- 1Department of Rehabilitation Medicine, First Hospital of Shanxi Medical University, Taiyuan, China
- 2First Clinical Medical College, Shanxi Medical University, Taiyuan, China
Objective: Balance dysfunction after stroke often results in individuals unable to maintain normal posture, limits the recovery of gait and functional independence. We explore the short-term effects of transcranial direct current stimulation (tDCS) on improving balance function and gait in stroke patients.
Methods: We systematically searched on PubMed, Web of Science, EMBASE, Cochrane Central Register of Controlled Trials, and Google Scholar for studies that explored the effects of tDCS on balance after stroke until August 2020. All involved studies used at least one measurement of balance, gait, or postural control as the outcome.
Results: A total of 145 studies were found, of which 10 (n = 246) met the inclusion criteria and included in our studies. The present meta-analysis showed that active tDCS have beneficial effects on timed up and go test (TUGT) [mean difference (MD): 0.35; 95% confidence interval (CI): 0.11 to 0.58] and Functional Ambulation Category (FAC) (MD: −2.54; 95% CI: −3.93 to −1.15) in stroke patients. However, the results were not significant on the berg balance scale (BBS) (MD: −0.20; 95% CI: −1.44 to 1.04), lower extremity subscale of Fugl-Meyer Assessment (FMA-LE) (MD: −0.43; 95% CI: −1.70 to 0.84), 10-m walk test (10 MWT) (MD: −0.93; 95% CI: −2.68 to 0.82) and 6-min walking test (6 MWT) (MD: −2.55; 95% CI: −18.34 to 13.23).
Conclusions: In conclusion, we revealed that tDCS might be an effective option for restoring walking independence and functional ambulation for stroke patients in our systematic review and meta-analysis.
Systematic Review Registration: CRD42020207565.
Introduction
Stroke is a severe central nervous system disease caused by cerebral ischemia or hemorrhage. Stroke often causes multiple health problems, such as decreased muscle strength, impaired proprioceptive capabilities, and impaired cognitive function (1, 2). These sensorimotor deficits will further affect the balance and postural control of stroke patients, which is the key to keeping the body upright and stable in different environments and conditions (3). Nearly 80% of stroke patients cannot successfully maintain balance and execute postural control (4). Another study has shown that 38% of stroke patients are still non-ambulatory at 6 months after onset (5). These patients show weight-bearing asymmetry toward the non-paretic leg, increased postural swing, abnormal joint movement, and cannot adjust their posture (6, 7). Besides, chronic stage stroke can also limit walking ability recovery and increase the risk of falling (8). Therefore, the recovery of balance and postural control is essential for patients to improve their activities of daily living, quality of life and prevent fall events after stroke (9).
Standard rehabilitation protocols focus on improving the patient's walking ability and using compensation strategies like a wheelchair or crutches to maintain balance. In the past several years, therapies based on motor learning and modulating neuroplasticity have greatly been developed. Treatments that used biofeedback training and repetitive task-specific training seem to be effective for patient activities (10). Still, both training types do not demonstrate superior results on clinical tests of balance capacity because the improvement of neural plasticity needs enough training intensity (11, 12). Such limitations in treatment have prompted researchers to find new options. Transcranial direct current stimulation (tDCS) is a promising, innovative, non-invasive method of neurostimulation in which a weak direct current (about 1–2 mA) is applied on the scalp with electrodes. The effect of tDCS is polarity specific, in which anodal stimulation causes an increase in the excitability of the motor cortex, and a cathode causes it to decrease (13).
The safety and effectiveness of tDCS technology have been proven in treating cognitive impairment, depression, and pain (14). It has also been confirmed that tDCS can improve motor learning and precision in healthy people (15) and improve upper limb function and fine motor control in stroke patients (16, 17). Similarly, Madhavan et al. (18) demonstrated that stroke patients' ankle movement had been significantly modulated after excitatory stimulation of the ipsilesional motor cortex. Sohn et al. (19) reported that excitatory tDCS could enhance postural stability in patients with sub-acute stroke, but a sham stimulation cannot. Although many current studies have shown positive results on the effect of tDCS. However, some studies showed the opposite results (20). These inconsistencies might be related to the lower sample size, the difference in evaluation methods, and the patient's pre-intervention status. In this study, we aimed to make a systematic review and meta-analysis to evaluate the effect of tDCS on balance following stroke patients.
Methods
Search Strategy
We conducted this meta-analysis to explore the effect of tDCS on balance in stroke patients following the Preferred Reporting Items for Systematic reviews and Meta-Analysis (PRISMA) guideline (21). Online databases (PubMed, Web of Science, EMBASE, Cochrane Central Register of Controlled Trials, and Google Scholar) were searched for articles until August 2020. We performed the literature search for studies that used tDCS to improve balance, gait, and postural control in stroke patients. The key search terms were: [“transcranial direct current stimulation” (MeSH term)] AND [“stroke” (MeSH term)] AND [“gait” (MeSH term) OR “Postural Balance” (MeSH term)]. The keyword “OR” was used for the combination of the MeSH term and Entry terms.
Inclusion Criteria and Exclusion Criteria
Our study followed these inclusion criteria: (1) randomized controlled trials (RCTs), crossover RCTs, and high-quality comparative studies focused on the tDCS effects in stroke patients' balance; (2) included at least one measurement of balance, gait, or postural control as the outcome; and (3) with sham stimulation as control.
The articles were excluded if they (1) were meta-analyses, reviews, study protocols, meeting abstracts, or case studies; (2) were animal experiments; and (3) had no sufficient data to calculate the effect size.
Data Collection
We extracted data from the studies as follows: authors and publication year, the type of study design, numbers of objects, the site of stimulation, sessions, characteristics of interventions and controls, and measured outcomes. If the outcome data were reported at multiple time points, we used those obtained immediately after the intervention.
The following outcome measures were chosen for our meta-analysis: (1) lower extremity subscale of Fugl-Meyer Assessment (FMA-LE) that tests the recovery of lower extremity motor impairment, (2) Berg Balance Scale (BBS) that examines the balance function, (3) Functional Ambulatory Category (FAC) that tests walking independence and functional ambulation, (4) timed up and go test (TUGT) that tests functional mobility, (5) 10-m walk test (10 MWT) that tests the effect of tDCS on walking speed, and (6) 6-min walking test (6 MWT) that tests the walking endurance.
Quality Assessment
We assessed the methodological quality of the included articles that follows the Cochrane Risk of Bias Tool (22). Two authors independently rated studies based on six domains: (1) sequence generation, (2) allocation concealment, (3) blinding of the participants, (4) blinding of the assessors, (5) method of addressing incomplete outcome data, and (6) selective reporting. The methodological quality assessment results have three levels in each domain: low risk, high risk, and unclear. We resolved any discrepancies during interpretation by discussion and mutual agreement.
Meta-Analysis
This meta-analysis used means and standard deviations of the difference between pre-intervention and post-intervention. When the means and standard deviations had not been directly provided, we used t-values, F-values, or p-values to calculate effect sizes. We conducted the pooled effect size calculation by using mean differences (MD) according to the outcome measures of studies. Heterogeneity between studies was assessed based on I2 statistics. We used a random-effects model when the threshold for heterogeneity was above 50%. Then, a sensitivity analysis was performed to control the heterogeneity by excluding one study at a time. When the heterogeneity threshold is below 50%, we used a fixed-effects model for the calculation. All statistical analyses were conducted using Review Manager 5.4.
Results
Search Results
The process of inclusion and exclusion is summarized in Figure 1. Finally, 10 articles were included for our systematic reviews and meta-analysis. Table 1 shows the characteristics of the included studies and their primary outcome measures. Seven RCTs, (23–25, 27–29, 32) two crossovers, (26, 31) and one active-control article, (30) with a total of 246 stroke patients, were included in this study. Among all the included literature, there were five articles that reported on TUGT, (23, 26–28, 31) five articles reported on FAC, (24, 28–30, 32) four articles reported on 10 MWT, (24, 26, 28, 32) four articles reported on 6MWT, (24, 25, 27, 32) four articles reported on BBS, (24, 27–29) four articles reported FMA-LE, (24, 26, 27, 29) three articles reported CME, (24, 27, 29) two articles reported on walking speed, (27, 30) two articles reported on POMA, (23, 26) and one article reported on MVC and FTSST (31). For those outcome measures reported in more than three articles, we calculated the pooled effect size.
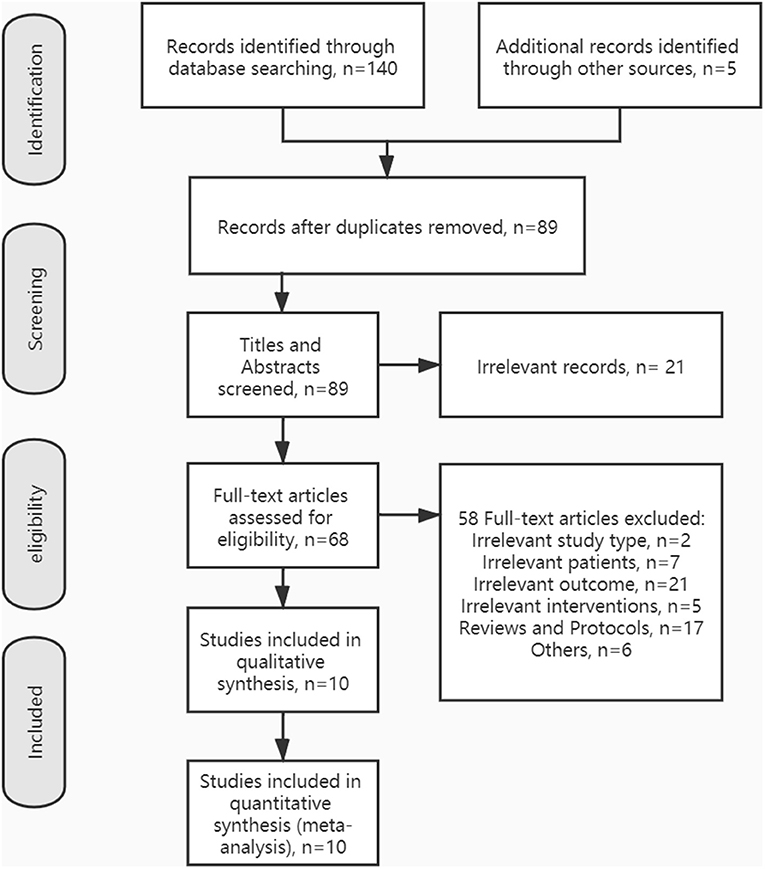
Figure 1. Preferred reporting items for systematic reviews and meta-analysis (PRISMA) flow diagram showing the literature search, inclusion, and exclusion process.
Quality Assessments
Figure 2 shows the methodological qualities of the trials with Cochrane recommendation. Among all the articles, one study had participants sequentially assigned to one of two groups, (30) and four studies clearly described the method of random allocation [two according to software-generated randomization scheme, (25, 32) one used the minimization method, (27) one used random table (24)], and the remaining studies only mentioned randomization without describing the specific implementation plan. Three studies described certain schemes of allocation concealment (24, 25, 27). Two studies had not sufficiently reported the blinding of participants and the blinding of outcome assessment (26, 27). One study had missing outcome data (27). None of the studies showed selective reporting results and other biases. Overall, the included studies were at a relatively low risk of bias.
Meta-Analysis Results
Lower Extremity Subscale of Fugl-Meyer Assessment
The meta-analysis of four trials and 115 participants showed no significant difference on FMA-LE in active tDCS compared with sham tDCS (MD: −0.43; 95% CI: −1.70 to 0.84; p = 0.51; I2 = 16%; inverse variance method with fixed-effects model) (Figure 3A).
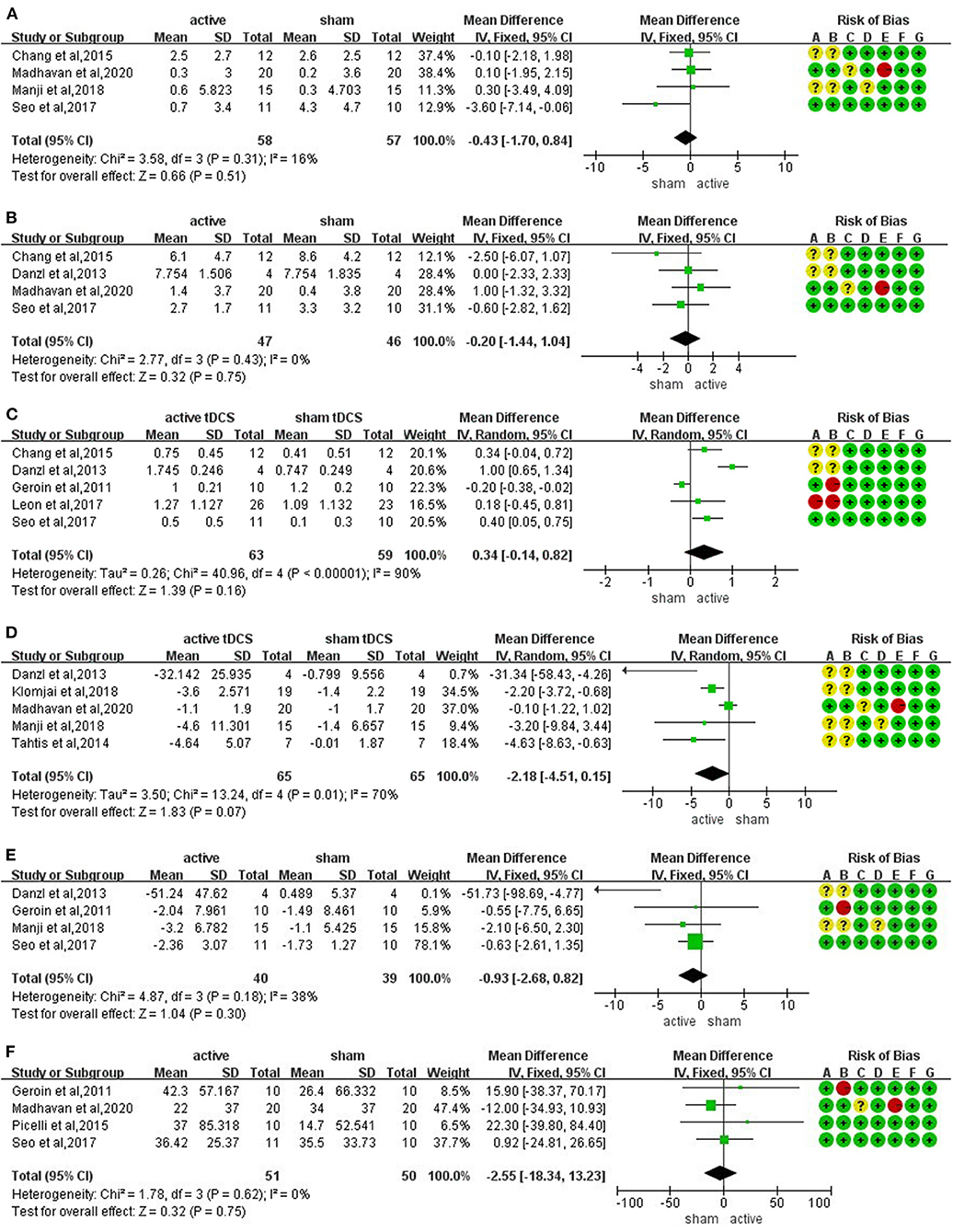
Figure 3. Forrest plots displaying the mean differences (MD) between active and sham groups. (A) Lower extremity subscale of Fugl-Meyer Assessment (FMA-LE). (B) berg balance scale (BBS). (C) functional ambulation category (FAC). (D) timed up and go test (TUGT). (E) 10-m walk test (10 MWT). (F) 6-min walking test (6 MWT).
Berg Balance Scale
The meta-analysis of four trials and 93 participants showed no significant difference on BBS in active tDCS compared with sham tDCS (MD: −0.20; 95% CI: −1.44 to 1.04; p = 0.75; I2 = 0%; inverse variance method with fixed-effects model) (Figure 3B).
Functional Ambulation Category
The meta-analysis of five trials and 122 participants showed no significant difference on FAC in active tDCS compared with sham tDCS (MD: 0.34; 95% CI: −0.14 to 0.82; p = 0.16; I2 = 90%; inverse variance method with random-effects model) (Figure 3C). The studies presented a high heterogeneity (I2 ≥ 50%). A sensitivity analysis was performed by excluding one study at a time. Two studies were excluded achieving I2 = 0%. The result showed a significant improvement on FAC scores in active tDCS compared with sham tDCS (MD: 0.35; 95% CI: 0.11 to 0.58; p = 0.005; I2 = 0%; inverse variance method with fixed-effects model) (Figure 4A).
Timed Up and Go Test
The meta-analysis of five trials and 130 participants showed no significant difference on TUGT in active tDCS compared with sham tDCS (MD: −2.18; 95% CI: −4.51 to 0.15; p = 0.07; I2 = 70%; inverse variance method with random-effects model) (Figure 3D). The studies presented a high heterogeneity (I2 ≥ 50%). A sensitivity analysis was performed by excluding one study at a time. One study was excluded achieving I2 = 47%. The result showed a significant reduction in timing when performing TUGT in active tDCS compared with sham tDCS (MD: −2.61; 95% CI: −4.00 to −1.22; p = 0.0002; I2 = 47%; inverse variance method with fixed-effects model) (Figure 4B).
10-m Walking Test
The meta-analysis of four trials and 79 participants showed no significant difference on 10 MWT in active tDCS compared with sham tDCS (MD: −0.93; 95% CI: −2.68 to 0.82; p = 0.30; I2 = 38%; inverse variance method with fixed-effects model) (Figure 3E).
6-min Walking Test
The meta-analysis of four trials and 101 participants showed no significant difference on 6 MWT in active tDCS compared with sham tDCS (MD: −2.55; 95% CI: −18.34 to 13.23; p = 0.75; I2 = 0%; inverse variance method with fixed-effects model) (Figure 3F).
Discussion
The present study included 10 trials to assess the effects of tDCS on balance capacity in stroke patients. Meta-analysis results showed an overall improvement in FAC and TUGT after implementing active tDCS, but no significant effects were found on FMA-LE, BBS, 10 MWT, and 6 MWT. The Functional Ambulation Category is used to classify locomotion ability depending on how much assistance the subject requires from others when walking (33, 34). Higher scores indicate better walking independence and functional ambulation. The TUGT tests functional mobility and correlates well with gait performance, lower limb weight-bearing, and walking endurance in stroke (35, 36). Although our studies do not directly show a significant effect of tDCS on balance, active tDCS still has some positive effects on walking independence, gait, and ambulation when compared with sham tDCS.
A decrease in cortical excitability often accompanies the occurrence of stroke. The decrease in cortical excitability is usually associated with a lesion of the affected brain or excessive inhibition from the contralateral hemisphere, or both. Previous studies have shown that the reorganization of motor circuits in the cerebral cortex after stroke was more crucial than the neural repair process (37). Therefore, regulating cortical excitability, activating latent neural pathways, or enhancing the efficiency of neural connection to accelerate brain function remodeling is the key to promote the recovery of motor function in stroke patients. So far, several studies have studied the effect of tDCS on the excitability of the motor cortex of lower limbs. Nitsche et al. discovered that tDCS could improve humans' cortical plasticity and motor function in stroke patients (13). Similarly, serval related studies also showed that tDCS applied to the lower limb representative area of the motor cortex could improve ankle fine motor control, (18) motor adaptability, (38) quadriceps muscle strength, (39) and increase the evoked potential amplitude of anterior tibial muscle (40).
Different from the unilateral cortical dominance of the upper limb, the bilateral hemispheres control the lower limb (41). Therefore, the hyperexcitability of the un-lesioned hemisphere relative to the lesioned hinders the lower limb's functional recovery after stroke. Relevant studies have also indicated that gait and balance performance in stroke populations depends on intact interhemispheric connections, (42) and asymmetry between cerebral hemispheres could be used as a prognostic indicator of motor recovery after stroke (43). As Enzinger et al. showed in their study, walking endurance in patients with chronic stroke could be improved by facilitating the bilateral hemispheric motor cortex, cingulate gyrus motor area, and caudate nucleus (44). This suggested that bilateral stimulation might be better than unilateral stimulation in improving balance and gait. Prior studies have found that bilateral tDCS stimulation was better than unilateral tDCS in regulating motor excitability (45), and bilateral tDCS could better reduce stroke patients' fall risk than unilateral tDCS (46). However, further studies are still needed to explore the detailed mechanism of bilateral tDCS in the rehabilitation of balance following stroke.
The underlying mechanism of tDCS in regulating stroke patients' balance function is related to cortical excitability. Weak direct current can lead to subthreshold regulation of membrane potential and regulates the resting membrane potentials toward depolarization or hyperpolarization (14), which affects cortical excitability. Besides, the interhemispheric imbalance after stroke will hinder the recovery of patients' functions. The use of tDCS (especially bilateral tDCS) can restore the two hemispheres' symmetry and improve the stroke population's gait and balance (42). The cerebellum plays an essential role in coordinating limb movements and maintaining body balance. Naro et al. found that cerebellar stimulation could activate the Purkinje cells, intensify its inhibition to cerebellar nuclei, and weaken the cerebral cortex's abnormal excitement (47). When the cerebellum is more involved in the movement's planning and coordination process, it improves the patient's balance function. There is an off-line therapeutic effect of tDCS, which is related to the regulation of synaptic plasticity. The main presentation is long-term potentiation (LTP) and long-term inhibition (LTP) mediated by the N-methyl-D-aspartic acid receptor (NMDA) system (48). Decreased regional cerebral blood flow (rCBF) after stroke is related to postural instability and increases the risk of falls (49). Studies have shown that when the dorsolateral prefrontal cortex (DLPFC) is stimulated by anodal tDCS, rCBF under the electrodes can be increased (50). In contrast, the rCBF significantly decreases under cathode, suggesting that tDCS can improve stroke patients' postural stability and balance function by increasing rCBF.
The current results are also consistent with previous meta-analyses (51), which assessed the effects of tDCS to improve the ambulation ability in stroke patients. The authors included 14 articles with 266 patients in their study, which showed that the FAC and TUGT improved significantly following active tDCS, but the effects on walking speed and walking endurance (6 MWT) were not significant in their study. Similarly, Li et al. revealed that the effects of tDCS on mobility and muscle strength after stroke were statistically significant, despite the results were not significant on balance function (SMD: 0.44; 95% CI: −0.06 to 0.94). (52). Accounting for the non-significant effect size on balance function may be because the maintenance of balance requires multiple factors (visual acuity, proprioception, inner ear function, foot position on the ground, etc.) that are not clear whether they are influenced by tDCS or not (19). In this case, tDCS alone is challenging to improve stroke patients' balance ability, and further studies are needed to evaluate all the factors affecting balance performance. Balance ability measured by BBS was considered the strongest predictor of 10-m and 6-min walking in stroke patients (53). Since tDCS cannot improve balance function, walking speed and walking endurance will also be limited. Besides, this study does not find that tDCS has more advantages in improving lower extremity motor impairment (FMA-LE) in stroke patients, which may be owed to motor impairment after stroke needs a long time to recover. However, this meta-analysis only includes the data immediately after the intervention. At present, the application and research of tDCS after stroke are gradually increasing. The application of tDCS requires the definition of multiple parameters, including the intensity of the stimulation current, duration, number of stimulations, position, and size of the electrodes. Through the combination of different parameters, the effects of tDCS are not the same. There are no unified standards for selecting stimulation parameters in clinical use, and careful considerations such as specific lesion locations, duration of onset, and safety are required. From the perspective of rehabilitation, tDCS, as an adjuvant treatment method, can be combined with functional training such as robotic-assisted gait training (RAGT), (24) constraint-induced movement therapy (CIMT), (54) and virtual reality (55–58), which combines neuromodulation and behavioral intervention to improve the patient's function. In sum, for patients with balance dysfunction after stroke, we should choose appropriate stimulation parameters and combine tDCS stimulation with traditional rehabilitation programs. These may help restore the balance function of stroke patients and make them back to society.
Several limitations should be noted when interpreting the results. (1) The long-term effect of tDCS on balance in stroke patients was not considered. (2) Since some trials did not provide the data directly, or the author did not reply to our data request, part of the data was extracted from the figures. The results may be biased. (3) Only the articles published in English were included. (4) The subgroup analyses were hard to implement because the number of included studies is small. (5) It was not considered the publication bias in the case of a small amount of included literature. In this META analysis, we explored the effects of tDCS on balance and gait in stroke patients based on multiple measurement indicators. In summary, the main conclusion drawn from our meta-analysis is that active tDCS have beneficial effects on FAC and TUGT in stroke patients (i.e., to improve walking independence and functional ambulation). Still, the improvement is not significant in the recovery of lower extremity motor impairment, walking endurance, gait speed, and overall balance function. Future research should focus on reproducing the results in a larger sample size and a long-time follow-up to understand the role of cognitive function in maintaining gait and balance in stroke patients and how tDCS can improve the balance function by affecting cognition. Furthermore, in future studies, we need to consider a variety of neurophysiological results, the effects of various factors on balance, and the use of imaging techniques.
Data Availability Statement
The original contributions presented in the study are included in the article/supplementary material, further inquiries can be directed to the corresponding author/s.
Author Contributions
KD: conceptualization, methodology, formal analysis, and writing-original draft. SM: quality assessment and project administration. ZG: quality assessment and formal analysis. RZ, PX, and EY: investigation and data curation. TL: validation, supervision, writing-reviewing, and editing. All authors contributed to the article and approved the submitted version.
Conflict of Interest
The authors declare that the research was conducted in the absence of any commercial or financial relationships that could be construed as a potential conflict of interest.
Abbreviations
10 MWT, 10-m walk test; 6 MWT, 6-min walking test; BBS, berg balance scale; CME, corticomotor excitability; FAC, functional ambulation category; FMA-LE, lower extremity of Fugl-Meyer assessment; FTSST, five-times-sit-to-stand; MVC, maximum voluntary contraction; POMA, performance-oriented mobility assessment; RCT, randomized controlled trial; TUGT, timed up and go test.
References
1. Benjamin EJ, Blaha MJ, Chiuve SE, Cushman M, Das SR, Deo R, et al. Heart disease and stroke statistics-2017 update: a report from the American Heart Association. Circulation. (2017) 135:e146–603. doi: 10.1161/CIR.0000000000000491
2. Smania N, Picelli A, Gandolfi M, Fiaschi A, Tinazzi M. Rehabilitation of sensorimotor integration deficits in balance impairment of patients with stroke hemiparesis: a before/after pilot study. Neurol Sci. (2008) 29:313–9. doi: 10.1007/s10072-008-0988-0
3. Balaban B, Tok F. Gait disturbances in patients with stroke. PM R. (2014) 6:635–42. doi: 10.1016/j.pmrj.2013.12.017
4. Tyson SF, Hanley M, Chillala J, Selley A, Tallis RC. Balance disability after stroke. Phys Ther. (2006) 86:30–8. doi: 10.1093/ptj/86.1.30
5. Kollen B, Kwakkel G, Lindeman E. Longitudinal robustness of variables predicting independent gait following severe middle cerebral artery stroke: a prospective cohort study. Clin Rehabil. (2006) 20:262–8. doi: 10.1191/0269215506cr910oa
6. Hugues A, Di Marco J, Janiaud P, Xue Y, Pires J, Khademi H, et al. Efficiency of physical therapy on postural imbalance after stroke: study protocol for a systematic review and meta-analysis. BMJ Open. (2017) 7:e013348. doi: 10.1136/bmjopen-2016-013348
7. Kerrigan DC, Gronley J, Perry J. Stiff-legged gait in spastic paresis. A study of quadriceps and hamstrings muscle activity. Am J Phys Med Rehabil. (1991) 70:294–300. doi: 10.1097/00002060-199112000-00003
8. Batchelor FA, Williams SB, Wijeratne T, Said CM, Petty S. Balance and gait impairment in transient ischemic attack and minor stroke. J Stroke Cerebrovasc Dis. (2015) 24:2291–7. doi: 10.1016/j.jstrokecerebrovasdis.2015.06.014
9. Geurts ACH, de Haart M, van Nes IJW, Duysens J. A review of standing balance recovery from stroke. Gait Posture. (2005) 22:267–81. doi: 10.1016/j.gaitpost.2004.10.002
10. Lee CH, Kim Y, Lee BH. Augmented reality-based postural control training improves gait function in patients with stroke: Randomized controlled trial. Hong Kong Physiother J. (2014) 32:51–7. doi: 10.1016/j.hkpj.2014.04.002
11. Lee SH, Byun SD, Kim CH, Go JY, Nam HU, Huh JS, et al. Feasibility and effects of newly developed balance control trainer for mobility and balance in chronic stroke patients: a randomized controlled trial. Ann Rehabil Med. (2012) 36:521–9. doi: 10.5535/arm.2012.36.4.521
12. de Rooij IJM, van de Port IGL, Meijer J-WG. Effect of virtual reality training on balance and gait ability in patients with stroke: systematic review and meta-analysis. Phys Ther. (2016) 96:1905–18. doi: 10.2522/ptj.20160054
13. Nitsche MA, Paulus W. Sustained excitability elevations induced by transcranial DC motor cortex stimulation in humans. Neurology. (2001) 57:1899–901. doi: 10.1212/WNL.57.10.1899
14. Lefaucheur J-P, Antal A, Ayache SS, Benninger DH, Brunelin J, Cogiamanian F, et al. Evidence-based guidelines on the therapeutic use of transcranial direct current stimulation (tDCS). Clin Neurophysiol. (2017) 128:56–92. doi: 10.1016/j.clinph.2016.10.087
15. Nitsche MA, Schauenburg A, Lang N, Liebetanz D, Exner C, Paulus W, et al. Facilitation of implicit motor learning by weak transcranial direct current stimulation of the primary motor cortex in the human. J Cogn Neurosci. (2003) 15:619–26. doi: 10.1162/089892903321662994
16. Kang N, Summers JJ, Cauraugh JH. Transcranial direct current stimulation facilitates motor learning post-stroke: a systematic review and meta-analysis. J Neurol Neurosurg Psychiatry. (2016) 87:345–55. doi: 10.1136/jnnp-2015-311242
17. Tedesco Triccas L, Burridge JH, Hughes AM, Pickering RM, Desikan M, Rothwell JC, et al. Multiple sessions of transcranial direct current stimulation and upper extremity rehabilitation in stroke: a review and meta-analysis. Clin Neurophysiol. (2016) 127:946–55. doi: 10.1016/j.clinph.2015.04.067
18. Madhavan S, Weber KA 2nd, Stinear JW. Non-invasive brain stimulation enhances fine motor control of the hemiparetic ankle: implications for rehabilitation. Exp Brain Res. (2011) 209:9–17. doi: 10.1007/s00221-010-2511-0
19. Sohn MK, Jee SJ, Kim YW. Effect of transcranial direct current stimulation on postural stability and lower extremity strength in hemiplegic stroke patients. Ann Rehabil Med. (2013) 37:759–65. doi: 10.5535/arm.2013.37.6.759
20. Steiner KM, Enders A, Thier W, Batsikadze G, Ludolph N, Ilg W, et al. Cerebellar tDCS does not improve learning in a complex whole body dynamic balance task in young healthy subjects. PLoS ONE. (2016) 11:e0163598. doi: 10.1371/journal.pone.0163598
21. Moher D, Liberati A, Tetzlaff J, Altman DG. Preferred reporting items for systematic reviews and meta-analyses: the PRISMA statement. BMJ. (2009) 339:b2535. doi: 10.1136/bmj.b2535
22. Higgins JPT, Thomas J, Chandler J, Cumpston M, Li T, Page MJ, et al. editors. Cochrane Handbook for Systematic Reviews of Interventions. 2nd ed. Chichester: John Wiley & Sons (2019).
23. Tahtis V, Kaski D, Seemungal BM. The effect of single session bi-cephalic transcranial direct current stimulation on gait performance in sub-acute stroke: a pilot study. Restor Neurol Neurosci. (2014) 32:527–32. doi: 10.3233/RNN-140393
24. Seo HG, Lee WH, Lee SH, Yi Y, Kim KD, Oh B-M. Robotic-assisted gait training combined with transcranial direct current stimulation in chronic stroke patients: A pilot double-blind, randomized controlled trial. Restor Neurol Neurosci. (2017) 35:527–36. doi: 10.3233/RNN-170745
25. Picelli A, Chemello E, Castellazzi P, Roncari L, Waldner A, Saltuari L, et al. Combined effects of transcranial direct current stimulation (tDCS) and transcutaneous spinal direct current stimulation (tsDCS) on robot-assisted gait training in patients with chronic stroke: a pilot, double blind, randomized controlled trial. Restor Neurol Neurosci. (2015) 33:357–68. doi: 10.3233/RNN-140474
26. Manji A, Amimoto K, Matsuda T, Wada Y, Inaba A, Ko S. Effects of transcranial direct current stimulation over the supplementary motor area body weight-supported treadmill gait training in hemiparetic patients after stroke. Neurosci Lett. (2018) 662:302–5. doi: 10.1016/j.neulet.2017.10.049
27. Madhavan S, Cleland BT, Sivaramakrishnan A, Freels S, Lim H, Testai FD, et al. Cortical priming strategies for gait training after stroke: a controlled, stratified trial. J Neuroeng Rehabil. (2020) 17:111. doi: 10.1186/s12984-020-00744-9
28. Danzl MM, Chelette KC, Lee K, Lykins D, Sawaki L. Brain stimulation paired with novel locomotor training with robotic gait orthosis in chronic stroke: a feasibility study. NeuroRehabilitation. (2013) 33:67–76. doi: 10.3233/NRE-130929
29. Chang MC, Kim DY, Park DH. Enhancement of cortical excitability and lower limb motor function in patients with stroke by transcranial direct current stimulation. Brain Stimul. (2015) 8:561–6. doi: 10.1016/j.brs.2015.01.411
30. Leon D, Cortes M, Elder J, Kumru H, Laxe S, Edwards DJ, et al. tDCS does not enhance the effects of robot-assisted gait training in patients with subacute stroke. Restor Neurol Neurosci. (2017) 35:377–84. doi: 10.3233/RNN-170734
31. Klomjai W, Aneksan B, Pheungphrarattanatrai A, Chantanachai T, Choowong N, Bunleukhet S, et al. Effect of single-session dual-tDCS before physical therapy on lower-limb performance in sub-acute stroke patients: a randomized sham-controlled crossover study. Ann Phys Rehabil Med. (2018) 61:286–91. doi: 10.1016/j.rehab.2018.04.005
32. Geroin C, Picelli A, Munari D, Waldner A, Tomelleri C, Smania N. Combined transcranial direct current stimulation and robot-assisted gait training in patients with chronic stroke: a preliminary comparison. Clin Rehabil. (2011) 25:537–48. doi: 10.1177/0269215510389497
33. Holden MK, Gill KM, Magliozzi MR. Gait assessment for neurologically impaired patients. Standards for outcome assessment. Phys Ther. (1986) 66:1530–9. doi: 10.1093/ptj/66.10.1530
34. Mehrholz J, Wagner K, Rutte K, Meissner D, Pohl M. Predictive validity and responsiveness of the functional ambulation category in hemiparetic patients after stroke. Arch Phys Med Rehabil. (2007) 88:1314–9. doi: 10.1016/j.apmr.2007.06.764
35. Podsiadlo D, Richardson S. The timed “Up & Go”: a test of basic functional mobility for frail elderly persons. J Am Geriatr Soc. (1991) 39:142–8. doi: 10.1111/j.1532-5415.1991.tb01616.x
36. Ng SS, Hui-Chan CW. The timed up & go test: its reliability and association with lower-limb impairments and locomotor capacities in people with chronic stroke. Arch Phys Med Rehabil. (2005) 86:1641–7. doi: 10.1016/j.apmr.2005.01.011
37. Ward N. Assessment of cortical reorganisation for hand function after stroke. J Physiol. (2011) 589:5625–32. doi: 10.1113/jphysiol.2011.220939
38. Kaski D, Quadir S, Patel M, Yousif N, Bronstein AM. Enhanced locomotor adaptation aftereffect in the “broken escalator” phenomenon using anodal tDCS. J Neurophysiol. (2012) 107:2493–505. doi: 10.1152/jn.00223.2011
39. Tanaka S, Takeda K, Otaka Y, Kita K, Osu R, Honda M, et al. Single session of transcranial direct current stimulation transiently increases knee extensor force in patients with hemiparetic stroke. Neurorehabil Neural Repair. (2011) 25:565–9. doi: 10.1177/1545968311402091
40. Jeffery DT, Norton JA, Roy FD, Gorassini MA. Effects of transcranial direct current stimulation on the excitability of the leg motor cortex. Exp Brain Res. (2007) 182:281–7. doi: 10.1007/s00221-007-1093-y
41. Luft AR, Smith GV, Forrester L, Whitall J, Macko RF, Hauser T-K, et al. Comparing brain activation associated with isolated upper and lower limb movement across corresponding joints. Hum Brain Mapp. (2002) 17:131–40. doi: 10.1002/hbm.10058
42. Sullivan EV, Adalsteinsson E, Hedehus M, Ju C, Moseley M, Lim KO, et al. Equivalent disruption of regional white matter microstructure in ageing healthy men and women. Neuroreport. (2001) 12:99–104. doi: 10.1097/00001756-200101220-00027
43. Agius Anastasi A, Falzon O, Camilleri K, Vella M, Muscat R. Brain symmetry index in healthy and stroke patients for assessment and prognosis. Stroke Res Treat. (2017) 2017:8276136. doi: 10.1155/2017/8276136
44. Enzinger C, Dawes H, Johansen-Berg H, Wade D, Bogdanovic M, Collett J, et al. Brain activity changes associated with treadmill training after stroke. Stroke. (2009) 40:2460–7. doi: 10.1161/STROKEAHA.109.550053
45. Halakoo S, Ehsani F, Hosnian M, Zoghi M, Jaberzadeh S. The comparative effects of unilateral and bilateral transcranial direct current stimulation on motor learning and motor performance: A systematic review of literature and meta-analysis. J Clin Neurosci. (2020) 72:8–14. doi: 10.1016/j.jocn.2019.12.022
46. Andrade SM, Ferreira JJ, de A, Rufino TS, Medeiros G, Brito JD, da Silva MA, et al. Effects of different montages of transcranial direct current stimulation on the risk of falls and lower limb function after stroke. Neurol Res. (2017) 39:1037–43. doi: 10.1080/01616412.2017.1371473
47. Naro A, Bramanti A, Leo A, Manuli A, Sciarrone F, Russo M, et al. Effects of cerebellar transcranial alternating current stimulation on motor cortex excitability and motor function. Brain Struct Funct. (2017) 222:2891–906. doi: 10.1007/s00429-016-1355-1
48. Cooke SF, Bliss TVP. Plasticity in the human central nervous system. Brain. (2006) 129:1659–73. doi: 10.1093/brain/awl082
49. Fitzgibbon-Collins LK, Noguchi M, Heckman GA, Hughson RL, Robertson AD. Acute reduction in cerebral blood velocity on supine-to-stand transition increases postural instability in young adults. Am J Physiol Heart Circ Physiol. (2019) 317:H1342–53. doi: 10.1152/ajpheart.00360.2019
50. Stagg CJ, Lin RL, Mezue M, Segerdahl A, Kong Y, Xie J, et al. Widespread modulation of cerebral perfusion induced during and after transcranial direct current stimulation applied to the left dorsolateral prefrontal cortex. J Neurosci. (2013) 33:11425–31. doi: 10.1523/JNEUROSCI.3887-12.2013
51. Tien H-H, Liu W-Y, Chen Y-L, Wu Y-C, Lien H-Y. Transcranial direct current stimulation for improving ambulation after stroke: a systematic review and meta-analysis. Int J Rehabil Res. (2020) 43:299–309. doi: 10.1097/MRR.0000000000000427
52. Li Y, Fan J, Yang J, He C, Li S. Effects of transcranial direct current stimulation on walking ability after stroke: A systematic review and meta-analysis. Restor Neurol Neurosci. (2018) 36:59–71. doi: 10.3233/RNN-170770
53. Patterson SL, Forrester LW, Rodgers MM, Ryan AS, Ivey FM, Sorkin JD, et al. Determinants of walking function after stroke: differences by deficit severity. Arch Phys Med Rehabil. (2007) 88:115–9. doi: 10.1016/j.apmr.2006.10.025
54. Figlewski K, Blicher JU, Mortensen J, Severinsen KE, Nielsen JF, Andersen H. Transcranial direct current stimulation potentiates improvements in functional ability in patients with chronic stroke receiving constraint-induced movement therapy. Stroke. (2017) 48:229–32. doi: 10.1161/STROKEAHA.116.014988
55. Massetti T, Crocetta TB, Silva TD da, Trevizan IL, Arab C, Caromano FA, et al. Application and outcomes of therapy combining transcranial direct current stimulation and virtual reality: a systematic review. Disabil Rehabil Assist Technol. (2017) 12:551–9. doi: 10.1080/17483107.2016.1230152
56. Kim YJ, Ku J, Cho S, Kim HJ, Cho YK, Lim T, et al. Facilitation of corticospinal excitability by virtual reality exercise following anodal transcranial direct current stimulation in healthy volunteers and subacute stroke subjects. J Neuroeng Rehabil. (2014) 11:1–12. doi: 10.1186/1743-0003-11-124
57. Lee SJ, Chun MH. Combination transcranial direct current stimulation and virtual reality therapy for upper extremity training in patients with subacute stroke. Arch Phys Med Rehabil. (2014) 95:431–8. doi: 10.1016/j.apmr.2013.10.027
Keywords: stroke, balance, transcranial direct current stimulation, meta-analysis, gait
Citation: Dong K, Meng S, Guo Z, Zhang R, Xu P, Yuan E and Lian T (2021) The Effects of Transcranial Direct Current Stimulation on Balance and Gait in Stroke Patients: A Systematic Review and Meta-Analysis. Front. Neurol. 12:650925. doi: 10.3389/fneur.2021.650925
Received: 12 January 2021; Accepted: 06 April 2021;
Published: 25 May 2021.
Edited by:
Andrea Martinuzzi, Eugenio Medea (IRCCS), ItalyReviewed by:
Marianna Capecci, Marche Polytechnic University, ItalyAntonino Naro, Centro Neurolesi Bonino Pulejo (IRCCS), Italy
Copyright © 2021 Dong, Meng, Guo, Zhang, Xu, Yuan and Lian. This is an open-access article distributed under the terms of the Creative Commons Attribution License (CC BY). The use, distribution or reproduction in other forums is permitted, provided the original author(s) and the copyright owner(s) are credited and that the original publication in this journal is cited, in accordance with accepted academic practice. No use, distribution or reproduction is permitted which does not comply with these terms.
*Correspondence: Tao Lian, dGFvbGlhbjEwMDBAMTI2LmNvbQ==