- 1Department of Electrical and Computer Engineering, University of Maryland, College Park, MD, United States
- 2Department of Psychological Sciences, University of Connecticut, Storrs, CT, United States
- 3Department of Neurology, The Johns Hopkins School of Medicine, Baltimore, MD, United States
- 4Department of Biology, University of Maryland, College Park, MD, United States
- 5Institute for Systems Research, University of Maryland, College Park, MD, United States
Stroke patients with hemiparesis display decreased beta band (13–25 Hz) rolandic activity, correlating to impaired motor function. However, clinically, patients without significant weakness, with small lesions far from sensorimotor cortex, exhibit bilateral decreased motor dexterity and slowed reaction times. We investigate whether these minor stroke patients also display abnormal beta band activity. Magnetoencephalographic (MEG) data were collected from nine minor stroke patients (NIHSS < 4) without significant hemiparesis, at ~1 and ~6 months postinfarct, and eight age-similar controls. Rolandic relative beta power during matching tasks and resting state, and Beta Event Related (De)Synchronization (ERD/ERS) during button press responses were analyzed. Regardless of lesion location, patients had significantly reduced relative beta power and ERS compared to controls. Abnormalities persisted over visits, and were present in both ipsi- and contra-lesional hemispheres, consistent with bilateral impairments in motor dexterity and speed. Minor stroke patients without severe weakness display reduced rolandic beta band activity in both hemispheres, which may be linked to bilaterally impaired dexterity and processing speed, implicating global connectivity dysfunction affecting sensorimotor cortex independent of lesion location. Findings not only illustrate global network disruption after minor stroke, but suggest rolandic beta band activity may be a potential biomarker and treatment target, even for minor stroke patients with small lesions far from sensorimotor areas.
Introduction
Motor impairment is present in many stroke survivors (1), but does not always take the form of significant weakness. Patients with “minor stroke” (2) and low National Institute of Health Stroke Scale (NIHSS) scores can exhibit normal strength but have disabling deficits manifesting as slowed response times and limited dexterity. This is common even in high functioning patients (3) and typically occurs bilaterally and independent of lesion location (4). Unlike hemiparesis, the underlying neural mechanisms for these processes are less well-understood. These minor stroke patients also report difficulty with concentration and attention which, paired with decreased motor dexterity and slowed response times, hinder their ability to successfully return to work and reintegrate back into society. Previously, we found that such patients have low amplitude responses to visual stimuli that are temporally dispersed, possibly indicating a disruption of cortical networks (4). In this study we investigate neural responses in the sensorimotor cortex of the same cohort of minor stroke patients compared to age-similar controls, to determine if they display abnormal beta band activity, possibly linked to mechanisms underlying reduced motor dexterity and slowed response times.
Measurements of cortical activity using electroencephalography (EEG) or magnetoencephalography (MEG) indicate that rolandic beta band (13–25 Hz) responses are intricately linked to motor function (5–7). Spontaneous rolandic beta band activity may reflect multiple functional mechanisms in sensorimotor cortex including intracortical inhibition, communication, motor imagery and motor planning (8–10). Abnormal beta band activity has been observed in stroke (11, 12), Parkinson's disease (13) and other sensorimotor disorders (14). Stroke patients with motor deficits have been found to display reduced beta responses, especially in the ipsi-lesional hemisphere, possibly due to abnormal disinhibition and increased excitation (15). It is well-established that beta band activity reduces during movement planning and execution (Event Related Desynchronization or ERD), and increases afterwards (Event Related Synchronization or ERS) in sensorimotor cortex (8, 9, 16). Although the neural mechanisms involved in these changes are not clear, prior work suggests that beta ERD may reflect cortical excitability and downregulation of inhibition while ERS may reflect active inhibition or a return to status quo after movement (17–19). Stroke patients with hemiparesis have decreased beta ERD/ERS, with a greater reduction in the ipsi-lesional hemisphere (11, 20) and abnormal cortical patterns and latencies (21). However, it is unclear whether patients with small lesions without significant hemiparesis, would also display such abnormalities in beta band activity and beta ERD/ERS, and if abnormalities would occur independent of whether the lesion affected traditional motor pathways. We have reason to hypothesize this will be the case, and that abnormalities will be bilateral, given their observed clinical deficits.
This study involved MEG data collected from stroke patients with small lesions with minor impairments in motor dexterity but no hemiparesis, and was motivated by several research questions. First, we address whether stroke patients with small infarcts display abnormal rolandic beta activity compared to controls using relative beta power and beta ERD/ERS during button press responses. Next, we explore whether abnormalities improve with time, using a subset of the patient cohort who return for a second visit ~6 months later. Finally, we investigate whether the lesion location influences beta band activity by separately analyzing responses in ipsi- and contra-lesional hemispheres, and use the pattern of abnormal beta to draw conclusions regarding potential mechanisms and future treatment implications.
Methods
Subject Population
Nine patients with acute ischemic stroke, 4–6 weeks post-infarct, and eight age-similar controls (age-matched within 5 years) without history of prior stroke were recruited for this MEG study [abnormalities in visual evoked responses from this population was reported in our previous study (4)]. The study was approved by the Johns Hopkins University institutional review board and all participants provided written informed consent. This study focused on patients with minor stroke to avoid potential confounding due to severe deficits and explore the role of small, predominantly subcortical infarcts in network dysfunction. Patients with significant hemiplegia or aphasia, large vessel occlusions (M1 and M2 branches), prior history of dementia or other neurological disease, incompletely treated psychiatric disease, or uncorrected vision or hearing loss were excluded. All patients underwent a comprehensive neurological examination by a board certified stroke neurologist (EBM) prior to participation in the study and did not display any other neurological injury, but did uniformly endorse new problems with attention, executive function, and processing speed. Deficits and corresponding MEG abnormalities were therefore judged to be secondary to their recent infarct.
Although definitions of “minor stroke” differ in the literature (2), this patient cohort was classified based on their low NIHSS scores, small lesion volumes (10 cc or less), and lack of severe motor disabilities, cortical features, or other factors as described above in the exclusion criteria. Admission NIHSS scores of up to 12 were included in order to capture small, deep, lacunar infarcts within the internal capsule that may lead to higher scores than lacunes in other brain areas; however, the vast majority of patients presented with NIHSS scores <4, and all NIHSS scores were <4 at follow-up prior to their initial MEG. Stroke patients were prescribed antiplatelet agents, statins, and agents for blood pressure and glycemic control for secondary stroke prevention. Two were also on antidepressants, along with one control participant.
The NIHSS (22), modified Rankin scale (mRS) (23), Barthel Index (24), Montreal Cognitive Assessment (MoCA) (25), and F-A-S verbal fluency test (26) were assessed at each visit to determine functional and cognitive impairment. Strength and hemiparesis was assessed through clinical examination, while motor dexterity and processing speed were captured using the grooved pegboard (27). Importantly, all patients exhibited low NIHSS scores and near normal neurological screening examinations, with the exception of their cognition (measured using the MoCA; impaired defined as ≤26, see Table 1). Cognitive deficits were mild and improved between visits. Motor examinations were significant for mildly impaired rapid alternating movements contralateral to the side of the infarct for some (n = 4), but with normal strength and tone without significant hemiparesis. However, patients uniformly displayed slowed bilateral motor responses during task performance and impaired dexterity as measured by the grooved pegboard. Six patients and six controls returned for a 2nd visit ~6 months post-infarct, before recruitment was halted due to the COVID-19 pandemic. A detailed description of patient characteristics is provided in Table 1. The anatomical locations and volumes of the lesions were determined during hospitalization using diffusion weighted MR imaging (locations provided in Table 2). Additional details regarding the patient population, lesion locations and clinical measures are provided in our previous work (4).
Experiment Design
To investigate neural responses during motor activity, MEG data was collected while subjects performed picture-word matching tasks with button responses. Subjects rested in a supine position with their head placed in the MEG sensor array and held a button box in each hand. In each trial, subjects saw an image, followed 4 s later by a word and were asked to quickly and accurately press a button using their finger to indicate if the word corresponded to the image (yes: left button, no: right button). The next trial was presented 2 s after the subject pressed the button. The subtasks had varying levels of difficulty (e.g., matching the picture to the name, matching the picture to a description word) and a full description of each subtask is provided in our previous work (4). Reaction times were measured from the time the word first appeared on the screen to the time the participant pushed the response button. Across subtasks, subjects completed a total of 156 trials. Resting state data were also acquired in order to investigate if baseline beta band activity was different in patients vs. controls. Resting state magnetic fields were recorded for ~1–2 min while the subjects rested with eyes open and fixated on a cross projected onto a screen ~2 feet in front of them.
MEG Recording and Preprocessing
MEG data was recorded using a 157 axial gradiometer whole head MEG system (Kanazawa Institute of Technology, Nonoichi, Ishikawa, Japan) while subjects rested in the supine position in a magnetically shielded room (VAC, Hanau, Germany). The data was recorded at a sampling rate of 1 kHz with a 200 Hz low pass filter, and a 60 Hz notch filter. All subsequent analyses were performed in mne-python (28, 29), eelbrain (30), and R software (31). The code is available at https://github.com/jpkulasingham/minor-stroke-beta. Saturating channels were excluded and the data was denoised using temporal signal space separation (32) to remove external noise. The MEG data was filtered from 1 to 40 Hz using an FIR filter (mne-python default settings), downsampled to 200 Hz, and independent component analysis was used to remove artifacts such as eye blinks, heartbeats, and muscle movements.
Neural Source Localization
The head shape of each subject was digitized using a Polhemus 3SPACE FASTRAK system, and head position was measured before and after the experiment using five marker coils. The marker coil locations and the digitized head shape were used to co-register the template FreeSurfer “fsaverage” brain (33) using rotation, translation and uniform scaling. Single trial MEG data was source localized in the “ico-4” surface source space, with current direction constrained to currents orthogonal to the white matter surface, using an inverse operator computed via Minimum Norm Estimation (MNE) (34), and a noise covariance estimated from empty room data. Neural sources in and around the pre- and post-central gyri were selected as the rolandic Region of Interest (ROI), using the “aparc” parcellation labels “precentral” and “postcentral” (35) (see Figure 1A).
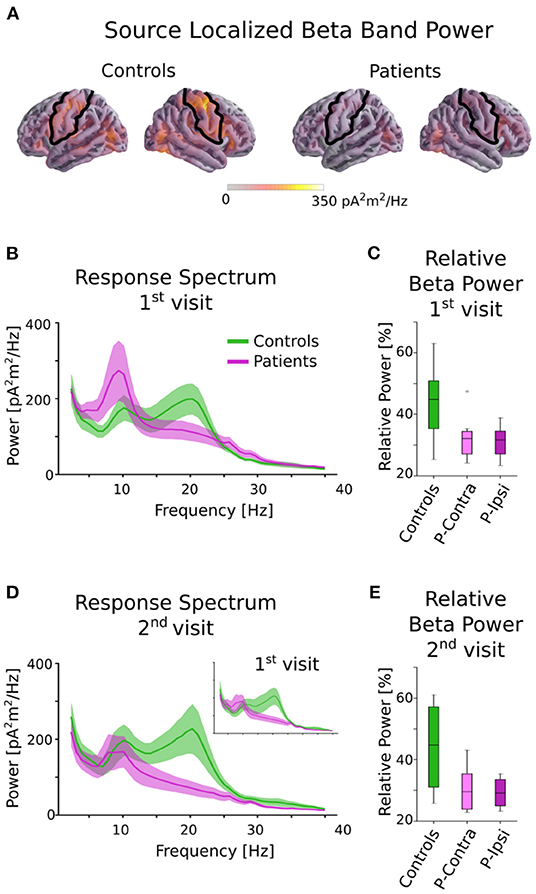
Figure 1. Beta power in controls and patients. (A) Source localized beta band power for controls and patients averaged across all tasks. The black outline indicates the ROI used for all further analysis. Although patients and controls have similar beta activity in occipital areas, controls have much stronger activity in the rolandic ROI. (B) Power spectral density of patients and controls averaged across all tasks in the central ROI for the 1st visit. There is a clear group difference in the beta range (13–25 Hz). (C) Relative beta power for controls and patients for the 1st visit, separated by ipsi-lesional and contra-lesional hemispheres. Clear differences between controls and patients are seen, but there are no notable differences within patients between ipsi-lesional and contra-lesional hemispheres. (D) Power spectral density for the 2nd visit. Note that only a subset of subjects returned for the 2nd visit. The 1st visit spectrum averaged across only this subset of subjects is shown as an inset for comparison. (E) Relative beta power for controls and patients for the 2nd visit, separated by ipsi-lesional and contra-lesional hemispheres. Patients show reduced power even after ~6 months postinfarct. There are no notable differences within patients for ipsi- and contra-lesional hemispheres.
Frequency Domain Analysis
The source localized continuous MEG data measured during each picture-word matching task and the resting state was segregated into 15 s intervals. For each interval, the power spectral density was computed using Welch's method (FFT length = 256 samples, with 50% overlap) and averaged across all segments and neural sources in the ROI. To account for individual variability in baseline frequency power, the relative beta power was computed by dividing the power in the beta range of 13–25 Hz by the total power in the range of 2–40 Hz. These relative beta powers were averaged across subtasks, log-transformed and were used for subsequent statistical analysis.
Event Related (De-)Synchronization Analysis
The source localized MEG data in the rolandic ROI were epoched −3 to 3 s before and after the response button was pushed during the picture-word matching tasks. The time-frequency spectrograms for these trials were computed using Morlet wavelets (20 frequency bands with log-spacing in the range of 6–35 Hz with the number of cycles in each band being equal to half the center frequency). The beta ERD was computed as the percentage decrease in average beta (13–25 Hz) power in the time range of −1 s to 0.5 s relative to the button-press, compared to the baseline average beta power (time range −3 to −2 s), consistent with established methods (9). The beta ERS was computed in a similar manner, as the percentage increase in average beta power over the baseline in the time range 0.5 to 2.5 s. The trial averaged ERD/ERS values for each subject (averaged across subtasks) were used for subsequent statistical analysis.
Statistical Analysis
Behavioral measures (reaction times and grooved peg board scores) were compared across patients and controls using t-tests with Bonferroni corrections for both the 1st visit (9 patients) and the 2nd visit (6 patients). Additionally, t-tests were used within the patient population to investigate if there were lateralization effects based on lesion hemisphere (ipsi- or contra-lesional sides). Correlations between behavioral performance and beta band measures were not investigated further given the small sample size.
To investigate group differences between patients and controls, the relative beta power in the rolandic ROI was analyzed with a linear mixed effects model using the “lme4” package in R (36), since these models are capable of accounting for individual variation using random effects and for missing data (for this dataset, several subjects were not present for a 2nd visit). This model was also used to test changes across visits, and across the picture-word matching tasks and resting state data. The dependent variable was relative beta log-power, with fixed effects of group (“control” or “patient”), task (“matching” or “resting”), and visit (“1st” or “2nd”), and a random intercept by subject. The full model with all interactions was tested for a significant difference over the reduced model without the highest-level interaction (group × task × visit) using the “drop1” function in the lmerTest package in R, which performs a Type II ANOVA with the degrees of freedom estimated using Satterthwaite's method (37). If the 3-way interaction was not significant, it was dropped from the model and the new model consisted of the 2-way interaction terms and the main effects. The same procedure with “drop1” was used to check for significant interactions in this new model. If none of the 2-way interaction terms were significant, the final model only consisted of main effects.
To investigate possible hemispheric differences due to lesion hemisphere in the patient population, the beta log-power was separated by hemisphere, and paired two-tailed t-tests were used to test for a significant difference between the ipsi- and contra-lesional hemispheres. Separate t-tests with Bonferroni correction were performed for the 1st visit (9 patients) and the 2nd visit (6 patients).
A linear mixed effects model was also used to investigate group differences and changes across visits. This model had fixed effects of group, visit, and metric (“ERD,” “ERS”) and random intercepts by subject. The same procedure as above was used to fit the models and determine significant interactions and effects, starting with the full model including the 3-way interaction. Finally, hemispheric differences in ERS and ERD due to lesion location were investigated within the patient population using paired two-tailed t-tests with Bonferroni correction for the 1st and 2nd visits similar to the above.
Results
Behavioral Outcomes
Nine patients with minor stroke and eight age-similar controls without prior history of stroke were recruited for this study. Controls were recruited with similar age (within 5 years, mean [SD]; controls = 58 [13.1]; patients = 59.8 [15.7]) and sex (n male, controls = 4, patients = 4), but controls had a higher level of education than patients (years of education: controls = 18.6 [3.6]; patients = 14.1 [4.4]). Patients performed worse in cognitive tasks compared to controls (MoCA controls = 29.5 [3], patients = 26.0 [4], Mann-Whitney U Test p = 0.005). The stroke severity scores for all patients were low at both visits (mean [SD] NIHSS 1st visit: 0.7 [1.1]; NIHSS 2nd visit: 0.2 [0.4], mRS 1st visit: 1 [0.5], 2nd visit: 0.5 [0.5], see Table 1). Patients were bilaterally slow on the grooved pegboard task for both visits as shown in Table 1 (reaction time in units of SD from the mean, ipsi-lesional 1st visit: −6.2 [5.6], 2nd visit: −3.3 [2.9]; contra-lesional 1st visit: −6.5 [5.1], 2nd visit: −6.3 [5.6]), though did demonstrate improvement. There were no significant differences between ipsi- and contra-lesional sides for grooved pegboard task performance [1st visit: t(8) = −0.15, p = 0.88; 2nd visit: t(5) = −1.84, p = 0.12]. The reaction times for the picture-word matching tasks were significantly longer for patients compared to controls for the first visit (mean [SD] controls = 1.04 [0.44] s, vs. patients = 2.17 [1.75] s; independent t-test on the reciprocal of the reaction times t(15) = 2.51, corrected p = 0.046, Cohen's d = 1.3). Although reaction times improved (decreased) for both patients and controls for the 2nd visit, there was no significant difference in the reaction time between the two visits (per subject difference between first and second visit: controls = 62 ms [143 ms], patients = −99 ms [318 ms]), and reaction times were still significantly different across groups for the 2nd visit [t(10) = 2.72, corrected p = 0.04, Cohen's d = 1.72]. There was also no difference in reaction times for the ipsi- and contra-lesional button presses in patients, which were both impaired [1st visit: t(8) = 0.88, p = 0.4; 2nd visit: t(5) = −0.95, p = 0.39]. Further details on reaction times for each subtask are provided in our previous work (4).
Beta Power Analysis
The MEG data during the picture-word matching tasks and resting state were source localized to the rolandic ROI and the (log-transformed) relative power in the beta frequency range of 13–25 Hz was computed (see Figure 1). A linear mixed effects model with fixed effects group (“patient” or “control”), visit (“1st” or “2nd”) and task (“matching” or “resting”) and a random intercept by subject revealed a significant main effect of group [F(1, 15) = 6.46, p = 0.023], but no other significant main effects [visit: F(1, 39) = 0.65, p = 0.42; task: F(1, 36) = 0.67, p = 0.42] or interactions [group × visit: F(1, 36) = 0.06, p = 0.80; group × task: F(1, 36) = 0.013, p = 0.91; visit × task: F(1, 39) = 0.93, p = 0.34; group × visit × task: F(1, 35) = 1.19, p = 0.28]. A post-hoc t-test averaged over tasks and visits revealed that controls had significantly higher relative beta power than patients [t(15) = 2.54, p = 0.023, Cohen's d = 1.31].
The effect of lesion hemisphere on the patients' beta activity was investigated by separating the relative beta power into ipsi- and contra-lesional hemispheres. The relative beta power was not significantly different across the hemispheres for either the 1st visit [picture-word matching: t(8) = 0.50, p = 0.63; resting: t(8) = 0.73, p = 0.49] or the 2nd visit [picture-word matching: t(5) = 0.83, p = 0.44; resting: t(5) = 0.45, p = 0.67]. Overall, patients had significantly reduced relative beta power for both the picture-word matching and resting tasks that did not improve for the 2nd visit, and was independent of lesion hemisphere.
Event Related (De)Synchronization Analysis
Beta ERS and ERD were calculated for the button responses during the picture-word matching tasks using Morlet wavelet spectrograms (see Figure 2; details in Methods). A linear mixed effects model for beta ERS/ERD with fixed effects group, visit, and metric (“ERS” or “ERD”) and a random intercept per subject was used to detect significant effects. The 3-way interaction was not significant [group × metric × visit F(1, 36) = 2.02, p = 0.16]. However, there was a significant interaction of group × metric [F(1, 37) = 13.5, p < 0.001] as well as significant main effects of group [F(1, 15) = 7.5, p = 0.015] and metric [F(1, 40) = 22.7, p < 0.001]. The other terms involving visit were not significant [group × visit: F(1, 41) = 1.62, p = 0.21; visit × metric: F(1, 37) = 0.34, p = 0.56; main effect of visit: F(1, 46) = 0.04, p = 0.84]. The significant interaction involving group and metric was analyzed further using post-hoc independent two-tailed t-tests with Bonferroni correction on the ERD and ERS averaged across visits and tasks. This revealed that patients had significantly lower ERS compared to controls (mean [SD] controls = 86.5 [44.3] %, patients = 39.9 [27.7] %, t(15) = 2.64, corrected p = 0.037, Cohen's d = 1.36). Although the ERD also showed a similar trend, with controls being larger than patients, this difference was not significant (controls = 30.0 [9.4] %, patients = 24.8 [9.6] %, t(15) = 1.12, corrected p = 0.28).
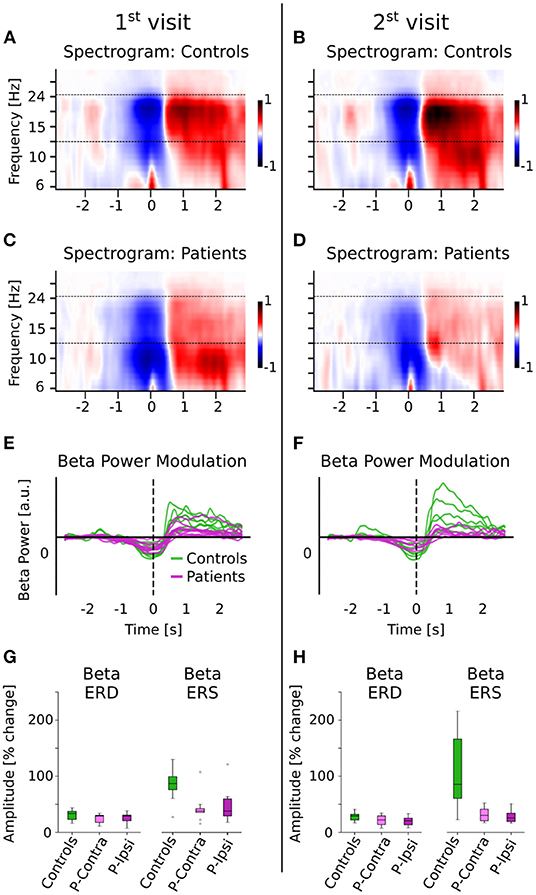
Figure 2. Beta ERD and ERS for controls and patients. (A–D) The spectrograms for controls and patients (normalized w.r.t. baseline activity) are shown. The movement (button press) occurred at time t = 0. The dashed line indicates the beta band (13–25 Hz) that was used for further analysis. (E,F) Beta power modulation per subject, computed as the average power in the beta band. Patients have reduced beta ERD/ERS. (G,H) Beta ERD/ERS (% change from baseline) for controls and patients, separated by ipsi- and contra-lesional hemispheres. Patients have reduced ERD and ERS compared to controls for both visits, but show no differences across hemispheres. The group difference is much larger for the ERS than for the ERD.
To avoid the confound of ERS/ERD effects being driven by group differences during the baseline time-period (denominator in ERS/ERD calculations), the baseline beta power was calculated separately and was found to be not significantly different across groups (independent t-test t(15) = 1.0, p = 0.33). However, the beta power relative to total power in 2–40 Hz in the baseline time-period was significantly different between groups [independent t-test t(15) = 2.54, p = 0.022], in line with the reduction of spontaneous relative beta power in patients as shown in Figure 1.
Finally, differences due to lesion location were tested by separating the ERD/ERS into ipsi- and contra-lesional hemispheres. There were no significant differences between the ipsi- and contra-lesional hemispheres within patients [ERD 1st visit: t(8) = −0.21, p = 0.84; ERD 2nd visit: t(5) = 1.62, p = 0.16; ERS 1st visit: t(8) = −0.82, p = 0.43; ERS 2nd visit: t(5) = 0.64, p = 0.55]. Overall, patients had significantly lower ERS (but not ERD), in a manner that was consistent across tasks and visits and independent of lesion hemisphere.
Discussion
Minor stroke patients displayed reduced beta activity in rolandic areas compared to controls, both during picture-word matching tasks and resting state. Although these patients did not have significant motor impairment or hemiparesis, they reported difficulties with concentration, attention and an overall slowness combined with a reduction of motor dexterity that hinders their ability to reintegrate well into society. We found that their rolandic beta activity was reduced bilaterally, independent of lesion hemisphere or location, and did not greatly improve with time. This is consistent with bilaterally slower reaction times and impaired grooved pegboard scores that improved but remained below the normative average for their second visit. Interestingly, group differences in beta ERS were much larger than in beta ERD. A similar pattern of persistently reduced visual evoked responses was found in the same patient cohort in our previous study (4). Overall, these results suggest a more global disruption, not tied to lesion location, possibly involving long range cortical networks or a global excitation/inhibition imbalance, even for patients with only minor strokes.
Reduced Beta Activity in Patients Compared to Controls
Minor stroke patients had significantly reduced relative beta power compared to controls in rolandic areas regardless of task. Although the beta ERD and ERS would be a significant component of the overall relative beta power during the picture-word matching tasks that involved button responses, we also found differences in relative beta power during the baseline period, which would not involve button press related motor beta activity. Spontaneous beta oscillations during resting were also reduced, consistent with prior work (15). This indicates that minor stroke patients have abnormal beta oscillations even without active movement.
Reduced Beta ERS/ERD in Patients Compared to Controls
Both beta ERS and ERD were reduced on average in minor stroke patients compared to controls, though only the ERS showed a significant difference. Prior studies have shown abnormal latencies, amplitudes and cortical patterns for both beta ERD and ERS in stroke patients with motor impairments (21, 38–41). The beta ERD/ERS is thought to reflect regulation of intracortical inhibition (17), although they may also arise from several other functions including motor planning and short-term memory (9). The changes in beta ERD may depend on the specific motor pathology (39), and may not be large enough to detect in our population of minor stroke patients. A more thorough analysis with a larger population is required to ascertain whether the beta ERD also shows a significantly reduced amplitude, like the ERS, in minor stroke patients.
Beta Abnormalities Independent of Lesion Hemisphere and Location
We found that for minor stroke patients, the reduction in beta activity was similar in both hemispheres, in contrast to some prior stroke studies that found a greater reduction of beta activity in the ipsi-lesional hemisphere (42, 43). The observed bilateral reduction in beta band activity agrees with slower reaction times for both hands on the grooved pegboard task in our patient population. Indeed, disinhibition and increased corticospinal excitability have been found in both hemispheres in cases of moderate to severe hemiparesis after unilateral stroke (44). Correspondingly, several studies do find bilateral abnormalities in beta activity due to stroke (12, 44–46), consistent with bilaterally impaired hand dexterity after unilateral stroke (47). Several explanations for the mechanisms underlying these bilateral impairments have been proposed, including bilateral disinhibition, cortical reorganization and bilateral involvement in motor planning and execution (46, 48). The bilateral disruption in our study occurred independent of lesion location, and the majority of infarcts were outside of the motor system, indicating that the bilateral abnormalities may be due to disruption of bilateral network connectivity leading to a global disruption. Further connectivity studies are warranted. This phenomenon has been reported in prior studies (49–51), most notably when examining patients with thalamic lesions leading to changes in bilateral cortical oscillations (52), but is further elucidated here with lesions in other locations, exhibiting a consistent behavioral phenotype.
Beta Abnormalities Are Consistent Across Visits
Cognitive performance and reaction times for the minor stroke patients improved when they returned for their second visit, though remained abnormal compared to controls. Beta band measures showed little improvement between visits, unlike other studies which found that improved recovery from stroke correlates with a return to healthy levels of beta band activity (20, 21, 46). However, most of these studies involved patients with moderate to severe motor impairments, and may not be comparable to our population of patients with only minor motor deficiencies. In our case, patients still performed slower than average on the grooved pegboard task even for the 2nd visit, indicating a persistent reduction in dexterity despite some improvement. The changes in beta band measures during recovery from minor strokes may be quite small, and perhaps longer studies (>1 year post infarct) are needed to detect such recovery effects. Alternatively, beta band abnormalities may persist due to mechanisms with long-term effects, such as network disruption (53). In summary, we show that MEG beta band activity is a meaningful measure of motor deficits, other than weakness, in patients with minor stroke, and may also provide insight into how a unilateral lesion results in bilateral symptomatology.
Considerations and Conclusions
Our study is not without limitations. Patients were not homogenous in terms of lesion location, though they were similar in stroke size, severity, and behavioral measures (NIHSS, small lesion volume, lack of severe motor disability and cortical features, slowed reaction times and dexterity). We did not find an association between anatomical measures and beta band abnormalities, providing further evidence that global network disruption can occur independent of lesion location and result in bilateral alterations in cerebral activation. This study has a limited number of subjects due to the need to stop recruitment during a pandemic, and the small sample size may have hindered proper investigations regarding the abovementioned concerns. Finally, two patients and one control were on antidepressants that theoretically could have altered cerebral function; nevertheless, group results were consistent regardless of medications and untreated depression has known effects on activity patterns (54, 55).
The bilateral reduction in beta activity is associated with an overall slowness and reduced dexterity in both hands and illustrates that even small infarcts may have bilateral impacts on motor function and sensorimotor activation, reflected in abnormal beta band activity. This work builds on previous studies that indicate that similar abnormalities are linked to a disruption of network connectivity or a global disinhibition of sensorimotor cortex, perhaps due to neural reorganization and compensatory mechanisms (44, 56–58). We believe this to be the case for our cohort as well, and given that many infarcts localize to areas not involving the motor system, this illustrates network disruption on a more global scale. We could not disentangle the composite mechanisms causing this abnormal beta activity, nor could we detect neural correlates of motor performance and improvement with time, perhaps due to our small sample size. Future studies with larger populations of minor stroke patients and more complex motor task designs could shed light on the underlying causes of abnormal beta band activity. In addition, formal connectivity studies to elucidate network dysfunction would aid in validation of our hypothesis. Measurements of beta band activity over the course of recovery could provide insight into accompanying neural connectivity changes. Investigating whether these small lesions far from motor cortex can consistently alter connectivity in remote areas could provide useful insights into the pathophysiology of these minor strokes and the mechanisms underlying bilateral motor impairments, and could lead to more effective methods of both motor rehabilitation and generalized stroke recovery.
Data Availability Statement
The data supporting the conclusions of this article are available at https://doi.org/10.13016/t8uf-k7nx.
Ethics Statement
The studies involving human participants were reviewed and approved by Johns Hopkins University Institutional Review Board. The patients/participants provided their written informed consent to participate in this study.
Author Contributions
EM and JS were involved in conception and design of the study. EM and SK recruited subjects. JK and SK designed and performed experiments and collected data. Data and statistical analysis was performed primarily by JK with the assistance and contribution of all authors. JK wrote the initial draft of the manuscript. All authors contributed to interpretation of results, methodology, and conclusions and were involved in manuscript revision and approved the final version.
Funding
This work was supported by an Innovative Research Grant through the American Heart Association (18IPA34170313) and National Science Foundation Grant SMA-1734892. This work is available online as a preprint (59).
Conflict of Interest
The authors declare that the research was conducted in the absence of any commercial or financial relationships that could be construed as a potential conflict of interest.
Publisher's Note
All claims expressed in this article are solely those of the authors and do not necessarily represent those of their affiliated organizations, or those of the publisher, the editors and the reviewers. Any product that may be evaluated in this article, or claim that may be made by its manufacturer, is not guaranteed or endorsed by the publisher.
References
1. Kelly-Hayes M, Beiser A, Kase CS, Scaramucci A, D'Agostino RB, Wolf PA. The influence of gender and age on disability following ischemic stroke: the Framingham study. J Stroke Cerebrovasc Dis. (2003) 12:119–26. doi: 10.1016/S1052-3057(03)00042-9
2. Fischer U, Baumgartner A, Arnold M, Nedeltchev K, Gralla J, De Marchis GM, et al. What is a minor stroke? Stroke. (2010) 41:661–6. doi: 10.1161/STROKEAHA.109.572883
3. Platz T, Prass K, Denzler P, Bock S, Mauritz K-H. Testing a motor performance series and a kinematic motion analysis as measures of performance in high-functioning stroke patients: reliability, validity, and responsiveness to therapeutic intervention. Arch Phys Med Rehabil. (1999) 80:270–7. doi: 10.1016/S0003-9993(99)90137-5
4. Marsh EB, Brodbeck C, Llinas RH, Mallick D, Kulasingham JP, Simon JZ, et al. Poststroke acute dysexecutive syndrome, a disorder resulting from minor stroke due to disruption of network dynamics. PNAS. (2020) 117:33578–85. doi: 10.1073/pnas.2013231117
5. Hari R, Forss N, Avikainen S, Kirveskari E, Salenius S, Rizzolatti G. Activation of human primary motor cortex during action observation: a neuromagnetic study. Proc Nat Acad Sci. (1998) 95:15061–5. doi: 10.1073/pnas.95.25.15061
6. Cheyne DO, MEG. studies of sensorimotor rhythms: a review. Exp Neurol. (2013) 245:27–39. doi: 10.1016/j.expneurol.2012.08.030
7. Tzagarakis C, Ince NF, Leuthold AC, Pellizzer G. Beta-band activity during motor planning reflects response uncertainty. J Neurosci. (2010) 30:11270–7. doi: 10.1523/JNEUROSCI.6026-09.2010
8. Pfurtscheller G, Stancák A, Neuper C. Post-movement beta synchronization. A correlate of an idling motor area? Electroencephalogr Clin Neurophysiol. (1996) 98:281–93. doi: 10.1016/0013-4694(95)00258-8
9. Kilavik BE, Zaepffel M, Brovelli A, MacKay WA, Riehle A. The ups and downs of beta oscillations in sensorimotor cortex. Exp Neurol. (2013) 245:15–26. doi: 10.1016/j.expneurol.2012.09.014
10. Pfurtscheller G, Neuper C, Brunner C, da Silva FL. Beta rebound after different types of motor imagery in man. Neurosci Lett. (2005) 378:156–9. doi: 10.1016/j.neulet.2004.12.034
11. Rossiter HE, Boudrias M-H, Ward NS. Do movement-related beta oscillations change after stroke? J Neurophysiol. (2014) 112:2053–8. doi: 10.1152/jn.00345.2014
12. Shiner CT, Tang H, Johnson BW, McNulty PA. Cortical beta oscillations and motor thresholds differ across the spectrum of post-stroke motor impairment, a preliminary MEG and TMS study. Brain Res. (2015) 1629:26–37. doi: 10.1016/j.brainres.2015.09.037
13. Heinrichs-Graham E, Wilson TW, Santamaria PM, Heithoff SK, Torres-Russotto D, Hutter-Saunders JAL, et al. Neuromagnetic evidence of abnormal movement-related beta desynchronization in parkinson's disease. Cerebral Cortex. (2014) 24:2669–78. doi: 10.1093/cercor/bht121
14. Leocani L, Comi G. Movement-related event-related desynchronization in neuropsychiatric disorders. In: Neuper C, Klimesch W, editors. Progress in Brain Research. Event-Related Dynamics of Brain Oscillations. Elsevier (2006). p. 351–66. doi: 10.1016/S0079-6123(06)59023-5
15. Tecchio F, Zappasodi F, Pasqualetti P, Tombini M, Salustri C, Oliviero A, et al. Rhythmic brain activity at rest from rolandic areas in acute mono-hemispheric stroke: a magnetoencephalographic study. Neuroimage. (2005) 28:72–83. doi: 10.1016/j.neuroimage.2005.05.051
16. Pfurtscheller G, Lopes da. Silva FH. Event-related EEG/MEG synchronization and desynchronization: basic principles. Clin Neurophysiol. (1999) 110:1842–57. doi: 10.1016/S1388-2457(99)00141-8
17. Takemi M, Masakado Y, Liu M, Ushiba J. Event-related desynchronization reflects downregulation of intracortical inhibition in human primary motor cortex. J Neurophysiol. (2013) 110:1158–66. doi: 10.1152/jn.01092.2012
18. Engel AK, Fries P. Beta-band oscillations—signalling the status quo? Curr Opin Neurobiol. (2010) 20:156–65. doi: 10.1016/j.conb.2010.02.015
19. Solis-Escalante T, Müller-Putz GR, Pfurtscheller G, Neuper C. Cue-induced beta rebound during withholding of overt and covert foot movement. Clin Neurophysiol. (2012) 123:1182–90. doi: 10.1016/j.clinph.2012.01.013
20. Parkkonen E, Laaksonen K, Parkkonen L, Forss N. Recovery of the 20 Hz rebound to tactile and proprioceptive stimulation after stroke. Neural Plast. (2018) 2018:e7395798. doi: 10.1155/2018/7395798
21. Tang C-W, Hsiao F-J, Lee P-L, Tsai Y-A, Hsu Y-F, Chen W-T, et al. β-Oscillations reflect recovery of the paretic upper limb in subacute stroke. Neurorehabil Neural Repair. (2020) 34:450–62. doi: 10.1177/1545968320913502
22. Brott T, Adams HP, Olinger CP, Marler JR, Barsan WG, Biller J, et al. Measurements of acute cerebral infarction: a clinical examination scale. Stroke. (1989) 20:864–70. doi: 10.1161/01.STR.20.7.864
23. Rankin J. Cerebral vascular accidents in patients over the age of 60: II. Prognosis. Scott Med J. (1957) 2:200–15. doi: 10.1177/003693305700200504
24. Wade DT, Collin C. The Barthel ADL Index: a standard measure of physical disability? Int Disabil Stud. (1988) 10:64–7. doi: 10.3109/09638288809164105
25. Nasreddine ZS, Phillips NA, Bédirian V, Charbonneau S, Whitehead V, Collin I, et al. The Montreal Cognitive Assessment, MoCA: a brief screening tool for mild cognitive impairment. J Am Geriatr Soc. (2005) 53:695–9. doi: 10.1111/j.1532-5415.2005.53221.x
26. Bush SS. Neurosensory Center comprehensive examination for aphasia. In: Kreutzer JS, DeLuca J, Caplan B, editors. Encyclopedia of Clinical Neuropsychology. New York, NY: Springer (2011). p. 1772–3.
27. Ashendorf L, Vanderslice-Barr JL, McCaffrey RJ. Motor tests and cognition in healthy older adults. Appl Neuropsychol. (2009) 16:171–6. doi: 10.1080/09084280903098562
28. Gramfort A. MEG and EEG data analysis with MNE-Python. Front Neurosci. (2013) 7:267. doi: 10.3389/fnins.2013.00267
29. Gramfort A, Luessi M, Larson E, Engemann DA, Strohmeier D, Brodbeck C, et al. Software for processing MEG and EEG data. Neuroimage. (2014) 86:446–60. doi: 10.1016/j.neuroimage.2013.10.027
30. Brodbeck C. Christianbrodbeck/Eelbrain. [Python]. (2020). Available online at: https://github.com/christianbrodbeck/Eelbrain (Accessed June 22, 2020).
31. R Core Team. R: A Language Environment for Statistical Computing. Vienna: R Foundation for Statistical Computing (2020). Available online at: https://www.R-project.org/
32. Taulu S, Simola J. Spatiotemporal signal space separation method for rejecting nearby interference in MEG measurements. Phys Med Biol. (2006) 51:1759–68. doi: 10.1088/0031-9155/51/7/008
34. Hämäläinen MS, Ilmoniemi RJ. Interpreting magnetic fields of the brain: minimum norm estimates. Med Biol Eng Comput. (1994) 32:35–42. doi: 10.1007/BF02512476
35. Desikan RS, Ségonne F, Fischl B, Quinn BT, Dickerson BC, Blacker D, et al. An automated labeling system for subdividing the human cerebral cortex on MRI scans into gyral based regions of interest. Neuroimage. (2006) 31:968–80. doi: 10.1016/j.neuroimage.2006.01.021
36. Kuznetsova A, Brockhoff PB, Christensen RHB. lmerTest package: tests in linear mixed effects models. J Stat Softw. (2017) 82:1–26. doi: 10.18637/jss.v082.i13
37. Satterthwaite FE. An approximate distribution of estimates of variance components. Biometr Bull. (1946) 2:110–4. doi: 10.2307/3002019
38. Monge-Pereira E, Molina-Rueda F, Rivas-Montero FM, Ibáñez J, Serrano JI, Alguacil-Diego IM, et al. Electroencephalography as a post-stroke assessment method: an updated review. Neurología. (2017) 32:40–9. doi: 10.1016/j.nrleng.2014.07.004
39. Platz T, Kim IH, Pintschovius H, Winter T, Kieselbach A, Villringer K, et al. Multimodal EEG analysis in man suggests impairment-specific changes in movement-related electric brain activity after stroke. Brain. (2000) 123(Pt. 12):2475–90. doi: 10.1093/brain/123.12.2475
40. Eder CF, Sokić D, Covičković-Šternić N, Mijajlović M, Savić M, Sinkjær T, et al. Symmetry of post-movement beta-ERS and motor recovery from stroke: a low-resolution EEG pilot study. Eur J Neurol. (2006) 13:1312–23. doi: 10.1111/j.1468-1331.2006.01550.x
41. Parkkonen E, Laaksonen K, Piitulainen H, Pekkola J, Parkkonen L, Tatlisumak T, et al. Strength of ~20-Hz rebound and motor recovery after stroke. Neurorehabil Neural Repair. (2017) 31:475–86. doi: 10.1177/1545968316688795
42. Tecchio F, Zappasodi F, Tombini M, Oliviero A, Pasqualetti P, Vernieri F, et al. Brain plasticity in recovery from stroke: an MEG assessment. Neuroimage. (2006) 32:1326–34. doi: 10.1016/j.neuroimage.2006.05.004
43. Bartur G, Pratt H, Soroker N. Changes in mu and beta amplitude of the EEG during upper limb movement correlate with motor impairment and structural damage in subacute stroke. Clin Neurophysiol. (2019) 130:1644–51. doi: 10.1016/j.clinph.2019.06.008
44. Manganotti P, Patuzzo S, Cortese F, Palermo A, Smania N, Fiaschi A. Motor disinhibition in affected and unaffected hemisphere in the early period of recovery after stroke. Clin Neurophysiol. (2002) 113:936–43. doi: 10.1016/S1388-2457(02)00062-7
45. Wilson TW, Fleischer A, Archer D, Hayasaka S, Sawaki L. Oscillatory MEG motor activity reflects therapy-related plasticity in stroke patients. Neurorehabil Neural Repair. (2010) 25:188–93. doi: 10.1177/1545968310378511
46. Graziadio S, Tomasevic L, Assenza G, Tecchio F, Eyre JA. The myth of the ‘unaffected' side after unilateral stroke: is reorganisation of the non-infarcted corticospinal system to re-establish balance the price for recovery? Exp Neurol. (2012) 238:168–75. doi: 10.1016/j.expneurol.2012.08.031
47. Nowak DA, Grefkes C, Dafotakis M, Küst J, Karbe H, Fink GR. Dexterity is impaired at both hands following unilateral subcortical middle cerebral artery stroke. Eur J Neurosci. (2007) 25:3173–84. doi: 10.1111/j.1460-9568.2007.05551.x
48. Schaefer SY, Haaland KY, Sainburg RL. Ipsilesional motor deficits following stroke reflect hemispheric specializations for movement control. Brain. (2007) 130:2146–58. doi: 10.1093/brain/awm145
49. Sharma N, Baron J-C, Rowe JB. Motor imagery after stroke: relating outcome to motor network connectivity. Ann Neurol. (2009) 66:604–16. doi: 10.1002/ana.21810
50. Grefkes C, Nowak DA, Eickhoff SB, Dafotakis M, Küst J, Karbe H, et al. Cortical connectivity after subcortical stroke assessed with functional magnetic resonance imaging. Ann Neurol. (2008) 63:236–46. doi: 10.1002/ana.21228
51. Westlake K, Nagarajan S. Functional connectivity in relation to motor performance and recovery after stroke. Front Syst Neurosci. (2011) 5:8. doi: 10.3389/fnsys.2011.00008
52. Mäkelä JP, Salmelin R, Kotila M, Salonen O, Laaksonen R, Hokkanen L, et al. Modification of neuromagnetic cortical signals by thalamic infarctions. Electroencephalogr Clin Neurophysiol. (1998) 106:433–43. doi: 10.1016/S0013-4694(98)00005-4
53. Nicolas K, Goodin P, Visser MM, Michie PT, Bivard A, Levi C, et al. Altered functional connectivity and cognition persists 4 years after a transient ischemic attack or minor stroke. Front Neurol. (2021) 12:836. doi: 10.3389/fneur.2021.612177
54. Knott V, Mahoney C, Kennedy S, Evans K. EEG power, frequency, asymmetry and coherence in male depression. Psychiatry Res Neuroimaging. (2001) 106:123–40. doi: 10.1016/S0925-4927(00)00080-9
55. Newson JJ, Thiagarajan TC. EEG frequency bands in psychiatric disorders: a review of resting state studies. Front Hum Neurosci. (2019) 12:521. doi: 10.3389/fnhum.2018.00521
56. Liepert J, Storch P, Fritsch A, Weiller C. Motor cortex disinhibition in acute stroke. Clin Neurophysiol. (2000) 111:671–6. doi: 10.1016/S1388-2457(99)00312-0
57. Shimizu T, Hosaki A, Hino T, Sato M, Komori T, Hirai S, et al. Motor cortical disinhibition in the unaffected hemisphere after unilateral cortical stroke. Brain. (2002) 125:1896–907. doi: 10.1093/brain/awf183
58. Rau C, Plewnia C, Hummel F, Gerloff C. Event-related desynchronization and excitability of the ipsilateral motor cortex during simple self-paced finger movements. Clin Neurophysiol. (2003) 114:1819–26. doi: 10.1016/S1388-2457(03)00174-3
Keywords: magnetoencephalography, minor stroke, beta band power, rolandic beta, Event Related Synchronization
Citation: Kulasingham JP, Brodbeck C, Khan S, Marsh EB and Simon JZ (2022) Bilaterally Reduced Rolandic Beta Band Activity in Minor Stroke Patients. Front. Neurol. 13:819603. doi: 10.3389/fneur.2022.819603
Received: 21 November 2021; Accepted: 14 February 2022;
Published: 28 March 2022.
Edited by:
Pavel Lindberg, INSERM U1266 Institut de Psychiatrie et Neurosciences de Paris, FranceReviewed by:
Jyrki Mäkelä, Hospital District of Helsinki and Uusimaa, FinlandEstelle Pruvost-Robieux, Groupe Hospitalier Universitaire Paris, France
Francesco Motolese, Campus Bio-Medico University, Italy
Copyright © 2022 Kulasingham, Brodbeck, Khan, Marsh and Simon. This is an open-access article distributed under the terms of the Creative Commons Attribution License (CC BY). The use, distribution or reproduction in other forums is permitted, provided the original author(s) and the copyright owner(s) are credited and that the original publication in this journal is cited, in accordance with accepted academic practice. No use, distribution or reproduction is permitted which does not comply with these terms.
*Correspondence: Joshua P. Kulasingham, am9zaHVhcGtAdGVycG1haWwudW1kLmVkdQ==
†These authors have contributed equally to this work and share last authorship