- 1Department of Neurology, Affiliated Hospital of Medical College, North Sichuan Medical College (University), Nanchong, China
- 2Department of Preventive Medicine, North Sichuan Medical College (University), Nanchong, China
Background: Theta burst stimulation (TBS), a type of patterned repetitive transcranial magnetic stimulation (rTMS), has several advantages, such as short time of single treatment and low stimulation intensity compared with traditional rTMS. Since the efficacy of TBS on the symptoms of Parkinson's disease (PD) was inconsistent among different studies, we systematically searched these studies and quantitatively analyzed the therapeutic effect of TBS for patients with PD.
Methods: We followed the recommended PRISMA guidelines for systematic reviews. Studies from PubMed, EMBASE, CENTRAL, and ClinicalTrials.gov from January 1, 2005 of each database to September 30, 2021 were analyzed. We also manually retrieved studies of reference.
Results: Eight eligible studies with 189 participants (received real TBS and/or sham TBS) were included. This metaanalysis found that TBS did not significantly improve Unified Parkinson's Disease Rating Scale part III (UPDRS-III) score in the “on” medicine state (SMD = −0.06; 95% CI, −0.37 to 0.25; p = 0.69; I2 = 0%), while, it brought significant improvement of UPDRS-III scores in the “off” medicine state (SMD = −0.37; 95% CI, −0.65 to −0.09; p < 0.01; I2 = 19%). Subgroup analysis found that merely continuous TBS (cTBS) over the supplementary motor area (SMA) brought significant improvement of UPDRS-III score (SMD = −0.63; 95% CI, −1.02 to −0.25; p < 0.01). TBS had insignificant effectiveness for upper limb movement disorder both in the “on” and “off” medicine status (SMD = −0.07; 95% CI, −0.36 to 0.22; p = 0.64; I2 = 0%; SMD = −0.21; 95% CI, −0.57 to 0.15; p = 0.26; I2 = 0%; respectively). TBS significantly improved slowing of gait in the “off” medicine status (SMD = −0.37; 95% CI, −0.71 to −0.03; p = 0.03; I2 = 0%). Subgroup analysis suggested that only intermittent TBS (iTBS) over the primary motor cortex (M1) + dorsolateral prefrontal cortex (DLPFC) had significant difference (SMD = −0.57; 95% CI, −1.13 to −0.01; p = 0.04). Additionally, iTBS over the M1+ DLPFC had a short-term (within 2 weeks) therapeutic effect on PD depression (MD = −2.93; 95% CI, −5.52 to −0.33; p = 0.03).
Conclusion: Our study demonstrated that cTBS over the SMA could significantly improve the UPDRS-III score for PD patients in the “off,” not in the “on,” medicine state. TBS could not bring significant improvement of upper limb movement dysfunction. ITBS over the M1+DLPFC could significantly improve the slowing of gait in the “off” medicine status. Additionally, iTBS over the M1+DLPFC has a short-term (within 2 weeks) therapeutic effect on PD depression. Further RCTs of a large sample, and excellent design are needed to confirm our conclusions.
Introduction
Parkinson's disease (PD) is the second most common neurodegenerative disorder. The prevalence of PD in industrialized countries is generally estimated at 0.3% of the entire population and ~1% of people over 60 years of age (1). The classical motor symptoms of PD include bradykinesia, muscular rigidity, rest tremor, and postural and gait impairment. Nonmotor dysfunction, such as depression, and cognitive impairment are also frequently present (2). PD is associated with functional deficits in multiple brain areas, including basal ganglia nuclei, cerebellum, and cortical areas (3).
Transcranial magnetic stimulation (TMS) is a non-invasive and painless method to stimulate the human brain (4). Repetitive TMS (rTMS) refers to the application of trains of regularly repeating TMS pulses. Repeated applications of it can produce even long-term effects that last for weeks to months (4–6). In addition to the local stimulatory effect on the cortical area, rTMS can also induce a distant effect on other cortical and subcortical brain regions, probably via the cortico-basal, ganglia–thalamocortical motor circuit (7, 8). The previous work showed that high-frequency (HF) rTMS targeting bilateral primary motor cortex (M1) regions could improve the motor performance in patients with PD (9–14), and HF rTMS of left dorsolateral prefrontal cortex (DLPFC) had an antidepressant effect on patients with PD (13, 15, 16). Relevant evidence-based guidelines also gave recommendations on the therapeutic effect of rTMS in motor symptoms and depression in patients with PD (17).
Theta burst stimulation (TBS), a variation of rTMS, may facilitate induction of plasticity mechanisms (18), which affords a short stimulation duration, low stimulation pulse intensity, and a possibility to improve rTMS efficiency (19). When TBS is delivered continuously (cTBS), it decreases cortical excitability, whereas intermittent TBS (iTBS) increases cortical excitability (20). Since TBS may have fewer adverse effects, such as seizures, impairment of hearing and cognition function (21), and shorter time of single intervention (within several minutes) compared with traditional rTMS, in recent years, an increasing number of studies have begun to explore the therapeutic effect of TBS on the motor and nonmotor symptoms in patients with PD (22–35). Nevertheless, there are inconsistencies of conclusion among these studies. Besides, the latest evidence-based guidelines did not include recommendations on the therapeutic effect of TBS in patients with PD (17), which brought dilemma to clinical practice. This systematic review and metaanalysis examined the studies on the therapeutic effect and tolerability of TBS for motor and nonmotor dysfunction in patients with PD.
Methods
Study Design
Our meta-analysis is according to the Preferred Reporting Items for Systematic Reviews and Meta-analyses (PRISMA) statement (36).
Study Search and Selection
We systematically retrieved relevant literature published in PubMed, EMBASE, and CENTRAL, and ClinicalTrials.gov from January 1, 2005 to September 30, 2021. Database searches were limited to articles published in English. Besides, we also manually retrieved studies of reference. The search terms we used to query the databases were as follows: (“Parkinson Disease” or “Parkinson's Disease” or “Parkinsonism”) and (“theta-burst stimulation” or “TBS” or “repetitive transcranial magnetic stimulation” or “rTMS” or “non-invasive stimulation)”. Studies were included if they met the “PICOS” as follows: population [patients with a diagnosis of idiopathic PD according to the UK PD Brain Bank criteria, (37)], intervention (received true patterned rTMS: TBS), comparators (sham TBS), outcome measure (clinical evaluation of motor and nonmotor symptoms), and study design (clinical trial). Studies were excluded if: (1) they were clear from the article title or abstract that did not meet the inclusion criteria, (2) they did not have data available (mean ± SD/SE) for effect size estimation or lacked sufficient reporting detail, such as conference abstract or presentation review articles, editorials, and other nonclinical trials.
Two investigators (BC and TZ) independently screened the titles and abstracts of the literature and decided whether the article should be further retrieved according to the inclusion and exclusion criteria. Those that could not be excluded were retrieved, and the full text was reviewed by the two reviewers (WZ and LS). For articles that may be included, if reported data were insufficient for data analysis, we contacted the corresponding author by email to request access to additional data. Any disagreements were resolved by discussion with a third reviewer (SZ).
Data Extraction
The following information was extracted by two reviewers (BC and TZ) independently, from included studies: first author, year of publication, study design, patients' age, sample size, PD duration, gender distribution, Hoehn and Yahr scale, timepoints of assessment, neuropsychological symptom assessment scale, TBS protocols, and study quality.
Quality Assessment
The quality assessments were performed with the PEDro scale (38), which is based on the Delphi List criteria (39) and is considered valid and reliable (38, 40). The PEDro scale assesses the methodological quality of a study based on important criteria, such as concealed allocation, intention-to-treat analysis, and the adequacy of follow-up. These characteristics make the PEDro scale a useful tool for assessing the quality of physical therapy and rehabilitation trials. The PEDro scale consisted of 11 items. One item on the PEDro scale (eligibility criteria) is related to external validity and is generally not used to calculate the method score (41, 42). Therefore, a score of 0–10 was allocated to each study (9–10: excellent; 6–8: good; 4–5: fair; and ≤ 3: poor) (43). Additionally, publication bias on included studies was assessed by the funnel plot and bias tests. If the plot is symmetrical or p > 0.05 from bias tests, it should be deemed not publication bias.
Data Synthesis and Statistical Analysis
The RevMan5.3 and Stata16 software were used to combine the data when at least two studies reported similar clinical outcomes. For quantitative synthesis, the effect size was calculated based on the mean prepost change in the treatment group minus the mean prepost change in the comparison group, divided by the pooled pretest standard deviation (44). If the unit of measurement was consistent across trials, the results were presented as the weighted mean difference (MD) with 95% confidence intervals (CIs) or else replaced by the standard mean difference (SMD). Data from the standard error of the mean (SEM) were converted to the standard deviation (SD) using sample size in the formula SD = SEM × √N (45). We used the random-effects model and fixed-effects model to calculate the pooled SMD and generated forest plots to display the single study and pooled-effect size. The Chi-square test and I2 statistic were used to assess heterogeneity among studies. Heterogeneity was considered significant if the p-value of the χ2 test was < 0.1 or I2 > 50% (46–48). If there was no significant heterogeneity, the fixed-effects model was used to pool data across the included studies; otherwise, the random-effects model was used (49, 50).
Results
Search Results
Our search strategy to query limited databases retrieved 1,360 studies, while, many of these were identified as duplicates. After screening the titles and abstracts, 375 records were excluded as they did not meet the inclusion criteria (“PICOS”) in our work, and we determined 59 articles for full-text reading. Finally, eight studies were included in our metaanalysis (23–25, 28–30, 32, 34). The literature selection is presented in Figure 1.
Study Characteristics
Eight eligible studies included for metaanalysis had 189 participants (received real TBS and/or sham TBS).
Four studies (24, 25, 30, 32) were parallel-controlled and four studies (23, 28, 29, 34) were crossover-controlled. Five studies gave single-session (23, 25, 28, 29, 34) and three studies gave multiple sessions (total of six to 42 sessions) (24, 30, 32). Four studies evaluated the immediate effects after TBS using clinical symptom assessment scales (23, 25, 28, 29, 34), and three studies conducted a follow-up of clinical effects range from 1 week to 1 month after TBS intervention (24, 30, 32). Two studies assessed the therapeutic effects of TBS only in the “on” anti-Parkinsonism medicine state (29, 51). While, two studies evaluated only in the “off” medicine status (under the anti-Parkinsonism medicine withdrawal status for at least 12 hours) (23, 34). Besides, four studies assessed the therapeutic effects of TBS both in the “on” and “off” medicine state (24, 25, 28, 32). The characteristics of the included studies are summarized in Table 1, and the TBS intervention parameters are summarized in Table 2.
Quality Assessment
The PEDro scores of the included studies ranged from 6 to 9, with mean scores of 7. Two studies were of excellent quality (24, 32) and five studies were of good quality. No studies (23, 25, 28–30, 34) were assessed as fair quality or poor quality. A detailed evaluation of the methodological quality of included studies for metaanalysis is provided in Table 3. Egger's test by Stata16 showed no significant publication bias for all clinical symptoms of quantitative analysis.
Effects of TBS on UPDRS-III Score
Six included studies provided the date of Unified Parkinson's Disease Rating Scale part III (UPDRS-III) score in the “on” and/or “off” medicine status (23, 24, 28, 30, 32, 34). The results showed that there was an insignificant difference in UPDRS-III score between the real TBS and the sham TBS in the “on” medicine state (SMD = −0.06; 95% CI, −0.37 to 0.25; P = 0.69; I2 = 0%. Figure 2A). Subgroup analysis based on different types of TBS (iTBS/cTBS) over the related brain targets (iTBS/cTBS- brain targets) showed insignificant differences among groups [iTBS- M1+DLPFC, SMD = −0.07; 95% CI, −0.41 to 0.26; p = 0.68, vs. cTBS-supplementary motor area (SMA), SMD = −0.01; 95% CI, −0.78 to 076; p = 0.98, Figure 2A]. Contrarily, there was a significant difference of UPDRS-III score between real TBS and sham TBS in the “off” medicine condition (SMD = −0.37; 95% CI, −0.65 to −0.09; p < 0.01; I2 = 19%, Figure 2B). Further subgroup analyses based on iTBS/cTBS-brain targets showed that merely cTBS-SMA brought significant improvement of UPDRS-III score (SMD = −0.63; 95% CI, −1.02 to −0.25; p < 0.01).
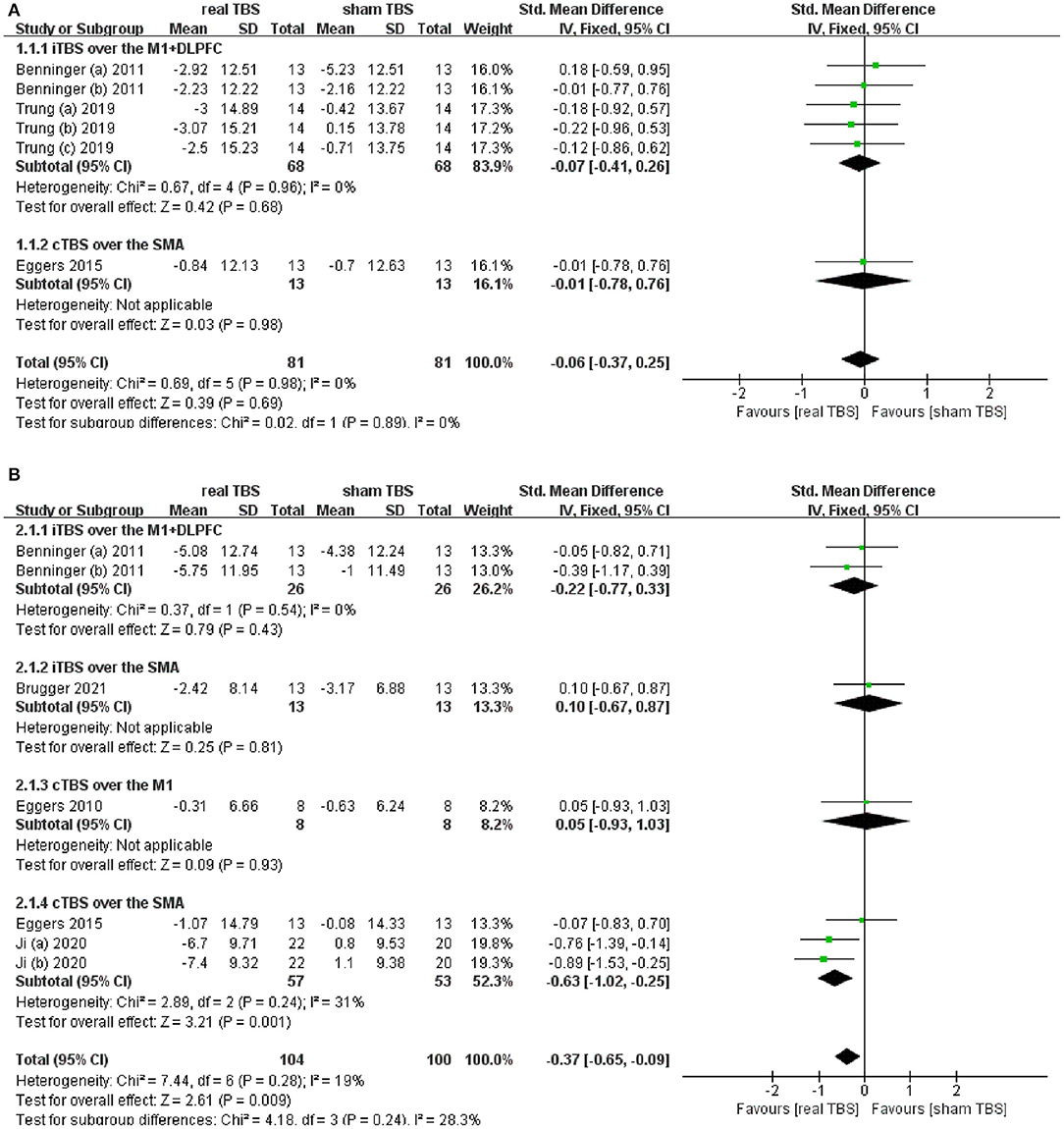
Figure 2. (A) Forest plot and metaanalysis of UPDRS-III score between real TBS and sham TBS in the “on” medicine state: subgroup analysis based on iTBS/cTBS-brain targets. (B) Forest plot and metaanalysis of UPDRS-III score between real TBS and sham TBS in the “off” medicine state: subgroup analysis based on iTBS/cTBS-brain targets.
Effects of TBS on Upper Limb Movement
Five studies reported changes in symptom scale score concerning upper limb motor dysfunction in “on” and/or “off” medicine status (23–25, 28–30). UPDRS-III bradykinesia of sequential hand and arm movement time items; UPDRS-III finger tapping, hand movement, and arm rigidity items; and Purdue Pegboard test (PPT) were involved totally. Results showed that there was an insignificant difference in the upper limb motor disorder scores between real TBS and sham TBS in the “on” medicine status (SMD = −0.07; 95% CI, −0.36 to 0.22; p = 0.64; I2 = 0%, Figure 3A). Subgroup analysis based on iTBS/cTBS-brain targets showed insignificant differences among groups [iTBS-M1+DLPFC, SMD = −0.26; 95% CI, −0.72 to 0.20; P = 0.26, vs. cTBS-SMA, SMD = 0.01; 95% CI, −0.53 to 0.56, P = 0.96, vs. iTBS- dorsal premotor cortex (PMd), SMD = 0.29; 95% CI, −0.43 to 1.01, p = 0.43, vs. cTBS-PMd, SMD = −0.10; 95% CI, −0.81 to 0.62; P = 0.79. Figure 3A]. Similarly, there was no significant difference in the “off” medicine status (SMD = −0.21; 95% CI, −0.57 to 0.15; P = 0.26; I2 = 0%, Figure 3B). Subgroup analysis based on iTBS/cTBS-brain targets shown insignificant differences among groups (iTBS-M1+DLPFC, SMD = −0.36; 95% CI, −0.91 to 0.19; P = 0.20, vs. cTBS-M1, SMD = 0.13; 95% CI, −0.85 to 1.11; P = 0.80, vs. cTBS- SMA, SMD = −0.16; 95% CI, −0.70 to 0.39; P = 0.57. Figure 3B).
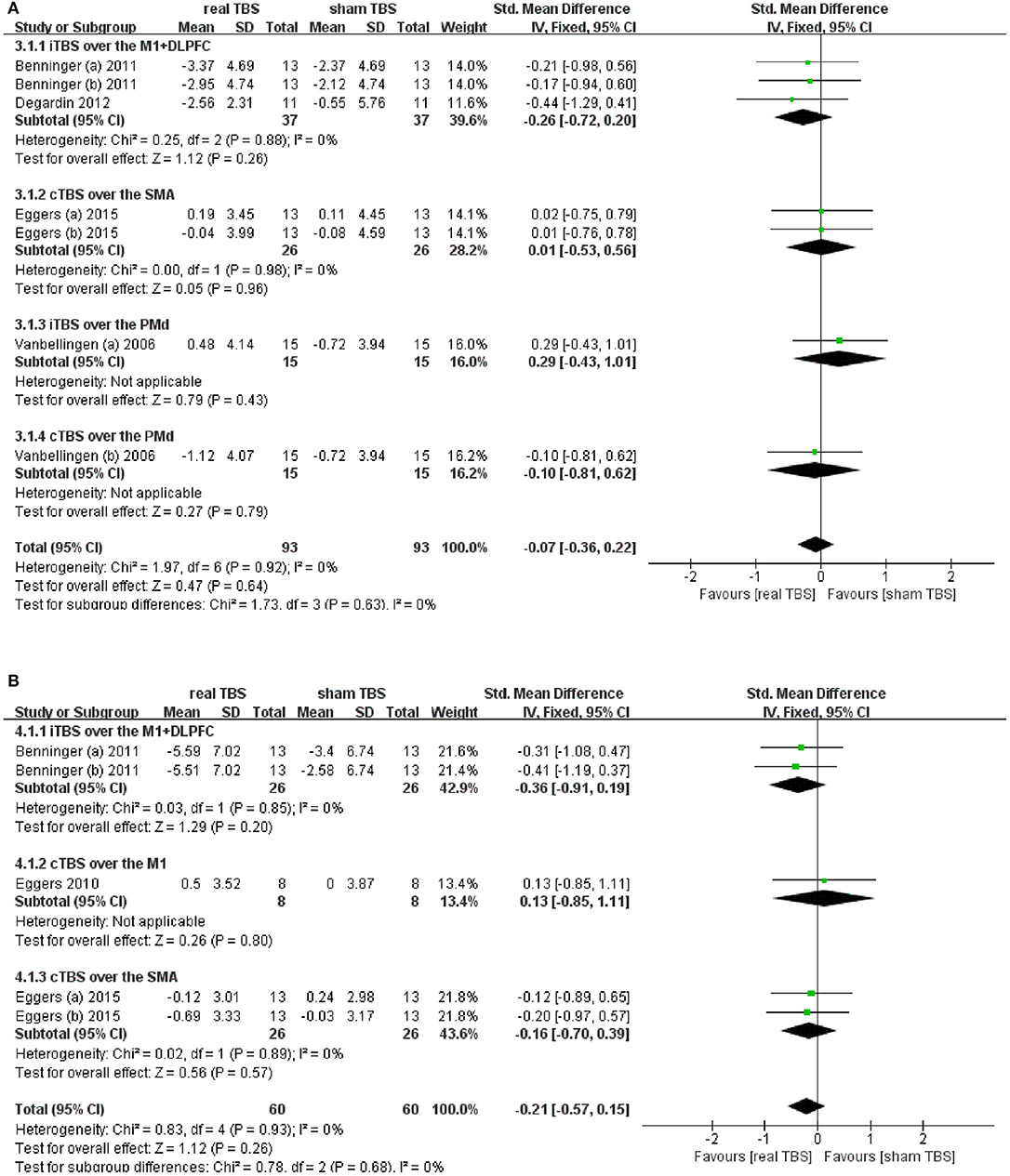
Figure 3. (A) Forest plot and metaanalysis of upper limb movement between real TBS and sham TBS in the “on” medicine state: subgroup analysis based on iTBS/cTBS-brain targets. (B) Forest plot and metaanalysis of upper limb movement between real TBS and sham TBS in the “off” medicine state: subgroup analysis based on iTBS/cTBS-brain targets.
Effects of TBS on Gait Disorders
Two included studies reported the data of assessing the therapeutic effect on slowing of gait (including the time to walk 10 meters and 20 meters) (24, 32). The meta-analysis shown a significant difference of gait disorder in real TBS relative to sham TBS in the “off” medicine status (SMD = −0.37; 95% CI, −0.71 to −0.03; P = 0.03; I2 = 0%, Figure 4). Subgroup analysis based on iTBS/cTBS-brain targets suggested that there was significant difference between groups (iTBS-M1+DLPFC, SMD = −0.57; 95% CI, −1.13 to −0.01; P = 0.04, vs. cTBS-SMA, SMD = −0.25; 95% CI, −0.68 to 0.18; p = 0.25, Figure 4).
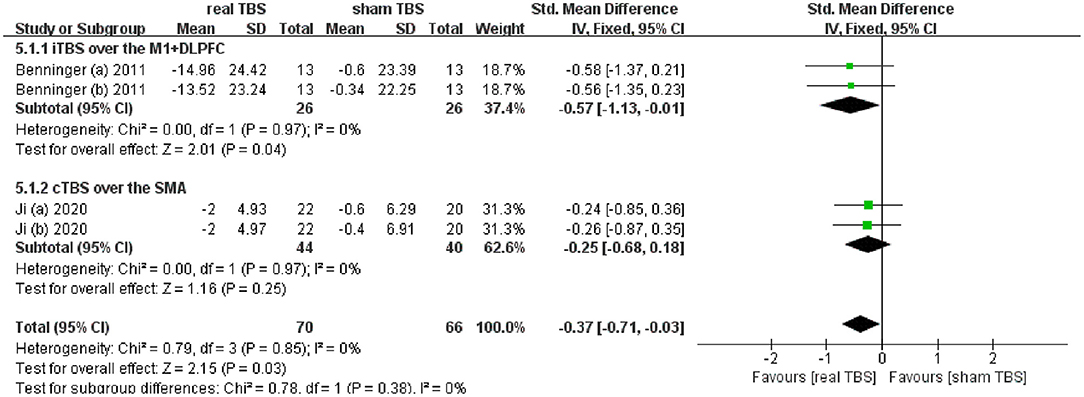
Figure 4. Forest plot and metaanalysis of gait disorder between real TBS and sham TBS in the “off” medicine state: subgroup analysis based on iTBS/cTBS-brain targets.
Effects of TBS on Depression
Two included studies reported the date of antidepressant effect by assessing beck depression inventory (BDI) scores (24, 30). The metaanalysis showed no significant difference in BDI scores for real TBS relative to sham TBS (MD = −2.03; 95% CI, −4.08 to 0.01; p = 0.05; I2 = 17%, Figure 5). Subgroup analysis based on different follow-up time showed that there was a significant difference between groups (within 2 weeks, MD = −2.93; 95% CI, −5.52 to −0.33; p = 0.03, vs. more than 2 weeks, MD = −0.55; 95% CI, −3.89 to 2.79; p = 0.75, Figure 5).
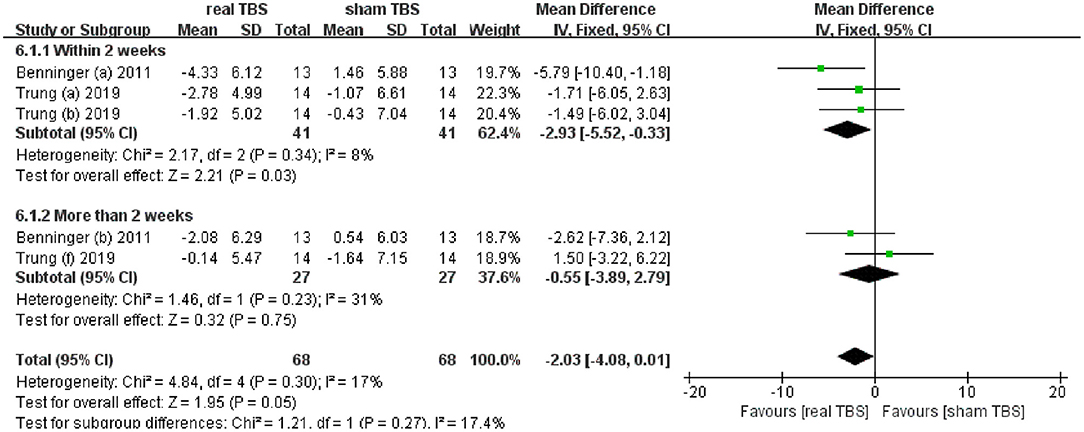
Figure 5. Forest plot and metaanalysis of depression between real TBS and sham TBS: subgroup analysis based on different follow-up times.
Effects of TBS on Cognitive Impairment and Dementia
Four included studies explored the therapeutic effect of TBS on cognitive dysfunction in patients with PD by evaluating the scores of cognitive domain scales (30, 31, 33, 35), and the results among these studies were inconsistent. We failed to synthesize data of cognitive disorders because we can only get details of the data (mean ± SD/SE) from one of the mentioned articles.
Safety/Adverse Events
Of the 14 studies included in this review, three studies (26, 30, 34) did not mention whether there were adverse events. Eleven studies (22–25, 27–29, 31–33, 35) reported that there were no serious adverse events, and one of the studies (33) reported uncomfortable sensation over local and adjacent areas of stimulation site during iTBS application, which was resolved by reducing the stimulation intensity (3–5%).
Discussion
To the best of our knowledge, this is the first metaanalysis to evaluate the therapeutic effect of TBS, patterned rTMS, on motor and nonmotor symptoms in patients with PD. This study provides evidence to demonstrate that iTBS-M1+DLPFC or cTBS-SMA did not significantly decrease the UPDRS-III score in the “on” medicine state, while, cTBS-SMA, not iTBS-M1+DLPFC, iTBS-SMA, and cTBS-M1, could significantly improve the UPDRS-III score of these patients in the “off” medicine state. TBS had insignificant efficacy for upper limb movement disorder both in the “on” medicine state (iTBS-M1+DLPFC, cTBS-SMA, cTBS-PMd, and iTBS-PMd), and in the “off” medicine state (iTBS-M1+DLPFC, cTBS-M1, and cTBS-SMA). ITBS-M1+DLPFC, not cTBS-SMA, significantly improved slowing of gait in PD patients in the “off” medicine status. Additionally, iTBS -M1+DLPFC had a short-term (within 2 weeks) significant antidepressant effect on patients with PD.
The UPDRS-III is a reliable and valid scale for the assessment of motor performance for patients with PD, which also correlates with disease severity and quality of daily life (52). Our results demonstrated that TBS did not significantly improve the UPDRS-III score in the “on” medicine state. While there was a significant therapeutic effect in the withdrawal medicine state. Considering that different types of TBS (iTBS/cTBS) over the related brain targets may produce a significant difference of therapeutic effect, we performed subgroup analysis based on iTBS/cTBS-brain targets in both the “on” and “off” medicine status, which found that iTBS- M1+DLPFC or cTBS-SMA did not significantly improve UPDRS-III score in the “on” medicine state. It is unexpected that facilitatory iTBS over the M1 did not improve total motor performance (17). Due to the limitation of the sample size in our study, the therapeutic effect of iTBS on the overall motor disorder in PD patients may be underestimated (type II error). Conversely, cTBS-SMA significantly improved the UPDRS-III score in the “off” medicine state. For two included studies, Ji et al. gave cTBS-SMA for consecutive 14 days, a total of 42 sessions, and the results showed that cTBS over the SMA had a significant improvement of UPDRS-III score with follow-up for 2 weeks (32), where change of UPDRS-III score reached a level that reflects a significant clinical improvement (53, 54), while in Eggers et al. work, single-session iTBS over the SMA did not significantly improve UPDRS-III score (28). Insufficient stimulation sessions may be an important reason. In our study, cTBS over the SMA improved the UPDRS-III score, which is consistent with previous studies that the improvement of motor symptoms was related to inhibiting the excitability of SMA (55–57). In terms of that cTBS-SMA significantly improved UPDRS-III in “off,” not in the “on,” medicine status, a possible mechanism is that dopamine has been suggested to have negative effects on the plasticity of the motor cortex in patients with PD (58). Most of the included studies in this meta-analysis defined “off” medicine state as anti-Parkinsonism medicine withdrawal status for at least 12 h, without considering the type of drug. It is necessary to select the scientific withdrawal time based on the different half-life of drugs and consider the equivalent dose of levodopa for confirming the difference of iTBS/cTBS over the corresponding brain targets in the “on” and “off” medicine state.
Upper limb motor dysfunction in patients with PD included a decrease concerning the speed and amplitude of movement, reaching and grasping deficits, and reduction of hand dexterity. Despite various upper limb dysfunctions that occur in PD, few studies reported treatment interventions for enhancing upper limb function (59). Our metaanalysis showed that TBS did not significantly improve the upper limb motor scores both in the “on” and “off” medicine state. Further analysis based on iTBS/cTBS-brain targets also found an insignificant difference among subgroups. PPT was chosen to explore upper limb movement and hand flexibility in two studies (23, 28), which has been demonstrated to correlate with disease severity, hand dexterity, and limitation of activity in patients with PD (60). Other studies selected the subitems of UPDRS-III concerning upper limb motor function (24, 25, 29). It is worth noting that Eggers et al. performed single-session cTBS over the SMA, and there was a significant difference in UPDRS-III score in the “off” medicine state (post-cTBS vs. pre-cTBS, p = 0.024), while did not have any significant effect in the “on” medicine status (28). As mentioned earlier, the possible mechanism may be the negative effect of dopaminergic drugs on the plasticity of the motor cortex. It is necessary to explore the efficacy of TBS on upper limb motor disorder in patients with PD by optimizing brain target and giving cumulative multiple-session TBS in the future.
For patients with PD, gait disorders and recurrent falls are common and cause disability in an advanced stage. Previous metaanalyses concluded modest efficacy of HF rTMS on motor performance in PD (61, 62). Controlled rTMS studies demonstrated positive effects on gait (63–65), suggesting more powerful stimulation protocols, such as TBS, could enhance efficacy (66, 67). Our results showed that TBS significantly improved the slowing of gait in PD in the “off” medicine state. Subgroup analysis based on iTBS/cTBS-brain targets found that iTBS-M1+DLPFC, not cTBS- SMA, had a significant therapeutic effect. Our results merely come from two studies (24, 32), and there was the limitation of a small sample size. In this metaanalysis, we failed to make a synthesis analysis for the date of freezing of gait (FOG). For PD patients, FOG is a refractory motor dysfunction resulting in an increased risk of falls. Two studies explored the efficacy of TBS on FOG by the gait parameter analysis (34, 68). The first study found that single-session iTBS- left premotor cortex did not improve FOG under normal medicine. The second study suggested that iTBS- SMA overall brought relative deterioration of gait, mainly in the time domain. The therapeutic effect of TBS on gait disorder in patients with PD needs to be further explored, and combined symptom scale of gaits and the gait parameter analysis are expected to be more effective for assessing improvement of gaits.
Depression is common in patients with PD, and it could even appear before the onset of motor symptoms. Previous studies regarding traditional rTMS found that HF rTMS over the DLPFC significantly improved PD depression, and the related guidelines also give recommendations for rTMS to intervene depression of patients with PD (13, 15–17). Our work found that iTBS-M1+DLPFC did not significantly improve the BDI score. However, subgroup analysis based on follow-up time found that it could bring short-term (within 2 weeks) therapeutic effect. For included two studies, Benninger et al. gave eight sessions of iTBS (within 2 weeks) over the M1+DLPFC (24), and Trung et al. performed six sessions of iTBS (within 1 week) over the left DLPFC (30). Whether more sessions and further optimized intervention targets can bring longer antidepressant effects requires further research.
Cognitive impairment and dementia are frequent in patients with PD (69). A growing number of researches support the view that cognitive decline in PD is mediated by degeneration and dysfunction of neural networks (70). Recent work assessing the efficacy of TBS in PD with mild cognitive impairment (PD-MCI) has shown mixed results. Hill et al. demonstrated that single-session iTBS-left DLPFC did not significantly improve working memory and executive function (31). Studies from Trung et al. and Lang et al. found that iTBS-left DLPFC had an insignificant effect on cognitive domain z-scores across time when comparing real with sham stimulation and correcting for multiple comparisons across cognitive domains (both received a total of six sessions iTBS within a week). However, the real iTBS group demonstrated a trend in the improvement of cognitive domain scores with 1-month follow-up compared with sham iTBS (30, 33). He and colleagues suggested that iTBS-left DLPFC for 10 consecutive weekdays brought significant improvement of repeatable battery for the assessment of neuropsychological status (RBANS) and Montreal cognitive assessment (MoCA) scores with a 3-month follow-up (p < 0.001 for both) (35). Considering that multiple-session iTBS over left DLPFC had a positive impact on cognitive scores, future research is promising.
It is worth mentioning that Bentley et al. explored the neurophysiology changes of DLPFC after iTBS on different deep brain stimulation (DBS) targets, subthalamic nucleus (STN), or globus pallidus interna (GPi) in seven patients with PD and found that GPi stimulation results in significantly greater theta power vs. STN stimulation (71). It is the first study that suggested TBS can be safely transmitted to human subcortical by DBS. A recent RCT demonstrated that TBS on DBS HF (200 Hz) and low-frequency (50 Hz) TBS with adapted stimulation amplitude were effective in the reduction of PD motor symptoms (akinesia, tremor, and rigidity) in 17 patients with PD (72), and had no serious adverse events.
Limitations and Future Directions
Our study has several limitations. Firstly, the total number of included participants was small, as mentioned above; hence interpreting results should be cautiously done. Secondly, interpretation of changes for behavioral assessment should be associated with reaching a level that reflects a significant clinical improvement. Third, several uncontrolled variables, such as disease stage, side of onset, age, and sex, exist that could confound the results and must be acknowledged. Lastly, we did not definite the optimal iTBS/cTBS-brain targets and parameters of TBS that could bring significant therapeutic effect due to the limitation of the data in these included studies. A further study combining TBS with different neuroimaging techniques may better discover the potential pathophysiological mechanisms of clinical benefit and optimize TBS treatment protocols. Compared with the figure of eight coils mainly used in our included studies, the double-cone coil has the advantage of a stronger magnetic field with higher penetration depth, which is worthy of further study. Additionally, future research should try to establish a more precise relationship between the TBS effect and PD patients' clinical and demographic characteristics, such as anti-Parkinsonism medicine regimen, stage of disease, side of onset, symptom subtype (e.g., specific cognitive domain impairment), age, and gender, for finding the optimal stimulation protocols for individualized TBS treatment. Lastly, multicenter, large sample research is necessary for the future to evaluate the application prospect of TBS on invasive brain stimulation for expanding the therapeutic window and enhancing clinical benefits in PD.
Conclusion
Our study demonstrated that cTBS-SMA could significantly improve the UPDRS-III score for patients with PD in the “off,” not in the “on,” medicine state, whereas TBS could not bring significant benefits to upper limb movement. ITBS- M1+DLPFC could significantly improve the slowing of gait in the “off” medicine status. Additionally, iTBS- M1+DLPFC has a short-term (within 2 weeks) therapeutic effect on PD depression. Since the limitations, such as small sample size and heterogeneity of assessment scale among included studies, further researches of a large sample, comprehensive evaluation, and multi-center excellent-designed RCTs are needed to confirm our research conclusions.
Data Availability Statement
The original contributions presented in the study are included in the article/supplementary material, further inquiries can be directed to the corresponding author.
Author Contributions
SZ developed the review concept. BC and TZ performed the data collection, analysis, and interpretation under the supervision of SZ and WZ. LS, YS, and WX contributed reagents, materials, and analysis. BC wrote the manuscript. All authors approved the final version of the manuscript for submission.
Funding
This work was supported by the Science Foundation of the Affiliated Hospital of North Sichuan Medical College (Grants No. 2019ZD014), the Primary Health Development Research Center of Sichuan Province Program (Grants Nos. SWFZ17-Z-13; SWFZ21-Y-29), and the Initial Scientific Research Foundation of Doctors of North Sichuan Medical College (Grants No. CBY16-QD08).
Conflict of Interest
The authors declare that the research was conducted in the absence of any commercial or financial relationships that could be construed as a potential conflict of interest.
Publisher's Note
All claims expressed in this article are solely those of the authors and do not necessarily represent those of their affiliated organizations, or those of the publisher, the editors and the reviewers. Any product that may be evaluated in this article, or claim that may be made by its manufacturer, is not guaranteed or endorsed by the publisher.
References
1. de Lau LML, Breteler MMB. Epidemiology of Parkinson's disease. Lancet Neurol. (2006) 5:525–35. doi: 10.1016/S1474-4422(06)70471-9
2. Kalia LV, Lang AE. Parkinson's disease. Lancet. (2015) 386:896–912. doi: 10.1016/S0140-6736(14)61393-3
3. Lang AE, Lozano AM. Parkinson's disease. First of two parts. N Engl J Med. (1998) 339:1044–53. doi: 10.1056/NEJM199810083391506
4. Ni Z, Chen R. Transcranial magnetic stimulation to understand pathophysiology and as potential treatment for neurodegenerative diseases. Transl Neurodegener. (2015) 4:22. doi: 10.1186/s40035-015-0045-x
5. Hallett M. Transcranial magnetic stimulation: a primer. Neuron. (2007) 55:187–99. doi: 10.1016/j.neuron.2007.06.026
6. Ridding MC, Rothwell JC. Is there a future for therapeutic use of transcranial magnetic stimulation? Nat Rev Neurosci. (2007) 8:559–67. doi: 10.1038/nrn2169
7. Kim JY, Chung EJ, Lee WY, Shin HY, Lee GH, Choe YS, et al. Therapeutic effect of repetitive transcranial magnetic stimulation in Parkinson's disease: analysis of [11C] raclopride PET study. Mov Disord. (2008) 23:207–11. doi: 10.1002/mds.21787
8. González-García N, Armony JL, Soto J, Trejo D, Alegría MA, Drucker-Colín R. Effects of rTMS on Parkinson's disease: a longitudinal fMRI study. J Neurol. (2011) 258:1268–80. doi: 10.1007/s00415-011-5923-2
9. Chou YH, Hickey PT, Sundman M, Song AW, Chen NK. Effects of repetitive transcranial magnetic stimulation on motor symptoms in Parkinson disease: a systematic review and meta-analysis. JAMA Neurol. (2015) 72:432–40. doi: 10.1001/jamaneurol.2014.4380
10. Zanjani A, Zakzanis KK, Daskalakis ZJ, Chen R. Repetitive transcranial magnetic stimulation of the primary motor cortex in the treatment of motor signs in Parkinson's disease: A quantitative review of the literature. Mov Disord. (2015) 30:750–8. doi: 10.1002/mds.26206
11. Yang C, Guo Z, Peng H, Xing G, Chen H, McClure MA, et al. Repetitive transcranial magnetic stimulation therapy for motor recovery in Parkinson's disease: a meta-analysis. Brain Behav. (2018) 8:e01132. doi: 10.1002/brb3.1132
12. Kim YW, Shin IS, Moon HI, Lee SC, Yoon SY. Effects of non-invasive brain stimulation on freezing of gait in parkinsonism: a systematic review with meta-analysis. Parkinsonism Relat Disord. (2019) 64:82–9. doi: 10.1016/j.parkreldis.2019.02.029
13. Li S, Jiao R, Zhou X, Chen S. Motor recovery and antidepressant effects of repetitive transcranial magnetic stimulation on Parkinson disease: a PRISMA-compliant meta-analysis. Medicine. (2020) 99:e19642. doi: 10.1097/MD.0000000000019642
14. Xie YJ, Gao Q, He CQ, Bian R. Effect of repetitive transcranial magnetic stimulation on gait and freezing of gait in parkinson disease: a systematic review and meta-analysis. Arch Phys Med Rehabil. (2020) 101:130–40. doi: 10.1016/j.apmr.2019.07.013
15. Xie CL, Chen J, Wang XD, Pan JL, Zhou Y, Lin SY, et al. Repetitive transcranial magnetic stimulation (rTMS) for the treatment of depression in Parkinson disease: a meta-analysis of randomized controlled clinical trials. Neurol Sci. (2015) 36:1751–61. doi: 10.1007/s10072-015-2345-4
16. Hai-Jiao W, Ge T, Li-Na Z, Deng C, Da X, Shan-Shan C, et al. The efficacy of repetitive transcranial magnetic stimulation for Parkinson disease patients with depression. Int J Neurosci. (2020) 130:19–27. doi: 10.1080/00207454.2018.1495632
17. Lefaucheur JP, Aleman A, Baeken C, Benninger DH, Brunelin J, Di Lazzaro V, et al. Evidence-based guidelines on the therapeutic use of repetitive transcranial magnetic stimulation (rTMS): an update (2014-2018). Clin Neurophysiol. (2020) 131:474–528. doi: 10.1016/j.clinph.2019.11.002
18. Suppa A, Huang YZ, Funke K, Ridding MC, Cheeran B, Di Lazzaro V, et al. Ten years of theta burst stimulation in humans: established knowledge, unknowns and prospects. Brain Stimul. (2016) 9:323–35. doi: 10.1016/j.brs.2016.01.006
19. Chu HT, Cheng CM, Liang CS, Chang WH, Juan CH, Huang YZ, et al. Efficacy and tolerability of theta-burst stimulation for major depression: A systematic review and meta-analysis. Prog Neuropsychopharmacol Biol Psychiatry. (2021) 106:110168. doi: 10.1016/j.pnpbp.2020.110168
20. Huang YZ, Edwards MJ, Rounis E, Bhatia KP, Rothwell JC. Theta burst stimulation of the human motor cortex. Neuron. (2005) 45:201–6. doi: 10.1016/j.neuron.2004.12.033
21. Rossi S, Antal A, Bestmann S, Bikson M, Brewer C, Brockmoller J, et al. Safety and recommendations for TMS use in healthy subjects and patient populations, with updates on training, ethical and regulatory issues: expert guidelines. Clin Neurophysiol. (2021) 132:269–306. doi: 10.1016/j.clinph.2020.10.003
22. Koch G, Brusa L, Carrillo F, Lo Gerfo E, Torriero S, Oliveri M, et al. Cerebellar magnetic stimulation decreases levodopa-induced dyskinesias in Parkinson disease. Neurology. (2009) 73:113–9. doi: 10.1212/WNL.0b013e3181ad5387
23. Eggers C, Fink GR, Nowak DA. Theta burst stimulation over the primary motor cortex does not induce cortical plasticity in Parkinson's disease. J Neurol. (2010) 257:1669–74. doi: 10.1007/s00415-010-5597-1
24. Benninger DH, Berman BD, Houdayer E, Pal N, Luckenbaugh DA, Schneider L, et al. Intermittent theta-burst transcranial magnetic stimulation for treatment of Parkinson disease. Neurology. (2011) 76:601–9. doi: 10.1212/WNL.0b013e31820ce6bb
25. Degardin A, Devos D, Defebvre L, Destée A, Plomhause L, Derambure P, et al. Effect of intermittent theta-burst stimulation on akinesia and sensorimotor integration in patients with Parkinson's disease. Eur J Neurosci. (2012) 36:2669–78. doi: 10.1111/j.1460-9568.2012.08158.x
26. Zamir O, Gunraj C, Ni Z, Mazzella F, Chen R. Effects of theta burst stimulation on motor cortex excitability in Parkinson's disease. Clin Neurophysiol. (2012) 123:815–21. doi: 10.1016/j.clinph.2011.07.051
27. Bologna M, Di Biasio F, Conte A, Iezzi E, Modugno N, Berardelli A. Effects of cerebellar continuous theta burst stimulation on resting tremor in Parkinson's disease. Parkinsonism Relat Disord. (2015) 21:1061–6. doi: 10.1016/j.parkreldis.2015.06.015
28. Eggers C, Günther M, Rothwell J, Timmermann L, Ruge D. Theta burst stimulation over the supplementary motor area in Parkinson's disease. J Neurol. (2015) 262:357–64. doi: 10.1007/s00415-014-7572-8
29. Vanbellingen T, Wapp M, Stegmayer K, Bertschi M, Abela E, Kübel S, et al. Theta burst stimulation over premotor cortex in Parkinson's disease: an explorative study on manual dexterity. J Neural Transm. (2016) 123:1387–93. doi: 10.1007/s00702-016-1614-6
30. Trung J, Hanganu A, Jobert S, Degroot C, Mejia-Constain B, Kibreab M, et al. Transcranial magnetic stimulation improves cognition over time in Parkinson's disease. Parkinsonism Relat Disord. (2019) 66:3–8. doi: 10.1016/j.parkreldis.2019.07.006
31. Hill AT, McModie S, Fung W, Hoy KE, Chung SW, Bertram KL. Impact of prefrontal intermittent theta-burst stimulation on working memory and executive function in Parkinson's disease: a double-blind sham-controlled pilot study. Brain Res. (2020) 1726:146506. doi: 10.1016/j.brainres.2019.146506
32. Ji GJ, Liu T, Li Y, Liu P, Sun J, Chen X, et al. Structural correlates underlying accelerated magnetic stimulation in Parkinson's disease. Hum Brain Mapp. (2020) 42:1670–81. doi: 10.1002/hbm.25319
33. Lang S, Gan LS, Yoon EJ, Hanganu A, Kibreab M, Cheetham J, et al. Theta-burst stimulation for cognitive enhancement in Parkinson's disease with mild cognitive impairment: a randomized, double-blind, sham-controlled trial. Front Neurol. (2020) 11:584374. doi: 10.3389/fneur.2020.584374
34. Brugger F, Wegener R, Baty F, Walch J, Kruger MT, Hagele-Link S, et al. Facilitatory rTMS over the supplementary motor cortex impedes gait performance in Parkinson patients with freezing of gait. Brain Sci. (2021) 11. doi: 10.3390/brainsci11030321
35. He W, Wang JC, Tsai PY. Theta burst magnetic stimulation improves parkinson's-related cognitive impairment: a randomised controlled study. Neurorehabil Neural Repair. (2021) 35:986–95. doi: 10.1177/15459683211041311
36. Page MJ, McKenzie JE, Bossuyt PM, Boutron I, Hoffmann TC, Mulrow CD, et al. The PRISMA 2020 statement: an updated guideline for reporting systematic reviews. BMJ. (2021) 372:n71. doi: 10.1136/bmj.n71
37. Hughes AJ, Daniel SE, Kilford L, Lees AJ. Accuracy of clinical diagnosis of idiopathic Parkinson's disease: a clinico-pathological study of 100 cases. J Neurol Neurosurg Psychiatry. (1992) 55:181–4. doi: 10.1136/jnnp.55.3.181
38. Maher CG, Sherrington C, Herbert RD, Moseley AM, Elkins M. Reliability of the PEDro scale for rating quality of randomized controlled trials. Phys Ther. (2003) 83:713–21. doi: 10.1093/ptj/83.8.713
39. Verhagen AP, de Vet HC, de Bie RA, Kessels AG, Boers M, Bouter LM, et al. The Delphi list: a criteria list for quality assessment of randomized clinical trials for conducting systematic reviews developed by Delphi consensus. J Clin Epidemiol. (1998) 51:1235–41. doi: 10.1016/S0895-4356(98)00131-0
40. de Morton NA. The PEDro scale is a valid measure of the methodological quality of clinical trials: a demographic study. Aust J Physiother. (2009) 55:129–33. doi: 10.1016/S0004-9514(09)70043-1
41. Gomes-Neto M, Saquetto MB, Silva CM, Carvalho VO, Ribeiro N, Conceição CS. Effects of respiratory muscle training on respiratory function, respiratory muscle strength, and exercise tolerance in patients poststroke: a systematic review with meta-analysis. Arch Phys Med Rehabil. (2016) 97:1994–2001. doi: 10.1016/j.apmr.2016.04.018
42. Li Y, Wei Q, Gou W, He C. Effects of mirror therapy on walking ability, balance and lower limb motor recovery after stroke: a systematic review and meta-analysis of randomized controlled trials. Clin Rehabil. (2018) 32:1007–21. doi: 10.1177/0269215518766642
43. Teasell R, Foley N, Salter K, Bhogal S, Jutai J, Speechley M. Evidence-based review of stroke rehabilitation: executive summary, 12th edition. Top Stroke Rehabil. (2009) 16:463–88. doi: 10.1310/tsr1606-463
44. Morris SB. Estimating effect sizes from pretest-posttest-control group designs. Organ Res Methods. (2007) 11:364–86. doi: 10.1177/1094428106291059
45. Portney LG, Watkins MP. Foundations of clinical research: applications to practice. Pearson/Prentice Hall Upper Saddle River, NJ (2009).
46. Higgins JP, Thompson SG. Quantifying heterogeneity in a meta-analysis. Stat Med. (2002) 21:1539–58. doi: 10.1002/sim.1186
47. Higgins JP, Thompson SG, Deeks JJ, DG A. Measuring inconsistency in meta-analyses. BMJ. (2003) 6:557–60. doi: 10.1136/bmj.327.7414.557
48. Huedo-Medina TB, Sanchez-Meca J, Marin-Martinez F, Botella J. Assessing heterogeneity in meta-analysis: Q statistic or I2 index? Psychol Methods. (2006) 11:193–206. doi: 10.1037/1082-989X.11.2.193
49. Mantel N, Haenszel W. Statistical aspects of the analysis of data from retrospective studies of disease. J Natl Cancer Inst. (1959) 22:719–48.
50. DerSimonian R, Laird N. Meta-analysis in clinical trials revisited. Contemp Clin Trials. (2015) 45:139–45. doi: 10.1016/j.cct.2015.09.002
51. Chung SW, Hill AT, Rogasch NC, Hoy KE, Fitzgerald PB. Use of theta-burst stimulation in changing excitability of motor cortex: a systematic review and meta-analysis. Neurosci Biobehav Rev. (2016) 63:43–64. doi: 10.1016/j.neubiorev.2016.01.008
52. Goetz CG, Tilley BC, Shaftman SR, Stebbins GT, Fahn S, Martinez-Martin P, et al. Movement disorder society-sponsored revision of the unified Parkinson's disease rating scale (MDS-UPDRS): scale presentation and clinimetric testing results. Mov Disord. (2008) 23:2129–70. doi: 10.1002/mds.22340
53. Schrag A, Sampaio C, Counsell N, Poewe W. Minimal clinically important change on the unified Parkinson's disease rating scale. Movement Disorders. (2006) 21:1200–7. doi: 10.1002/mds.20914
54. Shulman LM, Gruber-Baldini AL, Anderson KE, Fishman PS, Reich SG, Weiner WJ. The clinically important difference on the unified Parkinson's disease rating scale. Arch Neurol. (2010) 67:64–70. doi: 10.1001/archneurol.2009.295
55. Trošt M, Su S, Su P, Yen R-F, Tseng H-M, Barnes A, et al. Network modulation by the subthalamic nucleus in the treatment of Parkinson's disease. Neuroimage. (2006) 31:301–7. doi: 10.1016/j.neuroimage.2005.12.024
56. Bradberry TJ, Metman LV, Contreras-Vidal JL, van den Munckhof P, Hosey LA, Thompson JLW, et al. Common and unique responses to dopamine agonist therapy and deep brain stimulation in Parkinson's disease: an H215O PET study. Brain Stimul. (2012) 5:605–15. doi: 10.1016/j.brs.2011.09.002
57. Mure H, Tang CC, Argyelan M, Ghilardi MF, Kaplitt MG, Dhawan V, et al. Improved sequence learning with subthalamic nucleus deep brain stimulation: evidence for treatment-specific network modulation. J Neurosci. (2012) 32:2804–13. doi: 10.1523/JNEUROSCI.4331-11.2012
58. Kishore A, Popa T, Velayudhan B, Joseph T, Balachandran A, Meunier S. Acute dopamine boost has a negative effect on plasticity of the primary motor cortex in advanced Parkinson's disease. Brain. (2012) 135:2074–88. doi: 10.1093/brain/aws124
59. Chung CL, Mak MK. Effect of repetitive transcranial magnetic stimulation on physical function and motor signs in Parkinson's disease: a systematic review and meta-analysis. Brain Stimul. (2016) 9:475–87. doi: 10.1016/j.brs.2016.03.017
60. Proud EL, Morris ME. Skilled hand dexterity in Parkinson's disease: effects of adding a concurrent task. Arch Phys Med Rehabil. (2010) 91:794–9. doi: 10.1016/j.apmr.2010.01.008
61. Fregni F, Simon DK, Wu A, Pascual-Leone A. Non-invasive brain stimulation for Parkinson's disease: a systematic review and meta-analysis of the literature. J Neurol Neurosurg Psychiatry. (2005) 76:1614–23. doi: 10.1136/jnnp.2005.069849
62. Elahi B, Elahi B, Chen R. Effect of transcranial magnetic stimulation on Parkinson motor function–systematic review of controlled clinical trials. Mov Disord. (2009) 24:357–63. doi: 10.1002/mds.22364
63. Lefaucheur JP, Drouot X, Von Raison F, Menard-Lefaucheur I, Cesaro P, Nguyen JP. Improvement of motor performance and modulation of cortical excitability by repetitive transcranial magnetic stimulation of the motor cortex in Parkinson's disease. Clin Neurophysiol. (2004) 115:2530–41. doi: 10.1016/j.clinph.2004.05.025
64. von Papen M, Fisse M, Sarfeld AS, Fink GR, Nowak DA. The effects of 1 Hz rTMS preconditioned by tDCS on gait kinematics in Parkinson's disease. J Neural Transm. (2014) 121:743–54. doi: 10.1007/s00702-014-1178-2
65. Du J, Tian L, Liu W, Hu J, Xu G, Ma M, et al. Effects of repetitive transcranial magnetic stimulation on motor recovery and motor cortex excitability in patients with stroke: a randomized controlled trial. Eur J Neurol. (2016) 23:1666–72. doi: 10.1111/ene.13105
66. Khedr EM, Rothwell JC, Shawky OA, Ahmed MA, Hamdy A. Effect of daily repetitive transcranial magnetic stimulation on motor performance in Parkinson's disease. Mov Disord. (2006) 21:2201–5. doi: 10.1002/mds.21089
67. Lomarev MP, Kanchana S, Bara-Jimenez W, Iyer M, Wassermann EM, Hallett M. Placebo-controlled study of rTMS for the treatment of Parkinson's disease. Mov Disord. (2006) 21:325–31. doi: 10.1002/mds.20713
68. Tard C, Devanne H, Defebvre L, Delval A. Single session intermittent theta-burst stimulation on the left premotor cortex does not alleviate freezing of gait in Parkinson's disease. Neurosci Lett. (2016) 628:1–9. doi: 10.1016/j.neulet.2016.05.061
69. Marder K. Cognitive impairment and dementia in Parkinson's disease. Mov Disord. (2010) 25 Suppl 1:S110–116. doi: 10.1002/mds.22787
70. Gratwicke J, Jahanshahi M, Foltynie T. Parkinson's disease dementia: a neural networks perspective. Brain. (2015) 138:1454–76. doi: 10.1093/brain/awv104
71. Bentley JN, Irwin ZT, Black SD, Roach ML, Vaden RJ, Gonzalez CL, et al. Subcortical intermittent theta-burst stimulation (iTBS) increases theta-power in dorsolateral prefrontal cortex (DLPFC). Front Neurosci. (2020) 14:41. doi: 10.3389/fnins.2020.00041
Keywords: Parkinson's disease, repetitive transcranial magnetic stimulation, theta burst stimulation, non-invasive brain stimulation, meta-analysis
Citation: Cheng B, Zhu T, Zhao W, Sun L, Shen Y, Xiao W and Zhang S (2022) Effect of Theta Burst Stimulation-Patterned rTMS on Motor and Nonmotor Dysfunction of Parkinson's Disease: A Systematic Review and Metaanalysis. Front. Neurol. 12:762100. doi: 10.3389/fneur.2021.762100
Received: 21 August 2021; Accepted: 10 December 2021;
Published: 12 January 2022.
Edited by:
Fabio Godinho, University of São Paulo, BrazilReviewed by:
Erwin Montgomery, McMaster University, CanadaWilliam Hutchison, University Health Network (UHN), Canada
Talyta Cortez-Grippe, Toronto Western Hospital, Canada in collaboration with reviewer WH
Copyright © 2022 Cheng, Zhu, Zhao, Sun, Shen, Xiao and Zhang. This is an open-access article distributed under the terms of the Creative Commons Attribution License (CC BY). The use, distribution or reproduction in other forums is permitted, provided the original author(s) and the copyright owner(s) are credited and that the original publication in this journal is cited, in accordance with accepted academic practice. No use, distribution or reproduction is permitted which does not comply with these terms.
*Correspondence: Shushan Zhang, c3VzYW40NDhAMTYzLmNvbQ==
†These authors have contributed equally to this work and share first authorship