- 1Center for Research and Education in Special Environments, Department of Exercise and Nutrition Sciences, School of Public Health and Health Professions, University at Buffalo, Buffalo, NY, United States
- 2Human Integrative Physiology Laboratory, Department of Kinesiology, School of Public Health, Indiana University, Bloomington, IN, United States
- 3Department of Psychiatry, Jacobs School of Medicine and Biomedical Sciences, University at Buffalo, Buffalo, NY, United States
- 4UBMD Department of Orthopaedics and Sports Medicine, University at Buffalo, Buffalo, NY, United States
Concussions have been shown to result in autonomic dysfunction and altered cerebral vascular function. We tested the hypothesis that concussed athletes (CA) would have altered cerebral vascular function during acute decreases and increases in blood pressure compared to healthy controls (HC). Ten CA (age: 20 ± 2 y, 7 females) and 10 HC (age: 21 ± 2 y, 6 females) completed 5 min of lower body negative pressure (LBNP; −40 mmHg) and 5 min of lower body positive pressure (LBPP; 20 mmHg). Protocols were randomized and separated by 10 min. Mean arterial pressure (MAP) and middle cerebral artery blood velocity (MCAv) were continuously recorded. Cerebral vascular resistance (CVR) was calculated as MAP/MCAv. Values are reported as change from baseline to the last minute achieved (LBNP) or 5 min (LBPP). There were no differences in baseline values between groups. During LBNP, there were no differences in the change for MAP (CA: −23 ± 18 vs. HC: −21 ± 17 cm/s; P = 0.80) or MCAv (CA: −13 ± 8 vs. HC: −18 ± 9 cm/s; P = 0.19). The change in CVR was different between groups (CA: −0.08 ± 0.26 vs. HC: 0.18 ± 0.24 mmHg/cm/s; P = 0.04). Total LBNP time was lower for CA (204 ± 92 s) vs. HC (297 ± 64 s; P = 0.04). During LBPP, the change in MAP was not different between groups (CA: 13 ± 6 vs. HC: 10 ± 7 mmHg; P = 0.32). The change in MCAv (CA: 7 ± 6 vs. HC: −4 ± 13 cm/s; P = 0.04) and CVR (CA: −0.06 ± 0.27 vs. HC: 0.38 ± 0.41 mmHg/cm/s; P = 0.03) were different between groups. CA exhibited impaired tolerance to LBNP and had a different cerebral vascular response to LBPP compared to HC.
Introduction
Nearly 3.8 million individuals sustain a sport-related concussion every year in the United States and many more are sustained via car crashes, falls, and military action (1). It has been proposed that following head trauma there is a neurometabolic cascade that ensues which may influence autonomic nervous system function as well as cerebral blood flow and cerebral vascular function (2, 3). Accumulating evidence has indicated that individuals who have sustained a concussion exhibit autonomic dysfunction (4) and altered cerebral blood flow (5–7). For instance, asymptomatic post-mild traumatic brain injury (mTBI) patients exhibited reduced cardiac baroreflex sensitivity (cBRS) at rest (4, 8) and upon standing compared to healthy controls (4). Similarly, cerebral autoregulatory dysfunction was found in acutely concussed athletes (i.e., ice hockey and American football) during squat-to-stand maneuvers (9) and in active professional boxers with mild to moderate chronic brain injury using the thigh cuff deflation technique (10). These investigations have studied patients at rest (4, 8, 11) or using experimental techniques that induce only acute decreases in blood pressure to assess cBRS (4) and cerebral vascular function (9, 10). However, other common stressors that occur outside of the lab, such as mental stress (12–14) and exercise (15), can cause acute increases in blood pressure. These increases in blood pressure might contribute to an exacerbation of concussion symptoms secondary to impaired blood pressure control and/or abnormal cerebral vascular function. Thus, it is important to assess cerebral vascular function and the control of blood pressure during both decreases and increases in blood pressure.
Lower body negative pressure (LBNP) and lower body positive pressure (LBPP) are physiological stressors that can be used to acutely decrease and increase arterial blood pressure, respectively. These techniques can therefore be used to investigate both cerebral blood flow responses and autonomic baroreflex function in individuals who have sustained a concussion. Unlike the sit-to-stand tests, which have been used to demonstrate impaired dynamic cerebral vascular function in concussed patients (9, 16), LBNP and LBPP do not activate the vestibular system. Vestibular system disturbances are common following a concussion and can lead to symptom exacerbation, primarily dizziness, which can confound cBRS and cerebral blood flow outcomes during assesments that involve postural changes (17). To the best of our knowledge, no one has experimentally induced a rise in blood pressure above resting values to examine cBRS and cerebral vascular function in symptomatic concussed athletes. Thus, LBNP and LBPP protocols are excellent techniques to assess alterations in blood pressure in concussion patients because these techniques circumvent the possible influence of vestibular activation and allow for a more clear interpretation of the data. Furthermore, sit-to-stand tests examine cerebral blood velocity responses to rapid oscillations in blood pressure (9) whereas LBPP acutely increases and sustains a rise in blood pressure. The combined use of LBNP and LBPP can better illuminate the baroreflex and cerebral vascular responses to changes in blood pressure in patients who have sustained a concussion. To this end, the primary aims of our study were to investigate cerebral vascular function and cardiac-baroreflex sensitivity during acute changes in arterial blood pressure in symptomatic concussed college athletes. We hypothesized that compared to healthy controls, concussed athletes would (1) have a greater reduction in cerebral blood velocity during LBNP, (2) have lower cBRS during LBNP, (3) have a greater increase in cerebral blood velocity during LBPP, and (4) have lower cBRS during LBPP.
Methods
Participants
Ten concussed college athletes (CA) (7 females; age: 20 ± 2 y; height: 177 ± 10 cm; weight: 77 ± 10 kg; BMI: 25 ± 3 kg/m2) and 10 healthy controls (HC) (6 females; age: 21 ± 2 y; height: 174 ± 7 cm; weight: 76 ± 15 kg; BMI: 25 ± 3 kg/m2) completed the study. Using feasibility data, we calculated the a priori sample size based on the cardiovascular responses to a sympathetic stressor in 10 CA and 10 HC. We calculated we would need 8 participants in each group using an effect size (f ) of 0.38, alpha of 0.05, and power of 0.80 to observe a significant group x time interaction effect. CA completed the study within 10 days of concussion (5 ± 3 days) diagnosis by a University at Buffalo physician and while symptomatic (SCAT-5 score: 23 ± 36; range: 2–85). All concussions were sport-related and diagnosed using the most recent Concussion in Sport Group guidelines (18), including history, concussion symptoms linked to a head injury via the Post-Concussion Symptom Inventory (19), or injury to another part of the body with forced transmission to the head, and impairments on a concussion-focused physical exam (20). The CA group self-reported to not have had a concussion within the previous year (with the exception of the most recent concussion). All CA participated in collegiate sports. Female athletes participated in rowing (n = 1), volleyball (n = 2), soccer (n = 2), and basketball (n = 2). Male athletes participated in football (n = 1), wrestling (n = 1), and lacrosse (n =1). The HC group self-reported to be physically active on most if not all days of the week via the International Physical Activity Questionnaire and self-reported no concussions within the previous year. We used healthy controls that did not participate in collegiate athletics to avoid the unknown effects of repetitive subconcussive head impacts on physiological function. Participants were excluded if they had a Glasgow Coma Score of <12, focal neurological deficit, used medications that affect blood pressure or the autonomic nervous system (beta-blockers, alpha-blockers, angiotensin converting enzyme inhibitors, angiotensin receptor blockers); existing autonomic, cardiovascular, metabolic, respiratory, or endocrine disorder; major depression; pregnancy; or breastfeeding. The concussed athletes were studied while still reporting post-concussion symptoms based on a symptom severity inventory (Sport Concussion Assessment Tool−5th Edition (SCAT-5)) (18). This 22-question Likert scale questionnaire assesses a variety of symptoms (None = 0, Moderate = 3, Severe = 6). The sum of these responses was calculated to express total symptom severity (0–132). All participants were fully informed of the experimental procedures prior to providing informed, written consent. The study was approved by the Institutional Review Board at the University at Buffalo and was performed in accordance with the standards set by the latest revision of the Declaration of Helsinki.
Experimental Approach
Participants completed one study visit that consisted of an LBNP protocol and an LBPP protocol. Participants reported to the laboratory after abstaining from alcohol, exercise, and caffeine for 12 h, and food for 2 h. Women of childbearing potential voided their bladder for a urine pregnancy test and reported the start date of their last menstrual cycle. It is important to note that menstrual cycle phase might influence cerebral vascular function (21, 22) and baroreflex function (23) however, we were unable to control for the menstrual cycle due to testing participants within the first 10 days of concussion diagnosis and menstrual cycle phase was not a focus of our investigation. After voiding their bladder, participants assumed the supine position and their lower body was sealed into an airtight chamber at the level of the iliac crest using a neoprene skirt prior to being instrumented to continuously record beat-to-beat blood pressure (photoplethysmography), heart rate (3-lead electrocardiogram), and end-tidal CO2 tension (capnography). Right MCAv was measured via transcranial Doppler sonography using a 2 MHz probe (DWL USA, Inc., Germany, Europe) while using insonation techniques described in detail by Willie et al. (24).
After instrumentation, participants completed a 5-min baseline period before commencement of the protocols. Prior to and following the LBNP and LBPP protocols, concussed athletes reported their concussion symptom severity using a visual analog scale (VAS; 0 = feel terrific, no symptoms; 10 = feel terrible, worst I ever felt). The order of the protocols was randomized by a research team member prior to enrollment using Excel. Protocols were separated by 5 min of recovery and 5 min of quiet resting baseline (>10 min between protocols). Eight CA (5 women) and six HC (2 women) completed LBPP first. During the LBNP protocol, an acute decrease in blood pressure was achieved by applying 40 mmHg of LBNP for 5 min (25–27). If systolic blood pressure sustained a fall below 80 mmHg along with a precipitous decrease in heart rate, or if participants reported any symptoms of pre-syncope (e.g., sweating, tunnel vision, nausea, dizziness), the LBNP protocol was terminated (28). During the LBPP protocol, an acute increase in blood pressure was achieved by applying 20 mmHg of LBPP for 5 minutes (29–31). The LBPP protocol was terminated if participants desired to self-terminate or if systolic blood pressure sustained an increase above 180 mmHg.
Data Acquisition
Heart rate (1,000 Hz), beat-to-beat blood pressure (1,000 Hz), MCAv (1,000 Hz) and end-tidal CO2 tension (62.5 Hz) were continuously recorded using a data acquisition system (Biopac MP150, AcqKnowledge 4.2.0, Goleta, CA). Stroke volume was estimated using Modelflow (32) and cardiac output was calculated as the product of heart rate and stroke volume. Cerebral vascular resistance (CVR) was calculated as MAP divided by MCAv. Mean values were extracted for the last 2 min of baseline and for every minute of the LBNP and LBPP protocols. The absolute change from baseline values were calculated for each minute of LBNP and LBPP. Total LBNP time was recorded (s) as an indicator of orthostatic tolerance. Due to early termination of LBNP, only participants that completed at least 3 min of the 5 min protocol were included in the anaylsis for cBRS (see below) (33). Total LBPP time was not analyzed since all participants completed the protocol (300 s). One healthy control was excluded from MCAv and cerebral vascular resistance analysis during LBNP due to excessive noise (>10 s of excluded data) (34).
Assessment of Cardiac-Baroreflex Sensitivity (cBRS)
Raw data files of ECG and beat-to-beat blood pressure were used for offline analysis of cardiac-baroreflex sensitivity (WinCPRS, Absolute Aliens Oy, Turku, Finland). Three minute and five minute recordings for LBNP and LBPP were included for analysis, respectively. Participants were excluded from LBNP analysis of cBRS if the test was terminated prior to reaching 3 min of negative pressure [excluded concussed athletes (n = 3); excluded healthy controls (n = 2)] (33). The ECG and blood pressure waveforms were visually inspected for artifact and ectopic beats. We used the sequence method to calculate cBRS (35, 36). Four successive increases or decreases in systolic blood pressure (SBP) (±1 mmHg) that corresponded with changes in R-R interval (RRI) (±5 ms) were identified. Sequences were deemed valid if the R2-value calculated from individual linear regression analyses between SBP and RRI was ≥0.85 (37). The time delay between SBP and RRI was set to 1 beat (38). Sequences were determined separately for Up-cBRS (i.e., concurrent increases in RRI and SBP) and Down-cBRS (i.e., concurrent decreases in RRI and SBP decreased).
Statistical Analysis
We used unpaired t-tests to determine if resting baseline values for cardiovascular and cerebrovascular variables and total LBNP time were different between CA and HC. We used two-way repeated measures ANOVAs with time as a within-subject effect and group as a between-subject effect to compare responses for LBPP between CA and HC. Mixed-effects ANOVAs were used to compare responses between CA and HC during LBNP due to missing data from terminating the test early (n = 9 total events). All ANOVA assumptions were visually verified (Q-Q plots and residual plots) prior to model interpretation and all assumptions were met and no corrections were made. If an ANOVA revealed a significant interaction or main effect (39), we used Bonferroni post-hoc analyses to determine where differences occurred. Additionally, if the Bonferroni post-hoc analyses revealed a difference between groups, we calculated the effect size, Cohen's d. A Kaplan-Meier survival analysis was used to assess the survival rate between CA and HC during the LBNP protocol. Lastly, we used paired t-tests to compare concussion symptom severity in the CA group before and after each protocol. Statistical analyses were performed using Prism software (version 8, GraphPad Software, La Jolla, CA). Statistical significance was set at P ≤ 0.05. Exact P-values are reported where appropriate. Values are reported as mean ± SD of the change from baseline.
Results
Lower Body Negative Pressure
There were no differences between groups for all variables at baseline (Table 1).
Cardiovascular Responses
Mean arterial pressure and systolic blood pressure decreased from baseline in CA (all P ≤ 0.006) and HC (all P ≤ 0.008) during LBNP. Diastolic blood pressure decreased from baseline in CA at 2 min (P = 0.003), 3 min (P = 0.055), and 4 min (P = 0.003), whereas HC decreased at 3 min (P = 0.019), 4 min (P = 0.045), and 5 min (P = 0.002). Heart rate increased from baseline in CA (all P < 0.001) and HC (all P < 0.001) during LBNP. Stroke volume decreased from baseline in CA (all P < 0.001) and HC (all P < 0.001) during LBNP. Cardiac output decreased from baseline in CA at 1 min of LBNP (P = 0.014) and decreased in HC at 2 min (P = 0.012) and 3 min (P = 0.002). The change in cardiovascular variables were not different between groups (P ≥ 0.278, Figures 1A–F).
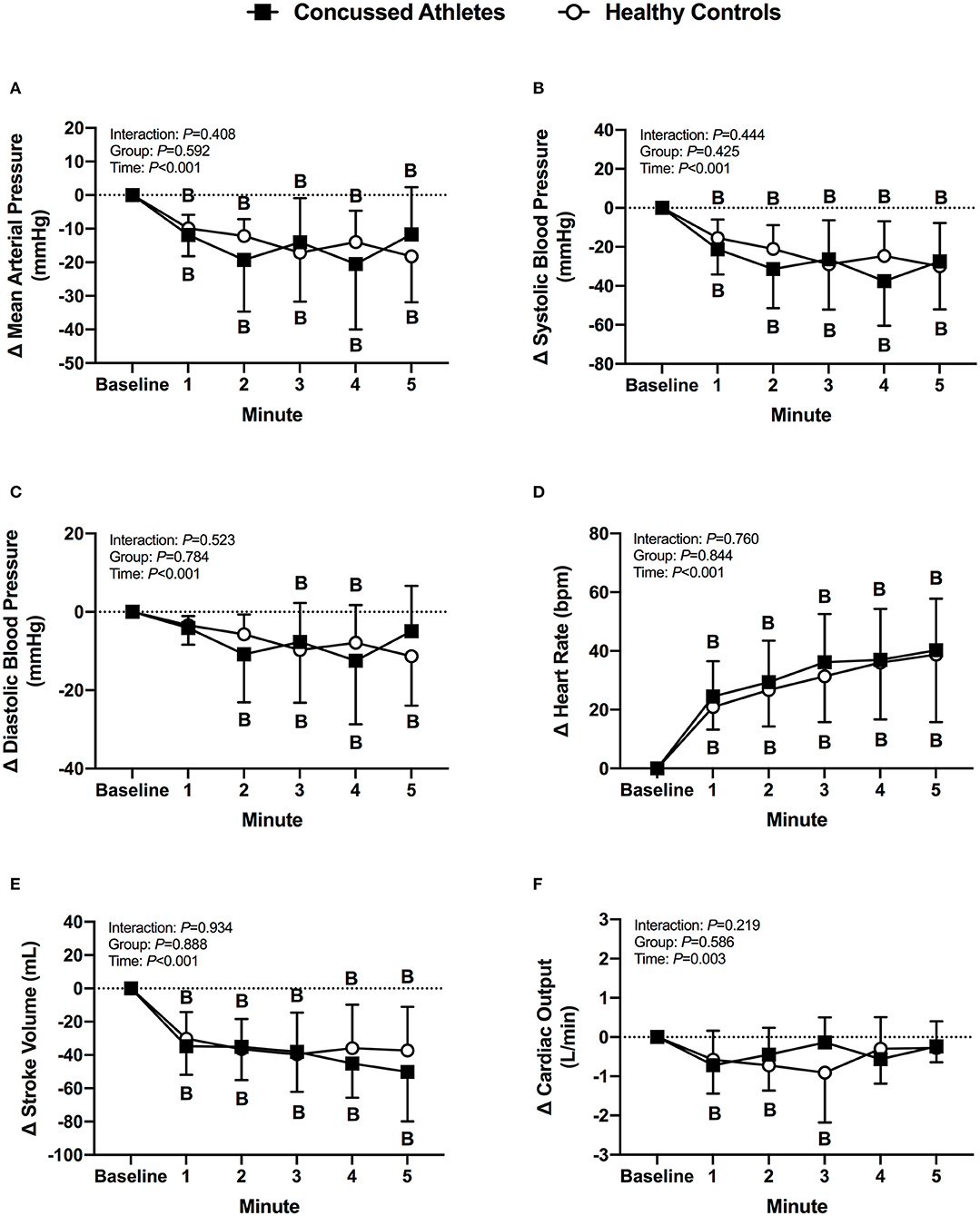
Figure 1. The change from baseline in mean arterial pressure (A), systolic blood pressure (B), diastolic blood pressure (C), heart rate (D), stroke volume (E), and cardiac output (F) at each minute of LBNP for concussed athletes (n = 10) and healthy controls (n = 10). Participants who experienced pre-syncopal signs and did not last the full minute are not included in the statistics for that time point. Concussed athletes were excluded at 3 min (n = 4), 4 min (n = 5), and 5 min (n = 7). Healthy controls were excluded at 3 min (n = 1) and 4 min (n = 2). B, different from Baseline, (P ≤ 0.05).
Cardiac-Baroreflex Sensitivity
RRI decreased from baseline in CA (P < 0.001) and HC (P = 0.002). Up-cBRS had a main effect of time (P = 0.015), but post-hoc-tests were unable to detect a difference from baseline in CA (P = 0.094) or HC (P = 0.239). Down-cBRS decreased from baseline in CA (P = 0.005) and HC (P = 0.005). The change in indices of cBRS were not different between groups (P ≥ 0.585, Figures 2A–C).
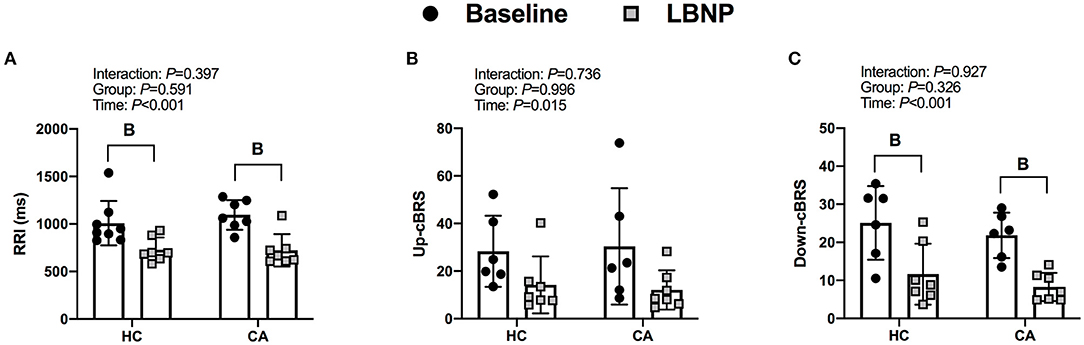
Figure 2. R-R interval (A), Up cardiac- baroreflex senstivity (B), and Down cardiac-baroreflex sensitivity (C) for Healthy Controls and Concussed Athletes at baseline and LBNP. B, different from Baseline, (P ≤ 0.05).
End-Tidal CO2 Tension
End-tidal CO2 tension decreased from baseline in CA (all P ≤ 0.036) and HC (all P ≤ 0.003) during LBNP. The change was not different between groups (P ≥ 0.246, Figure 3A).
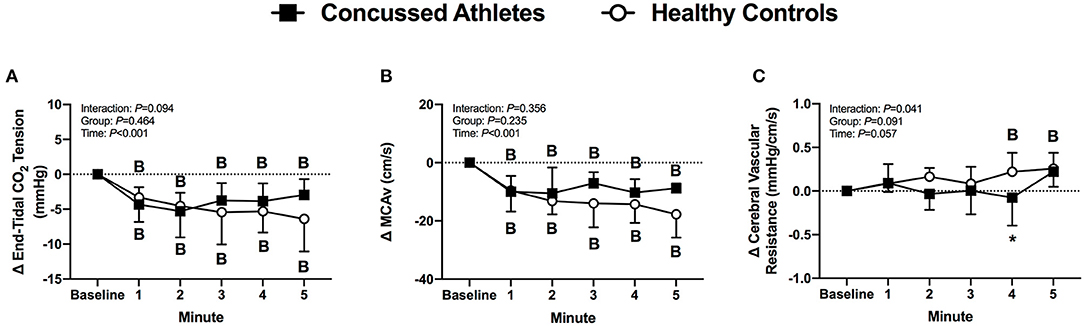
Figure 3. The change from baseline in end-tidal CO2 tension (A), MCAv (B) and cerebral vascular resistance (C) at each minute of LBNP for concussed athletes (n = 10) and healthy controls (n = 9; one excluded due to poor TCD signal). Participants who experienced pre-syncopal signs and did not last the full minute are not included in the statistics for that time point. Concussed athletes were excluded at 3 min (n = 4), 4 min (n = 5), and 5 min (n = 7). Healthy controls were excluded at 3 min (n = 1) and 4 min (n = 2). B, different from Baseline (P ≤ 0.05). *Different from Healthy Controls (P ≤ 0.05).
Cerebral Vascular Responses
MCAv decreased from baseline in CA (all P ≤ 0.003) and HC (all P < 0.001) during LBNP, but the change was not different between groups (P ≥ 0.431, Figure 3B). Cerebral vascular resistance did not change from baseline in CA (all P ≥ 0.730), whereas HC increased at 4 min (P = 0.037), and 5 min of LBNP (P = 0.008). The change in cerebral vascular resistance was different between groups at 4 min of LBNP (P = 0.033, d = 1.08, Figure 3C).
Total LBNP Time, Survival Rate, and Concussion Symptoms
Total LBNP time was different between CA (204 ± 92 s) and HC (270 ± 64 s; P = 0.040, d = 0.83) (Figure 4A). The Kaplan-Meier survival curve with the log-rank (Mantel-Cox) test revealed a median survival time that was different between CA (200 s) and HC (300 s; P = 0.049) (Figure 4B). CA experienced an exacerbation of symptoms from pre-LBNP (2 ± 2) to post-LBNP (4 ± 2; P = 0.006) (Figure 5).
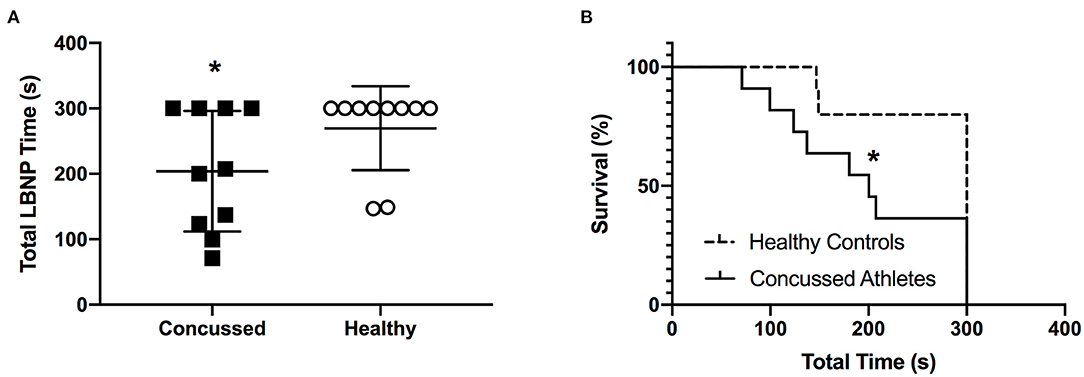
Figure 4. Total LBNP time (A) and Kaplan-Meier survival curve (B) for Concussed Athletes vs. Healthy Controls. Values are mean ± SD. *Different from Healthy Controls (P ≤ 0.05).
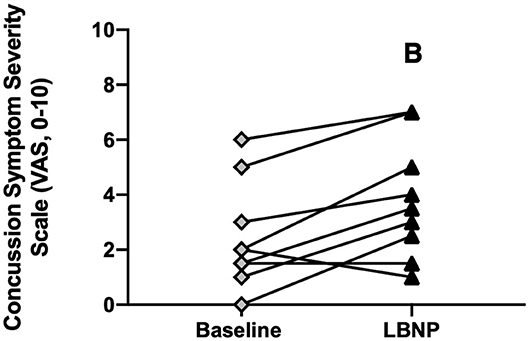
Figure 5. Concussion symptom severity rating (Visual Analog Scale, 0–10) at baseline and after LBNP for concussed athletes (n = 10). Values are mean ± SD. B, different from baseline (P ≤ 0.05).
Lower Body Positive Pressure
There were no differences between groups for all variables at baseline (Table 1).
Cardiovascular Responses
Mean arterial pressure, systolic blood pressure, and diastolic blood pressure increased from baseline in CA (all P < 0.001) and HC (all P ≤ 0.008) during LBPP. Heart rate increased from baseline in CA only at 5 min of LBNP (P = 0.006), whereas HC had an increase in heart rate at 2 min through 5 min of LBPP (all P < 0.001). Stroke volume decreased from baseline in CA (all P < 0.001) and HC (all P < 0.001) during LBPP. Cardiac output decreased from baseline in CA (all P < 0.001) during LBPP, but there was no change from baseline in HC (P > 0.999). The change in cardiovascular variables were not different between groups (P ≥ 0.064, Figures 6A–F).
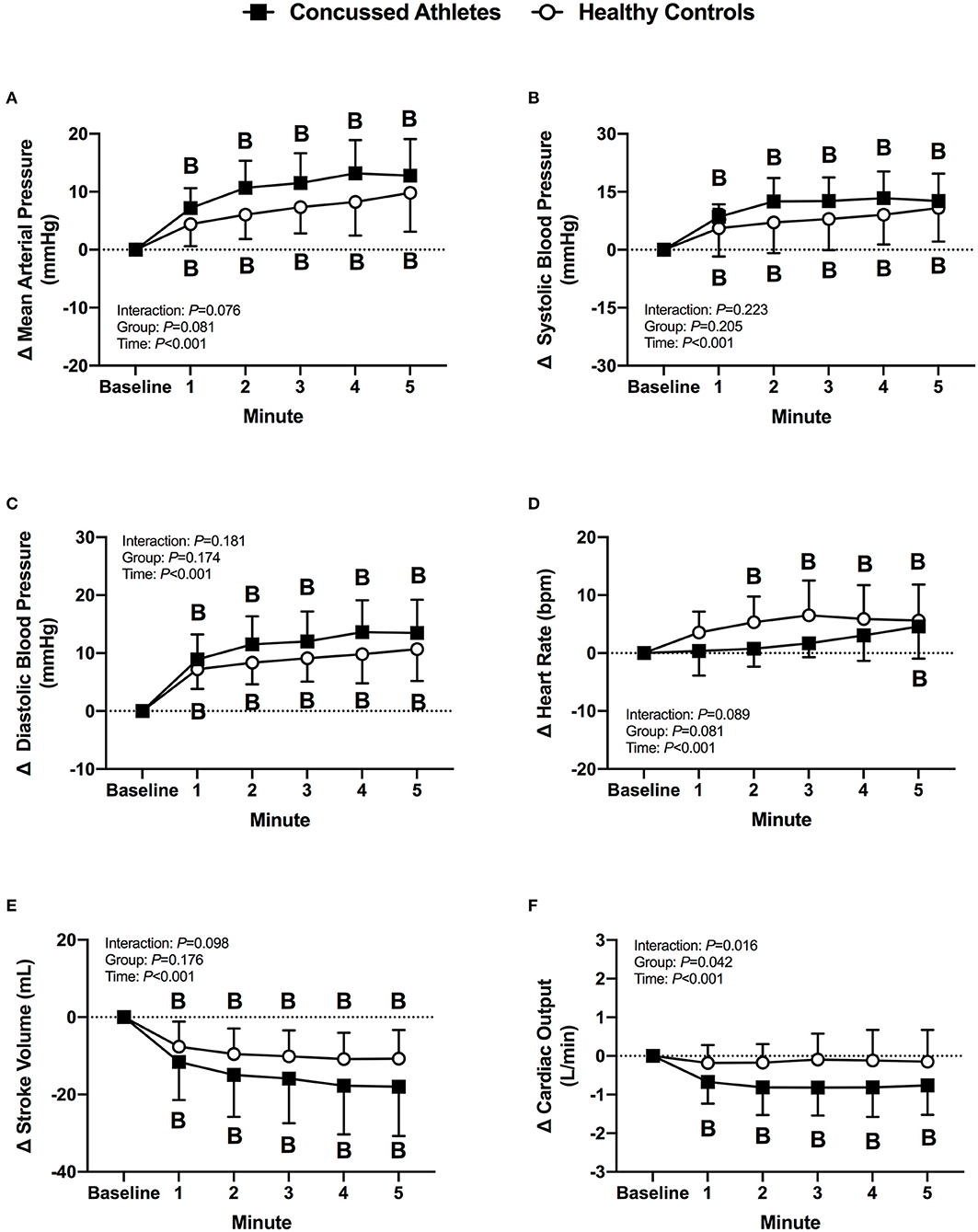
Figure 6. The change from baseline in mean arterial pressure (A), systolic blood pressure (B), diastolic blood pressure (C), heart rate (D), stroke volume (E), and cardiac output (F) at each minute of LBPP for concussed athletes (n = 10) and healthy controls (n = 10). B, different from Baseline (P ≤ 0.05).
Cardiac-Baroreflex Sensitivity
RRI decreased from baseline in HC (P < 0.001) but did not change in CA (P = 0.108). Up-cBRS did not change from baseline in CA (P = 0.358) or HC (P = 0.064). Down-cBRS was not different over time (P = 0.191) or between groups (P = 0.941). The change in indices of cBRS was not different between groups (P ≥ 0.257, Figures 7A–C).
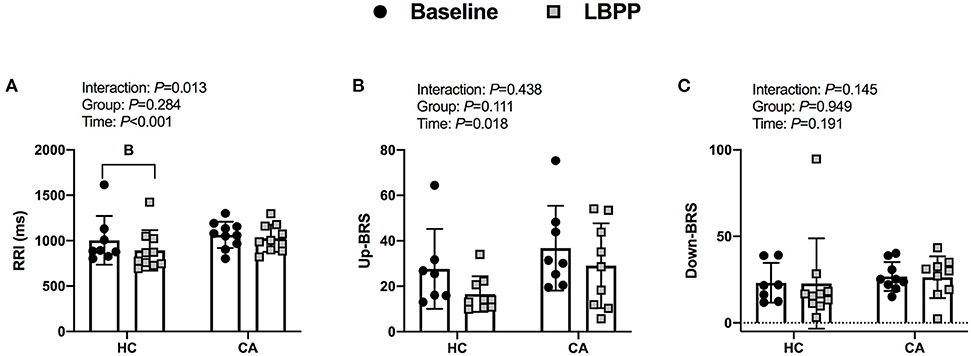
Figure 7. Indices of cardiac-baroreflex sensitivity for Healthy Controls and Concussed Athletes at baseline and LBPP. B, different from Baseline, (P ≤ 0.05).
End-Tidal CO2 Tension
End-tidal CO2 tension decreased from baseline in CA at 1 min (P = 0.044) and 2 min (P = 0.027) of LBPP, whereas HC decreased during LBPP (all P ≤ 0.028, Figure 7A). The change in end-tidal CO2 tension was not different between groups (P ≥ 0.302).
Cerebral Vascular Responses
MCAv did not change in CA (all P ≥ 0.193), but decreased in HC at 2 min of LBPP (P = 0.039). The change in MCAv was different between groups at 2 through 5 min of LBPP (2 min: P = 0.012, d = 1.38; 3 min: P = 0.039, d = 1.39; 4 min: P = 0.023, d = 1.60; 5 min: P = 0.036, d = 1.17) (Figure 8B). Cerebral vascular resistance did not change in CA (all P > 0.999), but increased in HC during LBPP (all P ≤ 0.049). The change in cerebral vascular resistance was not different between groups (P ≥ 0.065, Figure 8C).
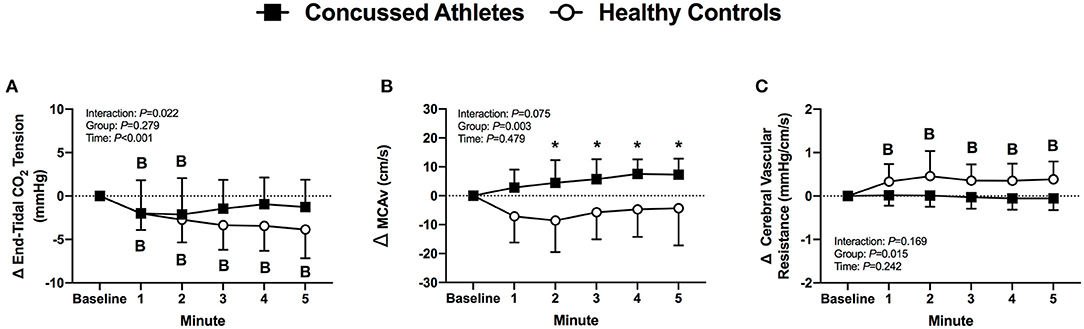
Figure 8. The change from baseline in end-tidal CO2 tension (A), MCAv (B) and cerebral vascular resistance (C) at each minute of LBPP. Values are mean ± SD. B, different from Baseline (P ≤ 0.05). *Different from Healthy Controls, (P ≤ 0.05).
Concussion Symptoms
CA experienced an exacerbation of symptoms from pre-LBPP (2 ± 2) to post-LBPP (3 ± 3, P = 0.004, Figure 9).
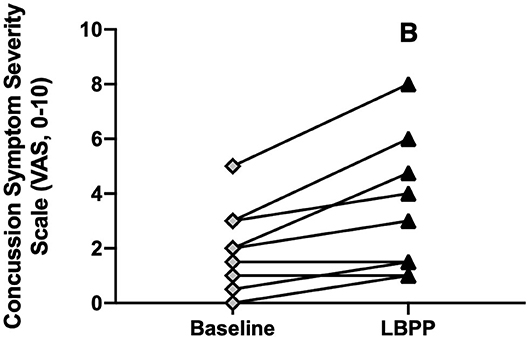
Figure 9. Concussion symptom severity rating (Visual Analog Scale, 0–10) at baseline and after LBPP for Concussed Athletes. Values are mean ± SD. B, different from baseline (P ≤ 0.05).
Discussion
We investigated the cardiovascular and cerebral vascular responses to acute periods of low and high blood pressure in symptomatic concussed college athletes and healthy controls. Our major novel findings are that concussed athletes demonstrated (1) greater orthostatic intolerance during an acute decrease in blood pressure via lower total LBNP time due to presyncopal signs and (2) different cerebral vascular responses during an acute increase in blood pressure due to an increase in MCAv compared to healthy controls.
Lower Body Negative Pressure
During an acute decrease in blood pressure, symptomatic concussed college athletes had significantly lower orthostatic tolerance compared to healthy controls. As expected, a majority of healthy controls tolerated the 5 min LBNP protocol; however, two healthy controls did not complete the protocol due to the onset of presyncopal symptoms. These results are similar to previous findings of relatively low orthostatic tolerance to a moderate LBNP challenge in young, healthy adults (28, 40–42). For example, Xiang et al. found that 33% of young healthy adults were classified as having low LBNP tolerance (42). We found that 50% of the concussed athletes exhibited presyncopal symptoms that reduced total LBNP time compared to healthy controls. The mechanisms that contributed to the low LBNP tolerance in the concussed athletes are not entirely clear based on the cardiovascular and cererbral vascular responses that we observed. We did not find any differences between groups for the blood pressure, cBRS, or MCAv responses (Figures 1–3). However, the healthy controls demonstrated some temporal increases in cerebral vascular resistance whereas the concussed athletes did not (Figure 3C).
The concussed athletes and healthy controls both exhibited reduced cBRS during the LBNP protocol, but we did not observe differences between groups. Previous evidence indicates that a concussion attenuates cBRS. La Fountaine and colleagues found that collegiate males diagnosed with a concussion have reduced spontaneous cBRS at 48 h and 1 wk post-injury compared to healthy matched controls during 5 min of quiet rest (11). Similarly, Hilz et al. reported an attenuation of cBRS in asymptomatic post-mTBI (5–43 months post-injury) during supine rest and upon standing (4). Additionally, it took longer for blood pressure to return to baseline values immediately following a Valsava maneuver in asymptomatic post-mTBI patients (4–98 months post-injury) vs. healthy controls, despite similar cardiovascular responses during the Valsalva maneuver (8). These data indicate altered blood pressure control in both symptomatic and asymptomatic mTBI patients. Our results contradict these previous findings which could be due to methodological differences and/or differences in injury recovery status as we tested within 5 ± 3 days of injury. For instance, La Fountaine and colleagues tested spontaneous cBRS at rest within 48 h and 1 wk of sport-related concussion (11), so it is unclear if baroreflex dysfunction would have been present during a perturbation. To that end, Hilz et al. (8) used postural changes to test asymptomatic post-mTBI patients whereas we tested symptomatic concussed athletes within 10 days of diagnosis using a technique that avoids vestibular activation. Despite our results differing with those of Hilz et al. (8) and La Fountaine et al. (11), animal models that have utilized the lateral fluid percussion brain injury model followed by a phenylephrine pressor test also found no change in baroreflex function within 30 min of the injury (43).
Lower Body Positive Pressure
The most novel finding from our LBPP protocol was that the healthy controls had an increase in cerebral vascular resistance whereas the concussed athletes did not (Figure 8C). Although statistically insignificant (P ≥ 0.065), the change in cerebral vascular resistance throughout LBPP was temporally different in concussed athletes and healthy controls. This was due primarily to lower MCAv responses in the healthy controls despite similar mean arterial pressure responses between groups (Figures 6A, 8B). We speculate that the healthy controls buffered the increase in mean arterial pressure to prevent overperfusion (i.e., increased MCAv) whereas the increased mean arterial pressure in the concussed athletes was likely translated to an increased cerebral perfusion, as indicated by the increase in MCAv during LBPP. Thus, the symptom exacerbation that the concussed atheletes experienced during LBPP could be explained by augmented MCAv. A rise in mean arterial pressure might cause sympathetically-induced cerebral vasoconstriction in order to prevent overperfusion. For instance, Bill and Linder demonstrated that stimulation of the cervical sympathetic chain prevented overperfusion in cats by attenuating cerebral vasodilation (44).
It is possible that other determinants of brain blood flow (e.g., end-tidal CO2 tension) could have contributed to the group differences we observed in MCAv during LBPP. Although not statistically different, the concussed athletes had a reduction in end-tidal CO2 tension of ~1 mmHg whereas healthy controls exhibited a decrease of ~4 mmHg during LBPP (Figure 8A). Using TCD, it has been shown that cerebral blood velocity decreases by 2% per mmHg decrease in end-tidal CO2 tension (45, 46). Hence, it is plasusible that the lower end-tidal CO2 tension that we observed might explain why healthy controls had lower MCAv during LBPP vs. the concussed athletes.
We postulate that the increase in MCAv contributed to the exacerbation of symptoms in the concussed college athletes following 5 min of LBPP. Previous evidence indicates a link between symptom severity and elevated regional CBF at rest using arterial spin labeling (6, 47, 48). However, our LBPP protocol augmented mean arterial pressure and MCAv, which provides a better link between cerebral perfusion pressure and concussion symptom severity. In this context, augmented CBF is a normal physiological response to aerobic exercise (49, 50). Patients who have reported persistent post-concussion symptoms exhibited an exacerbated MCAv response to aerobic exercise (51), which is thought to contribute to symptom exacerbation and exercise intolerance following concussion (52–54). However, treadmill exercise testing also activates the vestibular system and could independently increase concussion symptoms (55, 56) whereas the LBPP protocol that we employed provides a more controlled experimental protocol to study the link between increased cerebral blood velocity and concussion symptom exacerbation.
During the LBPP protocol, we observed an increase in heart rate that was temporally different in the concussed athletes and healthy controls. Although not significantly different between groups, healthy controls exhibited an increase in heart rate throughout most of the LBPP protocol (2–5 min) whereas concussed athletes did not (5 min only). We posit that the increase in heart rate along with the rise in mean arterial pressure was due to intramuscular pressure induced by LBPP that activated muscle afferents, which subsequently stimulated the cardiovascular control center. This response has been shown to override the baroreflex mediated decreased heart rate associated with increased blood pressure (29, 57–64). The temporal differences in the heart rate response that could be due to several reasons. First, intramuscular pressure sensitive receptors could be impaired in the concussed athletes. Second, afferent transduction to the cardiovascular control center could be altered in the concussed athletes. Third, the responsiveness of the heart to sympathetic stimulation could be impaired in concussed athletes. We think these reasons are unlikely because we are not aware of peripheral receptors, afferent transduction, and/or end-organ responsiveness to be influenced by a concussion. Therefore, we think there are alterations within the cardiovascular control center within the brainstem of concussed athletes. Although we did not use advanced imaging to determine the location of brain trauma, we have previously found abnormalities in the brainstem of concussed athletes using diffusion tensor imaging (65, 66). Concussion-induced brainstem changes might contribute to altered autonomic control of the cardiovascular system in the concussed athletes. We (67, 68) and others (4, 69–72) have found that autonomic control of the heart during a physioloigcal stressor is altered following concussion and might persist well-beyond symptom resolution (73). Thus, the association among structural brain abnormalities and autonomic function following a concussion warrant further investigation.
Experimental Considerations
There are several experimental considerations that pertain to the interpretation of our data. First, we did not sport-match the physically active healthy controls to the concussed athletes so there might have been a difference in fitness that contributed to the attenuated LBNP tolerance in the concussed athletes. Highly trained endurance athletes tend to have lower tolerance to orthostatic stress due to structural and mechanical cardiac changes that result in reduced stroke volume due to a steeper slope of the left cardiac pressure-stroke volume relationship (74) or to an altered central venous pressure-central blood volume relation (75) during an orthostatic challenge. However, most of the concussed athletes did not participate in traditional endurance sports (i.e., track, cycling, etc.). We recruited physically active healthy participants who were not currently participating in recreational collision sports to serve as controls in order to avoid the unknown physiological effects of repetitive subconcussive hits on orthostatic tolerance and cerebral vascular function. Second, we only recorded concussion symptom severity before and after each protocol from the concussed athletes. We cannot conclude that the exacerbation of concussion-like symptoms experienced during LBNP and LBPP would not have been experienced by the healthy controls. Thus, it remains unclear if symptom exacerbation following the protocols occurs only in acutely concussed athletes or if it is a normal response to experiencing a brief period of central hypo- and hypervolemia. The visual analog symptom severity scale also does not provide information on categorical symtpoms (e.g., headache, dizziness, nausea). Therefore, we cannot provide insight whether only pre-testing symptoms were exacerbated throughout the test, if new symptoms arose from pre- to post-testing, or if categorical symtpoms differed between the protocols. Third, TCD is a non-invasive tool used to measure beat-to-beat cerebral blood velocity of various intra-cranial arteries, primarly to assess responses to changes in arterial CO2 and/or blood pressure. TCD does not provide a measure of artery diameter and thus the assumption is made that changes in cerebral blood velocity directly reflect changes in CBF (i.e., artery diameter remains constant). Giller et al. reported small changes in MCA diameter (~2-2.5%) with alterations in arterial CO2 (±10 mmHg) and blood pressure (±18 mmHg) during craniotomy (76). On the other hand, MRI data revealed constant MCA diameter during a hypercapnia protocol that increased PETCO2 by ~8 mmHg (77). We cannot confirm if the changes observed in MCAv are due to alterations in artery diameter or cause changes in cerebral blood flow. Fourth, we did not clamp end-tidal CO2 tension to match baseline values. Thus, we cannot draw conclusions on the mechanisms that contributed to the MCAv during decreases and increases in blood pressure since end-tidal CO2 tension was not constant throughout the protocols. Although this approach reduces our capability to fully understand changes in cerebral blood flow regulation, we think it does enhance our ability to understand the physiology of concussion patients outside of the laboratory (e.g., greater external validity). Fifth, women were tested at different phases of the menstrual cycle due to our criteria of testing within the first 10 days of injury while symptomatic. It is possible that menstrual cycle phase influenced our findings. Sixth, because of the relatively large number of early LBNP terminations in the CA group, we have a low number of participants for some of the statistical comparisions. Lastly, we did not reassess CA after they were clinically cleared to return to play. This approach would have allowed us to determine if CA returned to physiological function that is exhibited by healthy controls prior to their return to play. Important emerging evidence indicates that physiological dysfunction persists in concussed patients beyond symptom resolution (4, 8, 73).
Perspectives and Significance
Recently concussed athletes can exhibit signs of exercise intolerance (78). There is mounting evidence that baroreflex dysfunction and altered cerebral vascular function are present following a concussion, which might contribute to exercise intolerance. At rest and during exercise, systemic blood pressure and intracranial pressure control are critical for maintaining blood flow to meet cerebral oxygen demand. The brain is susceptible to hypo- and/or hyperperfusion if systemic blood pressure (i.e., baroreflex) and/or intracranial pressure (i.e., cerebral autoregulation) control is/are impaired. In the present study, we assessed the cardiovascular and cerebral vascular responses to acute increases and decreases in blood pressure in symptomatic concussed college athletes vs. recreationally active healthy controls. Our data suggest a greater prevalence of orthostatic intolerance and different MCAv responses during the first 10 days after sustaining a sport-related concussion, both of which were associated with symptom exacerbation. These findings provide insight into the mechanism(s) for concussion symptoms (e.g., dizziness and headache) as these protocols simulate the blood pressure responses to postural changes (i.e., sit-to-stand) and aerobic exercise without activating the vestibular system. Although we recognize that more research is warranted, the prevalence of orthostatic intolerance observed in symptomatic concussed athletes demonstrates that examining the physiological response to a stressor might be an additional and useful tool to assist clinicians with their return-to-play decisions.
Conclusion
Although our LBNP protocol was not designed to test orthostatic tolerance, we found that symptomatic concussed athletes experienced attenuated orthostatic tolerance compared to recreationally active healthy controls. Additionally, LBPP induced similar changes in mean arterial pressure and end-tidal CO2 tension between groups, however MCAv was elevated only in symptomatic concussed athletes which could have contributed to concussion symptom exacerbation.
Data Availability Statement
The raw data supporting the conclusions of this article will be made available by the authors, without undue reservation.
Ethics Statement
The studies involving human participants were reviewed and approved by University at Buffalo Institutional Review Board. The patients/participants provided their written informed consent to participate in this study.
Author Contributions
ZS, BW, JL, and BJ conceived the study and designed the experiment. MO'L, JS, ZS, and BJ performed experiments and collected the data. MW, MO'L, and JS extracted and analyzed data. MW and BJ performed statistical analyses. MW drafted the manuscript. MW, MO'L, JS, ZS, BW, JL, and BJ edited and approved the final manuscript. All authors contributed to the article and approved the submitted version.
Funding
This study was supported by Grant No. UL1TR001412 from the National Center for Advancing Translational Sciences of the National Institutes of Health Pilot Grants Award Program BJ and Grant No. 1R01NS094444 from the National Institute of Neurological Disorders and Stroke of the National Institutes of Health JL.
Disclaimer
The content is solely the responsibility of the authors and does not necessarily represent the official views of the National Institutes of Health.
Conflict of Interest
The authors declare that the research was conducted in the absence of any commercial or financial relationships that could be construed as a potential conflict of interest.
Acknowledgments
We thank the participants for completing the study. We also thank Mr. Suman Sarker, MPH, for his technical assistance and Mr. Joel Greenshields, MS, for his statistical assistance.
References
1. Esterov D, Greenwald BD. Autonomic dysfunction after mild traumatic brain injury. Brain Sci. (2017) 7:100. doi: 10.3390/brainsci7080100
2. Giza CC Hovda DA The new neurometabolic cascade of concussion. Neurosurgery. (2014) 75(Suppl. 4):S24–33. doi: 10.1227/NEU.0000000000000505
3. Kenney K, Amyot F, Haber M, Pronger A, Bogoslovsky T, Moore C, et al. Cerebral vascular injury in traumatic brain injury. Exp Neurol. (2016) 275:353–66. doi: 10.1016/j.expneurol.2015.05.019
4. Hilz MJ, DeFina PA, Anders S, Koehn J, Lang CJ, Pauli E, et al. Frequency analysis unveils cardiac autonomic dysfunction after mild traumatic brain injury. J Neurotrauma. (2011) 28:1727–38. doi: 10.1089/neu.2010.1497
5. Wang Y, Nelson LD, LaRoche AA, Pfaller AY, Nencka AS, Koch KM, et al. Cerebral blood flow alterations in acute sport-related concussion. J Neurotrauma. (2016) 33:1227–36. doi: 10.1089/neu.2015.4072
6. Churchill NW Hutchison MG Graham SJ Schweizer TA Symptom correlates of cerebral blood flow following acute concussion. Neuroimage Clin. (2017) 16:234–9. doi: 10.1016/j.nicl.2017.07.019
7. Becelewski J, Pierzchala K. Dynamic assessment of blood flow in pre-cranial and intracranial arteries in patients with mild head injuries. Neurol Neurochir Pol. (2002) 36:1135–45; discussion 1146–8.
8. Hilz MJ, Liu M, Koehn J, Wang R, Ammon F, Flanagan SR, et al. Valsalva maneuver unveils central baroreflex dysfunction with atlered blood pressure control in persons with a history of mild traumatic brain injury. BMC Neurology. (2016) 16:61. doi: 10.1186/s12883-016-0584-5
9. Wright AD Smirl JD Bryk K Fraser S Jakovac M van Donkelaar P Sport-related concussion alters indices of dynamic cerebral autoregulation. Front Neurol. (2018) 9:196. doi: 10.3389/fneur.2018.00196
10. Bailey DM, Jones DW, Sinnott A, Brugniaux JV, New KJ, Hodson D, et al. Impaired cerebral haemodynamic function associated with chronic traumatic brain injury in professional boxers. Clin Sci (Lond). (2013) 124:177–89. doi: 10.1042/CS20120259
11. La Fountaine MF Hohn AN Testa AJ Weir JP Attenuation of spontaneous baroreceptor sensitivity after concussion. Med Sci Sports Exerc. (2019) 51:792–7. doi: 10.1249/MSS.0000000000001833
12. Anderson EA, Sinkey CA, Mark AL. Mental stress increases sympathetic nerve activity during sustained baroreceptor stimulation in humans. Hypertension. (1991) 17:III43–9. doi: 10.1161/01.HYP.17.4_Suppl.III43
13. Callister R, Suwarno NO, Seals DR. Sympathetic activity is influenced by task difficulty and stress perception during mental challenge in humans. J Physiol. (1992) 454:373–87. doi: 10.1113/jphysiol.1992.sp019269
14. Carter JR Ray CA Sympathetic neural responses to mental stress: responders nonresponders and sex differences. Am J Physiol Heart Circ Physiol. (2009) 296:H847–53. doi: 10.1152/ajpheart.01234.2008
15. Le VV, Mitiku T, Sungar G, Myers J, Froelicher V. The blood pressure response to dynamic exercise testing: a systematic review. Prog Cardiovasc Dis. (2008) 51:135–60. doi: 10.1016/j.pcad.2008.07.001
16. Moir ME, Balestrini CS, Abbott KC, Klassen SA, Fischer LK, Fraser DD, et al. An investigation of dynamic cerebral autoregulation in adolescent concussion. Med Sci Sports Exerc. (2018) 50:2192–9. doi: 10.1249/MSS.0000000000001695
17. Mucha A, Fedor S, DeMarco D. Vestibular dysfunction and concussion. Handb Clin Neurol. (2018) 158:135–44. doi: 10.1016/B978-0-444-63954-7.00014-8
18. McCrory P, Meeuwisse W, Dvorak J, Aubry M, Bailes J, Broglio S, et al. Consensus statement on concussion in sport-the 5(th) international conference on concussion in sport held in Berlin, October 2016. Br J Sports Med. (2017) 51:838–47. doi: 10.1136/bjsports-2017-098065
19. Sady MD, Vaughan CG, Gioia GA. Psychometric characteristics of the postconcussion symptom inventory in children and adolescents. Arch Clin Neuropsychol. (2014) 29:348–63. doi: 10.1093/arclin/acu014
20. Haider MN, Leddy JJ, Du W, Macfarlane AJ, Viera KB, Willer BS. Practical management: brief physical examination for sport-related concussion in the outpatient setting. Clin J Sport Med. (2020) 30:513–7. doi: 10.1097/JSM.0000000000000687
21. Peltonen GL, Harrell JW, Aleckson BP, LaPlante KM, Crain MK, Schrage WG. Cerebral blood flow regulation in women across menstrual phase: differential contribution of cyclooxygenase to basal, hypoxic, and hypercapnic vascular tone. Am J Physiol Regul Integr Comp Physiol. (2016) 311:R222–31. doi: 10.1152/ajpregu.00106.2016
22. Abidi S, Nili M, Serna S, Kim S, Hazlett C, Edgell H. Influence of sex, menstrual cycle, and oral contraceptives on cerebrovascular resistance and cardiorespiratory function during Valsalva or standing. J Appl Physiol (1985). (2017) 123:375–86. doi: 10.1152/japplphysiol.00035.2017
23. Tanaka M, Sato M, Umehara S, Nishikawa T. Influence of menstrual cycle on baroreflex control of heart rate: comparison with male volunteers. Am J Physiol Regul Integr Comp Physiol. (2003) 285:R1091–7. doi: 10.1152/ajpregu.00162.2003
24. Willie CK, Macleod DB, Shaw AD, Smith KJ, Tzeng YC, Eves ND, et al. Regional brain blood flow in man during acute changes in arterial blood gases. J Physiol. (2012) 590:3261–75. doi: 10.1113/jphysiol.2012.228551
25. Stevens PM, Lamb LE. Effects of lower body negative pressure on the cardiovascular system. Am J Cardiol. (1965) 16:506–15. doi: 10.1016/0002-9149(65)90027-5
26. Hoffler GW, Wolthuis RA, Johnson RL. Apollo space crew cardiovascular evaluations. Aerosp Med. (1974) 45:807–23.
27. Wolthuis RA, Bergman SA, Nicogossian AE. Physiological effects of locally applied reduced pressure in man. Physiol Rev. (1974) 54:566–95. doi: 10.1152/physrev.1974.54.3.566
28. Rickards CA, Johnson BD, Harvey RE, Convertino VA, Joyner MJ, Barnes JN. Cerebral blood velocity regulation during progressive blood loss compared with lower body negative pressure in humans. J Appl Physiol (1985). (2015) 119:677–85. doi: 10.1152/japplphysiol.00127.2015
29. Shi X, Crandall CG, Raven PB. Hemodynamic responses to graded lower body positive pressure. Am J Physiol. (1993) 265:H69–73. doi: 10.1152/ajpheart.1993.265.1.H69
30. Perry BG, Schlader ZJ, Raman A, Cochrane DJ, Lucas SJ, Mundel T. Middle cerebral artery blood flow velocity in response to lower body positive pressure. Clin Physiol Funct Imaging. (2013) 33:483–8. doi: 10.1111/cpf.12046
31. Yoshida H, Hamner JW, Ishibashi K, Tan C. Relative contributions of systemic hemodynamic variables to cerebral autoregulation during orthostatic stress. J Appl Physiol (1985). (2018) 124:321–9. doi: 10.1152/japplphysiol.00700.2017
32. Wesseling KH, Jansen JR, Settels JJ, Schreuder JJ. Computation of aortic flow from pressure in humans using a nonlinear, three-element model. J Appl Physiol (1985). (1985) 74:2566–73. doi: 10.1152/jappl.1993.74.5.2566
33. Pinna GD, Maestri R. Reliability of transfer function estimates in cardiovascular variability analysis. Med Biol Eng Comput. (2001) 39:338–47. doi: 10.1007/BF02345289
34. Claassen JA, Meel-van den Abeelen AS, Simpson DM, Panerai RB. Transfer function analysis of dynamic cerebral autoregulation: a white paper from the International Cerebral Autoregulation Research Network. J Cereb Blood Flow Metab. (2016) 36:665–80. doi: 10.1177/0271678X15626425
35. Bertinieri G, di Rienzo M, Cavallazzi A, Ferrari AU, Pedotti A, Mancia G. A new approach to analysis of the arterial baroreflex. J Hypertens Suppl. (1985) 3:S79–81.
36. Laude D, Baudrie V, Elghozi JL. Tuning of the sequence technique. IEEE Eng Med Biol Mag. (2009) 28:30–4. doi: 10.1109/MEMB.2009.934630
37. Parati DRM, Mancia G. How to measure baroreflex sensitivity: from the cardiovascular laboratory to daily life. J Hypertens. (2000) 18:7–19. doi: 10.1097/00004872-200018010-00003
38. Gross V, Plehm R, Tank J, Jordan J, Diedrich A, Obst M, et al. Heart rate variability and baroreflex function in AT2 receptor-disrupted mice. Hypertension. (2002) 40:207–13. doi: 10.1161/01.HYP.0000027279.69240.75
39. Tybout AM, Sternthal B. Can I test for simple effects in the presence of an insignificant interaction? J Consumer Psychol. (2001) 10:9–10. doi: 10.2307/1480447
40. Hinojosa-Laborde C, Rickards CA, Ryan KL, Convertino VA. Heart rate variability during simulated hemorrhage with lower body negative pressure in high and low tolerant subjects. Front Physiol. (2011) 2:85. doi: 10.3389/fphys.2011.00085
41. Johnson BD, van Helmond N, Curry TB, van Buskirk CM, Convertino VA, Joyner MJ. Reductions in central venous pressure by lower body negative pressure or blood loss elicit similar hemodynamic responses. J Appl Physiol (1985). (2014) 117:131–41. doi: 10.1152/japplphysiol.00070.2014
42. Xiang L, Hinojosa-Laborde C, Ryan KL, Rickards CA, Convertino VA. Time course of compensatory physiological responses to central hypovolemia in high- and low-tolerant human subjects. Am J Physiol Regul Integr Comp Physiol. (2018) 315:R408–16. doi: 10.1152/ajpregu.00361.2017
43. McMahon CG, Kenny R, Bennett K, Little R, Kirkman E. Effect of acute traumatic brain injury on baroreflex function. Shock. (2011) 35:53–8. doi: 10.1097/SHK.0b013e3181e687c6
44. Bill A, Linder J. Sympathetic control of cerebral blood flow in acute arterial hypertension. Acta Physiol Scand. (1976) 96:114–21. doi: 10.1111/j.1748-1716.1976.tb10176.x
45. Hoiland RL, Fisher JA, Ainslie PN. Regulation of the cerebral circulation by arterial carbon dioxide. Compr Physiol. (2019) 9:1101–54. doi: 10.1002/cphy.c180021
46. Ide K, Eliasziw M, Poulin MJ. Relationship between middle cerebral atery blood velocity and end-tidal PCO2 in the hypocapnic-hypercapnic range in humans. J Appl Physiol (1985). (1985) 95:129–37. doi: 10.1152/japplphysiol.01186.2002
47. Lin CM, Tseng YC, Hsu HL, Chen CJ, Chen DY, Yan FX, et al. Arterial spin labeling perfusion study in the patients with subacute mild traumatic brain injury. PLoS ONE. (2016) 11:e0149109. doi: 10.1371/journal.pone.0149109
48. Barlow K, Lorenzo M, Calson H, Marc L. Cerebral perfusion changes in children with and without post-concussion syndrome following a mild traumatic brain injury using arterial spin labelling. Neurology. (2016) 86:P3.321.
49. Ide K, Secher NH. Cerebral blood flow and metabolism during exercise. Prog Neurobiol. (2000) 61:397–414. doi: 10.1016/S0301-0082(99)00057-X
50. Querido JS, Sheel AW. Regulation of cerebral blood flow during exercise. Sports Med. (2007) 37:765–82. doi: 10.2165/00007256-200737090-00002
51. Clausen M, Pendergast DR, Willer B, Leddy J. Cerebral blood flow during treadmill exercise is a marker of physiological postconcussion syndrome in female athletes. J Head Trauma Rehabil. (2016) 31:215–24. doi: 10.1097/HTR.0000000000000145
52. Kozlowski KF, Graham J, Leddy JJ, Devinney-Boymel L, Willer BS. Exercise intolerance in individuals with postconcussion syndrome. J Athl Train. (2013) 48:627–35. doi: 10.4085/1062-6050-48.5.02
53. Leddy JJ, Kozlowski K, Donnelly JP, Pendergast DR, Epstein LH, Willer B. A preliminary study of subsymptom threshold exercise training for refractory post-concussion syndrome. Clin J Sport Med. (2010) 20:21–7. doi: 10.1097/JSM.0b013e3181c6c22c
54. Leddy JJ, Kozlowski K, Fung M, Pendergast DR, Willer B. Regulatory and autoregulatory physiological dysfunction as a primary characteristic of post-concussion syndrome: implications for treatment. NeuroRehabilitation. (2007) 22:199–205. doi: 10.3233/NRE-2007-22306
55. Haider MN, Johnson SL, Mannix R, Macfarlane AJ, Constantino D, Johnson BD, et al. The buffalo concussion bike test for concussion assessment in adolescents. Sports Health. (2019) 11:492–7. doi: 10.1177/1941738119870189
56. Wallace B, Lifshitz J. Traumatic brain injury and vestibulo-ocular function: current challenges and future prospects. Eye Brain. (2016) 8:153–64. doi: 10.2147/EB.S82670
57. McWilliam PN, Yang T. Inhibition of cardiac vagal component of baroreflex by group III and IV afferents. Am J Physiol. (1991) 260:H730–4. doi: 10.1152/ajpheart.1991.260.3.H730
58. Mitchell JH, Kaufman MP, Iwamoto GA. The exercise pressor reflex: its cardiovascular effects, afferent mechanisms, and central pathways. Annu Rev Physiol. (1983) 45:229–42. doi: 10.1146/annurev.ph.45.030183.001305
59. McCloskey DI, Mitchell JH. Reflex cardiovascular and respiratory responses originating in exercising muscle. J Physiol. (1972) 224:173–86. doi: 10.1113/jphysiol.1972.sp009887
60. Osterziel KJ, Julius S, Brant DO. Blood pressure elevation during hindquarter compression in dogs is neurogenic. J Hypertens. (1984) 2:411–7. doi: 10.1097/00004872-198408000-00014
61. Julius S. The blood pressure seeking properties of the central nervous system. J Hypertens. (1988) 6:177–85. doi: 10.1097/00004872-198803000-00001
62. Osterziel KJ, Julius S, Brant D Pressure increase to external hind quarter compression in dogs: a facilitative regulatory response. J Hypertens. (1986) 4:S54–6.
63. Sanchez R, Julius S, Malayan S, Hamlin M, Elkins M, Brant D, et al. Sustained blood pressure elevation to lower body compression in pigs and dogs. Hypertension Dallas. (1982) 4:782. doi: 10.1161/01.HYP.4.6.782
64. Nishiyasu T, Nagashima K, Nadel ER, Mack GW. Effects of posture on cardiovascular responses to lower body positive pressure at rest and during dynamic exercise. J Appl Physiol (1985). (1998) 85:160–7. doi: 10.1152/jappl.1998.85.1.160
65. Polak P, Leddy JJ, Dwyer MG, Willer B, Zivadinov R. Diffusion tensor imaging alterations in patients with postconcussion syndrome undergoing exercise treatment: a pilot longitudinal study. J Head Trauma Rehabil. (2015) 30:E32–42. doi: 10.1097/HTR.0000000000000037
66. Hirad AA, Bazarian JJ, Merchant-Borna K, Garcea FE, Heilbronner S, Paul D, et al. A common neural signature of brain injury in concussion and subconcussion. Sci Adv. (2019) 5:eaau3460. doi: 10.1126/sciadv.aau3460
67. Johnson BD, O'Leary MC, McBryde M, Sackett JR, Schlader ZJ, Leddy JJ. Face cooling exposes cardiac parasympathetic and sympathetic dysfunction in recently concussed college athletes. Physiol Rep. (2018) 6:e13694. doi: 10.14814/phy2.13694
68. Johnson BD, Sackett JR, Schlader ZJ, Leddy JJ. Attenuated cardiovascular responses to the cold pressor test in concussed collegiate athletes. J Athl Train. (2020) 55:124–31. doi: 10.4085/1062-6050-573-18
69. King ML, Lichtman SW, Seliger G, Ehert FA, Steinberg JS. Heart-rate variability in chronic traumatic brain injury. Brain Inj. (1997) 11:445–53. doi: 10.1080/026990597123421
70. Gall B, Parkhouse W, Goodman D. Heart rate variability of recently concussed athletes at rest and exercise. Med Sci Sports Exerc. (2004) 36:1269–74. doi: 10.1249/01.MSS.0000135787.73757.4D
71. La Fountaine MF Heffernan KS Gossett JD Bauman WA De Meersman RE Transient suppression of heart rate complexity in concussed athletes. Auton Neurosci. (2009) 148:101–3. doi: 10.1016/j.autneu.2009.03.001
72. Abaji JP, Curnier D, Moore RD, Ellemberg D. Persisting effects of concussion on heart rate variability during physical exertion. J Neurotrauma. (2016) 6:e13694. doi: 10.1089/neu.2015.3989
73. Nadir HM, Johnson BD, Horn Emily C, Leddy John J, Wilber Charles G, Reed Emma L, et al. Blunted cardiac parasympathetic activation in student athletes with a remote history of concussion: a pilot study. Front Neurol. (2020) 11:1156. doi: 10.3389/fneur.2020.547126
74. Levine BD, Lane LD, Buckey JC, Friedman DB, Blomqvist CG. Left ventricular pressure-volume and frank-starling relations in endurance athletes. Implications for orthostatic tolerance and exercise performance. Circulation. (1991) 84:1016–23. doi: 10.1161/01.CIR.84.3.1016
75. Ogoh S, Volianitis S, Nissen P, Wray DW, Secher NH, Raven PB. Carotid baroreflex responsiveness to head-up tilt-induced central hypovolaemia: effect of aerobic fitness. J Physiol. (2003) 551:601–8. doi: 10.1113/jphysiol.2003.046029
76. Giller CA, Bowman G, Dyer H, Mootz L, Krippner W. Cerebral arterial diameters during changes in blood pressure and carbon dioxide during craniotomy. Neurosurgery. (1993) 32:737–41; discussion 741–2. doi: 10.1097/00006123-199305000-00006
77. Serrador JM, Picot PA, Rutt BK, Shoemaker JK, Bondar RL. MRI measures of middle cerebral artery diameter in conscious humans during simulated orthostasis. Stroke. (2000) 31:1672–8. doi: 10.1161/01.STR.31.7.1672
Keywords: mild traumatic brain injury, cerebral blood flow, blood pressure, baroreflex, autonomic function
Citation: Worley ML, O'Leary MC, Sackett JR, Schlader ZJ, Willer B, Leddy JJ and Johnson BD (2021) Preliminary Evidence of Orthostatic Intolerance and Altered Cerebral Vascular Control Following Sport-Related Concussion. Front. Neurol. 12:620757. doi: 10.3389/fneur.2021.620757
Received: 23 October 2020; Accepted: 08 March 2021;
Published: 09 April 2021.
Edited by:
Ramon Diaz-Arrastia, University of Pennsylvania, United StatesReviewed by:
Alexa Walter, University of Pennsylvania, United StatesAnthony P. Kontos, University of Pittsburgh, United States
Copyright © 2021 Worley, O'Leary, Sackett, Schlader, Willer, Leddy and Johnson. This is an open-access article distributed under the terms of the Creative Commons Attribution License (CC BY). The use, distribution or reproduction in other forums is permitted, provided the original author(s) and the copyright owner(s) are credited and that the original publication in this journal is cited, in accordance with accepted academic practice. No use, distribution or reproduction is permitted which does not comply with these terms.
*Correspondence: Blair D. Johnson, YmozM0BpbmRpYW5hLmVkdQ==