- 1Department of Physical Therapy, College of Health Sciences, Dankook University, Cheonan, South Korea
- 2Department of Physical Medicine and Rehabilitation, College of Medicine, Yeungnam University, Daegu, South Korea
- 3Department of Health, Graduate School, Dankook University, Cheonan, South Korea
- 4Department of Physical Therapy, Yeungnam University College, Daegu, South Korea
Background and Purpose: Parieto-insular vestibular cortex (PIVC) injury can cause symptoms such as abnormal gait and affects the integration and processing of sensory inputs contributing to self-motion perception. Therefore, this study investigated the association of the vestibular pathway in the gait and motor function recovery process in patients with PIVC injury using diffusion tensor imaging (DTI).
Methods: We recruited 28 patients with stroke with only PIVC injury and reconstructed the PIVC using a 1.5-T scanner for DTI. Fractional anisotropy (FA), mean diffusivity (MD), and tract volume were measured. The functional ambulatory category (FAC) test was conducted, and motricity index (MI) score was determined. These were conducted and determined at the start (phase 1), end of rehabilitation (phase 2), and during the follow-up 6 months after onset.
Results: Although the tract volume of PIVC showed a decrease in subgroup A, all of DTI parameters were not different between two subgroups in affected side (p > 0.05). The results of MI and FAC were significantly different according to the recovery process (p < 0.05). In addition, FA of the PIVC showed a positive correlation with FAC in phase 2 of the recovery process on the affected side. On the unaffected side, FA of the PIVC showed a significant negative correlation with MI in all processes (p < 0.05).
Conclusion: The degree of projection pathways to PIVC injury at onset time seems to be related to early restoration of gait function. Moreover, we believe that early detection of the projection pathway for PIVC injury using DTI would be helpful in the clinical evaluation and prediction of the prognosis of patients with PIVC injury.
Introduction
The parieto-insular vestibular cortex (PIVC) is an ascending pathway that controls equilibrium in the human vestibular system (1, 2). The PIVC is located in the posterior parietal operculum/retroinsular region and extends into posterior sections (2–5). This area is the core region for integrating and processes vestibular and somatosensory information in the cerebral cortex when the head and body positions change. In particular, the PIVC is involved in the processing of self-motion perception, estimation of verticality, processing of visual motion, and motion coherent with gravitational vector (6, 7). Self-motion recognition is essential for monitoring body movements, especially balance and gait. Several studies have reported that PIVC injury can cause symptoms such as extremity weakness/obesity, imbalance, and abnormal gait. In addition, pathology of the PIVC affects the integration and processing of sensory inputs from the vestibular, visual, and somatosensory systems, contributing to self-motion perception (8, 9).
Although previous studies have reported that patients with PIVC injury have an impairment of motor function, no studies have reported an association between tract injury and recovery of motor function in PIVC. However, several studies reported on the relationship between the degree of damage to the corticospinal tract (CST) and the recovery of motor function (10–13). In 2016, Kumar et al. reported that the integrity of CST could predict the recovery process of upper limb motor function (13). In a previous study, diffusion tensor imaging (DTI) was used to assess the degree of CST injury and demonstrated a correlation between the motor impairment at each phase (acute: 3–7 days, subacute: 30 days, and chronic: 90 days) of ischemic stroke. The degree of tract damage and the motor National Institutes of Health Stroke Scale score showed a negative correlation for each phase, and DTI-derived CST assessment could become a marker of motor impairment level (10). Therefore, previous studies suggested that the degree of early tract injury could be used as an indicator to predict motor function recovery.
DTI quantifies diffusion in multiple directions and enables anatomical structures to be visualized using imaging water diffusion patterns (14, 15). Recently, studies have reconstructed PIVC in three dimensions, and several studies have reported on the location and function of the PIVC. However, no study has assessed the relationship between motor function associated with PIVC injury. Given the importance of the PIVC in gait and motor function, demonstrating the relationship of recovery following PIVC injury could provide useful information for the rehabilitation field.
In the current study, we investigated the association of the vestibular pathway with changes in the gait and motor function recovery process in patients with PIVC injury using DTI.
Materials and Methods
Subjects
Thirty-six patients with stroke (16 male, 20 female; mean age 63.03 ± 12.06 years) with only PIVC injury on magnetic resonance imaging were recruited for this study at Yeungnam University Hospital (Figure 1A). Inclusion criteria were as follows: (1) first ever stroke, (2) no brain injury due to traumatic injury, (3) PIVC injury due to infarction and hemorrhage, (4) hemiparesis at the time of DTI scanning, and (5) capable of undergoing the functional evaluation. Eight patients were excluded from this study because they were not capable of undergoing functional evaluation. The patients were classified into the following two subgroups based on the continuity of the projection pathway at PIVC injury level on DTI: subgroup A, 16 patients with a discontinuous projection pathway to the PIVC, and subgroup B, 12 patients with a continuous projection pathway to the PIVC at PIVC injury level. A summary of the demographic features according to subgroup A and subgroup B is presented in Table 1. All patients provided informed consent before undergoing DTI and the functional evaluation. The study was approved by the institutional review board of Dankook University (DKU 2020-07-009).
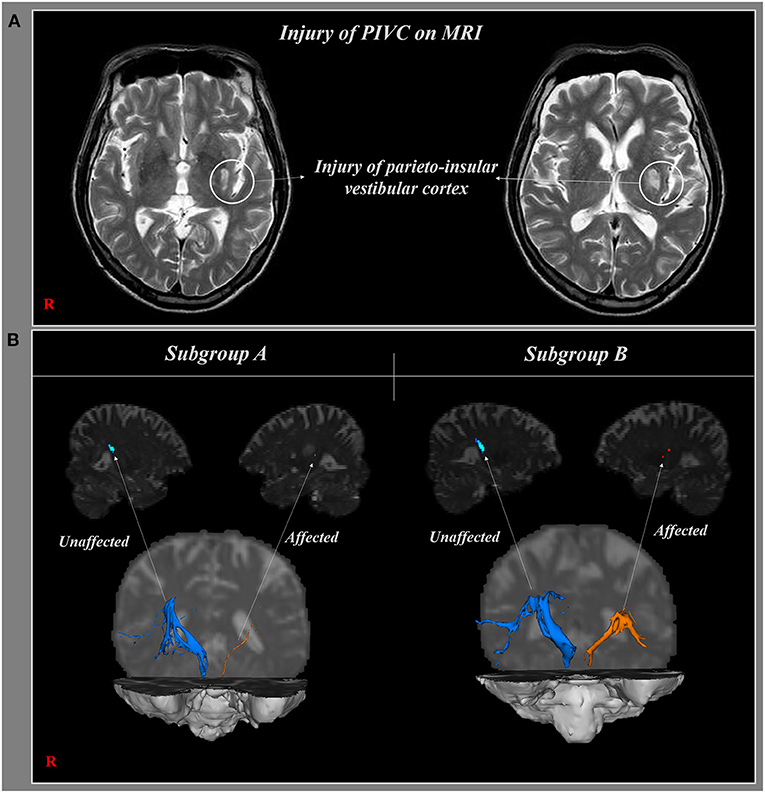
Figure 1. (A) Brain magnetic resonance imaging (MRI) shows injury of the projection pathway to parieto-insular vestibular cortex (PIVC). (B) Reconstructed projection pathways to PIVC in subgroup (A,B); the pathway was affected due to stroke (orange), and was not affected (blue).
Diffusion Tensor Image
Acquisition of DTI data was performed using a six-channel head coil on a 1.5 T Philips Gyro scan Intera (Philips, Best, The Netherlands) with single-shot echo-planar imaging. For each of the 32 non-collinear diffusion sensitizing gradients, 67 contiguous slices were collected parallel to the anterior commissure-posterior commissure line. The imaging parameters were as follows: acquisition matrix = 96 × 96; reconstructed matrix = 192 × 192; field of view = 240 × 240 mm2; TR = 10,726 ms; TE = 76 ms; parallel imaging reduction factor (SENSE factor) = 2; EPI factor = 49; b = 1,000 s/mm2; NEX = 1; and a slice thickness of 2.5 mm with no gap (acquired voxel size 1.3 × 1.3 × 2.5 mm3) (16, 17).
Probabilistic Fiber Tracking
The diffusion-weighted imaging data was analyzed using the Oxford Centre for Functional Magnetic Resonance Imaging of the Brain (FMRIB) Software Library (FSL; www.fmrib.ox.ac.uk/fsl). Affine multi-scale two-dimensional registration was used to correct the head motion effect and image distortion due to eddy current. Fiber tracking used a probabilistic image method based on a multifiber model, and performed in this study utilizing image routines implemented in FMRIB Diffusion (5,000 streamline samples, 0.5 mm step lengths, curvature thresholds = 0.2) (18).
The projection pathway to the core vestibular cortex was determined by selection of fibers passing through seed and two target regions of interest (ROI). The seed ROI and target ROI of the projection pathway to PIVC were determined as follows: seed ROI—the vestibular nuclei at the level of pons equivalent to Deiters' nuclei and Schwalbe's nuclei, and target ROI—two of the target ROI were placed on posterior parietal operculum and thalamus (Figure 2) (19). The 5,000 samples were generated from the seed voxel, and the results were visualized at the threshold of 1 streamline through each voxel for analysis. The values of fractional anisotropies (FA), mean diffusivities (MD), and tract volume (voxel number) of the projection pathway to PIVC were measured.
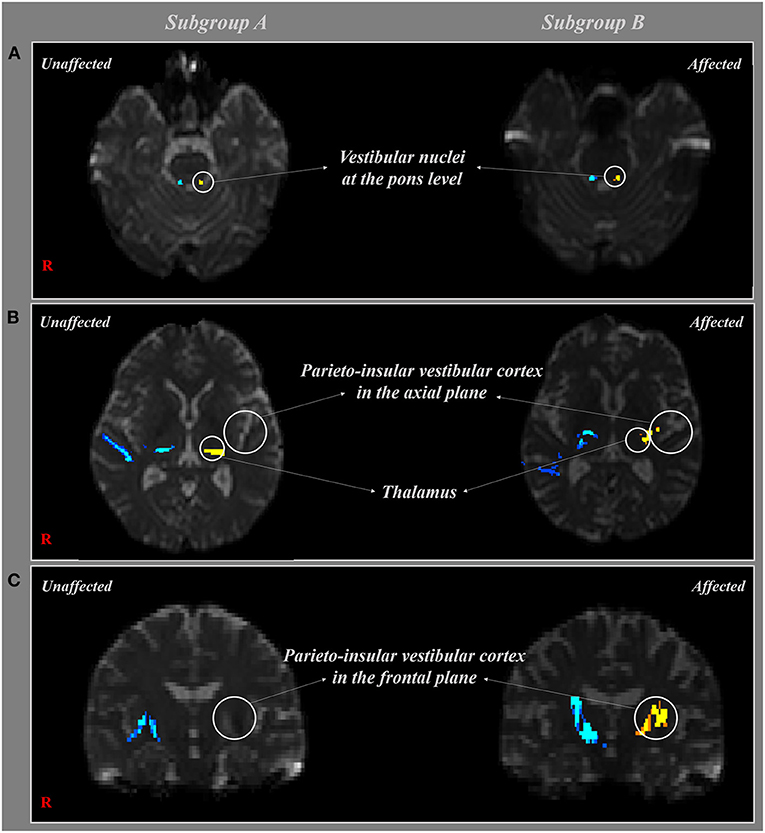
Figure 2. The projection pathway to parieto-insular vestibular cortex (PIVC) is shown at each level. The affected side (yellow and red) and unaffected side (blue) are shown in two subgroups according to the type of diffusion tensor imaging (DTI) tractography. (A) The projection pathway to PIVC that passes by the vestibular nuclei at the pons level, (B) thalamus and PIVC in the axial plane. (C) The projection pathway to PIVC that passes by the PIVC in the frontal plane.
Functional Evaluation
This study measured the ability of motor function using the functional ambulatory category (FAC) test and motricity index (MI) score with well-established reliabilities and validities (20, 21). Functional evaluation was conducted at the start of rehabilitation (phase 1, 13.21 ± 5.12 days), end of rehabilitation (phase 2, 40.57 ± 15.47 days), and at follow-up 6 months after onset (follow-up). The MI score was used to assess the motor function of the affected upper and lower extremities (maximum score: 100) (22). The FAC test was used to evaluate ambulatory function (23). FAC was designed to examine the levels of assistance and included six ordinal and hierarchical categories: 0 (non-ambulatory), 1 (needs continuous support from one person to help in carrying weight), 2 (needs intermittent support from one person to help with balance), 3 (needs only verbal supervision), 4 (help is required on stairs and uneven surfaces), and 5 (can walk independently anywhere).
Statistical Analysis
SPSS software (ver. 20.0; SPSS, Inc., Chicago, IL, USA) was used to analyze the results. Independent t-tests were used to compare the significant differences in DTI parameters after injury between subgroups A and B. Two-way repeated measures analysis of variance (ANOVA) was used to compare the significant differences in functional evaluation according to the recovery process between the two groups. The non-parametric Spearman correlation analysis was used to investigate the association between PIVC injury in DTI scanning and motor function according to the recovery process. The level of statistical significance was set at p < 0.05 (uncorrected).
Results
Diffusion Tensor Image
The results of DTI parameters between subgroups A and B were as follows. On the affected side, there were no significant differences in the mean FA and MD values of the PIVC between subgroups A and B (p > 0.05). Although the tract volume of the reconstructed projection pathway to the PIVC was less in subgroup A than in subgroup B, there were no significant differences between the two subgroups (p > 0.05) (Figures 1B, 2). In addition, no DTI parameters were significantly different between the two subgroups on the unaffected side (p > 0.05) (Table 2).
Functional Evaluations
The results of MI and FAC between subgroups A and B according to the recovery process are shown in Table 3. The results of the two-way repeated measures ANOVA indicated that there was no significant difference between subgroups A and B according to the recovery process (interaction time × group) in MI and FAC, respectively (F = 0.359, p = 0.620; F = 0.504, p = 0.571). In the case of the main effect of time, the results of MI and FAC were significantly different according to the recovery process (F = 37.032, p < 0.001; F = 70.596, p < 0.001, respectively).
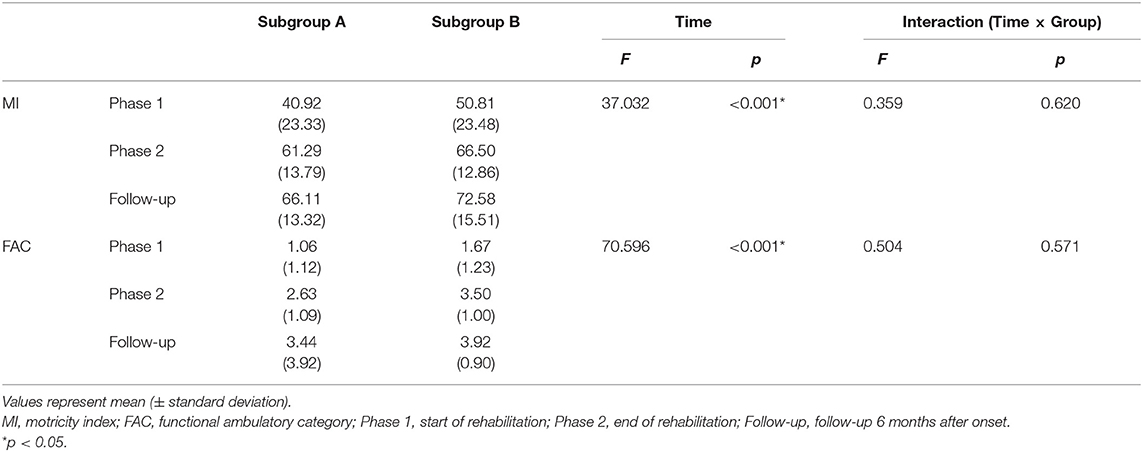
Table 3. Comparison of the results of MI and FAC between subgroup A and subgroup B according to recovery process.
Correlation According to the Recovery Process
A summary of the correlation between DTI parameters of the reconstructed projection pathway to the PIVC and functional evaluations according to the recovery process is shown in Tables 4, 5. On the affected side, the mean FA of the PIVC showed a positive correlation with FAC in phase 2 of the recovery process (r = 0.466, p = 0.012). Conversely, the mean FA values of PIVC were not correlated with phase 1 (r = 0.282, p = 0.146) and during follow-up (r = 0.113, p = 0.566) (Figure 3A). In addition, there was no correlation between the mean FA of DTI and the results of MI (p > 0.05). All DTI parameters except for FA values were also not significantly correlated with functional evaluations (p > 0.05) (Table 4). On the unaffected side, the mean FA values of the PIVC were significantly negatively correlated with MI in phase 1 (r = −0.457, p = 0.014), phase 2 (r = −0.476, p = 0.011), and during follow-up (r = −0.510, p = 0.004) (Figure 3B). The mean MD values of the PIVC were significantly negatively correlated with FAC in phase 2 (r = −0.434, p = 0.021). On the other hand, there was no correlation between mean FA values of DTI and FAC (p > 0.05). As with the affected side, all DTI parameters except for FA and MD values in the unaffected side were not significantly correlated with functional evaluations (p > 0.05) (Table 5).
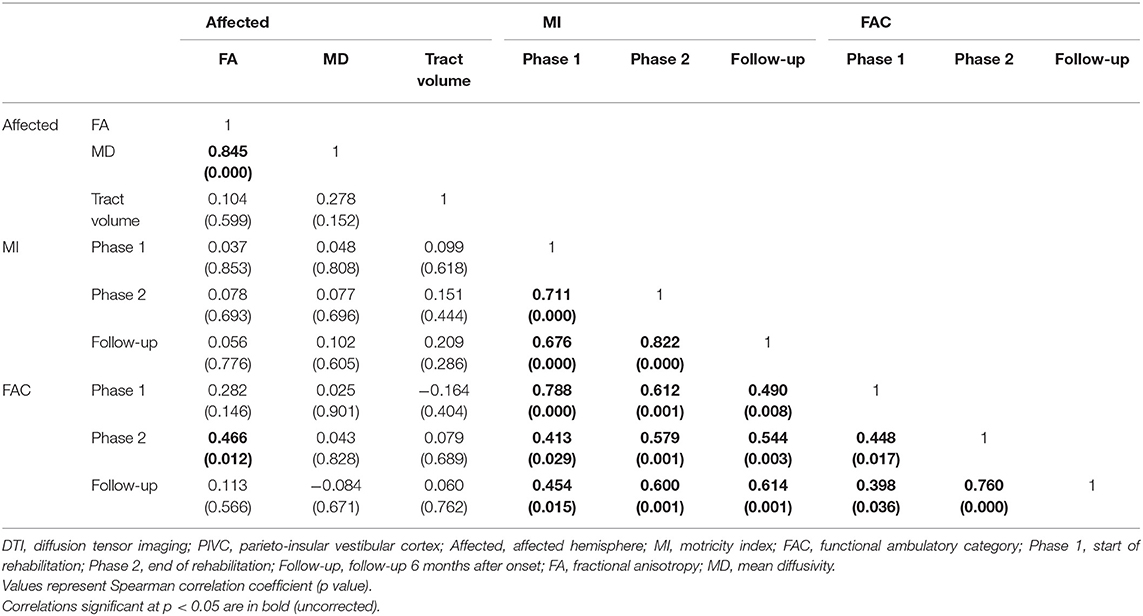
Table 4. Correlation between motor function and DTI parameters of PIVC in the affected hemisphere according to recovery process.
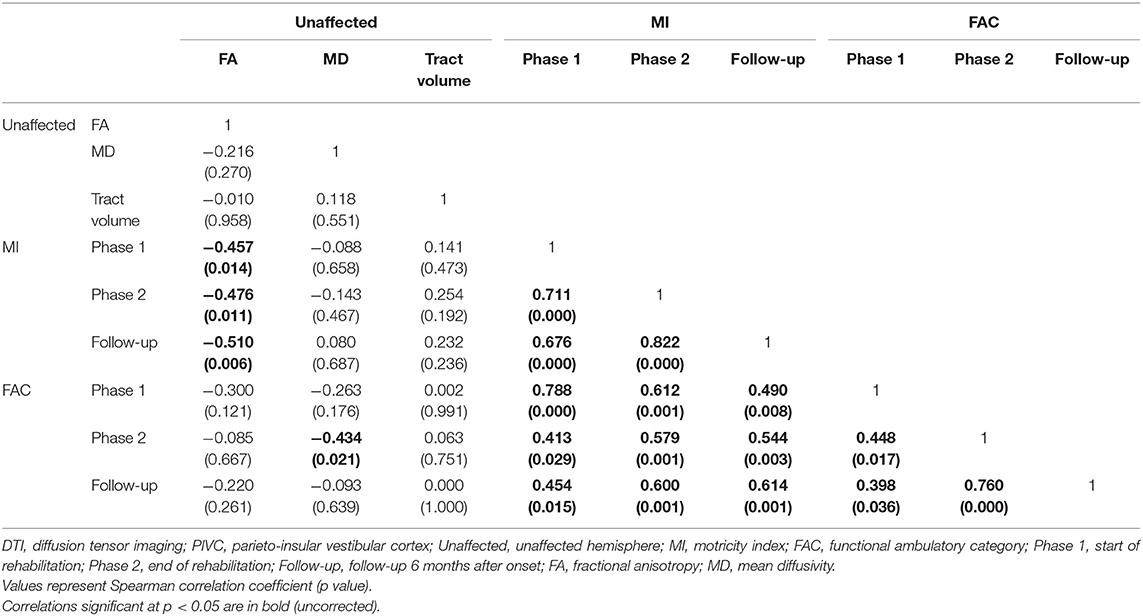
Table 5. Correlation between motor function and DTI parameters of PIVC in the unaffected hemisphere according to recovery process.
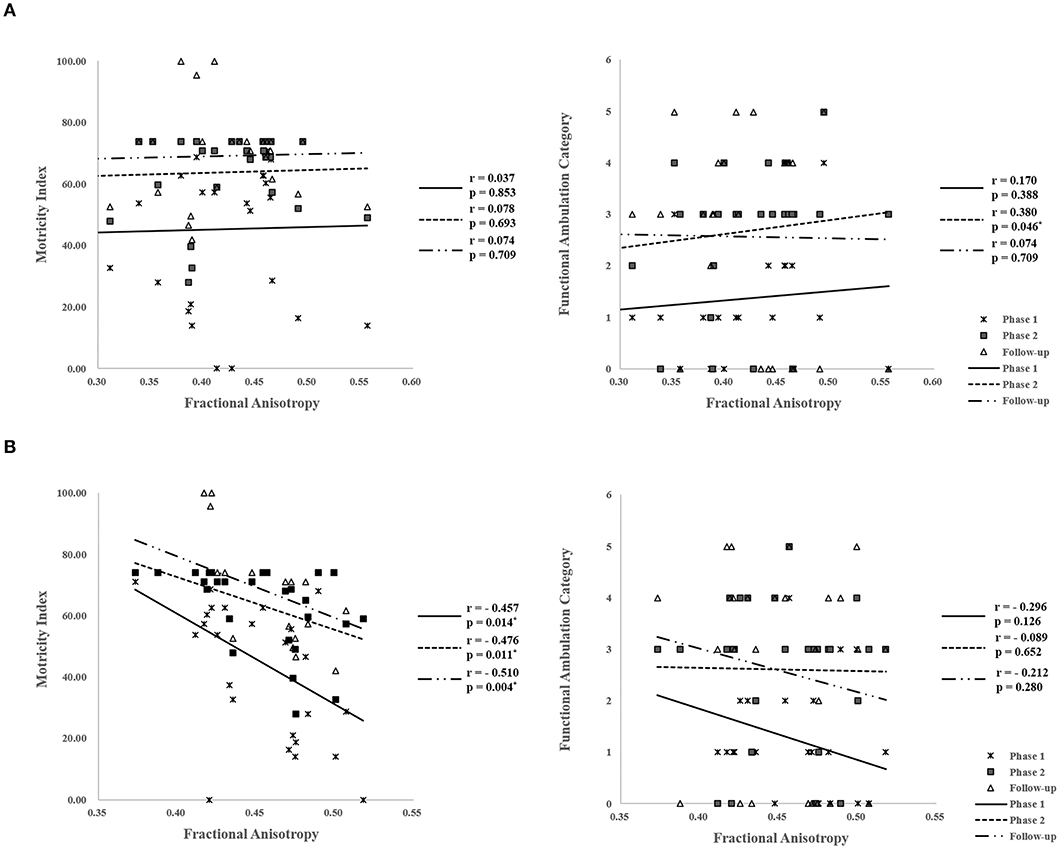
Figure 3. (A) Affected hemisphere. Correlations between motor function and fractional anisotropy (FA) of diffusion tensor imaging (DTI) parameters in the affected hemisphere according to recovery process. (B) Unaffected hemisphere. Correlations between motor function and fractional anisotropy (FA) of diffusion tensor imaging (DTI) parameters in the unaffected hemisphere according to recovery process. *p < 0.05.
Discussion
In the current study, we investigated the recovery process of motor function according to the degree of PIVC injury through a follow-up study for 6 months from onset. For this, we reconstructed the projection pathway to the PIVC as the core vestibular cortex in onset time and measured the motor function using FAC and MI from phase 1 to follow-up. We found that in phase 2, the FAC score was higher in subgroup B (patients with the continuous projection pathway to the PIVC) than in subgroup A (patients with the discontinuous projection pathway to the PIVC). In the correlation analysis, on the affected side, only FA values of projection pathways to the PIVC in onset time showed a mild positive correlation with FAC in phase 2. The FA value indicates the degree of directionality of water diffusion (24, 25). It represents white matter organization, and increased FA is related to increased myelination (25–27). We assumed that the increased FA values without change in tract volumes and MD values seem to indicate increased integration of neural fibers. Hence, the degree of projection pathways to PIVC injury in onset time seems to be related to the degree of recovery regarding gait function until the subacute stage. However, on the unaffected side, the FA values of the PIVC showed a significant negative correlation with MI from phase 1 to follow-up. Neuroplasticity is induced through hyperactivation of the PIVC tract without restoration of motor function to compensate for the affected side (28–30).
The PIVC is a core region of vestibular input into the cortical regions of the central vestibular system (31–33). In particular, the PIVC is involved in the integration and processing of sensory inputs from the vestibular, visual, and somatosensory systems, contributing to self-motion perception (8, 9, 31–33). Self-motion perception is essential for monitoring body movements, especially balance and walking (33). When vestibular inputs are absent or in conflict owing to an injury of the vestibular cortex region, the brain may generate an inaccurate self-motion perception in these patients (33). Hence, PIVC lesions can cause symptoms such as extremity weakness/numbness, imbalance, and gait abnormalities (17).
In DTI studies, Yeo et al. (34) demonstrated patients with injury of the vestibulospinal tract following lateral medullary syndrome (e.g., loss of consciousness, ataxia, postural instability, confusion, headache, incoordination, and visual deficits) (4). In 2019, Yeo et al. showed the relationship between the vestibular neural pathway, including the projection pathway to the PIVC and the vestibulospinal tract and balance according to aging. Moreover, DTI parameters in the vestibular neural pathway are associated with age-related reductions in balance ability (34). Together, these results commonly show that the PIVC is associated with central vestibular disorders and impairment of body movement, especially balance and gait function because PIVC encodes the vestibular signals contributing to self-motion perception (33). These results are consistent with the current findings showing that the degree of projection pathways for PIVC injury is associated with gait function, which requires complex integration of multisensory input and musculoskeletal systems (35, 36). Consequently, as far as we are aware, this is the first DTI study to demonstrate the degree of PIVC injury related to recovery of motor function from onset to follow-up.
Several previous studies have reported that patients with bilateral or unilateral loss of vestibular function are immediately ataxic, with severe postural instability (30, 37). These studies commonly report that over weeks and months, vestibular function such as postural stability and balance control improves through the process of vestibular compensation using vision, light touch, sensory bio-feedback, and potentiation of remaining vestibular function (30, 37, 38). Moreover, the recovery of postural stability and balance control after the loss of vestibular function is dependent on the remaining vestibular function (39, 40). Thus, we assume that the subgroup A appears to have improved gait function due to vestibular compensation, and subgroup B appears to have improved gait function due to recovery of the remaining vestibular function. Moreover, according to our results, the degree of the remaining projection pathway to the PIVC seems to accelerate the time-course of gait function recovery by using the remaining vestibular function. Conversely, even if the patients with a discontinuous projection pathway to the PIVC showed slow recovery of gait function, it seems that the gait function was eventually restored by vestibular compensation.
In the current study, the FA values of the PIVC were negatively correlated with MI from phase 1 to follow-up. Several studies have reported that persistent motor cortex activity in the unaffected hemisphere has been associated with poor motor function (41–45). In 2010, Kwak et al. reported that changes in the FA value of the unaffected side were not correlated with the motor function of the affected side. The results of the current study appear to be consistent with those of previous studies. Thus, it can be regarded as induced neuroplasticity by the hyperactivation of the PIVC tract without restoration of motor function to compensate for the affected side (28–30).
There are several studies for prediction of motor function using DTI in stroke. In 1999, Yang et al. demonstrated that the diffusion anisotropy ratio (affected/unaffected hemisphere) measured within 12 h of stroke onset correlated with the Barthel index at 3 months after stroke onset in 26 patients with cerebral infarction (46). Subsequently, Gillard et al. (47) reported that information obtained from DTI morphology (white matter distortion) in the early stage (11 h to 2 weeks after onset) of stroke (10 patients) provided useful information for predicting motor outcome at 4 months after onset (47). We believe that the findings of the current study are consistent with the findings of previous studies that attempted to predict motor outcome using a DTI study. Consequently, we believe that early detection of the projection pathway for PIVC injury using DTI would be helpful in clinical evaluation and prediction of prognosis for patients with PIVC injury.
The present study has a number of limitations that warrant consideration. First, we reconstructed the projection pathway to the PIVC only in onset time except phase 1, phase 2, and follow-up. Second, we could not consider other neural tracts related to gait function and balance, such as the vestibulospinal tract, corticoreticular pathway, and corticospinal tract. Third, we could not consider other clinical evaluations to measure the walking and balance function specifically, such as spatiotemporal parameters and the burg balance scale. Another limitation is that DTI analysis is operator dependent, and due to fiber complexity, this may result in false-positive or false-negative results for the fiber track. Further studies would be necessary, including follow-up DTI results and more neural tracts that are related to walking ability.
We investigated the recovery process of motor function according to the degree of PIVC injury through a follow-up study for 6 months from onset. According to the results, the degree of projection pathways to PIVC injury in onset time seems to be related to early restoration regarding gait function. Especially, it was found that the increased FA value of the PIVC in the affected hemisphere in onset time seems to be related to restoration regarding gait function until the subacute stage. Moreover, we believe that early detection of the projection pathway for PIVC injury using DTI would be helpful in the clinical evaluation and prediction of prognosis for patients with PIVC injury.
Data Availability Statement
The raw data supporting the conclusions of this article will be made available by the authors, without undue reservation.
Ethics Statement
The studies involving human participants were reviewed and approved by the institutional review board of Dankook University (DKU 2020-07-009). The patients/participants provided their written informed consent to participate in this study.
Author Contributions
SP and SO: writing—original draft preparation, methodology, and investigation. SY: writing—reviewing and editing, and conceptualization. SJ: data curation and project administration. IC: writing—original draft preparation and visualization. All authors contributed to the article and approved the submitted version.
Funding
This research was supported by Basic Science Research Program through the National Research Foundation of Korea (NRF), funded by the Ministry of Education, Science and Technology (2021R1A2C1095047).
Conflict of Interest
The authors declare that the research was conducted in the absence of any commercial or financial relationships that could be construed as a potential conflict of interest.
Publisher's Note
All claims expressed in this article are solely those of the authors and do not necessarily represent those of their affiliated organizations, or those of the publisher, the editors and the reviewers. Any product that may be evaluated in this article, or claim that may be made by its manufacturer, is not guaranteed or endorsed by the publisher.
References
1. Chou YH, Wagenaar RC, Saltzman E, Giphart JE, Young D, Davidsdottir R, et al. Effects of optic flow speed and lateral flow asymmetry on locomotion in younger and older adults: a virtual reality study. J Gerontol B Psychol Sci Soc Sci. (2009) 64:222–31. doi: 10.1093/geronb/gbp003
2. Putcha D, Ross RS, Rosen ML, Norton DJ, Cronin-Golomb A, Somers DC, et al. Functional correlates of optic flow motion processing in Parkinson's disease. Front Integr Neurosci. (2014) 8:57. doi: 10.3389/fnint.2014.00057
3. Eickhoff SB, Weiss PH, Amunts K, Fink GR, Zilles K. Identifying human parieto-insular vestibular cortex using fMRI and cytoarchitectonic mapping. Hum Brain Mapp. (2006) 27:611–21. doi: 10.1002/hbm.20205
4. Yeo SS, Jang SH, Kwon JW. Lateral medullary syndrome following injury of the vestibular pathway to the core vestibular cortex: diffusion tensor imaging study. Neurosci Lett. (2018) 665:147–51. doi: 10.1016/j.neulet.2017.12.010
5. Glasauer S, Dieterich M, Brandt T. Computational neurology of gravity perception involving semicircular canal dysfunction in unilateral vestibular lesions. Prog Brain Res. (2019) 248:303–17. doi: 10.1016/bs.pbr.2019.04.010
6. Guldin WO, Grüsser OJ. Is there a vestibular cortex? Trends Neurosci. (1998) 21:254–9. doi: 10.1016/S0166-2236(97)01211-3
7. Frank SM, Sun L, Forster L, Tse PU, Greenlee MW. Cross-Modal attention effects in the vestibular cortex during attentive tracking of moving objects. J Neurosci. (2016) 36:12720–8. doi: 10.1523/JNEUROSCI.2480-16.2016
8. Ferre ER, Bottini G, Haggard P. Vestibular inputs modulate somatosensory cortical processing. Brain Struct Funct. (2012) 217:859–64. doi: 10.1007/s00429-012-0404-7
9. Della-Justina HM, Gamba HR, Lukasova K, Nucci-Da-Silva MP, Winkler AM, Amaro E Jr. Interaction of brain areas of visual and vestibular simultaneous activity with fMRI. Exp Brain Res. (2015) 233:237–52. doi: 10.1007/s00221-014-4107-6
10. Laundre BJ, Jellison BJ, Badie B, Alexander AL, Field AS. Diffusion tensor imaging of the corticospinal tract before and after mass resection as correlated with clinical motor findings: preliminary data. AJNR Am J Neuroradiol. (2005) 26:791–6. doi: 10.2463/mrms.10.239
11. Maraka S, Jiang Q, Jafari-Khouzani K, Li L, Malik S, Hamidian H, et al. Degree of corticospinal tract damage correlates with motor function after stroke. Ann Clin Transl Neurol. (2014) 1:891–9. doi: 10.1002/acn3.132
12. Qin Y, Gu JW, Li GL, Xu XH, Yu K, Gao FB. Cerebral vasospasm and corticospinal tract injury induced by a modified rat model of subarachnoid hemorrhage. J Neurol Sci. (2015) 358:193–200. doi: 10.1016/j.jns.2015.08.1536
13. Kumar P, Kathuria P, Nair P, Prasad K. Prediction of upper limb motor recovery after subacute ischemic stroke using diffusion tensor imaging: a systematic review and meta-analysis. J Stroke. (2016) 18:50–9. doi: 10.5853/jos.2015.01186
14. Wang Q, Yap PT, Wu G, Shen D. Diffusion tensor image registration using hybrid connectivity and tensor features. Hum Brain Mapp. (2014) 35:3529–46. doi: 10.1002/hbm.22419
15. Wang Y, Shen Y, Liu D, Li G, Guo Z, Fan Y, et al. Evaluations of diffusion tensor image registration based on fiber tractography. Biomed Eng Online. (2017) 16:9. doi: 10.1186/s12938-016-0299-2
16. Jang SH, Lim HW, Yeo SS. The neural connectivity of the intralaminar thalamic nuclei in the human brain: a diffusion tensor tractography study. Neurosci Lett. (2014) 579:140–4. doi: 10.1016/j.neulet.2014.07.024
17. Yeo SS, Jang SH, Kwon JW. Central vestibular disorder due to ischemic injury on the parieto-insular vestibular cortex in patients with middle cerebral artery territory infarction: observational study. Medicine. (2017) 96:e9349. doi: 10.1097/MD.0000000000009349
18. Smith SM, Jenkinson M, Woolrich MW, Beckmann CF, Behrens TE, Johansen-Berg H, et al. Advances in functional and structural MR image analysis and implementation as FSL. Neuroimage. (2004) 23:S208–19. doi: 10.1016/j.neuroimage.2004.07.051
19. Kirsch V, Keeser D, Hergenroeder T, Erat O, Ertl-Wagner B, Brandt T, et al. Structural and functional connectivity mapping of the vestibular circuitry from human brainstem to cortex. Brain Struct Funct. (2016) 221:1291–308. doi: 10.1007/s00429-014-0971-x
20. Demeurisse G, Demol O, Robaye E. Motor evaluation in vascular hemiplegia. Eur Neurol. (1980) 19:382–9. doi: 10.1159/000115178
21. Cunha IT, Lim PA, Henson H, Monga T, Qureshy H, Protas EJ. Performance-based gait tests for acute stroke patients. Am J Phys Med Rehabil. (2002) 81:848–56. doi: 10.1097/00002060-200211000-00008
22. Orihuela-Espina F, Roldan GF, Sanchez-Villavicencio I, Palafox L, Leder R, Sucar LE, et al. Robot training for hand motor recovery in subacute stroke patients: a randomized controlled trial. J Hand Ther. (2016) 29:51–7. doi: 10.1016/j.jht.2015.11.006
23. Pohl M, Mehrholz J, Ritschel C, Ruckriem S. Speed-dependent treadmill training in ambulatory hemiparetic stroke patients: a randomized controlled trial. Stroke. (2002) 33:553–8. doi: 10.1161/hs0202.102365
24. Assaf Y, Pasternak O. Diffusion tensor imaging (DTI)-based white matter mapping in brain research: a review. J Mol Neurosci. (2008) 34:51–61. doi: 10.1007/s12031-007-0029-0
25. Neil JJ. Diffusion imaging concepts for clinicians. J Magn Reson Imaging. (2008) 27:1–7. doi: 10.1002/jmri.21087
26. Blumenfeld-Katzir T, Pasternak O, Dagan M, Assaf Y. Diffusion MRI of structural brain plasticity induced by a learning and memory task. PLoS ONE. (2011) 6:e20678. doi: 10.1371/journal.pone.0020678
27. O'donnell LJ, Westin CF. An introduction to diffusion tensor image analysis. Neurosurg Clin N Am. (2011) 22:185–96. doi: 10.1016/j.nec.2010.12.004
28. Kwak SY, Yeo SS, Choi BY, Chang CH, Jang SH. Corticospinal tract change in the unaffected hemisphere at the early stage of intracerebral hemorrhage: a diffusion tensor tractography study. Eur Neurol. (2010) 63:149–53. doi: 10.1159/000281108
29. Jang SH, Chang CH, Lee J, Kim CS, Seo JP, Yeo SS. Functional role of the corticoreticular pathway in chronic stroke patients. Stroke. (2013) 44:1099–104. doi: 10.1161/STROKEAHA.111.000269
30. Deveze A, Bernard-Demanze L, Xavier F, Lavieille JP, Elziere M. Vestibular compensation and vestibular rehabilitation. Current concepts and new trends. Neurophysiol Clin. (2014) 44:49–57. doi: 10.1016/j.neucli.2013.10.138
31. Lopez C, Blanke O. The thalamocortical vestibular system in animals and humans. Brain Res Rev. (2011) 67:119–46. doi: 10.1016/j.brainresrev.2010.12.002
32. Hitier M, Besnard S, Smith PF. Vestibular pathways involved in cognition. Front Integr Neurosci. (2014) 8:59. doi: 10.3389/fnint.2014.00059
33. Pfeiffer C, Serino A, Blanke O. The vestibular system: a spatial reference for bodily self-consciousness. Front Integr Neurosci. (2014) 8:31. doi: 10.3389/fnint.2014.00031
34. Yeo SS, Kwon JW, Cho IH. Associations between age-related changes in the core vestibular projection pathway and balance ability: a diffusion tensor imaging study. Behav Neurol. (2020) 2020:2825108. doi: 10.1155/2020/2825108
35. Lubetzky-Vilnai A, Kartin D. The effect of balance training on balance performance in individuals poststroke: a systematic review. J Neurol Phys Ther. (2010) 34:127–37. doi: 10.1097/NPT.0b013e3181ef764d
36. Dunsky A, Zeev A, Netz Y. Balance performance is task specific in older adults. Biomed Res Int. (2017) 2017:6987017. doi: 10.1155/2017/6987017
37. Horak FB. Postural compensation for vestibular loss. Ann N Y Acad Sci. (2009) 1164:76–81. doi: 10.1111/j.1749-6632.2008.03708.x
38. Peterka RJ, Statler KD, Wrisley DM, Horak FB. Postural compensation for unilateral vestibular loss. Front Neurol. (2011) 2:57. doi: 10.3389/fneur.2011.00057
39. Allum JH. Recovery of vestibular ocular reflex function and balance control after a unilateral peripheral vestibular deficit. Front Neurol. (2012) 3:83. doi: 10.3389/fneur.2012.00083
40. Lacour M, Bernard-Demanze L. Interaction between vestibular compensation mechanisms and vestibular rehabilitation therapy: 10 recommendations for optimal functional recovery. Front Neurol. (2015) 5:285. doi: 10.3389/fneur.2014.00285
41. Turton A, Wroe S, Trepte N, Fraser C, Lemon RN. Contralateral and ipsilateral EMG responses to transcranial magnetic stimulation during recovery of arm and hand function after stroke. Electroencephalogr Clin Neurophysiol. (1996) 101:316–28. doi: 10.1016/0924-980X(96)95560-5
42. Netz J, Lammers T, Homberg V. Reorganization of motor output in the non-affected hemisphere after stroke. Brain. (1997) 120:1579–86. doi: 10.1093/brain/120.9.1579
43. Marshall RS, Perera GM, Lazar RM, Krakauer JW, Constantine RC, Delapaz RL. Evolution of cortical activation during recovery from corticospinal tract infarction. Stroke. (2000) 31:656–61. doi: 10.1161/01.STR.31.3.656
44. Calautti C, Leroy F, Guincestre JY, Baron JC. Dynamics of motor network overactivation after striatocapsular stroke: a longitudinal PET study using a fixed-performance paradigm. Stroke. (2001) 32:2534–42. doi: 10.1161/hs1101.097401
45. Jang SH, Kim YH, Cho SH, Chang Y, Lee ZI, Ha JS. Cortical reorganization associated with motor recovery in hemiparetic stroke patients. Neuroreport. (2003) 14:1305–10. doi: 10.1097/01.wnr.0000078700.79393.09
46. Yang Q, Tress BM, Barber PA, Desmond PM, Darby DG, Gerraty RP, et al. Serial study of apparent diffusion coefficient and anisotropy in patients with acute stroke. Stroke. (1999) 30:2382–90. doi: 10.1161/01.STR.30.11.2382
Keywords: vestibular compensation, parieto-insular vestibular cortex (PIVC), diffusion tensor imaging-fiber tractography, motor function recovery, continuity
Citation: Park SY, Yeo SS, Jang SH, Cho IH and Oh S (2021) Associations Between Injury of the Parieto-Insular Vestibular Cortex and Changes in Motor Function According to the Recovery Process: Use of Diffusion Tensor Imaging. Front. Neurol. 12:740711. doi: 10.3389/fneur.2021.740711
Received: 13 July 2021; Accepted: 01 October 2021;
Published: 03 November 2021.
Edited by:
Alessia Sarica, University of Magna Graecia, ItalyReviewed by:
Mark W. Greenlee, University of Regensburg, GermanyHisham Mohammed, Burke Neurological Institute (BNI), United States
Copyright © 2021 Park, Yeo, Jang, Cho and Oh. This is an open-access article distributed under the terms of the Creative Commons Attribution License (CC BY). The use, distribution or reproduction in other forums is permitted, provided the original author(s) and the copyright owner(s) are credited and that the original publication in this journal is cited, in accordance with accepted academic practice. No use, distribution or reproduction is permitted which does not comply with these terms.
*Correspondence: Seunghue Oh, Z3JhbmRodWVAeW5jLmFjLmty