- 1Vanderbilt Sports Concussion Center, Department of Neurological Surgery, Vanderbilt University Medical Center, Nashville, TN, United States
- 2Department of Medical Statistics, London School of Hygiene and Tropical Medicine, London, United Kingdom
- 3School of Public Health, Physiotherapy and Sports Science, University College Dublin, Dublin, Ireland
- 4Grossman School of Medicine, New York University, New York, NY, United States
- 5Priority Research Center for Stroke and Brain Injury, School of Medicine and Public Health, University of Newcastle, Callaghan, NSW, Australia
- 6Department of Physical Medicine and Rehabilitation, Harvard Medical School, Boston, MA, United States
- 7Department of Physical Medicine and Rehabilitation, Spaulding Rehabilitation Hospital, Charlestown, MA, United States
- 8MassGeneral Hospital for Children Sports Concussion Program, Boston, MA, United States
- 9Department of Physical Medicine and Rehabilitation, Spaulding Research Institute, Charlestown, MA, United States
Background: Individuals with migraine disorders may be affected differently by concussions compared to individuals without migraine disorders. Prior studies on this topic have had mixed results. The purpose of this study was to systematically examine clinical outcomes following a sport-related concussion in athletes who have a pre-injury history of migraines.
Methods: All studies published prior to 15 May 2021 that examined pre-injury migraines as a possible predictor of clinical recovery from concussion were included. The search included (i) sport/athlete-related terms, (ii) concussion-related terms, and (iii) diverse predictor/modifier terms. After removing duplicates, 5,118 abstracts were screened, 538 full-text articles were reviewed, and 27 articles were included for narrative synthesis without meta-analysis (n = 25 with unique samples). Risk of bias was assessed using the domain-based Quality In Prognosis Studies (QUIPS) tool.
Results: Most studies did not find pre-injury migraines to be associated with concussion outcome, but several of these studies had small or very small sample sizes, as well as other methodological weaknesses. Risk of bias varied greatly across studies. Some of the larger, better-designed studies suggested pre-injury migraines may be a risk factor for worse concussion outcome. Most articles examined pre-injury migraines as an exploratory/secondary predictor of concussion outcome; very few were designed to examine migraine as the primary focus of the study. Migraine history was predominantly based on self-report and studies included minimal information about migraine (e.g., age of onset, frequency/severity, past treatment). Effect sizes were usually not reported or able to be calculated from reported study data.
Conclusion: There is some evidence to suggest that pre-injury migraines may be a vulnerability factor for a worse outcome following concussion, with studies having the lowest risk of bias reporting a positive association. Future studies should focus on improving methodological quality when assessing the relationship between pre-injury migraines and concussion outcome and better characterizing pre-injury migraine status.
Systematic Review Registration: https://www.crd.york.ac.uk/prospero/display_record.php?ID=CRD42019128292, identifier: PROSPERO 2019 CRD42019128292.
Introduction
There is considerable interest in better understanding factors that may influence recovery following sport-related concussion, including pre-existing health conditions, injury-related factors, and acute symptomatology (1–3). A pre-injury history of migraine headaches is one such factor. Migraine headaches, which are commonly characterized by a unilateral throbbing sensation accompanied with light/noise sensitivity, nausea, and vomiting (4) occur in approximately 8% of youth (5) and 14% of adults (6). Middle school student athletes with migraines have a higher prevalence of prior concussion compared to those without migraines (31% vs. 13%) (7), suggesting that youth with migraines may be at a greater risk for sustaining concussion. This finding is important because individuals who have sustained prior concussions are at a higher risk of sustaining a future concussion (8) and having multiple concussions is associated with greater baseline symptom reporting (9) and a subset may be at increased risk for experiencing persistent symptoms following a subsequent concussion (10). Furthermore, athletes with pre-existing migraine disorders, regardless of prior concussion history, report more symptoms at baseline in the absence of a recent concussion (9). They also are more likely to endorse symptom provocation on vestibular/ocular-motor screening tests, in the absence of a concussion, during preseason baseline testing (11). Moreover, they report more symptoms acutely following a concussion than athletes without migraine histories (12). These findings indicate that individuals with migraine disorders may be affected differently by concussions compared to individuals without migraine disorders.
A 2017 systematic review examining a variety of factors that may be associated with clinical outcome following a concussion (1) identified 10 studies published prior to June 2016 that analyzed whether having pre-injury migraines was a risk factor for protracted recovery following concussion (10, 13–21). Pre-existing migraine history was not a primary risk factor of interest in nearly all of these studies. Only one study (10) showed that migraine history is associated with worse clinical outcome; a greater proportion of youth with pre-injury migraines (43%) experienced persistent post-concussive symptoms at 28 days post-injury compared to those without pre-injury migraines (28%). However, significant variability in the methodology of these studies may have influenced the conclusions of the prior systematic review (1). Moreover, the prior systematic review focused broadly on any and all potential risk factors for worse outcome and did not examine any individual risk factor in depth. Additionally, many studies examining risk factors for a worse clinical recovery have been published since 2017, necessitating an updated review. Thus, the purpose of the present systematic review is to provide a thorough and targeted examination of whether pre-injury migraines may be a vulnerability factor for a protracted recovery following concussion, characterize potential gaps in the literature, and propose ways to advance our understanding of the association between pre-injury migraine and clinical outcome following sport-related concussion. In addition to the aforementioned goals, this systematic review expands on the prior review because it includes additional studies published since June 2016 and evaluates the risk of bias for the included studies.
Materials and Methods
The original systematic review (1) protocol was prospectively registered in the PROSPERO database for systematic reviews (protocol ID: CRD42016041479). The updated systematic review protocol was also registered (protocol ID: CRD42019128292). This systematic review is consistent with the guidelines from the Preferred Reporting Items for Systematic Reviews and Meta-Analyses (PRISMA) Statement (22).
Search Strategy
Articles were identified by online database searching, by hand-searching reference lists of identified studies, and by performing cited reference searches (see Figure 1). The online databases that were searched included PubMed, MEDLINE®, PsycINFO®, CINAHL, Cochrane Library, EMBASE, SPORTDiscus, Scopus, and Web of Science. A total of three separate searches were conducted, each covering English-language articles published from (i) database inception to 1 June 2016; (ii) 1 January 2016 to 1 February 2019; and (iii) 1 February 2019 to 15 May 2021. The searches were otherwise identical. The key search terms consisted of two main themes, (i) sport and athlete-related terms and (ii) brain concussion related terms, as follows: sport, sports [MeSH]), athletic, athlete, player and craniocerebral trauma, brain injuries, brain concussion, sports concussion, athletic injuries, mild traumatic brain injury, mTBI, traumatic brain injury, TBI, brain concussion, concussion, multiple concussions, repeated concussion, repetitive concussion, cumulative concussions, concussion history, brain damage, prognosis, outcome, recovery, risk factor, injury incidence, sex differences, gender, genetics, ApoE, BDNF, S100B, GFAP, severity, loss of consciousness, LOC, post-traumatic amnesia, PTA, amnesia, retrograde amnesia, seizure, seizures, learning disorder, ADHD, level of education, migraine, mental health, sleep disorders, medications, cervical injury, vestibular injury, psychological reactions, anxiety, depression, headaches, intractable headaches, magnetic resonance imaging, MRI, computed tomography, and CT. This search strategy was deliberately broad in order to replicate the methods employed in the original systematic review (1), and to ensure that relevant articles that did not explicitly mention “migraine” in the title/abstract were not accidentally excluded. In the original systematic review, we found that several articles examined multiple predictors, many of which were not discussed in the abstract and were, instead, discussed elsewhere in the manuscript, such as in the Results section or in tables or figures.
Article Selection and Data Extraction
When the search was repeated for the purpose of this systematic review (1), we discovered an error such that 7,617 records were reported when there were in fact 4,746 records in the original search, as reflected in Figure 1 of this paper. For the original and third searches, each title and abstract were examined for inclusion by two authors (GI and AG). For the second search, titles and abstracts were reviewed by one author (GI). Articles were identified for possible inclusion if they (i) assessed humans, and (ii) examined if pre-injury migraine was associated with outcome following sport-related concussion (in any capacity—that is, as a primary prognostic, confounding, mediating, or effect modifying variable). For all studies identified during the initial title/abstract screening, the full-text article was downloaded and reviewed by two authors to determine whether it met criteria for inclusion (original search: AG and GI; second search: DT and AG; third search: DT and NC). Reference lists of previously published reviews on predictors of concussion recovery were also examined for articles that may fit the inclusion criteria.
Outcome from sport-related concussion was defined in a variety of ways based on the methodology of the studies included in the review (e.g., days until symptom-resolution/return to baseline, days to return to school or sports, cognitive recovery/return to baseline). Studies that examined acute symptomatology/outcome in the first several days following a concussion but did not address clinical recovery or persistent symptoms were excluded. Four authors (NC, AG, DT, and NH) completed the extraction of information from the articles (i.e., first author, year of publication, the PubMed identification number, number of total participants, number of concussed participants, proportion of female participants, age, setting, study design, definition of outcome, the period for assessing the clinical outcome, findings regarding the association between migraine and outcome, number of participants with pre-injury migraines, method/definition for assessing pre-injury migraines, number of migraines in the past year before the index concussion, age of onset of migraines, whether migraines were differentiated from other headaches types, findings/effect size, and family history of migraine).
Risk of Bias and Level of Evidence
Risk of bias was rated independently for each article by two authors (DT and FB) using the Quality In Prognosis Studies (QUIPS) tool (23). QUIPS is a domain-based tool that evaluates risk of bias in prognostic studies, which can influence the direction and magnitude of study results. The authors developed a rating rubric before evaluating the studies. Discrepancies were resolved through discussion. The level of evidence for each article was rated independently by two authors (DT and AG) using the Oxford Classification for Evidence-Based Medicine (24).
Qualitative Synthesis and Likelihood Ratio Estimation
Heterogeneous subpopulations, comparators, outcomes, recovery timeframes, and operational definitions of pre-injury migraine used across studies precluded quantitative aggregation in the form of meta-analysis. Therefore, we performed a synthesis without meta-analysis by descriptively synthesizing included studies and systematically reporting study findings by study design, participant subpopulation (e.g., age range), pre-injury migraine definition, recovery timeframe, and clinical outcome (25, 26).
In addition, we used a likelihood heuristic to assess the probability of observing a specific number of statistically significant and non-significant studies reporting an association between migraine history and clinical outcome. This heuristic yields a likelihood ratio (LR) that estimates the weight of the evidence in support of the null hypothesis (i.e., migraine history is not associated with clinical outcome following concussion) vs. the alternative hypothesis (i.e., migraine history is associated with worse clinical outcome) (27). The likelihood heuristic has been used to examine associations between sport-related concussion and clinical outcomes in prior systematic reviews (3, 28). To calculate LRs, an a priori alpha (e.g., 0.05) and statistical power value (e.g., 0.80) are selected. Next, the number of studies (k) that reported a statistically significant result for various outcomes (e.g., symptom duration or time to return to sports) are summed and entered as a proportion of the overall number of studies reporting on a specific outcome (n). We used an LR calculator (https://lakens.shinyapps.io/likelihood) that has been developed to assist in synthesizing results from multiple studies (27). Two sets of analyses, with varying parameters, were conducted. First, an alpha level of 0.05 and power level of 0.80 were selected, reflecting near optimal study designs. Second, an alpha level of 0.25 and power level of 0.45 were selected, which is more likely to capture shortcomings inherent to several of the study designs included in this systematic review. The LR effect size estimates were summarized as follows: LRs > 8 and 32 are considered benchmarks of moderate and strong evidence, respectively (27, 29).
Results
As shown in Figure 1, the search revealed 7,946 articles. There were 5,118 unique articles after removing duplicates. Based on a review of the titles and abstracts of these articles, 4,580 were excluded because they did not examine sport-related concussion or were not an empirical research study [e.g., review articles, editorials, chapters, commentaries, letters to the editor). This narrowed the pool of articles to 538, which were downloaded and reviewed in full. A total of 27 articles met inclusion criteria. Detailed information about these studies is included in Tables 1, 2. Three of these articles were from the same research group and included an identical sample with similar analyses and findings (15, 30, 31). For summary purposes, these three studies were grouped and discussed as one study. For the 25 studies with unique samples, there were 8,692 athletes who sustained a sport-related concussion. At least 1,015 of these athletes reported a pre-injury history of migraines (11.8%; n = 1,015/8,582); one study did not report the number of individuals with pre-injury migraines (32)]. There was substantial methodological variability across studies (see Tables 1, 2). Study designs included prospective cohort studies (12/25; 48%), retrospective cohort studies (10/25, 44%), case-control studies (2/25; 8%), and a case series (1/25; 4%). Outcomes also varied. Many studies defined recovery based on symptom resolution (11/25; 44%; including studies that deemed persistent symptoms as “post-concussion syndrome”), while other studies defined outcome as return to play or school (7/25; 28%), clinical recovery (e.g., resolution of symptoms and cognitive and/or balance difficulties, 3/25; 12%), presence of a new psychiatric diagnosis (1/25; 4%), vestibular symptom changes (1/25; 4%), and self-reported perceived recovery time (2/25; 8%). Some studies reported two or more outcomes. Statistically, some studies examined recovery time as a continuous variable, while other studies created a dichotomous variable based on recovery at various timepoints (i.e., 10 days, 14 days, 21 days, 28 days, 60 days, 3 months).
Level of Evidence and Risk for Bias Assessment
Level of evidence ratings and results of the QUIPS are summarized in Table 3. The level of evidence for most studies was rated as 3 (i.e., 22/25 studies), and three studies were rated a 4 making the average level of evidence 3.1. Regarding risk of bias, most studies (21/25; 84%) were rated as having a moderate overall risk of bias. Two studies (8%) had a low overall risk of bias and two studies (8%) had a high overall risk of bias. Seven studies (28%) were rated as having low risk of selection bias and 17 studies (68%) were at moderate risk of selection bias due to inadequate methods to identify the target population and unclear or inadequate participation by eligible individuals. Almost all studies (92%) were at moderate risk of prognostic factor misclassification predominantly due to an unclear definition and method of assessing the prognostic factor (i.e., pre-injury migraine). Nine studies (36%) were at low risk of bias due to outcome measurement and 15 studies (60%) were at moderate risk of bias due to outcome measurement that was attributable to uncertainty about the reliability of the outcome measurement method (e.g., participants had to recall the last date on which they experienced post-concussion symptoms). Almost all studies (21/25; 84%) were at moderate risk of bias regarding the potential presence of confounding variables because such variables were not incorporated in analyses involving pre-injury migraines and outcome (e.g., biological sex, concussion history). Almost half of the studies (10/25; 40%) had low risk of outcome and analytical reporting bias while most other studies (14/25; 56%) had a moderate risk of bias due to potential incomplete reporting of study outcome measures and statistical analyses.
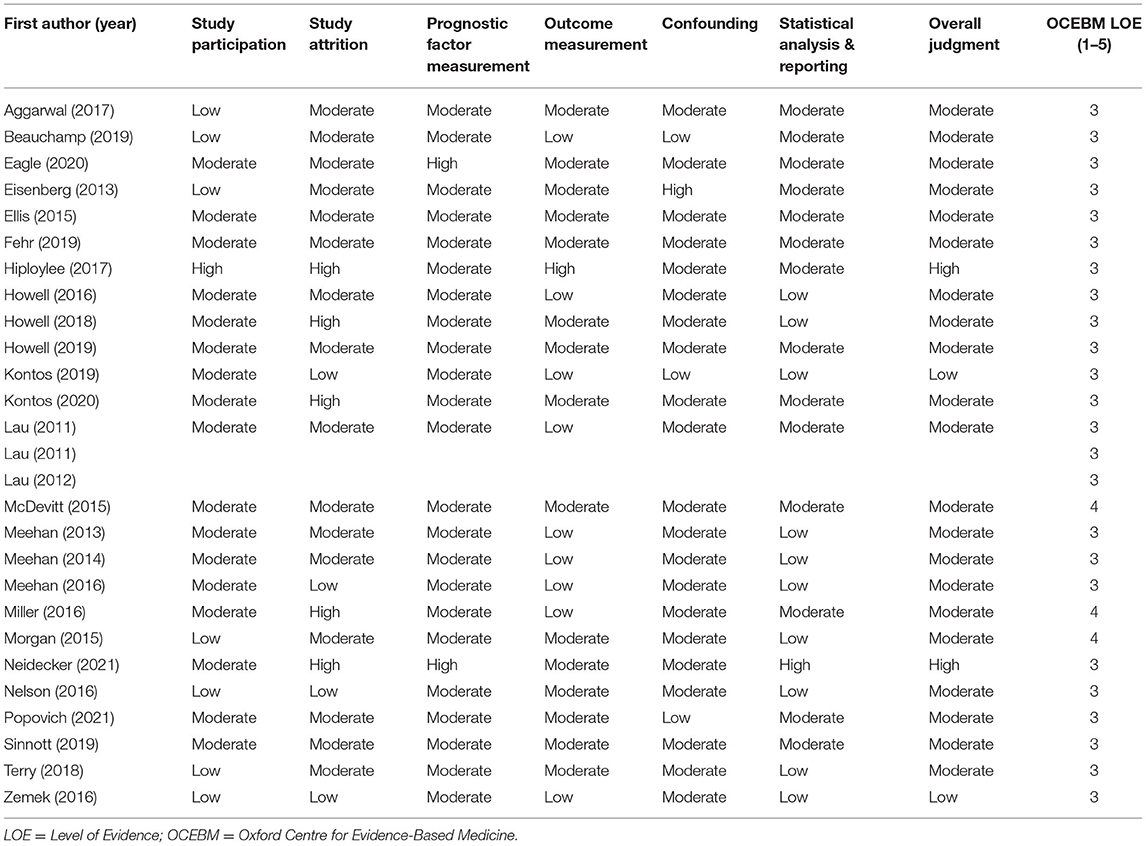
Table 3. Risk of bias, as rated by the Quality in Prognostic Studies (QUIPS), and oxford centre for evidence-based medicine level of evidence.
Pre-injury Migraines and Clinical Outcome: Overall Findings
Of the 25 studies, most (k = 19, 76%) did not find that pre-injury migraines were a risk factor for a worse clinical outcome following a sport-related concussion, two studies (8%) had both negative and positive findings, and four studies (16%) had positive findings; of note, one study did not conduct statistical analyses, (33) but based on the raw data reported in the paper, we conducted chi-squared tests and the p-values were >0.05; this study was included as one of the 19 studies with null results. Across all studies, 24% (k = 6/25) reported at least one result (e.g., symptom duration, time to return to play) showing an association between pre-injury migraine history and worse clinical outcome. There was a large amount of variability in how each study defined the outcome.
Likelihood ratios favored the null hypothesis with strong magnitude when you assume ideal study parameters and weak magnitude when you assume more realistic study parameters (see Table 4). When analyses were restricted to studies that included 20 or more and 50 or more individuals with a pre-injury history of migraine, 35.7% (k = 5/14) and 42.3% of studies (k = 3/7) had at least one positive finding, respectively. For studies that included over 50 people with pre-injury migraines, the likelihood ratios supported the alternative hypothesis (i.e., that there was a positive association between pre-injury migraine history and worse clinical outcome) with weak-to-moderate magnitude.
Narrative Review of Studies
In prospective cohort studies examining high school athletes, similar rates of athletes with and without pre-injury migraines had returned to play at 14 days (30) and at 28 days post injury (19, 20). Similarly, a prospective cohort study that examined symptom recovery time in high school and college athletes did not find an association between pre-injury history of migraines and time to recover (21). In specialty sport concussion clinic studies, pre-injury migraines were not associated with a longer recovery [i.e., >28 days (14, 34, 35); self-reported symptom recovery (36–39)] with the median recovery time for the entire sample being between 23 and 40 days across three studies (37–39). Pre-injury migraines were, however, associated with prolonged vestibular-ocular difficulties at 11–21 days following concussion (40). Another study in a specialty sport concussion clinic showed that amongst younger children (i.e., ages 8–12), those with migraines had a higher likelihood of having symptoms at 28 days following injury (24%) compared to those without migraines (4%; p = 0.025); however, this finding was not apparent in youth ages 13–18 (8% vs. 10% prolonged recovery, p = 0.81) (41). A different adolescent concussion clinic study reported that those with a “history of headaches or migraines” (36% of the entire sample) showed a higher likelihood of prolonged recovery at 30 days (OR = 4.02) (42). Adolescents with concussion seen in university settings did not have a higher likelihood of prolonged recovery (43) or greater time to return to play (44). In a study that examined adolescent and young adult athletes recruited from a hospital-based concussion program, those with a pre-injury history of migraines were not more likely to have a prolonged recovery (i.e., return to play > 60 days; n = 2/10, 20%) compared to those who did not have a pre-injury history of migraines (n = 18/77, 23.4%; p = 0.83) (18).
In a prospective surveillance study that followed players in high school and collegiate athletics, those with migraines took a median of 6 days to return to academics without accommodations and 15.5 days to return to athletics fully, compared to 5 days (for academics) and 14 days (for athletics) in those without migraines (45). Those with and without pre-injury migraines did not differ on their time to return to academics or athletics (45) when recovery time was treated as a continuous variable. However, slightly lower percentages of athletes with migraine histories returned to academics after 7 days (57% vs. 68%, p =0.02), 14 days (75% vs. 88%, p < 0.001), and 21 days post injury (89% vs. 94%, p = 0.03) (45). When the authors stratified this analysis by gender, this effect was significant in girls and women with pre-injury migraines, but not boys and men (45).
In a retrospective chart review that examined whether high school athletes experienced prolonged recovery [post-concussion syndrome (PCS) diagnosis] at 3 months post injury, the development of PCS was not predicted by a history of migraines (p = 0.09) (13). However, pre-injury migraine was overrepresented in the PCS group, as one-fifth (20%) of the PCS group had a history of migraines, while 8.8% of the non-PCS patients had a history of migraines (13).
In one study of adolescents and young adults recruited from an emergency room setting (64% sports injuries), the median recovery time for those with a pre-injury history of migraines was 13 days, which matched the median recovery time of the entire sample (16). Additionally, a similar proportion of those with migraines had symptoms at 28 days post injury (35%) compared to the entire sample (32%; p = 0.72) (16). A different prospective study that examined sports concussions in patients ages 12–17 who presented to an emergency department did not show a higher likelihood of prolonged recovery (46). In contrast, another study examined youth ages 5–18 who presented to emergency departments across Canada and found a higher portion of the migraine group (42.6%) had symptoms at 28 days post injury, compared to 28.1% of the group without migraines (10). The association between pre-injury migraine and persistent symptoms was significant in both univariate and multivariate models.
Those with pre-injury migraines did not have a higher likelihood of developing a psychiatric diagnosis following a concussion compared to those without pre-injury migraines (17). One study examined patients who all had persistent symptoms for at least 3 months following their injury. Patients were sent a questionnaire regarding their recovery by mail months to years following their injury. Some patients reported they never recovered. There was no difference in the proportion of patients with pre-injury migraines in the recovered vs. the not recovered groups (p = 0.94) (32).
Family History of Migraines and Clinical Outcome
Three studies assessed the association between a family history of migraines and recovery from concussion. One study examining high school athletes showed that the development of PCS at 3-months following injury was associated with a family history of migraine (p = 0.003), and that 35% of the PCS group had a family history of migraines compared to 11.3% of the control group (though, as noted above, in this same study the youth's own pre-injury migraine status was not associated with PCS) (13). The other two studies, both set in emergency departments, did not find an association between family history of migraines and time to recovery (p = 0.62) (16) or post-concussion symptoms at 28 days post injury (p = 0.08) (10). One study assessed if patients had a “personal or family history of migraine,” and did not find that migraines were predictive of time to symptom resolution (43).
Sample Size
One study did not report the number of participants with migraines (32) The number of participants with migraines in each study ranged from 4 to 204, with an average of 42.3 participants with migraines (total = 1,015; median = 29; based on the 24 studies reporting their sample size). Ten of the 24 studies (41.7%) included fewer than 20 participants who had pre-injury migraines. The two studies with the largest sample sizes of people with pre-injury migraines [n = 117 (45) and n = 204 (10)] were two of the six studies that showed positive findings between pre-injury migraines and worse clinical outcome following concussion.
Limited Clinical Information Relating to Pre-injury Migraines
All studies lacked basic clinical information related to pre-injury migraines, limiting the ability to understand how pre-injury migraines may be related to a worse outcome from a sport-related concussion. In 17 studies (68%), pre-injury history of migraines was assessed based on self- or parent-report, likely with a single item. It was not specified whether a definition of a migraine was provided to these athletes when they completed this question. Additionally, the specific wording related to this question was not clearly specified for all studies and thus likely differed across studies (e.g., “Has a physician treated you for migraines?,” “Do you have migraines?,” “Have you been diagnosed with a migraine disorder?”). Five studies (20%) used retrospective chart reviews, which may indicate that a diagnosis of migraine was rendered by a physician, but this cannot be determined from the information provided in the studies. Two studies (8%) based pre-injury migraine status on a clinical examination [one null (21), one significant (40)], and only three studies (12%) specified the migraines needed to be physician-diagnosed [two null (35, 36), one significant (10)]. No studies reported the age of onset for the migraines, current frequency/severity, or other important clinical features.
Discussion
This systematic review identified 27 studies that examined the association between pre-injury migraines and outcome following concussion, of which 25 comprised unique samples. Across these studies, an average of 11.8% of athletes reported a pre-injury history of migraine. It is essential to appreciate that only one study was designed specifically to examine migraine as a risk factor (45). All other studies included migraine as a secondary or exploratory variable; some studies discussed their rationale for examining pre-injury migraines (10, 20, 34–37, 41–43, 46), while others did not (13, 14, 16–19, 21, 30, 32, 33, 38–40, 44). Most studies (n = 19/25, 76%) did not find a statistically significant association between having pre-injury migraines and a prolonged recovery or worse clinical outcome following concussion, two studies had both significant and null findings, and four studies showed only significant findings.
A few studies found an association between pre-injury migraine and clinical outcome from concussion. The largest study examined in this systematic review, conducted in emergency departments across Canada, was also one of the two studies with an overall risk of bias judgment of “low” using the QUIPS tool (10). In that study, 42.6% of youth with a history of pre-injury physician-diagnosed migraines had persistent symptoms 28 days following their concussion, compared to 28.1% of youth without pre-injury migraines (OR = 1.9, p < 0.001) (10). Moreover, in a multivariable model that also included demographic factors (e.g., age, sex), history of prior concussions with symptoms that lasted more than 1 week, and acute post-injury symptoms (e.g., poor balance; headache, noise sensitivity, fatigue, answering questions slowly), pre-injury migraine history was independently associated with the presence of symptoms 4 weeks following injury (OR = 1.7, p < 0.001) (10). In the only other study with an overall “low” risk of bias, which examined athletes ages 12–23, the authors reported that patients with a pre-injury history of migraines (n = 41) had a longer recovery time (M = 5.5 weeks, SD = 4.2), taking, on average, about a week and a half longer to recover compared to those without pre-injury migraines (M = 4.0 weeks, SD = 2.7) (37). This finding was present in a univariable analysis as well as a multivariable model that also included initial symptom burden, posttraumatic migraine symptoms, biological sex, and age (i.e., dichotomized as <8 years old) (43). The two other studies that found pre-injury migraines as significantly associated with outcome had important methodological limitations, like combining headache and migraine history (36% of the sample) (42), which limits the generalizability of their findings, or examining a very small sample of people with pre-injury migraines (i.e., n = 8 total, with n = 5 in the statistical analysis) using a non-traditional definition of recovery (i.e., vestibular deficits at 11–21 days post-injury) (40).
Two studies had mixed findings. An observational study of high school and collegiate athletes examined full return to academics without accommodations and athletics (45) This study had the second largest migraine sample and was the only study with a primary aim to examine pre-injury migraines as a risk factor for prolonged recovery; most other studies included a variety of other potential prognostic factors and analyzed pre-injury migraine status in an exploratory or secondary manner. In this study (45), there were no statistically significant differences in the overall recovery times between athletes with and without pre-injury migraines. However, a lower proportion of those with pre-injury migraines fully returned to school at 7, 14, and 21 days post injury (45). This finding extends a prior study that showed high school athletes with pre-injury migraines appear to have greater symptoms in the first 3 days following concussion compared to non-migraineurs (12). Taken together, these studies suggest that athletes with pre-injury migraines have greater acute symptomatology following concussion, which may influence proximal outcomes (e.g., return to school) more than distal outcomes (e.g., return to play). Previous studies have consistently shown that greater acute symptom burden (45) and the presence of acute headaches/migraines (45, 47) are associated with a prolonged recovery. The final study with positive findings (41) found that 71% (n = 5/7) of youth athletes with pre-injury migraines (ages 8–12) had symptoms >28 days compared to only 26% of youth athletes without migraines (n = 16/61), though this analysis was based a very small sample of migraineurs and the finding did not replicate in the age 13–18 group (41).
The majority of studies did not report statistically significant differences in concussion outcome based on pre-injury migraine status, regardless of how it was defined. However, one of the most notable things about this body of literature is the variability in methodological rigor and sample size. Many studies included fewer than 20 patients with pre-injury migraines (13, 14, 17, 18, 21, 30, 34, 40, 43) [in some analyses (41)], and several had <10 patients with a migraine condition (21, 34, 40, 43) [in some analyses (14, 41)]. These studies likely lacked the statistical power to detect differences between groups and are unlikely to embody a representative or generalizable subsample of individuals with pre-injury migraine. Further, these studies had variable methods, such that there were a variety of outcomes examined (e.g., symptom resolution, return to play, return to academics, PCS diagnosis, development of a psychiatric disorder) at a variety of different time points. Empirical ratings of risk of bias in these studies were mostly classified as having a “moderate” risk of bias and only one study was classified as having a “low” risk of bias. Therefore, it is reasonable to interpret these null findings with caution, and that these methodological issues limit our current ability to render a conclusion about the relationship between pre-injury migraines and concussion outcome with certainty.
Potential Mechanisms
The mechanism by which some athletes with pre-injury migraines might have a longer or more complicated recovery from a concussion is not fully understood, but there are several noteworthy findings that suggest pre-injury migraines may be a risk modifier for a complicated concussion recovery. In both athletes and civilians, those with pre-injury migraines are more likely to have headaches and migraines following a concussion (48, 49) and post-injury headache and migraine are independent risk factors for a prolonged recovery from concussion (10, 50–54). Additionally, primary migraine disorders and post-traumatic headaches (which often manifest as having migraine-features) have similar pathophysiological profiles, involving alterations in ion flow, cerebral metabolism, and neurotransmitters/neuropeptides (55–59). It is possible that some of the pathophysiological processes underlying primary migraine disorders are more vulnerable to trauma, leading to an amplified neurovascular response (60). This could lead to worse acute symptoms following concussion for those with pre-injury migraines (12), and worse acute symptoms is one of the most consistent and robust predictors of prolonged concussion recovery (1).
Limitations
There are several important limitations to consider when interpreting the results of this systematic review. In most studies, pre-injury migraine status was based on a single self-report item; very few studies used data based on a clinical examination by a trained medical professional or a structured clinical interview for headaches [e.g., the Headache-Attributed Restriction, Disability, Social Handicap and Impaired Participation (HARDSHIP) questionnaire and its diagnostic algorithm (61)]. Other clinical factors, such as the age of onset of the migraine disorder or pre-injury migraine frequency, were not reported in any of these studies. Thus, those athletes with pre-existing migraines are likely to be heterogeneous in their migraine frequency and medication status, which may affect the study outcome. It is also important to consider that there might be a gender-by-migraine interaction girls/women are more likely to have migraine conditions (62), report more symptoms during baseline preseason testing (9), have a higher prevalence of headaches/migraines following concussion (63), and might be at risk for prolonged recovery compared to boys/men (1). Thus, stratifying analyses in future migraine studies by gender may be useful. Further, those with pre-injury migraines, overall, report a greater severity of concussion-like symptoms and higher symptom provocation scores on vestibular/ocular-motor screens in the absence of a recent concussion during pre-season baseline testing (9, 11). It is possible that when athletes with pre-injury migraines sustain a concussion, some of their prolonged post-injury symptoms and functional difficulties may be misattributed solely to the effects of concussion, but may actually reflect a variety of factors associated with having a migraine disorder more broadly. As noted earlier, many studies analyzed a small number of people with pre-injury migraines and were likely underpowered to detect statistically significant differences between groups. Additionally, due to the variability across studies and their outcomes, quantitative analyses or meta-analytic synthesis of effect sizes could not be conducted. Lastly, like most systematic reviews, only published studies were examined. There is a chance that these conclusions are subject to publication bias.
Knowledge Gaps and Directions for Future Research
There are several knowledge gaps that could be addressed in future research studies that examine the association between pre-injury migraines and clinical outcome from concussion. Many studies in this review recruited participants from specialty concussion clinics or hospital settings, while the most common setting in the United States for youth to receive a concussion diagnosis is from a primary care provider/pediatrician (64). Studies that sample individuals from specialty concussion clinics or emergency departments may represent a select and non-representative subset of individuals who sustain concussion, such that those who receive hospital care or specialty concussion services may have had more severe injury characteristics and/or are experiencing an atypical recovery. Designing studies that sample patients from more naturalistic and representative settings, such as primary care offices or prospectively following athletes via an injury surveillance system, will increase generalizability.
Further, studies should ensure they sample enough participants with migraines to achieve the statistical power necessary to detect differences between groups, if present. Additionally, future studies could more comprehensively describe the clinical characteristics of those with pre-injury migraines, by including empirically-supported migraine assessments, as well as measuring and reporting pre-injury migraine frequency, current migraine medication status, age of migraine onset, and pre-injury migraine functional impairment and disability. Future studies may also wish to examine the interplay between pre-injury migraines, prior concussions, and concussion recovery. It is possible that an athlete might develop a chronic migraine condition following a prior concussion, return to play, and then sustain a subsequent concussion. It will be important to study the clinical outcomes and recovery from concussion among these individuals.
The extant literature to date is inconclusive as to whether pre-injury migraine clearly confers risk for worse clinical outcome following a sport-related concussion—but it seems very likely that, at minimum, a subgroup of student athletes with pre-injury migraine are at increased risk. This would be most obvious for those who develop a mixed phenotype of post-traumatic headache, including worsening of their migraine condition, combined with difficulties with post-injury anxiety or depression. In the future, better prognostication—based on multiple possible risk factors such as pre-injury migraine, pre-injury mental health problems, and acute post-traumatic headache severity and the severity of other post-concussion symptoms—might, if implemented during the first few days following injury, allow for early targeted precision rehabilitation aimed at promoting a swifter and durable clinical recovery.
Data Availability Statement
The original contributions presented in the study are included in the article, further inquiries can be directed to the corresponding author.
Author Contributions
DT and GI conceptualized the study. DT, GI, AG, and NC screened abstracts. DT, FB, NH, AG, and NC extracted data and/or rated included studies. DT, FB, NH, NC, and GI all wrote portions of the manuscript. All authors contributed to the article and approved the submitted version.
Funding
This study was funded in part by the National Football League for a program of research entitled The Spectrum of Concussion: Predictors of Clinical Recovery, Treatment and Rehabilitation, and Possible Long-Term Effects (PI Iverson). The funder was not involved in the study design, collection, analysis, interpretation of data, the writing of this article or the decision to submit it for publication. GI acknowledges unrestricted philanthropic support from the Mooney-Reed Charitable Foundation, Heinz Family Foundation, Boston Bolts, National Rugby League, ImPACT Applications, Inc., and the Spaulding Research Institute. None of the above entities were involved in the study design, collection, analysis, interpretation of data, the writing of this article, or the decision to submit it for publication.
Conflict of Interest
GI serves as a scientific advisor for NanoDx® Inc., Sway Operations, LLC, and Highmark, Inc. He has a clinical and consulting practice in forensic neuropsychology, including expert testimony, involving individuals who have sustained mild TBIs (including athletes). He has received research funding from several test publishing companies, including ImPACT Applications, Inc., CNS Vital Signs, and Psychological Assessment Resources (PAR, Inc.). He has received research funding as a principal investigator from the National Football League, and salary support as a collaborator from the Harvard Integrated Program to Protect and Improve the Health of National Football League Players Association Members. DT has served as a consultant for REACT Neuro, Inc., and has a consulting practice in forensic neuropsychology involving individuals who have sustained mild TBIs (including athletes). AG serves as a scientific advisor for HitIQ, Ltd. He has a clinical practice in neuropsychology involving individuals who have sustained sport-related concussion (including current and former athletes). He is a contracted concussion consultant to Rugby Australia. He is a member of the World Rugby Concussion working group and the Australian Football League (AFL) Concussion Scientific Advisory Committee. He has received travel funding or been reimbursed by professional sporting bodies, and commercial organisations for discussing or presenting sport-related concussion research at meetings, scientific conferences, workshops, and symposiums. He has received research funding from the National Rugby League (NRL) and the Australian Institute of Sport (AIS) for the Retired Professional Rugby League Players Brain Health research program. Other previous research funding support includes: the NSW Sporting Injuries Committee, the Brain Foundation (Australia), and the Australian-American Fulbright Commission (Postdoctoral Award). He has received salary support from the Hunter Medical Research Institute (HMRI).
The remaining authors declare that the research was conducted in the absence of any commercial or financial relationships that could be construed as a potential conflict of interest.
Publisher's Note
All claims expressed in this article are solely those of the authors and do not necessarily represent those of their affiliated organizations, or those of the publisher, the editors and the reviewers. Any product that may be evaluated in this article, or claim that may be made by its manufacturer, is not guaranteed or endorsed by the publisher.
References
1. Iverson GL, Gardner AJ, Terry DP, Ponsford JL, Sills AK, Broshek DK, et al. Predictors of clinical recovery from concussion: a systematic review. Br J Sports Med. (2017) 51:941–8. doi: 10.1136/bjsports-2017-097729
2. Cook NE, Iaccarino MA, Karr JE, Iverson GL. Attention-deficit/hyperactivity disorder and outcome after concussion: a systematic review. J Dev Behav Pediatr. (2020) 41:571–82. doi: 10.1097/DBP.0000000000000808
3. Iverson GL, Williams MW, Gardner AJ, Terry DP. Systematic review of preinjury mental health problems as a vulnerability factor for worse outcome after sport-related concussion. Orthop J Sports Med. (2020) 8:2325967120950682. doi: 10.1177/2325967120950682
4. Headache Classification Committee of the International Headache Society (IHS). The international classification of headache disorders. Cephalalgia. (2013) 33:629–808. doi: 10.1177/0333102413485658
5. Abu-Arafeh I, Razak S, Sivaraman B, Graham C. Prevalence of headache and migraine in children and adolescents: a systematic review of population-based studies. Dev Med Child Neurol. (2010) 52:1088–97. doi: 10.1111/j.1469-8749.2010.03793.x
6. Burch RC, Loder S, Loder E, Smitherman TA. The prevalence and burden of migraine and severe headache in the United States: updated statistics from government health surveillance studies. Headache. (2015) 55:21–34. doi: 10.1111/head.12482
7. Terry DP, Wojtowicz M, Cook NE, Maxwell BA, Zafonte R, Seifert T, et al. Factors associated with self-reported concussion history in middle school athletes. Clin J Sport Med. (2018) 30:S69–74. doi: 10.1097/JSM.0000000000000594
8. Abrahams S, Mc Fie S, Patricios J, Posthumus M. September Av, Fie SM, et al. Risk factors for sports concussion: an evidence-based systematic review. Br J Sports Med. (2014) 48:91–7. doi: 10.1136/bjsports-2013-092734
9. Iverson GL, Silverberg ND, Mannix R, Maxwell BA, Atkins JE, Zafonte R, et al. Factors associated with concussion-like symptom reporting in high school athletes. JAMA Pediatr. (2015) 169:1132–40. doi: 10.1001/jamapediatrics.2015.2374
10. Zemek R, Barrowman N, Freedman SBB, Gravel J, Gagnon I, McGahern C, et al. Clinical risk score for persistent post-concussion symptoms among children with acute concussion in the ED. JAMA. (2016) 315:1014. doi: 10.1001/jama.2016.1203
11. Moran RN, Covassin T, Wallace J. Premorbid migraine history as a risk factor for vestibular and oculomotor baseline concussion assessment in pediatric athletes. J Neurosurg Pediatr. (2019) 23:465–70. doi: 10.3171/2018.10.PEDS18425
12. Terry DP, Reddi P, Cook NE, Seifert TD, Maxwell BA, Zafonte R, et al. Acute effects of concussion in youth with pre-existing migraines. Clin J Sport Med. (2021) 31:430–7. doi: 10.1097/JSM.0000000000000791
13. Morgan CD, Zuckerman SL, Lee YM, King L, Beaird S, Sills AK, et al. Predictors of post-concussion syndrome after sports-related concussion in young athletes: a matched case-control study. J Neurosurg Pediatr. (2015) 15:589–98. doi: 10.3171/2014.10.PEDS14356
14. Miller JH, Gill C, Kuhn EN, Rocque BG, Menendez JY, O'Neill JA, et al. Predictors of delayed recovery following pediatric sports-related concussion: a case-control study. J Neurosurg Pediatr. (2016) 17:491–6. doi: 10.3171/2015.8.PEDS14332
15. Lau BC, Collins MW, Lovell MR. Cutoff scores in neurocognitive testing and symptom clusters that predict protracted recovery from concussions in high school athletes. Neurosurgery. (2012) 70:371–9. doi: 10.1227/NEU.0b013e31823150f0
16. Eisenberg MA, Andrea J, Meehan W, Mannix R. Time interval between concussions and symptom duration. Pediatrics. (2013) 132:8–17. doi: 10.1542/peds.2013-0432
17. Ellis MJ, Ritchie LJ, Koltek M, Hosain S, Cordingley D, Chu S, et al. Psychiatric outcome after pediatric sports-related concussion. J Neurosurg Pediatr. (2015) 16:709–18. doi: 10.3171/2015.5.PEDS15220
18. McDevitt J, Tierney RT, Phillips J, Gaughan JP, Torg JS, Krynetskiy E. Association between GRIN2A promoter polymorphism and recovery from concussion. Brain Injury. (2015) 29:1674–81. doi: 10.3109/02699052.2015.1075252
19. Meehan WP, Mannix RC, Stracciolini A, Elbin RJ, Collins MW. Symptom severity predicts prolonged recovery after sport-related concussion, but age and amnesia do not. J Pediatr. (2013) 163:721–5. doi: 10.1016/j.jpeds.2013.03.012
20. Meehan WP, Mannix R, Monuteaux MC, Stein CJ, Bachur RG. Early symptom burden predicts recovery after sport-related concussion. Neurology. (2014) 83:2204–10. doi: 10.1212/WNL.0000000000001073
21. Nelson LD, Tarima S, LaRoche AA, Hammeke TA, Barr WB, Guskiewicz K, et al. Preinjury somatization symptoms contribute to clinical recovery after sport-related concussion. Neurology. (2016) 86:1856–63. doi: 10.1212/WNL.0000000000002679
22. Moher D, Liberati A, Tetzlaff J, Altman DG. PRISMA Group. Preferred reporting items for systematic reviews and meta-analyses: the PRISMA statement. PloS Med. (2009) 6:e1000097. doi: 10.1371/journal.pmed.1000097
23. Hayden JA, van der Windt DA, Cartwright JL, Côté P, Bombardier C. Assessing bias in studies of prognostic factors. Ann Intern Med. (2013) 158:280–6. doi: 10.7326/0003-4819-158-4-201302190-00009
24. Centre for Evidence-Based Medicine. OCEBM Levels of Evidence. Available online at: https://www.cebm.net/index.aspx?o=5653 (accessed December 15, 2021).
25. Campbell JC. Health consequences of intimate partner violence. Lancet. (2002) 359:1331–6. doi: 10.1016/S0140-6736(02)08336-8
26. Campbell M, Katikireddi SV, Sowden A, McKenzie JE, Thomson H. Improving Conduct and Reporting of Narrative Synthesis of Quantitative Data (ICONS-Quant): protocol for a mixed methods study to develop a reporting guideline. BMJ Open. (2018) 8:e020064. doi: 10.1136/bmjopen-2017-020064
27. Lakens D, Etz AJ. Too true to be bad: when sets of studies with significant and non-significant findings are probably true. Soc Psychol Personal Sci. (2017) 8:875–81. doi: 10.1177/1948550617693058
28. Büttner F, Terry DP, Iverson GL. Using a likelihood heuristic to summarize conflicting literature on predictors of clinical outcome following sport-related concussion. Clin J Sport Med. (2021) 31:e476–83. doi: 10.1097/JSM.0000000000000825
30. Lau BC, Collins MW, Lovell MR. Sensitivity and specificity of subacute computerized neurocognitive testing and symptom evaluation in predicting outcomes after sports-related concussion. Am J Sports Med. (2011) 39:1209–16. doi: 10.1177/0363546510392016
31. Lau BC, Kontos AP, Collins MW, Mucha A, Lovell MR. Which on-field signs/symptoms predict protracted recovery from sport-related concussion among high school football players? Am J Sports Med. (2011) 39:2311–8. doi: 10.1177/0363546511410655
32. Hiploylee C, Dufort PA, Davis HS, Wennberg RA, Tartaglia MC, Mikulis D, et al. Longitudinal study of post-concussion syndrome: not everyone recovers. J Neurotrauma. (2017) 34:1511–23. doi: 10.1089/neu.2016.4677
33. Neidecker JM, Gealt DB, Lambert K, Luksch JR, Weaver MD. First-time sports-related concussion recovery revisited: management changes and impact on recovery. J Osteopa Med. (2021) 121:49–56. doi: 10.1515/jom-2020-0106
34. Meehan WP, O'Brien MJ, Geminiani E, Mannix R. Initial symptom burden predicts duration of symptoms after concussion. J Sci Med Sport. (2016) 19:722–5. doi: 10.1016/j.jsams.2015.12.002
35. Howell DR, Zemek R, Brilliant AN, Mannix RC, Master CL, Meehan WP. Identifying persistent post-concussion symptom risk in a pediatric sports medicine clinic. Am J Sports Med. (2018) 46:3254–61. doi: 10.1177/0363546518796830
36. Howell DR, Potter MN, Kirkwood MW, Wilson PE, Provance AJ, Wilson JC. Clinical predictors of symptom resolution for children and adolescents with sport-related concussion. J Neurosurg. (2019) 24:54–61. doi: 10.3171/2018.11.PEDS18626
37. Kontos AP, Elbin RJ, Sufrinko A, Marchetti G, Holland CL, Collins MW. Recovery following sport-related concussion: integrating pre- and post-injury factors into multidisciplinary care. J Head Trauma Rehabil. (2019) 34:394–401. doi: 10.1097/HTR.0000000000000536
38. Kontos AP, Jorgensen-Wagers K, Trbovich AM, Ernst N, Emami K, Gillie B, et al. Association of time since injury to the first clinic visit with recovery following concussion. JAMA Neurol. (2020) 77:435–40. doi: 10.1001/jamaneurol.2019.4552
39. Fehr SD, Nelson LD, Scharer KR, Traudt EA, Veenstra JM, Tarima SS, et al. Risk factors for prolonged symptoms of mild traumatic brain injury: a pediatric sports concussion clinic cohort. Clin J Sport Med. 29:11–7. doi: 10.1097/JSM.0000000000000494
40. Sinnott AM, Elbin RJ, Collins MW, Reeves VL, Holland CL, Kontos AP. Persistent vestibular-ocular impairment following concussion in adolescents. J Sci Med Sport. (2019) 22:1292–7. doi: 10.1016/j.jsams.2019.08.004
41. Howell DR, O'Brien MJ, Beasley MA, Mannix RC, Meehan WP. Initial somatic symptoms are associated with prolonged symptom duration following concussion in adolescents. Acta Paediatr. (2016) 105:e426–32. doi: 10.1111/apa.13486
42. Eagle SR, Puligilla A, Fazio-Sumrok V, Kegel N, Collins MW, Kontos AP. Association of time to initial clinic visit with prolonged recovery in pediatric patients with concussion. J Neurosurg Pediatr. (2020) 26:165–70. doi: 10.3171/2020.2.PEDS2025
43. Aggarwal SS, Ott SD, Padhye NS, Meininger JC, Armstrong TS. Clinical and demographic predictors of concussion resolution in adolescents: a retrospective study. Appl Neuropsychol Child. (2019) 8:50–60. doi: 10.1080/21622965.2017.1381099
44. Popovich M, Almeida A, Freeman J, Eckner JT, Alsalaheen B, Lorincz M, et al. Use of supervised exercise during recovery following sports-related concussion. Clin J Sport Med. (2021) 31:127–32. doi: 10.1097/JSM.0000000000000721
45. Terry DP, Huebschmann NA, Maxwell BA, Cook NE, Mannix R, Zafonte R, et al. Preinjury migraine history as a risk factor for prolonged return to school and sports following concussion. J Neurotrauma. (2019) 36:142–51. doi: 10.1089/neu.2017.5443
46. Beauchamp MH, Tang K, Yeates KO, Anderson P, Brooks BL, Keightley M, et al. Predicting wellness after pediatric concussion. J Int Neuropsychol Soc. (2019) 25:375–89. doi: 10.1017/S1355617719000286
47. Mihalik JP, Register-Mihalik J, Kerr ZY, Marshall SW, McCrea MC, Guskiewicz KM. Recovery of post-traumatic migraine characteristics in patients after mild traumatic brain injury. Am J Sports Med. (2013) 41:1490–6. doi: 10.1177/0363546513487982
48. Lucas S, Hoffman JM, Bell KR, Walker W, Dikmen S. Characterization of headache after traumatic brain injury. Cephalalgia. (2012) 32:600–6. doi: 10.1177/0333102412445224
49. Packard RC. Epidemiology and pathogenesis of post-traumatic headache. J Head Trauma Rehabil. (1999) 14:9–21. doi: 10.1097/00001199-199902000-00004
50. Asplund CA, McKeag DB, Olsen CH. Sport-related concussion: factors associated with prolonged return to play. Clin J Sport Med. (2004) 14:339–43. doi: 10.1097/00042752-200411000-00003
51. Benson BW, Meeuwisse WH, Rizos J, Kang J, Burke CJ, A. prospective study of concussions among national hockey league players during regular season games: the NHL-NHLPA concussion program. CMAJ. (2011) 183:905–11. doi: 10.1503/cmaj.092190
52. Register-Mihalik JK, Mihalik JP, Guskiewicz KM. Association between previous concussion history and symptom endorsement during preseason baseline testing in high school and collegiate athletes. Sports Health. (2009) 1:61–5. doi: 10.1177/1941738108325920
53. Mihalik JP, Lengas E, Register-Mihalik JK, Oyama S, Begalle RL, Guskiewicz KM. The effects of sleep quality and sleep quantity on concussion baseline assessment. Clin J Sport Med. (2013) 23:343–8. doi: 10.1097/JSM.0b013e318295a834
54. Mihalik JP, Stump JE, Collins MW, Lovell MR, Field M, Maroon JC. Post-traumatic migraine characteristics in athletes following sports-related concussion. J Neurosurg. (2005) 102:850–5. doi: 10.3171/jns.2005.102.5.0850
55. Gilkey SJ, Ramadan NM, Aurora TK, Welch KM. Cerebral blood flow in chronic post-traumatic headache. Headache. (1997) 37:583–7. doi: 10.1046/j.1526-4610.1997.3709583.x
56. Giza CC, Kutcher JS, Ashwal S, Barth J, Getchius TSD, Gioia GA, et al. Summary of evidence-based guideline update: evaluation and management of concussion in sports: report of the guideline development subcommittee of the American academy of neurology. Neurology. (2013) 80:2250–7. doi: 10.1212/WNL.0b013e31828d57dd
57. Lauritzen M. Pathophysiology of the migraine aura. The spreading depression theory. Brain. (1994) 117:199–210. doi: 10.1093/brain/117.1.199
58. Lucas S. Headache management in concussion and mild traumatic brain injury. PM R. (2011) 3:S406–12. doi: 10.1016/j.pmrj.2011.07.016
59. Packard RCC, Ham LPP. Pathogenesis of post-traumatic headache and migraine: a common headache pathway? Headache. (1997) 37:142–52. doi: 10.1046/j.1526-4610.1997.3703142.x
60. Kamins J, Charles A. Post-traumatic headache: basic mechanisms and therapeutic targets. Headache. (2018) 58:811–26. doi: 10.1111/head.13312
61. Steiner TJ, Gururaj G, Andrée C, Katsarava Z, Ayzenberg I, Yu SY, et al. Diagnosis, prevalence estimation and burden measurement in population surveys of headache: presenting the HARDSHIP questionnaire. J Headache Pain. (2014) 15:3. doi: 10.1186/1129-2377-15-3
62. Vetvik KG, MacGregor EA. Sex differences in the epidemiology, clinical features, and pathophysiology of migraine. Lancet Neurol. (2017) 16:76. doi: 10.1016/S1474-4422(16)30293-9
63. Hoffman JM, Lucas S, Dikmen S, Braden CA, Brown AW, Brunner R, et al. Natural history of headache after traumatic brain injury. J Neurotrauma. (2011) 28:1719–25. doi: 10.1089/neu.2011.1914
Keywords: migraine, concussion, sport-related concussion, traumatic brain injury, systematic review, prolonged recovery
Citation: Terry DP, Büttner F, Huebschmann NA, Gardner AJ, Cook NE and Iverson GL (2022) Systematic Review of Pre-injury Migraines as a Vulnerability Factor for Worse Outcome Following Sport-Related Concussion. Front. Neurol. 13:915357. doi: 10.3389/fneur.2022.915357
Received: 07 April 2022; Accepted: 23 May 2022;
Published: 20 June 2022.
Edited by:
Darrin Jason Lee, University of Southern California, United StatesReviewed by:
Gokul Krishna, University of Arizona, United StatesRyan N. Moran, University of Alabama, United States
Copyright © 2022 Terry, Büttner, Huebschmann, Gardner, Cook and Iverson. This is an open-access article distributed under the terms of the Creative Commons Attribution License (CC BY). The use, distribution or reproduction in other forums is permitted, provided the original author(s) and the copyright owner(s) are credited and that the original publication in this journal is cited, in accordance with accepted academic practice. No use, distribution or reproduction is permitted which does not comply with these terms.
*Correspondence: Douglas P. Terry, ZG91Z2xhcy50ZXJyeUB2dW1jLm9yZw==