- 1College of Health and Life Sciences, Hamad Bin Khalifa University, Doha, Qatar
- 2Child and Adolescent Mental Health Service, Hamad Medical Corporation, Doha, Qatar
Tourette syndrome (TS) and obsessive-compulsive disorder (OCD) are two neuropsychiatric disorders that frequently co-occur. Previous evidence suggests a shared genetic diathesis underlying the comorbidity of TS and OCD. This review aims to comprehensively summarize the current literature on the genetic factors linked with TS and its comorbidities, with a focus on OCD. Family studies, linkage analysis, cytogenetic studies, and genome-wide association studies (GWAS) have played a pivotal role in identifying common and rare genetic variants connected with TS and OCD. Although the genetic framework of TS and OCD is complex and multifactorial, several susceptibility loci and candidate genes have been identified that might play a crucial role in the pathogenesis of both disorders. Additionally, post-infectious environmental elements have also been proposed to contribute to the development of TS-OCD, although the dynamics between genetic and environmental factors is not yet fully understood. International collaborations and studies with well-defined phenotypes will be crucial in the future to further elucidate the genetic basis of TS and OCD and to develop targeted therapeutic strategies for individuals suffering from these debilitating conditions.
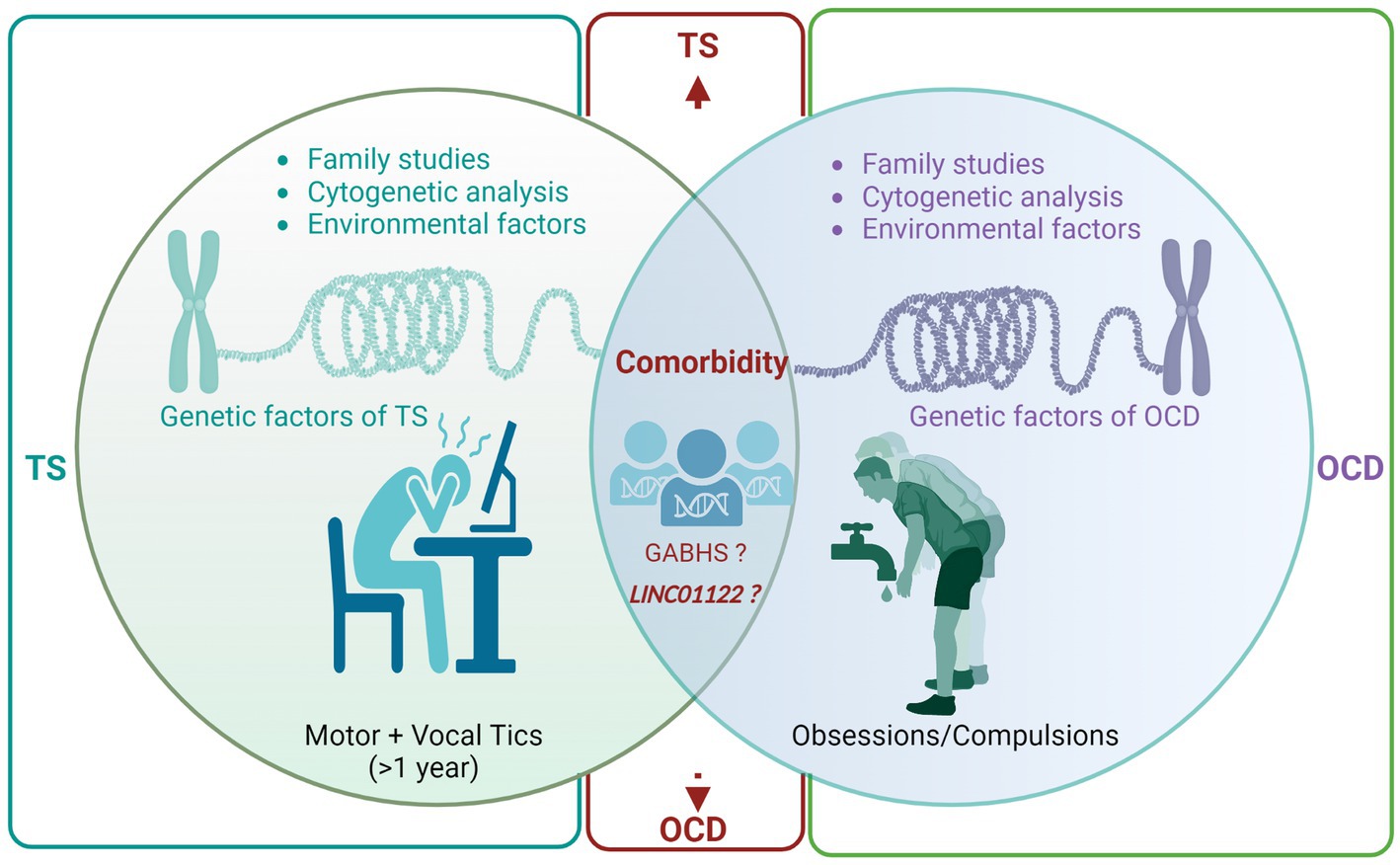
Graphical Abstract. Genetic studies indicate a complex interplay of multiple genes contributing to TS and OCD, with a potential shared genetic diathesis in certain families leading to the manifestation of either or both disorders. GWAS have identified significant genetic overlap between TS and OCD, including SNPs in the LINC01122 gene, suggesting a common genetic nexus for both conditions. Environmental factors such as post-infection autoimmune reactions (GABHS) and psychosocial stressors play a role in exacerbating TS and OCD symptoms, highlighting the need for a holistic approach to understanding these disorders. The evolving field of pharmacogenomics in TS and OCD treatment was discussed, with emphasis placed on the importance of personalized pharmacological interventions tailored to individual genetic variations, particularly in drug metabolism pathways. The emerging role of epigenetic modulation in TS and OCD comorbidity was explored, with emphasis placed on the need for comprehensive research into epigenetic mechanisms and their potential impact on diagnosis and treatment approaches in the future. GABHS, Group a beta-hemolytic streptococci; GWAS, Genome-wide associated studies; LINC01122, Long intergenic non-protein coding RNA 1122; SNP, Single nucleotide polymorphism; OCD, obsessive-compulsive disorder; TS, Tourette syndrome.
Introduction
More than a hundred years ago, the pioneering French neurologist, Gilles de la Tourette, delineated Tourette syndrome (TS) in nine individuals exhibiting repetitive motor and vocal tics during their childhood (1). For a considerable period, TS was erroneously deemed psychogenic until the late 1960s when Drs. Arthur and Elaine Shapiro reignited scientific curiosity in the disorder by proposing its organic nature (1). Subsequent research has tremendously enhanced our comprehension of TS, categorizing it as a multifaceted neurodevelopmental and neuropsychiatric disorder. TS is estimated to afflict 0.1 to 1% of the general population and 1% of school-going children, exhibiting a conspicuous male bias, being 2–10 times more prevalent in males than females (2, 3). A significant proportion of TS patients concurrently suffer from other psychopathologies, notably obsessive-compulsive disorder (OCD), marked by obsessions and/or compulsions that substantially hinder daily functioning (4). Intriguingly, Gilles de la Tourette’s initial characterization of TS noted the presence of obsessions and compulsions among the affected individuals, thereby implying a shared genetic predisposition (1).
Tourette syndrome (TS), attention deficit hyperactivity disorder (ADHD), autism spectrum disorder (ASD), and OCD are intricate neurodevelopmental disorders with a myriad of common and rare genetic factors influencing their onset (5–9). Although recent studies have pinpointed 12 significant genetic loci for ADHD (10) and five for ASD (11, 12), only a single significant genetic locus has been recently discerned for TS (13), and, strikingly, none for OCD (14).
This concise review endeavors to elucidate the prevailing understanding of the most prominent concepts associated with the genetic underpinnings of TS and OCD. Moreover, the review accentuates the conceivable influence of environmental risk factors on the manifestation of these neuropsychiatric conditions. Lastly, the review contemplates the directions for forthcoming research on TS and OCD genetics, encompassing the potential identification of novel therapeutic targets. Ultimately, a more profound comprehension of the complex interplay between genetic and environmental determinants precipitating the onset of these disorders is imperative for devising more efficacious interventions and enhancing the prognosis for affected individuals.
Heritability and familial clustering of Tourette syndrome: evidence from family-based studies
Robust evidence underscores the heritability of TS, a neurodevelopmental condition. Family studies have invariably demonstrated the familial aggregation of TS and associated tic disorders, evidenced by a higher prevalence of the disorder among immediate relatives relative to the general population (15). For instance, the prevalence of TS is approximately 5% in sisters and exceeds 10% in brothers, which signifies a ten-to thirty-fold elevated risk compared to the general population, considering that the estimated prevalence estimate of TS in child population varies between 0.3 and 0.9% (16). Additionally, gender-specific phenotypes have been recognized, with females exhibiting a propensity toward obsessive-compulsive disorders or behaviors, whereas males predominantly manifest TS and tics. Furthermore, genomic imprinting appears to modulate the phenotypic expression of TS, as illustrated by a study revealing a parent-of-origin effect, whereby an earlier onset is observed in offspring inheriting the disorder from their mother (17, 18).
While the familial distribution of TS suggests a genetic association, other determinants, such as infectious or environmental risk factors, might also be contributory (19). Twin studies have yielded unambiguous evidence of the proportional contributions of genetics and the environment to TS, revealing a notably increased concordance in monozygotic twins in comparison to dizygotic twins. Concordance rates among monozygotic twins have been reported to range from 53 to 56% and even reach 100% when the study methodology encompassed firsthand patient assessment and the diagnosis of chronic tics in conjunction with TS (20–22).
Numerous investigations have endeavored to elucidate the mode of TS’s inheritance, with segregation analyses proposing its transmission as an autosomal dominant trait with incomplete penetrance (23–25). Conversely, other studies have posited that TS may emanate from a major effect gene, with supplementary contributions from the genetic background and environmental determinants (26). However, a coherent depiction of the precise mode of inheritance remains elusive (27). TS likely originates from the interaction of multiple gene alleles, each exerting relatively modest effects compared to Mendelian disorders, and epigenetic influences culminating in diverse phenotypic outcomes.
Interestingly, the paradox of pronounced heritability without identified definitive mutations is not unique to TS. Essential Tremor (ET) is another classical example where, despite clear heritability and extensive research, the responsible mutations remain elusive (28). Several candidate genes have been implicated in ET, much like in TS, but a conclusive link has yet to be established (28). This phenomenon is not uncommon, as only a fraction of familial diseases, such as hereditary ataxias or dementias, have confirmed mutations (29, 30). The acknowledgment of this paradox enriches our understanding of the complexities involved in genetic research and the heritability of certain disorders.
Interplay of genetic factors in Tourette syndrome and associated psychiatric disorders
In clinical and population-based samples, the occurrence of TS is more frequently associated with comorbidities rather than as an isolated condition, illustrating the concept that comorbidity is the ‘exception rather than the rule’. A notable comorbidity is ADHD, a neurodevelopmental disorder characterized by a persistent pattern of inattention and/or hyperactivity-impulsivity that impairs functioning or development, which has a prevalence of 54.3% among individuals with TS (4, 31). This concurrent manifestation significantly contributes to disruptive behaviors and academic challenges (32). The potential shared genetic etiology between these two neurodevelopmental disorders has been a subject of debate (33). Although there is evidence supporting the independent transmission of these disorders within families, the high comorbidity rate suggests the possibility of a shared genetic component, at least in certain TS-ADHD subgroups. A latent class analysis involving 952 individuals from 222 TS families indicated that those with TS, ADHD, and OCD might have the most heritable form of the disorder (34). Moreover, seven genes, namely DRD2, HRH3, MAOB, BDNF, SNAP25, and SLC6A4, have been identified in the pathogenesis of both TS and ADHD, hinting at a shared pathogenic pathway (35).
Additionally, there is a higher incidence of co-occurrence of TS and ASD compared to the general population (36). A study into genomic copy number variations (CNVs) in TS showed a notable overlap between rare CNVs associated with TS and ASD, albeit no such overlay was observed with schizophrenia or intellectual disability (37). Additionally, these neurodevelopmental disorders share genetic underpinnings involving genes such as CNTNAP2 and IMMP2L, as well as the neurexin gene family (38).
The coexistence of depressive symptoms with TS may be attributed to either a shared genetic predisposition or the psychological ramifications of living with tics. A Taiwanese study involving 1,337 TS patients revealed a significantly higher incidence of depression (4.85 times more) among TS patients compared to those without TS (39). The underlying cause of this shared genetic association remains unclear. Some researchers postulate that depression is integral to TS and that both disorders share a similar genetic foundation (40). Conversely, other experts contend that TS and depression are genetically distinct, and depression may arise from the emotional struggles associated with managing a chronic illness (41). Table 1 provides an overview of studies exploring the genomics of comorbid TS and other mental health disorders.
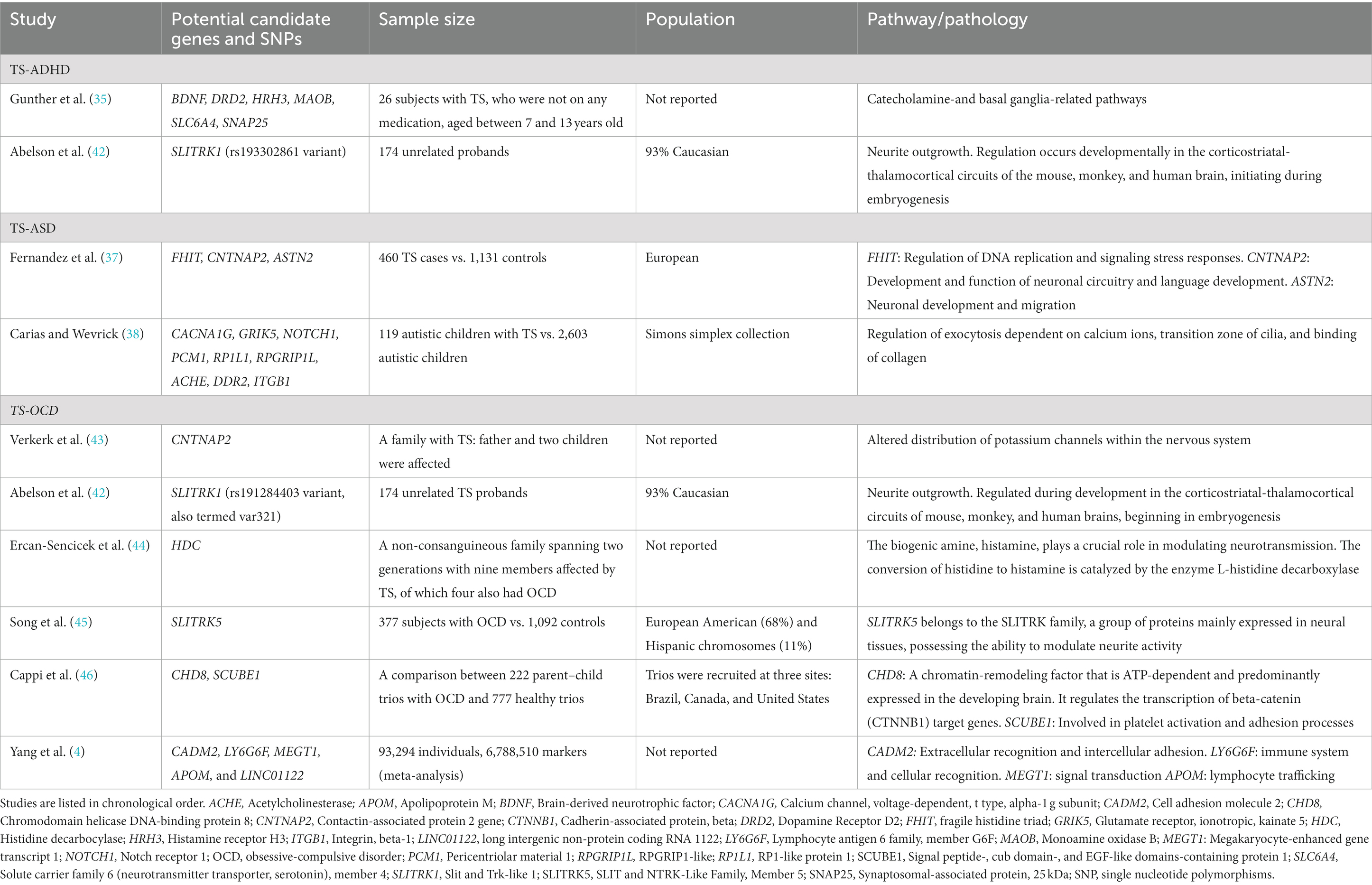
Table 1. Shows studies highlighting the genomics of comorbid Tourette syndrome with other psychiatric disorders.
Decoding the genetic nexus between TS and OCD
Family studies
Studies conducted globally have estimated that OCD affects 2–3% of the population throughout their lifetime (47). family studies have revealed that 30 to 60% of probands with TS also meet the diagnostic criteria for OCD (23, 48), signifying an elevated risk of OCD in family members of individuals with TS. However, this elevated risk does not necessarily infer a singular genetic predisposition leading to different manifestations. It could be attributed to common pathophysiological mechanisms or aggregation of environmental hazards rather than a shared genetic background. Nonetheless, several studies reported higher OCD rates (without tics) among TS patients’ relatives who do not exhibit obsessive-compulsive symptoms compared to unaffected families (21, 23, 48–50). Conversely, family studies involving OCD probands consistently revealed increased tic disorder rates (50–52). Moreover, multiple clinical case series indicated that individuals with tic-related OCD frequently exhibit symmetry and precision obsessions, a need to repeat activities for completion, or a ‘just right’ sensation (53, 54). In summary, the high OCD prevalence in TS patients and their families, obsessive-compulsive symptom patterns in TS pedigrees, and a distinct natural history of tic-related OCD indicate that a shared genetic diathesis in some families could manifest as TS, OCD, or both.
Linkage analysis
The implication of genetics in disorders was elucidated through research on families and twins, which necessitated identifying specific genetic loci involved. The autosomal dominant inheritance pattern observed in studies marked TS as a prime candidate for linkage analysis. Yet, despite years of parametric linkage studies, no specific genetic locus, except for the HDC gene, which codes for the enzyme L-histidine decarboxylase, has been pinpointed in the etiology of this neurodevelopmental disorder. L-histidine decarboxylase is the rate-limiting enzyme in histamine biosynthesis (55). A rare functional mutation in the HDC gene resulting in loss of enzyme activity was identified by Ercan-Sencicek et al. in a nonconsanguineous two-generation family with nine affected members, four of whom had OCD (44). Additionally, Fernandez et al. found a robust association between rare copy variants in families with TS and histaminergic signaling, notably in the H1 receptor pathways (37). These significant findings implicate that histaminergic neurotransmission could be involved in TS’s pathobiology, not only in this particular family but possibly on a broader scale.
Histamine (HA) signaling within the central nervous system is mediated by four 7-transmembrane G protein-coupled receptors, both pre-synaptically (predominantly H3 and H4) and post-synaptically (H1–H3), with presynaptic HA receptors being responsible for regulating the release of HA and dopamine (56). Existing evidence suggests a counter-regulatory role of HA, wherein decreased HA results in increased dopamine signaling and vice versa (57). The H2 and H3 receptors, enriched in the striatum and cortex, are critical brain regions implicated in TS (55). In TS, an excess of dopamine in the striatum is believed to stimulate the thalamocortical circuits, a theory substantiated by the effectiveness of dopamine blockers in suppressing TS symptoms (58). Recently, activating the histaminergic afferent system, particularly H3, has ameliorated obsessive-compulsive-like behaviors (59). These findings hold promise for developing innovative therapeutic strategies for TS and OCD.
Consanguinity
Investigating the potential contribution of recessive genetic variations to reduced penetrance of TS is a complex task. However, autozygosity, which occurs when both alleles at a genetic location are inherited from a single ancestor, allows the application of homozygosity mapping even in small families. This technique has been used to detect recessive variations in highly consanguineous populations (60). Motlagh et al. identified 12 Iranian families with TS characterized by early emergence of vocal tics, coprolalia, and, often, coexisting OCD (61). Analyzing the genetic constitution of affected and unaffected family members could uncover rare recessive variations associated with the comorbidity of TS and OCD.
Cytogenetic studies
Cytogenetic techniques such as karyotyping, fluorescent in situ hybridization, and array comparative genomic hybridization are valuable tools for detecting chromosomal irregularities like translocations, deletions, and duplications. By identifying genes located near these disrupted regions, potential genes that may increase susceptibility can be identified for further study. Notable chromosomal abnormalities linked with TS familes have been found on several chromosomes, including 2p12, 3p21.3, 7q35-36, 8p21.4, partial trisomy 9, 13q31, and 18q22.3 (43, 62–64).
State and colleagues described a patient with chronic tics (CT) and OCD, who displayed a specific inversion on chromosome 18q22 (62). The telomeric end of this inversion was close (within 1 Mb) to a translocation breakpoint earlier noted by another pedigree study (65). Although the inversion did not structurally alter any genes, functional analysis of two transcripts in that area showed changes in replication timing. This hints at the influence of epigenetics on gene activity, indicating that genes in this zone might be relevant for TS.
Cuker et al. described a translocation t (2, 18)(p12;q22) in a 14-year-old female exhibiting pronounced symptoms of OCD and CT (64). Interestingly, this patient’s breakpoint on chromosome 18 was in proximity (approximately 5 Mb) to two previously recognized rearrangements linked to TS, OCD, and CT, suggesting that this particular chromosomal region may house pivotal genes associated with the TS/OCD symptom range (62, 65).
In another case, Ververk and colleagues studied a family with a history of TS and OCD, identifying a complicated chromosomal anomaly that affected the CNTNAP2 gene, a massive gene in our genome (43). This gene, which comprises 25 exons and spans 2.0 Mb, codes for a membrane protein situated at a distinct point at the nodes of Ranvier of axons (66). They proposed that CNTNAP2 could be a key gene for TS/OCD due to its role in signal transmission, a process known to be disrupted in this combined disorder (43).
Genome-wide associated studies (GWAS)
The initial genome-wide examination of large and rare CNVs in OCD has identified a recurrent deletion in the 16p13.11 genomic region (67). This specific deletion appeared in individuals with both OCD and TS and those with only OCD, suggesting a potential genetic association with both conditions. Furthermore, the study unveiled genomic alterations in the 22q11 region in several participants with TS and OCD, corroborating findings from an earlier report on velocardiofacial syndrome (68).
A recent meta-analysis investigated the genetic overlap between TS and OCD using GWAS data (4). This research identified 21 significant genome-wide SNPs located in the LINC01122 gene on chromosome 2p16.1, all manifesting a consistent directional impact on both conditions. Notably, this specific region was absent in prior significant findings from a comprehensive analysis spanning eight psychiatric disorders, implying its potential uniqueness to the TS-OCD linkage (69). These results allude to a substantial genetic convergence between TS and OCD, potentially guiding the formulation of innovative therapeutic approaches. Concurrently, the study brought to light four novel genes—CADM2, LY6G6F, MEGT1, and APOM—previously associated with diverse neurological activities. Nevertheless, subsequent in-depth functional evaluations are necessary to clarify the exact functions of these genes in predisposing individuals to TS and OCD, as well as their viability as treatment targets.
Figure 1 provides a detailed visualization of the extensive research conducted to understand the genetic relationship between TS and OCD.
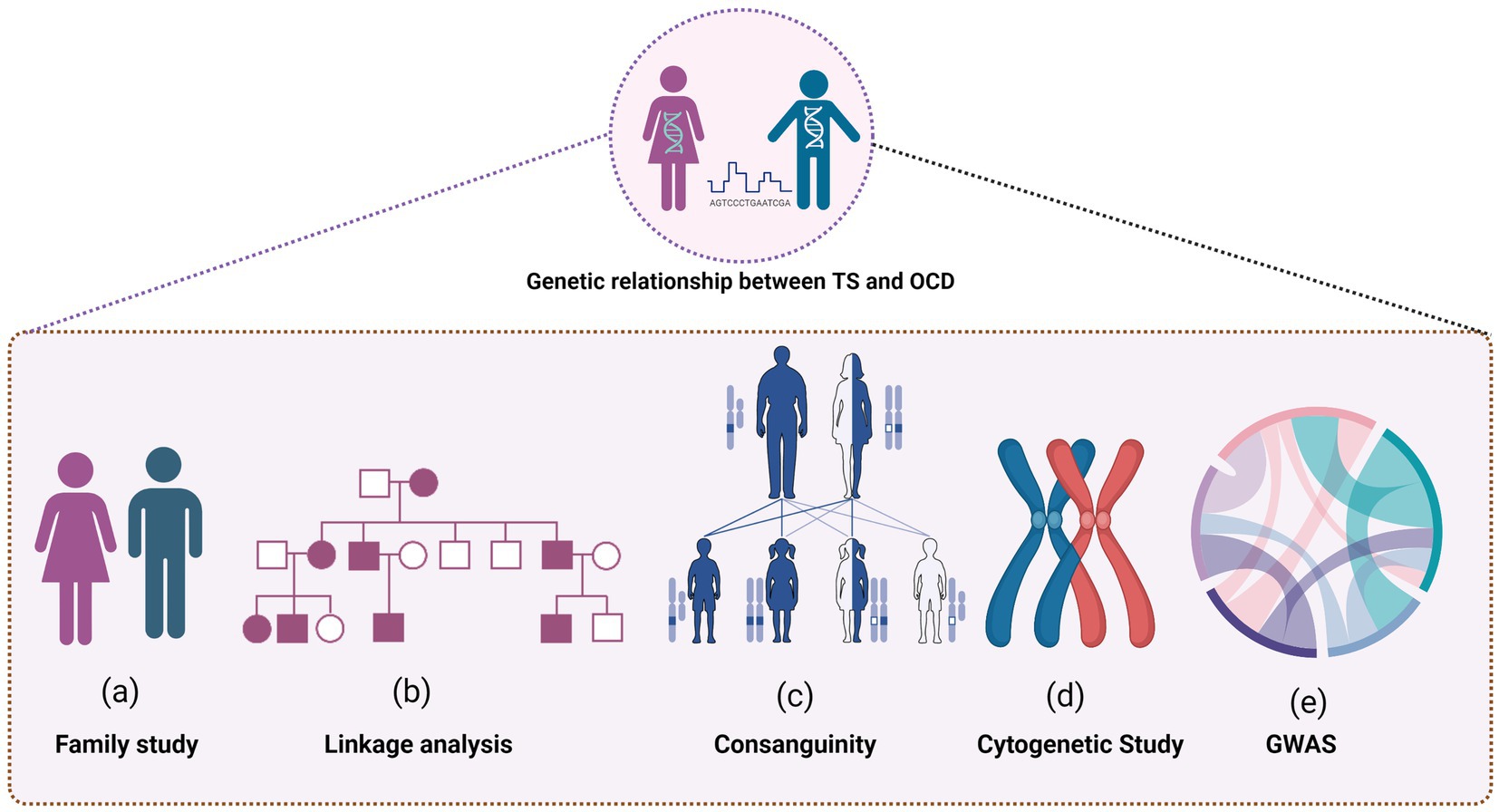
Figure 1. Several research methodologies were employed to uncover the intricate connections, including family studies to explore the hereditary nature of TS and OCD, and linkage analysis to pinpoint the specific genetic loci involved. The role of consanguinity was also examined, shedding light on how shared genetic backgrounds could influence the prevalence of TS and OCD. Cytogenetic studies offered insights into chromosomal variations and abnormalities associated with the conditions, contributing to the broader understanding of their genetic underpinnings. Furthermore, the figure highlights the findings from Genome-Wide Association Studies (GWAS), which helped to identify potential genetic markers and risk alleles associated with the development of both disorders.
Environmental factors
Multiple environmental contributors have been proposed to influence the onset of TS, including complications during pregnancy and delivery, androgen exposure, thermal stress and fatigue, post-infection autoimmune reactions, and psychosocial distress. This review will focus specifically on the last two.
The proposition that post-infectious autoimmune responses might cause TS and OCD originated in the 1800s (70) and remains a contentious subject in contemporary research (71). It is recognized that Group A beta-hemolytic streptococci (GABHS) can spark immune-driven conditions in genetically susceptible individuals. In 1998, Swedo and colleagues documented a cohort of patients manifesting OCD and TS symptoms concurrent with GABHS exposure (19). They postulated that GABHS-triggered immune reactions target the basal ganglia, a neural region previously implicated in other movement disorders. Consequently, they introduced the concept that Pediatric Autoimmune Neuropsychiatric Disorder Associated with Streptococcal infection (PANDAS) could be a discrete clinical category encompassing certain TS and OCD cases.
Moreover, TS patients frequently report elevated psychosocial stress, and latent class modeling on longitudinal datasets indicated that preceding stress events might amplify the intensity of obsessive-compulsive manifestations (72). Interestingly, Lin et al. found that GABHS infections could modestly predict future exacerbations in tic and OCD symptom intensity and further strengthen the prognostic value of existing psychosocial stress on ensuing tic and OCD symptom intensification (73). Buse et al. proposed a hypothesis suggesting stress might modulate tic pathology via dopaminergic and noradrenergic signaling pathways and immune mechanisms (74). They also indicated the possibility of mutual interactions between psychosocial stress and TS-associated aberrations in neurotransmission or immune responses. A study by Adams et al. demonstrated that stress can worsen OCD symptoms, impacting neural pathways like the corticostriatal and limbic systems, which results in neuronal growth in specific regions and shrinkage in others (75). Such neural shifts may foster an imbalance in purposeful versus routine/habitual behaviors, which could underlie the development and manifestation of OCD characteristics. Collectively, these insights underline the intricate relationships between GABHS infections, psychosocial stressors, and the severity of TS and OCD symptoms, highlighting opportunities for innovative therapeutic strategies.
Future directions and conclusion
Epigenetic dimensions
The domain of epigenetic modulation is rapidly emerging as a potentially pivotal area for decoding the intertwined etiologies of TS and OCD comorbidity. Existing evidence compellingly delineates specific ‘developmental windows’ wherein the genetically anchored micro-circuitry of crucial limbic-hypothalamic-midbrain architectures is vulnerable to early environmental factors. These early exposures appear to profoundly dictate an individual’s reactivity to psychosocial challenges and their aptitude for nurturing subsequent generations. Investigations have shown that these initial environmental interactions can alter methylation patterns within the promoter region of the glucocorticoid receptor gene, influencing the hypothalamic–pituitary–adrenal (HPA) stress response. Notably, pronounced maternal stress during gestation has been linked to a more complex form of TS (76). In tandem, cross-sectional and longitudinal research concerning TS and early-onset OCD imply a heightened sensitivity of these conditions to psychosocial stress (72). TS-affected individuals have been observed to exhibit an augmented stress reaction mediated by the HPA pathway (77, 78), accompanied by increased cerebrospinal fluid (CSF) concentrations of corticotropin-releasing hormone (79). Such observations resonate with epigenetic manifestations, as described by Meaney et al. (80).
Pharmacogenomic perspectives
Today, physicians are moving away from a “one size fits all” model to one that considers individual patient differences. The ultimate goal of pharmacogenomics is to maximize efficacy and minimize adverse effects. Practice parameters have suggested a difference in pharmacological interventions between tic-associated and non-tic-associated OCD. For example, while selective serotonin reuptake inhibitors (SSRIs), metabolized by the CYP2D6 and CYP2C19 enzymes, are efficacious for treating OCD, their response appears to be less favorable for tic-associated OCD (81, 82). Conversely, alpha-2a agonists are proficient in addressing tics but not OCD (83). A meta-analysis found that there is significant inter-ethnic variation in the prevalence of phenotypes predicted by CYP2D6 and CYP2C19, which are clinically relevant for pharmacological treatment, and thus pre-emptive pharmacogenetic testing should be considered for patients undergoing therapy with drugs metabolized by these pathways (84). Following this, The U.S. Food & Drug Administration (FDA) then recommended therapeutic recommendations for some pharmacogenetic associations of SSRIs, such as citalopram, escitalopram, and fluvoxamine, as well as clomipramine, considering the patient’s metabolizing status (85). Therefore, pharmacogenomic-based prescribing remains an exciting field to empower psychiatrists and neurologists to deliver better, more personalized care to patients with comorbid TS and OCD.
Conclusion
Research spanning over the past 40 years has shown that the genetic landscape of TS is considerably more diverse and intricate than previously perceived. Significant strides in discerning the genetic underpinnings of TS and its concurrent disorders like OCD have been achieved by leveraging family-based studies, segregation analyses, linkage analysis, GWAS, and investigations into rare genetic variants. Existing data indicates a genetic overlap between TS and OCD. The susceptibility to TS-OCD may be shaped by the cumulative impact of several genes interplaying with distinct environmental determinants, such as psychosocial and infectious stressors. Larger international genetic, epigenetic, and pharmacogenomic studies with well-defined phenotypes in the current era of novel genetic discoveries, will have tremendous ramifications for the tailored diagnosis and therapeutic approaches to comorbid TS and OCD.
Author contributions
MK: Writing – original draft, Writing – review & editing, Conceptualization, Methodology, Funding acquisition. FA: Visualization, Writing – review & editing. YK: Writing – review & editing. FM: Funding acquisition, Resources, Supervision, Writing – original draft, Writing – review & editing.
Funding
The author(s) declare financial support was received for the research, authorship, and/or publication of this article. The publication of this article was funded by the Qatar National Library. MK, FA, and FM were supported by the College of Health and Life Sciences (CHLS), Hamad Bin Khalifa University (HBKU), Qatar Foundation. MK and YK were further supported by the Child and Adolescent Mental Health Service, Hamad Medical Corporation, Qatar. All figures were prepared using Biorender.
Conflict of interest
The authors declare that the research was conducted in the absence of any commercial or financial relationships that could be construed as a potential conflict of interest.
Publisher’s note
All claims expressed in this article are solely those of the authors and do not necessarily represent those of their affiliated organizations, or those of the publisher, the editors and the reviewers. Any product that may be evaluated in this article, or claim that may be made by its manufacturer, is not guaranteed or endorsed by the publisher.
References
1. Shapiro, A , Shapiro, E , Young, J , and Feinberg, T . Gilles de la Tourette syndrome, 2nd ed. - Psyc NET. (1988). Available at: https://psycnet.apa.org/record/1988-97189-000 (Accessed January 13, 2023).
2. Robertson, MM . The prevalence and epidemiology of Gilles de la Tourette syndrome. Part 1: the epidemiological and prevalence studies. J Psychosom Res. (2008) 65:461–72. doi: 10.1016/j.jpsychores.2008.03.006
3. Keen-Kim, D , and Freimer, NB . Genetics and epidemiology of Tourette syndrome. J Child Neurol. (2006) 21:665–71. doi: 10.1177/08830738060210081101
4. Yang, Z , Wu, H , Lee, PH , Tsetsos, F , Davis, LK , Yu, D, et al. Investigating shared genetic basis across Tourette syndrome and comorbid neurodevelopmental disorders along the impulsivity-compulsivity Spectrum. Biol Psychiatry. (2021) 90:317–27. doi: 10.1016/j.biopsych.2020.12.028
5. Paschou, P , Fernandez, TV , Sharp, F , Heiman, GA , and Hoekstra, PJ . Genetic susceptibility and neurotransmitters in Tourette syndrome. Int Rev Neurobiol. (2013) 112:155. doi: 10.1016/B978-0-12-411546-0.00006-8
6. Faraone, SV , Asherson, P , Banaschewski, T , Biederman, J , Buitelaar, JK , Ramos-Quiroga, JA, et al. Attention-deficit/hyperactivity disorder. Nat Rev Dis Prim. (2015) 1:15020. doi: 10.1038/nrdp.2015.20
7. Robertson, MM , Eapen, V , Singer, HS , Martino, D , Scharf, JM , Paschou, P, et al. Gilles de la Tourette syndrome. Nat Rev Dis Prim. (2017) 3:16097. doi: 10.1038/nrdp.2016.97
8. Mahjani, B , Bey, K , Boberg, J , and Burton, C . Genetics of obsessive-compulsive disorder. Psychol Med. (2021) 51:2247–59. doi: 10.1017/S0033291721001744
9. Malik, S , Khan, YS , Sahl, R , Elzamzamy, K , and Nazeer, A . Genetics of autism spectrum disorder: an update. Psychiatr Ann. (2019) 49:109–14. doi: 10.3928/00485713-20190212-01
10. Demontis, D , Walters, RK , Martin, J , Mattheisen, M , Als, TD , Agerbo, E, et al. Discovery of the first genome-wide significant risk loci for attention deficit/hyperactivity disorder. Nat Genet. (2019) 51:63–75. doi: 10.1038/s41588-018-0269-7
11. Autism Spectrum Disorders Working Group of The Psychiatric Genomics Consortium . Meta-analysis of GWAS of over 16,000 individuals with autism spectrum disorder highlights a novel locus at 10q24.32 and a significant overlap with schizophrenia. Mol Autism. (2017) 8:21. doi: 10.1186/s13229-017-0137-9
12. Grove, J , Ripke, S , Als, TD , Mattheisen, M , Walters, RK , Won, H, et al. Identification of common genetic risk variants for autism spectrum disorder. Nat Genet. (2019) 51:431–44. doi: 10.1038/s41588-019-0344-8
13. Yu, D , Sul, JH , Tsetsos, F , Nawaz, MS , Huang, AY , Zelaya, I, et al. Interrogating the genetic determinants of Tourette’s syndrome and other tiC disorders through genome-wide association studies. Am J Psychiatry. (2019) 176:217–27. doi: 10.1176/appi.ajp.2018.18070857
14. Arnold, PD , Askland, KD , Barlassina, C , Bellodi, L , Bienvenu, OJ , Black, D, et al. Revealing the complex genetic architecture of obsessive-compulsive disorder using meta-analysis. Mol Psychiatry. (2018) 23:1181–8. doi: 10.1038/mp.2017.154
15. Leckman, JF , Bloch, MH , Scahill, L , and King, RA . Tourette syndrome: The self under siege. J Child Neurol. (2006) 21:642–9. doi: 10.1177/08830738060210081001
16. Scharf, JM , Miller, LL , Gauvin, CA , Alabiso, J , Mathews, CA , and Ben-Shlomo, Y . Population prevalence of Tourette syndrome: a systematic review and meta-analysis. Mov Disord. (2015) 30:221–8. doi: 10.1002/mds.26089
17. Lewin, AB , Murphy, TK , Storch, EA , Conelea, CA , Woods, DW , Scahill, LD, et al. A phenomenological investigation of women with Tourette or other chronic tic disorders. Compr Psychiatry. (2012) 53:525–34. doi: 10.1016/j.comppsych.2011.07.004
18. Eapen, V , O’Neill, J , Gurling, HMD , and Robertson, MM . Sex of parent transmission effect in Tourette’s syndrome: evidence for earlier age at onset in maternally transmitted cases suggests a genomic imprinting effect. Neurology. (1997) 48:934–7. doi: 10.1212/WNL.48.4.934
19. Swedo, SE , Leonard, HL , Garvey, M , Mittleman, B , Allen, AJ , Perlmutter, S, et al. Pediatric autoimmune neuropsychiatric disorders associated with streptococcal infections: clinical description of the first 50 cases. Am J Psychiatry. (1998) 155:264–71. doi: 10.1176/ajp.155.2.264
20. Hyde, TM , Aaronson, BA , Randolph, C , Ricker, KC , and Weinberger, DR . Relationship of birth weight to the phenotypic expression of gilles de la Tourette’s syndrome in monozygotic twins. Neurology. (1992) 42:652–8. doi: 10.1212/WNL.42.3.652
21. Walkup, JT , Leckman, JF , Price, RA , Hardin, M , Ort, SI , and Cohen, DJ . The relationship between obsessive-compulsive disorder and Tourette’s syndrome: a twin study. Psychopharmacol Bull. (1988) 24:375–9.
22. Price, RA , Kidd, KK , Cohen, DJ , Pauls, DL , and Leckman, JF . A twin study of Tourette syndrome. Arch Gen Psychiatry. (1985) 42:815–20. doi: 10.1001/archpsyc.1985.01790310077011
23. Pauls, DL , and Leckman, JF . The inheritance of Gilles de la Tourette’s syndrome and associated Behaviors. N Engl J Med. (1986) 315:993–7. doi: 10.1056/NEJM198610163151604
24. Baron, M , Shapiro, E , Shapiro, A , and Rainer, JD . Genetic analysis of Tourette syndrome suggesting major gene effect. Am J Hum Genet. (1981) 33:767–75.
25. Curtis, D , Robertson, MM , and Gurling, HMD . Autosomal dominant gene transmission in a large kindred with Gilles de la Tourette syndrome. Br J Psychiatry. (1992) 160:845–9. doi: 10.1192/bjp.160.6.845
26. Walkup, JT , Labuda, MC , Singer, HS , Brown, J , Riddle, MA , and Hurko, O . Family study and segregation analysis of Tourette syndrome: evidence for a mixed model of inheritance. Am J Hum Genet. (1996) 59:684–93.
27. Seuchter, SA , Hebebrand, J , Klug, B , Knapp, M , Lehmkuhl, G , Poustka, F, et al. Complex segregation analysis of families ascertained through Gilles de la Tourette syndrome. Genet Epidemiol. (2000) 18:33–47. doi: 10.1002/(SICI)1098-2272(200001)18:1<33::AID-GEPI3>3.0.CO;2-2
28. Clark, LN , and Louis, ED . Essential tremor. Handb Clin Neurol. (2018) 147:229. doi: 10.1016/B978-0-444-63233-3.00015-4
29. Sandford, E , and Burmeister, M . Genes and genetic testing in hereditary ataxias. Genes (Basel). (2014) 5:586. doi: 10.3390/genes5030586
30. Loy, CT , Schofield, PR , Turner, AM , and Kwok, JBJ . Genetics of dementia. Lancet. (2014) 383:828–40. doi: 10.1016/S0140-6736(13)60630-3
31. Khoodoruth, MAS , Ouanes, S , and Khan, YS . A systematic review of the use of atomoxetine for management of comorbid anxiety disorders in children and adolescents with attention-deficit hyperactivity disorder. Res Dev Disabil. (2022) 128:104275. doi: 10.1016/j.ridd.2022.104275
32. Eddy, CM , Rizzo, R , and Cavanna, AE . Neuropsychological aspects of Tourette syndrome: a review. J Psychosom Res. (2009) 67:503–13. doi: 10.1016/j.jpsychores.2009.08.001
33. Eapen, V , and Robertson, MM . Gilles de la Tourette syndrome and attention deficit hyperactivity disorder: no evidence for a genetic relationship. Neuropsychiatry Neuropsychol Behav Neurol. (1996) 9:192–6.
34. Grados, MA , and Mathews, CA . Latent class analysis of Gilles de la Tourette syndrome using comorbidities: clinical and genetic implications. Biol Psychiatry. (2008) 64:219–25. doi: 10.1016/j.biopsych.2008.01.019
35. Gunther, J , Tian, Y , Stamova, B , Lit, L , Corbett, B , Ander, B, et al. Catecholamine-related gene expression in blood correlates with tic severity in tourette syndrome. Psychiatry Res. (2012) 200:593–601. doi: 10.1016/j.psychres.2012.04.034
36. Baron-Cohen, S , Scahill, VL , Izaguirre, J , Hornsey, H , and Robertson, MM . The prevalence of Gilles de la Tourette syndrome in children and adolescents with autism: a large scale study. Psychol Med. (1999) 29:1151–9. doi: 10.1017/S003329179900896X
37. Fernandez, TV , Sanders, SJ , Yurkiewicz, IR , Ercan-Sencicek, AG , Kim, YS , Fishman, DO, et al. Rare copy number variants in tourette syndrome disrupt genes in histaminergic pathways and overlap with autism. Biol Psychiatry. (2012) 71:392–402. doi: 10.1016/j.biopsych.2011.09.034
38. Carias, KV , and Wevrick, R . Clinical and genetic analysis of children with a dual diagnosis of Tourette syndrome and autism spectrum disorder. J Psychiatr Res. (2019) 111:145–53. doi: 10.1016/j.jpsychires.2019.01.023
39. Chou, IC , Lin, HC , Lin, CC , Sung, FC , and Kao, CH . Tourette syndrome and risk of depression: a population-based cohort study in Taiwan. J Dev Behav Pediatr. (2013) 34:181–5. doi: 10.1097/DBP.0b013e3182829f2b
40. Comings, DE , and Comings, BG . A controlled family history study of Tourette’s syndrome, III: affective and other disorders. J Clin Psychiatry. (1990) 51:288–91.
41. Pauls, DL , Leckman, JF , and Cohen, DJ . Evidence against a genetic relationship between Tourette’s syndrome and anxiety, depression, panic and phobic disorders. Br J Psychiatry. (1994) 164:215–21. doi: 10.1192/bjp.164.2.215
42. Abelson, JF , Kwan, KY , O’Roak, BJ , Baek, DY , Stillman, AA , Morgan, TM, et al. Medicine: sequence variants in SLITRK1 are associated with Tourette’s syndrome. Science (80. (2005) 310:317–20. doi: 10.1126/science.1116502
43. Verkerk, AJMH , Mathews, CA , Joosse, M , Eussen, BHJ , Heutink, P , and Oostra, BA . CNTNAP2 is disrupted in a family with Gilles de la Tourette syndrome and obsessive compulsive disorder. Genomics. (2003) 82:1–9. doi: 10.1016/S0888-7543(03)00097-1
44. Ercan-Sencicek, AG , Stillman, AA , Ghosh, AK , Bilguvar, K , O’Roak, BJ , Mason, CE, et al. L-histidine decarboxylase and Tourette’s syndrome. N Engl J Med. (2010) 362:1901–8. doi: 10.1056/NEJMoa0907006
45. Song, M , Mathews, CA , Stewart, SE , Shmelkov, SV , Mezey, JG , Rodriguez-Flores, JL, et al. Rare synaptogenesis-impairing mutations in SLITRK5 are associated with obsessive compulsive disorder. PLoS One. (2017) 12:e0169994. doi: 10.1371/journal.pone.0169994
46. Cappi, C , Oliphant, ME , Péter, Z , Zai, G , Conceição do Rosário, M , CAW, S, et al. De novo damaging DNA coding mutations are associated with obsessive-compulsive disorder and overlap with Tourette’s disorder and autism. Biol Psychiatry. (2020) 87:1035–44. doi: 10.1016/j.biopsych.2019.09.029
47. Kessler, RC , Berglund, P , Demler, O , Jin, R , Merikangas, KR , and Walters, EE . Lifetime prevalence and age-of-onset distributions of DSM-IV disorders in the National Comorbidity Survey Replication. Arch Gen Psychiatry. (2005) 62:593–602. doi: 10.1001/archpsyc.62.6.593
48. Hebebrand, J , Klug, B , Fimmers, R , Seuchter, SA , Wettke-Schäfer, R , Deget, F, et al. Rates for tic disorders and obsessive compulsive symptomatology in families of children and adolescents with Gilles de la Tourette syndrome. J Psychiatr Res. (1997) 31:519–30. doi: 10.1016/S0022-3956(97)00028-9
49. Pauls, DL , Raymond, CL , Stevenson, JM , and Leckman, JF . A family study of Gilles de la Tourette syndrome. Am J Hum Genet. (1991) 48:154–63.
50. Do Rosario-Campos, MC , Leckman, JF , Curi, M , Quatrano, S , Katsovitch, L , Miguel, EC, et al. A family study of early-onset obsessive-compulsive disorder. Am J Med Genet B Neuropsychiatr Genet. (2005) 136B:92–7. doi: 10.1002/ajmg.b.30149
51. Nestadt, G , Samuels, J , Riddle, M , Bienvenu, OJ , Liang, KY , Labuda, M, et al. A family study of obsessive-compulsive disorder. Arch Gen Psychiatry. (2000) 57:358–63. doi: 10.1001/archpsyc.57.4.358
52. Pauls, DL , Alsobrook, JP , Goodman, W , Rasmussen, S , and Leckman, JF . A family study of obsessive-compulsive disorder. Am J Psychiatry. (1995) 152:76–84. doi: 10.1176/ajp.152.1.76
53. Woods, DW , Piacentini, J , Himle, MB , and Chang, S . Premonitory urge for tics scale (PUTS): initial psychometric results and examination of the premonitory urge phenomenon in youths with tic disorders. J Dev Behav Pediatr. (2005) 26:397–403. doi: 10.1097/00004703-200512000-00001
54. Kwak, C , Dat Vuong, K , and Jankovic, J . Premonitory sensory phenomenon in Tourette’s syndrome. Mov Disord. (2003) 18:1530–3. doi: 10.1002/mds.10618
55. Haas, HL , Sergeeva, OA , and Selbach, O . Histamine in the nervous system. Physiol Rev. (2008) 88:1183–241. doi: 10.1152/physrev.00043.2007
56. Panula, P , Chazot, PL , Cowart, M , Gutzmer, R , Leurs, R , Liu, WLS, et al. International Union of Basic and Clinical Pharmacology. XCVIII Histamine Receptors Pharmacol Rev. (2015) 67:601. doi: 10.1124/pr.114.010249
57. Ferrada, C , Ferré, S , Casadó, V , Cortés, A , Justinova, Z , Barnes, C, et al. Interactions between histamine H3 and dopamine D2 receptors and the implications for striatal function. Neuropharmacology. (2008) 55:190–7. doi: 10.1016/j.neuropharm.2008.05.008
58. Gilbert, DL , and Jankovic, J . Pharmacological treatment of Tourette syndrome. J Obsessive Compuls Relat Disord. (2014) 3:407–14. doi: 10.1016/j.jocrd.2014.04.006
59. Zhang, XY , Peng, SY , Shen, LP , Zhuang, QX , Li, B , Xie, ST, et al. Targeting presynaptic H3 heteroreceptor in nucleus accumbens to improve anxiety and obsessive-compulsive-like behaviors. Proc Natl Acad Sci U S A. (2020) 117:32155–64. doi: 10.1073/pnas.2008456117
60. Alkuraya, FS . Discovery of rare homozygous mutations from studies of consanguineous pedigrees. Curr Protoc Hum Genet. (2012) 75:6.12.1–6.12.13. doi: 10.1002/0471142905.HG0612S75
61. Motlagh, MG , Seddigh, A , Dashti, B , Leckman, JF , and Alaghband-Rad, J . Consanguineous Iranian kindreds with severe Tourette syndrome. Mov Disord. (2008) 23:2079–83. doi: 10.1002/mds.22261
62. State, MW , Greally, JM , Cuker, A , Bowers, PN , Henegariu, O , Morgan, TM, et al. Epigenetic abnormalities associated with a chromosome 18 (q21-q22) inversion and a Gilles de la Tourette syndrome phenotype. Proc Natl Acad Sci U S A. (2003) 100:4684–9. doi: 10.1073/pnas.0730775100
63. Petek, E , Windpassinger, C , Vincent, JB , Cheung, J , Boright, AP , Scherer, SW, et al. Disruption of a novel gene (IMMP2L) by a breakpoint in 7q31 associated with Tourette syndrome. Am J Hum Genet. (2001) 68:848–58. doi: 10.1086/319523
64. Cuker, A , State, MW , King, RA , Davis, N , and Ward, DC . Candidate locus for Gilles de la Tourette syndrome/obsessive compulsive disorder/chronic tic disorder at 18q22. Am J Med Genet A. (2004) 130A:37–9. doi: 10.1002/ajmg.a.30066
65. Boghosian-Sell, L , Comings, DE , and Overhauser, J . Tourette syndrome in a pedigree with a 7; 18 translocation: identification of a YAC spanning the translocation breakpoint at 18q22.3. Am J Hum Genet. (1996) 59:999–1005.
66. Poliak, S , Gollan, L , Martinez, R , Custer, A , Einheber, S , Salzer, JL, et al. Caspr2, a new member of the neurexin superfamily, is localized at the juxtaparanodes of myelinated axons and associates with K+ channels. Neuron. (1999) 24:1037–47. doi: 10.1016/S0896-6273(00)81049-1
67. McGrath, LM , Yu, D , Marshall, C , Davis, LK , Thiruvahindrapuram, B , Li, B, et al. Copy number variation in obsessive-compulsive disorder and tourette syndrome: a cross-disorder study. J Am Acad Child Adolesc Psychiatry. (2014) 53:910–9. doi: 10.1016/j.jaac.2014.04.022
68. Robertson, MM , Shelley, BP , Dalwai, S , Brewer, C , and Critchley, HD . A patient with both Gilles de la Tourette’s syndrome and chromosome 22q11 deletion syndrome: clue to the genetics of Gilles de la Tourette’s syndrome? J Psychosom Res. (2006) 61:365–8. doi: 10.1016/j.jpsychores.2006.06.011
69. Lee, PH , Anttila, V , Won, H , Feng, YCA , Rosenthal, J , Zhu, Z, et al. Genomic relationships, novel loci, and pleiotropic mechanisms across eight psychiatric disorders. Cells. (2019) 179:1469. doi: 10.1016/j.cell.2019.11.020
71. Hoekstra, PJ , Anderson, GM , Limburg, PC , Korf, J , Kallenberg, CGM , and Minderaa, RB . Neurobiology and neuroimmunology of Tourette’s syndrome: an update. Cell Mol Life Sci. (2004) 61:886–98. doi: 10.1007/s00018-003-3320-4
72. Lin, H , Katsovich, L , Ghebremichael, M , Findley, DB , Grantz, H , Lombroso, PJ, et al. Psychosocial stress predicts future symptom severities in children and adolescents with Tourette syndrome and/or obsessive-compulsive disorder. J Child Psychol Psychiatry Allied Discip. (2007) 48:157–66. doi: 10.1111/j.1469-7610.2006.01687.x
73. Lin, H , Williams, KA , Katsovich, L , Findley, DB , Grantz, H , Lombroso, PJ, et al. Streptococcal upper respiratory tract infections and psychosocial stress predict future tic and obsessive-compulsive symptom severity in children and adolescents with Tourette syndrome and obsessive-compulsive disorder. Biol Psychiatry. (2010) 67:684–91. doi: 10.1016/j.biopsych.2009.08.020
74. Buse, J , Kirschbaum, C , Leckman, JF , Münchau, A , and Roessner, V . The modulating role of stress in the onset and course of Tourette’s syndrome: a review. Behav Modif. (2014) 38:184–216. doi: 10.1177/0145445514522056
75. Adams, TG , Kelmendi, B , Brake, CA , Gruner, P , Badour, CL , and Pittenger, C . The role of stress in the pathogenesis and maintenance of obsessive-compulsive disorder. Chronic Stress. (2018) 2. doi: 10.1177/2470547018758043
76. Leckman, JF , Dolnansky, ES , Hardin, MT , Clubb, M , Walkup, JT , Stevenson, J, et al. Perinatal factors in the expression of Tourette’s syndrome: an exploratory study. J Am Acad Child Adolesc Psychiatry. (1990) 29:220–6. doi: 10.1097/00004583-199003000-00010
77. Corbett, BA , Mendoza, SP , Baym, CL , Bunge, SA , and Levine, S . Examining cortisol rhythmicity and responsivity to stress in children with Tourette syndrome. Psychoneuroendocrinology. (2008) 33:810–20. doi: 10.1016/j.psyneuen.2008.03.014
78. Chappell, P , Riddle, M , Anderson, G , Scahill, L , Hardin, M , Walker, D, et al. Enhanced stress responsivity of tourette syndrome patients undergoing lumbar puncture. Biol Psychiatry. (1994) 36:35–43. doi: 10.1016/0006-3223(94)90060-4
79. Chappell, P , Leckman, J , Goodman, W , Bissette, G , Pauls, D , Anderson, G, et al. Elevated cerebrospinal fluid corticotropin-releasing factor in Tourette’s syndrome: comparison to obsessive compulsive disorder and normal controls. Biol Psychiatry. (1996) 39:776–83. doi: 10.1016/0006-3223(95)00221-9
80. Meaney, MJ , Szyf, M , and Seckl, JR . Epigenetic mechanisms of perinatal programming of hypothalamic-pituitary-adrenal function and health. Trends Mol Med. (2007) 13:269–77. doi: 10.1016/j.molmed.2007.05.003
81. Pringsheim, T , Okun, MS , Müller-Vahl, K , Martino, D , Jankovic, J , Cavanna, AE, et al. Practice guideline recommendations summary: treatment of tics in people with Tourette syndrome and chronic tic disorders. Neurology. (2019) 92:896–906. doi: 10.1212/WNL.0000000000007466
82. Federal Drug Administration . Table of pharmacogenomic biomarkers in drug Labeling|FDA. United States Government (2019)1–53. Available at: https://www.fda.gov/drugs/science-and-research-drugs/table-pharmacogenomic-biomarkers-drug-labeling (Accessed March 11, 2023).
83. Roessner, V , Eichele, H , Stern, JS , Skov, L , Rizzo, R , Debes, NM, et al. European clinical guidelines for Tourette syndrome and other tic disorders—Version 2.0. Part III: pharmacological treatment. Eur Child Adolesc Psychiatry. (2022) 31:425–41. doi: 10.1007/s00787-021-01899-z
84. Koopmans, AB , Braakman, MH , Vinkers, DJ , Hoek, HW , and van Harten, PN . Meta-analysis of probability estimates of worldwide variation of CYP2D6 and CYP2C19. Transl Psychiatry. (2021) 11:1–16. doi: 10.1038/s41398-020-01129-1
85. Federal Drug Administration . Table of pharmacogenetic associations |FDA. Food Drug Administation (2022)1–2. Available at: https://www.fda.gov/medical-devices/precision-medicine/table-pharmacogenetic-associations (Accessed March 11, 2023).
Keywords: Tourette sydrome, obsessive – compulsive disorder, shared genetic factors, neurogenetics, neuropsychiatic genetics, genetics, epigenetics, genomics
Citation: Khoodoruth MAS, Ahammad F, Khan YS and Mohammad F (2023) The shared genetic risk factors between Tourette syndrome and obsessive-compulsive disorder. Front. Neurol. 14:1283572. doi: 10.3389/fneur.2023.1283572
Edited by:
Félix Javier Jiménez-Jiménez, Hospital Universitario del Sureste, SpainReviewed by:
Alfredo Bellon, Penn State Milton S. Hershey Medical Center, United StatesPedro J. Garcia-Ruiz, University Hospital Fundación Jiménez Díaz, Spain
Copyright © 2023 Khoodoruth, Ahammad, Khan and Mohammad. This is an open-access article distributed under the terms of the Creative Commons Attribution License (CC BY). The use, distribution or reproduction in other forums is permitted, provided the original author(s) and the copyright owner(s) are credited and that the original publication in this journal is cited, in accordance with accepted academic practice. No use, distribution or reproduction is permitted which does not comply with these terms.
*Correspondence: Farhan Mohammad, bW9oYW1tYWRmYXJoYW5AaGJrdS5lZHUucWE=