- 1Department of Anesthesiology, Xuanwu Hospital, Capital Medical University, Beijing, China
- 2Department of Neurosurgery, Xuanwu Hospital, Capital Medical University, Beijing, China
- 3Department of Vascular Ultrasound, Xuanwu Hospital, Capital Medical University, Beijing, China
Background: Patients undergoing carotid endarterectomy (CEA) for severe carotid stenosis are vulnerable to postoperative delirium, a complication frequently associated with poor outcome. This study investigated the impact of processed electroencephalogram (EEG)-guided anesthesia management on the incidence of postoperative delirium in patients undergoing CEA.
Methods: This single-center, prospective, randomized clinical trial on 255 patients receiving CEA under general anesthesia compared the outcomes of patient state index (PSI) monitoring [SEDLine Brain Function Monitor (Masimo, Inc, Irvine, CA)] (standard group, n = 128) with PSI combined with density spectral array(DSA) -guided monitoring (intervention group, n = 127) to reduce the risk of intraoperative EEG burst suppression. All patients were monitored by continuous transcranial Doppler ultrasound (TCD) and near-infrared spectroscopy (NIRS) to avoid perioperative cerebral hypoperfusion or hyperperfusion. According to the surgical process, EEG suppression time was calculated separately for three stages: S1 (from anesthesia induction to carotid artery clamping), S2 (from clamping to declamping), and S3 (from declamping to the end of surgery). The primary outcome was incidence of postoperative delirium according to the Confusion Assessment Method algorithm during the first 3 days post-surgery, and secondary outcomes were other neurologic complications and length of hospital stay.
Results: There were no episodes of cerebral hypoperfusion or hyperperfusion according to TCD and NIRS monitoring in either group during surgery. The incidence of postoperative delirium within 3 days post-surgery was significantly lower in the intervention group than the standard group (7.87 vs. 28.91%, P < 0.01). In the intervention group, the total EEG suppression time and the EEG suppression time during S2 and S3 were shorter (Total, 0 “0” vs. 0 “1.17” min, P = 0.04; S2, 0 “0” vs. 0 “0.1” min, P < 0.01; S3, 0 “0” vs. 0 “0” min, P = 0.02). There were no group differences in incidence of neurologic complications and length of postoperative hospital stay.
Conclusion: Processed electroencephalogram-guided general anesthesia management, consisting of PSI combined with DSA monitoring, can significantly reduce the risk of postoperative delirium in patients undergoing CEA. Patients, especially those exhibiting hemodynamic fluctuations or receiving surgical procedures that disrupt cerebral perfusion, may benefit from the monitoring of multiple EEG parameters during surgery.
Clinical Trial Registration: www.ClinicalTrials.gov, identifier: NCT03622515.
Key Points
• Question: Can EEG-guided general anesthesia management reduce the risk of postoperative delirium in high-risk patients undergoing CEA?
• Findings: Processed electroencephalography-guided anesthesia management, including PSI combined with DSA monitoring, can significantly reduce postoperative delirium incidence in patients undergoing CEA.
• Meaning: This randomized clinical trial suggests that slight changes in anesthetic management may play a major role in modulating postoperative delirium risk in patients undergoing CEA under general anesthesia. Vulnerable patients, especially those exhibiting hemodynamic fluctuations or undergoing surgical procedures that disrupt cerebral perfusion, may benefit from the monitoring of multiple EEG parameters during surgery.
Introduction
Carotid endarterectomy (CEA) is the gold standard treatment for patients with severe carotid stenosis (CS) to reduce the risk of stroke (1–4). The safety and long-term efficacy of CEA for the management of carotid artery disease has been demonstrated in large randomized controlled trials (5–8). However, cerebral blood supply may be severely disrupted by anesthesia and surgical manipulations during CEA, and cerebral function is highly vulnerable to even brief changes in oxygen and blood supply. Cerebral vascular diseases may further increase the risk of perioperative neurological dysfunction. For instance, there is a significant association between carotid artery stenosis and postoperative delirium (9, 10), an acute state of mental confusion defined by alterations in attention, consciousness, and disorganized thinking (11, 12). Postoperative delirium (POD) is a common yet serious geriatric syndrome that afflicts 10–60% of patients after major surgery (13–16) and up to 91% of the critically ill (17). Further, POD is associated with worse early and long-term prognosis as well as greater healthcare costs (11, 14, 17–19). Therefore, the United Kingdom National Institute for Health and Care Excellence, the American Geriatric Society, the American College of Surgeons, and the American Society of Anesthesiologists have all identified the prevention of postoperative delirium as a public health priority (20–23).
Risk factors for delirium are usually divided into predisposing and precipitating (24). Predisposing factors include preoperative vulnerabilities, while precipitating factors are potentially reversible events occurring throughout the perioperative period. POD incidence increases with longer cumulative intraoperative electroencephalogram (EEG) suppression duration (25, 26), and processed EEG monitoring during surgery may lower POD rate and prevent or minimize EEG suppression by using minimal anesthetic doses (27–31). A burst suppression pattern on the EEG indicates severe inhibition of neuronal activity and metabolic rate. Patients with severe CS are prone to vascular cerebral injury, and may therefore be particularly vulnerable to EEG suppression (32, 33). It is not clear, however, whether processed EEG-guided anesthesia management can reduce POD incidence in patients undergoing CEA and if this effect depends on decreasing EEG suppression.
The objectives of this study were to investigate the effect of processed EEG-guided anesthesia management on the incidence of POD in patients receiving CEA under general anesthesia. The primary hypothesis was that processed EEG-guided anesthesia management can effectively reduce the likelihood of the incidence of POD during the first 3 days following CEA.
Methods
Research Design
This is a single-center, prospective, randomized clinical trial with two parallel arms. The research protocol was approved by the Ethics Committee of Xuanwu Hospital of Capital Medical University (LYS[2018]053) and written informed consent was obtained from all subjects participating in the trial. The trial was registered prior to patient enrollment at clinicaltrials.gov (NCT03622515, Principal investigator: Na Xu, Date of registration: August 8, 2018). All surgeries were conducted at Xuanwu Hospital (Beijing, China). Results are reported according to CONSORT guidelines.
Participants
Study candidates were screened by investigators the day before surgery (or on Friday for those scheduled for surgery the following Monday). We recruited patients at our institution from August 2018 to December 2019. All surgeries were performed by the same surgical and anesthesia team. Inclusion criteria included patients scheduled to undergo elective CEA under general anesthesia, fluency in Chinese Mandarin, an anticipated length of hospital stay over 3 days after surgery, and willingness to comply with the research protocol. Exclusion criteria were as follows: (1) declining preoperative condition (such as unstable angina, acute myocardial infarction, and heart function NYHA III to IV) within 4–6 weeks before surgery, (2) severe hepatic dysfunction (Child–Pugh grade C) or renal failure (requirement for renal replacement therapy), (3) history of schizophrenia, Parkinson's disease, or traumatic brain injury, (4) inability to perform neurocognitive testing, (5) preoperative delirium according to the Confusion Assessment Method (CAM) algorithm (34), (6) preoperative cognitive impairment according to the Chinese Mini-Mental State Examination (MMSE) corrected for education level (illiterate ≤ 19 points, 1–6 years of primary school ≤ 22 points, middle school or above ≤ 26 points) and Montreal Cognitive Assessment (MoCA) score (illiterate ≤ 13 points, primary school ≤ 19 points, middle school or above ≤ 24 points) (35, 36), (7) preoperative depression according the Self-rating Depression Scale (SDS, score > 41 points) or preoperative anxiety according to the Self-rating Anxiety Scale (SAS, score > 41 points) (37, 38), (8) change in surgical procedure after anesthesia, (9) return to the intensive care unit following surgery, (10) conditions that caused severe hemodynamic fluctuations (such as severe allergic reactions or major bleeding), and (11) accidental discharge.
Baseline Data Collection
Baseline data included demographics, co-morbidities, and relevant physical and laboratory findings. Preoperative physical condition was evaluated using the American Society of Anesthesiologists (ASA) Physical Status Classification System (39). Activities of daily living were assessed using the Barthel Index (score range 0–100, with higher score indicating better independent function) (40). Cognitive functions were assessed using the MMSE and MoCA. Anxiety and depression were assessed using the SAS and SDS because several studies have identified preoperative depression (41–43) and anxiety (44) as risk factors for postoperative delirium incidence or duration. Delirium status was assessed with the CAM. Pre-surgical tests of baseline general cognition, delirium, anxiety, and depression were conducted by a neuropsychologist blinded to group allocation (see below).
Randomization and Blinding
Patients were assigned to intervention and control arms before surgery using a computerized random number generator at a 1:1 ratio. A seed was not specified, and blocks were not used in randomization. Randomization was conducted after patient consent for research participation during the preoperative interview. Both patients and research associates conducting preoperative testing and postoperative outcome assessments were also blinded to group assignment.
Anesthesia and Surgery
General anesthesia was induced in all patients by intravenous etomidate 0.15 mg·kg−1, and sufentanil 0.2 mg·kg−1, and maintained by continuous propofol infusion. In the standard monitoring group, propofol dosage was 50–80 mcg·kg−1·min−1, while in the intervention group, dosage was adjusted according to sedative depth as described in the next section (Intervention). All patients received remifentanil and dexmedetomidine as well as rocuronium (0.6 mg·kg−1) or cisatracurium (0.15 mg·kg−1) injection for muscle relaxation. Patients were mechanically ventilated with FiO2 50%, and SaO2 was maintained at more than 95%. Goal-directed fluid and vasoconstrictive drug therapies were conducted to maintain stroke volume variability below 13% and regulated patients' blood pressure within 180 mmHg according to transcranial Doppler ultrasound (TCD, China Shenzhen Delica Medical Equipment, ShenZhen, China) and near-infrared spectroscopy (NIRS, Cas Medical Systems, Inc, Branford, Connecticut, USA). Intraoperative warming devices were used to maintain nasopharyngeal temperature between 36°C and 37°C. Perioperative care was standardized according to institutional routines for all patients.
All CEA procedures were performed by the same team of 4 experienced neurosurgeons who have worked for more than 15 years. As there is a correlation between intraoperative hypotension and incidence of delirium (45, 46), all patients were monitored by TCD and NIRS during CEA to minimize the risks of perioperative cerebral hypoperfusion and hyperperfusion. Regional cerebral oxygenation (rSO2) was also monitored by NIRS and mean flow velocity of the middle cerebral artery (MFVMCA) by TCD. The NIRS probes were placed on the bilateral forehead. Cerebral ischemia was deemed to have occurred if the ipsilateral MFVMCA was reduced by >50% compared to baseline or rSO2 decreased by 20% from baseline during the clamping process (47–50). An increase in MFVMCA of 100% after carotid declamping compared to baseline was considered indicative of cerebral hyperperfusion (51). Anesthesiologists managed cerebral hypoperfusion or hyperperfusion during CEA by regulating the patient's blood pressure, which was elevated or reduced by 10% before and after declamping the carotid artery, respectively. Carotid shunting was conducted based on TCD monitoring and the surgeon's discretion.
Intervention
During surgery, patients from both the groups were monitored using the SEDLine Brain Function Monitor (Masimo, Inc, Irvine CA), which uses symmetrical bifrontal electrodes to measure four channels of raw EEG data with separate displays for electromyogram (EMG), artifacts (e.g., patient motion), burst suppression ratio (BSR), and density spectral array (DSA). The SEDLine monitor also estimates sedative depth from digital EEG waves using a proprietary algorithm and displays it as a dimensionless parameter called the patient state index (PSI, ranging 0–100, with 100 indicating wakefulness and 0 isoelectric EEG) (52). Even though there are some raw EEG patterns clearly visible on the spectrogram plots, we are nowhere close to being able to describe the cerebrum depending on the information obtained from the processed EEG. Therefore, in the intervention group, we visually analyzed and inspected DSA as an intervention indicator for EEG burst suppression rather than EEG suppression waveforms (53, 54). Whenever the DSA indicated burst suppression, propofol dosage was reduced 5 mcg·kg−1·min−1, as clinically permitted. In order to prevent intraoperative awareness, if lowering propofol was found to cause PSI to reach 60, but DSA still showed burst suppression, we stopped adjusting propofol and adjusted blood pressure according to the stage of surgery to ensure cerebral perfusion. For instance, blood pressure was elevated by 5% before declamping the carotid artery or decreased by 5% after declamping. In the standard group, the monitor screen was masked, all EEG data and spectrograms were blinded, and only PSI values were displayed. Anesthesiologists performed anesthesia by conventional methods and maintained the PSI at 25–50 in the standard group.
Outcome Assessments
Measurement of Delirium
Delirium was assessed by trained research team members preoperatively and daily on the first three postoperative days using the Chinese version of CAM algorithm, which has demonstrated good reliability and validity among the Chinese population (55). Postoperative visits were conducted between 10:00 A.M. and 16:00 P.M. at the patient's bedside. Delirium was defined by acute onset with fluctuating course, inattention, disorganized thinking, and (or) altered level of consciousness.
Measurement of EEG Suppression
The raw EEG data, PSI, and BSR were obtained from all patients using the SEDLine monitor. The EEG recordings were initiated from anesthesia induction, and ended at the completion of surgical manipulation. All EEG data were then edited and saved. The experienced neurophysiologists independently reviewed the intraoperative EEG traces acquired by the SEDLine® monitoring system for the entire duration of the operation, recorded whether or not burst suppression was present, and calculated the cumulative duration of EEG suppression in minutes. The neurologists recorded an epoch as having a burst suppression pattern if there was at least 5 s of suppression of the EEG tracing present in a given 30 s epoch (56). We recorded the cumulative duration of total EEG suppression and the duration of EEG suppression for three surgical stages: S1 (from anesthesia induction to carotid artery clamping), S2 (from clamping to declamping), and S3 (from declamping to the end of surgery).
The anesthesiologists received training by neurophysiologists in reading and interpreting the DSA before the study was initiated to ensure that all anesthesiologists could reach 100% consensus on the interpretation of the DSA. Additionally, the training process was repeated at 6-month intervals during the study.
Magnetic Resonance Image Data Acquisition
Cerebral microembolization is a significant contributor to postoperative delirium (57, 58). Therefore, magnetic resonance imaging examinations were conducted before and 24 h following surgery using a Clinical 3-Tesla whole-body MR imager (Verio; Siemens Medical Solutions, Erlangen, Germany). In accordance with previous studies (59–62), new ischemic cerebral lesions were defined as hyperintense regions on post-intervention diffusion-weighted images that were not present on pretreatment images. Ischemic lesions (number and total volume of hyperintense regions) were evaluated by a radiologist and neurologist both blinded to the research protocol, and disagreements were resolved by consensus.
Measurement of Anesthetic Doses
To determine whether processed EEG-guidance resulted in propofol dosage reduction, we calculated the cumulative doses of all anesthetics according to intraoperative electronic medical records.
Measurement of Vital Signs
Mean arterial blood pressure (MAP), PSI, end-expiratory carbon dioxide partial pressure (PETCO2), bilateral rSO2, and bilateral MFVMCA were recorded at three time-points, immediately after general anesthesia induction and before carotid artery clamping as baseline (T1), immediately after clamping (T2), and after declamping and subsequent stabilization of cerebral perfusion (T3).
Measurement of Secondary Outcomes
Physical and neurological examinations were conducted by a neurologist blinded to group allocation before and for the first 3 days after surgery. Examinations included evaluation of neurological deficits according to the National Institutes of Health Stroke Scale. From the beginning of anesthesia to 3 days after surgery, we also monitored adverse events such as intraoperative movement or awareness. Finally, length of hospital stay was recorded.
Statistical Analysis
Postoperative Outcome Analysis
The Kolmogorov–Smirnov test was used to check for the normality of all continuous variables. Continuous datasets with a normal distribution were compared by independent-samples t-test, and continuous datasets with non-normal distributions by independent-samples Mann–Whitney U-tests. Categorical data were compared by χ2 test or Fisher exact test as indicated. Measurement data at each time point were compared between the two groups by analysis of variance with repeated measures (RT-ANOVA). Normally distributed continuous variables are reported as mean ± standard deviation (s.d.) and non-normally distributed continuous variables as median (interquartile range, i.q.r.).
Statistical analyses were conducted using SPSS® Version 21(IBM Inc. Chicago, IL, USA). All tests were two-tailed, and a two-sided P < 0.050 was considered statistically significant.
Sample Size Calculation
We calculated the sample size based on the expected incidence of the primary outcome (postoperative delirium). In a pilot study, 20 patients meeting the inclusion criteria were included and randomly divided into an intervention group and standard care group, with 10 patients in each group. One patient in the standard care group had postoperative delirium, whereas patients in the intervention group did not have any postoperative neurological complications. Therefore, postoperative neurological complications rates where 0% in the interventional group (to avoid errors, the incidence was increased of 0.5% for calculating the sample size) and 10% in the standard group. We set type I error α = 0.05 and the type II error β = 0.1. From this, the sample size of each group was estimated at 91 cases using the sample size software PASS11 (NCSS, Caseville, Utah, United States). Assuming a 20% loss to follow-up, we had planned to enroll at least 220 patients undergoing CEA.
Results
Patient Recruitment
This clinical trial was conducted from August 2018 to December 2019. Overall, 366 patients were deemed eligible and 272 patients were included, with patients randomly and equally divided into processed EEG-guided anesthetic management (intervention) and standard anesthesia care groups (Figure 1). Anesthesiologists adhered to the protocol for all 136 patients assigned to the intervention group. During the study period, five surgeries were changed to carotid stenting (four cases in the intervention group and one in the standard care group, and they did not receive any of the study intervention in either group), 10 cases did not complete the postoperative CAM test (five cases in each group), and two cases in the standard care group were transferred to the intensive care unit after surgery. Therefore, 255 patients were included in the final analysis, 127 in the intervention group and 128 in the standard care group (Figure 1). Preoperative demographic, surgical, and intraoperative variables for each group are summarized in Tables 1, 2. Baseline variables, such as age, sex ratio, preoperative co-morbidity, and cognitive and mood assessments, among others (Table 1), and intraoperative variables, such as surgery duration, anesthesia duration, and clamping duration, among others, were well-matched between the groups (all P > 0.05) (Table 2). In addition, intraoperative anesthesia dose did not differ between groups.
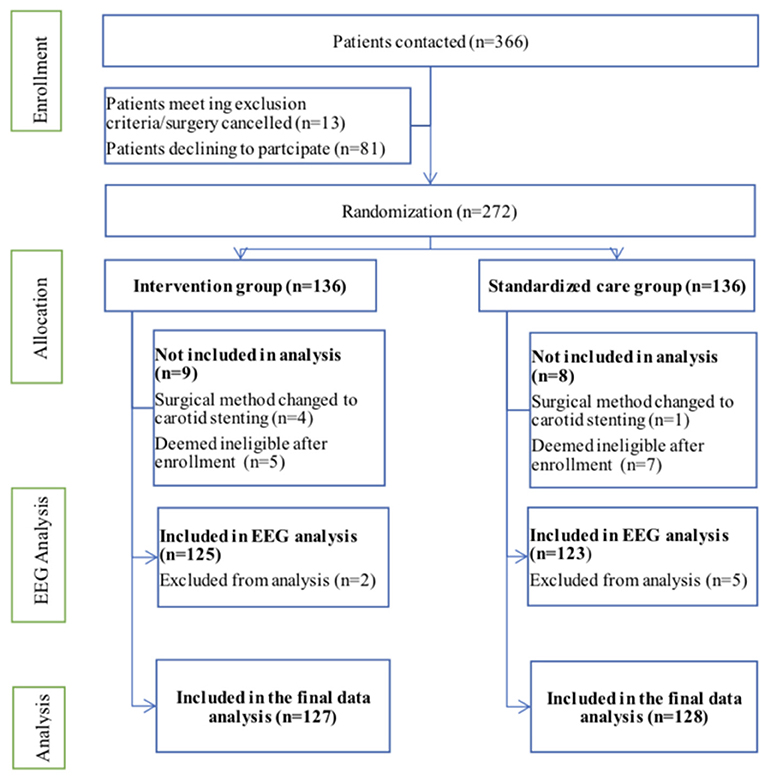
Figure 1. Flow diagram depicting patient recruitment for this clinical trial. EEG, electroencephalography.
Incident Delirium
The incidence of postoperative delirium was significantly lower among patients receiving processed EEG-guided anesthesia management compared with that in the standard care group [10 of 127 (7.87%) vs. 37 of 128 (28.91%), P < 0.01] (Table 3).
Intraoperative EEG Suppression
Seven patients were excluded from EEG analysis because of corrupted data. The total duration of EEG suppression was significantly shorter in the intervention group than the standard care group [0 (0) vs. 0 (1.17), P = 0.04] (Table 3). The effect of the intervention on EEG suppression differed according to surgical stage, with significantly shorter durations during S2 and S3 among the intervention group patients compared to the standard group patients, while there was no significant difference in duration during S1.
Other Outcomes and Safety of the Intervention
There were no significant differences in incidence of new cerebral infarctions according to symptoms and neuroimaging, incidence of intracerebral hemorrhage according to neuroimaging, and hospital stay duration between groups (Table 3). No intraoperative movement or awareness was observed in any patient during surgery and no deaths occurred after surgery.
Intraoperative Monitoring Values at Different Time-Points
There were also no significant differences in MAP, PETCO2, bilateral MFVMCA and rSO2 between groups at corresponding time-points (Figures 2–7), while PSI was higher in the intervention group at all measurement time-points (P < 0.05) (Figure 8). There were differences in MAP, PETCO2, PSI, ipsilateral MFVMCA, and bilateral rSO2 at all time-points within the two groups. MAP, PETCO2 and contralateral rSO2 at T2 were higher than at T1 and T3 (P < 0.05); PSI, ipsilateral rSO2 and MFVMCA at T2 were lower than at T1 and T3 (P < 0.05). While contralateral MFVMAC was not significantly different at all time-points in the two groups (Table 4).
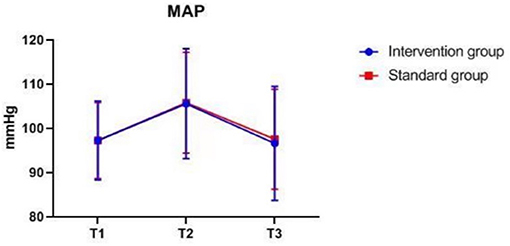
Figure 2. MAP over time. MAP, mean arterial blood pressure. The measurement time interval after general anesthesia but before clamping the carotid artery was recorded as baseline reference T1, the time interval after clamping but before declamping was recorded as T2, and the time interval after the declamping and stabilization of cerebral perfusion but before completion of surgery was recorded as T3.
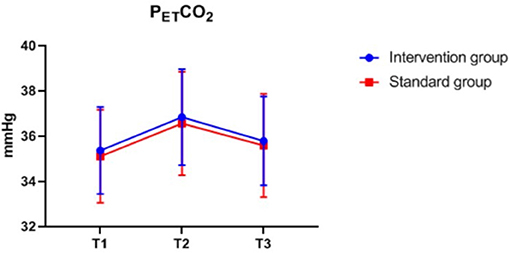
Figure 3. PETCO2 over time. PETCO2, end-expiratory carbon dioxide partial pressure. The measurement time interval after general anesthesia but before clamping the carotid artery was recorded as baseline reference T1, the time interval after clamping but before declamping was recorded as T2, and the time interval after the declamping and stabilization of cerebral perfusion but before completion of surgery was recorded as T3.
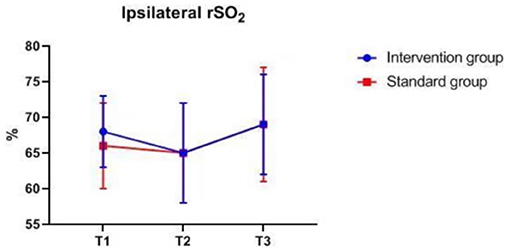
Figure 4. Ipsilateral rSO2 over time. rSO2, regional cerebral oxygenation. The measurement time interval after general anesthesia but before clamping the carotid artery was recorded as baseline reference T1, the time interval after clamping but before declamping was recorded as T2, and the time interval after the declamping and stabilization of cerebral perfusion but before completion of surgery was recorded as T3.
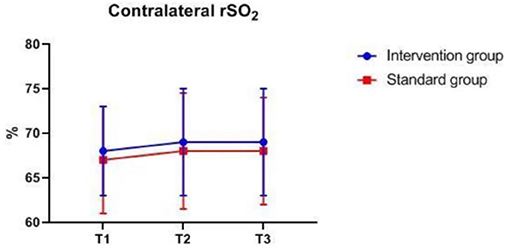
Figure 5. Contralateral rSO2 over time. rSO2, regional cerebral oxygenation. The measurement time interval after general anesthesia but before clamping the carotid artery was recorded as baseline reference T1, the time interval after clamping but before declamping was recorded as T2, and the time interval after the declamping and stabilization of cerebral perfusion but before completion of surgery was recorded as T3.
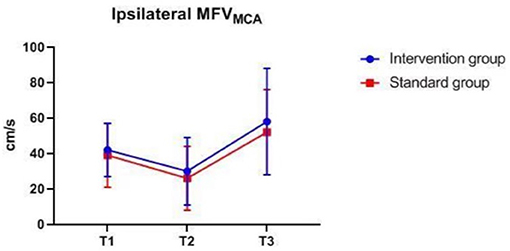
Figure 6. Ipsilateral MFVMAC over time. MFVMAC, mean flow velocity of middle cerebral artery. The measurement time interval after general anesthesia but before clamping the carotid artery was recorded as baseline reference T1, the time interval after clamping but before declamping was recorded as T2, and the time interval after the declamping and stabilization of cerebral perfusion but before completion of surgery was recorded as T3. MFVMAC, bilateral mean flow velocity of middle cerebral artery.
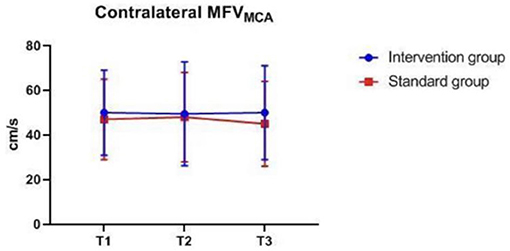
Figure 7. Contralateral MFVMAC over time. MFVMAC, mean flow velocity of middle cerebral artery. The measurement time interval after general anesthesia but before clamping the carotid artery was recorded as baseline reference T1, the time interval after clamping but before declamping was recorded as T2, and the time interval after the declamping and stabilization of cerebral perfusion but before completion of surgery was recorded as T3. MFVMAC, bilateral mean flow velocity of middle cerebral artery.
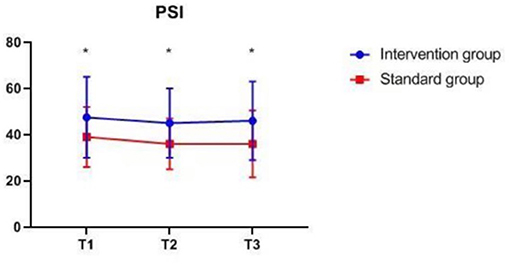
Figure 8. PSI at corresponding time points. PSI, patient state index, *P < 0.05 from standard group (statistically significant). The measurement time interval after general anesthesia but before clamping the carotid artery was recorded as baseline reference T1, the time interval after clamping but before declamping was recorded as T2, and the time interval after the declamping and stabilization of cerebral perfusion but before completion of surgery was recorded as T3.
Discussion
We demonstrated that in patients undergoing CEA, processed EEG-guided anesthesia management with PSI combined with DSA reduces the incidence of POD compared to using PSI alone. Based on previous studies on POD (11, 14, 17–19), we suggest that not only the index from a processed EEG but also an index with additional values (in this case, DSA) to guide anesthesia management protocol may improve outcomes among CEA patients.
The SEDLine monitor provides computed quantitative EEG indices based on retrospective analysis of a diagnostic EEG database of sedated patients. Since regulatory approval in 2002, these indices have been shown to independently predict deep sedation as assessed by other clinical metrics such as the Ramsay Sedation Score and Modified Observer's Assessment of Alertness/Sedation Scale (52, 63–65). Our findings of reduced POD incidence using processed EEG-guided anesthesia management are in accord with previous studies on other surgical populations (27–29, 66, 67). In contrast, the Electroencephalography Guidance of Anesthesia (ENGAGES) trial of older adults undergoing cardiac or non-cardiac surgery found no reduction in delirium incidence using this EEG-based intervention (68). Possible explanations for this discrepancy include differences in baseline conditions between cohorts and the unique effects of cardiopulmonary bypass on the cerebrum. A comprehensive description of predisposing factors is critical for investigations on POD, especially those including patients with multiple etiologies. Leung and colleagues recently reported the results of their clinical trial in older adults undergoing non-cardiac surgery, and reported similar rates of postoperative delirium between patients randomized to receive EEG-guided anesthetic management vs. those without the monitor (66). Unlike our study, most of the patients included in this study were undergoing spinal surgery, and the surgical process had little effect on the cerebral circulation. In contrast to the two trials, our patient groups were well-matched demographically and clinically at baseline, and received similar surgical procedures with comparable impacts on cerebral circulation, permitting similar anesthesia management (except for the EEG-based intervention). Another possible explanation for this discrepancy is that anesthesia requirements may be highly patient-specific due to individual sensitivity to anesthetic depth (69). EEG suppression is also a function of cerebral perfusion. The patients recruited in our study all had severe carotid artery stenosis and exhibited baseline characteristics predictive of greater sensitivity to anesthesia depth and POD compared to the two cohort. Thus, patient vulnerability is an important consideration when identifying patients most suitable for processed EEG monitoring. A slight change in anesthetic management may play a major role in modulating postoperative delirium risk in patients with cerebral ischemia (or patients at a risk of cerebral ischemia).
In this study, both groups of patients used the index from a processed EEG, and the intervention group combined the index with additional values (in this case the DSA) to guide anesthesia. It was observed that using the PSI alone did not result in as good outcomes and BS avoidance, possibly due to underestimation of EEG suppression using the monitoring (56). Patients may have benefited from BSR and PSI monitoring, visual tracking of the EEG, and the individualized real-time feedback of an anesthetic cerebral state provided by the EEG spectrogram or raw waveform patterns (70–72), thereby achieving superior anesthesia depth monitoring and reducing POD risk. Anesthesiologists may be able to manage patients' anesthetic cerebral state better by monitoring multiple EEG parameters. The occurrence of EEG burst suppression during maintenance may be predictive of POD (73). Lower EEG suppression duration may have directly contributed to the reduced POD incidence in the intervention group; hence, vulnerable patients may have derived a greater benefit. The PSI of the intervention group is higher than that of the standard care group, however, if only a single PSI index is observed and maintained at a high level, EEG suppression may not be minimized, which may not be enough to significantly reduce the risk of POD.
The EEG suppression is also a function of cerebral perfusion, in other words, the lower the perfusion, the more suppressed the EEG. Our patients had severe carotid artery stenosis and relatively insufficient cerebral perfusion. The severe fluctuations of cerebral blood flow (CBF) during CEA may have further aggravated hypoperfusion or led to cerebral hyperperfusion. In our study, the combined monitoring of TCD and NIRS may minimize the influence of the patient's hemodynamics on cerebral perfusion. However, the largest changes in CBF occurred during S2 and S3, and the duration of EEG suppression was significantly lower during these stages in the intervention group. In the intervention group, while adjusting the anesthesia dose to reduce EEG burst suppression, we also paid close attention to the effect on CBF. When adjusting the anesthetic dose, burst suppression could not be minimized if CBF changes were unfavorable. Therefore, even if the TCD and NIRS monitors did not show hypoperfusion or hyperperfusion, we still slightly regulated blood pressure according to the surgical stage. Although we observed no difference in MAP at each time point between groups, small adjustments may have slightly improved CBF, reduce EEG suppression, and thereby reduce POD.
Anesthetic dosage can be reduced using processed EEG (27, 74), and previous studies have shown potentially neurotoxic effects of general anesthetics, including propofol (75, 76). Therefore, it is conceivable that POD risk was reduced by lower anesthetic levels. However, the present results do not support this premise, at least for the anesthetic doses used and for this specific population. All patients had severe CS and were prone to vascular events which had been identified as one of the postoperative risk factors for the development of delirium. In addition to adjusting the anesthetic dose during the perioperative period, we also adjusted blood pressure according to the operation stage to avoid potential cerebral perfusion problems that may lead to burst suppression. Moreover, we conducted multimodal monitoring on all patients, which helped us obtain more comprehensive information to identify the extent of individual cerebral perfusion. We adjusted the physiological functions of patients according to anesthetics/techniques and surgical procedures, and strictly controlled arterial blood pressure (77) to avoid insufficient or excessive cerebral perfusion risk related to POD. All the monitoring we used is non-invasive and has no significant risk of injury to patient. Therefore, we conclude that patients with carotid artery stenosis can benefit from multimodal monitoring during surgery to reduce the onset of EEG suppression.
The difference in MAP, PETCO2, ipsilateral MFVMCA, and bilateral rSO2 at all time-points is due to differences in blood pressure management measures taken at different surgical stages to ensure that the cerebral perfusion is within desired limits. Despite the difference in contralateral rSO2, there was no difference in MFVMCA, likely because TCD monitoring reflects MFVMCA, while NIRS monitors the oxygenation of the frontal cortex mainly supplied by the anterior communicating artery (ACA). Since the contralateral MFVMCA is not affected by the surgery, there is no difference at all time-points. The NIRS monitoring value may have been influenced by oxygen metabolism of extracranial origin as well as by changes in blood pressure and arterial oxygen saturation. The differences in PSI within group at corresponding time-points are likely because the largest changes in CBF during clamping and declamping of the carotid artery may also affect EEG, leading to changes in PSI.
Potential Limitations
The study has several limitations. First, as a single-center trial, the generalizability of the results may be limited. Second, postoperative delirium has no objective biomarker and so may be difficult to diagnose in certain cases (78). Third, POD was assessed only over 3 days, so transient and later incidences could have been missed. However, the preponderance of evidence suggests that most cases occur within the first 3 days after anesthesia and surgery (14, 79). Fourth, the anesthesiologists were not blinded to group assignment; therefore, when caring for patients in the intervention group, they might have been more cautious in the management of the patient's overall hemodynamic parameters. However, the following measures were adopted to avoid bias. The combined monitoring of TCD and NIRS may minimize the influence of the patient's hemodynamics on cerebral perfusion. There was no difference in MAP, bilateral MFVMCA and rSO2 at different time-points between the two groups. The anesthetists did not participate in postoperative follow-up, and the investigators responsible for postoperative follow-up and delirium assessments were blinded to group assignment and did not participate in anesthesia or perioperative care. The anesthetists and investigators also did not communicate patient information. Fifth, these findings may not apply to patients receiving inhalational anesthetics.
Summary
This randomized clinical trial suggests that electroencephalography-guided anesthesia management with both a quantitative EEG index and DSA can reduce postoperative delirium incidence in patients undergoing CEA, especially those vulnerable to disruption of cerebral perfusion and EEG suppression. A slight change in anesthetic management may play a significant role in modulating postoperative delirium risk in patients with ischemic cerebral disease (or patients at risk of cerebral ischemia). In turn, reducing POD incidence may improve outcome.
Data Availability Statement
The original contributions presented in the study are included in the article/supplementary material, further inquiries can be directed to the corresponding author/s.
Ethics Statement
The studies involving human participants were reviewed and approved by the Ethics Committee of Xuanwu Hospital of Capital Medical University (LYS[2018]053). The patients/participants provided their written informed consent to participate in this study.
Author Contributions
NX was responsible for the implementation of the project, patient recruitment, patient interviews, data collection, data entry, statistical analyses, and article writing. L-XL, D-XY, JW, Y-HM, TT, and X-LS performed patient recruitment, patient interviews, and data collection. L-QJ and YH reviewed and edited the final manuscript. T-LW managed the project and provided funding. All authors contributed to manuscript revision, read, and approved the submitted version.
Funding
This work was supported by Beijing Municipal Administration of Hospitals Clinical Medicine Development of Special Funding Support (ZYLX201818), Beijing Municipal Health Commission (Jing2019-2), and Xuanwu Hospital Funding Support (XWJL-2018017) of Capital Medical University.
Conflict of Interest
The authors declare that the research was conducted in the absence of any commercial or financial relationships that could be construed as a potential conflict of interest.
Acknowledgments
The authors sincerely thank all colleagues in the Departments of Neurosurgery, Vascular Ultrasound, and Anesthesiology at Xuanwu Hospital of Capital Medical University, Beijing, for their cooperation. The authors wish to express special appreciation to Ying Han (Department of Neurology, Xuanwu Hospital, Capital Medical University, Beijing) for neuropsychological consultation and personnel training.
Abbreviations
CEA, carotid endarterectomy; EEG, electroencephalogram; TCD, transcranial Doppler ultrasound; NIRS, near-infrared spectroscopy; CS, carotid stenosis; POD, postoperative delirium; CAM, Confusion Assessment Method; MMSE, Mini-Mental State Examination; MoCA, Montreal Cognitive Assessment; SDS, Self-rating Depression Scale; SAS, Self-rating Anxiety Scale; ASA, American Society of Anesthesiologists; rSO2, regional cerebral oxygenation; MFVMCA, mean flow velocity of the middle cerebral artery; BSR, burst suppression ratio; DSA, density spectral array; PSI, patient state index; MAP, mean arterial blood pressure; PETCO2, end-expiratory carbon dioxide partial pressure; SD, standard deviation; IQR, interquartile range; OR, odds ratio; ACA, anterior communicating artery; CBF, cerebral blood flow; CAS, carotid artery stenting; EMG, electromyogram.
References
1. Malcharek MJ, Ulkatan S, Marinò V, Geyer M, Lladò-Carbò E, Perez-Fajardo G, et al. Intraoperative monitoring of carotid endarterectomy by transcranial motor evoked potential: a multicenter study of 600 patients. Clin Neurophysiol. (2013) 124:1025–30. doi: 10.1016/j.clinph.2012.10.014
2. Pennekamp CW, Moll FL, de Borst GJ. The potential benefits and the role of cerebral monitoring in carotid endarterectomy. Curr Opin Anaesthesiol. (2011) 24:693–7. doi: 10.1097/ACO.0b013e32834c7aa1
3. Pulli R, Dorigo W, Barbanti E, Azas L, Russo D, Matticari S, et al. Carotid endarterectomy with contralateral carotid artery occlusion: is this a higher risk subgroup? Eur J Vasc Endovasc Surg. (2002) 24:63–8. doi: 10.1053/ejvs.2002.1612
4. Brott TG, Halperin JL, Abbara S, Bacharach JM, Barr JD, Bush RL, et al. 2011 ASA/ACCF/AHA/AANN/AANS/ACR/ASNR/CNS/SAIP/SCAI/SIR/SNIS/ SVM/SVS guideline on the management of patients with extracranial carotid and vertebral artery disease: executive summary. Stroke. (2011) 42:e420–63. doi: 10.1161/STR.0b013e3182112d08
5. North American Symptomatic Carotid Endarterectomy Trial Collaborators, Barnett HJM, Taylor DW, Haynes RB, Sackett DL, Peerless SJ, et al. Beneficial effect of carotid endarterectomy in symptomatic patients with high-grade carotid stenosis. N Engl J Med. (1991) 325:445–53. doi: 10.1056/NEJM199108153250701
6. Endarterectomy for asymptomatic carotid artery stenosis. Executive committee for the asymptomatic carotid atherosclerosis study. JAMA. (1995) 273:1421–8.
7. Randomised trial of endarterectomy for recently symptomatic carotid stenosis: final results of the MRC European Carotid Surgery Trial (ECST). Lancet. (1998) 351:1379–87.
8. Barnett HJ, Taylor DW, Eliasziw M, Fox AJ, Ferguson GG, Haynes RB, et al. Benefit of carotid endarterectomy in patients with symptomatic moderate or severe stenosis. North American symptomatic carotid endarterectomy trial collaborators. N Engl J Med. (1998) 339:1415–25. doi: 10.1056/NEJM199811123392002
9. Monk TG, Weldon BC, Garvan CW, Dede DE, van der Aa MT, Heilman KM, et al. Predictors of cognitive dysfunction after major non-cardiac surgery. Anesthesiology. (2008) 108:18–30. doi: 10.1097/01.anes.0000296071.19434.1e
10. Miyazaki S, Yoshitani K, Miura N, Irie T, Inatomi Y, Ohnishi Y, et al. Risk factors of stroke and delirium after off-pump coronary artery bypass surgery. Interactive Cardiovasc Thorac Surg. (2011) 12:379–83. doi: 10.1510/icvts.2010.248872
11. Inouye SK. The dilemma of delirium: clinical and research controversies regarding diagnosis and evaluation of delirium in hospitalized elderly medical patients. Am J Med. (1994) 97:278–88. doi: 10.1016/0002-9343(94)90011-6
13. Lipowski ZL. Delirium in the elderly patient. N Engl J Med. (1989) 320:578–82. doi: 10.1056/NEJM198903023200907
14. Khadka J, McAlinden C, Pesudovs K. Cognitive trajectories after postoperative delirium. N Engl J Med. (2012) 367:30–9. doi: 10.1056/NEJMoa1112923
15. Inouye SK, Westendorp RG, Saczynski JS. Delirium in elderly people. Lancet. (2014) 383:911–22. doi: 10.1016/S0140-6736(13)60688-1
16. Bin Abd Razak HR, Yung WY. Postoperative delirium in patients undergoing total joint arthroplasty: a systematic review. J Arthroplasty. (2015) 30:1414–7. doi: 10.1016/j.arth.2015.03.012
17. Salluh JI, Wang H, Schneider EB, Nagaraja N, Yenokyan G, Damluji A, et al. Outcome of delirium in critically ill patients: systematic review and meta-analysis. BMJ. (2015) 350:h2538. doi: 10.1136/bmj.h2538
18. Crocker E, Beggs T, Hassan A, Denault A, Lamarche Y, Bagshaw S, et al. Long-term effects of postoperative delirium in patients undergoing cardiac operation: a systematic review. Ann Thoracic Surg. (2016) 102:1391–9. doi: 10.1016/j.athoracsur.2016.04.071
19. Mashour GA, Woodrum DT, Avidan MS. Neurological complications of surgery and anaesthesia. Br J Anaesthesia. (2015) 114:194–203. doi: 10.1093/bja/aeu296
20. American Society of Anesthesiologists. Perioperative Brain Health Initiative Website. (2018). Available online at: https://www.asahq.org/brainhealthinitiative (accessed September 16, 2018).
21. Mohanty S, Rosenthal RA, Russell MM, Neuman MD, Ko CY, Esnaola NF. Optimal perioperative management of the geriatric patient: a best practices guideline from the American College of Surgeons NSQIP and the American Geriatrics Society. J Am College Surgeons. (2016) 222:930–47. doi: 10.1016/j.jamcollsurg.2015.12.026
22. O'Mahony R, Murthy L, Akunne A, Young J. Synopsis of the National Institute for Health and Clinical Excellence guideline for prevention of delirium. Ann Internal Med. (2011) 154:746–51. doi: 10.7326/0003-4819-154-11-201106070-00006
23. National Institute for Health and Care Excellence. Delirium in Adults. London: National Institute for Health and Care Excellence (2014).
24. Inouye SK, Charpentier PA. Precipitating factors for delirium in hospitalized elderly persons. Predictive model and interrelationship with baseline vulnerability. JAMA. (1996) 275:852–7.
25. Fritz BA, Kalarickal PL, Maybrier HR, Muench MR, Dearth D, Chen Y, et al. Intraoperative electroencephalogram suppression predicts postoperative delirium. Anesthesia Analgesia. (2016) 122:234–42. doi: 10.1213/ANE.0000000000000989
26. Soehle M, Dittmann A, Ellerkmann RK, Baumgarten G, Putensen C, Guenther U. Intraoperative burst suppression is associated with postoperative delirium following cardiac surgery: a prospective, observational study. BMC Anesthesiol. (2015) 15:61. doi: 10.1186/s12871-015-0051-7
27. Chan MT, Cheng BC, Lee TM, Gin T. BIS-guided anesthesia decreases postoperative delirium and cognitive decline. J Neurosurg Anesthesiol. (2013) 25:33–42. doi: 10.1097/ANA.0b013e3182712fba
28. Radtke FM, Franck M, Lendner J, Krüger S, Wernecke KD, Spies CD. Monitoring depth of anaesthesia in a randomized trial decreases the rate of postoperative delirium but not postoperative cognitive dysfunction. Br J Anaesthesia. (2013) 1:i98–105. doi: 10.1093/bja/aet055
29. MacKenzie KK, Britt-Spells AM, Sands LP, Leung JM. Processed electroencephalogram monitoring and postoperative delirium: a systematic review and meta-analysis. Anesthesiology. (2018) 129:417–27. doi: 10.1097/ALN.0000000000002323
30. Sieber FE, Zakriya KJ, Gottschalk A, Blute MR, Lee HB, Rosenberg PB, et al. Sedation depth during spinal anesthesia and the development of postoperative delirium in elderly patients undergoing hip fracture repair. Mayo Clin Proc. (2010) 85:18–26. doi: 10.4065/mcp.2009.0469
31. Whitlock EL, Torres BA, Lin N, Helsten DL, Nadelson MR, Mashour GA, et al. Postoperative delirium in a substudy of cardiothoracic surgical patients in the BAG-RECALL clinical trial. Anesthesia Analgesia. (2014) 118:809–17. doi: 10.1213/ANE.0000000000000028
32. Seder DB, Fraser GL, Robbins T, Libby L, Riker RR. The bispectral index and suppression ratio are very early predictors of neurological outcome during therapeutic hypothermia after cardiac arrest. Inten Care Med. (2010) 36:281–8. doi: 10.1007/s00134-009-1691-1
33. Umegaki N, Hirota K, Kitayama M, Yatsu Y, Ishihara H, Mtasuki A. A marked decrease in bispectral index with elevation of suppression ratio by cervical haematoma reducing cerebral perfusion pressure. J Clin Neurosci. (2003) 10:694–6. doi: 10.1016/j.jocn.2002.11.001
34. Inouye SK, van Dyck CH, Alessi CA, Balkin S, Siegal AP, et al. Clarifying confusion: the confusion assessment method. A new method for detection of delirium. Ann Internal Med. (1990) 113:941–8. doi: 10.7326/0003-4819-113-12-941
35. Katzman R, Zhang MY, Ouang-Ya-Qu N, Wang ZY, Liu WT, Yu E, et al. A Chinese version of the Mini-Mental State Examination; impact of illiteracy in a Shanghai dementia survey. J Clin Epidemiol. (1988) 41:971–8. doi: 10.1016/0895-4356(88)90034-0
36. Roalf DR, Moberg PJ, Xie SX, Wolk DA, Moelter ST, Arnold SE. The Montreal Cognitive Assessment, MoCA: a brief screening tool for mild cognitive impairment. J Am Geriatr Soc. (2005) 53:695–9. doi: 10.1111/j.1532-5415.2005.53221.x
37. Zung WW. A self-rating depression scale. Arch Gene Psychiatry. (1965) 12:63–70. doi: 10.1001/archpsyc.1965.01720310065008
38. Zung WW. A rating instrument for anxiety disorders. Psychosomatics. (1971) 12:371–9. doi: 10.1016/S0033-3182(71)71479-0
39. American Society of Anesthesiologists. New classification of physical status. Anesthesiology. (1963) 24:111.
40. Collin C, Wade DT, Davies S, Horne V. The Barthel ADL index: a reliability study. Int Disabil Stud. (1988) 10:61–3. doi: 10.3109/09638288809164103
41. Rudolph JL, Marcantonio ER. Review articles: postoperative delirium: acute change with long-term implications. Anesthesia Analgesia. (2011) 112:1202–11. doi: 10.1213/ANE.0b013e3182147f6d
42. Kazmierski J, Kowman M, Banach M, Fendler W, Okonski P, Banys A, et al. Incidence and predictors of delirium after cardiac surgery: Results from The IPDACS Study. J Psychosomat Res. (2010) 69:179–85. doi: 10.1016/j.jpsychores.2010.02.009
43. Leung JM. Postoperative delirium: are there modifiable risk factors? Eur J Anaesthesiol. (2010) 27:403–5. doi: 10.1097/EJA.0b013e3283340a99
44. Wada S, Inoguchi H, Sadahiro R, Matsuoka YJ, Uchitomi Y, Sato T, et al. Preoperative anxiety as a predictor of delirium in cancer patients: a prospective observational cohort study. World J Surg. (2019) 43:134–42. doi: 10.1007/s00268-018-4761-0
45. Wang J, Li Z, Yu Y, Li B, Shao G, Wang Q. Risk factors contributing to postoperative delirium in geriatric patients postorthopedic surgery. Asia-Pacific Psychiatry. (2015) 7:375–82. doi: 10.1111/appy.12193
46. Siepe M, Pfeiffer T, Gieringer A, Zemann S, Benk C, Schlensak C, et al. Increased systemic perfusion pressure during cardiopulmonary bypass is associated with less early postoperative cognitive dysfunction and delirium. Eur J Cardio-Thoracic Surg. (2011) 40:200–7. doi: 10.1016/j.ejcts.2010.11.024
47. Samra SK, Dy EA, Welch K, Dorje P, Zelenock GB, Stanley JC. Evaluation of a cerebral oximeter as a monitor of cerebral ischemia during carotid endarterectomy. Anesthesiology. (2000) 93:964–70. doi: 10.1097/00000542-200010000-00015
48. Rowed DW, Houlden DA, Burkholder LM, Taylor AB. Comparison of monitoring techniques for intraoperative cerebral ischemia. Can J Neurol Sci. (2004) 31:347–56. doi: 10.1017/s0317167100003437
49. Grubhofer G, Plöchl W, Skolka M, Czerny M, Ehrlich M, Lassnigg A. Comparing Doppler ultrasonography and cerebral oximetry as indicators for shunting in carotid endarterectomy. Anesthesia Analgesia. (2000) 91:1339–44. doi: 10.1097/00000539-200012000-00006
50. Ali AM, Green D, Zayed H, Halawa M, El-Sakka K, Rashid HI. Cerebral monitoring in patients undergoing carotid endarterectomy using a triple assessment technique. Interact Cardiovasc Thoracic Surg. (2011) 12:454–7. doi: 10.1510/icvts.2010.235598
51. Sundt TM Jr, Sharbrough FW, Anderson RE, Michenfelder JD. Correlation of cerebral blood flow and electroencephalographic changes during carotid endarterectomy: with results of surgery and hemodynamics of cerebral ischemia. Mayo Clin Proc. (1981) 56:533–43.
52. Drover D, Ortega HR. Patient state index. Best practice & research. Clin Anaesthesiol. (2006) 20:121–8. doi: 10.1016/j.bpa.2005.07.008
53. Ching S, Purdon PL, Vijayan S, Kopell NJ, Brown EN. A neurophysiological-metabolic model for burst suppression. Proc Natl Acad Sci USA. (2012) 109:3095–100. doi: 10.1073/pnas.1121461109
54. Lewis LD, Ching S, Weiner VS, Peterfreund RA, Eskandar EN, Cash SS, et al. Local cortical dynamics of burst suppression in the anaesthetized brain. Brain. (2013) 136:2727–37 doi: 10.1093/brain/awt174
55. Leung J.l, Leung V. c., Leung CM, Pan PC. Clinical utility and validation of two instruments (the Confusion Assessment Method Algorithm and the Chinese version of Nursing Delirium Screening Scale) to detect delirium in geriatric inpatients. Gene Hospital Psychiatry. (2008) 30:171–6. doi: 10.1016/j.genhosppsych.2007.12.007
56. Muhlhofer WG, Zak R, Kamal T, Rizvi B, Sands LP, Yuan M, et al. Burst-suppression ratio underestimates absolute duration of electroencephalogram suppression compared with visual analysis of intraoperative electroencephalogram. Br J Anaesthesia. (2017) 118:755–61. doi: 10.1093/bja/aex054
57. Bokeriia LA, Golukhova EZ, Polunina AG. Postoperative delirium in cardiac operations: microembolic load is an important factor. Ann Thoracic Surg. (2009) 88:349–50. doi: 10.1016/j.athoracsur.2009.02.031
58. Donald W, Reza M. Intraoperative cerebral high-intensity transient signals and postoperative cognitive function: a systematic review. Am J Surg. (2009) 198:295–7. doi: 10.1016/j.amjsurg.2008.11.019
59. Flach HZ, Ouhlous M, Hendriks JM, Van Sambeek MR, Veenland JF, Koudstaal PJ, et al. Cerebral ischemia after carotid intervention. J Endovasc Ther. (2004) 11:251–7. doi: 10.1583/03-1128.1
60. Henry M, Amor M, Masson I, Henry I, Tzvetanov K, Chati Z, et al. Ischemic cerebral lesions after carotid surgery and carotid stenting. Eur J Vasc Endovasc Surg. (2007) 33:430–5. doi: 10.1016/j.ejvs.2006.11.012
61. Bonati LH, Jongen LM, Haller S, Flach HZ, Dobson J, Nederkoorn PJ, et al. New ischaemic brain lesions on MRI after stenting or endarterectomy for symptomatic carotid stenosis: a substudy of the International Carotid Stenting Study (ICSS). Neurology. (2010) 9:353–62. doi: 10.1016/S1474-4422(10)70057-0
62. Schnaudigel S, Gröschel K, Pilgram SM, Kastrup A. New brain lesions after carotid stenting versus carotid endarterectomy: a systematic review of the literature. Stroke. (2008) 39:1911–9. doi: 10.1161/STROKEAHA.107.500603
63. Soehle M, Ellerkmann RK, Grube M, Kuech M, Wirz S, Hoeft A, et al. Comparison between bispectral index and patient state index as measures of the electroencephalographic effects of sevoflurane. Anesthesiology. (2008) 109:799–805. doi: 10.1097/ALN.0b013e3181895fd0
64. Schneider G, Heglmeier S, Schneider J, Tempel G, Kochs EF. Patient State Index (PSI) measures depth of sedation in intensive care patients. Inten Care Med. (2004) 30:213–6. doi: 10.1007/s00134-003-2092-5
65. Soehle M, Kuech M, Grube M, Wirz S, Kreuer S, Hoeft A, et al. Patient state index vs bispectral index as measures of the electroencephalographic effects of propofol. Br J Anaesthesia. (2010) 105:172–8. doi: 10.1093/bja/aeq155
66. Tang CJ, Jin Z, Sands LP, Pleasants D, Tabatabai S, Hong Y, et al. ADAPT-2: A randomized clinical trial to reduce intraoperative EEG suppression in older surgical patients undergoing major noncardiac surgery. Anesthesia Analgesia. (2020) 131:1228–36. doi: 10.1213/ANE.0000000000004713
67. Punjasawadwong Y, Chau-In W, Laopaiboon M, Punjasawadwong S, Pin-On P. Processed electroencephalogram and evoked potential techniques for amelioration of postoperative delirium and cognitive dysfunction following non-cardiac and non-neurosurgical procedures in adults. Cochrane Datab Systematic Rev. (2018) 5:CD011283. doi: 10.1002/14651858.CD011283.pub2
68. Wildes TS, Mickle AM, Ben Abdallah A, Maybrier HR, Oberhaus J, Budelier TP, et al. Effect of electroencephalography-guided anesthetic administration on postoperative delirium among older adults undergoing major surgery: the ENGAGES randomized clinical trial. JAMA. (2019) 321:473–483. doi: 10.1001/jama.2018.22005
69. Iwakiri H, Nishihara N, Nagata O, Matsukawa T, Ozaki M, Sessler DI. Individual effect-site concentrations of propofol are similar at loss of consciousness and at awakening. Anesthesia Analgesia. (2005) 100:107–10. doi: 10.1213/01.ANE.0000139358.15909.EA
70. Purdon PL, Pavone KJ, Akeju O, Smith AC, Sampson AL, Lee J, et al. The ageing brain: Age-dependent changes in the electroencephalogram during propofol and sevoflurane general anaesthesia. Br J Anaesthesia. (2015) 115(Suppl. 1):i46–57. doi: 10.1093/bja/aev213
71. Purdon PL, Zhou DW, Akeju O, Brown EN. In reply. Anesthesiology. (2015) 123:725–8. doi: 10.1097/ALN.0000000000000794
72. Purdon PL, Sampson A, Pavone KJ, Brown EN. Clinical electroencephalography for anesthesiologists: part i: background and basic signatures. Anesthesiology. (2015) 123:937–60. doi: 10.1097/ALN.0000000000000841
73. Hesse S, Kreuzer M, Hight D, Gaskell A, Devari P, Singh D, et al. Association of electroencephalogram trajectories during emergence from anaesthesia with delirium in the postanaesthesia care unit: an early sign of postoperative complications. Br J Anaesthesia. (2019) 122:622–34. doi: 10.1016/j.bja.2018.09.016
74. Luo C, Zou W. Cerebral monitoring of anaesthesia on reducing cognitive dysfunction and postoperative delirium: a systematic review. J Int Med Res. (2018) 46:4100–10. doi: 10.1177/0300060518786406
75. Berger M, Nadler JW, Friedman A, McDonagh DL, Bennett ER, Cooter M, et al. The effect of propofol versus isoflurane anesthesia on human cerebrospinal fluid markers of Alzheimer's disease: results of a randomized trial. J Alzheimer's Dis. (2016) 52:1299–310. doi: 10.3233/JAD-151190
76. Tang JX, Baranov D, Hammond M, Shaw LM, Eckenhoff MF, Eckenhoff RG. Human Alzheimer and inflammation biomarkers after anesthesia and surgery. Anesthesiology. (2011) 115:727–32. doi: 10.1097/ALN.0b013e31822e9306
77. Joshi B, Ono M, Brown C, Brady K, Easley RB, Yenokyan G, et al. Predicting the limits of cerebral autoregulation during cardiopulmonary bypass. Anesthesia Analgesia. (2012) 114:503–10. doi: 10.1213/ANE.0b013e31823d292a
78. Li T, Wieland LS, Oh E, Neufeld KJ, Wang NY, Dickersin K, et al. Design considerations of a randomized controlled trial of sedation level during hip fracture repair surgery: a strategy to reduce the incidence of postoperative delirium in elderly patients. Clin Trials. (2017) 14:299–307. doi: 10.1177/1740774516687253
Keywords: delirium, carotid endarterectomy, electroencephalogram-guided, general anesthesia, cerebral perfusion, monitoring
Citation: Xu N, Li L-X, Wang T-L, Jiao L-Q, Hua Y, Yao D-X, Wu J, Ma Y-H, Tian T and Sun X-L (2021) Processed Multiparameter Electroencephalogram-Guided General Anesthesia Management Can Reduce Postoperative Delirium Following Carotid Endarterectomy: A Randomized Clinical Trial. Front. Neurol. 12:666814. doi: 10.3389/fneur.2021.666814
Received: 16 February 2021; Accepted: 09 June 2021;
Published: 12 July 2021.
Edited by:
Jacqueline Leung, University of California, San Francisco, United StatesReviewed by:
Wolfgang Muhlhofer, University of Alabama at Birmingham, United StatesRochelle Zak, University of California, San Francisco, United States
Copyright © 2021 Xu, Li, Wang, Jiao, Hua, Yao, Wu, Ma, Tian and Sun. This is an open-access article distributed under the terms of the Creative Commons Attribution License (CC BY). The use, distribution or reproduction in other forums is permitted, provided the original author(s) and the copyright owner(s) are credited and that the original publication in this journal is cited, in accordance with accepted academic practice. No use, distribution or reproduction is permitted which does not comply with these terms.
*Correspondence: Tian-Long Wang, d190bDU1OTVAaG90bWFpbC5jb20=