- Department of General Neurology, University Clinic of Neurology, Medical University of Graz, Graz, Austria
Multiple sclerosis is a multifactorial disease of the central nervous system with both genetic and environmental causes. The exact disease mechanisms are still unclear. Consequently, studies of possible treatment and preventive measures cover a large setting of heterogeneous approaches. Vitamin D is one of these approaches, and in many trials the relation of vitamin D serum levels and multiple sclerosis disease risk and activity describes different effects with sometimes inconsistent findings. Animal models are substantial for the research of disease mechanisms, and many of the drugs that are currently in use in multiple sclerosis have been developed, tested, or validated via animal studies. Especially when clinical studies show contradicting findings, the use of standardized settings and information about the mechanistic background is necessary. For this purpose, animal models are an essential tool. There is a variety of different experimental settings and types of animal models available, each of them with own strengths but also weaknesses. This mini-review aims to overview results of vitamin D studies in different animal models and sums up the most important recent findings.
Introduction
Multiple sclerosis (MS) is a chronic demyelinating disease of the central nervous system (CNS), affecting about 2.5 million people worldwide. It is an autoimmune disease with targeted myelin attack that causes demyelination (1, 2). Even though disease-modifying medications are capable to reduce disease severity, the disease continues to worsen over the patient's life span. Both genetic and environmental factors contribute to disease development, but the exact mechanisms are still not fully understood. Experimental autoimmune encephalomyelitis (EAE) in rodents is the favored model for exploring neuroinflammatory aspects of the disease, while toxin-induced demyelinating models like the cuprizone model are able to elucidate the cellular mechanism of de- and remyelination (2–5). Vitamin D (vitD), or the lack of it, is one frequently discussed environmental factor associated with MS, and its immunomodulatory ability has been widely demonstrated (6, 7). Despite numerous studies suggesting a beneficial effect of vitD intake in MS, there is still a controversy whether the supplementation can be used therapeutically (7). This work will discuss and summarize recent data from animal models on this topic. For overview, in Table 1 and Figure 1 the chemical and metabolic background of the vitD metabolism is summed up.
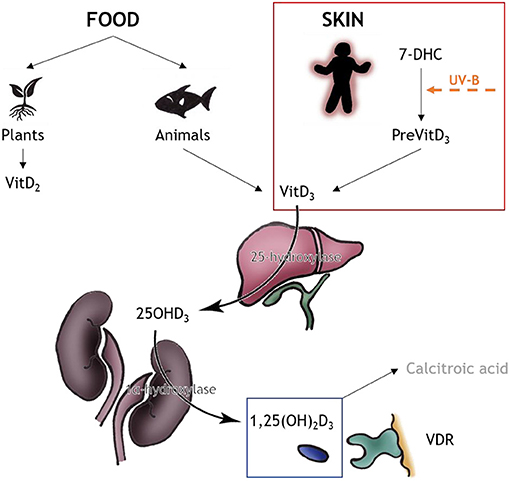
Figure 1. VitD metabolism. VitD can be obtained from two sources, food (minor part) and skin (major part). The source for vitD2 are plants and fungi, and the source of vitD3 are animals, especially fatty fish. The major source of vitD3 is produced nonenzymatically via the human skin: 7-DHC converts to PreVitD which isomerizes via heat to vitD3. In the liver, vitD3 is converted to 25OHD3 via 25-hydroxylase; in the kidneys the biologically active form 1,25(OH)2D3 is produced via 1alpha-hydroxylase. The active product either can bind to VDR for correspondent outcome or is converted to calcitroic acid for storage. Information upon vitD metabolism obtained from (8); drawings by MT Haindl.
Disease Prevention and Risk Reduction
Most studies investigating the capability of vitD to prevent MS are based on EAE findings. There is prophylactic potential of the association of myelin oligodendrocyte glycoprotein (MOG) peptide and active vitD against EAE. Vaccination with a mixture of MOG associated with vitD determines a reduction in CNS inflammation, dendritic cell maturation, clinical score, body weight loss, and production of cytokines, indicating that this association tones down the autoimmune response and prevents EAE. In other autoimmune conditions, there is a similar effect expectable (9). However, not only immunization with a MOG-vitD mixture has preventive effects. Also, very early intervention with the active form of vitD controls neuroinflammation during EAE development and was shown to decrease prevalence, clinical score, inflammation, and demyelination. Furthermore, a reduced major histocompatibility complex class II (MHCII) expression in macrophages and microglia as well as the level of oxidative stress and messenger RNA (mRNA) expression for caspase-1, interleukin (IL)-1β, and others was observed. These effects are accompanied by stabilization of blood–spinal cord barrier permeability, indicating that early intervention with vitD can control the neuroinflammatory process which is one of the hallmarks of EAE and MS (6). Conversely, low vitD levels are associated with increased risk of MS, suggesting the possibility of a gene–environment interaction in MS pathogenesis. VitD supplementation downregulates the MHCII expression in EAE. The impact of vitD on one master regulator of MHCII expression was investigated in 2020 on EAE rats. An inverse vitD dose-dependent effect on demyelination and inflammatory infiltration of the CNS, as well as downregulation of some pro-inflammatory genes, indicated an impact of vitD on pathophysiology and immune response during EAE. A modulatory effect of vitD regarding genetic variances in MS is therefore most likely probably relevant for the human disease as well (10). Additionally, vitD may reduce the MS risk in part through a mechanism involving myeloid cell vitD production and CTLA-4 upregulation in CNS-infiltrating T-cells. Humans with CTLA-4-inactivating mutations have an incompletely penetrant cellular phenotype with hyperactive effector CD4+ T-cells and a complex immune dysregulation syndrome. Another EAE study found out that CTLA-4 might act as a vitD-regulated immunological checkpoint in MS prevention (11).
Influence on T-Cells
The protective effect of vitD associates with decreased proliferation of CD4+ T-cells and a lower frequency of pathogenic T-helper (Th) 17 cells. Multiple pathways, critical for T-cell activation and differentiation, seem to be affected by vitD. For example, Jak/Stat, Erk/Mapk, and pi3K/Akt/mTor signaling pathway genes were downregulated upon vitD supplementation. VitD might modulate MS risk by changing myelin-reactive T-cell expression patterns as observed in EAE. Additionally, the role of vitD supplementation for prevention or treatment of autoimmune diseases in general is supported because CD4+ T-cells are driving target organ destruction in autoimmune diseases and many of the autoimmune loci are shared by multiple autoimmune diseases (7). However, the influence of vitD on T-cells seems to act not only via metabolic pathways but also upon dendritic cells (DCs). DCs mediate immune response via their antigen presentation function, driving T-cell differentiation. VitD has the ability to induce tolerogenic DCs (VD3-DCs), increasing the negative regulatory signaling pathway programmed death 1 (PD1)/programmed death ligand 1 (PDL1). The expression of PD1 and PDL1 increases significantly after vitD treatment, enhancing the activation of this pathway. As a result, the activation of T-cells can be inhibited and the number of Tregs is increased, promoting immune tolerance (12). The induction of VD3-DCs further inhibits the infiltration of T helper type 1 (Th1) and Th17 cells into the spinal cord and increases the proportions of regulatory T-cells and regulatory B-cells in peripheral immune organs, thereby attenuating EAE (13). One dose of calcitriol plus vitD is able to reverse EAE, resulting in increased CD4+ T-cell transcripts, Helios protein, CD4+ Helios+ FoxP3+ Treg, and global DNA methylation. Calcitriol might drive a transition from CD4+ T-cell to Treg cell dominance, recycling homocysteine to methionine, reducing homocysteine toxicity, maintaining DNA methylation, and stabilizing CD4+ Helios+ FoxP3+ Treg. Structural similarity in the responsible vitD-promoters even suggests a similar regulatory mechanism for humans (14). CD4+ T-cells have a cooperative amplification loop promoting CD4+Helios+FoxP3+ Treg development, and this process is disturbed when the vitD pathway is impaired (15).
Because of the role of T-cells in MS, glucocorticoids remain the most commonly used substance in treating acute MS relapses. However, in approximately 30% of patients, a limited efficacy of glucocorticoids is reported, often in patients with low serum vitD levels. VitD increases glucocorticoid-induced apoptosis of T-cells via upregulation of the glucocorticoid receptor (GCR). With the help of two different EAE models with reduced or absent GCR signaling, it was demonstrated that there are synergistic effects of vitD and glucocorticoids, probably mediated through mTORc1 signaling. Severe vitD deficiency is associated with downregulation of an mTORc1 inhibitor in human T-cells. In animals with T-cell-specific depletion of mTORc1 and in animals receiving a specific mTORc1 inhibitor, the synergistic effects of vitD/glucocorticoids on GCR upregulation, T-cell apoptosis, and therapeutic efficacy in EAE failed (16).
Beside the direct influence of vitD on T-cells, also related molecules such as cytokines and chemokines can induce powerful changes. For example vitD increases the production of IL-4, IL-10, and TGF-β while decreasing IFNγ, IL-6, TNFα, and IL-17 production accompanied with a deviated balance between Th1/Th2 and Th17/Treg to Th2 and Treg under middle and high doses of vitD (17). Accordingly, vitD downregulates the expression of some Th17 cell-related cytokines, key inflammatory chemokines, and chemokine receptors in EAE considering a possible therapeutic potential of vitD in future treating MS (18).
Remyelination
There is also recent literature available describing the effects of vitD on remyelination. Most data are available on the cuprizone model, since it is the easiest way of studying de- and remyelination. One study found that there is a significant increase MOG and 2′,3′-cyclic-nucleotide 3′-phosphodiesterase (CNPase) expression in vitD-supplemented cuprizone-exposed mice compared to control groups. MOG is a minor component of the myelin sheath, but it has an important autoantigen link to the pathogenesis of EAE whereas the protein CNPase is one of the main proteins of myelin and its appearance seems to be one of the earliest events of oligodendrocyte differentiation and myelination. VitD may play a role in the process of remyelination by increasing MOG and CNPase expression in the cortex (19). In another study, axonal damage during de- and remyelination in the cuprizone mouse model was investigated. The authors found significantly higher neurofilament preservation in the high dose-supplemented inactive vitD group in comparison to the low dose-supplemented group. High doses of active vitD, however, given after the demyelination phase as well as during remyelination did not influence axonal regeneration, while inactive vitD, given before and during cuprizone exposure, seems to have a protective effect on axons (20). VitD might even have the ability to trigger neuronal stem cell differentiation (21).
VitD and MS Progression
After a disease duration of about 20 years, most MS patients enter the progressive state of the disease with a steady worsening of clinical neurological symptoms. Only little data are available upon the question whether vitD could be a reasonable support during progressive MS. Some clinical studies suggest a protective role of higher vitD levels on myelin content in progressive MS and an association between a low vitD status at the beginning of MS and the early entry to the progressive disease state (22, 23). A most recent study however could not confirm these assumption—vitD levels were not associated with the severity of optical coherence tomography findings or low-contrast letter acuity in their group of progressive MS patients (24). Clinical studies may furthermore be hampered by the possibility that severely affected progressive MS patients may have limited sunlight exposure as a consequence of their disease rather than as a cause. This demonstrates the need of more mechanistic knowledge of the mode of action of vitD in progressive MS. Unfortunately, there is no animal study addressing this research question so far. More studies making use of recently established animal models of progressive MS would be most welcome to elucidate the mechanistical background of how vitD could affect this disease state (25, 26).
Issues and Problems
VitD Controversy
Even though the majority of animal studies affirm a beneficial effect of vitD in experimental animal models of MS, there is also a small list of literature suggesting that vitD is not capable of positively influencing autoimmune diseases. VitD and sunlight have each been reported to protect against the development of EAE. Since exposure of ultraviolet (UV) light also causes the generation of vitD, studies investigated whether the UV-based suppression of EAE results, at least in part, from the production of vitD. One study examined UV suppression of EAE in mice devoid of vitD receptor (VDR) and mice unable to produce 7-DHC. UV light suppression of EAE occurred in the absence of vitD production and in the absence of VDR (27). However, it is possible that the UV suppression of EAE can further be influenced by the active form of vitD. The presence of active vitD surprisingly actually counteracted the suppressive effect of UV in one study (28). Further investigations should focus on identifying the pathway responsible for the protective action of UV in EAE and presumably human MS (27). Two independent research groups have demonstrated unexpectedly that vitD deficiency blocks EAE development. In one study, the suppression of EAE is even reported as a result from hypercalcemia and not as an effect of the active form of vitD (28). Another study revealed that a NBUVB light at 311 nm is responsible for the EAE suppression, and this wavelength does not produce vitD. There are suggestions upon a mechanism of EAE suppression independent of vitD, whereas a remaining question is still whether the active form of vitD has any impact on the NBUVB suppression of EAE (28, 29). These findings emphasize the need of further mechanistic research to gain a better understanding of EAE suppression and the role of vitD and light.
VitD Supplementation: Attention Should Be Paid to Adequate Dosage
The problem of potential overdosing vitD resulting in hypercalcemia is a critical aspect to this topic. Moderate supplementation of vitD reduces the severity of subsequent EAE in mice, associated with an expansion of Tregs. Direct exposure of T-cells to vitD metabolites inhibits their activation. On the other hand, high doses of vitD (200 nmol/l) in mice result in fulminant EAE with massive CNS infiltration. This is caused by mild hypercalcemia only observed in animals receiving high, but not medium, doses of vitD (30). Because of this problem, one study investigated the therapeutic potential of Paricalcitol (Pari) on EAE, since it is a non-hypercalcemic vitD2 analogue, capable of promoting anti-inflammatory activity in kidney and heart diseases. In this study, severity, apoptosis and neuropathology of EAE were reduced via Pari accompanied by inhibition of glial cell activation, cellular infiltration, pro-inflammatory molecules, and activation of nuclear factor κB (NF-κB). This phenomenon could further be reduced by suppressing NF-κB with its inhibitor and Pari in combination (31). On the contrary, another study found a lower production of proinflammatory cytokines and reduced inflammation only in the EAE/vitD group, not in the Pari group. The authors thus suggest, that vitD, but not Pari, has the potential to be used as a preventive therapy to control MS severity (32). Another approach to bypass the problem of vitD overdose suggests a combination of vitamin A (vitA) and vitD. The combinatory treatment with vitA and vitD using the optimal synergistic effects with low doses could be beneficial in addressing the side effects and possibly paving the way for a more efficient MS therapy. One study demonstrated a significant different cytokine gene expression profile in the treated and control groups, suggesting a benefit of this treatment approach (33).
Experimental Animal Models and Problems in Translation
Results from animal models have to be critically validated. Common EAE models reflect important aspects of MS, but one has to consider that these models are mainly based on inflammation induced by autoreactive CD4+ T-cells whereas results from clinical trials in MS indicate that CD8+ T-cells and B-lymphocytes may play an important role in MS. In EAE, the inflammatory demyelinating disease burns out when the peripheral brain antigen depot has been removed. Therefore, it is most likely that in human MS, a persistent trigger within or outside the CNS is required for chronic disease propagation (4). This emphasizes the need of a variety of carefully selected animal models to cover different aspects of different phases of MS. However, especially concerning progressive MS, data are currently scarce.
VitD and Clinical Data in MS
Most of the clinical data regarding vitD and MS focus on its ability to reduce the risk of MS development. The suggestion that low vitD serum level is one MS risk factor is nowadays mainly accepted. One important question however remains upon its actions once the disease has started. Many studies concerning with this question are unfortunately insufficiently powered, most often without a long-lasting follow-up or with methodological bias, hindering conclusive results. Nevertheless, it appears highly likely that vitD is able to decrease components of the inflammatory pathway of the disease. Of course, further scientific validation is needed; a systematic vitD supplementation of MS patients has already been recommended in clinical practice, anyway (34). In comparison to the evidence of benefit of vitD supplementation in early MS, there is little known about the role of vitD in the progressive disease phase. Even though it is well known that these patients commonly suffer from low vitD serum levels, there is still the requirement of long-term observational studies (23, 35).
Discussion
Numerous animal studies attest benefits of vitD. Based on the current state of knowledge, vitD supplementation may be considered as a preventative measure for decreasing the risk for developing autoimmune diseases and potentially as adjunctive therapy (7). Figure 2 sums up the most recent findings discussed in this work. Studies on the beneficial effect of vitD in EAE suggest that treatment with vitD before EAE induction or from peak disease is effective at reducing disease severity. This beneficial effect may be mediated at least in part through the attenuation of T-cells, reduction of axonal and neuronal loss, and support of oligodendrocyte maturation (36, 37). Some other recent studies focused on genetic variations and how vitD could intervene. So far, it has been shown that vitD promotes negative feedback regulation of Toll-like receptor (TLR) signaling in macrophages in vitro, which in turn ameliorates inflammation. Naturally occurring allelic differences in the Vra4locus/Mhc2ta could be relevant for the efficacy of vitD in modulation of MS-like neuroinflammation and potentially even in MS, but sufficient information about response to vitD within the Vra4 gene locus is still missing (10). Data from EAE allow the conclusion that vitD synthesis by activated microglial cells and macrophages in the CNS preserves neurological function by dampening the inflammatory process. VitD seems to support the CTLA-4 immunological checkpoint to prevent immune-mediated neurological damage. Additionally, the protective effect of vitD seems to involve epigenetic mechanisms (DNA methylation), which may provide a molecular basis for cellular memory that mediates long-term effects and suggests potential for future combined therapies (7). VitD and VDR are closely associated with the development of autoimmune diseases. There might be unknown factors capable of regulating VDR. It is already known that miRNAs are associated with VDRs. Even though the involvement of miRNAs in human diseases strengthened our understanding of pathogenesis, candidates for miRNAs that have the potential to control autoimmune pathomechanisms remain limited (38). Those strong regulatory molecules could become powerful tools of autoimmune disease management, if the exact mechanisms are elucidated in further studies. Beside validated findings on vitD beneficial effects, one important aspect is the reasonable application of vitD. Findings of studies regarding dose dependence of vitD suggest that vitD at moderate levels may exert a direct regulatory effect, while continuous high-dose vitD treatment could trigger MS disease activity by raising T-cell excitatory calcium (30, 39). This is indeed a very important lesson from animal studies since hypercalcemia was reported also in humans supplemented with high doses of vitD (40, 41). Monitoring the vitD level in MS patients thus is crucial to ensure positive effects of the supplementation. Another important topic is the impact of vitD on different MS treatments. Current MS treatments are found to be directly or indirectly linked to NF-κB pathways and act to adjust the immune system. MS is associated with constitutive activation of NF-κB, which results in excessive expression of related effector molecules, driving inflammation, and there is a very complex association of this factor and different cytokine patterns involved in EAE progression too (31). Pari could be one potential NF-κB blocker, and other vitD analogues might act in a similar way. Again, most of our knowledge about NF-κB is based on results from animal studies and further animal studies will be needed to investigate the mechanism further (31, 32). A similar issue concerns MS therapies working by increasing PDL1 expression. The PD1/PDL1 pathway might act as a key player in the mechanism of demyelination and autoimmune response due to its regulation of antigen-presenting cell and T-cell interaction. VD3-DCs attenuate the clinical symptoms of EAE by increasing the activation of the PD/PDL1 signaling pathway. However, the specific mechanisms of this signaling pathway still remain unsolved and further research is necessary in order to apply VD3-DCs to clinical practice (12). Translation of data from preclinical models to humans is always to be used with caution. However, it is still necessary to investigate pathophysiological mechanisms with the help of animal models. Even though most preclinical assays indicated a strong potential of vitD as a useful agent for EAE prevention or therapy, clinical trials with patients revealed mixed data (32). One possible reason is the missing knowledge in how vitD exactly acts in many different signaling pathways. Another interesting research goal is to elucidate gender aspects of vitD effects (42, 43). In general, autoimmune diseases are characterized by a significant female bias. This is also the case in MS where more females are affected (43). This sexual dimorphism in autoimmune diseases seems to be related to sex hormones, which differently affect the immune system. In general, males show higher immunosuppression may be due to androgens, and females show a higher immunoreactivity and competence likely related to estrogens. This leads to a greater resilience to infections but also to a higher risk for developing autoimmune diseases (42, 43). Additionally, the outcome of vitD status in MS is determined by gene-by-sex interactions (44). Thus, gender and sex hormones could be included as variables when evaluating the potential power of vitD to influence autoimmune diseases (42, 43). Beside all these findings, one has to consider that there is a developmental stage-dependent efficiency of vitD to ameliorate neuroinflammation, suggesting that childhood and adolescence should be the target for the most effective preventive vitD treatment (45).
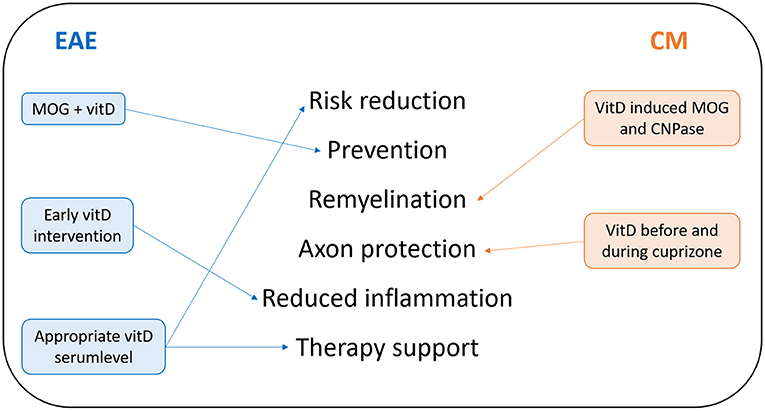
Figure 2. Summary of recent findings of protective vitD effects. Most results upon vitD effects in MS-related animal models were obtained by EAE and the cuprizone model (CM). Lessons from EAE are written on the left side in blue; lessons from CM are shown in orange on the right side. In the middle of this summary, recent research findings of protective effects of vitD are listed.
Conclusion
The majority of literature suggests a beneficial role of vitD at least in therapy of MS related animal models. When it comes to the translation of these findings to the human situation, the most important aspect to be considered is the right dosage, to avoid negative side effects. Nevertheless, for an effective treatment or support of MS therapies with the help of vitD and probably other vitamins, further studies are necessary. Especially if and how exactly vitD could intervene in pathophysiological mechanisms of progressive MS remains largely unsolved.
Author Contributions
MH wrote the original draft and generated the figures. All authors contributed to the writing of this article, approved the submitted version, were involved in developing the plan for the article, and in reviewing and editing the manuscript.
Conflict of Interest
The authors declare that the research was conducted in the absence of any commercial or financial relationships that could be construed as a potential conflict of interest.
Publisher's Note
All claims expressed in this article are solely those of the authors and do not necessarily represent those of their affiliated organizations, or those of the publisher, the editors and the reviewers. Any product that may be evaluated in this article, or claim that may be made by its manufacturer, is not guaranteed or endorsed by the publisher.
References
1. Wasnik S, Sharma I, Baylink DJ, Tang X. Vitamin D as a potential therapy for multiple sclerosis: where are we? Int J Mol Sci. (2020) 21:3102. doi: 10.3390/ijms21093102
2. Burrows DJ, McGown A, Jain SA, De Felice M, Ramesh TM, Sharrack B, et al. Animal models of multiple sclerosis: from rodents to zebrafish. Mult Scler. (2019) 25:306–24. doi: 10.1177/1352458518805246
3. Bjelobaba I, Begovic-Kupresanin V, Pekovic S, Lavrnja I. Animal models of multiple sclerosis: focus on experimental autoimmune encephalomyelitis. J Neurosci Res. (2018) 96:1021–42. doi: 10.1002/jnr.24224
4. Lassmann H, Bradl M. Multiple sclerosis: experimental models and reality. Acta Neuropathol. (2017) 133:223–44. doi: 10.1007/s00401-016-1631-4
5. Procaccini C, De Rosa V, Pucino V, Formisano L, Matarese G. Animal models of multiple sclerosis. Eur J Pharmacol. (2015) 759:182–91. doi: 10.1016/j.ejphar.2015.03.042
6. de Oliveira LRC, Mimura LAN, Fraga-Silva TFC, Ishikawa LLW, Fernandes AAH, Zorzella-Pezavento SFG, et al. Calcitriol prevents neuroinflammation and reduces blood-brain barrier disruption and local macrophage/microglia activation. Front Pharmacol. (2020) 11:161. doi: 10.3389/fphar.2020.00161
7. Zeitelhofer M, Adzemovic MZ, Gomez-Cabrero D, Bergman P, Hochmeister S., N'diaye M, et al. Functional genomics analysis of vitamin D effects on CD4+ T cells in vivo in experimental autoimmune encephalomyelitis. Proc Natl Acad Sci U S A. (2017) 114:E1678–87. doi: 10.1073/pnas.1615783114
8. Bikle DD. Vitamin D metabolism, mechanism of action, and clinical applications. Chem Biol. (2014) 21:319–29. doi: 10.1016/j.chembiol.2013.12.016
9. Mimura LA, Chiuso-Minicucci F, Fraga-Silva TF, Zorzella-Pezavento SF, França TG, Ishikawa LL, et al. Association of myelin peptide with vitamin D prevents autoimmune encephalomyelitis development. Neuroscience. (2016) 317:130–40. doi: 10.1016/j.neuroscience.2015.12.053
10. Hochmeister S, Aeinehband S, Dorris C, Berglund R, Haindl MT, Velikic V, et al. Effect of vitamin D on experimental autoimmune neuroinflammation is dependent on haplotypes comprising naturally occurring allelic variants of CIITA (Mhc2ta). Front Neurol. (2020) 11:600401. doi: 10.3389/fneur.2020.600401
11. Spanier JA, Nashold FE, Nelson CD, Praska CE, Hayes CE. Vitamin D3-mediated resistance to a multiple sclerosis model disease depends on myeloid cell 1,25-dihydroxyvitamin D3synthesis and correlates with increased T cell CTLA-4 expression. J Neuroimmunol. (2020) 338:577105. doi: 10.1016/j.jneuroim.2019.577105
12. Cao Q, Zheng C, Xie Z, Liu L, Zhu J, Jin T. The change of PD1, PDL1 in experimental autoimmune encephalomyelitis treated by 1,25(OH)2D3. J Neuroimmunol. (2020) 338:577079. doi: 10.1016/j.jneuroim.2019.577079
13. Xie Z, Chen J, Zheng C, Wu J, Cheng Y, Zhu S, et al. 1,25-dihydroxyvitamin D3 -induced dendritic cells suppress experimental autoimmune encephalomyelitis by increasing proportions of the regulatory lymphocytes and reducing T helper type 1 and type 17 cells. Immunology. (2017) 152:414–24. doi: 10.1111/imm.12776
14. Moore JR, Hubler SL, Nelson CD, Nashold FE, Spanier JA, Hayes CE. 1,25-Dihydroxyvitamin D3 increases the methionine cycle, CD4+ T cell DNA methylation and Helios+Foxp3+ T regulatory cells to reverse autoimmune neurodegenerative disease. J Neuroimmunol. (2018) 324:100–14. doi: 10.1016/j.jneuroim.2018.09.008
15. Spanier JA, Nashold FE, Mayne CG, Nelson CD, Hayes CE. Vitamin D and estrogen synergy in Vdr-expressing CD4(+) T cells is essential to induce Helios(+)FoxP3(+) T cells and prevent autoimmune demyelinating disease. J Neuroimmunol. (2015) 286:48–58. doi: 10.1016/j.jneuroim.2015.06.015
16. Hoepner R, Bagnoud M, Pistor M, Salmen A, Briner M, Synn H, et al. Vitamin D increases glucocorticoid efficacy via inhibition of mTORC1 in experimental models of multiple sclerosis. Acta Neuropathol. (2019) 138:443–56. doi: 10.1007/s00401-019-02018-8
17. Haghmorad D, Yazdanpanah E, Tavaf MJ, Zargarani S, Soltanmohammadi A, Mahmoudi MB, et al. Prevention and treatment of experimental autoimmune encephalomyelitis induced mice with 1, 25-dihydroxyvitamin D3. Neurol Res. (2019) 41:943–57. doi: 10.1080/01616412.2019.1650218
18. Jafarzadeh A, Azizi SV, Arabi Z, Ahangar-Parvin R, Mohammadi-Kordkhayli M, Larussa T, et al. Vitamin D down-regulates the expression of some Th17 cell-related cytokines, key inflammatory chemokines, and chemokine receptors in experimental autoimmune encephalomyelitis. Nutr Neurosci. (2019) 22:725–37. doi: 10.1080/1028415X.2018.1436237
19. Mashayekhi F, Salehi Z. Administration of vitamin D3 induces CNPase and myelin oligodendrocyte glycoprotein expression in the cerebral cortex of the murine model of cuprizone-induced demyelination. Folia Neuropathol. (2016) 54:259–64. doi: 10.5114/fn.2016.62535
20. Nystad AE, Torkildsen Ø, Wergeland S. Effects of vitamin D on axonal damage during de- and remyelination in the cuprizone model. J Neuroimmunol. (2018) 321:61–5. doi: 10.1016/j.jneuroim.2018.05.016
21. Tiwari S, Lapierre J, Ojha CR, Martins K, Parira T, Dutta RK, et al. Signaling pathways and therapeutic perspectives related to environmental factors associated with multiple sclerosis. J Neurosci Res. (2018) 96:1831–46. doi: 10.1002/jnr.24322
22. Abbatemarco JR, Fox RJ, Li H, Ontaneda D. Vitamin D and MRI measures in progressive multiple sclerosis. Mult Scler Relat Disord. (2019) 35:276–82. doi: 10.1016/j.msard.2019.08.014
23. Muris AH, Rolf L, Broen K, Hupperts R, Damoiseaux J, Smolders J, et al. Low vitamin D status at diagnosis is associated with an early conversion to secondary progressive multiple sclerosis. J Steroid Biochem Mol Biol. (2016) 164:254–7. doi: 10.1016/j.jsbmb.2015.11.009
24. Abbatemarco JR, Fox RJ, Li H, Bermel RA, Ontaneda D. Vitamin D levels and visual system measurements in progressive multiple sclerosis: a cross-sectional study. Int J MS Care. (2021) 23:53–8. doi: 10.7224/1537-2073.2020-005
25. Üçal M, Haindl MT, Adzemovic MZ, Strasser J, Theisl L, Zeitelhofer M, et al. Widespread cortical demyelination of both hemispheres can be induced by injection of pro-inflammatory cytokines via an implanted catheter in the cortex of MOG-immunized rats. Exp Neurol. (2017) 294:32–44. doi: 10.1016/j.expneurol.2017.04.014
26. Haindl MT, Ücal M, Fazekas F, Hochmeister S. Overview of promising rat models for cortical lesion research- 2006 until now. J Neurol Neuromed. (2018) 3:8–12. doi: 10.29245/2572.942X/2018/5.1218
27. Irving AA, Marling SJ, Seeman J, Plum LA, DeLuca HF. UV light suppression of EAE (a mouse model of multiple sclerosis) is independent of vitamin D and its receptor. Proc Natl Acad Sci U S A. (2019) 116:22552–5. doi: 10.1073/pnas.1913294116
28. Wang Y, Marling SJ, Martino VM, Prahl JM, Deluca HF. The absence of 25-hydroxyvitamin D3-1α-hydroxylase potentiates the suppression of EAE in mice by ultraviolet light. J Steroid Biochem Mol Biol. (2016) 163:98–102. doi: 10.1016/j.jsbmb.2016.04.010
29. de Abreu DAF, El Ibrahim C, Boucraut J, Khrestchatisky M, Féron F. Severity of experimental autoimmune encephalomyelitis is unexpectedly reduced in mice born to vitamin D-deficient mothers. J Steroid Biochem Mol Biol. (2010) 121:250–3. doi: 10.1016/j.jsbmb.2010.03.006
30. Häusler D, Weber MS. Vitamin D supplementation in central nervous system demyelinating disease-enough is enough. Int J Mol Sci. (2019) 20:218. doi: 10.3390/ijms20010218
31. Zhang D, Qiao L, Fu T. Paricalcitol improves experimental autoimmune encephalomyelitis (EAE) by suppressing inflammation via NF-κB signaling. Biomed Pharmacother. (2020) 125:109528. doi: 10.1016/j.biopha.2019.109528
32. Mimura LAN, Fraga-Silva TFC, Oliveira LRC, Ishikawa LLW, Borim PA, Machado CM, et al. Preclinical therapy with Vitamin D3 in experimental encephalomyelitis: efficacy and comparison with paricalcitol. Int J Mol Sci. (2021) 22:1914. doi: 10.3390/ijms22041914
33. Parastouei K, Solaymani-Mohammadi F, Shiri-Shahsavar MR, Chahardoli R, Nasl-Khameneh AM, Zarandi MB, et al. The effect of calcitriol and all-trans retinoic acid on T-bet, IFN-γ, GATA3 and IL-4 genes expression in experimental autoimmune encephalomyelitis. APMIS. (2020) 128:583–92. doi: 10.1111/apm.13073
34. Pierrot-Deseilligny C, Souberbielle JC. Vitamin D and multiple sclerosis: an update. Mult Scler Relat Disord. (2017) 14:35–45. doi: 10.1016/j.msard.2017.03.014
35. Shoemaker TJ, Mowry EM. A review of vitamin D supplementation as disease-modifying therapy. Mult Scler. (2018) 24:6–11. doi: 10.1177/1352458517738131
36. Sloka S, Zhornitsky S, Silva C, Metz LM, Yong VW. 1,25-Dihydroxyvitamin D3 protects against immune-mediated killing of neurons in culture and in experimental autoimmune encephalomyelitis. PLoS ONE. (2015) 10:e0144084. doi: 10.1371/journal.pone.0144084
37. Shirazi HA, Rasouli J, Ciric B, Wei D, Rostami A, Zhang GX. 1,25-Dihydroxyvitamin D3 suppressed experimental autoimmune encephalomyelitis through both immunomodulation and oligodendrocyte maturation. Exp Mol Pathol. (2017) 102:515–21. doi: 10.1016/j.yexmp.2017.05.015
38. Long HC, Wu R, Liu CF, Xiong FL, Xu Z, He D, et al. MiR-125a-5p regulates Vitamin D receptor expression in a mouse model of experimental autoimmune encephalomyelitis. Neurosci Bull. (2020) 36:110–20. doi: 10.1007/s12264-019-00418-0
39. Häusler D, Torke S, Peelen E, Bertsch T, Djukic M, Nau R, et al. High dose vitamin D exacerbates central nervous system autoimmunity by raising T-cell excitatory calcium. Brain. (2019) 142:2737–55. doi: 10.1093/brain/awz190
40. Malihi Z, Wu Z, Stewart AW, Lawes CM, Scragg R. Hypercalcemia, hypercalciuria, and kidney stones in long-term studies of vitamin D supplementation: a systematic review and meta-analysis. Am J Clin Nutr. (2016) 104:1039–51. doi: 10.3945/ajcn.116.134981
41. Marcus JF, Shalev SM, Harris CA, Goodin DS, Josephson SA. Severe hypercalcemia following vitamin d supplementation in a patient with multiple sclerosis: a note of caution. Arch Neurol. (2012) 69:129–32. doi: 10.1001/archneurol.2011.1199
42. Crescioli C, Minisola S. Vitamin D: autoimmunity and gender. Curr Med Chem. (2017) 24:2671–86. doi: 10.2174/0929867323666161220105821
43. Magyari M. Gender differences in multiple sclerosis epidemiology and treatment response. Dan Med J. (2016) 63:B5212.
44. Krementsov DN, Asarian L, Fang Q, McGill MM, Teuscher C. Sex-specific gene-by-Vitamin D interactions regulate susceptibility to central nervous system autoimmunity. Front Immunol. (2018) 9:1622. doi: 10.3389/fimmu.2018.01622
Keywords: multiple sclerosis, vitamin D, animal models-rodent, autoimmune diseases, therapy
Citation: Haindl MT and Hochmeister S (2021) Vitamin D in Multiple Sclerosis—Lessons From Animal Studies. Front. Neurol. 12:757795. doi: 10.3389/fneur.2021.757795
Received: 12 August 2021; Accepted: 20 September 2021;
Published: 20 October 2021.
Edited by:
Ruth Dobson, Queen Mary University of London, United KingdomReviewed by:
Ermelinda De Meo, San Raffaele Hospital (IRCCS), ItalyShuhei Nishiyama, Massachusetts General Hospital and Harvard Medical School, United States
Copyright © 2021 Haindl and Hochmeister. This is an open-access article distributed under the terms of the Creative Commons Attribution License (CC BY). The use, distribution or reproduction in other forums is permitted, provided the original author(s) and the copyright owner(s) are credited and that the original publication in this journal is cited, in accordance with accepted academic practice. No use, distribution or reproduction is permitted which does not comply with these terms.
*Correspondence: Sonja Hochmeister, c29uamEuaG9jaG1laXN0ZXJAbWVkdW5pZ3Jhei5hdA==