- 1School of Integrated Traditional Chinese and Western Medicine, Binzhou Medical University, Yantai, China
- 2College of Pharmacy, Shihezi University, Shihezi, China
Cancer poses a serious threat to human health and overall well-being. Conventional cancer treatments predominantly encompass surgical procedures and radiotherapy. Nevertheless, the substantial side effects and the emergence of drug resistance in patients significantly diminish their quality of life and overall prognosis. There is an acute need for innovative, efficient therapeutic agents to address these challenges. Plant-based herbal medicines and their derived compounds offer promising potential for cancer research and treatment due to their numerous advantages. Solanum nigrum (S. nigrum), a traditional Chinese medicine, finds extensive use in clinical settings. The steroidal compounds within S. nigrum, particularly steroidal alkaloids, exhibit robust antitumor properties either independently or when combined with other drugs. Many researchers have delved into unraveling the antitumor mechanisms of the active components present in S. nigrum, yielding notable progress. This literature review provides a comprehensive analysis of the research advancements concerning the active constituents of S. nigrum. Furthermore, it outlines the action mechanisms of select monomeric anticancer ingredients. Overall, the insights derived from this review offer a new perspective on the development of clinical anticancer drugs.
1 Introduction
Cancer remains a leading cause of mortality worldwide (1). There were an estimated 18.1 million new cancer cases and 9.9 million cancer deaths globally in 2020 (2). According to Cancer Facts & Figures 2023 released by American Cancer Society, over 1.9 million new cancer cases are expected to be diagnosed, and approximately 609,820 deaths from cancer are expected in the US in 2023 (3). Furthermore, global projections indicate that the cancer burden is expected to increase by 50% in 2040 compared with that in 2020, primarily due to an aging population (4). Fortunately, there is promising hope in the battle against cancer, with natural medicines derived from plants and microrganisms offering new avenues of exploration. Solanum nigrum (S. nigrum), a frequently used herb with antitumor potential in clinical practice, has garnered attention. Within S. nigrum, steroidal alkaloids, particularly steroids, represent the crucial chemical constituents responsible for the antitumor properties of S. nigrum (5).
Cancer cells are characterized by distinct traits, including sustaining proliferative signals, evading growth inhibitors, resisting programmed cell death, enabling replicative immortality, inducing angiogenesis, promoting metastasis and invasion, remodeling cellular metabolism, and acquiring the ability to evade immune surveillance (6). Patients with primary tumors typically undergo treatments such as surgery, radiotherapy, and chemotherapy (7). Chemotherapeutic agents are cytotoxic drugs commonly used in treating cancer. These drugs target rapidly growing and proliferating cells and promote some of the key factors that impair mitosis and enhance apoptosis during cell division (8). However, chemotherapeutic agents lead to poor prognoses and adverse side effects (9). Common side effects include bone marrow suppression, alopecia (hair loss), fatigue, neuropathy, dermatological issues, and gastrointestinal disorders (10). Traditional Chinese medicine is gradually gaining recognition for its relatively low side effects and antitumor properties.
S. nigrum, the largest genus in the Solanaceae family, is an annual herb widely distributed in China (11). It can be used for medicinal purposes, either in its fresh or in its dried form. S. nigrum is known for its diverse therapeutic effects, including heat-clearing and toxin-removing properties, reduction of swelling and lumps, anti-inflammatory and diuretic effects, and helping produce saliva and slake thirst (12). In the Ayurvedic tradition of India, S. nigrum has been used to treat intestinal diseases, ulcers, diarrhea, and skin conditions (13). Modern pharmacological studies have provided evidence of S. nigrum’s antitumor, anti-inflammatory, antioxidant, and antihypertensive properties (14). Furthermore, various active molecules extracted from S. nigrum exhibit anticancer effects.
We present a review of the research data from the past decade obtained from PubMed and Web of Science. We conducted a thorough analysis to identify the individual active constituents within S. nigrum. Additionally, we explored the antitumor properties and underlying mechanisms of S. nigrum in combination with other drugs against different types of tumors.
2 Chemical composition of S. nigrum
As of 2022, S. nigrum has been found to contain a total of 188 chemicals, encompassing steroidal alkaloids, steroidal saponins, glycoproteins, organic acids, lignins, polysaccharides, and polyphenols (15). Among these, steroidal alkaloids and steroidal saponins are the primary active components responsible for its antitumor properties. The steroidal alkaloids in S. nigrum primarily consist of three glycosides: solanines, solasonine, and solamargine (SM). These glycosides are primarily present in immature fruits and have been extensively studied in the field of natural products (16).
Predominantly, the alkaloids found in the entire S. nigrum herb are steroidal alkaloids, all sharing the fundamental steroidal skeleton structure of cyclopentanoperhydrophenanthrene. This structural motif is currently the subject of widespread research as a potential antitumor active ingredient (17). The fruits of S. nigrum are particularly rich in steroidal alkaloids, with the highest content found in the unripe green fruits. As the fruits ripen, their alkaloid composition undergoes changes, leading to a reduction in alkaloid content. As early as 1982, Japanese researchers employed ethanol to isolate two steroidal alkaloids, solasonine and SM, from unripe fruits. These glycosides are linked by glycosidic bonds and share the same glycoside, solasodine (18). Since then, numerous steroidal alkaloids have been identified in S. nigrum fruits, including compounds such as 7α-OH-kekasianine, 7α-OH SM, 7α-OH solasonine, 12β,27-dihydroxy solanine-3-O-β-D-glucopyranoside, and 27-solasonine-3-O-β-D-glucopyranosyl-(1→4)-α-L-rhamnopyranoside-(1→2)-[α-L-rhamnopyranoside-(1→4)]-β-D-glucopyranoside (19, 20).
3 Pharmacological effects of S. nigrum
Modern pharmacological studies have revealed that S. nigrum extracts obtained using different solvents yield distinct pharmacological effects. For example, the aqueous extract of S. nigrum demonstrates the ability to mitigate angiotensin-II-induced cardiac hypertrophy and improve cardiac health (21). Furthermore, the aqueous extract can inhibit the growth of breast, ovarian, and liver cancer cells by influencing the expression of numerous tumor-related genes (22–30). Additionally, it augments the tumor-inhibitory efficacy of cisplatin, adriamycin, and docetaxel on human ovarian cancer cells (26, 27). Nirmal et al. have demonstrated the antihistaminic and antiallergic effects of the petroleum ether extract of S. nigrum fruit, highlighting its potential as a therapeutical agent for asthma (14).
The ethanolic extract of S. nigrum has exhibited in vitro inhibitory effects on melanoma cells. In in vivo experiments, a 1% aqueous extract of S. nigrum significantly reduced tumor mass in tumor-bearing mice, achieving a tumor inhibition rate exceeding 50%. Additionally, it effectively inhibited lung metastasis in melanoma (31).
Research on the antitumor effects of individual compounds within S. nigrum has predominantly focused on solanine, solasonine, SM, and solasodine. Furthermore, α-solanine has displayed antitumor effects on non–small cell lung cancer (NSCLC) (32). Pharmacological studies have demonstrated that SM exhibits inhibitory effects on gastric, hepatocellular, and lung cancers (33–35). Notably, uttroside B, a saponin isolated from S. nigrum, has demonstrated superior anti–liver cancer efficacy over sorafenib, a first-line anticancer drug and antitumor angiogenesis targeted drug (36). Furthermore, uttroside B exhibits fewer toxic effects on normal cells (37). Solasonine has been shown to inhibit pancreatic cancer cells (38). S. nigrum has a long history of use in cancer treatment, with its extracts displaying significant antitumor pharmacological activities. Consequently, further research into the isolation and purification of the antitumor active components of S. nigrum holds great significance.
In this study, we have reviewed research conducted over the past two decades on the active components of S. nigrum with antitumor properties. Additionally, we have explored the mechanisms underlying the antitumor actions of its four key antitumor monomer active components. The molecular structure of these four components was shown in Figure 1.
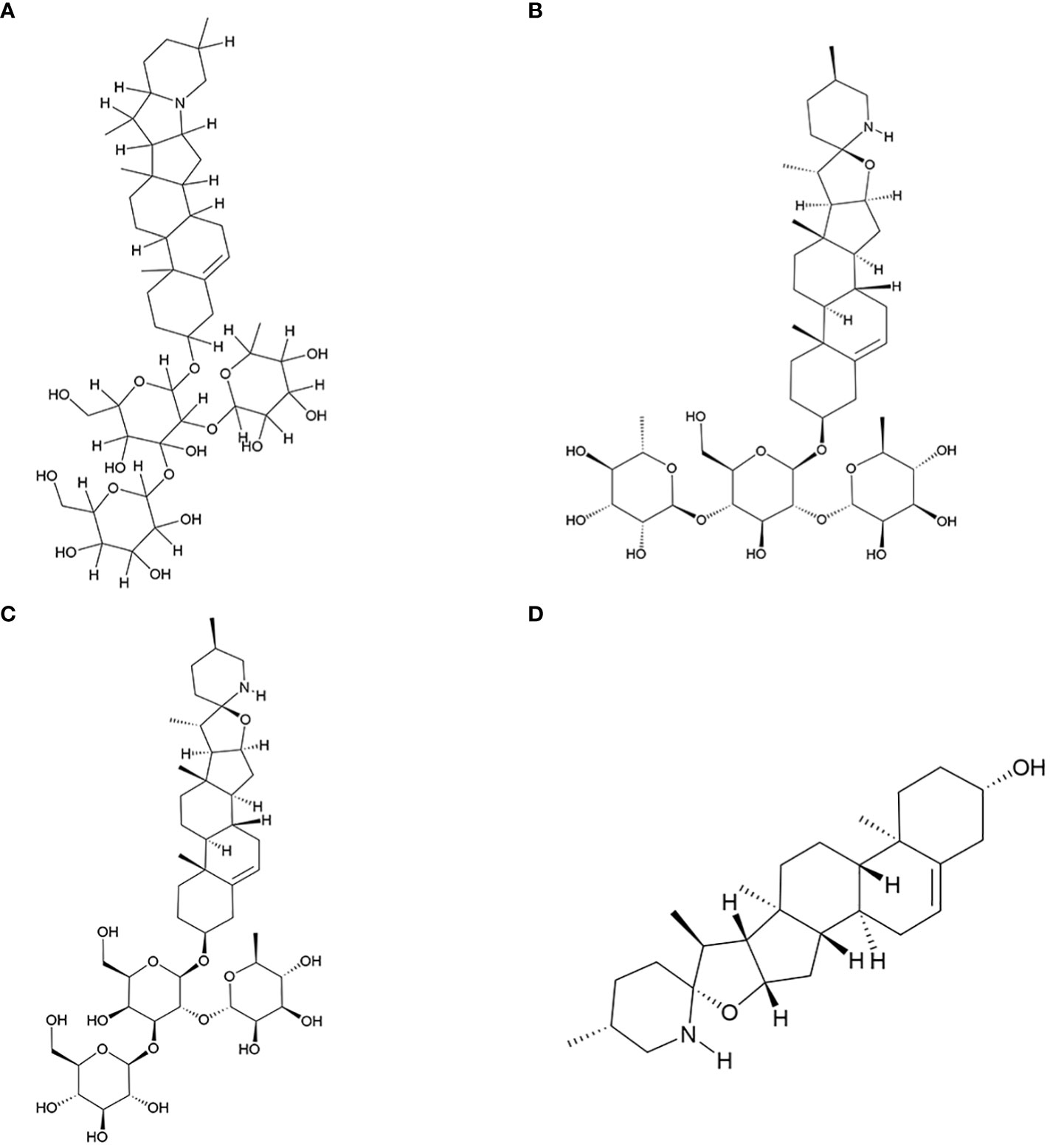
Figure 1 Key antitumor monomer of S. nigrum. (A) Solanine; (B) Solamargine; (C) Solasonine; (D) Solasodine.
4 Antitumor active monomers from S. nigrum
4.1 Solanine
Solanine, also referred to as α-solanine, possesses a range of beneficial properties, including antidiabetic, antiallergic, anti-inflammatory, antiviral, antibacterial, antiprotozoan, and antifungal activities. Its anticancer potential is evident through its ability to induce apoptosis and hinder cell growth, migration, and invasion both in vitro and in vivo (39). Notably, its therapeutic efficacy against liver cancer has been thoroughly investigated.
4.1.1 Anti–liver cancer activity of solanine
Matrix metalloproteinases (MMPs) play a pivotal role in tumor growth and metastasis. Solanine effectively inhibits MMPs, thereby impeding tumor development and stemness. This action involves the downregulation of E-cadherin and upregulation of N-cadherin, resulting in reduced tumor invasiveness and proliferation of HepG2 cells (40). miR-21, a well-established cancer marker, exhibits high expression levels in invasive cancer cells. Remarkably, solanine treatment significantly reduces miR-21 expression, subsequently inhibiting the migration and invasion of liver cancer cells (40). Additionally, solanine intervenes in the immune escape mediated by liver cancer Treg cells through the transforming growth factor (TGF)-β/Smad signaling pathway, ultimately enhancing the immune response (41). In synergy with cisplatin, solanine induces apoptosis, intensifies cell cycle arrest in liver cancer cells, enhances caspase-3 and caspase-7 activity, and attenuates the expression of Bcl-2 and survivin, thereby promoting apoptosis and inhibiting cancer cell growth (42). Furthermore, solanine fosters the expression of ASK1 and TBP-2 and augments their kinase activities by inducing reactive oxygen species (ROS) production in HepG2 cells, ultimately driving apoptosis in these cells (43).
4.1.2 Anti–esophageal cancer activity of solanine
In a study by Wu et al., solanine effectively suppresses the proliferation, migration, and invasion of esophageal cancer cells (EC9706 and Eca109) in vitro in a dose- and time-dependent manner. Moreover, solanine triggers apoptosis by activating caspase-3 and caspase-7 and suppresses MMP-2 and MMP-9 expression in a dose-dependent manner. The administration of solanine significantly elevates E-cadherin expression levels, suggesting its ability to inhibit metastasis by influencing cellular protein hydrolysis activation and adhesion capabilities. Solanine treatment also reduces the expression of the apoptosis-related protein Bcl-2, enhances Bax expression, and promotes apoptosis, thus emerging as a potential agent for esophageal cancer prevention and treatment (39). Additionally, solanine downregulates survivin expression by upregulating miR-138 expression, consequently enhancing the radiosensitivity of EC cells and positioning solanine as a promising radiosensitizer (44).
4.1.3 Anti–breast cancer activity of solanine
Solanine treatment is further characterized by increased Bax expression, decreased Bcl-2 levels, and diminished platelet–endothelial cell adhesion molecule (CD31) expression in cancer cells. These actions contribute significantly to anti-angiogenesis, leading to the reduction or disappearance of mammary tumors and the inhibition of tumor progression in mice (45). The anticancer effects of solanine may involve the inhibition of the NF-κB pathway (46). Subsequently, a specialized nanoparticle (DNS) with high solubility capacity was developed. DNS treatment upregulated Bcl-2 expression while downregulating Bax, MMP-2, MMP-9, mTOR, and Akt levels in cancer cells. These findings underscore solanine’s antiangiogenic effect on cancer cells through the activation of the PI3K-Akt pathway (47). Hence, solanine emerges as a promising candidate drug for breast cancer treatment.
4.1.4 Other antitumor activities of solanine
S100P overexpression is known to play a role in promoting tumorigenesis and metastasis in various cancer models (48). S100P is overexpressed in colorectal cancer cells. However, solanine counters this effect by inhibiting S100P expression. Solanine-induced inhibition results in cell cycle arrest at the G0/G1 phase, increased production of ROS, and apoptosis induction in tumor cells. Additionally, solanine has demonstrated its ability to suppress cancer cell proliferation, migration, and stemness by reducing the activity and expression of MMP-2 and MMP-9 (49, 50). Moreover, solanine has been found to elevate ROS levels in lung cancer A549, prostate cancer DU145 cells, and squamous carcinoma KB cells. It activates cellular autophagy by downregulating Akt/mTOR expression (51).
The above-mentioned studies collectively highlight solanine’s inhibitory effect on various human cancer cells, positioning it as a potential effective antitumor drug in the future. This anticancer activity is attributed to solanine’s ability to modulate different cellular targets, which vary among different cancer cells and at different concentrations. The above antitumor mechanism of solanine was shown in Figure 2.
4.2 Solamargine
SM is a small-molecule steroidal alkaloid isolated from nightshade plants. Its fundamental chemical structure comprises one glycosidic element linked to three sugar groups through a glycosidic bond, with a molecular formula of C45H73NO15 (52). SM is widely acknowledged as a potent active ingredient in antitumor therapy. SM exhibits robust anticancer activity against various types of cancer, including gastric, lung, liver, and prostate cancers; melanoma; cholangiocarcinoma; and hypopharyngeal squamous cell carcinoma.
4.2.1 Anti–gastric cancer activity of solamargine
SM exerts significant inhibitory effects on gastric cancer cells (33, 53). It induces the expression of caspase-7 and disrupts the G2/M cell cycle to promote cancer cell apoptosis. Furthermore, it upregulates the expression of lncNEAT1_2 and lncPINT by inhibiting the Erk1/2 MAPK signaling pathway, leading to suppressed viability of gastric cancer cells. In vivo studies have demonstrated a marked increase in early apoptotic cells and a significant reduction in tumor volume following SM treatment, establishing SM’s potential as a therapeutic agent for gastric cancer (33).
4.2.2 Anti–lung cancer activity of solamargine
In lung cancer, SM acts by inhibiting the expression of SP1 and p65 proteins through the suppression of the PI3K-Akt signaling pathway. This leads to a dose-dependent suppression of the growth of H299 and A549 human lung cancer cells (35). Additionally, SM inhibits DNMT1 protein expression by increasing Erk1/2 phosphorylation, subsequently suppressing c-Jun protein expression and tumor proliferation (54).
HOX transcript antisense RNA (HOTAIR) is often overexpressed in lung cancer and correlates with metastasis and poor prognosis. It promotes the proliferation, survival, invasion, metastasis, and drug resistance of lung cancer cells (55). SM inhibits the proliferation and induces apoptosis of NSCLC cells through inhibition of long-stranded non-coding RNA (HOTAIR). Additionally, SM promotes the expression of miR-214-3p while inhibiting the expression of its downstream target PDPK1. The interplay between HOTAIR and miR-214-3p inhibits NSCLC cell growth (56). Furthermore, the combination of SM and cisplatin exhibits synergistic effects in inhibiting cisplatin-resistant lung cancer cell lines (57), enhancing the antitumor effects of gefitinib and erlotinib (58).
4.2.3 Anti–liver cancer activity of solamargine
Higher expression of Ki67 and PCNA in cells typically indicates increased cell proliferation (59). Treatment of the HepG2 cell line with SM led to reduced expression of Ki67, PCNA, and Bcl-2 proteins while elevating the expression levels of Bax, caspase-3, and caspase-9. As a result, SM is believed to inhibit liver cancer cell proliferation and induce apoptosis by activating the Bcl-2/Bax and caspase signaling pathways (60). Moreover, at high concentrations, SM may effectively treat liver cancer by modulating the LIF/miR-192-5p/CYR61/Akt axis, inducing autophagy and apoptosis. At low concentrations, SM can repolarize M2 macrophages into an M1-like phenotype through LIF/p-Stat3 signaling, inhibiting epithelial–mesenchymal transition (EMT) in hepatoma cells, thereby reducing the invasion and migration abilities of hepatoblastoma cells (34, 61).
MUC1 plays a pivotal role in the transcriptional regulation of genes associated with tumor invasion, metastasis, angiogenesis, proliferation, apoptosis, drug resistance, inflammation, and immune regulation (62–69). According to Tang et al., SM can hinder the growth of hepatocellular carcinoma (HCC) through the HOTTIP-TUG1/miR-4726-5p/MUC1 signaling pathway. Additionally, the combination of SM and sorafenib synergistically inhibits MUC1 protein expression, enhancing the anticancer effect of sorafenib (70).
4.2.4 Other antitumor activities of solamargine
In human castration-resistant prostate cancer (CRPC), SM promotes the phosphorylation of AMPKα and decreases the expression of MUC1 and NF-κB p65 proteins. This leads to the suppression of CRPC cell growth through AMPKα-mediated inhibition of p65 (71). When combined with docetaxel, SM enhances the antitumor effect of docetaxel (72). According to Zhang et al., the treatment of human cholangiocarcinoma cells QBC939 with SM increases the expression of apoptosis-related proteins, inhibiting cell viability. This suggests that SM may induce apoptosis in human cholangiocarcinoma QBC939 cells through the mitochondrial pathway (73).
SM exerts its antitumor effects through multiple mechanisms, including the tumor suppressor pathway, caspase activation pathway, mitochondrial pathway, apoptosis receptor pathway, protein kinase pathway, and signaling pathways that promote invasion/migration and multidrug resistance (Figure 3).
4.3 Solasonine
Solasonine, with the molecular formula C45H73NO16, is another typical steroidal alkaloid found in S. nigrum. It is commonly used in the treatment of skin diseases and various cancers, and extensive pharmacological studies have been conducted on it.
4.3.1 Anti–liver cancer activity of solasonine
Mortalin, a protein overexpressed in various cancers, sequesters p53 into the cytoplasm, preventing its translocation to the nucleus and inhibiting its cellular functions. Inhibiting the mortalin-p53 interaction is a novel strategy against tumors (74). Solasonine inhibits this interaction, inducing apoptosis in HCC cell lines expressing p53 (HepG2) or in those not expressing p53 (Hep3b). Thus, the apoptotic activity of solasonine can be mediated through both p53-dependent and p53-independent pathways (75). SP1, a direct target of miR-375-3p, can be co-regulated by miR-375-3p and CCAT1. Solasonine can inhibit CCAT1 and SP1 by activating miR-375-3p. Subsequently, IRF5 protein expression is suppressed, inhibiting the proliferation of HepG2 liver cancer cells. Therefore, IRF5 may be a potential target for the treatment of liver cancer (76–79).
4.3.2 Anti-osteosarcoma activity of solasonine
Tumor cells ferment large amounts of glucose into lactic acid even in the presence of oxygen, a phenomenon known as the Warburg effect or aerobic glycolysis, to supply energy (80). Glycolysis is believed to be driven by oncogenic signaling pathways. The Wnt/β-catenin pathway is essential for the development of various embryos. Aberrant activation of this pathway is associated with the development and progression of many human malignancies. Wnt/β-catenin/Snail has been shown to activate EMT, which is closely associated with tumor cell invasion and metastasis (81). According to Wang et al., solasonine upregulates E-cadherin expression and downregulates N-cadherin expression, inhibiting EMT in cells and effectively restraining the migration and invasion of osteosarcoma cells. This inhibition of aerobic glycolysis in osteosarcoma cells, along with reduced lactate secretion and glucose consumption and increased ROS production in cell supernatants, is mediated by solasonine through the Wnt/β-Catenin/Snail pathway in an ALDOA-dependent manner. These effects inhibit cancer cell proliferation and migration in both in vivo and in vitro settings (82).
4.3.3 Other antitumor activities of solasonine
Solasonine, either alone or in combination, can trigger apoptosis in the SGC-7901 human gastric cancer cell line through the mitochondrial apoptosis pathway. Furthermore, when combined with cisplatin, solasonine enhances cisplatin’s activity, resulting in improved therapeutic outcomes (83). Moreover, solasonine is effective at inhibiting cancer cell proliferation and increasing sensitivity to chemotherapeutic drugs by targeting miR-486-5p, thereby increasing its expression. miR-486-5p, which post-transcriptionally regulates PI3KR1, plays a regulatory role in gastric cancer. These findings underscore solasonine ‘s antigastric cancer effects by modulating the miR-486-5p/PI3KR1 axis (84). The above antitumor mechanism of solasonine was shown in Figure 4.
4.4 Solasodine
Solasodine, with a molecular formula of C27H43NO2, is a steroidal alkaloidal sapogenin known for its pharmacological effects such as antioxidant properties (85) and anticonvulsant activity (86). Many studies have highlighted the potent cytotoxicity of solasonine against various types of tumor cells, which is described in the subsequent sections.
4.4.1 Anti–pancreatic cancer activity of solasodine
In clinical treatment, solasodine (CTX) is commonly employed due to its potent anticancer activity against pancreatic cancer (87). In a SW1990 tumor-bearing mouse model, solasodine exhibited superior efficacy in inhibiting tumor growth over CTX, while exerting lower toxicity to normal cells. Solasodine achieved this by inhibiting the Cox-2/Akt/GSK3β signaling pathway in pancreatic cancer cells, leading to increased expression of cyt-C, caspase-9, caspase-3, and Bax and decreased expression of Bcl-2 in a dose-dependent manner. Additionally, solasodine stimulated the immune response, significantly elevating serum levels of TNF-α, IL-2, and IFN-γ in tumor-bearing mice in vivo (88).
4.4.2 Anti–gastric cancer activity of solasodine
Solasodine demonstrated a dose-dependent downregulated expression of caspase-3, caspase-7, caspase-9, and poly-ADP-ribose polymerase (PARP) in GC cells HGC27. It also reduced the levels of GPX4 and SLC7A11, although it did not alter the levels of ROS and malondialdehyde. Moreover, solasodine promoted apoptosis and ferroptosis in cancer cells, effectively inhibiting tumor growth. Through the inhibition of the RhoA/STAT 3/NF-κB pathway and reduction of CLDN 2 through the AMPK pathway, solasodine suppressed metastasis and inhibited EMT in GC cells. Hence, solasodine presents itself as a potential antitumor drug for GC (89).
4.4.3 Anti–colorectal cancer activity of solasodine
Solasodine can inhibit human colorectal cancer cells by targeting the AKT/GSK-3β/β-catenin axis and inducing apoptosis in rectal cancer cells through the activation of the caspase cascade (90). Treatment of rectal cancer HCT 116 cells with varying concentrations of solasodine resulted in reduced protein levels of tumor stemness markers, including CD133, CD44, Nanog, Oct-4, and Sox-2. Additionally, these treatments inhibited TGF-β1-induced invasion and migration of HCT 116 cells. These results suggest that solasodine can reverse the stemness of colorectal cancer cells in vitro and in vivo, while also suppressing the metastasis and invasion of cancer cells, thereby exerting its anticancer effects (91).
In conclusion, solasodine exhibits potent antitumor effects and holds promise as a potentially active drug for cancer treatment. The above antitumor mechanism of solasonine was shown in Figure 5.
5 Future prospects
Traditional Chinese medicine has emerged as a significant strategy for cancer treatment, offering a novel therapeutic approach characterized by its ability to target multiple components, affect various signaling pathways, and reduce side effects. Astragalus membranaceus, S. nigrum, Lotus plumule, and Ligusticum wallichii are prominent traditional Chinese medicines widely utilized in clinical antitumor treatments. Due to its inherent toxicity, S. nigrum is frequently combined with other drugs in clinical settings, resulting in remarkable antitumor effects, substantial medicinal value, and promising prospects for further development. In recent years, fewer in-depth studies have explored the pharmacological mechanisms of the active monomer components within S. nigrum. Therefore, future research should prioritize investigating this aspect. Combining individual components with traditional chemotherapeutic drugs such as solanine, SM, and cisplatin, has proven to enhance tumor suppression efficiency and mitigate drug resistance in tumor cells. This innovative approach opens up a fresh avenue for investigating the anticancer properties of S. nigrum. Notably, a series of derivatives have recently been identified based on the active monomer components, demonstrating significant anticancer pharmacological activities. The development of new derivatives and targeted dosage forms also holds substantial research potential.
6 Conclusion
This study provides a comprehensive review of the four anti-tumor active components found in the Chinese medicinal herb S. nigrum, along with their molecular mechanisms of action (Table 1). S. nigrum is widely utilized in clinical Chinese medicine and traditional folk Chinese herbal formulas. A wealth of evidence supports the notion that the active components in S. nigrum can effectively inhibit cancer cells, reverse drug resistance in tumors, and reduce the stemness of tumor stem cells. The antitumor effects and mechanisms of S. nigrum primarily encompass the inhibition of cell proliferation, cell cycle arrest, induction of apoptosis, suppression of EMT and tumor metastasis, reversal of drug resistance, and enhancement of the efficacy of radiotherapy and targeted therapy. This review delves into the antitumor mechanisms of the active monomer components in S. nigrum, offering valuable theoretical insights for the rational utilization and further development of S. nigrum in cancer treatment.
Author contributions
HZ: Writing – original draft. J-LL: Writing – original draft. Q-SZ: Conceptualization, Project administration, Supervision, Writing – review & editing. JL: Conceptualization, Project administration, Supervision, Writing – review & editing.
Funding
The author(s) declare that no financial support was received for the research, authorship, and/or publication of this article.
Conflict of interest
The authors declare that the research was conducted in the absence of any commercial or financial relationships that could be construed as a potential conflict of interest.
Publisher’s note
All claims expressed in this article are solely those of the authors and do not necessarily represent those of their affiliated organizations, or those of the publisher, the editors and the reviewers. Any product that may be evaluated in this article, or claim that may be made by its manufacturer, is not guaranteed or endorsed by the publisher.
References
1. Kumar MS, Adki KM. Marine natural products for multi-targeted cancer treatment: A future insight. BioMed Pharmacother (2018) 105:233–45. doi: 10.1016/j.biopha.2018.05.142
2. Sung H, Ferlay J, Siegel RL, Laversanne M, Soerjomataram I, Jemal A, et al. Global cancer statistics 2020: globocan estimates of incidence and mortality worldwide for 36 cancers in 185 countries. CA: A Cancer J Clin (2021) 71(3):209–49. doi: 10.3322/caac.21660
3. Siegel RL, Miller KD, Wagle NS, Jemal A. Cancer statistics, 2023. CA: A Cancer J Clin (2023) 73(1):17–48. doi: 10.3322/caac.21763
4. Cao W, Chen HD, Yu YW, Li N, Chen WQ. Changing profiles of cancer burden worldwide and in China: A secondary analysis of the global cancer statistics 2020. Chin(Engl) (2021) 134(7):783–91. doi: 10.1097/cm9.0000000000001474
5. Liu LY, Yang YK, Wang JN, Ren JG. Steroidal alkaloids from solanum nigrum and their cytotoxic activities. Phytochemistry (2022) 202:113317. doi: 10.1016/j.phytochem.2022.113317
6. Hanahan D. Hallmarks of cancer: new dimensions. Cancer Discovery (2022) 12(1):31–46. doi: 10.1158/2159-8290.Cd-21-1059
7. Yamashina T, Ishihara R, Nagai K, Matsuura N, Matsui F, Ito T, et al. Long-term outcome and metastatic risk after endoscopic resection of superficial esophageal squamous cell carcinoma. Am J Gastroenterol (2013) 108(4):544–51. doi: 10.1038/ajg.2013.8
8. Acharya S, Dilnawaz F, Sahoo SK. Targeted epidermal growth factor receptor nanoparticle bioconjugates for breast cancer therapy. Biomaterials (2009) 30(29):5737–50. doi: 10.1016/j.biomaterials.2009.07.008
9. Qian L, Su W, Wang Y, Dang M, Zhang W, Wang C. Synthesis and characterization of gold nanoparticles from aqueous leaf extract of alternanthera sessilis and its anticancer activity on cervical cancer cells (Hela). Artif Cells Nanomed Biotechnol (2019) 47(1):1173–80. doi: 10.1080/21691401.2018.1549064
10. Chan HK, Ismail S. Side effects of chemotherapy among cancer patients in a Malaysian general hospital: experiences, perceptions and informational needs from clinical pharmacists. Asian Pac J Cancer Prev (2014) 15(13):5305–9. doi: 10.7314/apjcp.2014.15.13.5305
11. Pei H, Yang J, Li W, Luo X, Xu Y, Sun X, et al. Solanum nigrum linn.: advances in anti-cancer activity and mechanism in digestive system tumors. Med Oncol (2023) 40(11):311. doi: 10.1007/s12032-023-02167-7
12. Chen X, Dai X, Liu Y, Yang Y, Yuan L, He X, et al. Solanum nigrum linn.: an insight into current research on traditional uses, phytochemistry, and pharmacology. Front Pharmacol (2022) 13:918071. doi: 10.3389/fphar.2022.918071
13. Jainu M, Devi CS. Antiulcerogenic and ulcer healing effects of solanum nigrum (L.) on experimental ulcer models: possible mechanism for the inhibition of acid formation. J Ethnopharmacol (2006) 104(1-2):156–63. doi: 10.1016/j.jep.2005.08.064
14. Nirmal SA, Patel AP, Bhawar SB, Pattan SR. Antihistaminic and antiallergic actions of extracts of solanum nigrum berries: possible role in the treatment of asthma. J Ethnopharmacol (2012) 142(1):91–7. doi: 10.1016/j.jep.2012.04.019
15. Jin J, Sun H, Wei H, Liu G. The anti-hepatitis drug ddb chemosensitizes multidrug resistant cancer cells in vitro and in vivo by inhibiting P-gp and enhancing apoptosis. Invest New Drugs (2007) 25(2):95–105. doi: 10.1007/s10637-006-9001-z
16. Xiang L, Wang Y, Yi X, He X. Steroidal alkaloid glycosides and phenolics from the immature fruits of solanum nigrum. Fitoterapia (2019) 137:104268. doi: 10.1016/j.fitote.2019.104268
17. Dey P, Kundu A, Chakraborty HJ, Kar B, Choi WS, Lee BM, et al. Therapeutic value of steroidal alkaloids in cancer: current trends and future perspectives. Int J Cancer (2019) 145(7):1731–44. doi: 10.1002/ijc.31965
18. Saijo R, Murakami K, Nohara T, Tomimatsu T, Sato A, Matsuoka K. Studies on the constituents of solanum plants. Ii. On the constituents of the immature berries of solanum nigrum L. (Author’s transl). Yakugaku Zasshi (1982) 102(3):300–5. doi: 10.1248/yakushi1947.102.3_300
19. Gu XY, Shen XF, Wang L, Wu ZW, Li F, Chen B, et al. Bioactive steroidal alkaloids from the fruits of solanum nigrum. Phytochemistry (2018) 147:125–31. doi: 10.1016/j.phytochem.2017.12.020
20. Yang YK, Liu LY, Wang WY, Wang JN, Lin L, Liu JX. [Two new steroidal alkaloids from ripe berries of solanum nigrum]. Zhongguo Zhong Yao Za Zhi (2022) 47(18):4966–71. doi: 10.19540/j.cnki.cjcmm.20220506.205
21. Lin HJ, Mahendran R, Huang HY, Chiu PL, Chang YM, Day CH, et al. Aqueous extract of solanum nigrum attenuates angiotensin-ii induced cardiac hypertrophy and improves cardiac function by repressing protein kinase C-ζ to restore hsf2 desumolyation and mel-18-igf-iir signaling suppression. J Ethnopharmacol (2022) 284:114728. doi: 10.1016/j.jep.2021.114728
22. Ling B, Xiao S, Yang J, Wei Y, Sakharkar MK, Yang J. Probing the antitumor mechanism of solanum nigrum L. Aqueous extract against human breast cancer mcf7 cells. Bioengineering (Basel) (2019) 6(4):112. doi: 10.3390/bioengineering6040112
23. Li J, Li Q, Feng T, Li K. Aqueous extract of solanum nigrum inhibit growth of cervical carcinoma (U14) via modulating immune response of tumor bearing mice and inducing apoptosis of tumor cells. Fitoterapia (2008) 79(7-8):548–56. doi: 10.1016/j.fitote.2008.06.010
24. Tai CJ, Wang CK, Tai CJ, Lin YF, Lin CS, Jian JY, et al. Aqueous extract of solanum nigrum leaves induces autophagy and enhances cytotoxicity of cisplatin, doxorubicin, docetaxel, and 5-fluorouracil in human colorectal carcinoma cells. Evid Based Complement Alternat Med (2013) 2013:514719. doi: 10.1155/2013/514719
25. Son YO, Kim J, Lim JC, Chung Y, Chung GH, Lee JC. Ripe fruit of solanum nigrum L. Inhibits cell growth and induces apoptosis in mcf-7 cells. Food Chem Toxicol (2003) 41(10):1421–8. doi: 10.1016/s0278-6915(03)00161-3
26. Wang CW, Chen CL, Wang CK, Chang YJ, Jian JY, Lin CS, et al. Cisplatin-, doxorubicin-, and docetaxel-induced cell death promoted by the aqueous extract of solanum nigrum in human ovarian carcinoma cells. Integr Cancer Ther (2015) 14(6):546–55. doi: 10.1177/1534735415588826
27. Wu YH, Chiu WT, Young MJ, Chang TH, Huang YF, Chou CY. Solanum incanum extract downregulates aldehyde dehydrogenase 1-mediated stemness and inhibits tumor formation in ovarian cancer cells. J Cancer (2015) 6(10):1011–9. doi: 10.7150/jca.12738
28. Wang CK, Lin YF, Tai CJ, Wang CW, Chang YJ, Choong CY, et al. Integrated treatment of aqueous extract of solanum nigrum-potentiated cisplatin- and doxorubicin-induced cytotoxicity in human hepatocellular carcinoma cells. Evid Based Complement Alternat Med (2015) 2015:675270. doi: 10.1155/2015/675270
29. Lai YJ, Tai CJ, Wang CW, Choong CY, Lee BH, Shi YC, et al. Anti-cancer activity of solanum nigrum (Aesn) through suppression of mitochondrial function and epithelial-mesenchymal transition (Emt) in breast cancer cells. Molecules (2016) 21(5):553. doi: 10.3390/molecules21050553
30. Churiyah C, Ningsih S, Firdayani F. The cytotoxic, apoptotic induction, and cell cycle arrest activities of solanum nigrum L. Ethanolic extract on mcf-7 human breast cancer cell. Asian Pac J Cancer Prev (2020) 21(12):3735–41. doi: 10.31557/apjcp.2020.21.12.3735
31. Wang HC, Wu DH, Chang YC, Li YJ, Wang CJ. Solanum nigrum linn. Water extract inhibits metastasis in mouse melanoma cells in vitro and in vivo. J Agric Food Chem (2010) 58(22):11913–23. doi: 10.1021/jf1022065
32. Zou T, Gu L, Yang L, Wei J, Zhao Y, Shen J, et al. Alpha-solanine anti-tumor effects in non-small cell lung cancer through regulating the energy metabolism pathway. Recent Pat Anticancer Drug Discovery (2022) 17(4):396–409. doi: 10.2174/1574892817666220113144635
33. Fu R, Wang X, Hu Y, Du H, Dong B, Ao S, et al. Solamargine inhibits gastric cancer progression by regulating the expression of lncneat1_2 via the mapk signaling pathway. Int J Oncol (2019) 54(5):1545–54. doi: 10.3892/ijo.2019.4744
34. Yin S, Jin W, Qiu Y, Fu L, Wang T, Yu H. Solamargine induces hepatocellular carcinoma cell apoptosis and autophagy via inhibiting lif/mir-192-5p/cyr61/akt signaling pathways and eliciting immunostimulatory tumor microenvironment. J Hematol Oncol (2022) 15(1):32. doi: 10.1186/s13045-022-01248-w
35. Chen Y, Tang Q, Wu J, Zheng F, Yang L, Hann SS. Inactivation of pi3-K/akt and reduction of sp1 and P65 expression increase the effect of solamargine on suppressing ep4 expression in human lung cancer cells. J Exp Clin Cancer Res (2015) 34:154. doi: 10.1186/s13046-015-0272-0
36. Wang X, Hu R, Song Z, Zhao H, Pan Z, Feng Y, et al. Sorafenib combined with stat3 knockdown triggers er stress-induced hcc apoptosis and cgas-sting-mediated anti-tumor immunity. Cancer Lett (2022) 547:215880. doi: 10.1016/j.canlet.2022.215880
37. Swetha M, Keerthana CK, Rayginia TP, Nath LR, Haritha NH, Shabna A, et al. Augmented efficacy of uttroside B over sorafenib in a murine model of human hepatocellular carcinoma. Pharm (Basel) (2022) 15(5):636. doi: 10.3390/ph15050636
38. Liang X, Hu C, Han M, Liu C, Sun X, Yu K, et al. Solasonine inhibits pancreatic cancer progression with involvement of ferroptosis induction. Front Oncol (2022) 12:834729. doi: 10.3389/fonc.2022.834729
39. Wang L, Sun QQ, Zhang SJ, Du YW, Wang YY, Zang WQ, et al. Inhibitory effect of α-solanine on esophageal carcinoma in vitro. Exp Ther Med (2016) 12(3):1525–30. doi: 10.3892/etm.2016.3500
40. Lin LT, Choong CY, Tai CJ. Solanine attenuated hepatocarcinoma migration and invasion induced by acetylcholine. Integr Cancer Ther (2020) 19:1534735420909895. doi: 10.1177/1534735420909895
41. Gao J, Ying Y, Wang J, Cui Y. Solanine inhibits immune escape mediated by hepatoma treg cells via the tgfβ/smad signaling pathway. BioMed Res Int (2020) 2020:9749631. doi: 10.1155/2020/9749631
42. El-Daly SM, Gouhar SA, Gamal-Eldeen AM, Abdel Hamid FF, Ashour MN, Hassan NS. Synergistic effect of α-solanine and cisplatin induces apoptosis and enhances cell cycle arrest in human hepatocellular carcinoma cells. Anticancer Agents Med Chem (2019) 19(18):2197–210. doi: 10.2174/1871520619666190930123520
43. Meng XQ, Zhang W, Zhang F, Yin SY, Xie HY, Zhou L, et al. Solanine-induced reactive oxygen species inhibit the growth of human hepatocellular carcinoma hepg2 cells. Oncol Lett (2016) 11(3):2145–51. doi: 10.3892/ol.2016.4167
44. Wu J, Wang L, Du X, Sun Q, Wang Y, Li M, et al. α-solanine enhances the chemosensitivity of esophageal cancer cells by inducing microrna−138 expression. Oncol Rep (2018) 39(3):1163–72. doi: 10.3892/or.2018.6187
45. Mohsenikia M, Alizadeh AM, Khodayari S, Khodayari H, Kouhpayeh SA, Karimi A, et al. The protective and therapeutic effects of alpha-solanine on mice breast cancer. Eur J Pharmacol (2013) 718(1-3):1–9. doi: 10.1016/j.ejphar.2013.09.015
46. Farhangi B, Alizadeh AM, Khodayari H, Khodayari S, Dehghan MJ, Khori V, et al. Protective effects of dendrosomal curcumin on an animal metastatic breast tumor. Eur J Pharmacol (2015) 758:188–96. doi: 10.1016/j.ejphar.2015.03.076
47. Mohsenikia M, Farhangi B, Alizadeh AM, Khodayari H, Khodayari S, Khori V, et al. Therapeutic effects of dendrosomal solanine on a metastatic breast tumor. Life Sci (2016) 148:260–7. doi: 10.1016/j.lfs.2016.02.008
48. Gibadulinova A, Tothova V, Pastorek J, Pastorekova S. Transcriptional regulation and functional implication of S100p in cancer. Amino Acids (2011) 41(4):885–92. doi: 10.1007/s00726-010-0495-5
49. Ni X, Chen J, Lu F, Yuan Z, Xu X, Hu Z, et al. Anti-cancer effect of α-solanine by down-regulating S100p expression in colorectal cancer cells. Recent Pat Anticancer Drug Discovery (2018) 13(2):240–7. doi: 10.2174/1574892813666180329163945
50. Yan X, Li M, Chen L, Peng X, Que ZJ, An HM, et al. α−Solanine inhibits growth and metastatic potential of human colorectal cancer cells. Oncol Rep (2020) 43(5):1387–96. doi: 10.3892/or.2020.7519
51. Hasanain M, Bhattacharjee A, Pandey P, Ashraf R, Singh N, Sharma S, et al. α-solanine induces ros-mediated autophagy through activation of endoplasmic reticulum stress and inhibition of akt/mtor pathway. Cell Death Dis (2015) 6(8):e1860. doi: 10.1038/cddis.2015.219
52. Munari CC, de Oliveira PF, Campos JC, Martins Sde P, Da Costa JC, Bastos JK, et al. Antiproliferative activity of Solanum lycocarpum alkaloidic extract and their constituents, Solamargine and Solasonine, in tumor cell lines. J Nat Med (2014) 68(1):236–41. doi: 10.1007/s11418-013-0757-0
53. Ding X, Zhu F, Yang Y, Li M. Purification, antitumor activity in vitro of steroidal glycoalkaloids from black nightshade (Solanum nigrum L.). Food Chem (2013) 141(2):1181–6. doi: 10.1016/j.foodchem.2013.03.062
54. Chen Y, Tang Q, Xiao Q, Yang L, Hann SS. Targeting ep4 downstream C-jun through erk1/2-mediated reduction of dnmt1 reveals novel mechanism of solamargine-inhibited growth of lung cancer cells. J Cell Mol Med (2017) 21(2):222–33. doi: 10.1111/jcmm.12958
55. Loewen G, Jayawickramarajah J, Zhuo Y, Shan B. Functions of lncrna hotair in lung cancer. J Hematol Oncol (2014) 7:90. doi: 10.1186/s13045-014-0090-4
56. Tang Q, Zheng F, Liu Z, Wu J, Chai X, He C, et al. Novel reciprocal interaction of lncrna hotair and mir-214-3p contribute to the solamargine-inhibited pdpk1 gene expression in human lung cancer. J Cell Mol Med (2019) 23(11):7749–61. doi: 10.1111/jcmm.14649
57. Han Y, Shi J, Xu Z, Zhang Y, Cao X, Yu J, et al. Identification of solamargine as a cisplatin sensitizer through phenotypical screening in cisplatin-resistant nsclc organoids. Front Pharmacol (2022) 13:802168. doi: 10.3389/fphar.2022.802168
58. Tang Q, Zhou Q, Li J, Yang X, Wang R, Wang X, et al. Solamargine enhanced gefitinib anti-tumor effect via regulating malat1/mir-141-3p/sp1/igfbp1 signaling pathway in non-small cell lung cancer. Carcinogenesis (2023) 44(6):497–510. doi: 10.1093/carcin/bgad028
59. Jayaraman S, Pazhani J, PriyaVeeraraghavan V, Raj AT, Somasundaram DB, Patil S, et al. Pcna and Ki67: Prognostic proliferation markers for oral cancer. Oral Oncol (2022) 130:105943. doi: 10.1016/j.oraloncology.2022.105943
60. Xie X, Zhu H, Yang H, Huang W, Wu Y, Wang Y, et al. Solamargine triggers hepatoma cell death through apoptosis. Oncol Lett (2015) 10(1):168–74. doi: 10.3892/ol.2015.3194
61. Xie X, Zhu H, Zhang J, Wang M, Zhu L, Guo Z, et al. Solamargine inhibits the migration and invasion of hepg2 cells by blocking epithelial-to-mesenchymal transition. Oncol Lett (2017) 14(1):447–52. doi: 10.3892/ol.2017.6147
62. Kufe DW. Mucins in cancer: function, prognosis and therapy. Nat Rev Cancer (2009) 9(12):874–85. doi: 10.1038/nrc2761
63. Nath S, Daneshvar K, Roy LD, Grover P, Kidiyoor A, Mosley L, et al. Muc1 induces drug resistance in pancreatic cancer cells via upregulation of multidrug resistance genes. Oncogenesis (2013) 2(6):e51. doi: 10.1038/oncsis.2013.16
64. Ahmad R, Raina D, Joshi MD, Kawano T, Ren J, Kharbanda S, et al. Muc1-C oncoprotein functions as a direct activator of the nuclear factor-kappab P65 transcription factor. Cancer Res (2009) 69(17):7013–21. doi: 10.1158/0008-5472.Can-09-0523
65. Hattrup CL, Gendler SJ. Muc1 alters oncogenic events and transcription in human breast cancer cells. Breast Cancer Res (2006) 8(4):R37. doi: 10.1186/bcr1515
66. Roy LD, Sahraei M, Subramani DB, Besmer D, Nath S, Tinder TL, et al. Muc1 enhances invasiveness of pancreatic cancer cells by inducing epithelial to mesenchymal transition. Oncogene (2011) 30(12):1449–59. doi: 10.1038/onc.2010.526
67. Sahraei M, Roy LD, Curry JM, Teresa TL, Nath S, Besmer D, et al. Muc1 regulates pdgfa expression during pancreatic cancer progression. Oncogene (2012) 31(47):4935–45. doi: 10.1038/onc.2011.651
68. Behrens ME, Grandgenett PM, Bailey JM, Singh PK, Yi CH, Yu F, et al. The reactive tumor microenvironment: muc1 signaling directly reprograms transcription of ctgf. Oncogene (2010) 29(42):5667–77. doi: 10.1038/onc.2010.327
69. Cascio S, Zhang L, Finn OJ. Muc1 protein expression in tumor cells regulates transcription of proinflammatory cytokines by forming a complex with nuclear factor-κb P65 and binding to cytokine promoters: importance of extracellular domain. J Biol Chem (2011) 286(49):42248–56. doi: 10.1074/jbc.M111.297630
70. Tang Q, Li X, Chen Y, Long S, Yu Y, Sheng H, et al. Solamargine inhibits the growth of hepatocellular carcinoma and enhances the anticancer effect of sorafenib by regulating hottip-tug1/mir-4726-5p/muc1 pathway. Mol Carcinog (2022) 61(4):417–32. doi: 10.1002/mc.23389
71. Xiang S, Zhang Q, Tang Q, Zheng F, Wu J, Yang L, et al. Activation of ampkα Mediates additive effects of solamargine and metformin on suppressing muc1 expression in castration-resistant prostate cancer cells. Sci Rep (2016) 6:36721. doi: 10.1038/srep36721
72. Ge J, Wang P, Ma H, Zhang J. Solamargine inhibits prostate cancer cell growth and enhances the therapeutic efficacy of docetaxel via akt signaling. J Oncol (2022) 2022:9055954. doi: 10.1155/2022/9055954
73. Zhang X, Yan Z, Xu T, An Z, Chen W, Wang X, et al. Solamargine derived from solanum nigrum induces apoptosis of human cholangiocarcinoma qbc939 cells. Oncol Lett (2018) 15(5):6329–35. doi: 10.3892/ol.2018.8171
74. Elwakeel A. Abrogating the interaction between P53 and mortalin (Grp75/hspa9/mthsp70) for cancer therapy: the story so far. Front Cell Dev Biol (2022) 10:879632. doi: 10.3389/fcell.2022.879632
75. Pham MQ, Tran THV, Pham QL, Gairin JE. In silico analysis of the binding properties of solasonine to mortalin and P53, and in vitro pharmacological studies of its apoptotic and cytotoxic effects on human hepg2 and hep3b hepatocellular carcinoma cells. Fundam Clin Pharmacol (2019) 33(4):385–96. doi: 10.1111/fcp.12447
76. Wang F, Li Y, Zhou J, Xu J, Peng C, Ye F, et al. Mir-375 is down-regulated in squamous cervical cancer and inhibits cell migration and invasion via targeting transcription factor sp1. Am J Pathol (2011) 179(5):2580–8. doi: 10.1016/j.ajpath.2011.07.037
77. Xu H, Jiang J, Zhang J, Cheng L, Pan S, Li Y. Microrna-375 inhibits esophageal squamous cell carcinoma proliferation through direct targeting of sp1. Exp Ther Med (2019) 17(3):1509–16. doi: 10.3892/etm.2018.7106
78. Wang Z, Gai Y, Zhou J, Liu J, Cui S. Mir-375 mediates the crf signaling pathway to regulate catecholamine biosynthesis by targeting sp1 in porcine adrenal gland. Stress (2019) 22(3):332–46. doi: 10.1080/10253890.2018.1561845
79. Liu Z, Ma C, Tang X, Tang Q, Lou L, Yu Y, et al. The reciprocal interaction between lncrna ccat1 and mir-375-3p contribute to the downregulation of irf5 gene expression by solasonine in hepg2 human hepatocellular carcinoma cells. Front Oncol (2019) 9:1081. doi: 10.3389/fonc.2019.01081
80. Vander Heiden MG, Cantley LC, Thompson CB. Understanding the warburg effect: the metabolic requirements of cell proliferation. Science (2009) 324(5930):1029–33. doi: 10.1126/science.1160809
81. Liang G, Fang X, Yang Y, Song Y. Silencing of cemip suppresses wnt/β-catenin/snail signaling transduction and inhibits emt program of colorectal cancer cells. Acta Histochem (2018) 120(1):56–63. doi: 10.1016/j.acthis.2017.11.002
82. Wang B, Zhou Y, Zhang P, Li J, Lu X. Solasonine inhibits cancer stemness and metastasis by modulating glucose metabolism via wnt/β-catenin/snail pathway in osteosarcoma. Am J Chin Med (2023) 51(5):1293–308. doi: 10.1142/s0192415x23500593
83. Li TC, Chen NJ, Chen YY, He BJ, Zhou ZF. Solasonine induces apoptosis of the sgc-7901 human gastric cancer cell line in vitro via the mitochondria-mediated pathway. J Cell Mol Med (2022) 26(12):3387–95. doi: 10.1111/jcmm.17343
84. Zhang Y, Han G, Cao Y, Zhang Y, Zhang X, Gong H. Solasonine inhibits gastric cancer proliferation and enhances chemosensitivity through microrna-486-5p. Am J Trans Res (2020) 12(7):3522–30.
85. Sharma T, Airao V, Panara N, Vaishnav D, Ranpariya V, Sheth N, et al. Solasodine Protects Rat Brain against Ischemia/Reperfusion Injury through Its Antioxidant Activity. Eur J Pharmacol (2014) 725:40–6. doi: 10.1016/j.ejphar.2014.01.005
86. Chauhan K, Sheth N, Ranpariya V, Parmar S. Anticonvulsant activity of solasodine isolated from solanum sisymbriifolium fruits in rodents. Pharm Biol (2011) 49(2):194–9. doi: 10.3109/13880209.2010.508499
87. Miyamoto R, Oda T, Hashimoto S, Kurokawa T, Inagaki Y, Shimomura O, et al. Cetuximab delivery and antitumor effects are enhanced by mild hyperthermia in a xenograft mouse model of pancreatic cancer. Cancer Sci (2016) 107(4):514–20. doi: 10.1111/cas.12888
88. Fan Y, Li Z, Wu L, Lin F, Shao J, Ma X, et al. Solasodine, isolated from solanum sisymbriifolium fruits, has a potent anti-tumor activity against pancreatic cancer. Drug Des Devel Ther (2021) 15:1509–19. doi: 10.2147/dddt.S266746
89. Su K, Yao X, Guo C, Qian C, Wang Y, Ma X, et al. Solasodine suppresses the metastasis of gastric cancer through claudin-2 via the ampk/stat3/nf-κb pathway. Chem Biol Interact (2023) 379:110520. doi: 10.1016/j.cbi.2023.110520
90. Zhuang YW, Wu CE, Zhou JY, Chen X, Wu J, Jiang S, et al. Solasodine inhibits human colorectal cancer cells through suppression of the akt/glycogen synthase kinase-3β/β-catenin pathway. Cancer Sci (2017) 108(11):2248–64. doi: 10.1111/cas.13354
Keywords: Solanum nigrum, antitumor, solanine, solamargine, solasonine, solasodine
Citation: Zhang H, Lv J-l, Zheng Q-s and Li J (2023) Active components of Solanum nigrum and their antitumor effects: a literature review. Front. Oncol. 13:1329957. doi: 10.3389/fonc.2023.1329957
Received: 30 October 2023; Accepted: 07 December 2023;
Published: 19 December 2023.
Edited by:
Samson Amos, Cedarville University, United StatesReviewed by:
Jaqueline Bazioli, University of Illinois Chicago, United StatesZebo Jiang, Zhuhai Hospital of Integrated Traditional Chinese & Western Medicine, China
Copyright © 2023 Zhang, Lv, Zheng and Li. This is an open-access article distributed under the terms of the Creative Commons Attribution License (CC BY). The use, distribution or reproduction in other forums is permitted, provided the original author(s) and the copyright owner(s) are credited and that the original publication in this journal is cited, in accordance with accepted academic practice. No use, distribution or reproduction is permitted which does not comply with these terms.
*Correspondence: Qiu-sheng Zheng, zqsyt@sohu.com; Jie Li, lijie5767@126.com
†These authors have contributed equally to this work