- 1Department of Neurosurgery, Dehua County Hospital, Quanzhou, China
- 2Department of Internal Medicine, Dehua County Hospital, Quanzhou, China
- 3Department of Neurosurgery, Yuebei People's Hospital, Shaoguan, China
- 4Department of Neurosurgery, Neurosurgery Research Institute, The First Affiliated Hospital, Fujian Medical University, Fuzhou, China
Purpose: To explore the value of partial pressure of carbon dioxide (PaCO2) levels in arterial blood for predicting immediate seizures (ISs) in patients with primary intracerebral hemorrhage (ICH).
Methods: Demographic information and clinical data from patients with primary ICH were prospectively collected, including arterial blood gas analysis. Immediate seizures (ISs) were determined as seizures in the first 24 h after admission. Univariate and multivariate analyses were performed to assess the association of PaCO2 levels with ISs. Propensity-score matching (PSM) analyses were adopted to reduce the baseline difference between ISs and non-ISs groups.
Results: A total of 596 patients with primary ICH were initially screened in this clinical study, 368 of whom fulfilled all the inclusion criteria [mean age, (60.46 ±12.78) years; 57.9% female patients]. ISs occurred in 30 of the 368 (8.15%) patients with primary ICH of this cohort. Patients with ISs had significantly lower PaCO2 levels [34.35(32.38–37.53) vs. 39.45(35.90–43.43), mmHg, p < 0.001] and were younger than those without ISs [(54.57±12.15 vs. 60.99 ±12.72) years, p = 0.008]. Multivariate analysis showed that lower initial PaCO2 (≤37.2 mmHg) level was a significant independent predictor of ISs [odds ratios (OR) 0.141, 95% confidence interval (CI) 0.057–0.351, p < 0.001], as well as younger age (OR 0.961, 95% CI 0.928–0.995, p = 0.023) and hematoma expansion (OR 0.340, 95% CI 0.134–0.863, p = 0.023). Receiver operating characteristic curve (ROC) analysis demonstrated that the optimal cutoff value of PaCO2 level for predicting ISs was 37.20 mmHg in patients with primary ICH (the area under the curve (AUC) was 0.760 with a corresponding sensitivity of 76.67% and specificity of 67.46%, 95%CI = 0.713–0.802, p < 0.001). After PSM, the matched ISs group had significantly lower PaCO2 levels compared with the matched non-ISs group [34.45(32.43–38.18) vs. 41.75(35.85–43.98) mmHg, p < 0.05] in the univariate analysis. The lower initial PaCO2 level was still independent of ISs following primary ICH.
Conclusions: The lower initial PaCO2 level was associated with an increased risk of ISs in patients with primary ICH.
Introduction
Intracerebral hemorrhage (ICH) occurs in 15–25% of all strokes and is the most devastating and untreatable type of hemorrhagic stroke with a high risk of disability and mortality (1, 2). Seizures are a frequent and intractable complication of ICH, and they vary widely among epidemiological studies, ranging from 2.5 to 28% (3–9), with the majority occurring at or near onset (10, 11). The reported literature generally classifies seizures by the time of onset as immediate (<24 h after admission), early (1–14 days), or late (>2 weeks) (12). Most animal models have shown that the first seizure occurred in the first 24 h post-ICH (13). Clinically, ~90% of the seizures occur within the first 3 days post-ICH (11, 14, 15). Following ICH, the risk factors for immediate seizures (ISs) include young age and lobar location of ICH (5, 12).
Carbon dioxide (CO2) plays a crucial role in neuronal metabolic activity, neurotransmitter function, and regulating cerebral blood flow (CBF) (16, 17). Acute hemorrhagic stroke, including ICH, appears to correlate with a significant decrease in CO2 levels (10). Published animal studies have demonstrated that CO2 levels influence tissue pH, which plays a vital role in developing acute epilepsy (18, 19). Previous literature indicated that CO2 concentrations and respiratory mechanisms might be associated with seizures (20–22). The hippocampus and cerebral cortex, frequently involved in seizures, are susceptible to CO2 and may mediate a neuroendocrine response to CO2 (19). Prior studies demonstrated that lower partial pressure of carbon dioxide (PaCO2) was associated with febrile convulsions or the absence of seizures in children (23, 24). One recent study revealed that early decreased PaCO2 levels in the first 24 h were independently associated with an increased incidence of acute seizures in patients receiving extracorporeal membrane oxygenation for respiratory failure (25). To date, the association of PaCO2 with ISs following ICH has not previously been reported in the literature (12, 26). Also, Hextrum et al. have reported that the initial PaCO2 level is a stronger predictor of disease progression than the 72-h nadir (27). We, therefore, sought to test the hypothesis whether the initial PaCO2 level was associated with ISs in patients with primary ICH.
Materials and Methods
Study Population
Patients with ICH presenting directly to Dehua County Hospital from January 7 2018 to 21 May 2021, were enrolled in the study. Immediate seizures (ISs) were determined as seizures in the first 24 h after admission (5, 12, 28). Neurosurgeons or nurses witnessed ISs in the hospital. The inclusion criteria were: (1) baseline computed tomography (CT) within 6 h after hemorrhage was performed in all patients, and a follow-up CT scan was performed within 24 h; (2) computerized tomography angiography (CTA) was completed within 72 h of admission to exclude cerebrovascular diseases such as intracranial aneurysms, cerebral arteriovenous malformations, and moyamoya disease; (3) arterial blood gas analysis was performed within 2 h of admission. The exclusion criteria were as follows: (1) patients underwent emergency surgery before follow-up CT;(2) patients with suspicious and pre-admission seizures described by family members; (3) patients with hemorrhage induced by brain infarction, vascular malformations, and a brain tumor; (4) an initial ICH volume <1 ml; (5) primary intraventricular hemorrhage (IVH); (6) acute kidney injury or chronic kidney disease; (7) historical modified Rankin Scale (mRS) scores >1; (8) malignant tumor. Study inclusion/exclusion is summarized in Figure 1.
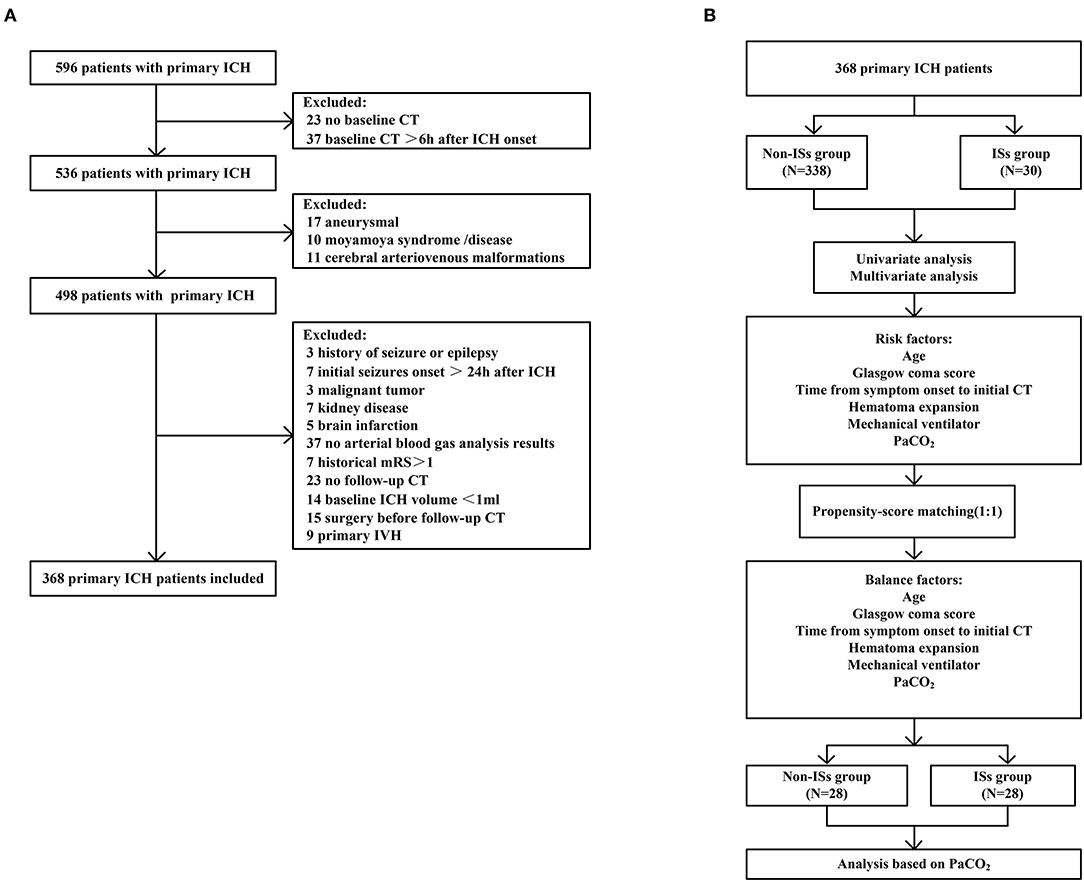
Figure 1. Flow diagram of the patient selection process. (A) Flowchart of patient inclusion; (B) Flowchart of propensity-score matching. ICH, intracerebral hemorrhage; IVH, intraventricular hemorrhage.
Antiepileptic drugs (AEDs), including sodium valproate, phenobarbital, or levetiracetam, were given if clinical seizures were observed. Multiple AEDs were used in combination when seizures were poorly controlled.
Data Collection
Demographic information and clinical data, including age, sex, medical history, vital admission signs, baseline Glasgow coma score (GCS) score, time from symptom onset to initial CT, baseline ICH volume, primary IVH, ICH location, arterial blood gas analysis, admission laboratory, hematoma expansion (HE), treatment received, mechanical ventilator, and all other data related to their hospitalization were prospectively collected. Arterial blood gas analysis, including PaCO2, was conducted within 2 h after arrival at our department and simultaneously obtained using i-STAT Analyzer (Abbott Park MN: 300-G, Singapore).
CT Scan Analysis
Patients with primary ICH were categorized into two groups for the statistical analysis: patients with deep or lobar ICH. Primary ICH with selective involvement of the thalamus, basal ganglia, internal capsule, deep periventricular white matter, or brain stem was defined as deep ICH, whereas ICH isolated in the cortex (with or without subcortical white matter involvement) was defined as lobar ICH (29). Follow-up CT was carried out within 24 h after hospital admission to identify a HE diagnosis. ICH volumes were calculated using a post-processing workstation (Advantage Workstation 4.6, GE Healthcare, Chicago, Illinois, USA) (30). HE was defined as a 33% increase in the hematoma volume or >6 mL on follow-up imaging (31).
Clinical Prognostic Assessment
Functional outcome was measured at 90 days after ICH onset with the modified Rankin Scale (mRS) score. A favorable outcome was defined as an mRS score of 0–2, and an unfavorable outcome was defined as an mRS score of 4 or greater (32).
Statistical Analysis
Normally distributed continuous variables were presented as mean ± standard deviation (SD), non-normally distributed continuous variables as median (interquartile range, IQR), and categorical variables as frequencies (percentages). Median with IQR was shown for violin plots. Categorical data were analyzed by chi-squared test or Fisher's exact test. Normally and non-normally distributed variables were analyzed for significance by Student's t-test or Mann–Whitney U test, respectively. The correlation was determined using the Spearman rank test. Risk factors with p < 0.1 in univariate analyses were included in the multivariate models. Moreover, the receiver operating characteristic (ROC) curve was plotted by MedCalc (MedCalc Software, Ostend, Belgium), and the area under the ROC curve (AUC) was calculated to evaluate the predictive power of PaCO2 for ISs. The best cutoff value of PaCO2 for predicting ISs was selected based on the ROC cutoff value, and patients were divided into “ ≤ optimal cutoff value” and “>optimal cutoff value.” Propensity score matching (PSM) analysis was implemented with a 1:1 nearest-neighbor matching algorithm to balance confounders between the two groups. Variables with p < 0.05 in the univariate analysis were entered into the PSM. All statistical analyses were performed using SPSS Statistics 25.0 software (SPSS Inc., Chicago, USA) and Prism 8.3.0 (GraphPad Software, San Diego, CA, USA). Differences were considered statistically significant when p < 0.05.
Results
As described in Figure 1 (the detailed flow of the selection process), a total of 596 patients with primary ICH were initially screened in this clinical study, 368 of whom fulfilled all the inclusion criteria [mean age (60.46 ±12.78) years; 57.9% female patients]. Thirty patients (8.15%) experienced ISs, and 53 patients developed early HE after primary ICH. The median baseline GCS score was 12.0 (7.0–15.0), and the median baseline ICH volume was 12.0 ml (5.0–27.00 ml). The median time from symptom onset to initial CT was 2 h (1–3 h), and the median time from admission to ISs onset was 3 h (1–7 h). Cumulative ISs rate using Kaplan–Meier analysis within 24 h after admission in the ISs group is illustrated in Figure 2A.
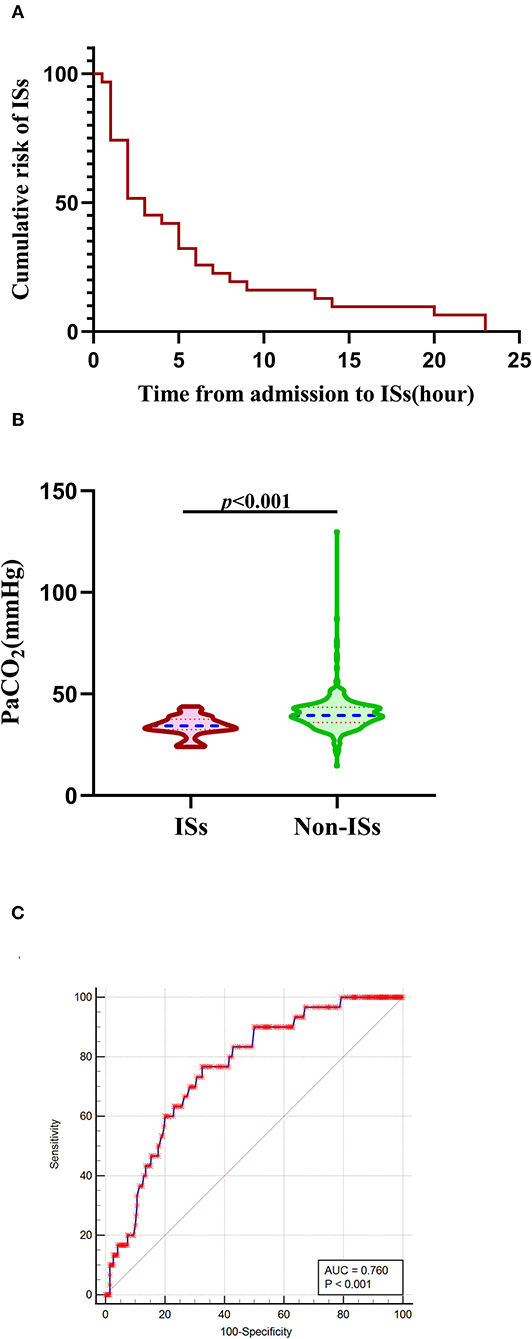
Figure 2. Association of initial PaCO2 levels with ISs. (A) The cumulative risk of ISs using Kaplan-Meier analysis within 24 h after admission in the ISs group. (B) PaCO2 levels in patients with ISs (n = 30) and non-ISs (n = 338). (C) ROC curve analysis for predicting ISs. The optimal cutoff value of PaCO2 level for predicting ISs was 37.20 mmHg in patients with primary ICH (the AUC was 0.760 with a corresponding sensitivity of 76.67% and specificity of 67.46%, 95%CI = 0.713–0.802, p < 0.001). Median with IQR was shown for all violin plots in panel B. Mann–Whitney tests were performed to compare differences between groups. AUC, area under the curve; ICH, intracerebral hemorrhage; ISs, immediate seizures; IQR, interquartile range; PaCO2, partial pressure of carbon dioxide; ROC, Receiver operating curve.
Baseline demographic and clinical characteristics are summarized in Table 1. The 368 patients with primary ICH were stratified into ISs-group (N = 30) and non-ISs group (N = 338). In the univariate analysis, significant differences were observed in age, mechanical ventilator (MV) within 24 h, GCS score, HE, and PaCO2 between the ISs and non-ISs groups (p < 0.05). The PaCO2 level was significantly lower in the ISs group than that in the non-ISs group [34.35 (32.38–37.53) vs. 39.45(35.90–43.43), mmHg, p < 0.001; Table 1 and Figure 2B]. Patients were younger in the ISs group than the non-ISs group (54.57±12.15 vs. 60.99 ±12.72, years, p = 0.008; Table 1). Multicollinearity analyses were carried out among the predictors included in the multivariate analysis model. A variable inflation factor (VIF) > 5 or tolerance <0.2 indicates the existence of multiple collinearity (33). We observed no significant collinearity between the covariates included in the multivariable models as judged by VIF and tolerance (Table 2). Therefore, six covariates were included in stepwise multivariate analysis. In the multivariate logistic regression model, lower initial PaCO2 (≤37.2 mm Hg) level was a significant independent predictor of ISs (OR 0.141, 95% CI 0.057–0.351, p < 0.001), as well as younger age [odds ratios (OR) 0.961, 95% confidence interval (CI) 0.928–0.995, p = 0.023; Table 3)] and HE (OR 0.340, 95% CI 0.134–0.863, p = 0.023; Table 3). However, baseline GCS score and MV within 24 h were not independent predictors of ISs (p > 0.05; Table 3). The Hosmer–Lemeshow test, used to access good-of-fit for multivariate models, indicated that the model fitted to a satisfactory extent (χ2=5.896, p = 0.659). Interestingly, Spearman correlation analysis detected a positive correlation of PaCO2 with GCS score (r = 0.1055, p = 0.0432). ROC analysis for assessing the ability of initial PaCO2 to identify ISs is shown in Figure 2C. The optimal cutoff value of the initial PaCO2 level for predicting ISs was 37.20 mmHg in patients with primary ICH [the area under the curve (AUC) was 0.760 with a corresponding sensitivity of 76.67% and specificity of 67.46%, 95%CI=0.713-0.802, p < 0.001; Figure 2C].
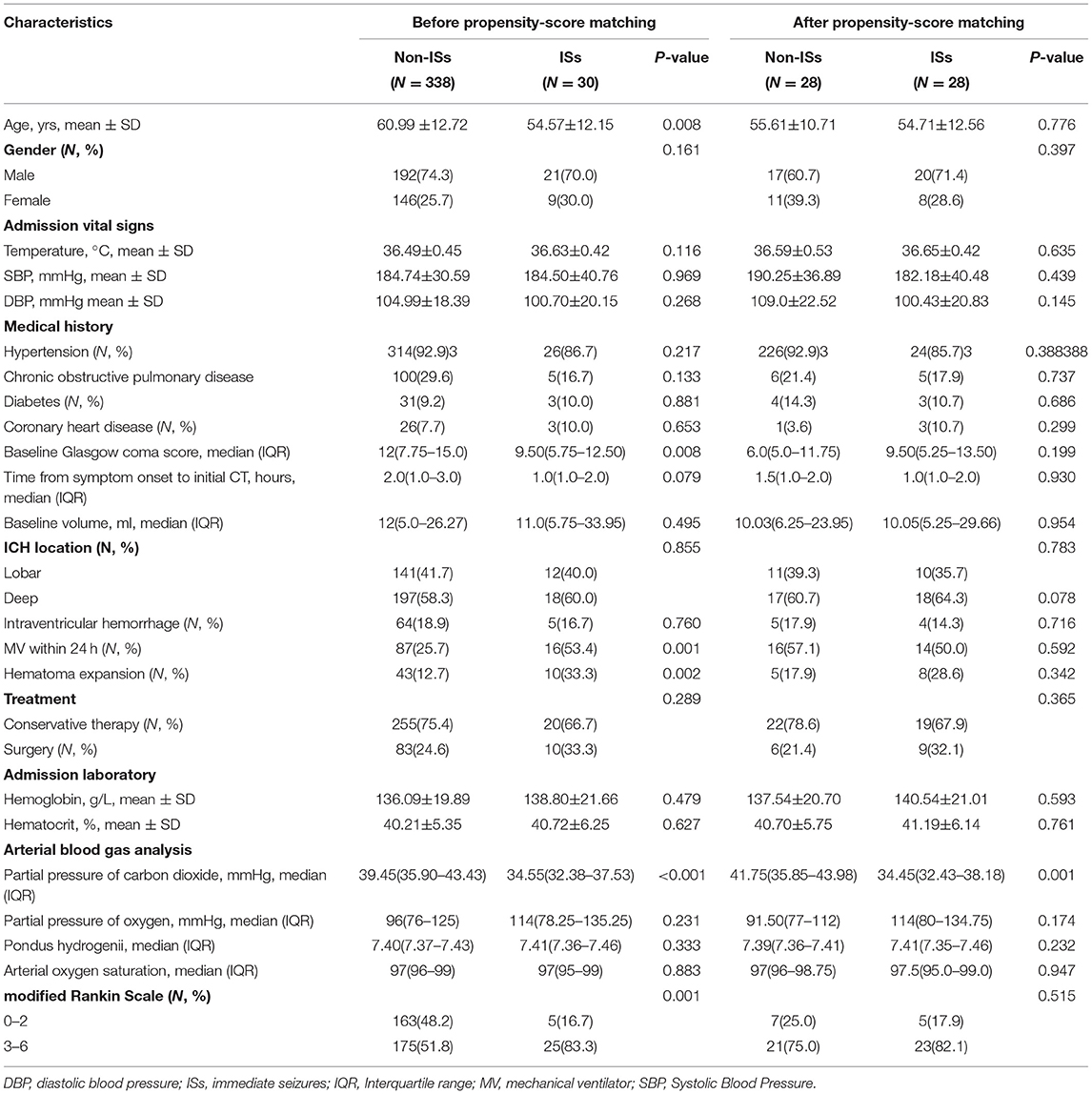
Table 1. Univariate analysis of association with ISs before and after propensity-score matching in spontaneous intracerebral hemorrhage patients.
To further account for significant differences in baseline characteristics between the ISs group and non-ISs group, we conducted a PSM. After PSM, two patients in the ISs group could not be matched. The significant differences in age, MV within 24 h, GCS score, and HE between the two groups were balanced. The matched ISs group had significantly lower PaCO2 levels compared with the matched non-ISs group [34.45(32.43–38.18) vs. 41.75(35.85–43.98) mmHg, p < 0.05; Table 1 and Figure 3A)] in the univariate analysis. The lower initial PaCO2 level was still an independent predictor of ISs. After PSM, the optimal cutoff value for initial PaCO2 levels as a predictor for ISs following primary ICH was determined as 39.4 mmHg (the AUC was 0.765 with a corresponding sensitivity of 89.29% and specificity of 60.71%, 95%CI = 0.632–0.868, p < 0.001; Figure 3B).
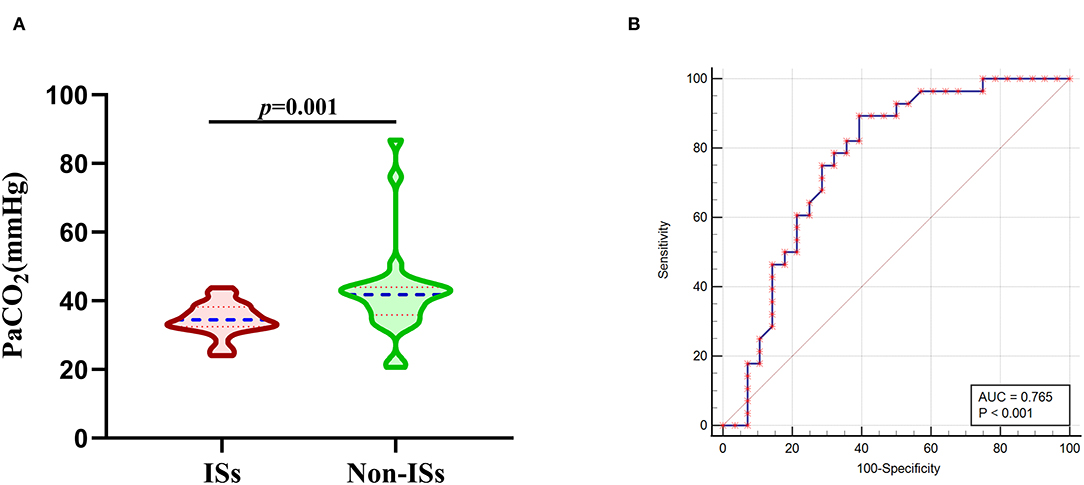
Figure 3. Association of initial PaCO2 levels with ISs after PSM. (A) PaCO2 levels in patients with ISs (n = 28) and non-ISs (n = 28) after propensity-score matching. (B) ROC curve analysis for predicting ISs after PSM. The optimal cutoff value for PaCO2 levels as a predictor for ISs following primary ICH was determined as 39.4mmHg (the AUC was 0.765 with a corresponding sensitivity of 89.29% and specificity of 60.71%, 95%CI = 0.632–0.868). Median with IQR was shown for all violin plots in (A). Mann-Whitney tests were performed to compare differences between groups. AUC, area under the curve; ICH, intracerebral hemorrhage; ISs, immediate seizures; IQR, interquartile range; ISs, immediate seizures; PaCO2, partial pressure of carbon dioxide; ROC, Receiver operating curve.
Patients with ISs had a statistically worse prognosis than patients without ISs (Table 1). For the functional outcome of mRS, the distribution of 90-day mRS of both groups is illustrated in Figure 4. Interestingly, no significant difference was witnessed between the two groups after PSM (Table 1).
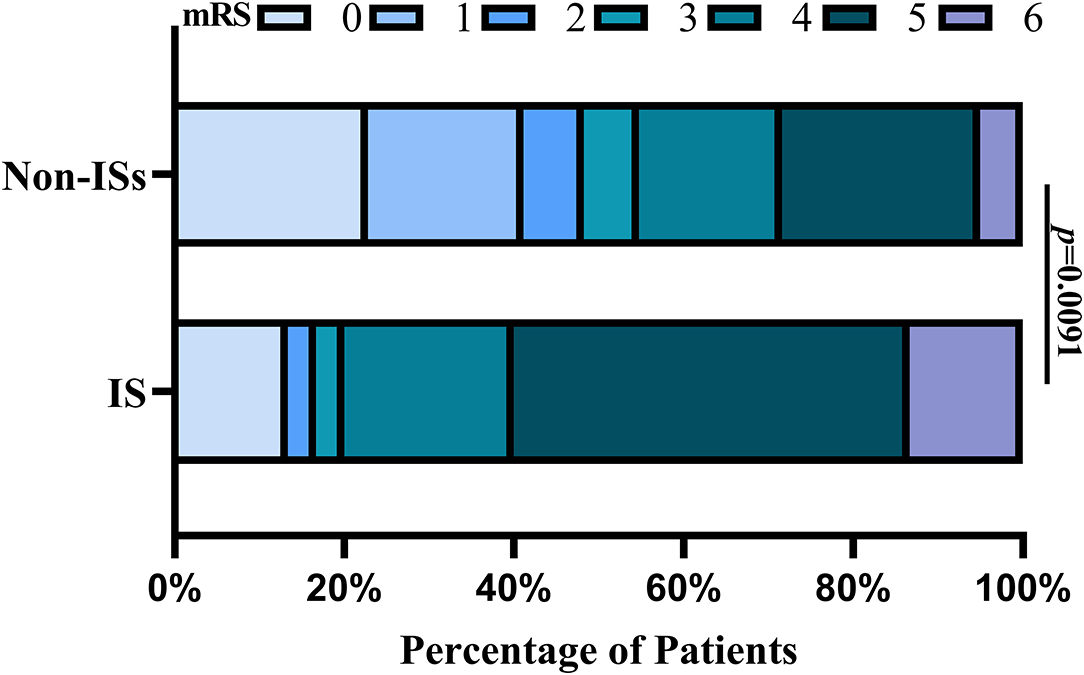
Figure 4. Distributions of mRS scores at 90 days between the ISs and non-ISS groups. Proportions of patients within each score category on the 7-point scale (where o indicates no symptoms and 6 indicates death) at 90 days. ISs, immediate seizures; mRS, modified Rankin Scale.
No statistically significant difference was observed in the PaCO2 level between a favorable outcome and an unfavorable outcome [39.55(36.13–43.07) vs. 39.00(34.63–43.08) mmHg p = 0.341]. ROC analysis indicated an AUC of 0.529 for PaCO2 levels as a prognostic predictor in patients with ICH.
Discussion
Four principal findings emerge from our study:(1) the lower initial PaCO2 (≤37.2 mmHg) level was a significant independent predictor of ISs in patients with primary ICH; (2) HE and younger age were significantly associated with ISs;(3) the initial PaCO2 level was positively associated with GCS score; and (4) patients with ISs had a statistically worse prognosis than patients without ISs, while no significant difference was witnessed between two groups after PSM. Following ICH, reported risk factors for ISs included age and HE (5, 12), which were balanced in this study. After PSM, there were no significant differences in age and HE between the two groups. The matched ISs group had lower initial PaCO2 levels compared with the matched non-ISs group in the univariate analysis. To the best of our knowledge, lower initial PaCO2 was first reported as a risk factor for ISs following ICH.
Acute hemorrhagic stroke was associated with a significant decrease in CO2 levels (10). Decreased PaCO2 can elevate the blood pH, which induces cerebral vasoconstriction in the brain and consequently reduces CBF and intracranial pressure (34, 35). Animal models frequently reported CO2 levels with epilepsy as a trigger for seizures (18, 19). Previous studies demonstrated that lower PaCO2 was associated with febrile convulsions or the absence seizure in children (23, 24). One recent study revealed that early decreased PaCO2 levels in the first 24 h were independently related to an increased incidence of acute seizures in patients receiving extracorporeal membrane oxygenation for respiratory failure (25). This study found that lower initial PaCO2 was a significant independent predictor of ISs following primary ICH, even after adjusting for confounders or PSM.
It has been well documented that CBF is associated with PaCO2 levels, and PaCO2 is one of the most decisive parameters that affect CBF (36). Middle cerebral artery (MCA) flow changes by 4% for every 1 mmHg increase or decrease in PaCO2 between 20 and 80 mmHg (16). Crucially, for every 1 mm Hg incremental lowering of PaCO2, CBF decreases by 3% (27). Prior study has hinted that an association between acute hemorrhagic stroke, lower PaCO2, and brain tissue hypoxia is purported to be driven by the onset of cerebral vasoconstriction (27, 37). Hextrum S and colleagues have reported that lower initial PaCO2 was associated with an increased risk of developing ischemic lesions (27). The vasoconstrictive effects of lower PaCO2 may be compounded by other factors that reduce CBF (acute blood pressure reduction in ICH, lowering intracranial pressure) to increase the risk of secondary ischemic injury (27), which results in the dysfunction of neuronal metabolic activity, thereby producing an acute epileptic seizure. Cerebral ischemia and reperfusion injury exacerbate seizures when CBF gradually returns to normal and subsequent oxygen and arterial PaCO2. In addition, the vasoconstrictive effects of lower PaCO2 reduce hemoglobin oxygen release, increasing neuronal excitability and possibly releasing excitatory toxins such as glutamate (38), which may induce ISs. Decreased PaCO2 can induce seizures by affecting cortical pH, γ-aminobutyric acid (GABA) release, and brain electrical activity (19). Lower cerebrospinal fluid (CSF) PaCO2 levels reduce cerebral blood volume and CBF through cerebral arterial vasoconstriction, which causes ischemia and hypoxia in brain tissue, contributing to ISs following ICH (39).
Clinical observations and experimental animal models have demonstrated that changes in CO2 have significant effects on neuronal excitability and seizure propensity in susceptible individuals (40–42). Reduced CO2 increases neuronal excitability in the hippocampus, contributing to acute seizures (40). The hippocampus, frequently involved in seizures, is susceptible to CO2 and mediates a neuroendocrine response to CO2 (40, 41). In the hippocampal slice preparation, decreasing CO2 levels reduced extracellular adenosine concentration and increased neuronal excitability via adenosine A1 receptors, adenosine triphosphate (ATP) receptors, and ecto ATPase, causing ISs (40).
As is well-known, inflammation response to ICH is a significant factor in acute seizures (26, 43). Shreds of evidence from clinical and experimental studies indicated that brain inflammation plays an essential role in seizures (26, 43). The elevation of inflammatory cytokines, such as tumor necrosis factor and interleukin, were also detected in patients with epilepsy and in epilepsy models (38, 43). Interestingly, lower PaCO2 can increase tumor necrosis factor and interleukin (38, 44). In the present study, decreased PaCO2 level was observed in the ISs group after ICH, supporting a possible link between PaCO2, inflammation, and seizures.
Interestingly, one unexpected finding is that the risk of ISs was no different between those with lobar and deep ICH, unlike the results of previous literature (12). The result is noteworthy for the following reasons. On the one hand, the classification of hematoma location in this study is different from previous literature (12). In a study by Cheng Qian et al. (12), which classified ICH locations into subcortical, thalamic, ganglionic, infratentorial (cerebellum and/or pons), and other hematomas, subcortical hematomas were associated with ISs. On the other hand, prior studies have focused on the relationship between early seizures (1–7 days or 1–14 days) and ICH location, rather than ISs (<24 h after admission) (15, 28). Early seizures and ISs have different temporal boundaries, and so the results are not universally identical.
The prognostic significance of PaCO2 levels in critically ill patients, including ICH, remains controversial. Hextrumet al. have previously demonstrated that lower initial PaCO2 was independently associated with a greater risk of in-hospital death in patients with ICH (27). A recent study has shown that decreased PaCO2 improves cerebral autoregulation and possible outcome in patients following ICH (45). Contrary to previous studies (25, 27, 45), we found that PaCO2 level was not associated with unfavorable outcomes. ROC analysis indicated an AUC of 0.529 for PaCO2 levels as a prognostic predictor in patients with ICH, indicating that the predictive value of PaCO2 level in predicting poor prognosis was weak. Lower initial PaCO2 level may be a marker of illness severity rather than a cause of worse clinical outcomes. This hypothesis supported the current study results of a positive correlation between the initial PaCO2 level and the commonly used disease severity scores-GCS score. The inconsistency of the data obtained in this study with previous studies may be due to the limited sample size and different inclusion and exclusion criteria.
The ISs incidence in our study is in line with the study of Szaflarski et al., demonstrating that 8.4% of patients with ICH had a seizure within the first 24 h of stroke onset in a population-based study (28). According to our definition of ISs, the incidence of ISs in the previous study is 8.4%. Whether there is an independent causal relationship between ISs and neurologic outcomes in patients with ICH remains controversial. Cheng Qian et al. have reported that ICH patients with ISs had the highest mortality rate (12). Conversely, Szaflarski JP et al. have demonstrated that ISs are not independent predictors of in-hospital mortality, which is consistent with the fact that ISs are predominantly lobar ICH without intraventricular spread and are smaller, implying a better prognosis (5). In the present study, patients with ISs had a statistically worse prognosis than patients without ISs at the 90-day follow-up after ICH onset.
This was a single-center clinical study and a hospital-based analysis with inherent limitations when interpreting the findings. Firstly, there were relatively few patients with ISs in the current research. Furthermore, PaCO2 levels of CSF had not been collected, and PaCO2 levels in arterial blood cannot directly reflect the actual level in the brain tissue. A single measurement may not fully reflect accurate PaCO2 levels over time. Continuous dynamic PaCO2 monitoring was the evident approach for improving accuracy. Thirdly, the sample sizes decreased, which may influence IS prediction accuracy for ISs due to the stringent inclusion/exclusion criteria. Fourthly, PaCO2 levels are within the normal range in some patients with ICH with ISs, so the result is not straightforward to interpret. More insight into detailed mechanisms awaits further study. Finally, the diagnosis of seizures was made clinically, and information on electroencephalography was not obtained.
Conclusions
The lower initial PaCO2 levels may be a crucial biomarker in predicting ISs in patients following ICH. The PaCO2 level is readily available as an indicator of blood gas analysis at admission and may help identify high-risk patients with ISs. However, further large-scale or randomized studies are needed to verify these findings and determine whether changes in PaCO2 levels over time are associated with the onset of ISs.
Data Availability Statement
The original contributions presented in the study are included in the article/supplementary material, further inquiries can be directed to the corresponding author/s.
Ethics Statement
The studies involving human participants were reviewed and approved by the Research Ethics Committee of Dehua County Hospital. The patients/participants provided their written informed consent to participate in this study.
Author Contributions
ZP and YZ designed the study. ZP, QZ, and YZ drafted the manuscript. ZP, CW, XC, and XL collected and analyzed data. YZ helped in the statistical analysis and result interpretation. XZ and YZ were identified as the guarantor of the article, taking responsibility for the integrity of the work as a whole, and prepared the figures and interpreted the results. JW, XZ, and YZ supervised the study and revised manuscript. All authors read and approved the final manuscript.
Funding
This study was supported by the Science and Technology Planning Project of Quanzhou City (No. 2019NO11S to ZP) and Fujian University of Traditional Chinese Medicine School Management Project Clinical Special Funding (No. XB2021113 to CW).
Conflict of Interest
The authors declare that the research was conducted in the absence of any commercial or financial relationships that could be construed as a potential conflict of interest.
Publisher's Note
All claims expressed in this article are solely those of the authors and do not necessarily represent those of their affiliated organizations, or those of the publisher, the editors and the reviewers. Any product that may be evaluated in this article, or claim that may be made by its manufacturer, is not guaranteed or endorsed by the publisher.
Acknowledgments
The authors thank Liangxin Chen for assisting in parts of the data collection.
References
1. Biffi A, Rattani A, Anderson CD, Ayres AM, Gurol EM, Greenberg SM, et al. Delayed seizures after intracerebral haemorrhage. Brain. (2016) 139:2694–705. doi: 10.1093/brain/aww199
2. Cordonnier C, Demchuk A, Ziai W, Anderson CS. Intracerebral haemorrhage: current approaches to acute management. Lancet. (2018) 392:1257–68. doi: 10.1016/S0140-6736(18)31878-6
3. Hu X, Fang Y, Li H, Liu W, Lin S, Fu M, et al. Protocol for seizure prophylaxis following intracerebral hemorrhage study (SPICH): a randomized, double-blind, placebo-controlled trial of short-term sodium valproate prophylaxis in patients with acute spontaneous supratentorial intracerebral hemorrhage. Int J Stroke. (2014) 9:814–7. doi: 10.1111/ijs.12187
4. Mullen MT, Kasner SE, Messe SR. Seizures do not increase in-hospital mortality after intracerebral hemorrhage in the nationwide inpatient sample. Neurocrit Care. (2013) 19:19–24. doi: 10.1007/s12028-012-9791-0
5. Passero S, Rocchi R. Si'mone Rossi, Ulivelli M, Vatti G: Seizures after spontaneous supratentorial intracerebral hemorrhage. Epilepsia. (2002) 43:1175–80. doi: 10.1046/j.1528-1157.2002.00302.x
6. Beghi E. The epidemiology of epilepsy. Neuroepidemiology. (2020) 54:185–91. doi: 10.1159/000503831
7. Graham NS, Crichton S, Koutroumanidis M, Wolfe CD, Rudd AG. Incidence and associations of poststroke epilepsy: the prospective South London Stroke Register. Stroke. (2013) 44:605–11. doi: 10.1161/STROKEAHA.111.000220
8. Sauro KM, Wiebe S, Dunkley C, Janszky J, Kumlien E, Moshe S, et al. The current state of epilepsy guidelines: a systematic review. Epilepsia. (2016) 57:13–23. doi: 10.1111/epi.13273
9. Serafini A, Gigli GL, Gregoraci G, Janes F, Cancelli I, Novello S, et al. Are early seizures predictive of epilepsy after a stroke? Results of a Population-Based Study. Neuroepidemiology. (2015) 45:50–8. doi: 10.1159/000382078
10. Hemphill JC, Greenberg SM, Anderson CS, Becker K, Bendok BR, Cushman M, et al. Guidelines for the management of spontaneous intracerebral hemorrhage: a guideline for healthcare professionals from the American Heart Association/American Stroke Association. Stroke. (2015) 46:2032–60. doi: 10.1161/STR.0000000000000069
11. Beghi E, D'Alessandro R, Beretta S, Consoli D, Crespi V, Delaj L, et al. Incidence and predictors of acute symptomatic seizures after stroke. Neurology. (2011) 77:1785–93. doi: 10.1212/WNL.0b013e3182364878
12. Qian C, Lopponen P, Tetri S, Huhtakangas J, Juvela S, Turtiainen HM, et al. Immediate, early and late seizures after primary intracerebral hemorrhage. Epilepsy Res. (2014) 108:732–9. doi: 10.1016/j.eplepsyres.2014.02.020
13. Germonpre C, Proesmans S, Bouckaert C, Stevens L, Sprengers M, Vonck K, et al. Acute symptomatic seizures following intracerebral hemorrhage in the rat collagenase model. Epilepsy Res. (2020) 164:106364. doi: 10.1016/j.eplepsyres.2020.106364
14. Angriman F, Tirupakuzhi Vijayaraghavan BK, Dragoi L, Lopez Soto C, Chapman M, Scales DC. Antiepileptic drugs to prevent seizures after spontaneous intracerebral hemorrhage. Stroke. (2019) 50:1095–9. doi: 10.1161/STROKEAHA.118.024380
15. De Herdt V, Dumont F, Hénon H, Derambure P, Vonck K, Leys D, et al. Early seizures in intracerebral hemorrhage Incidence, associated factors, and outcome. Neurology. (2011) 77:1794–800. doi: 10.1212/WNL.0b013e31823648a6
16. Salinet ASM, Minhas JS, Panerai RB, Bor-Seng-Shu E, Robinson TG. Do acute stroke patients develop hypocapnia? A systematic review and meta-analysis. J Neurol Sci. (2019) 402:30–9. doi: 10.1016/j.jns.2019.04.038
17. Claassen J, Thijssen DHJ, Panerai RB, Faraci FM. Regulation of cerebral blood flow in humans: physiology and clinical implications of autoregulation. Physiol Rev. (2021) 101:1487–559. doi: 10.1152/physrev.00022.2020
18. Totola LT, Takakura AC, Oliveira JA, Garcia-Cairasco N, Moreira TS. Impaired central respiratory chemoreflex in an experimental genetic model of epilepsy. J Physiol. (2017) 595:983–99. doi: 10.1113/JP272822
19. Shi X-Y, Hu L-Y, Liu M-J, Zou L-P. Hypercapnia-induced brain acidosis: effects and putative mechanisms on acute kainate induced seizures. Life Sci. (2017) 176:82–7. doi: 10.1016/j.lfs.2017.03.018
20. Seyal M, Bateman LM. Ictal apnea linked to contralateral spread of temporal lobe seizures: Intracranial EEG recordings in refractory temporal lobe epilepsy. Epilepsia. (2009) 50:2557–62. doi: 10.1111/j.1528-1167.2009.02245.x
21. Seyal M, Bateman LM, Albertson TE, Lin TC, Li CS. Respiratory changes with seizures in localization-related epilepsy: analysis of periictal hypercapnia and airflow patterns. Epilepsia. (2010) 51:1359–64. doi: 10.1111/j.1528-1167.2009.02518.x
22. Sirsi D, Nadiminti L, Packard MA, Engel M, Solomon GE. Apneic seizures: a sign of temporal lobe hemorrhage in full-term neonates. Pediatr Neurol. (2007) 37:366–70. doi: 10.1016/j.pediatrneurol.2007.06.004
23. Kilicaslan B, Erol I, Ozkale Y, Saygi S, Sariturk C. Association between hypocapnia and febrile seizures. J Child Neurol. (2014) 29:599–602. doi: 10.1177/0883073813513070
24. Wirrell EC Camfield PR Gordon KE Camfield CS Dooley JM Hanna Hanna BD: Will a critical level of hyperventilation-induced hypocapnia always induce an absence seizure? Epilepsia. (1996) 37:459–62. doi: 10.1111/j.1528-1157.1996.tb00592.x
25. Yiorgos Alexandros Cavayas, Munshi L, Sorbo Ld, Fan E. The early change in paco2 after extracorporeal membrane oxygenation initiation is associated with neurological complications. Am J Respir Crit Care Med. (2020) 201:1525–35. doi: 10.1164/rccm.202001-0023OC
26. Zhang C, Wang X, Wang Y, Zhang JG, Hu W, Ge M, et al. Risk factors for post-stroke seizures: a systematic review and meta-analysis. Epilepsy Res. (2014) 108:1806–16. doi: 10.1016/j.eplepsyres.2014.09.030
27. Hextrum S, Minhas JS, Liotta EM, Sorond FA, Naidech AM, Maas MB. Hypocapnia, ischemic lesions, and outcomes after intracerebral hemorrhage. J Neurol Sci. (2020) 418:117139. doi: 10.1016/j.jns.2020.117139
28. Szaflarski JP, Rackley AY, Kleindorfer DO, Khoury J, Woo D, Miller R, et al. Incidence of seizures in the acute phase of stroke: a population-based study. Epilepsia. (2008) 49:974–81. doi: 10.1111/j.1528-1167.2007.01513.x
29. Biffi A, Anderson CD, Jagiella JM, Schmidt H, Kissela B, Hansen BM, et al. APOE genotype and extent of bleeding and outcome in lobar intracerebral haemorrhage: a genetic association study. Lancet Neurol. (2011) 10:702–9. doi: 10.1016/S1474-4422(11)70148-X
30. Zhang YB, Zheng SF, Yao PS, Chen GR Li GH, Li SC, Zheng YF, et al. Lower ionized calcium predicts hematoma expansion and poor outcome in patients with hypertensive intracerebral hemorrhage. World Neurosurg. (2018) 118:e500–4. doi: 10.1016/j.wneu.2018.06.223
31. Demchuk AM, Dowlatshahi D, Rodriguez-Luna D, Molina CA, Blas YS, Dzialowski I, et al. Prediction of haematoma growth and outcome in patients with intracerebral haemorrhage using the CT-angiography spot sign (PREDICT): a prospective observational study. Lancet Neurol. (2012) 11:307–14. doi: 10.1016/S1474-4422(12)70038-8
32. Morotti A, Brouwers HB, Romero JM, Jessel MJ, Vashkevich A, Schwab K, et al. Intensive blood pressure reduction and spot sign in intracerebral hemorrhage: a secondary analysis of a randomized clinical trial. JAMA Neurol. (2017) 74:950–60. doi: 10.1001/jamaneurol.2017.1014
33. O'brien RM. A caution regarding rules of thumb for variance inflation factors. Qual Quang. (2007) 41:673–90. doi: 10.1007/s11135-006-9018-6
34. Dibue M, Spoor JKH, Dremmen M, von Sass CF, Hanggi D, Steiger HJ, et al. Sudden death in epilepsy: there is room for intracranial pressure. Brain Behav. (2020) 10:e01838. doi: 10.1002/brb3.1838
35. McGuigan PJ, Shankar-Hari M, Harrison DA, Laffey JG, McAuley DF. The interaction between arterial oxygenation and carbon dioxide and hospital mortality following out of hospital cardiac arrest: a cohort study. Crit Care. (2020) 24:336. doi: 10.1186/s13054-020-03039-6
36. Zohdi H, Scholkmann F, Wolf U. Frontal cerebral oxygenation asymmetry: intersubject variability and dependence on systemic physiology, season, and time of day. Neurophotonics. (2020) 7:025006. doi: 10.1117/1.NPh.7.2.025006
37. Meng L, Gelb A. Regulation of cerebral autoregulation by carbon dioxide. Anesthesiology. (2015) 122:196–205. doi: 10.1097/ALN.0000000000000506
39. Suzuki H, Shiba M, Nakatsuka Y, Nakano F, Nishikawa H. Higher cerebrospinal fluid ph may contribute to the development of delayed cerebral ischemia after aneurysmal subarachnoid hemorrhage. Transl Stroke Res. (2017) 8:165–73. doi: 10.1007/s12975-016-0500-8
40. Dulla CG, Dobelis P, Pearson T, Frenguelli BG, Staley KJ, Masino SA. Adenosine and ATP link PCO2 to cortical excitability via pH. Neuron. (2005) 48:1011–23. doi: 10.1016/j.neuron.2005.11.009
41. Balestrino M, Somjen GG. Concentration of carbon dioxide, interstitial pH and synaptic transmission in hippocampal formation of the rat. J Physol. (1988) 396:247–66. doi: 10.1113/jphysiol.1988.sp016961
42. Guaranha MS, Garzon E, Buchpiguel CA, Tazima S, Yacubian EM, Sakamoto AC. Hyperventilation revisited: physiological effects and efficacy on focal seizure activation in the era of video-EEG monitoring. Epilepsia. (2005) 46:69–75. doi: 10.1111/j.0013-9580.2005.11104.x
43. Liu Y, Wang T, Liu X, Wen Y, Xu T, Yu X, et al. Overexpression of zinc-alpha2-glycoprotein suppressed seizures and seizure-related neuroflammation in pentylenetetrazol-kindled rats. J Neuroinflammation. (2018) 15:92. doi: 10.1186/s12974-018-1132-6
44. Curley G, Kavanagh BP, Laffey JG. Hypocapnia and the injured brain: more harm than benefit. Crit Care Med. (2010) 38:1348–59. doi: 10.1097/CCM.0b013e3181d8cf2b
Keywords: intracerebral hemorrhage, carbon dioxide, epilepsy, risk factor, stroke
Citation: Pan Z, Zhong Q, Wang C, Wang J, Chen X, Li X, Zhang X and Zhang Y (2022) Association Between Partial Pressure of Carbon Dioxide and Immediate Seizures in Patients With Primary Intracerebral Hemorrhage: A Propensity-Matched Analysis. Front. Neurol. 13:865207. doi: 10.3389/fneur.2022.865207
Received: 29 January 2022; Accepted: 08 March 2022;
Published: 21 April 2022.
Edited by:
Zhibin Chen, Monash University, AustraliaReviewed by:
Archana Hinduja, The Ohio State University, United StatesNanya Hao, Sichuan University, China
John-Paul Nicolo, Royal Melbourne Hospital, Australia
Copyright © 2022 Pan, Zhong, Wang, Wang, Chen, Li, Zhang and Zhang. This is an open-access article distributed under the terms of the Creative Commons Attribution License (CC BY). The use, distribution or reproduction in other forums is permitted, provided the original author(s) and the copyright owner(s) are credited and that the original publication in this journal is cited, in accordance with accepted academic practice. No use, distribution or reproduction is permitted which does not comply with these terms.
*Correspondence: Xintong Zhang, 67185761@qq.com; Yibin Zhang, zyb2008qz@163.com
†These authors have contributed equally to this work