- 1Research Group MOVANT, Department of Rehabilitation Sciences and Physiotherapy, Faculty of Health Sciences, University of Antwerp, Wilrijk, Belgium
- 2M2OCEAN Lab, The Multidisciplinary Motor Centre Antwerp, Faculty of Health Sciences, University of Antwerp, Edegem, Belgium
- 3RevArte Rehabilitation Hospital, Edegem, Belgium
- 4Department of Neurology, Neurovascular Reference Center, Antwerp University Hospital, Edegem, Belgium
- 5Research Group Translational Neurosciences, Faculty of Medicine and Health Sciences, University of Antwerp, Wilrijk, Belgium
- 6Department of Rehabilitation Medicine and Amsterdam Neuroscience, Amsterdam Movement Sciences, Amsterdam University Medical Centre, Amsterdam, Netherlands
- 7Department of Physical Therapy and Human Movement Sciences, Feinberg School of Medicine, Northwestern University, Chicago, IL, United States
- 8Department of Neurorehabilitation, Amsterdam Rehabilitation Research Centre, Reade, Amsterdam, Netherlands
Introduction: Although most stroke survivors show some spontaneous neurological recovery from motor impairments of the most-affected leg, the contribution of this leg to standing balance control remains often poor. Consequently, it is unclear how spontaneous processes of neurological recovery contributes to early improvements in standing balance.
Objective: We aim to investigate (1) the time course of recovery of quiet stance balance control in the first 12 weeks poststroke and (2) how clinically observed improvements of lower limb motor impairments longitudinally relate to this limb's relative contribution to balance control.
Methods and Analysis: In this prospective longitudinal study, a cohort of 60 adults will be recruited within the first 3 weeks after a first-ever hemispheric stroke and mild-to-severe motor impairments. Individual recovery trajectories will be investigated by means of repeated measurements scheduled at 3, 5, 8, and 12 weeks poststroke. The Fugl-Meyer Motor Assessment and Motricity Index of the lower limb serve as clinical measures of motor impairments at the hemiplegic side. As soon as subjects are able to stand independently, bilateral posturography during quietly standing will be measured. First, the obtained center-of-pressure (COP) trajectories at each foot will be used for synchronization and contribution measures that establish (a-)symmetries between lower limbs. Second, the COP underneath both feet combined will be used to estimate overall stability. Random coefficient analyses will be used to model time-dependent changes in these measures and, subsequently, a hybrid model will be used to investigate longitudinal associations with improved motor impairments.
Discussion: The current study aims to investigate how stroke survivors “re-learn” to maintain standing balance as an integral part of daily life activities. The knowledge gained through this study may contribute to recommending treatment strategies for early stroke rehabilitation targeting behavioral restitution of the most-affected leg or learning to compensate with the less-affected leg.
Introduction
Background
Stroke is a major cause for acquired serious disability in adults worldwide (1). It is well known that stroke affects standing balance, for example posturographic studies revealed that stroke survivors show increased body sway reflected by center-of-pressure (COP) movements (2–5). Recovering the ability to maintain standing balance is seen as a prerequisite for regaining walking independence (6), and residual deficits are associated with limited community ambulation (7, 8) and falls (9–11). Accordingly, falls remain a major problem with approximately 50 to 70% of community-dwelling stroke survivors experiencing a fall recently after being discharged home from rehabilitation facilities (9, 10, 12). As such, deficient standing balance control may contribute to chronic disability and fall-related injury resulting in greater economic costs due to the need of care (13) and poor quality of life (14).
Improving stroke rehabilitation services requires a profound understanding about the behavioral changes underlying impaired as well as improved balance control execution, together with the time windows in which they develop. This may enable clinicians to define appropriate treatment targets and rehabilitation goals early onwards. From this perspective, particularly explorative longitudinal research with repeated measurements early in time is warranted to distinguish between behavioral restitution and compensation when control of standing balance is restored (15, 16). As stroke recovery is a complex process that likely involves both spontaneous and learning-dependent mechanisms (17, 18), this research should be based on a commonly shared framework for classifying and using uniform terminology.
The WHO's international classification of function, disability and health (ICF) model may serve as such framework by categorizing the consequences of stroke in terms of body functions, activities and participation (17, 19). Following the ICF, we will discuss available literature and, subsequently, the design of an ongoing observational study into the time course and mechanisms underlying standing balance recovery. For this purpose, we distinguish between recovery of motor impairments of the most-affected lower limb (i.e., ICF level of body functions) and an improved ability to maintain standing balance as an integral part of daily life activities (i.e., ICF level of activities).
Time Course of Lower Limb Recovery
Unfortunately, only few longitudinal studies have been designed to investigate prospectively the time course of recovery of lower limb function (20–23) and activities (24–26) early after stroke. These studies suggest that recovery of motor impairments, such as synergy-dependency (20, 22, 23) and weakness (21, 23), mainly occurs in the first 5 weeks and levels off between 8 to 12 weeks poststroke (20–22). However, most survivors experience residual impairments in dissociating voluntary foot and leg movements from intra-limb synergies (27, 28) and muscle strength (23) which is associated with a decreased contribution of this leg to standing balance control (29–32). These early time-dependent changes remain poorly understood, but can be conceptualized as spontaneous neurological recovery as reflected by the passage of time (23).
Similar regularities in recovery patterns of activities involving the lower limbs have been shown. Likewise, most improvements in walking (6, 24–26) and other daily life activities (21, 23) were seen in the first weeks and regaining standing balance is found imperative to such recovery (6). One might suggest that spontaneous neurological recovery is associated with, if not determinant for these rapid improvements. This would mean that the ability to complete these tasks is restored with the same movement repertoire, or quality, the patient had before the stroke (i.e., behavioral restitution) (18, 19, 33). However, previous longitudinal research is largely limited to outcomes showing the mere accomplishment on these tasks. As these measures are unable to discriminate how the task is performed (19, 34, 35), little has been learned so far about the time course of recovery regarding qualitative aspects of movement when standing balance is restored.
Mechanisms Underlying Recovery of Standing Balance Control
As recently suggested (28), a number of severely affected subjects fail to show neurological recovery (28), leaving them entirely dependent on learning to use alternative ways to maintain their standing balance (i.e., behavioral compensation). However, even “well-recovered” patients with near normal clinical scores may show a disproportional reliance on the less-affected leg to balance control (31, 36). This is illustrated by more recent posturographic studies showing that bilateral COP displacements at the feet separately, as a reflection of the anticipatory modulation of ankle muscle activity aiming to minimize body sway (37, 38), are poorly synchronized (32, 39) and unequal (29–31, 40, 41) as compared to healthy controls. It seems that neurological recovery, even if occurring, is rarely complete and an even larger portion of survivors may depend on using compensation strategies to deal with residual impairments.
In favor of this notion, few longitudinal studies did show that standing balance improves in many patients without any concomitant improvements in anticipatory control of most-affected leg muscles in response to rapid arm movements (34, 42) or without restitution of symmetric exertion of corrective COP movements during unperturbed, quiet stance (2, 30, 40). However, these studies did unfortunately not start in the first weeks and assessed subjects at only few arbitrarily chosen time-points, leaving longitudinal associations with neurological recovery entirely underexplored.
As it remains unknown how stroke survivors “re-learn” to maintain standing balance in the early recovery phase when motor functions at the hemiplegic side improve, there is a need for early-started longitudinal research incorporating sensitive measures of task performance next to clinical scales. These studies may eventually progress our understanding of how spontaneous neurological recovery contributes to the re-acquisition of bipedal balance skills early after stroke onset.
Objectives
In the current manuscript, we describe an observational cohort study that aims to prospectively investigate the time course of recovery of quiet stance balance control in the first 12 weeks after a first-ever hemispheric stroke. The relatively low functional demands of this condition (as compared to perturbed stance or walking) enables us to describe the process of balance skill re-acquisition even in more-severely impaired subjects and in the time window of spontaneous neurological recovery. Simultaneously, we will clinically observe the time course of recovery of lower limb motor impairments to investigate longitudinal associations with this leg's contribution to balance control.
The pre-defined research questions and hypotheses are:
• What is the time course of recovery in the first 12 weeks poststroke in terms of balance control (a-)symmetries and overall stability during quiet stance? (i.e., project A).
First, we hypothesize that improvements in balance control symmetries toward values seen in healthy controls, as assessed through bilateral posturography (i.e., between-limb synchronization and contribution measures of corrective COP movements), are restricted to the first 5 weeks poststroke. Second, we hypothesize that an improved ability to maintain a stable standing position following traditional posturographic measures of body sway (i.e., stance stability) may develop beyond the time window and up until 12 weeks poststroke. As such, these later changes are hypothesized to reflect compensatory body stabilization exerted through the less-affected leg.
• How are improvements in muscle synergies and ankle strength of the most-affected leg longitudinally associated with this leg's contribution to quiet stance balance control in the first 12 weeks poststroke? (i.e., project B).
First, we hypothesize that clinical improvements in muscle synergies (i.e., Fugl-Meyer Motor Assessment) and strength (i.e., Motricity Index) at the hemiplegic side are mainly seen within the first 5 weeks poststroke, in accordance with previous longitudinal studies investigating time-dependent change (20, 23). Second, we hypothesize to find within this time window longitudinal associations between recovery on these scales and improved between-limb symmetry in balance control. This means that clinically observed improvements in motor impairments relate over the first weeks to an improved ability of this leg to contribute to corrective COP movements while standing quietly.
Methods
The protocols of prospective repeated-measurement studies from which participants will be recruited for this longitudinal investigation are registered online (ClinicalTrials.gov Identifier: NCT03728036; NCT03727919). These studies are designed and executed in adherence to the STROBE statements and were approved by the local Ethics Committee of the Antwerp University Hospital (Edegem, Belgium) in accordance with the declaration of Helsinki (main ethics committee protocol number: 18/25/305; Belgium trial registration number: B300201837010). The responsible ethics committees of all other involved clinical sites were asked for advice and additional approval before the study started.
Participants
In total, this study will include a cohort of 60 patients after a first-ever hemispheric stroke of ischemic or hemorrhagic cause within, at most, 3 years of recruitment. This cohort will be recruited from the stroke units at the University Hospital Antwerp (Edegem, Antwerp, Belgium), the GZA Hospital campus St Augustinus (Wilrijk, Antwerp, Belgium) and the General Hospital Geel (Antwerp, Belgium). All cooperating hospitals are located in the larger Antwerp region in Flanders, Belgium. Stroke survivors will be screened and asked to participate as soon as possible and within the first 3 weeks after stroke onset. Only survivors who are, or will be admitted to one of the involved rehabilitation facilities (RevArte, Edegem, Antwerp, Belgium or the rehabilitation hospital Geel, Geel, Antwerp, Belgium) for inpatient treatment (eventually followed by outpatient treatment) are considered for inclusion, as the repeated measurements will be performed there. In addition, ten middle-aged (i.e., 45 to 65 years) healthy adults (gender equally distributed) with no known musculoskeletal or neurological injury or illness that may affect balance control will be recruited to serve as controls for the interpretation of posturographic measures of quiet stance balance control.
Before entry, an information brochure about the study's aim, content and potential risks, together with information about the investigators is provided to each prospective subject (and if adequate to her/his family). If the subject feels sufficiently informed and agrees to participate, an informed consent is signed and eligibility is determined according to the following criteria.
Inclusion Criteria
• A first-ever, CT- or MRI-confirmed, supra-tentorial stroke in the anterior or middle cerebral arteria territory of ischemic or hemorrhagic cause;
• Impaired motor functions of the lower limb, defined as a score of > 0 on the NIHSS item 6 (i.e., at least “drift of the leg within 5 s without a fall”) within 3 days after stroke onset (or self-reported if data is missing), and a MI-LE score of ≤ 91 (i.e., at least “movement against resistance but weaker” in one item) at the moment of inclusion;
• Age between 18 and 90 years;
• Sufficient motivation to participate.
Exclusion Criteria
• Dependent in daily life activities before stroke onset, defined as a pre-morbid Functional Ambulation Category score of <4 and a modified Rankin Scale score of >1;
• Having a preexisting orthopedic or neurological condition that affects motor functions of the lower limbs and/or standing balance control;
• Severe cognitive or communication deficits that may hinder informed consent and execution of the study;
• Not Dutch, German or English speaking prior to the stroke incident.
Design
The current recovery study is a non-interventional observational study meaning that no systematic interventions are applied next to usual care. For the duration of inpatient rehabilitation, this consists of 30- to 60-min sessions of physical and occupational therapy each workday following local guidelines.
After eligible subjects are included within the first 3 weeks poststroke, serial measurements will be scheduled at 3, 5, 8, and 12 weeks poststroke. Clinical evaluations are performed by the same assessor for each participant to decrease the effect of inter-rater variability when measuring within-subject change. In total, two physical therapists are available for clinical testing who underwent a training period to standardize assessment procedures prior to the study start. As soon as subjects are able to stand independently barefoot over at least 30 seconds, bilateral posturography will be performed during a standardized quiet stance task. For this purpose, either two separate force-plates (Type OR 6-7, AMTI, MA, US; measurement frequency 1 kHz) arranged in a side-by-side configuration as part of a stationary movement analysis laboratory (M2OCEAN, University Hospital Antwerp, Edegem, Belgium) or a portable pressure-plate system (0.5 m footscan 3D, RS Scan, Belgium; measurement frequency 500 Hz) will be used. This provides the advantage of executing this study in multiple (clinical) settings to facilitate recruitment and continuous data acquisition. Importantly, repeated within-subject measurements are always performed with the same equipment and these measurements are performed by a trained physiotherapist who is educated in using the measuring instruments.
Data Collection and Processing
During the uptake procedure, the participant's demographics including sex and age together with information about the stroke lesion in terms of type (i.e., infarct or bleeding) and side (i.e., left or right hemisphere affected) is collected. Length of stay in the rehabilitation hospital and discharge destination will be recorded throughout the study.
Before each balance assessment, the subject's body weight and length is measured. Next, the subject's bare feet will be positioned in parallel with a 8.4 cm heel-to-heel distance and in a 9° toe-out angle on the measuring plate (40). Manual support will be provided during standing up until the subject feels comfortable to stand independently. Now, the subject is instructed to maintain this position for 40 s with the arms alongside the trunk and the eyes open. They are asked to look straight ahead at a visual target at an approximately 2-meter distance. Subjects are encouraged to adopt a spontaneous, stable posture rather than standing as symmetric as possible. Three trials will be performed and if the subject got distracted, lost balance or moved the arms or head in a way that is not related to balance, the trial is excluded.
From each eligible trial, the first 10 s will be removed to prevent the influence of starting effects. Previously it was shown that 30 s quiet stance registration yield excellent test-retest reliability (43). Limb-specific COP trajectories will be calculated based on raw force components and low-pass filtered using a zero-lag, second-order Butterworth filter with a 10 Hz cutoff frequency. For the calculation of synchronization and contribution measures, these trajectories will be split into an anteroposterior (AP) and mediolateral (ML) signal based on the orientation of the feet by rotating the reference system. The COP underneath both feet combined will be used to estimate overall stance stability. For this purpose, we focus on velocity parameters as these are shown to have greatest reliability (44, 45) which has been confirmed for bilateral posturography in stroke survivors (46). These studies also show that usually two to three trials are sufficient to reach reliable data (45, 46) which is of relevance for this project since instability is pronounced early after stroke allowing to acquire only few standing attempts. All data processing (and parameter calculation, as documented below) will be done by using custom-made Matlab scripts (The MathWorks Inc., MA, USA).
Outcomes
Poststroke recovery is a complex matter and the inconsistent use of terminology in the literature creates opportunities for confusion. Therefore, we first define the chosen outcome variables following the ICF framework (see Figure 1) and differentiate between dependent and independent variables for subsequent analyses.
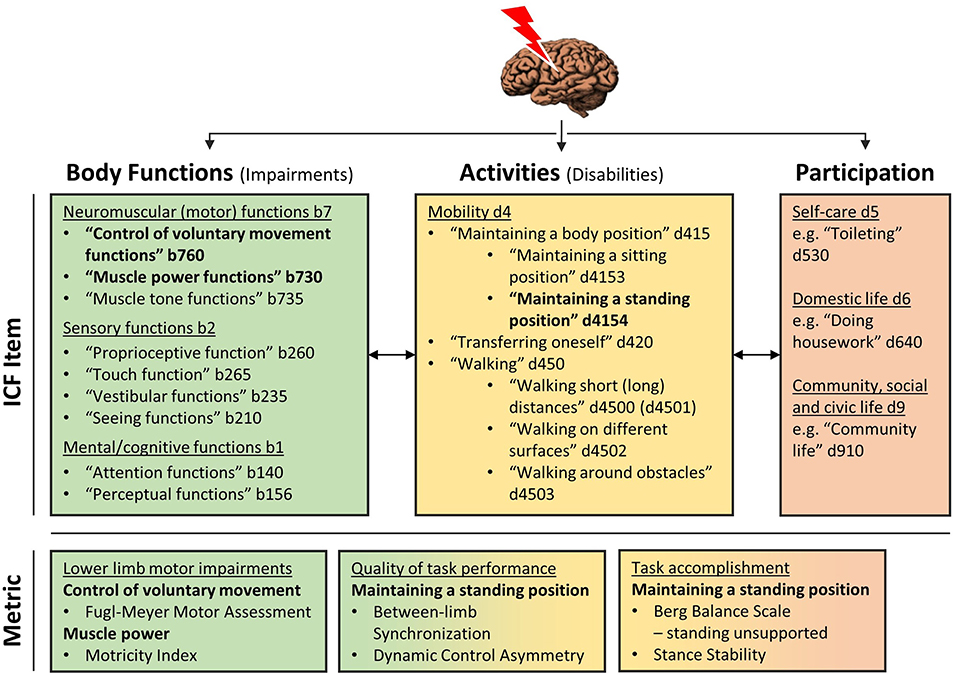
Figure 1. ICF model. The upper panel provides an overview of ICF items that are required for (i.e., level of body functions, colored green) and dependent on (i.e., level of activities, colored yellow, and participation, colored red) standing balance control. The items that are directly assessed in the current study are highlight in bolt. In the lower panel metrics corresponding to these items of interest are provided. The color-coding indicates that metrics of quality of task performance and task accomplishment are both primarily situated on the level of activities, whereas the first indicates how movement execution functions (i.e., level of body functions) are assembled to execute a balance task. Contrary, task accomplishment means if a subjects is successful in maintaining standing balance, irrespective of the underlying control strategy, as a determinant of independence in daily life activities and participation (i.e., level of participation).
Independent Variables of Outcome
The main independent variables for modeling longitudinal associations with balance control measures (i.e., project B) are time-dependent, or dynamic, and situated on the ICF level of body functions (see Table 1). On this level, recovery means the restitution of rudimental functions of movement execution (19, 35), such as synergy-dependency and strength which we define as follows.
Dynamic Variables
Although different definitions and constructs of muscle synergies exists in the literature (47), we refer to synergies as the clinical phenomenon of “pathological intra-limb synergies” meaning the loss of independent joint control leading to the emergence of stereotypical flexor and extensor movements (48) (i.e., ICF item b760 “Control of voluntary movement functions”). As such, muscle synergies are defined as an “increased co-activation between muscles in the paretic limb that can be elicited voluntarily” (48). Second, muscle strength is defined as the ability to produce a “maximum voluntary force or torque” through a muscle or muscle group contraction around a single joint (i.e., ICF item b730 “Muscle power functions”) (49). The following standardized clinical scales will be used to address these functions.
• Fugl Meyer motor assessment–lower extremity (FMA-LE): Muscle synergies will be assessed by means of scores on the FMA-LE, ranging from 0 (i.e., unable to move the limb or evoke tendon reflexes) to 34 points (i.e., able to selectively flex the knee and ankle joint in standing and normal reflexes). This scale has been reported to have excellent inter-rater [Pearson's r = 0.96 (50); ICC = 0.91, 0.97–1.0 95% CI (51)] and intra-rater reliability [ICC = 0.99, 0.91–1.0 95% CI (51)] when assessed in stroke survivors. Moreover, we use the standardization method of See et al. (52) to improve scoring consistency.
• Motricity Index–lower extremity (MI-LE): Muscle strength in hip flexion, knee extension and ankle dorsiflexion direction will be assessed with the MI-LE. Active range of motion and force against manual resistance will be compared between both sides and evaluated with scores varying between 0 (i.e., no voluntary muscle contraction) and 33 points (i.e., full movement against gravity and equal strength) for each item. This scale has shown excellent intra-rater [ICC = 0.93, 0.84–0.97 95% CI (53); Spearman's rho = 0.87 (54)] and inter-rater reliability [Spearman's rho = 0.87 (54)] in stroke survivors.
Fixed Variables
Fixed, or time-dependent variables that are hypothesized to be potential confounders will be used as additional covariates. This includes the subject's age and gender at inclusion, as well as their Body Mass Index (BMI) (see Table 1). Body weight is serially assessed at each occasion and if significant fluctuations are seen over time, the BMI may be added as a dynamic variable. In addition, the stroke type and side will be added. Lastly, the used equipment for serial subject-specific measurements will be evaluated as a potential confounder.
Dependent Variables of Outcome
Dependent variables of outcome will be investigated in this study on the time course of recovery (i.e., project A) and longitudinal associations (i.e., project B). These outcomes are situated on the ICF level of activities where recovery comprises a general improvement in the ability to execute purposeful movements in a task context (19, 35). In the current study, the task of maintaining a quiet standing posture (i.e., ICF item d4154) will be evaluated which is defined as “the ability to control the body's center-of-mass relative to the base of support in fairly predictable and non-changing conditions” (55). For this purpose, we use and distinguish metrics addressing the quality of performance from those showing the mere accomplishment on this task.
Quality of Task Performance
Quality of task performance is “defined through a direct comparison of a patient's motor execution of a task […] to able-bodied control subjects” (35). This means that the closer the movement matches those seen in controls, the better the quality. With regard to standing balance, quality of performance is best reflected by measures that establish the (a-)symmetry between the most- and less-affected side considering that healthy balance control is characterized by equal output generated through the legs in the form of corrective COP movements (39, 56, 57). For this purpose, synchronization and contribution measures will be calculated that show how well both limbs act together (32, 39, 56) and are equally involved (31, 57) in maintaining standing balance. As COP in the ML direction is less meaningful for bipedal balance control (56), we focus on the sagittal plane.
• Between-limb synchronization: Between-limb synchronization is a measure of the temporal structure and similarity between bilateral COP (39, 56). For this purpose, the mean position is subtracted from left and right AP COP trajectories and, next, a cross-correlation at zero-phase leg on a frame-by-frame basis is calculated. This measure therefore shows how well COP displacements are alike, or synchronized.
• Dynamic control asymmetry (DCA): DCA is a symmetry index of the root mean square (RMS) of the AP COP velocities for each leg separately (31, 57). A score of zero indicates equal contribution of both legs to balance control and positive or negative values indicating a relatively larger involvement of the less- or most-affected leg, respectively. We use the following equation: Symmetry index: 2x (RMS COP AP velocity less-affected- RMS COP AP velocity most-affected) / (RMS COP AP velocity less-affected + RMS COP AP velocity most-affected).
Task Accomplishment
Metrics on task accomplishment are designed to show if a patient re-acquired the ability to complete the task irrespective of the underlying control strategy. With regard to standing balance, this is exemplified first by the level of independence following clinical scales and second by (traditional) posturographic measures of body sway that show how well a subject can stabilize their center-of-mass within the base of support. Therefore, these outcome variables will be used to address the process of regaining and optimizing the ability to maintain standing balance.
• Berg Balance Scale - standing unsupported item (BBS-s): The BBS-s assesses the ability to maintain assesses the ability to maintain a quiet standing posture without using the arms or support by another person. A score is assigned based on the level of independence needed to complete this task, where a score of 0 indicates no standing ability and a score varying between 1 and 4 indicate independent stance over 30 s to 2 min. The BBS has been reported to have excellent internal consistency as reported by a systematic review (58).
• Stance stability: To investigate overall stability when subjects attempt to stand quietly, we will calculate the root mean square (RMS) velocity of the net COP (i.e., combining two feet together without correction for feet orientation) in AP and ML direction. As the position of the net COP and the vertical representation of the body's center-of-mass correlate (59) these metrics reflect the ability to stand with minimal body sway.
Data Analysis
Descriptive Analysis
Subject's demographics at baseline together with lesion characteristics will be descriptively analyzed. The length of stay in the inpatient rehabilitation facility and discharge destination will be reported. In addition, adherence to the study protocol will be illustrated by reporting the number of subjects leaving the study prematurely and reasons for dropping out entirely or missing assessments. We will use the BBS-s to show how soon subjects recovered standing balance and were able to participate in posturographic assessments.
Statistical Analysis
Project A
In project A, we aim to investigate time-dependent changes in metrics of quiet stance balance control. To estimate how each parameter is changing as a function of time poststroke, we use a random coefficient analysis (or mixed model analysis) with “time” of measurements as the main fixed effect (JMP Pro, version 15). Additionally, “time” will be entered as an independent covariate in form of an subject-specific slope (i.e., the interaction term “participant*time”) to adjust for dependency of repeated observations. However, the greatest advantage of this method is its flexibility in dealing with missing values. The latter may result from subjects being unable to stand at first occasions, being unavailable due to hospital discharge or transfers, or by no longer corresponding to eligibility criteria for example due to a recurrent stroke or other sudden medical condition (potentially) affecting outcome variables. Moreover, the value on the addressed metric at 3 weeks poststroke will be added to account for inter-subjects variability.
In addition, fixed covariates that are hypothesized to be potential confounders will be entered in the model. This includes “gender,” “age” and “BMI” considering their influence on standing balance control. [i.e., females and elderly tend to show greater body sway (60) and obesity is associated with instability (61)]. Second, stroke “side” and “type” will be added, as right-sided lesions typically result in greater balance deficits (62) and subjects with hemorrhagic strokes may display delayed recovery (63). Lastly, “equipment” is added as a potential confounder considering technical variations in measuring instruments.
Statistical analysis of the difference in each measure of quiet stance balance control between subjects and healthy controls will be performed using the Mann-Whitney U test for each measuring system separately.
Project B
After describing the recovery time course, we aim to investigate longitudinal associations between motor impairments in terms of leg muscle synergies (i.e., FMA-LE) and ankle strength (i.e., ankle item of the MI-LE) serving as independent variables, and the DCA which is the dependent variable. First, we analyze the pattern of neurological recovery following these clinical scales by using similar methods as outlined above. Second, we will apply a recently discussed hybrid model (64) to investigate longitudinal associations over the first 12 weeks poststroke. This method has the advantage of disentangling the between- and within-subject effects of this relation.
The between-subjects covariate score is determined as the individual average value over time of the independent variables which reveals the association irrespective of the development over time. On the other hand, the within-subject covariate is calculated as the observed value at each time point minus the individual average (i.e., deviation score). By this, we can estimate whether longitudinal changes of the dependent and independent variables within a subject are associated, i.e., are increasing scores on the FMA-LE or MI-LE ankle item related to changes following the DCA. Similar covariates will be entered in the model and tested on significance.
For all statistical tests, the likelihood ratio test will be used to examine the need to enter random effects into the model and the Wald test will be used to obtain P-values for regression coefficients in the final model. A 2-tailed significance level of 0.05 will be used for all analyses.
Sample Size Justification
To the best of our knowledge, this is the first study to prospectively investigate changes in the variables over time early after stroke. This certainly limits the effectiveness of a sample size determination based on a power analysis. Therefore, we determine and justify our sample size of n = 60 based on recruitment (2.2 participants/month) and drop-out (15%) rates as seen during the first year of recruitment. Considering that we will include not more than 3 to 4 (main) covariates in our random coefficient and hybrid models, we meet the “rule of thumb” saying that 10 subjects per variable are sufficient to perform bivariate and multivariate regression analyses.
Trial Status
Participant recruitment began in January 2019 in the University Hospital Antwerp and the RevArte rehabilitation hospital, in the GZA Sint-Augustinus hospital in April 2019 with a temporal suspension of recruitment in all involved sites between March and September 2020 due to Covid-19 measures. In response, an additional partnership with the General Hospital Geel in January 2021 was set-up. By now (October 2021), 52 stroke survivors were recruited indicating feasibility of reaching the desired sample size within the proposed recruitment period.
Discussion
In this manuscript, we describe the design of an ongoing observational study with repeated measurements in time. This study aims to prospectively investigate individual recovery trajectories in a cohort of 60 mild-to-severely impaired subjects early after a first-ever, ischemic or hemorrhagic hemispheric stroke. Bilateral posturography will be used to measure balance control (a-) symmetries and overall stability during a quiet stance task to investigate the time course of recovery following these posturographic measures within subjects (i.e., project A) and, subsequently, longitudinal associations with recovery of lower limb motor impairments (i.e., project B). The knowledge gained through this study may contribute to our understanding of how progress of time as a reflection of spontaneous recovery contributes to regaining standing balance control through the most-affected leg, as well as dependency on compensatory stabilization exerted through the less-affected leg.
Time Course of Lower Limb Recovery
Recent upper limb recovery studies (65, 66) attest to the effectiveness of incorporating sensitive and specific task performance measures into longitudinal research. Based on repeated kinematic measures of a reaching task, it was shown that recovery of movement quality with the hemiplegic arm plateaus in most patients over the first 5 weeks poststroke (65, 66). This suggests that further task improvements are most likely explained on the basis of compensatory mechanisms, such as increased trunk movements to assist arm and hand transport (19). If assuming that neurological recovery regarding the upper and lower limb develops in parallel, as suggested by previous clinical research (20, 22, 23), a similar distinct time window of behavioral restitution of standing balance control through the most-affected leg might be expected.
Previous posturographic studies already showed that the DCA shows little tendency to diminish over inpatient rehabilitation (40) resulting in a poor contribution of the most-affected leg to balance control (29, 31, 32, 41). Acknowledging that recovery of standing balance may extend far beyond the first weeks, e.g., improvements are seen in response to specific training even in the chronic stage (67), it seems that learning to compensate with the intact leg drives the reacquisition of functional balance skills after stroke. In favor of this notion, few longitudinal studies report consistent improvements over the first months in timed muscle activation with the less-affected leg to effectively correct balance after perturbations (34, 42, 68). Simultaneous changes at the hemiplegic side are often absent (34, 42, 68). However, how progress of time contributes to the relative involvement of the most-affected limb to balance control and, consequently, when such compensation need to emerge has hardly been investigated early after stroke. This makes project A of the current study unique.
Mechanisms Underlying Recovery of Standing Balance Control
When standing, even smallest movements of the body must be corrected to avoid excessive sway and eventually a fall. As such, fine motor control is demanded for effective balance control. However, balance-related leg muscle activation is often disturbed after stroke. Abnormal intra-limb coordination patterns (68, 69) and delayed muscle onset (34, 42, 68) characterize reactive balance control through the hemiplegic leg, and inter-limb muscle activity about the ankle joints is poorly synchronized (11, 32, 39) and unequal exerted (2, 29–31, 40) during unperturbed stance. What determines poor muscle control as seen during balancing tasks is unknown, but may involve synergy-dependency (30, 31).
Already in 1951, Twitchell showed based on meticulous clinical observations that regaining control over the hemiplegic limb goes through synergy-dependent stages (48). This is later confirmed by longitudinal studies (20, 22, 23) showing progressively increasing scores on the FMA-LE over the first weeks poststroke. One might suspect a relationship between such clinical gains and an improved ability to execute functional movements with this leg, but this has been investigated cross-sectionally only with regard to standing balance (30–32). Although a relation was suggested, it is considered weak (31, 32). It was recently even shown that patients with near normal scores on the FMA-LE may show considerable control asymmetries (31). Although speculative, one may suggest that subtle fine motor control impairments go undetected by these scales, while it remains entirely unknown how this relation develops early after stroke. Since this knowledge has implications for rehabilitation practice, project B can be regarded as being innovative and of clinical relevance.
Clinical and Scientific Significance
It is important for rehabilitation clinicians to distinguish improved standing balance resulting from behavioral restitution of the most-affected limb and compensatory stabilization through the less-affected limb. Historical treatment concepts strive to restore normal movement patterns (70), and even recent therapies such as feedback-based balance training involve teaching patients to stand as symmetric as possible (71). This might be questioned acknowledging that many stroke survivors seem unable to restore symmetric balance control strategies (31, 40). These patients may even benefit from some asymmetric loading to make corrective COP movements at the less-affected side more effective (57). From this perspective, the knowledge gained through this study may further direct how stroke survivors should be trained early onwards. This may eventually result in faster reaching of independence in daily life activities to enable patients to engage as early as possible in more intensive, semi-supervised therapies (72) and supported discharge (73).
However, implications may go far beyond clinical rehabilitation practice alone. An improved understanding of recovery that distinguishes behavioral restitution from compensation will contribute to the design of rehabilitation devices as well as development of sensitive measurements of quality of movement. The latter will improve future trial design regarding the choice of outcome measures (16). Specifically, addressing effectiveness of novel behavioral and pharmacological treatments based on such measures is warranted (35). Moreover, interpretation of neuroimaging may greatly benefit from this knowledge. Current literature argues the importance of knowledge about the associations between behavioral improvements and changes in brain activity and connectivity (35), yet the neural correlates of behavioral restitution remain so far unknown.
Study Limitations
The study described in the current report also has some limitations. Although the number of participants that will be included in the current study is greater as compared to previous prospective balance recovery studies (30, 34, 40, 42, 68) [with ranges between n = 13 (68) and n = 37 (40)], the desired sample size is limited. Second, since we use one specific balance condition it is not possible to determine whether results can be generalized to other balance tasks. Recent literature suggests that increasing challenges may reduce the degree of asymmetry in bipedal balance control (74) and balancing in everyday life environments requires rather reactive control skills (11). The results may therefore not fully capture the upper boundary of neurological recovery at the hemiplegic side and translation to dynamic balance conditions remains unknown. However, incorporating more-challenging paradigms may lead to a greater amount of missing values since many subacute patients with hemiparesis are not able to safely withstand perturbations when standing (34, 42) or perform dynamic tasks such as walking (75) until several weeks after stroke. Third, repeated measurements were performed with either force-plates or a mobile pressure-plate system. This enables us to perform measures in various (clinical) settings and recruit more broadly. However, while both systems can extract bilateral COP profiles, technical variations differ. To control for this, focus lies on within-subjects time series analyses and, additionally, we will add “equipment” as a covariate to our regression models. Fourth, measurements are restricted to the first 12 weeks. Yet, it might be of interest to further continue measurements acknowledging that few studies revealed that about 15% of survivors improve (76) and 25% will deteriorate beyond the first 6 months (77). Lastly, the study did not monitor the type and amount of therapy provided to each participant and we are unable to correct for these factors. The lack of using uniform guidelines for stroke rehabilitation in Flanders, Belgium could lead to differences in how subjects are treated in cooperating facilities. Moreover, mildly-affected subjects may be discharged earlier and receive less intensive outpatient therapy afterwards. However, evidence that current rehabilitation interventions impact neurological recovery is still lacking so far (17, 78), particularly if provided as part of standard care which is shown to be low-dosed (79, 80). Since usual care has not been systematically modified, differences in rehabilitation treatment are expected to have a limited impact on outcomes of the current study.
Author Contributions
JS, ST, and WS participated in the planning and initial conception of this study. GK and LY participated in further conceptual work and designing the study. JS wrote the initial manuscript, is responsible for the study process and day-to-day study management under guidance of WS. All authors were involved in drafting the manuscript and have approved the final version for publication.
Funding
SJ receives funding by the Fonds Wetenschappelijk Onderzoek (FWO), Flanders, Belgium in order to execute the described research project and communicate results (application nr.: 1S64819N).
Conflict of Interest
The authors declare that the research was conducted in the absence of any commercial or financial relationships that could be construed as a potential conflict of interest.
Publisher's Note
All claims expressed in this article are solely those of the authors and do not necessarily represent those of their affiliated organizations, or those of the publisher, the editors and the reviewers. Any product that may be evaluated in this article, or claim that may be made by its manufacturer, is not guaranteed or endorsed by the publisher.
References
1. Donkor ES. Stroke in the 21(st) century: a snapshot of the burden, epidemiology, and quality of life. Stroke Res Treat. (2018) 2018:3238165. doi: 10.1155/2018/3238165
2. Mizrahi J, Solzi P, Ring H, Nisell R. Postural stability in stroke patients: vectorial expression of asymmetry, sway activity and relative sequence of reactive forces. Med Biol Eng Comput. (1989) 27:181–90. doi: 10.1007/BF02446228
3. Dickstein R, Abulaffio N. Postural sway of the affected and nonaffected pelvis and leg in stance of hemiparetic patients. Arch Phys Med Rehabil. (2000) 81:364–7. doi: 10.1016/S0003-9993(00)90085-6
4. Marigold DS, Eng JJ. The relationship of asymmetric weight-bearing with postural sway and visual reliance in stroke. Gait Posture. (2006) 23:249–55. doi: 10.1016/j.gaitpost.2005.03.001
5. Peurala SH, Kononen P, Pitkanen K, Sivenius J, Tarkka IM. Postural instability in patients with chronic stroke. Restor Neurol Neurosci. (2007) 25:101–8.
6. Kollen B, van de Port I, Lindeman E, Twisk J, Kwakkel G. Predicting improvement in gait after stroke: a longitudinal prospective study. Stroke. (2005) 36:2676–80. doi: 10.1161/01.STR.0000190839.29234.50
7. Michael KM, Allen JK, Macko RF. Reduced ambulatory activity after stroke: the role of balance, gait, and cardiovascular fitness. Arch Phys Med Rehabil. (2005) 86:1552–6. doi: 10.1016/j.apmr.2004.12.026
8. van de Port IG, Kwakkel G, Lindeman E. Community ambulation in patients with chronic stroke: how is it related to gait speed? J Rehabil Med. (2008) 40:23–7. doi: 10.2340/16501977-0114
9. Mackintosh SF, Hill KD, Dodd KJ, Goldie PA, Culham EG. Balance score and a history of falls in hospital predict recurrent falls in the 6 months following stroke rehabilitation. Arch Phys Med Rehabil. (2006) 87:1583–9. doi: 10.1016/j.apmr.2006.09.004
10. Simpson LA, Miller WC, Eng JJ. Effect of stroke on fall rate, location and predictors: a prospective comparison of older adults with and without stroke. PLoS ONE. (2011) 6:e19431. doi: 10.1371/journal.pone.0019431
11. Mansfield A, Wong JS, McIlroy WE, Biasin L, Brunton K, Bayley M, et al. Do measures of reactive balance control predict falls in people with stroke returning to the community? Physiotherapy. (2015) 101:373–80. doi: 10.1016/j.physio.2015.01.009
12. Forster A, Young J. Incidence and consequences of falls due to stroke: a systematic inquiry. BMJ. (1995) 311:83–6. doi: 10.1136/bmj.311.6997.83
13. Joo H, George MG, Fang J, Wang G. A literature review of indirect costs associated with stroke. J Stroke Cerebrovasc Dis. (2014) 23:1753–63. doi: 10.1016/j.jstrokecerebrovasdis.2014.02.017
14. Carod-Artal FJ, Egido JA. Quality of life after stroke: the importance of a good recovery. Cerebrovasc Dis. (2009) 27 Suppl 1:204–14. doi: 10.1159/000200461
15. Bernhardt J, Hayward KS, Kwakkel G, Ward NS, Wolf SL, Borschmann K, et al. Agreed definitions and a shared vision for new standards in stroke recovery research: the stroke recovery and rehabilitation roundtable taskforce. Neurorehabil Neural Repair. (2017) 31:793–9. doi: 10.1177/1545968317732668
16. Winters C, Kwakkel G, van Wegen EEH, Nijland RHM, Veerbeek JM, Meskers CGM. Moving stroke rehabilitation forward: the need to change research. NeuroRehabilitation. (2018) 43:19–30. doi: 10.3233/NRE-172393
17. Langhorne P, Bernhardt J, Kwakkel G. Stroke rehabilitation. Lancet. (2011) 377:1693–702. doi: 10.1016/S0140-6736(11)60325-5
18. Krakauer JW, Carmichael ST, Corbett D, Wittenberg GF. Getting neurorehabilitation right: what can be learned from animal models? Neurorehabil Neural Repair. (2012) 26:923–31. doi: 10.1177/1545968312440745
19. Levin MF, Kleim JA, Wolf SL. What do motor “recovery” and “compensation” mean in patients following stroke? Neurorehabil Neural Repair. (2009) 23:313–9. doi: 10.1177/1545968308328727
20. Duncan PW, Goldstein LB, Horner RD, Landsman PB, Samsa GP, Matchar DB. Similar motor recovery of upper and lower extremities after stroke. Stroke. (1994) 25:1181–8. doi: 10.1161/01.STR.25.6.1181
21. Jorgensen HS, Nakayama H, Raaschou HO, Vive-Larsen J, Stoier M, Olsen TS. Outcome and time course of recovery in stroke. part II: time course of recovery the copenhagen stroke study. Arch Phys Med Rehabil. (1995) 76:406–12. doi: 10.1016/S0003-9993(95)80568-0
22. Verheyden G, Nieuwboer A, De Wit L, Thijs V, Dobbelaere J, Devos H, et al. Time course of trunk, arm, leg, and functional recovery after ischemic stroke. Neurorehab Neural Re. (2008) 22:173–9. doi: 10.1177/1545968307305456
23. Kwakkel G, Kollen B, Twisk J. Impact of time on improvement of outcome after stroke. Stroke. (2006) 37:2348–53. doi: 10.1161/01.STR.0000238594.91938.1e
24. Jorgensen HS, Nakayama H, Raaschou HO, Olsen TS. Recovery of walking function in stroke patients: the copenhagen stroke study. Arch Phys Med Rehabil. (1995) 76:27–32. doi: 10.1016/S0003-9993(95)80038-7
25. Wade DT, Wood VA, Hewer RL. Recovery after stroke–the first 3 months. J Neurol Neurosurg Psychiatry. (1985) 48:7–13. doi: 10.1136/jnnp.48.1.7
26. Friedman PJ. Gait recovery after hemiplegic stroke. Int Disabil Stud. (1990) 12:119–22. doi: 10.3109/03790799009166265
27. Smith MC, Byblow WD, Barber PA, Stinear CM. Proportional recovery from lower limb motor impairment after stroke. Stroke. (2017) 48:1400–3. doi: 10.1161/STROKEAHA.116.016478
28. Veerbeek JM, Winters C, van Wegen EEH, Kwakkel G. Is the proportional recovery rule applicable to the lower limb after a first-ever ischemic stroke? PLoS ONE. (2018) 13:e0189279. doi: 10.1371/journal.pone.0189279
29. Genthon N, Rougier P, Gissot AS, Froger J, Pelissier J, Perennou D. Contribution of each lower limb to upright standing in stroke patients. Stroke. (2008) 39:1793–9. doi: 10.1161/STROKEAHA.107.497701
30. Roerdink M, Geurts AC, de Haart M, Beek PJ. On the relative contribution of the paretic leg to the control of posture after stroke. Neurorehabil Neural Repair. (2009) 23:267–74. doi: 10.1177/1545968308323928
31. Roelofs JMB, van Heugten K, de Kam D, Weerdesteyn V, Geurts ACH. Relationships between affected-leg motor impairment, postural asymmetry, and impaired body sway control after unilateral supratentorial stroke. Neurorehabil Neural Repair. (2018) 32:953–60. doi: 10.1177/1545968318804405
32. Mansfield A, Mochizuki G, Inness EL, McIlroy WE. Clinical correlates of between-limb synchronization of standing balance control and falls during inpatient stroke rehabilitation. Neurorehabil Neural Repair. (2012) 26:627–35. doi: 10.1177/1545968311429688
33. Kwakkel G, Kollen B, Lindeman E. Understanding the pattern of functional recovery after stroke: facts and theories. Restor Neurol Neurosci. (2004) 22:281–99.
34. Garland SJ, Willems DA, Ivanova TD, Miller KJ. Recovery of standing balance and functional mobility after stroke. Arch Phys Med Rehabil. (2003) 84:1753–9. doi: 10.1016/j.apmr.2003.03.002
35. Kwakkel G, van Wegen EEH, Burridge JH, Winstein CJ, van Dokkum LEH, Alt Murphy M, et al. Standardized measurement of quality of upper limb movement after stroke: consensus-based core recommendations from the second stroke recovery and rehabilitation roundtable. Neurorehabil Neural Repair. (2019) 33:951–8. doi: 10.1177/1545968319886477
36. Patterson KK, Inness E, McIlroy WE, Mansfield A. A retrospective analysis of post-stroke berg balance scale scores: how should normal and at-risk scores be interpreted? Physiother Can. (2017) 69:142–9. doi: 10.3138/ptc.2015-73
37. Gatev P, Thomas S, Kepple T, Hallett M. Feedforward ankle strategy of balance during quiet stance in adults. J Physiol. (1999) 514:915–28. doi: 10.1111/j.1469-7793.1999.915ad.x
38. Masani K, Popovic MR, Nakazawa K, Kouzaki M, Nozaki D. Importance of body sway velocity information in controlling ankle extensor activities during quiet stance. J Neurophysiol. (2003) 90:3774–82. doi: 10.1152/jn.00730.2002
39. Mansfield A, Danells CJ, Inness E, Mochizuki G, McIlroy WE. Between-limb synchronization for control of standing balance in individuals with stroke. Clin Biomech. (2011) 26:312–7. doi: 10.1016/j.clinbiomech.2010.10.001
40. de Haart M, Geurts AC, Huidekoper SC, Fasotti L, van Limbeek J. Recovery of standing balance in postacute stroke patients: a rehabilitation cohort study. Arch Phys Med Rehabil. (2004) 85:886–95. doi: 10.1016/j.apmr.2003.05.012
41. van Asseldonk EH, Buurke JH, Bloem BR, Renzenbrink GJ, Nene AV, van der Helm FC, et al. Disentangling the contribution of the paretic and non-paretic ankle to balance control in stroke patients. Exp Neurol. (2006) 201:441–51. doi: 10.1016/j.expneurol.2006.04.036
42. Garland SJ, Ivanova TD, Mochizuki G. Recovery of standing balance and health-related quality of life after mild or moderately severe stroke. Arch Phys Med Rehabil. (2007) 88:218–27. doi: 10.1016/j.apmr.2006.11.023
43. Le Clair K, Riach C. Postural stability measures: what to measure and for how long. Clin Biomech. (1996) 11:176–8. doi: 10.1016/0268-0033(95)00027-5
44. Geurts AC, Nienhuis B, Mulder TW. Intrasubject variability of selected force-platform parameters in the quantification of postural control. Arch Phys Med Rehabil. (1993) 74:1144–50.
45. Lafond D, Corriveau H, Hebert R, Prince F. Intrasession reliability of center of pressure measures of postural steadiness in healthy elderly people. Arch Phys Med Rehabil. (2004) 85:896–901. doi: 10.1016/j.apmr.2003.08.089
46. Gray VL, Ivanova TD, Garland SJ. Reliability of center of pressure measures within and between sessions in individuals post-stroke and healthy controls. Gait Posture. (2014) 40:198–203. doi: 10.1016/j.gaitpost.2014.03.191
47. McMorland AJ, Runnalls KD, Byblow WD. A neuroanatomical framework for upper limb synergies after stroke. Front Hum Neurosci. (2015) 9:82. doi: 10.3389/fnhum.2015.00082
48. Twitchell TE. The restoration of motor function following hemiplegia in man. Brain. (1951) 74:443–80. doi: 10.1093/brain/74.4.443
49. Bohannon RW. Muscle strength and muscle training after stroke. J Rehabil Med. (2007) 39:14–20. doi: 10.2340/16501977-0018
50. Duncan PW, Propst M, Nelson SG. Reliability of the fugl-meyer assessment of sensorimotor recovery following cerebrovascular accident. Phys Ther. (1983) 63:1606–10. doi: 10.1093/ptj/63.10.1606
51. Sullivan KJ, Tilson JK, Cen SY, Rose DK, Hershberg J, Correa A, et al. Fugl-Meyer assessment of sensorimotor function after stroke: standardized training procedure for clinical practice and clinical trials. Stroke. (2011) 42:427–32. doi: 10.1161/STROKEAHA.110.592766
52. See J, Dodakian L, Chou C, Chan V, McKenzie A, Reinkensmeyer DJ, et al. A standardized approach to the Fugl-Meyer assessment and its implications for clinical trials. Neurorehabil Neural Repair. (2013) 27:732–41. doi: 10.1177/1545968313491000
53. Fayazi M, Dehkordi SN, Dadgoo M, Salehi M. Test-retest reliability of Motricity Index strength assessments for lower extremity in post stroke hemiparesis. Med J Islam Repub Iran. (2012) 26:27–30.
54. Collen FM, Wade DT, Bradshaw CM. Mobility after stroke: reliability of measures of impairment and disability. Int Disabil Stud. (1990) 12:6–9. doi: 10.3109/03790799009166594
55. Shumay-Cook AW. Motor Control: Translating research into practice. Lippincott Williams And Wilkins (2017).
56. Winter DA, Francois P, Stergiou P, Powell C. Medial-lateral and anteriorposterior motor responses associated with centre of pressure changes in quiet stance. Neurosci Res Commun. (1993) 12:141–8.
57. Anker LC, Weerdesteyn V, van Nes IJ, Nienhuis B, Straatman H, Geurts AC. The relation between postural stability and weight distribution in healthy subjects. Gait Posture. (2008) 27:471–7. doi: 10.1016/j.gaitpost.2007.06.002
58. Blum L, Korner-Bitensky N. Usefulness of the berg balance scale in stroke rehabilitation: a systematic review. Phys Ther. (2008) 88:559–66. doi: 10.2522/ptj.20070205
59. Winter DA, Patla AE, Prince F, Ishac M, Gielo-Perczak K. Stiffness control of balance in quiet standing. J Neurophysiol. (1998) 80:1211–21. doi: 10.1152/jn.1998.80.3.1211
60. Kim JW, Eom GM, Kim CS, Kim DH, Lee JH, Park BK, et al. Sex differences in the postural sway characteristics of young and elderly subjects during quiet natural standing. Geriatr Gerontol Int. (2010) 10:191–8. doi: 10.1111/ggi.12453
61. Hue O, Simoneau M, Marcotte J, Berrigan F, Dore J, Marceau P, et al. Body weight is a strong predictor of postural stability. Gait Posture. (2007) 26:32–8. doi: 10.1016/j.gaitpost.2006.07.005
62. Laufer Y, Sivan D, Schwarzmann R, Sprecher E. Standing balance and functional recovery of patients with right and left hemiparesis in the early stages of rehabilitation. Neurorehabil Neural Repair. (2003) 17:207–13. doi: 10.1177/0888439003259169
63. Schepers VP, Ketelaar M, Visser-Meily AJ, de Groot V, Twisk JW, Lindeman E. Functional recovery differs between ischaemic and haemorrhagic stroke patients. J Rehabil Med. (2008) 40:487–9. doi: 10.2340/16501977-0198
64. Twisk JWR, de Vente W. Hybrid models were found to be very elegant to disentangle longitudinal within- and between-subject relationships. J Clin Epidemiol. (2019) 107:66–70. doi: 10.1016/j.jclinepi.2018.11.021
65. Cortes JC, Goldsmith J, Harran MD, Xu J, Kim N, Schambra HM, et al. A short and distinct time window for recovery of arm motor control early after stroke revealed with a global measure of trajectory kinematics. Neurorehabil Neural Repair. (2017) 31:552–60. doi: 10.1177/1545968317697034
66. van Kordelaar J, van Wegen E, Kwakkel G. Impact of time on quality of motor control of the paretic upper limb after stroke. Arch Phys Med Rehabil. (2014) 95:338–44. doi: 10.1016/j.apmr.2013.10.006
67. van Duijnhoven HJ, Heeren A, Peters MA, Veerbeek JM, Kwakkel G, Geurts AC, et al. Effects of exercise therapy on balance capacity in chronic stroke: systematic review and meta-analysis. Stroke. (2016) 47:2603–10. doi: 10.1161/STROKEAHA.116.013839
68. Kirker SG, Jenner JR, Simpson DS, Wing AM. Changing patterns of postural hip muscle activity during recovery from stroke. Clin Rehabil. (2000) 14:618–26. doi: 10.1191/0269215500cr370oa
69. Badke MB, Duncan PW. Patterns of rapid motor responses during postural adjustments when standing in healthy subjects and hemiplegic patients. Phys Ther. (1983) 63:13–20. doi: 10.1093/ptj/63.1.13
70. Langhammer B, Stanghelle JK. Can physiotherapy after stroke based on the Bobath concept result in improved quality of movement compared to the motor relearning programme. Physiother Res Int. (2011) 16:69–80. doi: 10.1002/pri.474
71. Geurts AC, de Haart M, van Nes IJ, Duysens J. A review of standing balance recovery from stroke. Gait Posture. (2005) 22:267–81. doi: 10.1016/j.gaitpost.2004.10.002
72. van Wissen K, Blanchard D. Circuit class therapy for improving mobility after stroke: A Cochrane review summary. Int J Nurs Stud. (2019) 97:130–1. doi: 10.1016/j.ijnurstu.2018.10.001
73. van den Berg M, Crotty MP, Liu E, Killington M, Kwakkel GP, van Wegen E. Early supported discharge by caregiver-mediated exercises and e-health support after stroke: a proof-of-concept trial. Stroke. (2016) 47:1885–92. doi: 10.1161/STROKEAHA.116.013431
74. Pollock CL, Hunt MA, Vieira TM, Gallina A, Ivanova TD, Garland SJ. Challenging standing balance reduces the asymmetry of motor control of postural sway poststroke. Motor Control. (2019) 23:327–43. doi: 10.1123/mc.2017-0098
75. Buurke JH, Nene AV, Kwakkel G, Erren-Wolters V, Ijzerman MJ, Hermens HJ. Recovery of gait after stroke: what changes? Neurorehabil Neural Repair. (2008) 22:676–83. doi: 10.1177/1545968308317972
76. Kwakkel G, Kollen BJ, Wagenaar RC. Long term effects of intensity of upper and lower limb training after stroke: a randomised trial. J Neurol Neurosurg Psychiatry. (2002) 72:473–9.
77. van de Port IG, Kwakkel G, Schepers VP, Lindeman E. Predicting mobility outcome one year after stroke: a prospective cohort study. J Rehabil Med. (2006) 38:218–23. doi: 10.1080/16501970600582930
78. Schroder J, Truijen S, Van Criekinge T, Saeys W. Feasibility and effectiveness of repetitive gait training early after stroke: a systematic review and meta-analysis. J Rehabil Med. (2019) 51:78–88. doi: 10.2340/16501977-2505
79. Rand D, Eng JJ. Disparity between functional recovery and daily use of the upper and lower extremities during subacute stroke rehabilitation. Neurorehabil Neural Repair. (2012) 26:76–84. doi: 10.1177/1545968311408918
Keywords: stroke recovery, motor recovery, force plate analysis, longitudinal regression analysis, standing balance, prospective longitudinal cohort study
Citation: Schröder J, Saeys W, Yperzeele L, Kwakkel G and Truijen S (2022) Time Course and Mechanisms Underlying Standing Balance Recovery Early After Stroke: Design of a Prospective Cohort Study With Repeated Measurements. Front. Neurol. 13:781416. doi: 10.3389/fneur.2022.781416
Received: 09 November 2021; Accepted: 17 January 2022;
Published: 21 February 2022.
Edited by:
Yves Vandermeeren, CHU Dinant Godinne UCL Namur, BelgiumReviewed by:
Akhila Veerubhotla, Kessler Foundation, United StatesPhilippe Desfontaines, Centre Hospitalier Chrétien, Belgium
Copyright © 2022 Schröder, Saeys, Yperzeele, Kwakkel and Truijen. This is an open-access article distributed under the terms of the Creative Commons Attribution License (CC BY). The use, distribution or reproduction in other forums is permitted, provided the original author(s) and the copyright owner(s) are credited and that the original publication in this journal is cited, in accordance with accepted academic practice. No use, distribution or reproduction is permitted which does not comply with these terms.
*Correspondence: Jonas Schröder, Sm9uYXMuc2Nocm9kZXJAdWFudHdlcnBlbi5iZQ==