- 1Cancer Metastasis Alert and Prevention Center, and Biopharmaceutical Photocatalysis of State Key Laboratory of Photocatalysis on Energy and Environment, College of Chemistry, Fujian Provincial Key Laboratory of Cancer Metastasis Chemoprevention and Chemotherapy, Fuzhou University, Fuzhou, China
- 2Institute of Oceanography, Minjiang University, Fuzhou, China
Successful adhesion of circulating tumor cells (CTCs) to microvascular endothelium of distant metastatic tissue is the key starting step of metastatic cascade that could be effectively chemoprevented as we demonstrated previously. Here, we hypothesize that the hetero-adhesion may produce secretory biomarkers that may be important for both premetastatic diagnosis and chemoprevention. We show that co-incubation of triple-negative breast cancer (TNBC) cell line MDA-MB-231 with human pulmonary microvascular endothelial monolayers (HPMEC) secretes Cyr61 (CCN1), primarily from MDA-MB-231. However, addition of metapristone (RU486 metabolite) to the co-incubation system inhibits Cyr61 secretion probably via the Cyr61/integrin αvβ1 signaling pathway without significant cytotoxicity on both MDA-MB-231 and HPMEC. Transfection of MDA-MB-231 with Cyr61-related recombinant plasmid or siRNA enhances or reduces Cyr61 expression, accordingly. The transfection significantly changes hetero-adhesion and migration of MDA-MB-231, and the changed bioactivities by overexpressed CYR61 could be antagonized by metapristone in vitro. Moreover, the circulating MDA-MB-231 develops lung metastasis in mice, which could be effectively prevented by oral metapristone without significant toxicity. The present study, for the first time, demonstrates that co-incubation of MDA-MB-231 with HPMEC secrets CYR61 probably via the CYR61/integrin αvβ1 signaling pathway to promote adhesion-invasion of TNBC (early metastatic step). Metapristone, by interfering the adhesion-invasion process, prevents metastasis from happening.
Introduction
Breast cancer is the most common cancer and the leading cause of related death among females worldwide (Akram et al., 2017; Barrios et al., 2018). Triple-negative breast cancer (TNBC) represents about 15% of all breast cancers and is largely refractory to current available therapies (Wein and Loi, 2017). Therefore, identifying biomarkers responsible for TNBC, and developing a novel cancer metastasis chemopreventive agent is critical for alternative breast cancer treatment approaches. Metastasis is a frequent occurrence in TNBC. The most important step in metastasis is the migration of cancer cells away from the primary tumor, and then adhesion to the endothelial cells, a process called tumor invasion (Valastyan and Weinberg, 2011). Studies indicate that the migration and invasion of pathogenesis of tumor cells depend on cross-communications between tumor cells and various endothelial cells residing in their microenvironment (Rodvold and Zanetti, 2016; Lambert et al., 2017). For example, several growth factors signaling pathways, secreted proteins or micro RNAs (miRNAs) and exosomes are functional mediators of tumor-endothelial interactions in metastasis (Miller and Grunewald, 2015; Yu et al., 2015a; Yu et al., 2015b; Zeng et al., 2018). However, the way invasive cancer cells diminish the endothelial barrier function still remains elusive.
Cysteine-rich protein 61 (CCN1/Cyr61), cysteine-rich, heparin-binding extracellular matrix-associated protein, is the first cloned member of cysteine-rich protein (CCN) family which includes connective tissue growth factor (CTGF, CCN2), nephroblastoma over-expressed protein (Nov, CCN3), Wnt-1-induced secreted protein 1 (WISP-1, CCN4), WISP-2 (CCN5) and WISP-3 (CCN6) (Lin et al., 2012; Jandova et al., 2012). As a secreted protein, Cyr61 connects with the extracellular matrix and the cell surface (Grzeszkiewicz et al., 2002) and is a communication media between cancer cells and the host which can reflect the changes arising due to cancer treatment (Bonin-Debs et al., 2004; Mbeunkui et al., 2006). The Cyr61 protein has been reported to mediate cell adhesion, stimulate chemostasis, augment growth factor-induced DNA synthesis, foster cell survival, and enhance angiogenesis (Hou et al., 2014). Overexpression of Cyr61 enhanced the growth and migration of glioma cells through activation of the ILK-mediated-catenin-TCF/Lef and the Akt signaling pathways (Wu et al., 2017). Silencing Cyr61 in invasive breast cancer cells caused a major loss of MMP-1 induction from stromal fibroblasts and inhibited the tumorigenicity of breast cancer cells (Nguyen et al., 2006). Researchers showed that Cyr61 can activate biochemical signal transduction through interacting with various integrins (Crockett et al., 2007; Su et al., 2010). While binding of integrin αvβ3 triggered cell adhesion and apoptosis, binding of integrin α6β1 induced senescence, and binding of integrin αvβ5 affected migration (Crockett et al., 2010; Lau, 2011). These reports indicated that the conformation of Cyr61 and integrins may play a vital role in metastasis.
Metapristone is the most predominant biological active metabolite of mifepristone (Heikinheimo et al., 1989; Teng et al., 2011), which has received considerable attention due to its anticancer activity in the recent years. Metapristone was developed as a novel cancer metastasis chemopreventive agent by us for its per-metastatic chemoprevention. In our previous studies, metapristone induced dose-dependent apoptosis, and interfered with adhesion of HT-29 cells to human umbilical vein endothelial cells (HUVECs) in vitro (Wang et al., 2014). Moreover, our studies demonstrated that metapristone inhibited TNBC cells migration and adhesion to endothelial cells through intervening the EMT-related signaling pathways (Yu et al., 2016). Inspired by our previous studies, we hypothesize that the anti-metastasis potential of metapristone is related with the bidirectional cross-talk between endothelial and tumor cells.
To test the hypothesis, we examined the effects of endothelial cells (HPMEC) on the aggressive phenotype of breast cancer cells (MDA-MB-231) using an in vitro co-culture system. We observed that the co-culture of HPMEC with MDA-MB-231 increased the expression of Cyr61 (CCN1), and the formation of Cyr61/integrin αvβ1 complex. This highlights an important contact in cell communication between malignant breast epithelial cells and the endothelium. This study also supports our hypothesis and reveals a novel function for metapristone in the prevention of breast cancer metastasis by intervening Cyr61/integrin αvβ1 signaling pathways. The study report is as follows.
Materials and Methods
Materials
Anti-Cyr61, Anti-ITGAV, Anti-ITGB1, and goat Anti-Rabbit lgG H&L were from Abcam. Human recombinant Cyr61 was obtained from GeneTex. SiRNA-Cyr61 and negative control siRNA were purchased from Sangon Biotech (Shanghai). Pierce Co-Immunoprecipitation (Co-IP) Kit (26149) was purchased from Thermo scientific. The recombinant plasmid of pcDNA3.1-Cyr61 was constructed by our lab.
Cell Culture
MDA-MB-231 cells were obtained from American Type Culture Collection (ATCC, Manassas, VA), and were incubated with Leibovitz’s L-15 medium (Catalog No. 30-2008) containing 10% FBS, 100 U/ml penicillin and 100 µg/ml streptomycin at 37 °C in a free gas exchange with atmospheric air. MCF-7 cells were purchased from the national experimental cell resource sharing service platform (Beijing) and cultured in RPMI-1640 medium with 10% FBS, 100 U/ml penicillin, and 100 µg/ml streptomycin. Human pulmonary microvascular endothelial cells (HPMEC) were purchased from PromoCell, and cultured in ECM with 10% FBS, 100 U/ml penicillin, and 100 µg/ml streptomycin at 37 °C in 5% CO2 atmosphere.
InVitro Cytotoxicity Studies
The in vitro cytotoxicity was determined as what we described previously (Lau, 2011; Shi et al., 1993). MDA-MB-231 cells were trypsinized and seeded on 96-well plates at 8×103 cells/well. After adhering of 24 h, doses of metapristone (0,10,25,50,75,100 µM) were added and incubated for another 12 and 24 h respectively. Then, 100 µl/well MTT (5 mg/ml) was added and incubated for 4 h in incubator. The MTT solution was aspirated and replaced with 100 µl/well dimethyl sulfoxide solution (DMSO). After shaking 10 to 30 min, the plates were measured at 570 nm using an infinite M200 Pro microplate reader (Tecan, Switzerland).
Co-Culture Model and iTRAQ Analysis
Metastasis, a process that cancer cells invade surrounding tissues and migrate to distal organs including lung, liver, brain, bone, and lymph nodes, is a major cause of mortality in breast cancer patients (Torre et al., 2015), and adhering to the vascular endothelium is a key step when this process starts (Dotan et al., 2009). Thus, in this study, we used co-culture model to stimulate the tumor microenvironment in vitro. HPMEC cells were seeded in 75 cm flasks with complete ECM culture. After overnight adhesion, MDA-MB-231 cells (1:2) were plated into the same flasks with serum-free and metapristone (50 µM). Then the flasks were incubated another 24 h. Each group of serum-free ECM culture media was incubated for iTRAQ analysis. The procedures for iTRAQ and further analysis are described as these labs previously (Adav et al., 2010; Yu et al., 2015a; Yu et al., 2015b).
Elisa Assay
Enzyme-linked immunosorbent assay (ELISA) was used to investigate the secretion levels of Cyr61 at different conditions. Concentrations of Cyr61 from each group were measured quantitatively using a sandwich ELISA as described (Adav et al., 2010). Briefly, 96-Well ELISA plates were coated with mouse anti-human Cyr61 (ab80112) and stored overnight at 4°C. After three washes in PBST, wells were blocked with 1% BSA in PBS-T for 2 h at room temperature. Next, the serum-free medium was added to duplicate wells and human recombination Cyr61 (GTX48189-PRO) protein was diluted into different concentrations with PBS as the standard. Simultaneously, an additional set of wells were coated with blank buffer to serve as a control. Then plates were incubated at 37°C for 2 h and followed by three washed in PBS-T, respectively. A rabbit anti-human Cyr61 mAb (Santa Cruz Biotechnology, sc-13100) was added and incubated at 37°C for 2 additional h. After washing, alkaline phosphatase-conjugated goat anti-rabbit IgG antibodies were added for 2 additional h, then 2 M sodium hydroxide solution were used for color development. Concentrations of Cyr61 were determined by detecting the absorbance at 405 nm using an infinite M200 Pro microquant plate reader (Tecan, Switzerland). Each test was repeated at least three times.
Cell Morphology Assay
5×104 HPMEC cells were cultured in a 35 mm cell culture dish (NEST, GBD-35-20) for 12 h and then co-cultured with 2.5×104 MDA-MB-231 cells with or without metapristone (50 µM). This system was taken for a time-lapse photography by the Leica TCS SP8 confocal microscope.
Plasmid Construction, siRNAs Synthesis, and Transient Transfection
The ORF of the human Cyr61 cDNA was amplified by RT-PCR using specific primers (sense, 5`-taa aag ctt atg agc tcc cgc atc gcc ag-3` and antisense, 5`-ccc ctc gag tta gtc cct aaa ttt gtg aat gtc-3`) that were designed based on the Cyr61 gene (GenBank ID: CR536519.1) by Takara (Shiga, Japan). The gel-purified PCR products were digested with the restriction enzymes, HindIII and XhoI (Takara, Dalian, China), and cloned into the eukaryotic expression vector, pcDNA3.1 (Invitrogen, American). The inserted sequence was confirmed by DNA sequencing.
According to the design rule for RNAi (Laganà et al., 2015), a fragment of Cyr61 gene (5`-AACAUCAGUGCACAUGTAUUG-3`) was used as the target siRNA (Cyr61-siRNA). As control, the sequence of Cyr61 siRNA was rearranged at random (5`-CAAUACAUGUGCACUGAUGUU-3`) to yield the corresponding random-siRNA (ram-siRNA). siRNAs were synthesized by Sangon Biotech Corporation (Shanghai, China).
Transient transfection of MDA-MB-231 cells with siRNA oligos (100 pmol) and recombinant plasmid pcDNA3.1-Cyr61 (4 µg/well) was carried out using Lipofectamineeq \o\ac(○,R)3000 Transfection Reagent Protocol (life technologies), according to the manufacturer’s instructions. Both of nontargeting siRNA and empty pcDNA3.1 vector were served as negative controls, respectively. These cells were harvested 24 h after transfection and used for further analysis.
Cell Adhesion Assay
The adhesion assay of MDA-MB-231 cells to the HPMECs was assessed according to the method described previously by this lab (Yu et al., 2015; Yu et al., 2016). Briefly, HPMECs were seeded in 24-well plates and grown to 90% confluence in the ECM medium. Then, TNF-α (final concentration: 10 ng/ml) was used to activate HPMECs for 4 h. Rhodamine 123-labled MDA-MB-231 cells were washed twice using PBS and resuspended by ECM medium with a dose of metapristone, and co-cultured with the HPMEC monlayers (1:8) in each well for 2 h. DMSO (0.1%) was used as the vehicle control. Then, nonadherent cells were removed by PBS and ten visual fields for each well were selected randomly and taken pictures using a fluorescence microscope (Zeiss, Germany). Mean inhibition of adhesion for 10 visual fields was calculated by using the equation: % of control adhesion = [the number of adhered cells in treated group/the number of adhered cells in the control group] × 100%.
Wound Healing Assay
MDA-MB-231 cells were seeded in 24-well plates with 5×104/ml cells in complete medium and were reached over 90% confluence. The scratch wound was generated by using a pipette tip, and the floating cells were removed through washing with PBS. Then the PBS was instead by L-15 with different concentrations of metapristone (0, 10, 50, 75 µM) and the wound healing was recorded by using a fluorescence microscope (Zeiss, Germany) at 0 and 24 h. DMSO (final concentration: 0.1%) as vehicle control was added after wounding. At indicated time points, motility was quantified by measuring the average extent of wound closure. Each sample was assayed in triplicate in three independent experiments.
RNA Extraction and Real-Time PCR
MDA-MB-231 cells (6×105) were seeded in complete L-15 medium in 6-well plates and incubated over 24 h. Then, the medium was renewed with L-15 in the presence of metapristone (0, 10, 50, 75 µM). After incubation of 24 h, total RNA was exacted with Trizol reagent (Invitrogen, American) according to the manufacturer’s protocol and 4 µg RNA was converted to cDNA using PrimeScript™ RT reagent kit (TaKaRa, Dalian, China). The housekeeping gene, β-actin served as the internal control. Each real-time PCR reaction contained SYBRR) Premx Ex Taq II (Tli RNaseH Plus), PCR Forward/Reverse Primer, cDNA solution and dH2O. All PCR reactions were performed in triplicate using the mean value being used to determine mRNA levels. Relative mRNA expression levels for each gene were analyzed using the 2-ΔΔCt method and normalized to the endogenous reference gene β-actin.
The main primers were as follows:
β-actin:
F: 5`-TGGCACCCAGCACAATGAA-3`
R: 5`-CTAAGTCATAGTCCGCCTAGAAGCA-3`
integrin αv
F: 5`-TTGTAAGTTGGCAGATCTTCCTAAGTT-3`
R: 5`-GATGGGTAGTGGCTGCACATAG-3`
integrin β1:
F: 5`-AATTGTGGGTGGTGCACAAAT-3`
R: 5`-TGGAGGGCAACCCTTCTTTT-3`
Cyr61:
F: 5`-TCT CGT TGC TCA TGA AATT-3`
R: 5`-TAG AGT GGG TAC ATC AAA GCT TCAG-3`
Western Blot Analysis
MDA-MB-231 cells cultured in the present of metapristone (0, 10, 50, 75 µM) were lysed by RIPA. Then, the lysates were supplemented with HALT protease and phosphatase inhibitor cocktail (Thermo Scientific). Antibodies used for western blot analysis include Cyr61, integrin αv, integrin β1, and β-actin. Immunodetection of electrophoresis-resolved proteins was accomplished using enhanced chemiluminescence based on standard protocols, and signals were quantified with a quantitative digital imaging system (Quantity One, Bio-Rad) based on at least five replicates.
Co-Immunoprecipitation
The formation of the Cyr61/integrin αvβ1 complex in MDA-MB-231 cells was analyzed by co-immunoprecipitation and western blot according to the manufacturer’s instructions. Briefly, cells were lysed with IP Lysis/Wash Buffer (0.025 M Tris, 0.15 M NaCl, 0.001 M EDTA, 1% NP-40, 5% glycerol; pH7.4, containing 10 mM protease inhibitors (PMSF). Cell lysates (500 µl/15 × 105 cells) were incubated with mouse anti-Cyr61 monoclonal antibody binding to aminolink plus coupling resin in pierce spin columns. Purified mouse IgG (Beyotime Biotechnology) was used as the negative control. The pierce spin columns were washed four times, and the bound proteins were released by Elution Buffer for western blot analysis with rabbi anti-integrin αv and rabbit anti-integrin β1.
Immunofluorescence Microscopy
MDA-MB-231 cells (1.0×105) grown on 35 mm cell culture dish (NEST, GBD-35-20) were rinsed three times using PBS, and fixed in 4% paraformaldehyde for 30 min. Cells were then washed three times with PBS, and permeabilized with 0.2% Triton x-100 for 10 min. After washed three times with PBS again, the cells were blocked with 10% BSA for 30 min, then were incubated with mouse anti-Cyr61 (1:100) and rabbit anti-integrin αv (1:500)/anti-integrin β1 (1:250) for 1–2 h in room temperature, rewashed, and incubated with FITC-conjugated goat anti-mouse IgG and CY3-conjugated goat anti-rabbit for 1 h. Finally, the cells were washed, and examined using a Leica TCS SP8 Spectral Confocal System.
InVivo Tumor Xenograft Study
Four or six-week-old female BALB/C nude mice were purchased from the Shanghai Laboratory Animal Center (Shanghai, China) and maintained under clean conditions. Then they were divided into experimental group and control group randomly, eight animals per group. Cells (5 × 106) were resuspended in 200 µl of PBS, and injected into the lateral tail veins of mice, which had been orally gavaged with vegetable oil in the present of a dose of metapristone, 0 mg/kg (control), 2.5 mg/kg and 50 mg/kg for 3–4 days, respectively. After 5–7 weeks gavage, the lungs were removed, washed with PBS and fixed in 10% neutral buffered formalin. The number of lung tumor nodules was counted by visual inspection using a magnifying glass, then were paraffin embedded and stained with hematoxylin and eosin (H&E). The further study was to test the expression of Cyr61 and integrin αvβ1 in lung tissue using immunohistochemical analysis assay. All of the animal experiments were performed in accordance with animal protocol procedures, approved by the Institutional Animal Care and Use Committee of Fuzhou University.
Statistical Analysis
Data are presented as the mean ± standard error of the mean (SEM) or means ± standard deviations (SD). Statistical analysis was performed using the student’s t-test and one-way analysis of variance. A P-value less than 0.05 was considered statistically significant.
Results
The Cytotoxicity Effect of Metapristone on the Growth of MDA-MB-231 and HPMEC Cells.
We evaluated the effect of various concentrations of metapristone on the viability of MDA-MB-231, MCF-7, and HPMEC cells for 12 and 24 h by MTT assay. As shown in Figures 1A–C, the viability of each group cells decreased in a dose- and time- dependent manner following metapristone treatment. Their IC50 values for metapristone (24 h) were 88.1 ± 3.2 (MDA-MB-231), 87.0 ± 2.7 (MCF-7), and 91.2± 2.5(HPMEC) µM, respectively.
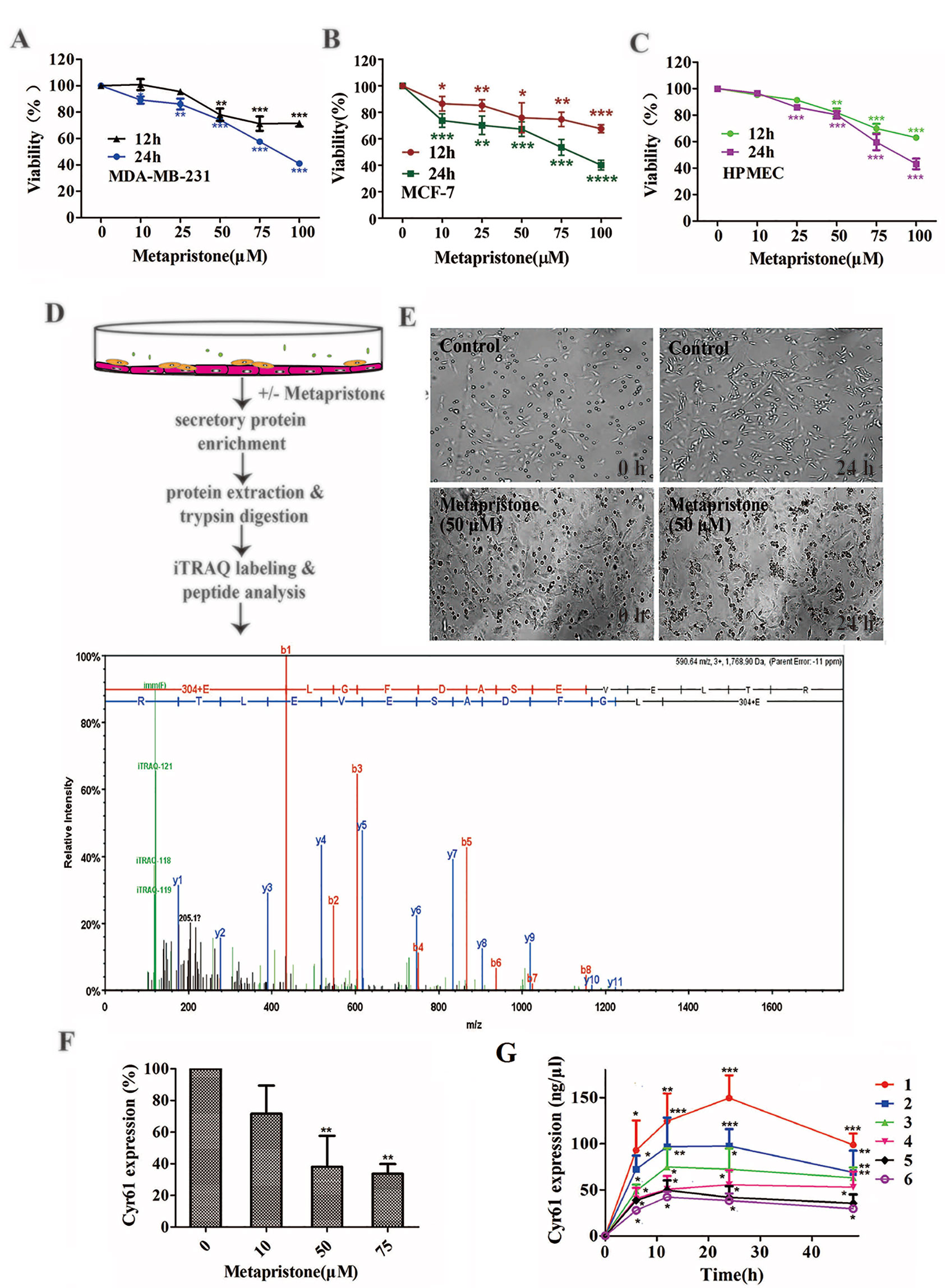
Figure 1 Secretion of Cyr61 from MDA-MB-231 and human pulmonary microvascular endothelial cells co-culture system and inhibition by metapristone of the secretion. (A) Effect of metapristone on MDA-MB-231 viability. (B) Effect of metapristone on MCF-7 viability. (C) Effect of metapristone on HPMEC viability. (D) Flow chart showing that MDA-MB-231 and HPMEC were co-cultured in the presence and absence of metapristone for 24 h followed by trypsin digestion and iTRAQ pharmacoproteomic analysis; The Cyr61 proteomic signal was shown at its corresponding m/z. (E) Confocal microscopy images showing the morphological changes by metapristone (50 µM) in the same spots where the two cell lines were co-cultured. (F) inhibition by metapristone of Cyr61 secretion. (G) time course of Cyr61 secretion (ELISA assay) under different conditions: 1) MDA-MB-231+ HPMEC; 2) MDA-MB-231+ HPMEC+ metapristone (50 µM); 3) MDA-MB-231; 4) MDA-MB-231+ metapristone (50 µM); 5) HPMEC; 6) HPMEC+ metapristone (50 µM). The data represent the mean± SEM (n = 3). *, P < 0.05, **, P < 0.01 and ***, P < 0.001 vs. the untreated control.
Overview of Quantitative Proteomics and ELISA Validation
iTRAQ analysis was performed on the purified proteins extracted from the supernatant of co-culture system with or without metapristone (50 µM) treatment to find the metapristone-mediated anti-metastasis proteins. As represented by the flow chart in Figure 1. In total, 105 secreted proteins showed significant differences in metapristone-treated co-culture system (P-value < 0.05). Among these differentially expressed proteins (DEPs), 77 were up-regulated (Table 1) and 28 were down-regulated (Table 2). Pharmacoproteomic study showed that the expression of Cyr61 was significantly down-regulated by metapristone by 60%, which was one of the most significantly changed proteins. To validate this, ELISA was performed. Compared with the control, the expression level of Cyr61 secreted from co-culture was down-regulated to 72%, 39%, and 36%, respectively, at 10, 50, and 75 µM of metapristone (Figure 1F), confirming that the proteomic data was reliable.
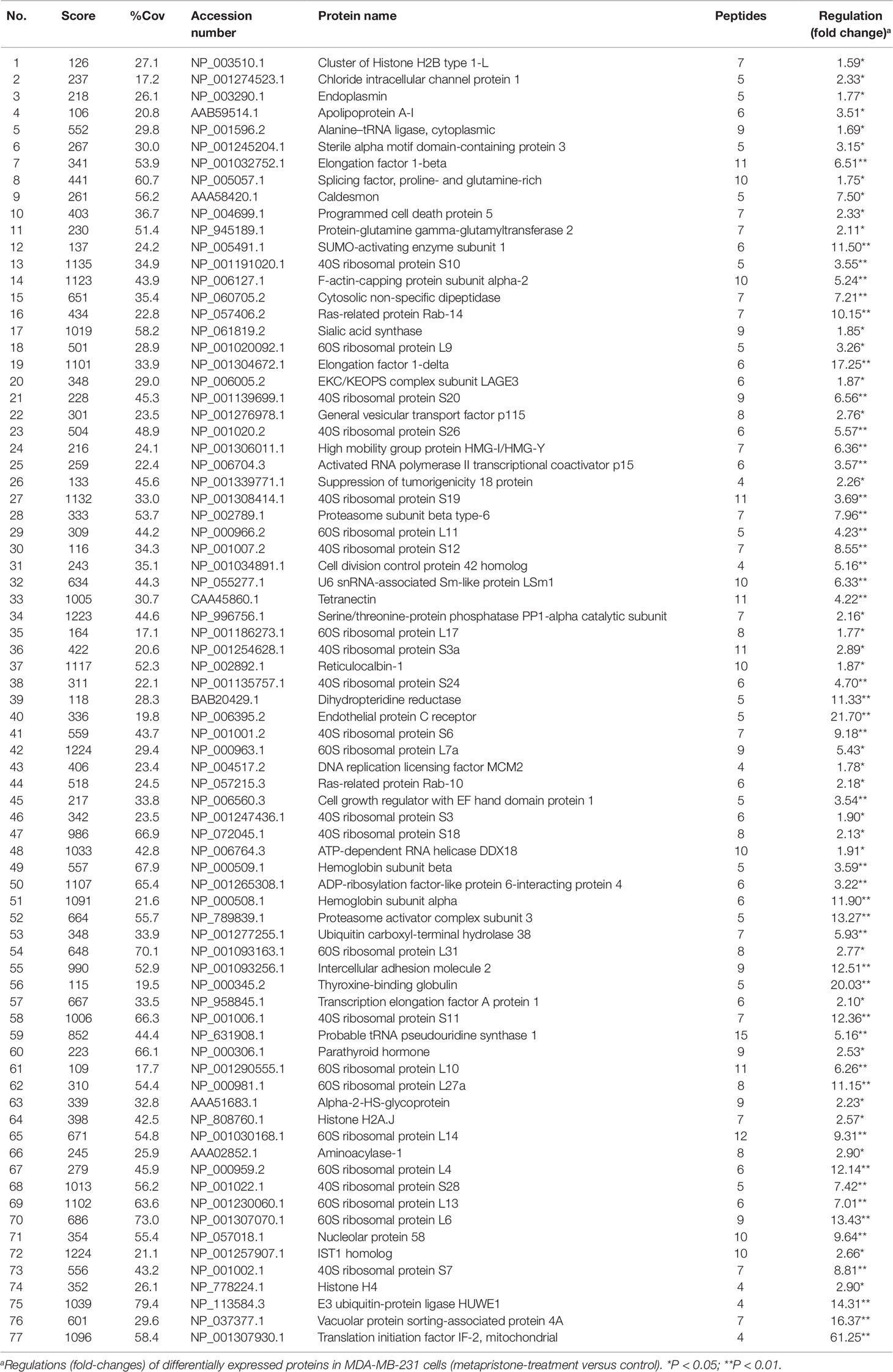
Table 1 Up-regulated proteins expressed in the conditioned media of MDA-MB-231 co-cultured with HPMECs.
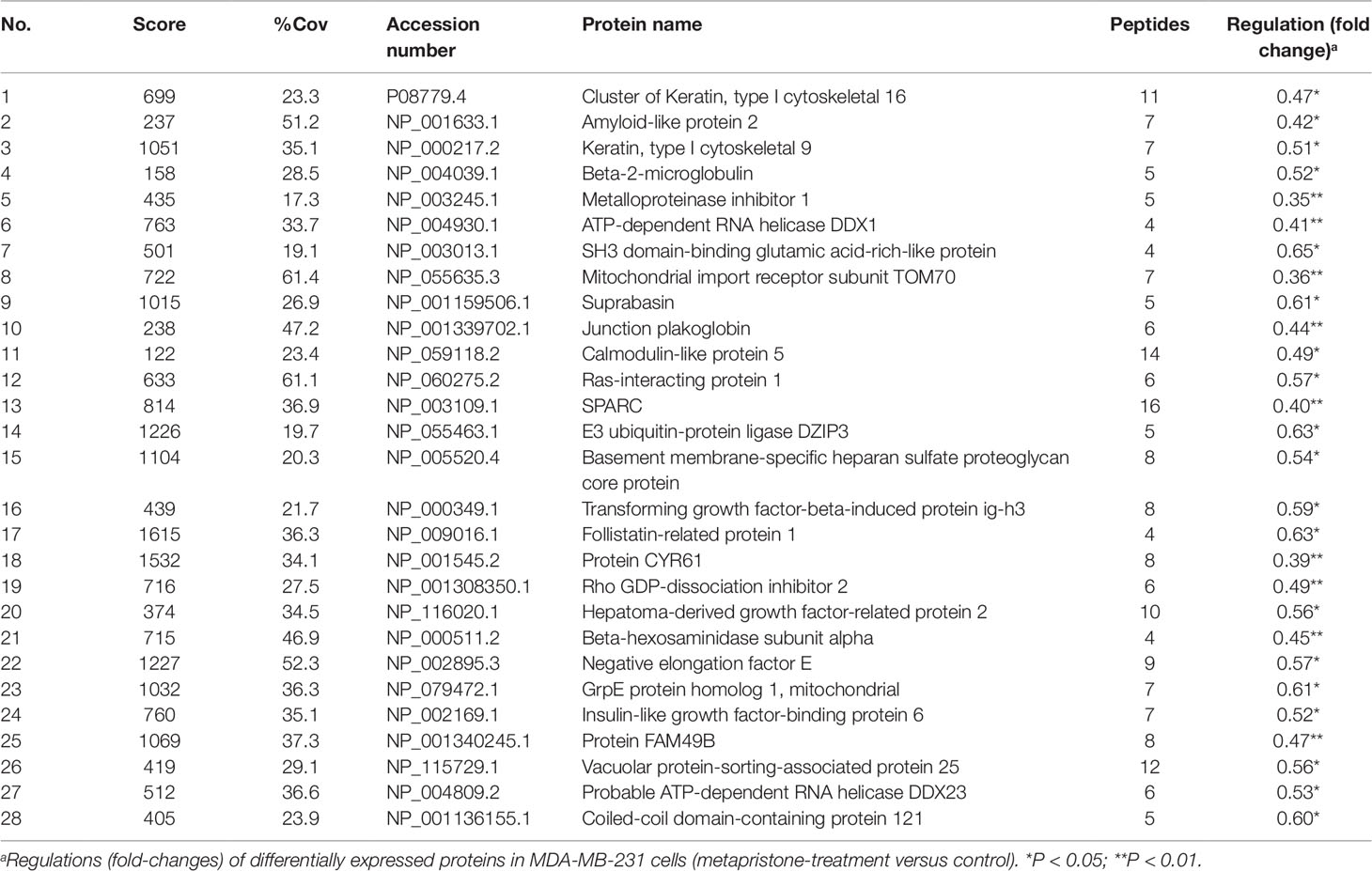
Table 2 Down-regulated proteins expressed in the conditioned media of MDA-MB-231 co-cultured with HPMECs.
The Origins of Cyr61 Secreted
To explore whether MDA-MB-231/HPMEC co-culture increases Cyr61 secretion, we tested the concentrations of Cyr61 in different groups at 0, 6, 12, 24, and 48 h by ELISA. As shown in Figure 1G, the secretion of Cyr61 increased at the beginning, then saturated or decreased progressively. Cyr61 expression in co-culture group was higher than that in MDA-MB-231 or HPMEC monocultures. Both MDA-MB-231 cells and HPMECs could secrete Cyr61, but the former secreted more. The secretions of Cyr61 in metapristone groups were lower than that in non-treatment groups. These results indicated that the co-culture system provided a better microenvironment for expression and secretion of Cyr61 compared with monoculture.
To further investigate the relationship between metapristone and co-culture model, a time-lapse photography was taken, which showed that MDA-MB-231 cells suspended in culture tended to adhere to HPMECs on the dish, but this tendency was interfered by metapristone. The co-culture system in the presence and absence of metapristone showed almost 0% adhesion. At 24 h after co-culture, the control group reached 45% adhesion, whereas the metapristone-treated group reached only 20% adhesion (Figure 1E).
Metapristone Inhibits Cyr61-Mediated Cell Migration and Adhesion
First, we constructed the “pcDNA3.1-Cyr61” plasmid and synthesized the “Cyr61-siRNA” (see Supplementary Information, Figure S1 and S2) to test the function of Cyr61 in metapristone-related anti-metastasis mechanics. Then, would healing assay was used to examine the effects of endogenous Cyr61 protein and metapristone on MDA-MB-231 cell motility. As shown in Figure 2A, the overexpression of Cyr61 enhanced cellular migration (170%) and the down-regulation of Cyr61 expression inhibited cellular migration (53%). MDA-MB-231 cells migration was inhibited by metapristone in a concentration-dependent manner by 74% (10 µM), 52% (50 µM), and 45% (75 µM), respectively (Figure 2). These results suggested that metapristone significantly inhibited Cyr61-mediated cell motility and wound closure at concentrations lowering than its IC50.
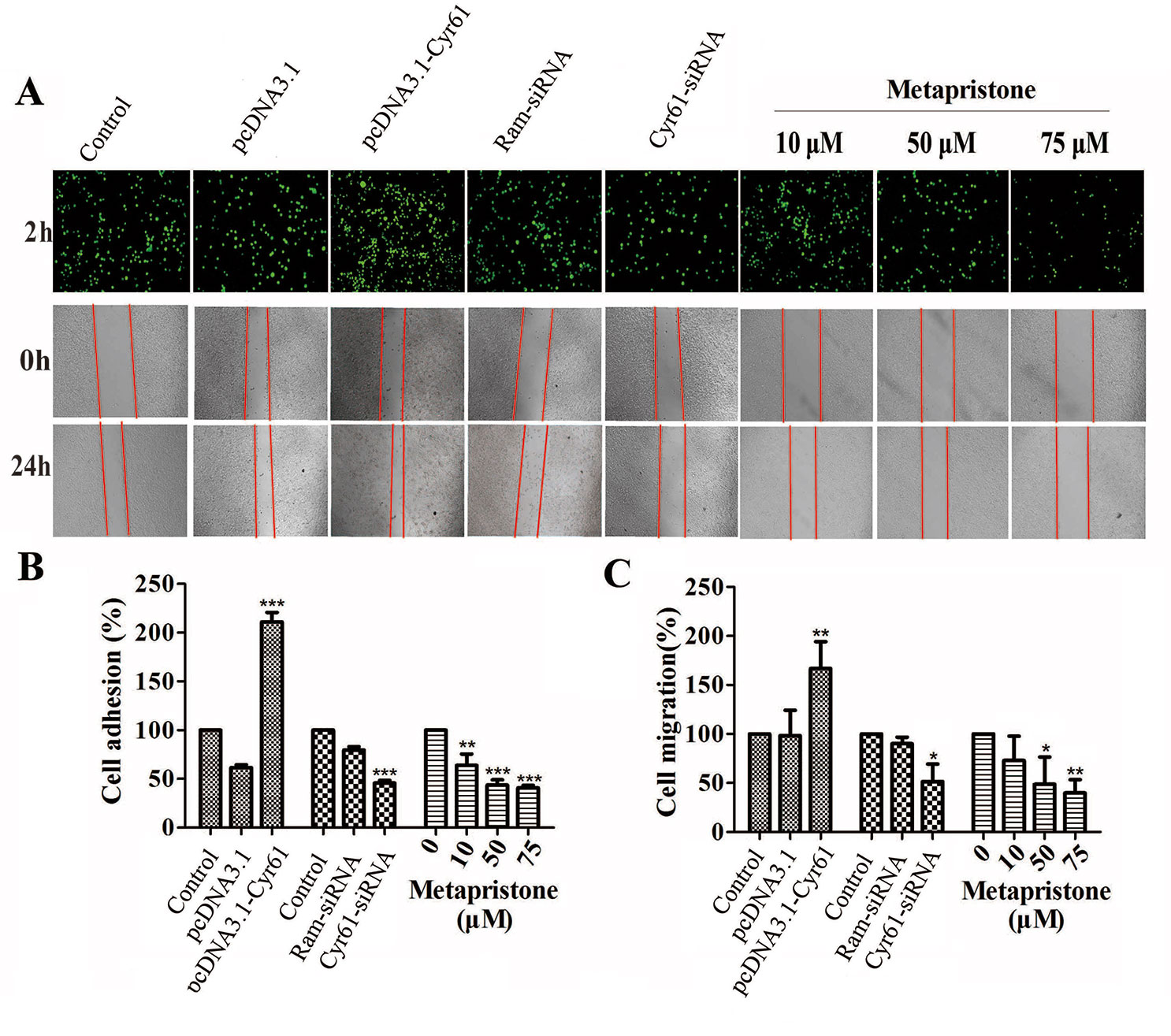
Figure 2 Cyr61-related cell adhesion and migration were mediated by metapristone. MDA-MB-231 cells were transfected with pcDNA3.1, pcDNA3.1-Cyr61 recombinant plasmid, or Ram-siRNA, Cyr61-siRNA for 36–48 h. (A) Upper panel: inhibition by metapristone of MDA-MB-231 cell adhesion to human pulmonary microvascular endothelial cells; note, the adhesion between pcDNA3.1-Cyr61 cancer cells and HPMECs was significantly enhanced. Whereas, the adhesion between Cyr-61-siRNA cancer cells and HPMECs was reduced in comparison with their controls. Metapristone caused concentration-dependent inhibition of the adhesion. Middle and lower panels: changes by metapristone in cell migration rate following the scratch assay. (B) Quantitative analysis of the adhesion between Cyr61-transfected cancer cells and HPMECs. (C) The MDA-MB-231 cells were transfected and treated differently, and the migration rate following the scratch assay was quantitatively determined; The data represent mean± SEM (n = 3), *, P < 0.05; **, P < 0.01; and ***, P < 0.001 vs. the controls.
It is well known that tumor cells adhesion to the ECM is a fundamental step in cancer metastasis (Gilkes et al., 2014; Conlon and Murray, 2019). The adhesion of MDA-MB-231 cells to HPMECs was examined to determine whether metapristone can regulate cell adhesion at a non-cytotoxic concentration by Cyr61 pathway. Compared with the control, the adhesion rate of MDA-MB-231 cells was 218%, 48%, 63%, 44%, and 41%, respectively, for transfection groups (pcDNA3.1-Cyr61, Cyr61-siRNA) and metapristone treatment groups (10, 50 and 75 µM) (Figures 2A, B). Metapristone markedly inhibited the adhesion of MDA-MB-231 cells to endothelial monolayers in a concentration-dependent manner through the Cyr61-dependent mechanism.
Metapristone Inhibits Adhesion and Migration of MDA-MB-231 Cells via the Cyr61/Integrin αvβ1 Signaling Pathway
Previous reports have indicated that the CCN family interacts with integrin receptors to modulate cell biological functions (Holbourn et al., 2008). Therefore, we sought to identify the cellular adhesion receptor(s) through which Cyr61 may function. By using co-immunoprecipitation assay, as shown in Figure 3, integrin αvβ1, as a new receptor for Cyr61 in the MDA-MB-231/HPMEC co-cultures was identified by immunoprecipitation combined with western blot analysis. We found that MDA-MB-231/HPMEC co-cultures promoted the formation of Cyr61/integrin αvβ1 complex, which was inhibited by metapristone significantly. The results were further confirmed by confocal microscopy co-localized methods using immunofluorescent staining of the CYR1, integrin αv, and integrin β1 proteins in MDA-MB-231 cells (Figure 3B).
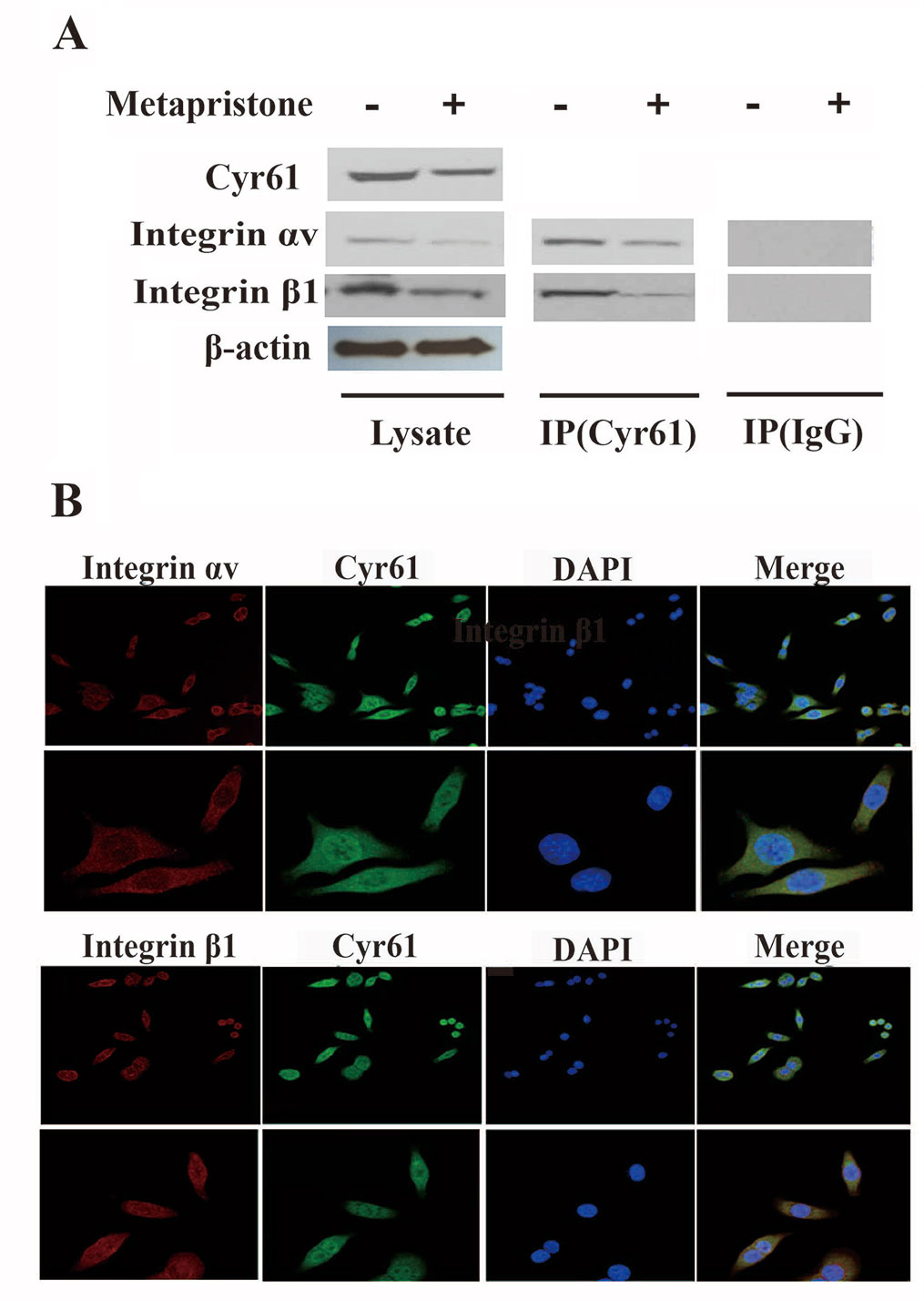
Figure 3 Formation of Cyr61/integrin αvβ1 complex in MDA-MB-231 cells. (A) Co-immunoprecipitation evidence: MDA-MB-231 cell lysates were added to aminolink-coupling resin columns that were individually coupled with anti-Cyr61 antibody (land 1 and 3), anti-integrin αv antibody (land 2), or anti-integrin β1 antibody (land 4). The bound complexes were eluted with the washing buffer and tested by western blotting with anti-integrin αv antibody (land 1), anti-Cyr61 antibody (land 2 and 4), or anti-integrin β1 antibody, individually. Samples of Lysis (before column; left), IgG, and Target (column elutes; right) after western blotting showed that the anti-Cyr61 antibody elute was integrin αv positive (land 1), integrin β1 positive (land 3), and Cyr61 positive (land 2 and 4). (B) Cyr61/integrin αvβ1 co-localization evidence: MDA-MB-231 cells were immunofluorescent-stained with Cyr61 (green), integrin αv (the upper two panels), or integrin β1 (the lower two panels; both in dark red) and DAPI (in blue for nuclear) dyes followed by confocal microscopic examination at amplification×20 (top panel) or ×40 (bottom panel). The merged images showed co-localization of Cyr61 with integrin αv and β1.
Furthermore, the effects of metapristone on the expressions of Cyr61, integrin αv, and integrin β1 on the protein and mRNA levels were determined using qRT-PCR and western blot analysis. The results showed that when the MDA-MB-231 cells were treated with different doses of metapristone, the expressions of Cyr61 and integrin αvβ1 were dose-dependently decreased (Figures 4A, B, D). As the secreted protein, the expression of Cyr61 in cell culture was detected by ELISA assay. As shown in Figure 4, the significantly reduced expression of the Cyr61 was observed in the presence of metapristone. The metapristone might regulate cell adhesion and migration through Cyr61/integrin αvβ1 pathway in MDA-MB-231 cells.
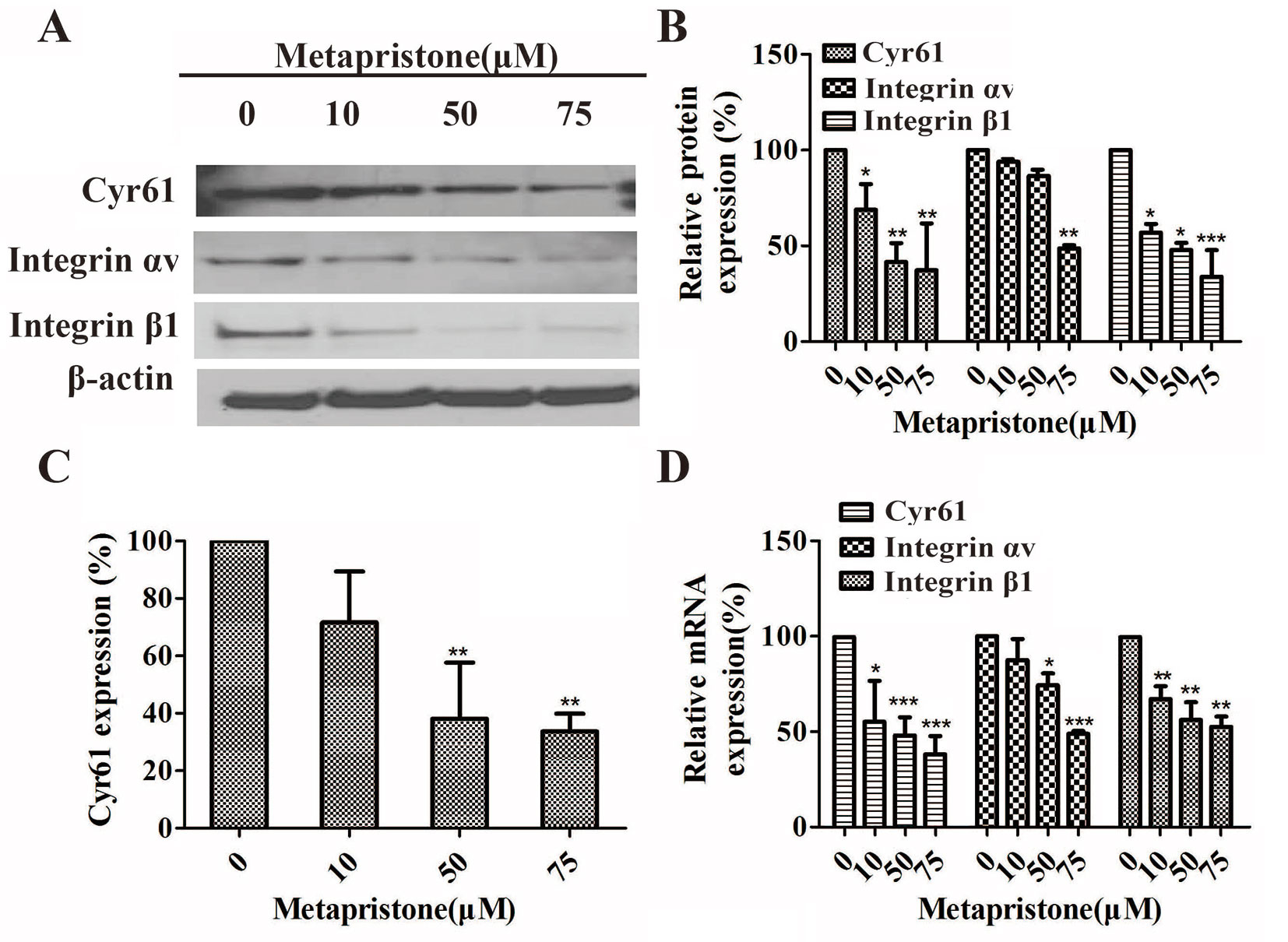
Figure 4 Inhibition by metapristone of expressions of Cyr61 and integrin αvβ1 in MDA-MB-231 cells. Western blot images (A) and the related quantitative analysis, (B) and quantitative ELISA analysis (C) of inhibition by metapristone of expressions of Cyr61 and integrin αvβ1 in MDA-MB-231 cells. (D) mRNA expression of Cyr61, integrin αv and β1 was inhibited by metapristone in a concentration-dependent manner. The data are expressed as the mean± SEM (n = 3). *, P < 0.05; **, P < 0.01, and ***, P < 0.001 vs. the untreated controls.
Effect of Metapristone on Experimental Lung Metastasis inVivo
The lungs are a frequent target of metastatic breast cancer cells (Landemaine et al., 2008; Oskarsson et al., 2011). Therefore, we further examined the anti-metastatic efficacy of metapristone on MDA-MB-231 cells using a xenograft mice model. Figure 5 showed representative images of pulmonary metastases of MDA-MB-231 breast cancer in each group. We obviously observed that pulmonary metastatic nodules and the rate of lung tumor-metastasis from the mice treated with the metapristone were less than those of mice in control group (Figures 5B, C). In addition, the histological examinations (hematoxylin-eosin staining of various lung sections) showed the metastatic nodules colonized in the lungs of metapristone treatment groups were smaller than that of non-treated group with lower density (Figure 5). Furthermore, there was obvious effect on the expressions of Cyr61 and integrin αvβ1 in these lung tissues (Figure 5) at different dose levels. By comparison, the positive areas of Cyr61 and integrin αvβ1 were decreased with high dose metapristone.
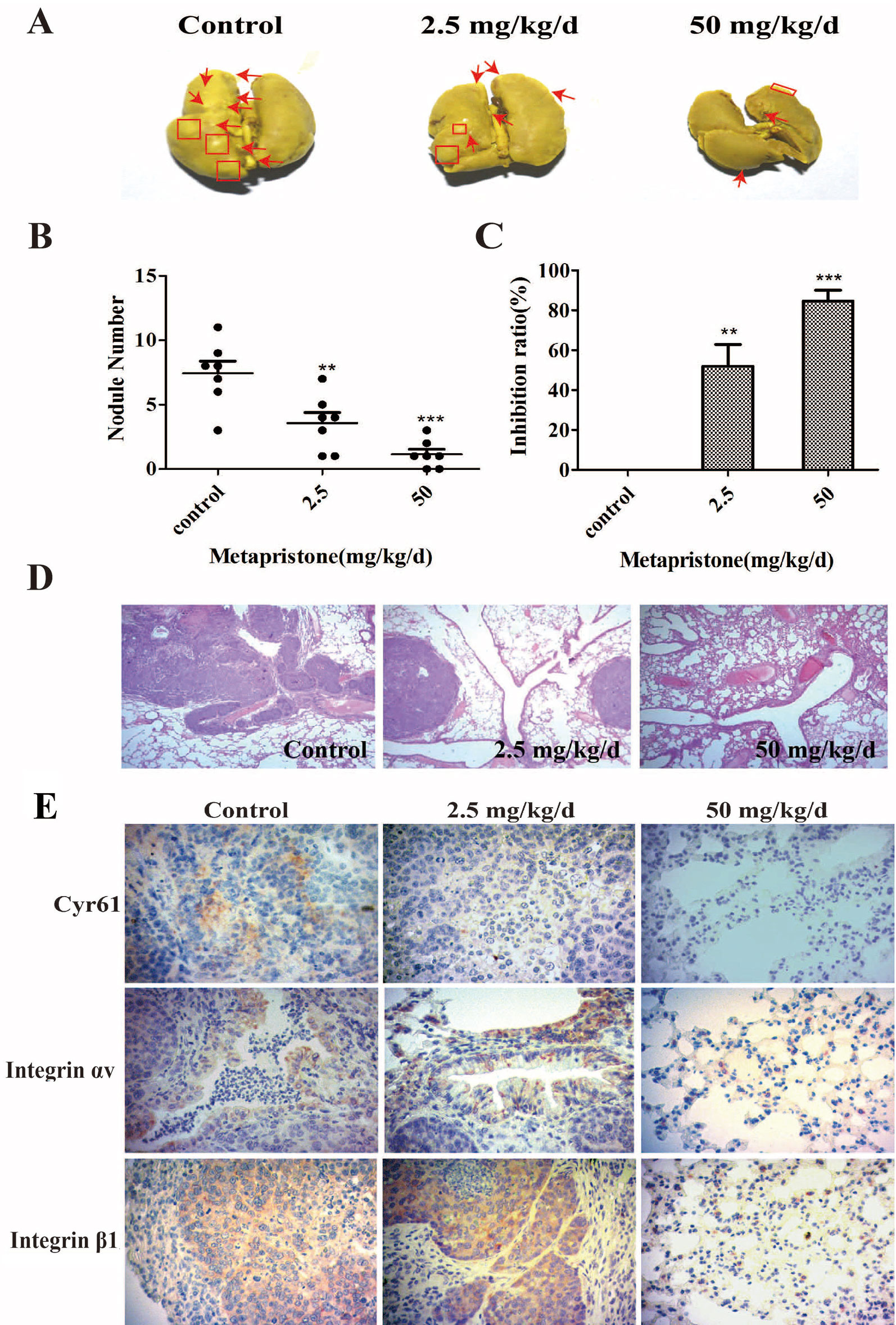
Figure 5 Metapristone inhibits lung metastasis of MDA-MB-231 cells via decreasing levels of Cyr61 and integrin αvβ1. (A) photography of mouse lung metastasis after six weeks of MDA-MB-231 inoculation via tail vein injection. The mice were pretreated with oral metapristone for three days before the inoculation followed by 6-week oral administration of metapristone. Control, drug vehicle; (B) quantitative comparison in mouse lung tumor nodules between the control and metapristone groups (n = 5/group); **P < 0.01, ***P < 0.001. (C) inhibition rate of mouse lung tumor metastasis after metapristone treatment; (D) hematoxylin–eosin staining of the lungs (amplification× 5); the arrows indicate metastatic foci that were significantly reduced by metapristone; (E) lung immunostaining with antibodies against Cyr61, integrin αv and integrin β1; the staining showed reduction in Cyr61/integrin avβ1 formation by metapristone.
Discussion
Tumor microenvironment plays an important role in directly stimulating malignant cell metastasis. For example, normal endothelial and tumor cells usually communicate indirectly before or directly after adhesion, through a complex network of interactions to drive cellular differentiation, tissue structures formation, cancer invasion, and metastasis. One function of this communication is to exchange both soluble and insoluble signaling molecules, such as secreted proteins, miRNAs and exosomes. However, the underlying mechanism is not well understood.
In this study, we used a MDA-MB-231/HPMEC co-culture model to investigate the secreted proteins involved in cell adhesion/invasion, the crucial procedures of cancer early metastasis (Devis et al., 2017). iTRAQ technology exhibited superb performance in the quantitative proteomic study (Astier, 2010). Using iTRAQ-based proteomic approach, we identified 105 secreted proteins, showing significant differences in metapristone (a potential cancer metastasis chemopreventives)-treated co-culture secretome compared to the control group (P-value < 0.05) (Figure 1 and Table 1). In particular, we found that MDA-MB-231/HPMEC co-cultures promoted the secretion levels of Cyr61 relative to MDA-MB-231 or HPMEC monocultures. In contrast, metapristone not only inhibited the Cyr61 secretion, but also prevented adhesion of MDA-MB-231 cells to HPMECs in morphology (Figure 1 and Figure 2).
Cyr61 (CCN1), as the first cloned member of cysteine-rich protein (CCN) family, is a secreted, cysteine-rich, heparin binding extracellular matrix-associated protein (Planque and Perbal, 2003; D’Antonio et al., 2010). To date, a number of reports describe that Cyr61 is involved in many cell biological functions. For example, Cyr61 has been identified to mediate cell adhesion, migration, proliferation, apoptosis, and angiogenesis. Cyr61 is highly expressed in breast cancer (Sánchez-Bailón et al., 2015; Mayer et al., 2017; Yang et al., 2018), and is without a doubt associated with expression stage, tumor size, positive lymph nodes and age (Xie et al., 2001). The analysis indicated that blocking Cyr61 might be a potent method for TNBC breast cancer treatment. We previously reported how metapristone inhibited the adhesion and migration of MDA-MB-231 breast cancer cells through EMT-related pathway (Yu et al., 2016). However, the other mechanism remains largely unknown. In the present study, the effect of metapristone induction on Cyr61 activity to interfere cell adhesion and migration was examined by the transfection of siRNA-Cyr61/pcDNA3.1-Cyr61 into MDA-MB-231 (see Supplementary Information). Our results showed that overexpression/knockdown of Cyr61 significantly increase/decrease adhesion and migration of MDA-MB-231 cells, respectively (Figure 2).
A number of the activities of Cyr61 can be attributed to its interaction with integrin receptors (Kireeva, 1998; Jedsadayanmata et al., 1999; Chen et al., 2000; Grzeszkiewicz et al., 2001; Schober et al., 2002). For example, primary human skin fibroblasts adhesion to Cyr61 is dependent on integrin α6β1 (Leu et al., 2003), and activation-dependent adhesion of blood platelets to Cyr61 is mediated through interaction with integrin α2β3 (Jedsadayanmata et al., 1999). To understand Cyr61’s action in MDA-MB-231/HPMEC co-cultures, we sought to identify the cellular adhesion receptor(s) through which Cyr61 may function. We provided the first demonstration of the identification integrin αvβ1 as a novel receptor for Cyr61 in MDA-MB-231/HPMEC co-cultures by immunoprecipation combined with western blot analysis (Figure 3). The expressions of Cyr61, integrin αv, and integrin β1 on the protein and mRNA levels were all down-regulated by metapristone in a dose-dependent manner. Furthermore, metapristone inhibited the formation of Cyr61/integrin αvβ1 complex, which are correlated with cell adhesion and migration (Figure 4). Moreover, the circulating MDA-MB-231, developing lung metastasis in mice, could be effectively prevented by oral metapristone without significant toxicity ( Figure 5). Also, our studies demonstrated the obvious inhibition effect on the expressions of Cyr61 and integrin αvβ1 in lung tissues after metapristone treatment.
Taken together, we have demonstrated for the first time that co-incubation of triple-negative breast cancer cell line MDA-MB-231 with HPMEC promotes the secretion of Cyr61 (CCN1), primarily from MDA-MB-231, which forms Cyr61/integrin αvβ1 complex. Moreover, our data show that metapristone, a new chemopreventive, has the ability to inhibit TNBC cells adhesion and migration through down-regulation of Cyr61 and the formation of Cyr61/integrin αvβ1 complex (Figure 6). Our data provide more details in understanding metastasis mechanism of microenvironment of tumor, especially under the tumor cells/endothelial cells co-culture condition, offer a cache of potential therapeutic targets, and more importantly, provide a molecular framework for clinical evaluation of metapristone as a potential cancer metastatic chemopreventive agent.
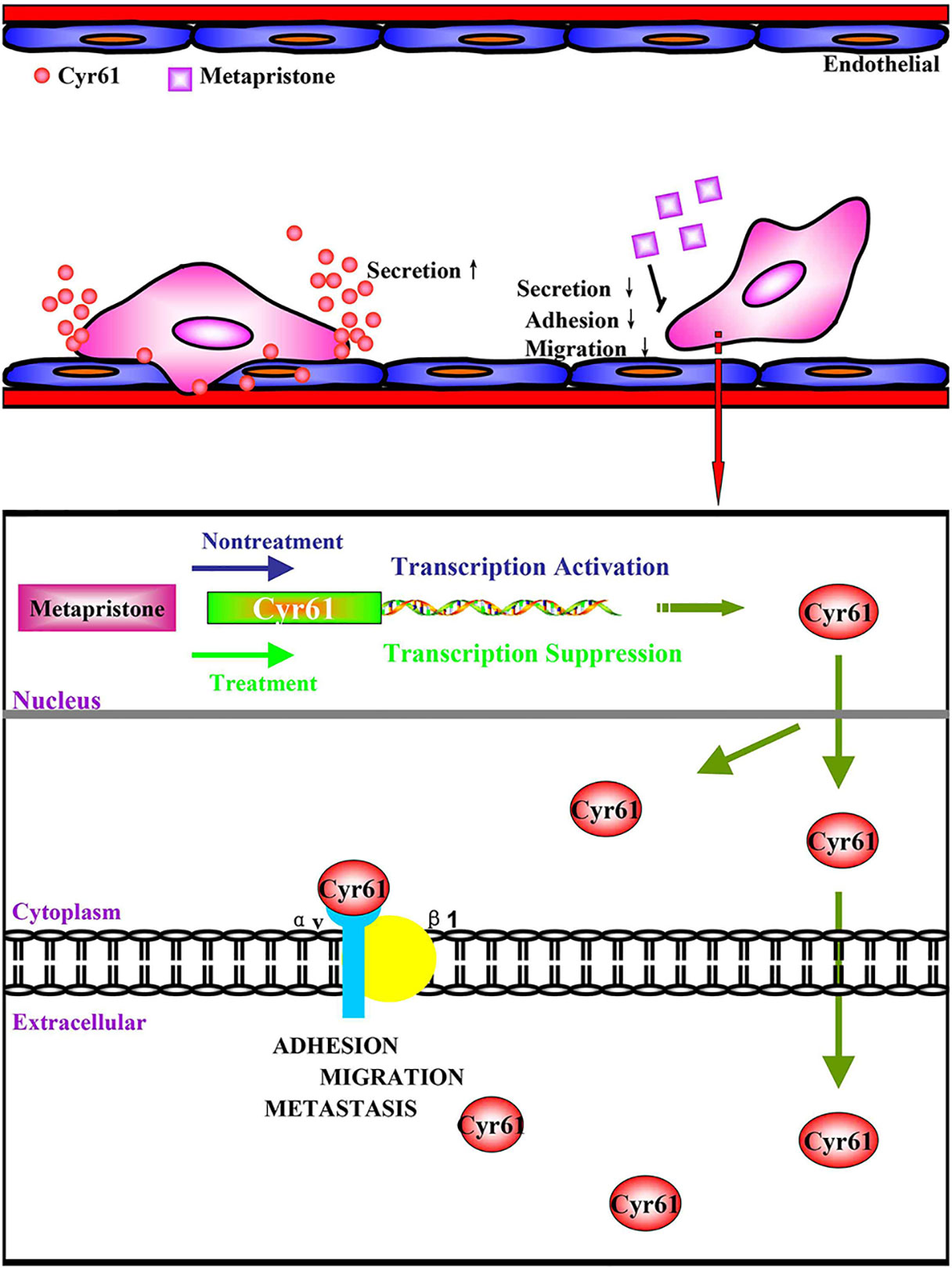
Figure 6 (A) possible mechanism of Cyr61 secretion and its inhibition by metapristone. Activated CTCs adhere to endothelial cells in the metastatic microenvironment, and the adhesion induces hetero-cellular communication and the resultant secretion of Cyr61 from CTCs, which forms Cyr61/integrin avβ1 complex to advance the adhesion/invasion metastasis. Metapristone inhibits the Cyr61 secretion, and the related adhesion/invasion process; (B) quantitative comparison in mouse lung tumor nodules between the control and metapristone groups (n = 5/group); (C) inhibition rate of mouse lung tumor metastasis after metapristone treatment; (D) hematoxylin–eosin staining of the lungs (amplification× 5); the arrows indicate metastatic foci that were significantly reduced by metapristone; (E) lung immunostaining with antibodies against Cyr61, integrin αv and integrin β1; the staining showed reduction in Cyr61/integrin avβ1 formation by metapristone.
Data Availability Statement
All datasets generated for this study are included in the article/Supplementary Material.
Ethics Statement
The animal study was reviewed and approved by The Experimental animal ethics committee, Fuzhou University.
Author Contributions
LJ and SY conceived and designed the experiments. SY and CY performed the pharmacoproteomic analysis. WW, SH, ML, JL, and YL carried out the cell biology experiments. XY and JM performed the animal experiments. CY and WW acquired and drew the pictures. LJ and SY wrote the manuscript. All authors read and approved the final manuscript.
Conflict of Interest
The authors declare that the research was conducted in the absence of any commercial or financial relationships that could be construed as a potential conflict of interest.
Acknowledgments
This work was supported by grants from National Natural Science Foundation of China (NSFC) U1505225, 81502617, 81273548; the grant from Ministry of Science and Technology of China (MOST 2015CB931804); the grant from Fujian Development and Reform Commission (project#829054, 2014/168), Fujian Science and Technology plan project (2015Y0071), the financial support from the program of China Scholarships Council (No. 201706655014), and China Postdoctoral Science Foundation (2015M582027).
The authors thank the Department of Chemistry and the Institute of Biomedical Science, Fudan University, Peoples Republic of China, for technical support.
Supplementary Material
The Supplementary Material for this article can be found online at: https://www.frontiersin.org/articles/10.3389/fphar.2019.01296/full#supplementary-material
References
Adav, S. S., Li, A. A., Manavalan, A., Punt, P., Sze, S. K. (2010). Quantitative iTRAQ secretome analysis of Aspergillus niger reveals novel hydrolytic enzymes. J. Proteome Res. 9, 3932–3940. doi: 10.1021/pr100148j
Akram, M., Iqbal, M., Daniyal, M., Khan, A. U. (2017). Awareness and current knowledge of breast cancer. Biol. Res. 50, 33. doi: 10.1186/s40659-017-0140-9
Astier, A. (2010). Recent developments of pharmacogenomics in the treatment of colorectal cancers. Annales Pharmaceutiques Françaises. 68, 233–253. doi: 10.1016/j.pharma.2010.04.001
Barrios, C. H., Reinert, T., Werutsky, G. (2018). Global breast cancer research: moving forward. Am. Soc. Clin. Oncol. Educ. Book. 38, 441–450. doi: 10.1200/EDBK_209183
Bonin-Debs, A. L., Boche, I., Gille, H., Brinkmann, U. (2004). Development of secreted proteins as biotherapeutic agents. Expert Opin. Biol. Therapy. 4, 551–558. doi: 10.1517/14712598.4.4.551
Chen, N., Chen, C. C., Lau, L. F. (2000). Adhesion of human skin fibroblasts to Cyr61 is mediated through integrin alpha 6beta 1 and cell surface heparan sulfate proteoglycans. J. Biol. Chem. 275, 24953–24961. doi: 10.1074/jbc.M003040200
Conlon, G. A., Murray, G. I. (2019). Recent advances in understanding the roles of matrix metalloproteinases in tumour invasion and metastasis. J. Pathol. 247, 629–640. doi: 10.1002/path.5225
Crockett, J. C., Schütze, N., Tosh, D., Jatzke, S., Duthie, A., Jakob, F., et al. (2007). The matricellular protein CYR61 inhibits osteoclastogenesis by a mechanism independent of alphavbeta3 and alphavbeta5. Endocrinology. 148, 5761–5768. doi: 10.1210/en.2007-0473
Crockett, J. C., Schütze, N., Tosh, D., Jatzke, S., Duthie, A., Jakob, F. (2010). Matricellular protein CCN1 activates a proinflammatory genetic program in murine macrophages. J. Immunol. 184, 3223–3232. doi: 10.4049/jimmunol.0902792
D’Antonio, K. B., Schultz, L., Albadine, R., Mondul, A. M., Platz, E. A., Netto, G. J., et al. (2010). Decreased expression of Cyr61 is associated with prostate cancer recurrence after surgical treatment. Clin. Cancer Res. Official J. Am. Assoc. Cancer Res. 16, 5908–5913. doi: 10.1158/1078-0432.CCR-10-1200
Devis, L., Moiola, C. P., Masia, N., Martinez-Garcia, E., Santacana, M., Stirbat, T. V., et al. (2017). Activated leukocyte cell adhesion molecule (ALCAM) is a marker of recurrence and promotes cell migration, invasion, and metastasis in early-stage endometrioid endometrial cancer. J. Pathol. 241, 475–487. doi: 10.1002/path.4851
Dotan, E., Cohen, S. J., Alpaugh, K. R., Meropol, N. J. (2009). Circulating tumor cells: evolving evidence and future challenges. Oncologist. 14, 1070–1082. doi: 10.1634/theoncologist.2009-0094
Gilkes, D. M., Semenza, G. L., Wirtz, D. (2014). Hypoxia and the extracellular matrix: drivers of tumour metastasis. Nat. Rev. Cancer. 14 (6), 430–439. doi: 10.1038/nrc3726
Grzeszkiewicz, T. M., Kirschling, D. J., Chen, N., Lau, L. F. (2001). CYR61 stimulates human skin fibroblast migration through integrin αvβ5 and enhances mitogenesis through integrin αvβ3, independent of its carboxyl-terminal Domain. J. Biol. Chem. 276, 21943–21950. doi: 10.1074/jbc.M100978200
Grzeszkiewicz, T. M., Lindner, V., Chen, N., Lam, S. C., Lau, L. F. (2002). The Angiogenic factor cysteine-rich 61 (CYR61, CCN1) supports vascular smooth muscle cell adhesion and stimulates chemotaxis through integrin alpha(6)beta(1) and cell surface heparan sulfate proteoglycans. Endocrinology. 143, 1441–1450. doi: 10.1210/endo.143.4.8731
Heikinheimo, O., Haukkamaa, M., Lähteenmäki, P. (1989). Distribution of RU486 and its demethylated metabolites in humans. J. Clin. Endocrinol. Metabolism 68, 270. doi: 10.1210/jcem-68-2-270
Holbourn, K. P., Acharya, K. R., Perbal, B. (2008). The CCN family of proteins: structure–function relationships. Trends Biochem. Sci. 33, 461–473. doi: 10.1016/j.tibs.2008.07.006
Hou, C. H., Lin, F. L., Hou, S. M., Liu, J. F. (2014). Cyr61 promotes epithelial-mesenchymal transition and tumor metastasis of osteosarcoma by Raf-1/MEK/ERK/Elk-1/TWIST-1 signaling pathway. Mol. Cancer 13, 236. doi: 10.1186/1476-4598-13-236
Jandova, J., Beyer, T. E., Meuillet, E. J., Watts, G. S. (2012). The matrix protein CCN1/CYR61 is required for αVβ5-mediated cancer cell migration. Cell Biochem. Function. 30, 687–695. doi: 10.1002/cbf.2853
Jedsadayanmata, A., Chen, C. C., Kireeva, M. L., Lau, L. F., Lam, S. C. (1999). Activation-dependent adhesion of human platelets to Cyr61 and Fisp12/mouse connective tissue growth factor is mediated through integrin alpha(IIb)beta(3). J. Biol. Chem. 274, 24321–24327. doi: 10.1074/jbc.274.34.24321
Kireeva, M. L. (1998). Adhesion of human umbilical vein endothelial cells to the immediate-early gene product Cyr61 is nediated through integrin alpha v beta 3. J. Biol. Chem. 273, 3090–3096. doi: 10.1074/jbc.273.5.3090
Laganà, A., Veneziano, D., Russo, F., Pulvirenti, A., Giugno, R., Croce, C. M., et al. (2015). Computational design of artificial RNA molecules for gene regulation. Methods Mol. Biol. 1269, 393–412. doi: 10.1007/978-1-4939-2291-8_25
Lambert, A. W., Pattabiraman, D. R., Weinberg, R. A. (2017). Emerging biological principles of metastasis. Cell. 168, 670–691. doi: 10.1016/j.cell.2016.11.037
Landemaine, T., Jackson, A., Bellahcène, A., Rucci, N., Sin, S., Abad, B. M., et al. (2008). A six-gene signature predicting breast cancer lung metastasis. Cancer Res. 68, 6092–6099. doi: 10.1158/0008-5472.CAN-08-0436
Lau, L. F. (2011). CCN1/CYR61: the very model of a modern matricellular protein. Cell. Mol. Life Sci. 68, 3149–3163. doi: 10.1007/s00018-011-0778-3
Leu, S. J., Liu, Y., Chen, N., Chen, C. C., Lam, S. C., Lau, L. F. (2003). Identification of a novel integrin α6β1 binding site in the angiogenic inducer CCN1 (CYR61). J. Biol. Chem. 278, 33801–33808. doi: 10.1074/jbc.M305862200
Lin, J., Huo, R., Wang, L., Zhou, Z., Sun, Y., Shen, B., et al. (2012). A novel anti-Cyr61 antibody inhibits breast cancer growth and metastasis in vivo.Cancer Immunol. Immunotherapy. 61, 677–687. doi: 10.1007/s00262-011-1135-y
Mayer, S., Erbes, T., Timme-Bronsert, S., Jaeger, M., Rücker, G., Kuf, F., et al. (2017). Clinical relevance of Cyr61 expression in patients with hormone-dependent breast cancer. Oncol. Lett. 14, 2334–2340. doi: 10.3892/ol.2017.6406
Mbeunkui, F., Fodstad, O., Pannell, L. K. (2006). Secretory protein enrichment and analysis: an optimized approach applied on cancer cell lines using 2D LC-MS/MS. J. Proteome Res. 5, 899–906. doi: 10.1021/pr050375p
Miller, I. V., Grunewald, T. G. P. (2015). Tumour-derived exosomes: tiny envelopes for big stories. Biol. Cell. 107, 287–305. doi: 10.1111/boc.201400095
Nguyen, N., Kuliopulos, A., Graham, R. A., Covic, L. (2006). Tumor-derived Cyr61(CCN1) promotes stromal matrix metalloproteinase-1 production and protease-activated receptor 1-dependent migration of breast cancer cells. Cancer Research. 66, 2658–2665. doi: 10.1158/0008-5472.CAN-05-2082
Oskarsson, T., Acharyya, S., Zhang, X. H., Vanharanta, S., Tavazoie, S. F., Morris, P. G., et al. (2011). Breast cancer cells produce tenascin C as a metastatic niche component to colonize the lungs. Nat. Med. 17, 867–874. doi: 10.1038/nm.2379
Planque, N., Perbal, B. (2003). A structural approach to the role of CCN (CYR61/CTGF/NOV) proteins in tumourigenesis. Cancer Cell International. 3, 15. doi: 10.1186/1475-2867-3-15
Rodvold, J. J., Zanetti, M. (2016). Tumor microenvironment on the move and the Aselli connection. Sci. Signaling. 9, fs13. doi: 10.1126/scisignal.aag2279
Sánchez-Bailón, M. P., Calcabrini, A., Mayoral-Varo, V., Molinari, A., Wagner, K. U., Losada, J. P., et al. (2015). Cyr61 as mediator of Src signaling in triple negative breast cancer cells. Oncotarget 6, 13520–13538. doi: 10.18632/oncotarget.3760
Schober, J. M., Chen, N., Grzeszkiewicz, T. M., Jovanovic, I., Emeson, E. E., Ugarova, T. P., et al. (2002). Identification of integrin alpha(M)beta(2) as an adhesion receptor on peripheral blood monocytes for Cyr61 (CCN1) and connective tissue growth factor (CCN2): immediate-early gene products expressed in atherosclerotic lesions. Blood. 99, 4457–4465. doi: 10.1182/blood.v99.12.4457
Su, J. L., Chiou, J., Tang, C. H., Zhao, M., Tsai, C. H., Chen, P. S. (2010). CYR61 regulates BMP-2-dependent osteoblast differentiation through the {alphav}{beta3}integrin/integrin-linked kinase/ERK pathway. J. Biol. Chem. 285, 31325–31336. doi: 10.1074/jbc.M109.087122
Teng, Y. N., Dong, R. Q., Wang, B. J., Liu, H. J., Jiang, Z. M., Wei, C. M., et al. (2011). Determinations of mifepristone and its metabolites and their pharmacokinetics in healthy female Chinese subjects. Acta Pharmaceutica Sinica 46, 1241–1245. doi: 10.1210/jcem-68-2-270
Torre, L. A., Bray, F., Siegel, R. L., Ferlay, J., Lortet-Tieulent, J., Jemal, A. (2015). Global cancer statistics. CA Cancer J. Clin. 65, 87–108. doi: 10.3322/caac.21262
Valastyan, S., Weinberg, R. A. (2011). Tumor metastasis: molecular insights and evolving paradigms. Cell. 147, 275–292. doi: 10.1016/j.cell.2011.09.024
Wang, J., Chen, J., Wan, L., Shao, J., Lu, Y., Zhu, Y., et al. (2014). Synthesis, spectral characterization, and in vitro cellular activities of metapristone, a potential cancer metastatic chemopreventive agent derived from mifepristone (RU486). Aaps Journal. 16 (2), 289–298. doi: 10.1208/s12248-013-9559-2
Wein, L., Loi, S. (2017). Mechanisms of resistance of chemotherapy in early-stage triple negative breast cancer (TNBC). Breast. 34 Suppl 1, S27–S30. doi: 10.1016/j.breast.2017.06.023
Wu, P., Ma, G., Zhu, X., Gu, T., Zhang, J., Sun, Y., et al. (2017). Cyr61/CCN1 is involved in the pathogenesis of psoriasis vulgaris via promoting IL-8 production by keratinocytes in a JNK/NF-κB pathway. Clin. Immunol. 174, 53–62. doi: 10.1016/j.clim.2016
Xie, D., Miller, C. W., O’Kelly, J., Nakachi, K., Sakashita, A., Said, J. W., et al. (2001). Breast cancer. Cyr61 is overexpressed, estrogen-inducible, and associated with more advanced disease. J. Biol. Chem. 276, 14187–14194. doi: 10.1074/jbc.M009755200
Yang, R., Chen, Y., Chen, D. (2018). Biological functions and role of CCN1/Cyr61 in embryogenesis and tumorigenesis in the female reproductive system (Review). Mol. Med. Rep. 17, 3–10. doi: 10.3892/mmr.2017.7880
Yu, S., Yang, X., Zhu, Y., Xie, F., Lu, Y., Yu, T., et al. (2015a). Systems pharmacology of mifepristone (RU486) reveals its 47 hub targets and network: Comprehensive analysis and pharmacological focus on FAK-Src-Paxillin complex. Sci. Rep. 5, 7830. doi: 10.1038/srep07830
Yu, S., Cao, H., Shen, B., Feng, J. (2015b). Tumor-derived exosomes in cancer progression and treatment failure. Oncotarget. 6, 37151–37168. doi: 10.18632/oncotarget.6022
Yu, S., Yan, C., Yang, X., He, S., Liu, J., Qin, C., et al. (2016). Pharmacoproteomic analysis reveals that metapristone (RU486 metabolite) intervenes E-cadherin and vimentin to realize cancer metastasis chemoprevention. Sci. Rep. 6, 22388. doi: 10.1038/srep22388
Keywords: MDA-MB-231/HPMEC co-culture, metapristone, CYR61, integrin αvβ1, metastasis chemoprevention
Citation: Yu S, Yan C, Wu W, He S, Liu M, Liu J, Yang X, Ma J, Lu Y and Jia L (2019) RU486 Metabolite Inhibits CCN1/Cyr61 Secretion by MDA-MB-231-Endothelial Adhesion. Front. Pharmacol. 10:1296. doi: 10.3389/fphar.2019.01296
Received: 01 August 2019; Accepted: 10 October 2019;
Published: 20 November 2019.
Edited by:
Katrin Sak, NGO Praeventio, Tartu, EstoniaReviewed by:
Srinivas V. Koduru, Penn State Milton S. Hershey Medical Center, United StatesQiyang Shou, Zhejiang Chinese Medical University, China
Copyright © 2019 Yu, Yan, Wu, He, Liu, Liu, Yang, Ma, Lu and Jia. This is an open-access article distributed under the terms of the Creative Commons Attribution License (CC BY). The use, distribution or reproduction in other forums is permitted, provided the original author(s) and the copyright owner(s) are credited and that the original publication in this journal is cited, in accordance with accepted academic practice. No use, distribution or reproduction is permitted which does not comply with these terms.
*Correspondence: Lee Jia, cmapcjia1234@163.com
†These authors have contributed equally to this work