1096/fasebj.9.10.7615157
37. Gao W, Liu H, Yuan J, Wu C, Huang D, Ma Y, et al. Exosomes derived from mature dendritic cells increase endothelial inflammation and atherosclerosis via membrane TNF-alpha mediated NF-kappaB pathway. J Cell Mol Med. (2016) 20:2318–27. doi: 10.1111/jcmm.12923
38. Stamatikos A, Dronadula N, Ng P, Palmer D, Knight E, Wacker BK, et al. ABCA1 overexpression in endothelial cells in vitro enhances apoai-mediated cholesterol efflux and decreases inflammation. Hum Gene Ther. (2019) 30:236–48. doi: 10.1089/hum.2018.120
39. Wacker BK, Dronadula N, Bi L, Stamatikos A, Dichek DA. Apo A-I (Apolipoprotein A-I) vascular gene therapy provides durable protection against atherosclerosis in hyperlipidemic rabbits. Arterioscler Thrombosis Vasc Biol. (2018) 38:206–17. doi: 10.1161/ATVBAHA.117.309565
40. Li B, Zang G, Zhong W, Chen R, Zhang Y, Yang P, et al. Activation of CD137 signaling promotes neointimal formation by attenuating TET2 and transferrring from endothelial cell-derived exosomes to vascular smooth muscle cells. Biomed Pharmacother Biomed Pharmacother. (2020) 121:109593. doi: 10.1016/j.biopha.2019.109593
41. Zhong X, Gao W, Wu R, Liu H, Ge J. Dendritic cell exosomeshuttled miRNA146a regulates exosomeinduced endothelial cell inflammation by inhibiting IRAK1: a feedback control mechanism. Mol Med Rep. (2019) 20:5315–23. doi: 10.3892/mmr.2019.10749
42. Pu K, Xie HY. Molecularly engineered macrophage-derived exosomes with inflammation tropism and intrinsic biosynthesis for atherosclerosis treatment. Angew Chem. Int. Ed. (2019) 59:4068–74. doi: 10.1002/anie.201913700
43. Zhang YG, Song Y, Guo XL, Miao RY, Fu YQ, Miao CF, et al. Exosomes derived from oxLDL-stimulated macrophages induce neutrophil extracellular traps to drive atherosclerosis. Cell cycle. (2019) 18:2674–84. doi: 10.1080/15384101.2019.1654797
44. Huang C, Huang Y, Zhou Y, Nie W, Pu X, Xu X, et al. Exosomes derived from oxidized LDL-stimulated macrophages attenuate the growth and tube formation of endothelial cells. Mol Med Rep. (2018) 17:4605–10. doi: 10.3892/mmr.2018.8380
45. Xie Z, Wang X, Liu X, Du H, Sun C, Shao X, et al. Adipose-derived exosomes exert proatherogenic effects by regulating macrophage foam cell formation and polarization. J Am Heart Assoc. (2018) 7:e007442. doi: 10.1161/JAHA.117.007442
46. Chen L, Yang W, Guo Y, Chen W, Zheng P, Zeng J, et al. Exosomal lncRNA GAS5 regulates the apoptosis of macrophages and vascular endothelial cells in atherosclerosis. PLoS ONE. (2017) 12:e0185406. doi: 10.1371/journal.pone.0185406
47. Chen L, Yao X, Yao H, Ji Q, Ding G, Liu X. Exosomal miR-103-3p from LPS-activated THP-1 macrophage contributes to the activation of hepatic stellate cells. FASEB J. (2020) 34:5178–92. doi: 10.1096/fj.201902307RRR
48. Caescu CI, Guo X, Tesfa L, Bhagat TD, Verma A, Zheng D, et al. Colony stimulating factor-1 receptor signaling networks inhibit mouse macrophage inflammatory responses by induction of microRNA-21. Blood. (2015) 125:e1–13. doi: 10.1182/blood-2014-10-608000
49. Sheedy FJ, Palsson-McDermott E, Hennessy EJ, Martin C, O'Leary JJ, Ruan Q, et al. Negative regulation of TLR4 via targeting of the proinflammatory tumor suppressor PDCD4 by the microRNA miR-21. Nat Immunol. (2010) 11:141–7. doi: 10.1038/ni.1828
Keywords: atherosclerosis, exosome, RNA, macrophage, endothelial cell
Citation: Yang W, Pan X and Ma A (2021) The Potential of Exosomal RNAs in Atherosclerosis Diagnosis and Therapy. Front. Neurol. 11:572226. doi: 10.3389/fneur.2020.572226
Received: 22 June 2020; Accepted: 02 December 2020;
Published: 11 February 2021.
Edited by:
Osama O. Zaidat, Northeast Ohio Medical University, United StatesReviewed by:
Jan Jack Gouda, Wright State University, United StatesLina Shehadeh, University of Miami, United States
Copyright © 2021 Yang, Pan and Ma. This is an open-access article distributed under the terms of the Creative Commons Attribution License (CC BY). The use, distribution or reproduction in other forums is permitted, provided the original author(s) and the copyright owner(s) are credited and that the original publication in this journal is cited, in accordance with accepted academic practice. No use, distribution or reproduction is permitted which does not comply with these terms.
*Correspondence: Aijun Ma, ZHJtYWpAMTI2LmNvbQ==; Xudong Pan, ZHJwYW4wMjJAMTYzLmNvbQ==