- 1School of Civil and Construction Engineering, Oregon State University, Corvallis, OR, United States
- 2Advanced Lighting Team, Pacific Northwest National Laboratory, Portland, OR, United States
- 3Lighting Research Solutions LLC, Cambridge, MA, United States
At its best, human-centric lighting considers the visual and non-visual effects of light in support of positive human outcomes. At its worst, it is a marketing phrase used to healthwash lighting products or lighting design solutions. There is no doubt that environmental lighting contributes to human health, but how might one practice human-centric lighting given both the credible potential and the implausible hype? Marketing literature is filled with promises. Technical lighting societies have summarized the science but have not yet offered design guidance. Meanwhile, designers are in the middle, attempting to distinguish credible knowledge from that which is dubious to make design decisions that affect people directly. This article is intended to: (1) empower the reader with fundamental understandings of ways in which light affects health; (2) provide a process for human-centric lighting design that can dovetail with the decision-making process that is already a part of a designer's workflow.
Introduction
Human-centric lighting is an idiom intended to describe lighting solutions that considers the traditional elements of lighting quality that are rooted in human vision while simultaneously incorporating new insights about the non-visual effects of light. Recently, Houser et al. (1) outlined the rise of human-centric lighting and its current status in lighting. That manuscript describes a range of visual and non-visual responses and the eye-brain pathways that drive them, outlines the agreed upon science that can inform the practice of human-centric lighting, and offers general guidance for the practice of human-centric lighting. That work asserts that human-centric lighting, also called integrative lighting (2, 3), is not a product feature, and that lighting products that claim to improve sleep or performance should be met with skepticism.
Instead, human-centric lighting begins with effective prioritization of design goals and is an outcome of good decision making at every step in the lighting design process. Human-centric lighting begins with project conceptualization, and continues through prioritization of design goals, architectural design (including daylighting design), lighting equipment specification, commissioning, and operation of the lighting systems. Successful implementation requires buy-in from all stakeholders involved in building design, construction, and operation, including occupants.
The stakeholders with the most at stake are occupants, since it is their health, well-being, and cognitive performance that we are concerned about. Yet, designers cannot influence light exposure when occupants are elsewhere, such as at home, in transit, or in other buildings. Individual outcomes will vary based on individual lifestyles and habits. As will be expanded upon later, the best that a designer can do is to provide the proper light at the proper time for the projects that they design.
Since many American adults spend about 90% of their time in buildings (4, 5), we believe that human-centric lighting should almost always be a part of a designer's scope and a building owner's desires. For the many people that spend their time indoors, days are dimmer and nights are brighter than would be experienced in nature (6–8). Thus, while natural light from the sky and sun is ideal for many health outcomes associated with light, electric lighting has a critical role in supporting human health in the modern world.
Implementing a successful human-centric lighting solution is complex. The goal of this work, therefore, is to outline the early stages of the design process for projects where human-centric lighting is deemed important. We offer a five-step process that will help organize information gathering and decision-making. Though no standard for biologically effective lighting has gone through the full consensus process required by ANSI, ISO, or IEC, we consider compliance with WELL v2 (9) and UL Design Guideline 24480 (10) as they may be appropriate for some projects. In support of informed decision making, we summarize relevant responses of people to light and lighting. Our review is intended to not overwhelm the reader with every study that links light with human physiology. Instead, we offer enough background to describe the unequivocal physiological responses to light, including why such responses may not always affect real-world outcomes. The content covered in this manuscript is complemented by reviews by others (1, 11–16).
Foundational Considerations
The goal of this section is to succinctly introduce the principles of human-centric lighting in support of conversational understanding of the most relevant considerations for lighting design.
The Stimulus Response Relationship Between Light and Human Outcomes
Figure 1 is a schematic of the stimulus response relationship between light and human outcomes. The top four categories are lighting variables that can be manipulated in the design of a light stimulus, whether in lighting experiments or in the design of the built environment. These categories are temporal pattern, light level, light spectrum, and spatial pattern.
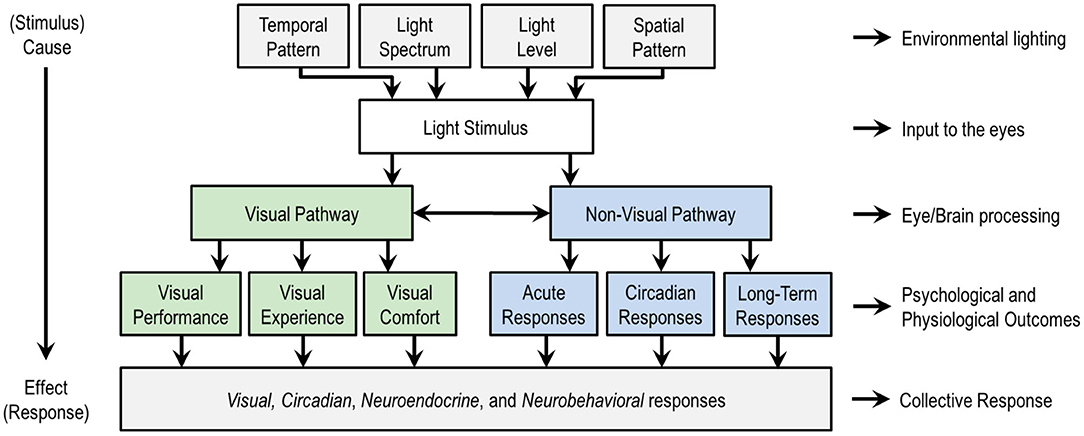
Figure 1. An overview of the stimulus (top) response (bottom) relationship between light and human responses, with a schematic subdivision of the visual and non-visual responses. At the top level, the temporal pattern relates the timing and duration of exposure to a light stimulus, spatial pattern refers to the spatial distribution of light in the three-dimensional light field, light spectrum refers to the spectral power distribution (SPD) that governs color qualities, and light level refers to the quantity of light in radiometric or photometric units. These four factors contribute to the biological potency of the light stimulus. Designers often vary factors together, though any of the factors can be disengaged from any other. Researchers usually vary just a small number of factors, sometimes only one, to isolate cause and effect. Non-lighting factors not shown such as age and chronotype moderate the effects of light on people and are important in practice. This figure only considers the effects of light through the eyes; the effects of optical radiation on or through the skin are not considered here. Figure inspired by de Kort and Veitch (17).
These four aspects of the light stimulus can be manipulated to influence two categories of human responses to light, visual and non-visual, as illustrated in the middle of Figure 1. A visual response is an eye-brain response that enables sight, contributing to visual performance, the visual experience including emotional responses, and visual comfort (or discomfort, as with glare). Non-visual responses might also be called non-image forming (NIF) effects of light, biological responses, or physiological responses. CIE adopted the phrase ipRGC-influenced light responses (18) and IES uses the phrase visual, circadian, neuroendocrine, and neurobehavioral responses (19). Circadian responses are internal biological processes that occur on a roughly 24-h period, such as the sleep-wake cycle. Neuroendocrine responses refer to how the brain regulates hormones, such as expression of melatonin. Neurobehavioral responses refer to the relationship between the action of the nervous system and human behavior. The IES terminology is adopted in the bottom of Figure 1 because this collection of responses encompasses all the ways that light may affect people through the eyes. Table 1 provides examples of physiological and psychophysical responses that are important to human health, well-being, cognition, and performance, and which may be influenced by light and lighting over different time periods.
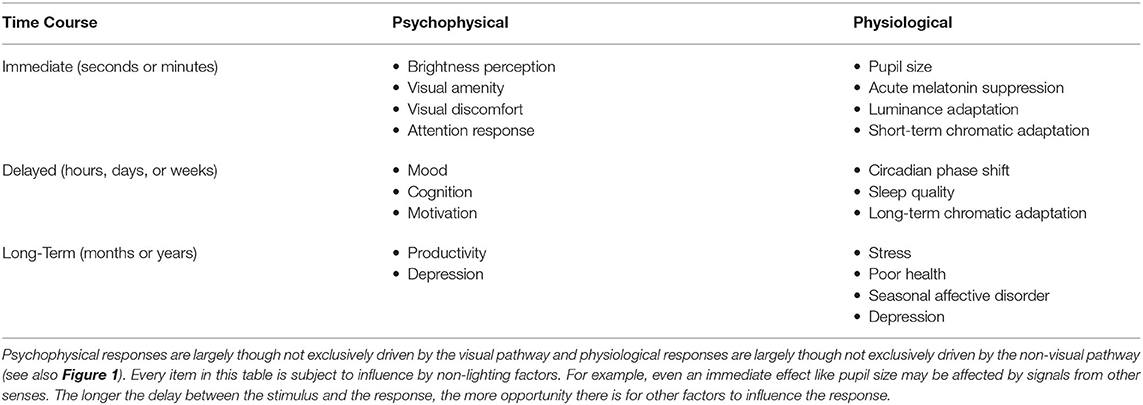
Table 1. Examples of psychophysical and physiological responses that are influenced by light and lighting.
The four categories of lighting variables identified in the top row of Figure 1 have unequal influences on non-visual responses to light, and thus contribute unequally to the biological potency of a light stimulus. Biological potency refers to the strength of influence of a light stimulus on a human biological response.
The temporal pattern is most important to non-visual responses because the brain tells time by observing nature's daily pattern of light and dark (20). The same light stimulus may be beneficial at one time of day but detrimental at another. For day-active people—that is, people who are active during the day, relatively less active in the evening, and sleep at night—bright light in the morning and during the day will support health, whereas bright light in the evening may delay the onset of sleep and be detrimental to health.
At any given time, the biological potency of a light stimulus can be altered first by adjusting light level and second by adjusting light spectrum. Brighter light with proportionally more short-wavelength radiation, coinciding with the melanopsin action spectrum, is more biologically potent than dimmer light with proportionally less short-wavelength radiation that coincides with the melanopsin action spectrum. At constant illuminance, broadband sources with a higher CCT are often more biologically potent than those with a lower CCT, though this depends upon how biological potency is quantified. CCT, however, is a one-dimensional reduction of a light source SPD that cannot reliably predict biological potency, a limitation that is especially apparent for color-mixed LEDs (21, 22) or any other SPD with pronounced peaks and valleys.
With respect to the spatial pattern of light, light exposure on the lower retina more effectively suppresses melatonin than light on the upper retina (23), and light exposure on the nasal side of the retina is more biologically potent than light exposure on the temporal side of the retina (24, 25). In building interiors, these effects are likely dwarfed by gaze direction. Looking toward a bright window will produce a more biologically potent light stimulus than looking toward a dimly lit interior wall. Lighting criteria for vision are at task locations and are often oriented horizontally (26), whereas criteria for circadian lighting design are at the plane of the occupant's eyes and are oriented vertically (9, 10, 27). There are opportunities for novel lighting solutions that balance the need to deliver light at the plane of an observer's eyes without causing visual discomfort.
Quantifying the Biological Potency of Light for Human-Centric Lighting Design
Visual and non-visual responses are driven by photoreceptors that reside in the retina and send signals to the brain. Visual responses in humans are largely driven by the rods and cones. Non-visual responses are largely driven by intrinsically photosensitive retinal ganglion cells (ipRGCs) (28, 29). The ipRGCs combine their own intrinsic response to light with extrinsic inputs from the rods and cones (30). Though the understanding of the balance between extrinsic and intrinsic signaling in the various ipRGC subtypes (31) is incomplete, it is known that all photoreceptors contribute to both visual and non-visual responses.
There are two prevalent methods for quantifying light as a non-visual stimulus: (1) based on the spectral response of the photopigments in the rods, cones, and ipRGCs (18, 32), (2) based on nocturnal suppression of the hormone melatonin (33–36).
Equivalent Melanopic Lux (EML) introduced by Lucas et al. (32) is computed as the product of Photopic Illuminance, E, and the Melanopic Ratio, R, where:
EML is expressed in units of melanopic lux (m-lux), which is not recognized by the International System of Units (SI). R is computed as the ratio of a light source's melanopsin-activating radiation to its photopic-activating radiation, multiplied by 1.218, which guarantees that R = 1.0 for the Equal Energy illuminant. R is unitless and ranges from ~0.45 to 1.70. On average, light sources with a higher CCT also have a higher value for R.
CIE has proscribed the use of EML and has proposed an SI compliant quantity as its replacement (18). Melanopic Equivalent Daylight Illuminance, melanopic EDI, , or “mel-EDI” for short, is the illuminance of standard daylight (D65), at a point, that provides equal melanopic irradiance as the test source. For example, a mel-EDI of 100 lx means that the light source under evaluation produces the same amount of melanopsin-activating radiation as 100 lx of daylight at 6,500 K. It is computed as the product of photopic illuminance, Ev, and the melanopic Daylight Efficacy Ratio, melanopic DER, , or “mel-DER” for short, where:
(mel-EDI) is expressed in units of lux, which is an SI-compliant unit. (mel-DER) is computed as the ratio of a test source's melanopic efficacy of luminous radiation to the melanopic efficacy of luminous radiation of D65. Melanopic DER is unitless and ranges from ~0.40 to 1.60. EML and mel-EDI are related by a scalar multiplier, where
A limitation of the Lucas et al. and CIE methods is that they are based solely on photopigment signals, yet it is unknown how photopigment signals are combined by photoreceptors and processed by the brain. Understanding of how EML and mel-EDI relate to non-visual outcomes in real-world settings is incomplete. The CIE method is the only consensus standard for characterizing the instantaneous biological potency of a light stimulus, but it comes with the caveat that quantities derived using the CIE system may not necessarily represent how light influences non-visual responses in real-world settings.
Circadian Stimulus (CS) is a non-linear model of human nocturnal melatonin suppression that is based on the quantity and spectrum of light and assumes 1-h of exposure time (33–36). CS models a hypothesized relationship between the retina and pineal gland as operating on a spectral opponency between the “blue” and “yellow” channels, from which Circadian Light, CLa, values are determined. CS is calculated by fitting CLa values to a four-parameter logistic function. CS is expressed as a decimal percentage of melatonin suppression. CS ranges from 0.00 (0%) to 0.70 (70%). Though CS is a measure of melatonin suppression, it is intended to be relevant for the regulation of circadian rhythms. A limitation of CS is that melatonin suppression and circadian phase shift are not proxies for each other (37), and CS does not necessarily represent how light influences other non-visual responses.
There are publicly available calculators for computing the above quantities. For a Microsoft Excel calculator to compute EML see IWBI (38). For a Microsoft Excel calculator to compute mel-EDI see CIE (39). CS can be computed using an online calculator (40) or Microsoft Excel (41). Computation of EML, mel-EDI and CS require two inputs, a light source's spectral power distribution (SPD) and photopic illuminance at the plane of the eye. In principal, these quantities can be measured on site—though the complexity and cost of doing so in a non-cursory way must be acknowledged. It should also be understood that instantaneous measurements may not be representative of mean light exposure, which is almost always transitory and varies with things such as view direction, instantaneous daylight exposure, and lamp aging factors such as lumen depreciation and spectral changes with time. There are recommendations for measurement and reporting of light exposure in experiments (42, 43), with some recommendations being transferable to field settings. Spectral lighting software is available to investigate some of these measures via simulation (44, 45).
Responses to Light That Matter
Humans have a wide range of visual and non-visual responses to light. Human outcomes most commonly relevant in applied lighting are visual performance, visual experience, visual comfort, circadian phase-shifting, and alertness. This prioritization is similar to the response headings in Figure 1, but not identical since here we are endeavoring to be more explicit. For example, while alertness, melatonin suppression, and pupil size can all be acute responses, we believe that alertness is more tangible in daily life.
Visual performance refers to the ability of the eye/brain system to gather and process visual information to perform a task. Visual experience refers to the perceptual response to illuminated environments, including evoked perceptions and emotions such as feelings of relaxation, tension, spaciousness, closure, and the like. Visual comfort is a reference to the perceptual response to the qualities and quantities of light in an environment, and whether they result in comfortable or uncomfortable seeing. Since visual discomfort is usually simpler to define than visual comfort, a visually comfortable environment is often defined as one that does not create visual discomfort. Circadian phase-shifting refers to the ability of light to advance or delay the circadian clock, and so sleep timing. Alertness refers to light's potential to moderate a person's state of sensory awareness and active attention. These five categories are not exhaustive and may not receive equal prioritization in a design solution. For example, the primary goal in retail lighting may be to encourage sales (46) and in open surgery visual performance will dominate the design criteria (47).
Visual performance, visual experience, and visual comfort have undergone decades of research and are the basis for lighting industry standards, recommended practices, and design guidelines. Of the various non-visual responses to light, alertness (48, 49), melatonin suppression (50, 51), and circadian phase shifting (51, 52) have been most extensively studied, mostly in laboratory settings. Pupillary response (53, 54), heart rate (55, 56), mood state (57, 58), and body temperature (55, 56) have also been studied, as has student performance in school settings (59–62), workspaces (63–66), and senior living centers (67). In this section we summarize considerations related to circadian entrainment, alertness, and performance of students and office workers since these considerations are especially relevant to how lighting design influences human health.
Circadian Entrainment
Circadian rhythms are biological rhythms with predictable changes in magnitude that repeat on a period of about 24 h. Examples include core body temperature, alertness, and the concentration of melatonin. Circadian entrainment is a stable relationship between a biological rhythm and an external environmental cue. A circadian phase shift is a change in the timing of circadian rhythms, where a phase-advance means that bedtime and wake-up time will move earlier in the day, and a phase-delay means that bedtime and wake-up time will move later in the day. The light/ dark cycle is the most important exogenous cue for entraining circadian rhythms. Reduced contrast between day and night can weaken circadian entrainment (6).
Acute suppression of the hormone melatonin is much easier to measure than changes in circadian rhythms and has been treated as a proxy measure in some of the studies endeavoring to study the effects of light on circadian health (33, 34, 36, 68, 69). Unfortunately, acute melatonin suppression by light may not be a suitable proxy for other physiological responses, such as circadian phase shifting and alertness (37, 70). The human circadian system adapts to prior light exposure (71), light exposure earlier in the day affects the biological potency of light later in the day (72–75), extended periods under dim light may negatively impact subsequent sleep (76), and there is considerable interindividual variability in the response to evening light (77). Collectively, these findings suggest nuance in the human circadian response to light, raising questions about the veracity of numerical design targets for circadian lighting design. For example, the CS targets in UL 24480 (10) are based on research that characterizes acute melatonin suppression after a 1-h exposure to light, yet compliance with the UL standard requires a minimum of a 2-h exposure of CS > 0.30 between 7 a.m. and 4 p.m., and is intended to support a broad range of health outcomes for all day-shift workers.
Daylight naturally provides bright days and dark nights, creating both the cycle and light/dark ratio that is essential for circadian entrainment (78, 79). Daylight offers other psychological and physiological benefits (80–82) and, whenever possible, should play a key role in human-centric lighting design (83).
Alertness
The potential to use light to enhance alertness and improve cognitive performance is of interest to educational institutions and knowledge-work environments. Organizations that operate 24/7 may also aspire to reduce errors and increase safety by using light to enhance nighttime alertness of employees.
The alerting response has largely been characterized in laboratory settings during nighttime. The acute alerting properties of light have been compared to that observed after caffeine consumption (84). In laboratory settings, light has reduced attentional lapses, decreased subjective sleepiness, improved alertness, and enhanced performance on some cognitive tests (11, 15, 85–87). The alerting effects of light may be stronger at night than during the day (87). During daytime hours, improvements in alertness may be minimal for well-rested people (88). Many studies of alertness and cognition have shown mixed results where some measures have improved but others have not (15, 89–92).
Alertness is commonly characterized using an objective assessment of sustained attention (93) or a subjective alertness measure (94). An advantage of these standardized tests is that they are sensitive to sleep deprivation and have a large base of prior literature for comparison. More complex tasks, however, may be resistant to the short-term alerting effects of light. The generalizability of simplistic assessments of attention to real-world outcomes is questionable.
In their review, Souman et al. (15) concluded that increasing the intensity of white light sometimes increases subjective ratings of alertness, that the effect of CCT on subjective alertness is unclear, and that no studies show a systematic relationship between alertness and wavelength. The meta-analysis by Brown (95) suggests a sigmoidal relationship between subjective sleepiness and mel-EDI (18). Others have shown that light without short-wavelength content can maintain subjective alertness without suppressing melatonin (96, 97), and that long-wavelength (red) light can elicit an alerting response during daytime hours without acute melatonin suppression (98). Few studies have tested the potential alerting benefits of architectural lighting relative to operational outcomes in real-world settings. Despite the consensus that light influences alertness, especially in controlled settings, and general guidance in the peer-reviewed literature (99), no consensus body has offered lighting criteria that implies a direct stimulus response relationship between light and alertness.
Student and Office Worker Performance
There is some evidence that lighting influences student performance. Early evidence demonstrated a positive connection between the presence of daylight in classrooms and student performance (100). More recently, classroom lighting with relatively more short-wavelength radiation was shown to improve cognitive processing speed in high school students (59), improve concentration in elementary school children (60), and improve oral reading fluency performance in third-grade students (61). Lighting that varies in color temperature and illuminance was shown to increase attention and reading speed of elementary and high school students (62). Collectively, these studies suggest that architectural lighting, including daylight, has the potential to positively impact student learning.
There is also some evidence that lighting influences knowledge worker performance. Lighting with a very high CCT of 17,000 K was employed within a shift-working call center (63) and in an office setting (64). Mills et al. found mean improvements on fatigue, alertness, daytime sleepiness, and work performance. Viola et al. found mean improvements on alertness, mood, daytime sleepiness, evening fatigue, and work performance. Office workers in windowless environments self-reported poorer well-being and sleep quality in comparison to workers with access to windows (65). View very likely plays a role, but the reported positive effects of daylight and view have not yet been disentangled (101). Figueiro et al. (66) linked daytime light exposure to the sleep quality and mood of office workers. Collectively, these studies suggest that architectural lighting, including daylight (and view), has the potential to influence office worker well-being and performance.
External Validity
External validity is the extent to which results can be applied to other people or contexts that differ from the specific circumstances of the experiment (102). Outside of laboratory settings, responses to light do not occur in isolation. The same light stimulus may simultaneously influence none or many biological responses. A biological response may or may not translate to a change in performance or overall health. Any of the outcomes mentioned above can be influenced by factors other than light, such as age, climate, diet, disease, exercise, genetics, medications, mental health, pregnancy, sleep habits, stress, and travel. Responses evoked by light may also be evoked by other sensory inputs, such as an attention response evoked by auditory (103) or olfactory (104) stimuli. While light is indeed potent, it is important to consider the effects of light in a broader context that includes other sensory and non-sensory inputs.
Of the field studies available, many have used participants with limited or less-common exposure to light, such as elders with limited mobility (67, 105–107), night shift workers (108–111), fatigued cancer survivors (112), hospitalized patients (113), and infants (114). In most of these studies, the benefit of lighting was modest or the outcome was mixed.
The least studied group are healthy adults that work during the day, sleep at night, and who have exposure to daylight. For this group, if electric light exposure is compliant with guidelines for vison (26, 115), it is unclear the degree to which changing the lighting will affect non-visual outcomes. While laboratory studies have demonstrated the capacity and potential of light to influence non-visual outcomes, more field studies are needed to understand the veracity of the effects in real-world settings.
A Five-Step Design Process for Human-Centric Lighting
Though there is still much to learn, enough is known today to at least offer a framework for addressing visual and non-visual outcomes through lighting design decisions. The five-step process outlined below augments the already well-established lighting design processes (116) and can be used to integrate human-centric lighting design concepts into design practice. Table 2 provides an overview of the process with examples for select application types.
Step 1: Characterize the Lighting Application
The first step is to establish the application's primary tasks and activities, when they occur, and the desired outcomes or operational goals. For example, healthcare environments are typified by the intent to prevent, cure, or treat illness. Operational goals will likely include improving patient health and well-being, which often requires visual evaluation, diagnostic testing, and interpersonal communication. Architectural design and human-centric lighting strategies should support these activities.
A thorough understanding of desired outcomes will guide prioritization of design criteria and facilitate rational design decisions when all outcomes cannot be simultaneously achieved. For example, in a healthcare environment, temporary visual discomfort of a patient may be acceptable if it increases the speed and efficacy with which a caregiver can diagnose and administer treatment (e.g., over-bed patient exam lights tends to be very bright when viewed from the perspective of a patient, but are necessary for the caregiver). In an office environment, such visual discomfort would likely affect productivity and would therefore be unacceptable. There is no one-size-fits all approach.
Step 2: Determine the Likely Sleep-Wake Cycle(s) of Occupants
Determine if the application includes day-active people, night-active people, or both. Occupants that are day-active/night-inactive have wake-sleep cycles that are largely synchronous with the day-night cycle. Their lighting needs may conflict with occupants that are night-active/day-inactive and who have wake-sleep cycles that are largely asynchronous with the day-night cycle. Day-active people benefit from light with high biological potency during the morning and daytime, low biological potency in the evening, and as little biological potency as possible at night. Night-active people need nighttime illumination to adequately and safely perform personal or professional tasks while minimizing the potential negative human outcomes associated with an asynchronous sleep-wake cycle and nighttime light exposure.
For example, education settings are likely to have predominately day-active occupants, 24/7 industrial facilities may have day-active or night-active people sequentially throughout the day as the work shift changes from day-shift, to second-shift, to night-shift, and healthcare facilities may include both day-active and night-active occupants simultaneously. Applications with both day-active and night-active people—either sequentially or simultaneously—may require specialized design solutions that include advanced lighting controls (e.g., controlling zones, scenes, intensity, spectrum) and/or architectural interventions (e.g., barriers to block obtrusive light, temporary or permanent partitions to create zonal workspaces) that rethink traditional architectural and spatial relationships.
It is especially challenging to address the needs of workers with rotating shifts, such as nurses that cycle between dayshifts and nightshifts. During periods of rotation, the circadian pacemaker is playing perpetual catch-up to the changing timing of the light exposure rhythms. The optimal lighting solution for an occupant with a rotating shift may differ from the optimal lighting solution for an occupant with a permanent shift, even when both people occupy the same environment at the same time and are performing comparable work tasks.
Step 3: Determine the Sleep Needs of Occupants
Determine if the application includes sleeping occupants. If yes, determine if the sleeping occupants will be day-active, night-active, or both. Darkness promotes sleep and spaces where occupants will sleep should be as dark as possible while providing enough light for safe navigation (27). For day-active people sleep occurs primarily in the evening and throughout the night—this may require window treatments to block intrusive light. For night active people, sleep occurs primarily during daytime hours and window treatments or eye coverings are almost certainly necessary.
For example, healthcare environments are likely to have a combination of occupant needs: day-active (or night-active) patients seeking treatment during the day (outpatient); day-active (or night-active) patients seeking treatment over several days (inpatient), with both active and sleep requirements; and the healthcare workers that provide care, and who may be day-active or night-active, depending on the shift. These groups have separate and distinct needs that change throughout the day and the interplay between conflicting needs by different occupants demands careful examination, including prioritization and a considered understanding of tradeoffs. See Zee and Goldstein (117) for guidance about using light and sleep hygiene practices to improve outcomes for people with non-traditional work schedules.
Step 4: Review Published Human-Centric Lighting Guidance
Human-centric lighting design can be informed by industry guidelines that endeavor to bridge the gap between scientific understanding and guidance for application. Organizations like the Society for Light and Lighting and the Illuminating Engineering Society (IES) provide standards that focus of visual outcomes (26, 115). The WELL Building Standard (WELL) (9) and Underwriters Laboratory (UL) (10) each recommend quantitative design targets for circadian lighting design. The WELL standard has changed threshold values over time; to our knowledge, the rationale for the threshold design targets and changes to the thresholds has never been disclosed. The IES maintains that, to ensure transparency and involvement of relevant constituencies, any recommended practice related to light and health should be a consensus document developed through an accredited ANSI process (118), which was not done with either the WELL or UL standards. DIN SPEC 67600 also provides design guidelines for biologically effective illumination (119). DIN SPEC 67600 was not developed through the full ISO consensus standards process, which is why it bears the “SPEC” modifier in the title (120).
The WELL criteria are based primarily on EML (32), while also allowing compliance with mel-EDI (18) or CS (36). UL's criteria are primarily expressed using CS while also allowing compliance using EML or photopic illuminance. Both WELL and UL have exposure times and durations associated with their recommendations. The UL standard is limited to promoting circadian entrainment for day-active and night-inactive people in commercial, educational, and industrial settings, while encouraging consideration of other legitimate design goals such as glare and color quality. The WELL standard is also intended to promote circadian entrainment, while also including explicit pass/fail criteria related to illuminance, glare, visual comfort, access to daylight, views, color quality, flicker, and personal control. Both systems endeavor to promote a comprehensive approach to human-centric lighting. The criteria related to circadian entrainment for the UL and WELL systems are provided in Table 3. The criteria are based on the temporal pattern of light exposure (time of day and duration of exposure), light level, spectrum, and the location where the light is delivered. While Table 3 is current as of the date of this article, it is prudent to check for updates since recommendations may change.
Brown et al. (27) provide recent and noteworthy light exposure recommendations for healthy adults with regular daytime schedule. They recommend mel-EDI ≥ 250 lx throughout the day, mel-EDI ≤ 10 lx in the 3 h before bedtime, and sleep environments as dark as possible (mel-EDI ≤ 1 lx). All measurements are at the plane of an observer's eye, simplified as a vertical plane at ≈ 1.2 m height. Insofar as possible, these recommendations should be applied daily at the same time of day. These recommendations are not intended to supersede existing guidelines related to visual function and safety; rather, they are additional criteria to be considered by lighting specifiers. The daytime criteria of Brown et al. (27) are higher in quantity and longer in duration than both the WELL and UL criteria.
WELL v2 (9), UL 24480 (10), and Brown et al. (27) provide pass/fail criteria that facilitate ease-of-use in practice but may promote a false sense of precision. Considerations of precision and accuracy are relevant (121) since they can complicate the debate about how to establish metrics and thresholds that serve both vision and autonomic body functions that are influenced by light. It is already appreciated that under many circumstances great precision is not needed when designing lighting systems. The IES Lighting Handbook (26) suggest that, at time of occupancy, illuminance measurements within 30% of the target are sufficiently accurate for most applications. Similar considerations are likely relevant for non-visual effects, since physiological responses are modulated by large relative changes more so than by small fractional changes. The key considerations in Well v2 (9), UL 24480 (10), and Brown et al. (27) focus on defining the magnitude of photic stimulation at the plane of the eye, linked to time of day, for a given length of time. While the recommendations available today are not identical, they are comparable in manner and degree, which we view as progress toward consensus. The fuzziness that exists today is not a problem since estimates need not be overly precise or accurate to be useful in lighting practice. We believe WELL v2 (9), UL 24480 (10), and Brown et al. (27) all move lighting practice in a positive direction by encouraging consideration of non-visual responses to light and by providing quantitative target that can inform the development of design criteria.
Step 5: Put It All Together
Once the application characteristics and operational goals have been defined, the occupants' sleep-wake cycles established, occupant sleep requirements have been determined, and published guidance has been reviewed, design criteria and numerical design targets can be established. Recommendations from WELL, UL, CIBSE, and IES provide guidance, but it is ultimately the duty of the design team, in consultation with the building owner, to balance the relative importance of visual and non-visual needs. The WELL and UL circadian lighting guidelines are specifically for day-active (night-inactive) people; stakeholders will need to use their best judgement when designing lighting systems for people who do not fit this profile or where multiple populations or people with different schedules occupy the same spaces.
Lighting design demands consideration of competing criteria. Prioritization may be needed to balance tradeoffs between visual and non-visual design goals. For example, bright light during the day is expected to better support non-visual outcomes for day-active people. But, if the light is so bright that it creates glare, then productivity may suffer. Higher light levels also require more energy, which may not be compatible with required energy codes (122). As another example, darkness is most desirable at nighttime for circadian health, but light may be needed to support safe navigation. Such difficult tradeoffs are best addressed by competent professionals and thoughtful clients that are willing to prioritize needs and define expected outcomes.
Design teams work on projects on a per-project basis, where a typical project may be a building or a subset of spaces within a building. Yet, people transition through many buildings and spaces through the course of a day, week, month, and year. Coworkers or classmates may have very different spectral diets (123), which will moderate the manner with which comparable workplace or school lighting affects individual outcomes. Individuals have different levels of control over their exposure to light. Some people may be able to take morning and lunchtime walks and limit screen use at night. Others may have limited mobility or inflexible work schedules. Even longitudinal position within a time zone affects cancer risk, likely due to varying degrees of circadian disruption (124). Though the design team cannot control these and other pertinent factors, designers have some control over the light stimulus received by occupants in the spaces they design. By providing light with appropriate qualities (e.g., intensity, spectrum, spatial pattern, controllability) at the right time of day, designers can support good outcomes even against this background of uncertainty.
Conclusions
For day-active people, visual and non-visual needs are generally synchronized. While light from the sun and sky naturally provides the cycle and light/dark ratio that supports circadian health, electric lighting has a critical role since most of us spend most of our time indoors (4, 5). Architecture, glazing, lighting equipment, and lighting control solutions can be used in combination to deliver biologically potent light during the day while minimizing light exposure as night, all while balancing traditional factors such as color quality, flicker, glare, psychological reinforcement, and visibility (26, 115). Perhaps the simplest guidance to support the health of day-active people is to provide light of high biologically potency during the day and low biological potency at night.
For night-active people, visual and non-visual needs are in conflict, requiring explicit prioritization. Many night-active people provide critical societal functions, such as night shift nurses and doctors, with visually demanding jobs that have a low tolerance for errors. For these people, factors like visibility and alertness may be prioritized over circadian entrainment, even while recognizing that circadian disruption from an asynchronous sleep-wake cycle is associated with long-term negative health outcomes (125). Lighting design solutions for night-active people demands complete consideration of tradeoffs.
Good outcomes are most likely when a knowledgeable team that includes designers, owners, and equipment manufacturers prioritize visual and non-visual design outcomes. Such teams are well-positioned to develop lighting solutions that deliver light of an appropriate amount and spectrum at the right time of day, for an appropriate length of time.
Because there is no one-size-fits-all solution, we suggested a framework to guide lighting design: characterize the lighting application, determine the likely sleep-wake cycle(s) of occupants, determine the sleep needs of the occupants, review published guidance to develop goals and design criteria that support visual and non-visual outcomes, then use this information to establish design criteria that will guide decisions in the latter stages of the design process. We hope that implementation of this process facilitates realization of what we all want—lighting solutions that support human outcomes.
Author Contributions
KH and TE contributed to conceptualization, writing, reviewing, and editing of this manuscript, including figures and tables. KH managed project administration. All authors contributed to the article and approved the submitted version.
Funding
The authors received funding from Energy Focus, Inc. for the preparation of this manuscript. Energy Focus, Inc. had no role in the conceptualization, preparation, or approval of this manuscript.
Conflict of Interest
KH is Editor in Chief of LEUKOS, the journal of the Illuminating Engineering Society, founder of Loucetios, LLC, and co-founder of Lyralux, Inc. TE is founder of Lighting Research Solutions, LLC.
References
1. Houser KW, Boyce PR, Zeitzer J, Herf M. Human centric lighting: myth, magic, or metaphor? Lighting Res Technol. (2020) Online before print. doi: 10.1177/1477153520958448
2. CIE. Commission Internationale de l'Eclairage. CIE Position Statement on Non-Visual Effects of Light: Recommending Proper Light and the Proper Time. 2nd ed. Vienna: CIE (2019).
3. ISO/CIE. International Standards Organization and Commission Internationale de l'Eclairage. Light and lighting—Integrative lighting—Non-visual effects. Under development (2020). Report No. ISO/CIE TR 21783.
4. Beyer KMM, Szabo A, Hoormann Stolley M. Time spent outdoors, activity levels, and chronic disease among American adults. J Behav Med. (2018) 41:494–503. doi: 10.1007/s10865-018-9911-1
5. Klepeis NE, Nelson WC, Ott WR, Robinson JP, Tsang AM, Switzer P, et al. The national human activity pattern survey (NHAPS): a resource for assessing exposure to environmental pollutants. J Expo Anal Environ Epidemiol. (2001) 11:231–52. doi: 10.1038/sj.jea.7500165
6. Wright KP, McHill AW, Birks BR, Griffin BR, Rusterholz T, Chinoy ED. Entrainment of the human circadian clock to the natural light-dark cycle. Curr Biol. (2013) 23:1554–8. doi: 10.1016/j.cub.2013.06.039
7. Stothard ER, McHill AW, Depner CM, Birks BR, Moehlman TM, Ritchie HK, et al. Circadian entrainment to the natural light-dark cycle across seasons and the weekend. Curr Biol. (2017) 27:508–13. doi: 10.1016/j.cub.2016.12.041
8. Chen S, Wei M, Dai Q, Huang Y. Estimation of possible suppression of melatonin production caused by exterior lighting in commercial business districts in metropolises. LEUKOS. (2020) 16:137–44. doi: 10.1080/15502724.2018.1523013
9. International Well Building Institute. WELL Building Standard v2, Q3 2020 Version. Section L03: Circadian Lighting Design. (2020). Available online at: https://v2.wellcertified.com/v/en/light/feature/3 (accessed November 2, 2020).
10. Underwriters Laboratory. Design Guidelines for Promoting Circadian Entrainment with Light for Day-Active People. UL Design Guideline 24480, Edition 1. Northbrook, IL: UL (2019).
11. Cajochen C. Alerting effects of light. Sleep Med Rev. (2007) 11:453–64. doi: 10.1016/j.smrv.2007.07.009
12. Golombek D, Rosenstein RE. Physiology of circadian entrainment. Physiol Rev. (2010) 90:1063–102. doi: 10.1152/physrev.00009.2009
13. Khademagha P, Aries MBC, Rosemann ALP, van Loenen EJ. Implementing non-image-forming effects of light in the built environment: a review of what we need. Build Environ. (2016) 108:263–272. doi: 10.1016/j.buildenv.2016.08.035
14. Münch M, Brøndsted AE, Brown SE, Gjedde A, Kantermann T, Martiny K, et al. Chapter 3. The effect of light on humans. In: Sanders S, Oberst J, editors. Changing Perspectives on Daylight: Science, Technology, and Culture. Washington, DC: Science/AAAS. (2017). p. 16–23.
15. Souman JL, Tinga AM, te Pas SF, van Ee R, Vlaskamp BNS. Acute alerting effects of light: a systematic literature review. Behav Brain Res. (2018) 337:228–39. doi: 10.1016/j.bbr.2017.09.016
16. Vetter C, Pattison PM, Houser KW, Herf M, Phillips AJK, Wright KPM, et al. A Review of Human Physiological Responses to Light: Implications for the Development of Integrative Lighting Solutions. LEUKOS. doi: 10.1080/15502724.2021.1872383
17. de Kort YAW, Veitch JA. From blind spot into the spotlight: introduction to the special issue ‘Light, lighting, and human behaviour'. J Environ Psychol. (2014) 39:1–4. doi: 10.1016/j.jenvp.2014.06.005
18. Commission Internationale de l'Eclairage. CIE S 026/E:2018 CIE System for Metrology of Optical Radiation for ipRGC-Influenced Responses to Light. Vienna: CIE (2018).
19. Illuminating Engineering Society. TM-18-18. Light and Human Health: An Overview of the Impact of Optical Radiation on Visual, Circadian, Neuroendocrine and Neurobehavioral Responses. New York, NY: IES (2018).
20. Roenneberg T, Kumar CJ, Merrow M. The human circadian clock entrains to sun time. Curr Biol. (2006) 17:R44–5. doi: 10.1016/j.cub.2006.12.011
21. Houser KW. Editorial: human centric lighting and semantic drift. LEUKOS. (2018) 14:213–4. doi: 10.1080/15502724.2018.1501234
22. Souman JL, Borra T, de Goijer I, Schlangen LJM, Vlaskamp BNS, Lucassen MP. Spectral tuning of white light allows for strong reduction in melatonin suppression without changing illumination level or color temperature. J Biol Rhythms. (2018) 33:420–31. doi: 10.1177/0748730418784041
23. Glickman G, Hanifin JP, Rollag MD, Wang J, Cooper H, Brainard GC. Inferior retinal light exposure is more effective than superior retinal exposure in suppressing melatonin in humans. J Biol Rhythms. (2003) 18:71–9. doi: 10.1177/0748730402239678
24. Visser EK, Beersma DGM, Daan S. Melatonin suppression by light in humans is maximum when the nasal part of the retina is illuminated. J Biol Rhythms. (1999) 14:116–21. doi: 10.1177/074873099129000498
25. Ruger M, Gordijn MCM, Beersma DGM, de Vries B, Daan S. Nasal versus temporal illumination of the human retina: effects on core body temperature, melatonin, and circadian phase. J Biol Rhythms. (2005) 20:60–70. doi: 10.1177/0748730404270539
26. DiLaura DL, Houser KW, Mistrick RG, Steffy GR editors. The Lighting Handbook, Reference and Application. 10th ed. New York, NY: Illuminating Engineering Society (2011).
27. Brown T, Brainard G, Cajochen C, Czeisler C, Hanifin J, Lockley S, et al. Recommendations for healthy daytime, evening, and night-time indoor light exposure. Preprints. (2020) doi: 10.20944/preprints202012.0037.v1
28. Berson DM, Dunn FA, Takao M. Phototransduction by retinal ganglion cells that set the circadian clock. Science. (2002) 295:1070–3. doi: 10.1126/science.1067262
29. Hattar S, Liao HW, Takao M, Derson DM, Yau KW. Melanopsin-containing retinal ganglion cells: architecture, projections, and intrinsic photosensitivity. Science. (2002) 296:1065–70. doi: 10.1126/science.1069609
30. Dacey DM, Liao HW, Peterson BB, Robinson FR, Smith VC, Pokorny J, et al. Melanopsin-expressing ganglion cells in primate retina signal colour and irradiance and project to the LGN. Nature. (2005) 433:749–54. doi: 10.1038/nature03387
31. Mure LS, Vinberg F, Hanneken A, Panda S. Functional diversity of human intrinsically photosensitive retinal ganglion cells. Science. (2019) 366:1251–5. doi: 10.1126/science.aaz0898
32. Lucas RJ, Peirson SN, Berson DM, Brown TM, Cooper HM, Czeisler CA, et al. Measuring and using light in the melanopsin age. Trends Neurosci. (2014) 37:1–9. doi: 10.1016/j.tins.2013.10.004
33. Rea MS, Figueiro MG, Bullough JD, Bierman A. A model of phototransduction by the human circadian system. Brain Res Rev. (2005) 50:213–28. doi: 10.1016/j.brainresrev.2005.07.002
34. Rea MS, Figueiro MG, Bierman A, Bullough JD. Circadian light. J Circadian Rhythms. (2010) 8:10. doi: 10.1186/1740-3391-8-2
35. Rea MS, Figueiro MG, Bierman A, Hamner R. Modelling the spectral sensitivity of the human circadian system. Lighting Res Technol. (2012) 44:386–96. doi: 10.1177/1477153511430474
36. Rea MS, Figueiro MG. Light as a circadian stimulus for architectural lighting. Lighting Res Technol. (2018) 50:497–510. doi: 10.1177/1477153516682368
37. Rahman SA, St Hilaire MA, Gronfier C, Chang AM, Santhi N, Czeisler CA, et al. Functional decoupling of melatonin suppression and circadian phase resetting in humans. J Physiol. (2018) 596:2147–57. doi: 10.1113/JP275501
38. International Well Building Institute. Melanopic Ratio (Microsoft Excel). (2020). Available online at: https://standard.wellcertified.com/sites/default/files/Melanopic Ratio.xlsx (accessed November 2, 2020).
39. Commission Internationale de l'Eclairage. Alpha Opic Toolbox (Microsoft Excel). (2020). Available online at: http://files.cie.co.at/CIE%20S%20026%20alpha-opic%20Toolbox.xlsx (accessed November 2, 2020)
40. Lighting Research Center. CS Calculator (Web Based). (2020). Available online at: https://www.lrc.rpi.edu/cscalculator/ (accessed November 2, 2020).
41. Lighting Research Center. CS Calculator (Microsoft Excel). (2020). Available online at: http://www.lrc.rpi.edu/resources/CSCalculator_2017_10_03_Mac.xlsm (accessed November 2, 2020)
42. Knoop M, Broszio K, Diakite A, Liedtke C, Niedling M, Rothert I, et al. Methods to describe and measure lighting conditions in experiments on non-image-forming aspects. LEUKOS. (2019) 15:163–79. doi: 10.1080/15502724.2018.1518716
43. Spitschan M, Stefani O, Blattner P, Gronfier C, Lockley SW, Lucas RJ. How to report light exposure in human chronobiology and sleep research experiments. Clocks Sleep. (2019) 1:280–9. doi: 10.3390/clockssleep1030024
44. University of Washington and ZGF Architects LLP. Lark Spectral Lighting Software. (2020). Available online at: https://faculty.washington.edu/inanici/Lark/Lark_home_page.html (accessed November 16, 2020).
45. Solemma Inc. Alfa Software. (2020). Avaliable online at: https://www.solemma.com/Alfa.html (accessed November 16, 2020).
46. Cuttle C, Brandston H. Evaluation of retail lighting. J Illum Eng Soc. (1995) 24:33–49. doi: 10.1080/00994480.1995.10748117
47. Mundinger JJ, Houser KW. Adjustable correlated colour temperature for surgical lighting. Lighting Res Technol. (2017) 51:280–90. doi: 10.1177/1477153517742682
48. Cajochen C, Zeitzer JM, Czeisler CA, Dijk DJ. Dose-response relationship for light intensity and ocular and electroencephalographic correlates of human alertness. Behav Brain Res. (2000) 115:75–83. doi: 10.1016/S0166-4328(00)00236-9
49. Lockley SW, Evans EE, Scheer FAJL, Brainard GC, Czeisler CA, Aeschbach D. Short-wavelength sensitivity for the direct effects of light on alertness, vigilance, and the waking electroencephalogram in humans. Sleep Physiol. (2006) 29:161–8. doi: 10.1093/sleep/29.2.161
50. Lewy AJ, Wehr TA, Goodwin FK, Newsome DA, Markey SP. Light suppresses melatonin secretion in humans. Science. (1980) 210:1267–9. doi: 10.1126/science.7434030
51. Zeitzer JM, Dijk DJ, Kronauer RE, Brown EN, Czeisler CA. Sensitivity of the human circadian pacemaker to nocturnal light: melatonin phase resetting and suppression. J Physiol. (2000) 526:695–702. doi: 10.1111/j.1469-7793.2000.00695.x
52. Czeisler CA, Kronauer RE, Allan JS, Duffy JF, Jewett ME, Brown EN, et al. Bright light induction of strong (type 0) resetting of the human circadian pacemaker. Science. (1989) 244:1328–33. doi: 10.1126/science.2734611
53. Gooley JJ, Ho Mien I, St Hilaire MA, Yeo SC, Chua EC, van Reen E, et al. Melanopsin and rod-cone photoreceptors play different roles in mediating pupillary light responses during exposure to continuous light in humans. J Neurosci. (2012) 32:14242–53. doi: 10.1523/JNEUROSCI.1321-12.2012
54. Spitschan M, Jain S, Brainard DH, Aguirre GK. Opponent melanopsin and S-cone signals in the human pupillary light response. PNAS. (2014) 111:15568–72. doi: 10.1073/pnas.1400942111
55. Cajochen C, Munch M, Kobialka S, Krauchi K, Steiner R, Oelhafen P, et al. High sensitivity of human melatonin, alertness, thermoregulation, and heart rate to short wavelength light. J Clin Endocrinol Metab. (2005) 90:1311–6. doi: 10.1210/jc.2004-0957
56. Prayag AS, Jost S, Avouac P, Dumortier D, Gronfier C. Dynamics of non-visual responses in humans: as fast as lightning? Front Neurosci. (2019) 13:16. doi: 10.3389/fnins.2019.00126
57. Golden RN, Gaynes BN, Ekstrom RD, Hamer RM, Jacobsen FM, Suppes T, et al. The efficacy of light therapy in the treatment of mood disorders: a review and meta-analysis of the evidence. Am J Psychiatry. (2005) 162:656–63. doi: 10.1176/appi.ajp.162.4.656
58. Bedrosian TA, Nelson RJ. Influence of the modern light environment on mood. Mol Psychiatry. (2013) 18:751–7. doi: 10.1038/mp.2013.70
59. Keis O, Helbig H, Streb J, Hille K. Influence of blue-enriched classroom lighting on students' cognitive performance. Trends Neurosci Educ. (2014) 3:86–92. doi: 10.1016/j.tine.2014.09.001
60. Sleegers PJC, Moolenaar NM, Galetzka M, Pruyn A, Sarroukh BE, van der Zande B. Lighting affects students' concentration positively: findings from three Dutch studies. Lighting Res Technol. (2013) 45:169–75. doi: 10.1177/1477153512446099
61. Mott MS, Robinson DH, Walden A, Burnette J, Rutherford AS. Illuminating the effects of dynamic lighting on student learning. Sage Open. (2012) 2:1–9. doi: 10.1177/2F2158244012445585
62. Barkmann C, Wessolowski N, Schulte-Markwort M. Applicability and efficacy of variable light in schools. Physiol Behav. (2012) 105:621–7. doi: 10.1016/j.physbeh.2011.09.020
63. Mills PR, Tomkins S, Schlangen LJM. The effect of high correlated colour temperature office lighting on employee wellbeing and work performance. J Circadian Rhythms. (2007) 5:9. doi: 10.1186/1740-3391-5-2
64. Viola AU, James LM, Schlangen LJM, Dijk DJ. Blue-enriched white light in the workplace improves self-reported alertness, performance and sleep quality. Scand J Work Environ Health. (2008) 34:297–306. doi: 10.5271/sjweh.1268
65. Boubekri M, Cheung IN, Reid KJ, Wang CH, Zee PC. Impact of windows and daylight exposure on overall health and sleep quality of office workers: a case-control pilot study. J Clin Sleep Med. (2014) 10:603–11. doi: 10.5664/jcsm.3780
66. Figueiro MG, Steverson B, Heerwagen J, Kampschroer K, Hunter CM, Gonzales K, et al. The impact of daytime light exposures on sleep and mood in office workers. Sleep Health. (2017) 3:204–15. doi: 10.1016/j.sleh.2017.03.005
67. Royer M, Ballentine NH, Eslinger PJ, Houser K, Mistrick R, Behr R, et al. Light therapy for seniors in long term care. JAMDA. (2012) 13:100–2. doi: 10.1016/j.jamda.2011.05.006
68. Brainard GC, Hanifin JP, Greeson JM, Byrne B, Glickman G, Gerner E, et al. Action spectrum for melatonin regulation in humans: evidence for a novel circadian photoreceptor. J Neurosci. (2001) 21:6405–12. doi: 10.1523/JNEUROSCI.21-16-06405.2001
69. Najjar RP, Chiquet C, Teikari P, Cornut PL, Claustrat B, Denis P, et al. Aging of non-visual spectral sensitivity to light in humans: compensatory mechanisms? PLoS ONE. (2014) 9:e85837. doi: 10.1371/journal.pone.0085837
70. Zeitzer JM. When is a proxy not a proxy? The foibles of studying non-image forming light. J Physiol. (2018) 596:2029–30. doi: 10.1113/JP276076
71. Chang A-M, Scheer FAJL, Czeisler CA. The human circadian system adapts to prior photic history. J Physiol. (2011) 589:1095–102. doi: 10.1113/jphysiol.2010.201194
72. Hébert M, Martin SK, Lee C, Eastman CI. The effects of prior light history on the suppression of melatonin by light in humans. J Pineal Res. (2002) 33:198–203. doi: 10.1034/j.1600-079X.2002.01885.x
73. Zeitzer JM, Friedman L, Yesavage JA. Effectiveness of evening phototherapy for insomnia is reduced by bright daytime light exposure. Sleep Med. (2011) 12:805–7. doi: 10.1016/j.sleep.2011.02.005
74. Kozaki T, Kubokawa A, Taketomi R, Hatae K. Effects of day-time exposure to different light intensities on light-induced melatonin suppression at night. J Physiol Anthropol. (2015) 34:5. doi: 10.1186/s40101-015-0067-1
75. te Kulve M, Schlangen LJM, van Marken Lichtenbelt WD. Early evening light mitigates sleep compromising physiological and alerting responses to subsequent late evening light. Sci Rep. (2019) 9:16064. doi: 10.1038/s41598-019-52352-w
76. Cajochen C, Reichert C, Maire M, Schlangen LJM, Schmidt C, Viola AU, et al. Evidence that homeostatic sleep regulation depends on ambient lighting conditions during wakefulness. Clocks Sleep. (2019) 1:517–31. doi: 10.3390/clockssleep1040040
77. Phillips AJK, Vidafar P, Burns AC, McGlashan EM, Anderson C, Rajaratnam SMW, et al. High sensitivity and interindividual variability in the response of the human circadian system to evening light. PNAS. (2019) 116:12019–24. doi: 10.1073/pnas.1901824116
78. Roenneberg T, Foster RG. Twilight times: light and the circadian system. Photochem Photobiol. (1997) 66:549–61. doi: 10.1111/j.1751-1097.1997.tb03188.x
79. Roenneberg T, Daan S, Merrow M. The art of entrainment. J Biol Rhythms. (2003) 18:183–94. doi: 10.1177/0748730403018003001
80. Boyce PR, Hunter C, Howlett O. The Benefits of Daylight Through Windows. Troy, NY: Rensselaer Polytechnic Institute (2003).
81. Solt J, Aarts MPJ, Andersen M, Appelt S, Bodart M, Kaempf J, et al. Chapter 4. Daylight in the built environment. In: Sanders S, Oberst J, editors. Changing Perspectives on Daylight: Science, Technology, and Culture. Washington, DC: Science/AAAS. (2017). p. 24–32.
82. Knoop M, Stefani O, Bueno B, Matusiak B, Hobday R, Wirz-Justice A, et al. Daylight: what makes the difference? Lighting Res Technol. (2020) 52:423–42. doi: 10.1177/1477153519869758
83. Aarts MPJ, Brown SA, Bueno B, Gjedde A, Mersch D, Münch M, et al. Chapter 5. Reinventing daylight. In: Sanders S, Oberst J, editors. Changing Perspectives on Daylight: Science, Technology, and Culture. Washington, DC: Science/AAAS (2017). p. 33–7.
84. Wright KP, Badia P, Myers BL, Plenzler SC, Hakel M. Caffeine and light effects on nighttime melatonin and temperature levels in sleep-deprived humans. Brain Res. (1997) 747:78–84. doi: 10.1016/S0006-8993(96)01268-1
85. Badia P, Myers B, Boecker M, Culpepper J, Harsh JR. Bright light effects on body temperature, alertness, EEG and behavior. Physiol Behav. (1991) 50:583–8. doi: 10.1016/0031-9384(91)90549-4
86. Cajochen C, Frey S, Anders D, Spati J, Bues M, Pross A, et al. Evening exposure to a light-emitting diodes (led)-backlit computer screen affects circadian physiology and cognitive performance. J Appl Physiol. (2011) 110:1432–8. doi: 10.1152/japplphysiol.00165.2011
87. Rahman SA, Flynn-Evans EE, Aeschbach D, Brainard GC, Czeisler CA, Lockley SW. Diurnal spectral sensitivity of the acute alerting effects of light. Sleep. (2014) 37:271–81. doi: 10.5665/sleep.3396
88. Lok R, Woelders T, Gordijn MCM, Hut RA, Beersma DGM. White light during daytime does not improve alertness in well-rested individuals. J Biol Rhythms. (2018) 33:637–48. doi: 10.1177/0748730418796036
89. Rahman SA St., Hilaire MA, Lockley SW. The effects of spectral tuning of evening ambient light on melatonin suppression, alertness and sleep. Physiol Behav. (2017) 177:221–9. doi: 10.1016/j.physbeh.2017.05.002
90. Segal AY, Sletten TL, Flynn-Evans EE, Lockley SW, Rajaratnam SMW. Daytime exposure to short- and medium-wavelength light did not improve alertness and neurobehavioral performance. J Biol Rhythms. (2016) 31:470–82. doi: 10.1177/0748730416659953
91. Sletten TL, Ftouni S, Nicholas CL, Magee M, Grunstein RR, Ferguson S, et al. Randomised controlled trial of the efficacy of a blue-enriched light intervention to improve alertness and performance in night shift workers. Occup Environ Med. (2017) 74:792–801. doi: 10.1136/oemed-2016-103818
92. Rodriguez-Morilla B, Madrid JA, Molina E, Perez-Navarro Correa A. Blue-enriched light enhances alertness but impairs accurate performance in evening chronotypes driving in the morning. Front Psychol. (2018) 9:688. doi: 10.3389/fpsyg.2018.00688
93. Dorrian J, Rogers NL, Dinges DF. Psychomotor vigilance performance: neurocognitive assay sensitive to sleep loss. In: Kushida CA, editor. Sleep Deprivation: Clinical Issues Pharmacology, and Sleep Loss Effects. Boca Raton, FL: CRC Press (2004). p. 67–98.
94. Akerstedt T, Gillberg M. Subjective and objective sleepiness in the active individual. Int J Neurosci. (1990) 52:29–37. doi: 10.3109/00207459008994241
95. Brown T. Melanopic illuminance defines the magnitude of human circadian light responses under a wide range of conditions. J Pineal Res. (2020) 69:e12655. doi: 10.1111/jpi.12655
96. Papamichael C, Skene DJ, Revell VL. Human nonvisual responses to simultaneous presentation of blue and red monochromatic light. J Biol Rhythms. (2012) 27:70–8. doi: 10.1177/0748730411431447
97. van de Werken M, Gimenez MC, de Vries B, Beersma DG, Gordijn MC. Short-wavelength attenuated polychromatic white light during work at night: limited melatonin suppression without substantial decline of alertness. Chronobiol Int. (2013) 30:843–54. doi: 10.3109/07420528.2013.773440
98. Sahin L, Figueiro MG. Alerting effects of short-wavelength (blue) and long-wavelength (red) lights in the afternoon. Physiol Behav. (2013) 116–117:1–7. doi: 10.1016/j.physbeh.2013.03.014
99. Figueiro MG, Nagare R, Price LLA. Non-visual effects of light: how to use light to promote circadian entrainment and elicit alertness. Lighting Res Technol. (2018) 50:38–62. doi: 10.1177/1477153517721598
100. Heschong L, Wright RL, Okura S. Daylighting impacts on human performance in school. J Illum Eng Soc. (2002) 31:101–14. doi: 10.1080/00994480.2002.10748396
101. Boubekri M, Lee J, MacNaughton P, Woo M, Schuyler L, Tinianov B, et al. The impact of optimized daylight and views on the sleep duration and cognitive performance of office workers. Int J Environ Res Public Health. (2020) 17:3219. doi: 10.3390/ijerph17093219
102. Veitch JA, Fotios SA, Houser KW. Judging the scientific quality of applied lighting research. LEUKOS. (2019) 15:97–114. doi: 10.1080/15502724.2018.1550365
103. Snyder JS, Gregg MK, Weintraub DM, Alain C. Attention, awareness, and the perception of auditory scenes. Front Psychol. (2012) 3:17. doi: 10.3389/fpsyg.2012.00015
104. Keller A. Attention and olfactory consciousness. Front Psychol. (2011) 2:13. doi: 10.3389/fpsyg.2011.00380
105. Figueiro MG, Plitnick BA, Lok A, Jones GE, Higgins P, Hornick TR, et al. Tailored lighting intervention improves measures of sleep, depression, and agitation in persons with Alzheimer's disease and related dementia living in long-term care facilities. Clin Interv Aging. (2014) 9:1527–37. doi: 10.2147/CIA.S68557
106. Hopkins S, Morgan PL, Schlangen LJM, Williams P, Skene DJ, Middleton B. Blue-enriched lighting for older people living in care homes: effect on activity, actigraphic sleep, mood and alertness. Curr Alzheimer Res. (2017) 14:1053–62. doi: 10.2174/1567205014666170608091119
107. Giggins OM, Doyle J, Hogan K, George M. The impact of cycled lighting intervention on nursing home residents: a pilot study. Gerontol Geriatr Med. (2019) 5:1–6. doi: 10.1177/2333721419897453
108. Lunn RM, Blask DE, Coogan AN, Figueiro MG, Gorman MR, Hall JE, et al. Health consequences of electric lighting practices in the modern world: a report on the National Toxicology Program's workshop on shift work at night, artificial light at night, and circadian disruption. Sci Total Environ. (2017) 607–608:1073–84. doi: 10.1016/j.scitotenv.2017.07.056
109. Hunter CM, Figueiro MG. Measuring light and night and melatonin levels in shift workers: a review of the literature. Biol Res Nurs. (2017) 19:365–74. doi: 10.1177/1099800417714069
110. Motamedzadeh M, Golmohammadi R, Kazemi R, Heidarimoghadam R. The effect of blue-enriched white light on cognitive performances and sleepiness of night-shift workers: a field study. Physiol Behav. (2017) 177:208–14. doi: 10.1016/j.physbeh.2017.05.008
111. Griepentrog JE, Labiner HE, Gunn SR, Rosengart MR. Bright environmental light improves the sleepiness of nightshift ICU nurses. Crit Care. (2018) 22:1–9. doi: 10.1186/s13054-018-2233-4
112. Wu LM, Amidi A, Valdimarsodittir H, Ancoli-Israel S, Liu L, Winkey G, et al. The effect of systematic light exposure on sleep in a mixed group of fatigued cancer survivors. J Clin Sleep Med. (2018) 14:31–9. doi: 10.5664/jcsm.6874
113. Giménez MC, Geerdinck LM, Versteylen M, Leffers P, Meekes GJBM, Herremans H, et al. Patient room lighting influences on sleep, appraisal and mood in hospitalized people. J Sleep Res. (2017) 26:236–46. doi: 10.1111/jsr.12470
114. Tsai SY, Thomas KA, Lentz MJ, Barnard KE. Light is beneficial for infant circadian entrainment: an actigraphic study. J Adv Nurs. (2011) 68:1738–47. doi: 10.1111/j.1365-2648.2011.05857.x
115. Chartered Institution of Building Service Engineers. SLL Lighting Handbook. London: CIBSE (2018). p. 519.
116. Illuminating Engineering Society. PS-12-19. IES Position on UL RP 24480 Regarding Light and Circadian Entrainment. (2020). Available online at: https://www.ies.org/about-outreach/position-statements/ (accessed November 2, 2020).
117. Zee PC, Goldstein CA. Treatment of shift work disorder and jet lag. Curr Treat Options Neurol. (2010) 12:396–411. doi: 10.1007/s11940-010-0090-9
118. American National Standards Institute/Illuminating Engineering Society. LP-7-20 Lighting Practice: The Lighting Design and Construction Process. New York, NY: IES (2020). p. 46.
119. Deutsches Institut für Normung. DIN SPEC 67600:2013-04 Biologically Effective Illumination – Design Guidelines. Berlin: DIN (2013).
120. Deutsches Institut für Normung. Standards Deliverables. (2020). Available online at: https://www.din.de/en/about-standards/din-standards/deliverables (accessed December 21, 2020).
121. Houser KW. Editorial: estimates, precision, accuracy, and adaptation in applied lighting. LEUKOS. (2020) 17:1–2. doi: 10.1080/15502724.2020.1834283
122. Safranek S, Collier JM, Wilkerson A, Davis RG. Energy impact of human health and wellness lighting recommendations for office and classroom applications. Energy Build. (2020) 226:110365. doi: 10.1016/j.enbuild.2020.110365
123. Webler FS, Spitschan M, Foster RG, Andersen M, Peirson SN. What is the ‘spectral diet' of humans? Curr Opin Behav Sci. (2019) 30:80–6. doi: 10.1016/j.cobeha.2019.06.006
124. Gu F, Xu S, Devesa SS, Zhang F, Kierman EB, Graubard BI, et al. Longitudinal position in a time zone and cancer risk in the United States. Cancer Epidemiol Biomark Prev. (2017) 26:1306–11. doi: 10.1158/1055-9965.EPI-16-1029
Keywords: human-centric lighting, lighting quality, non-visual action of light, circadian light effects, alertness
Citation: Houser KW and Esposito T (2021) Human-Centric Lighting: Foundational Considerations and a Five-Step Design Process. Front. Neurol. 12:630553. doi: 10.3389/fneur.2021.630553
Received: 17 November 2020; Accepted: 06 January 2021;
Published: 27 January 2021.
Edited by:
Manuel Spitschan, University of Oxford, United KingdomReviewed by:
Oliver Stefani, University Psychiatric Clinic Basel, SwitzerlandKatharina Wulff, Umeå University, Sweden
Ruth Kelly Waskett, Hoare Lea, United Kingdom
Copyright © 2021 Houser and Esposito. This is an open-access article distributed under the terms of the Creative Commons Attribution License (CC BY). The use, distribution or reproduction in other forums is permitted, provided the original author(s) and the copyright owner(s) are credited and that the original publication in this journal is cited, in accordance with accepted academic practice. No use, distribution or reproduction is permitted which does not comply with these terms.
*Correspondence: Kevin W. Houser, a2V2aW4uaG91c2VyQG9yZWdvbnN0YXRlLmVkdQ==