- 1Department of Radiology Children's Hospital of Chongqing Medical University, National Clinical Research Center for Child Health and Disorders, Ministry of Education Key Laboratory of Child Development and Disorders, Chongqing Key Laboratory of Pediatrics, Chongqing, China
- 2GE Healthcare, MR Research China, Beijing, China
- 3Department of Cardiovascular and Thoracic Surgery, Children's Hospital of Chongqing Medical University, Chongqing, China
Objective: To explore the application of three-dimensional pseudocontinuous arterial spin labeling (3D-PCASL) perfusion imaging in the brains of children with autism and to understand the characteristics of cerebral blood perfusion in children with autism.
Methods: A total of 320 children with autism (160 men and 160 women) aged between 2 and 18 years and 320 age- and sex-matched healthy children participated in the study. All children were scanned by 3.0 T magnetic resonance axial T1 fluid-attenuated inversion recovery (FLAIR), T2 FLAIR, 3D-T1, and 3D-PCASL sequences. After postprocessing, cerebral blood flow (CBF) values in each brain region of children with autism and healthy children at the same age were compared and analyzed. Furthermore, CBF characteristics in each brain region of autistic children at various ages were determined.
Results: The CBF values of the frontal lobe, hippocampus, temporal lobe, and caudate nucleus of children with autism are lower than those of healthy children (P < 0.05). Additionally, as the ages of children with autism increase, the number of brain regions with decreased CBF values gradually increases. A receiver operating characteristic (ROC) analysis results show that the CBF values of the frontal lobe, hippocampus, temporal lobe, and caudate nucleus can distinguish children with autism [area under the ROC curve (AUC) > 0.05, P < 0.05].
Conclusion: The 3D-PCASL shows lower brain CBF values in children with autism.
Clinical Trial Registration: www.ClinicalTrials.gov, identifier: ChiCTR2000034356.
Key Points
- Three-dimensional pseudocontinuous arterial spin labeling shows lower brain CBF values in children with autism.
- The temporal lobe, frontal lobe, hippocampus, and caudate nucleus can distinguish children with autism [area under the ROC curve (AUC) > 0.5, P < 0.05].
- The temporal lobe, frontal lobe, hippocampus, and caudate nucleus regions may be the first areas to show cerebral blood flow changes in autistic children.
Introduction
Autism spectrum disorder (ASD) refers to a group of developmental disorders that start in early childhood, with high incidence and unclear causes (1). ASD is a multifactorial disease related to genetics, neurodevelopment, social psychology, etc. (2, 3). Some pathogenic factors have not been clearly identified (4, 5). For example, studies have found that some brain regions of children with autism have abnormal anatomical structures or abnormal brain function activities (6, 7), but whether cerebral blood perfusion in abnormal brain regions is also abnormal is unknown.
In previous studies, some researchers have used single-photon emission computed tomography (SPECT) to determine cerebral blood perfusion in children with ASD (8, 9). SPECT is an instrument that uses radioisotopes as tracers to obtain blood flow values in various regions of children's brains, which is harmful to the human body, and children are at a high risk of adverse effects due to γ-ray (radioactive isotope decay produces γ- ray) radiation exposure (10, 11). Therefore, identifying a technology with no impact on children's bodies to determine their brain blood flow is a study hotspot for medical researchers.
Three-dimensional pseudocontinuous arterial spin labeling (3D-PCASL) is a technique for image perfusion that uses continuous labeling (12, 13). This technique is non-invasive and can be used to perform functional imaging and evaluation of the whole brain repeatedly without the need for contrast agent injection. It has been widely used in clinical practice (14, 15). At present, more reports are available on the application of 3D-PCASL technology to adult brain examinations (16), while fewer reports are available on its application to pediatric brain examinations, with an especially low number of reports on its application to head examinations in children with autism (17, 18).
In this study, 3D-PCASL cerebral blood perfusion imaging was used to obtain the blood perfusion value of each brain region of the children's brains. The blood perfusion values of various brain regions of children with autism and healthy children aged 2–18 years were compared and analyzed to determine differences in the blood perfusion value of each brain area between children with autism and healthy children such that the brain blood perfusion characteristics of children with autism can be accurately identified as soon as possible, children with autism can receive a timely diagnosis and reasonable treatment, the severity of the illness can be improved, and the burden on the family and society can be reduced.
Materials and Methods
Ethics Statement
The study protocol was approved by the Human Ethics Committee of the Children's Hospital of Chongqing Medical University (No. 2018-47). Written informed consent was obtained from the parents or guardians of all the children before the examinations.
Study Participants
Study group: A total of 431 children with autism aged 2 to 18 years were selected, from June 2018 to March 2021, and 320 children (men 160, women 160) were included in the study. Control group: A total of 401 healthy children aged 2 to 18 years were selected from February 2019 to April 2021, and 320 children (men 160, women 160) were included in the study. Both groups of children were divided into eight subgroups with 40 in each subgroup according to age (2, 3, 4, 5, 6–8, 9–10, 11–14, 15–18 years old). Children who were excluded from the study had abnormal brain lesions or did not cooperate with sedation, resulting in breathing artifacts on imaging (Table 1).
The inclusion criteria for children in the control group: a body mass index of 15–18 kg/m2, right-handedness, no neurological disease, no other organ-related diseases, no other diseases that may affect brain function and structure, and no abnormalities on routine head MRI.
The inclusion criteria for the children in the study group: a body mass index of 15–18 kg/m2 and right-handedness. The children were required to meet the diagnostic criteria of the Diagnostic and Statistical Manual of Mental Disorders (DSM-V) for autism (19), with no neurological disease, no other organ-related diseases, no other diseases that may affect brain function and structure, no history of medical treatment, and no abnormalities on routine head MRI; the children with ASD in the study group were diagnosed at the outpatient clinic of a physician with an associate senior title or above in the Children's Developmental Behavior and Child Health Department and the Department of Psychology of Children's Hospital of Chongqing Medical University. Children with ASD included in the study had to meet the diagnostic criteria for autism in the fifth edition of the Diagnostic and Statistical Manual of Mental Disorders and have a Childhood Autism Rating Scale (CARS) score ≥30.
All children who did not cooperate with the examination were examined in the sedation center of our hospital after sedation. The sedation method was as follows: dexmedetomidine 3 μg/kg intranasally + chloral hydrate 40 mg/kg orally. If the sedation depth was not sufficient after 20 min, dexmedetomidine 1 μg/kg was administered intranasally again, and if necessary, chloral hydrate 20 mg/kg was administered orally.
Imaging Data Collection and Postprocessing
In this study, a GE 3.0 T magnetic resonance (Discovery MR750; GE Medical Systems, Milwaukee, WI, USA) scanner with an eight-channel head-neck joint coil was used. Children who did not cooperate with the examination were sent to the sedation center for sedation and axial T1 FLAIR, T2 FLAIR, T2 weighted imaging (WI), 3D-T1, and 3D-PCASL sequence scans after deep sleep were achieved. 3D-PCASL: repetition time (TR): 4,628 ms, field of view (FOV): 25 cm, time of echo (TE): 10.4 ms, number of excitations (NEX): three times, slice thickness: 4.2 mm, 32 slices, post labeling delay (PLD): 1,525 ms, and scanning time: 4 min 29 s. 3D-T1: TR: 450 ms, FOV: 25 cm, TE: 3.1 ms, NEX: 1 time, slice thickness: 1 mm, 152 slices, and scanning time: 3 min 43 s. The range of all sequence scanning was from skull top to skull base, including the whole brain.
Data Analysis
To calculate the quantitative parameters, including the gray matter volume (GMV), white matter volume (WMV), and CBF values of different brain regions, we followed an atlas-based image processing approach. CBF maps were further calculated from the 3D-PCASL data using an AW4.6 GE Workstation. Second, we performed rigid registration between CBF maps and 3D-T1WI data using SPM12 software (http://www.fil.ion.ucl.ac.uk/spm/) based on MATLAB (MathWorks, Natick, MA, USA). Third, 3D-T1WI images were segmented and nonlinearly normalized into MNI space using the CAT12 toolbox (http://www.neuro.uni-jena.de/cat/) implemented in SPM12 to obtain tissue probability maps and normalized CBF quantitative maps. Finally, the brain was parcellated into 38 anatomical regions based on the automated anatomical labeling atlas (AAL-3) for quantitative parameter extraction. Brain regional volumes and CBF can be extracted by averaging the values from voxels in specific brain regions. The estimated global GMV and WMV were further normalized by correction of the intracranial volume (Figures 1, 2).
Statistical Analysis
In this study, SPSS 25.0 statistical software was used, and the measurement data were expressed as the mean and standard deviation. The 3D-PCASL perfusion CBF value of the same brain region in children of the same age in the study group and the control group was compared by two-independent samples t-tests (P < 0.05 indicates that a difference was statistically significant); the volume of the same brain region in children of the same age in the study group and the control group was compared by two-independent samples t-tests (P < 0.05 indicates that a difference was statistically significant).
A receiver operating characteristic (ROC) curve was used to evaluate the diagnostic value of the CBF value for the diagnosis of childhood autism. An area under the ROC curve (AUC) > 0.5 with statistical significance was considered indicative of diagnostic value. A value closer to one corresponded to a higher diagnostic value.
Results
Comparison of CBF Values in the Same Age Group and the Same Brain Region
In the group with 2-year-old children, the CBF values of the temporal lobe, hippocampus, and putamen in children with autism were lower than those in healthy children (P < 0.05); in the group containing 3-year-old children, the CBF values of the frontal lobe, temporal lobe, hippocampus, and putamen in children with autism were lower than those in healthy children (P < 0.05); in the group with 4-year-old children, the CBF values of the temporal lobe, frontal lobe, thalamus, hippocampus, putamen, and caudate nucleus in children with autism were lower than those in healthy children (P < 0.05); in the group containing 5-year-old children, the CBF values of the temporal lobe, thalamus, hippocampus, putamen, caudate nucleus, substantia nigra, and red nucleus in children with autism were lower than those in healthy children (P < 0.05); and in the group with 6–18-year-old subjects, the CBF values of the frontal lobe, temporal lobe, thalamus, hippocampus, caudate nucleus, substantia nigra, and red nucleus in children with autism were lower than those in healthy children (P < 0.05) (Table 2, Figures 3, 4).
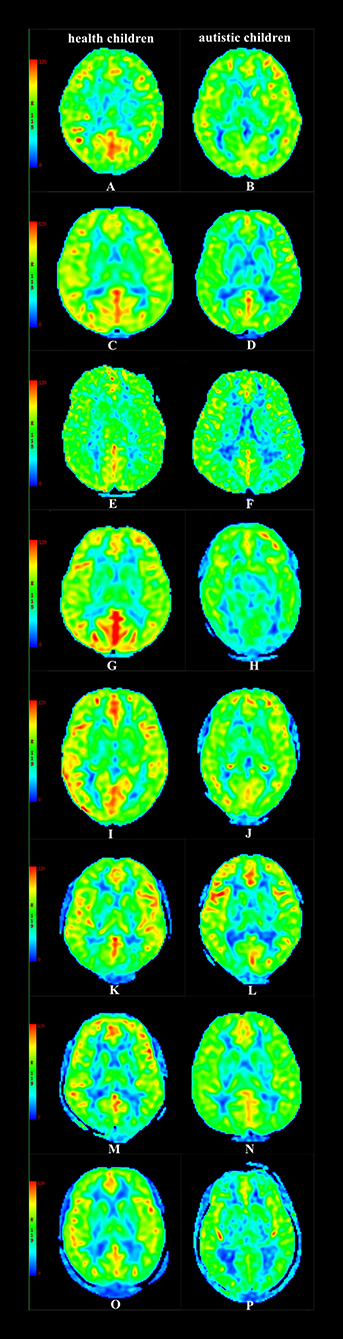
Figure 4. CBF maps (A) Male, 2.3 y, healthy children. (B) Female, 2.4 y, autistic children. (C) Male, 3.3 y, healthy children. (D) Male, 3.6 y, autistic children. (E) Female, 4.5 y, healthy children. (F) Male, 4.4 y, autistic children. (G) Male, 5.6 y, healthy children. (H) Male, 5.4 y, autistic children. (I) Female, 6.9 y, healthy children. (J) Male, 6.7 y, autistic children. (K) Male, 9.7 y, healthy children. (L) Male, 9.9 y, autistic children. (M) Male, 12.7 y, healthy children. (N) Famle, 12.8 y, autistic children. (O) Male, 16.8 y, healthy children. (P) Male, 16.6 y, autistic children.
Volume Comparison of the Same Age Group and the Same Brain Region
In the group with 2–5-year-old children, no statistically significant difference in the volume of the same brain region was found between children with autism and healthy children in the same age group; in the group containing 6- to 8-year-olds, the volumes of the frontal lobe, temporal lobe, hippocampus, and putamen in healthy children were smaller than those in children with autism (P < 0.05); in the group with 9- to 10-year-old children, the volumes of the temporal lobe, frontal lobe, thalamus, hippocampus, putamen, and caudate nucleus in healthy children were smaller than those in children with autism (P < 0.05); in the group with 11-to 18-year-old subjects, the volumes of the frontal lobe, temporal lobe, thalamus, hippocampus, putamen, caudate nucleus, substantia nigra, and red nucleus in healthy children were smaller than those in children with autism (P < 0.05) (Table 3).
The ROC analysis results showed that the CBF values of the frontal lobe, hippocampus, temporal lobe, and caudate nucleus could distinguish children with autism (AUC > 0.05, P < 0.05), and the AUC value of the temporal lobe was the highest (Figure 5, Table 4).
Discussion
The study results imply that the decrease in the CBF value in children with autism will increase with age. The decreases in CBF values in these brain regions may be related to cognitive, language, and motor retardation in children with autism (when a child with autism is ~2 years old, compared with a healthy child, the gaps in cognitive, language, and motor development are smaller. As the age of children with autism increases, the gaps between their cognitive, language, and motor development gradually increases compared with that in healthy children, eventually leading to a decrease in blood perfusion and a gradual increase in the number of affected brain regions) (20–23).
The study results show that except for the 2-year-old and 5-year-old groups, the CBF value of the frontal lobe in autistic children in the other groups was lower than that in healthy children, and the CBF value for blood perfusion in the 3-year-old group was significantly lower than that in the 4-year-old group. The reason for this phenomenon may be that the growth and development of the frontal lobe of autistic children begin to slow down from the age of ~3 years, resulting in a decline in frontal lobe blood flow and perfusion, and then the growth and development of the frontal lobe gradually recover and finally return to normal at the age of ~5 years. However, after the age of 6 years, the frontal lobe's blood flow and perfusion of children with autism gradually decrease (24–27).
In this study, we selected the basal ganglia, frontal lobe, temporal lobe, hippocampus, and other brain regions as key research areas because these are important neurological functional areas (28). The basal ganglia of the brain, which is located deep in the brain, controls and regulates motor functions together with the cerebral cortex and cerebellum (29). The frontal lobe is the most developed brain lobe and has a complex structure and extensive neural connections, including movement, memory, judgment, analysis, thinking, and other functions (30). Abnormalities in the frontal lobe can cause abnormalities in movement, memory, and other functions (31). The hippocampus is closely related to the functions of learning, memory, emotion, and movement, and abnormalities in the hippocampus can cause abnormalities in its functions (32, 33). The results of the study showed that the CBF values of the caudate nucleus, basal ganglia, hippocampus, frontal lobe, temporal lobe, and other brain regions were lower than those in healthy children. The decrease in the CBF value may affect the development of nerve cells, leading to abnormal neurodevelopment in the brain and ultimately leading to abnormal behavior in children with autism (34, 35).
The study results show no statistically significant difference in the volume of the same brain region in children with autism at the same age in the 2- to 5-year-old group, however, the CBF value for blood perfusion in some brain regions of children with autism of the same age was lower than that in the corresponding brain regions of healthy children, suggesting that the decrease in the CBF value for cerebral perfusion in some brain regions of children with autism is unrelated to the volumes of brain regions and may be related to cognitive, language, and motor retardation in children with autism (36, 37); the study results show that in the 6- to 18-year-old group, the volumes of some brain regions of healthy children in the same age group were lower than those in the corresponding brain regions of children with autism, possibly because as the ages of children with autism increases, cerebral blood perfusion decreases, the number of affected brain regions increases, and long-term cerebral blood perfusion is insufficient, which eventually leads to abnormal brain regions (38, 39).
The study results show that the temporal lobe, frontal lobe, hippocampus, and caudate nucleus can distinguish children with autism (AUC > 0.5, P < 0.05). Therefore, these four brain regions can be used as key brain regions for brain imaging diagnosis in children with autism. If the CBF values of the above four brain regions are reduced and the clinical symptoms are consistent with the characteristics of autism, the clinician can diagnose the child with autism and give reasonable treatment in time to improve the condition of the child.
Limitations of This Study
This study is not a multicenter study, and the results of the study may be regional; some of the children included in the study were examined after sedation, which may affect the state of cerebral blood flow and ultimately lead to a certain deviation in the study results (Ogawa found that patients' brain CBF values decreased after sedation). The above shortcomings will be further studied in the future (40, 41).
In summary, 3D-PCASL shows lower brain CBF values in children with autism, and with increases in the ages of children with autism, the number of brain regions with decreased CBF values gradually increases.
Data Availability Statement
Publicly available datasets were analyzed in this study. This data can be found here: Figshare, https://figshare.com/s/9ecf5f9eeafd809fe53b.
Ethics Statement
The study protocol was approved by the Human Ethics Committee of the Children's Hospital of Chongqing Medical University (No. 2018-47). Written informed consent to participate in this study was provided by the participants' legal guardian/next of kin.
Author Contributions
ST, ZP, and LH: experimental design and project management. XL: statistical analysis and image analysis. QR and LW: data acquisition and data analysis. LN: software support. All authors contributed to the article and approved the submitted version.
Funding
This study was supported by the Chongqing Municipal Education Commission (No. KJQN202000425) and National Clinical Research Center for Child Health and Disorders (No. NCRCCHD-2021-YP-07).
Conflict of Interest
LN was employed by GE Healthcare and MR Research China.
The remaining authors declare that the research was conducted in the absence of any commercial or financial relationships that could be construed as a potential conflict of interest.
Publisher's Note
All claims expressed in this article are solely those of the authors and do not necessarily represent those of their affiliated organizations, or those of the publisher, the editors and the reviewers. Any product that may be evaluated in this article, or claim that may be made by its manufacturer, is not guaranteed or endorsed by the publisher.
Acknowledgments
The authors would like to thank LN (GE Healthcare, MR Research China, Beijing) for providing software support.
Abbreviations
MRI, magnetic resonance imaging; 3D-PCASL, three-dimensional pseudocontinuous arterial spin labeling; ASD, autism spectrum disorder; DSM-V, Diagnostic and Statistical Manual of Mental Disorders, Fifth Edition; MCV, mean corpuscular volume; VBM, voxel-based morphology; CBF, cerebral blood flow; PLD, post labeling delay; FLAIR, fluid attenuated inversion recovery; T2WI, weighted imaging; TR, repetition time; FOV, field of view; TE, time of echo; NEX, number of excitations.
References
1. Gabrielsen TP, Anderson JS, Stephenson KG, Beck J, King JB, Kellems R, et al. Functional MRI connectivity of children with autism and low verbal and cognitive performance. Mol Autism. (2018) 27:67–75. doi: 10.1186/s13229-018-0248-y
2. Cox AD, Virues-Ortega J, Julio F, Martin TL. Establishing motion control in children with autism and intellectual disability: applications for anatomical and functional. MRI J Appl Behav Anal. (2017) 50:8–26. doi: 10.1002/jaba.351
3. Peterson BS, Zargarian A, Peterson JB, Goh S, Sawardekar S, Williams SCR, et al. Hyperperfusion of frontal white and subcortical gray matter in autism spectrum disorder. Biol Psychiatry. (2019) 85:584–95. doi: 10.1016/j.biopsych.2018.11.026
4. Dell'Osso L, Lorenzi P, Carpita B. Autistic traits and illness trajectories. Clin Pract Epidemiol Ment Health. (2019) 30:94–8. doi: 10.2174/1745017901915010094
5. Nuntanee S, Daranee S. Effect of motorized elephant-assisted therapy program on balance control of children with autism spectrum disorder. Occup Ther Int. (2019) 2019:5914807. doi: 10.1155/2019/5914807
6. Dean DC, Freeman A, Lainhart J. The development of the social brain in baby siblings of children with autism. Curr Opin Psychiatry. (2020) 33:110–6. doi: 10.1097/YCO.0000000000000572
7. Sen B, Borle NC, Greiner R, Brown MRG. A general prediction model for the detection of ADHD and Autism using structural and functional MRI. PLoS ONE. (2018) 13:e0194856. doi: 10.1371/journal.pone.0194856
8. Kowalewska B, Drozdz W, Kowalewski L. Positron emission tomography (PET) and single-photon emission computed tomography (SPECT) in autism research: literature review. Ir J Psychol Med. (2021) 5:1–15. doi: 10.1017/ipm.2021.15
9. Kaneta T. PET and SPECT imaging of the brain: a review on the current status of nuclear medicine in Japan. Jpn J Radiol. (2020) 38:343–57. doi: 10.1007/s11604-019-00901-8
10. Juhász C, John F. Utility of MRI, PET, and ictal SPECT in presurgical evaluation of non-lesional pediatric epilepsy. Seizure. (2020) 77:15–28. doi: 10.1016/j.seizure.2019.05.008
11. Mir A, Baradie RA, Alnaim A, Moinuddin A, Khan MI, Jallul T, et al. Utility of single-photon emission computed tomography (SPECT) in presurgical evaluation of children: a single-center experience. Epilepsy Res. (2020) 167:106445. doi: 10.1016/j.eplepsyres.2020.106445
12. Zeng Q, Jiang B, Shi F, Ling C, Dong F, Zhang J. 3D Pseudocontinuous arterial spin-labeling MR imaging in the preoperative evaluation of gliomas. AJNR Am J Neuroradiol. (2017) 38:1876–83. doi: 10.3174/ajnr.A5299
13. Sogabe S, Satomi J, Tada Y, Kanematsu Y, Kuwayama K, Yagi K, et al. Intra-arterial high signals on arterial spin labeling perfusion images predict the occluded internal carotid artery segment. Neuroradiology. (2017) 59:587–94. doi: 10.1007/s00234-017-1828-9
14. Wong AM-C, Yeh C-H, Liu H-L, Wu T-W, Lin K-L, Wang H-S, et al. Arterial spin-labeling perfusion imaging of children with subdural hemorrhage: perfusion abnormalities in abusive head trauma. J Neuroradiol. (2017) 44:281–7. doi: 10.1016/j.neurad.2017.02.003
15. Zhang N, Gordon ML, Goldberg TE. Cerebral blood flow measured by arterial spin labeling MRI at resting state in normal aging and Alzheimer's disease. Neurosci Biobehav Rev. (2017) 72:168–175. doi: 10.1016/j.neubiorev.2016.11.023
16. Li D, Liu Y, Zeng X, Xiong Z, Yao Y, Liang D, et al. Quantitative study of the changes in cerebral blood flow and iron deposition during progression of alzheimer's disease. J Alzheimers Dis. (2020) 78:439–52. doi: 10.3233/JAD-200843
17. Tang S, Liu X, He L, Liu B, Qin B, Feng C. Application of a 3D pseudocontinuous arterial spin-labeled perfusion MRI scan combined with a postlabeling delay value in the diagnosis of neonatal hypoxic-ischemic encephalopathy. PLoS ONE. (2019) 14:e0219284. doi: 10.1371/journal.pone.0219284
18. Tang S, Liu X, He L, Liu B, Qin B, Feng C. Application of postlabeling delay time in 3-dimensional pseudocontinuous arterial spin-labeled perfusion imaging in normal children. J Comput Assist Tomogr. (2019) 43:697–707. doi: 10.1097/RCT.0000000000000911
19. Wakefield JC. Diagnostic issues and controversies in DSM-5: return of the false positives problem. Annu Rev Clin Psychol. (2016) 12:105–32. doi: 10.1146/annurev-clinpsy-032814-112800
20. Oliver LD, Moxon-Emre I, Lai MC, Grennan L, Voineskos AN, Ameis SH. Social cognitive performance in schizophrenia spectrum disorders compared with autism spectrum disorder: a systematic review, meta-analysis, and meta-regression. JAMA Psychiatry. (2021) 78:281–92. doi: 10.1001/jamapsychiatry.2020.3908
21. Ozsivadjian A, Hollocks MJ, Magiati I, Happé F, Baird G, Absoud M. Is cognitive inflexibility a missing link? the role of cognitive inflexibility, alexithymia and intolerance of uncertainty in externalising and internalising behaviours in young people with autism spectrum disorder. J Child Psychol Psychiatry. (2021) 62:715–24. doi: 10.1111/jcpp.13295
22. Uddin LQ. Brain mechanisms supporting flexible cognition and behavior in adolescents with autism spectrum disorder. Biol Psychiatry. (2021) 89:172–83. doi: 10.1016/j.biopsych.2020.05.010
23. An K-M, Ikeda T, Hirosawa T, Yaoi K, Yoshimura Y, Hasegawa C. Decreased grey matter volumes in unaffected mothers of individuals with autism spectrum disorder reflect the broader autism endophenotype. Sci Rep. (2021) 11:10001. doi: 10.1038/s41598-021-89393-z
24. Williams CM, Peyre H, Toro R, Beggiato A, Ramus F. Adjusting for allometric scaling in ABIDE I challenges subcortical volume differences in autism spectrum disorder. Hum Brain Mapp. (2020) 41:4610–29. doi: 10.1002/hbm.25145
25. Famitafreshi H, Karimian M. Overview of the recent advances in pathophysiology and treatment for autism. CNS Neurol Disord Drug Targets. (2018) 17:590–4. doi: 10.2174/1871527317666180706141654
26. Molnar-Szakacs I, Kupis L, Uddin LQ. Neuroimaging markers of risk and pathways to resilience in autism spectrum disorder. Biol Psychiatry Cogn Neurosci Neuroimaging. (2021) 6:200–10. doi: 10.1016/j.bpsc.2020.06.017
27. Pan PY, Bölte S, Kaur P, Jamil S, Jonsson U. Neurological disorders in autism: a systematic review and meta-analysis. Autism. (2021) 25:812–30. doi: 10.1177/1362361320951370
28. Riva D, Taddei M, Bulgheroni S. The neuropsychology of basal ganglia. Eur J Paediatr Neurol. (2018) 22:321–6. doi: 10.1016/j.ejpn.2018.01.009
29. Bostan AC, Dum RP, Strick PL. Functional anatomy of basal ganglia circuits with the cerebral cortex and the cerebellum. Prog Neurol Surg. (2018) 33:50–61. doi: 10.1159/000480748
30. Yanagisawa N. Functions and dysfunctions of the basal ganglia in humans. Proc Jpn Acad Ser B Phys Biol Sci. (2018) 94:275–304. doi: 10.2183/pjab.94.019
31. Pierce JE, Péron J. The basal ganglia and the cerebellum in human emotion. Soc Cogn Affect Neurosci. (2020) 15:599–613. doi: 10.1093/scan/nsaa076
32. Oades RD. Frontal, temporal and lateralized brain function in children with attention-deficit hyperactivity disorder: a psychophysiological and neuropsychological viewpoint on development. Behav Brain Res. (1998) 94:83–95. doi: 10.1016/S0166-4328(97)00172-1
33. Alsultan F, Alaboudi M, Almousa A, Alajaji R, Bashir S. Effects of transcranial direct current stimulation over frontal, parietal and cerebellar cortex for cognitive function during fasting in healthy adults. IBRO Rep. (2020) 25:129–35. doi: 10.1016/j.ibror.2020.03.002
34. Sousa AMM, Meyer KA, Santpere G, Gulden FO, Sestan N. Evolution of the human nervous system function, structure, and development. Cell. (2017) 170:226–47. doi: 10.1016/j.cell.2017.06.036
35. Matsudaira I, Kawashima R, Taki Y. [Structural Brain Development in Healthy Children and Adolescents]. Brain Nerve. (2017) 69:539–45. doi: 10.11477/mf.1416200780
36. Sathyanesan A, Zhou J, Scafidi J, Heck DH, Sillitoe RV, Gallo V. Emerging connections between cerebellar development, behaviour and complex brain disorders. Nat Rev Neurosci. (2019) 20:298–313. doi: 10.1038/s41583-019-0152-2
37. Khundrakpam BS, Lewis JD, Zhao L, Chouinard-Decorte F, Evans AC. Brain connectivity in normally developing children and adolescents. Neuroimage. (2016) 1:192–203. doi: 10.1016/j.neuroimage.2016.03.062
38. Dow-Edwards D, MacMaster FP, Peterson BS, Niesink R, Andersen S, Braams BR. Experience during adolescence shapes brain development: From synapses and networks to normal and pathological behavior. Neurotoxicol Teratol. (2019) 11:106834. doi: 10.1016/j.ntt.2019.106834
39. Clark GD. Brain development and the genetics of brain development. Neurol Clin. (2002) 20:917–39. doi: 10.1016/S0733-8619(02)00024-5
40. Liang P, Xu Y, Lan F, Ma D, Li K. Decreased cerebral blood flow in mesial thalamus and precuneus/PCC during midazolam induced sedation assessed with ASL. Neuroinformatics. (2018) 16:403–10. doi: 10.1007/s12021-018-9368-y
Keywords: brain, MRI, children, autism, three-dimensional arterial spin labeling
Citation: Tang S, Liu X, Ran Q, Nie L, Wu L, Pan Z and He L (2022) Application of Three-Dimensional Pseudocontinuous Arterial Spin Labeling Perfusion Imaging in the Brains of Children With Autism. Front. Neurol. 13:851430. doi: 10.3389/fneur.2022.851430
Received: 09 January 2022; Accepted: 24 January 2022;
Published: 23 February 2022.
Edited by:
Anna Maria Lavezzi, University of Milan, ItalyReviewed by:
Song'An Shang, Nanjing Medical University, ChinaNeetu Soni, University of Rochester, United States
Copyright © 2022 Tang, Liu, Ran, Nie, Wu, Pan and He. This is an open-access article distributed under the terms of the Creative Commons Attribution License (CC BY). The use, distribution or reproduction in other forums is permitted, provided the original author(s) and the copyright owner(s) are credited and that the original publication in this journal is cited, in accordance with accepted academic practice. No use, distribution or reproduction is permitted which does not comply with these terms.
*Correspondence: Zhengxia Pan, cGFuemhlbmd4aWEwMDVAc2luYS5jb20=; Ling He, NDgwMDU2QGhvc3BpdGFsLmNxbXUuZWR1LmNu