- 1Center of Medicine, All-Russian Research Institute of Medicinal and Aromatic Plants (VILAR), Moscow, Russia
- 2Division of Clinical Pharmacology, Department of Pediatrics, University of Utah School of Medicine, Salt Lake City, UT, United States
- 3Department of Pediatrics, Boonshoft School of Medicine, Dayton Children’s Hospital, Wright State University, Dayton, OH, United States
- 4Department of Pharmaceutics & Pharmaceutical Chemistry, College of Pharmacy, University of Utah, Salt Lake City, UT, United States
Humans used plants for thousand of years as food, drugs, or fuel to keep homes warm. People commonly used fruits and roots, and other parts of the plant were often wasted. This review aims to discuss the potential of rational stem-to-stern use of three highly versatile and valuable plants with hepatoprotective properties. Milk thistle (Silybum marianum L. Gaertn.), artichoke (Cynara cardunculus), and chicory (Cichorium intybus L.) have well-characterized hepatoprotective properties. These plants have been chosen since liver diseases are significant diseases of concern worldwide, and all parts of plants can be potentially utilized. Artichoke and chicory are commonly used as food or dietary supplements and less often as phytodrugs. Various dietary supplements and phytodrugs prepared from milk thistle (MT) fruits/seeds are well-known to consumers as remedies supporting liver functions. However, using these plants as functional food, farm animal feed, is not well-described in the literature. We also discuss bioactive constituents present in various parts of these plants, their pharmacological properties. Distinct parts of MT, artichoke, and chicory can be used to prepare remedies and food for humans and animals. Unused plant parts are potentially wasted. To achieve waste-free use of these and many other plants, the scientific community needs to analyze the complex use of plants and propose strategies for waste-free technologies. The government must stimulate companies to utilize by-products. Another problem associated with plant use as a food or source of phytodrug is the overharvesting of wild plants. Consequently, there is a need to use more active cultivation techniques for plants.
Introduction
Humans have been using plants as food and remedies for thousands of years. About 80% of the world’s population uses medicinal plants and phytodrugs to treat various diseases (Ekor, 2014). Based on ethnopharmacological studies conducted by Sun et al., plants have a critical role in people’s diets, with roots and fruits most commonly used. Currently, wild and cultivated plants are used by the food, pharmaceutical, and cosmetic industries (Schmidt, 2012; Nofal et al., 2019; Rahman et al., 2019). The demand for wild plants, specially harvested in ecologically clean areas, is growing. Therefore, overharvesting of wild plants can lead to their endangerment (United Plant Savers, 2021). Often distinct parts of the plant are used as food or medicine. This raises the question of what happens to by-products and whether it is possible to develop waste-free processes of plant handling. Parts of the plants that may be wasted can be used as a source of food, functional food, or feed for farm animals. Therefore, researchers urge companies to introduce ethically reasonable use of plants.
According to the encyclopedia Britannica, food is a “substance consisting essentially of protein, carbohydrate, fat, and other nutrients used in the body of an organism to sustain growth and vital processes and to furnish energy” (Britannica Encyclopaedia., 2020). The Japanese government first introduced the term “functional food” in the early 1980s (Martirosyan and Singh, 2015). Later functional food was introduced to the European and American markets (Martirosyan and Singh, 2015). A function food “may provide a health benefit beyond the traditional nutrients it contains” (Thomas and Earl, 1994). In 1994, a new category of natural products, a dietary supplement, was introduced to consumers (1994). The Dietary Supplement Health and Education Act (DSHEA) describes dietary supplements as preparations intended to supplement the diet and may contain plants, vitamins, minerals, amino acids, tissue from organs, enzymes, and probiotics (1994). Dietary supplements are usually minimally regulated by medical authorities. According to the United States Food and Drug Administration (US FDA), about 50% of United States adults regularly use medicinal plants/herbs (Gottlieb, 2019). Phytodrugs, another category of natural products, are purified extracts from various parts of the plant or single-molecule phytochemicals isolated from natural products. These products are tightly regulated by regulatory authorities worldwide (Enioutina et al., 2020). The US FDA considers the highly purified extracts prepared from medicinal plants as botanical drugs and similarly regulates them as conventional synthetic drugs (Enioutina et al., 2020).
The primary purpose of this article is to discuss the potential of rational “stem-to-stern” use of plants. To prove the potential of rational use, we have chosen three plants with confirmed hepatoprotective properties. These plants are milk thistle (MT, Silybum marianum L. Gaertn.), artichoke (Cynara cardunculus), and Chicory (Cichorium intybus L.) (Baginskaya et al., 2000; Abenavoli et al., 2010; Li et al., 2014; Ben Salem et al., 2019; Achufusi and Patel, 2020; Mukhtar et al., 2021; Perovic et al., 2021; Song et al., 2021). The term “hepatoprotective” is often used to describe the ability of a drug or plant to prevent liver damage, whereas “antihepatotoxic” drugs can prevent or treat liver damage done by hepatotoxic substances. In general, these terms are often used interchangeably. In this article, we have chosen to use the term “hepatoprotective” plants.
The chief reason for selecting these plants is that liver disorders, including cirrhosis, viral hepatitis, hepatocellular carcinoma, and drug-, heavy metal-, and alcohol-induced liver injuries, are significant diseases of concern worldwide (Rehm et al., 2013; Asrani et al., 2019; Bashir et al., 2021). One-third to nearly 90% of patients with liver diseases or cancer diagnoses used herbal products with hepatoprotective properties (Fenclova et al., 2019).
Another reason is that the consumers widely use these plants as food, food substitute, phytodrugs, or dietary supplements. Artichoke flowers are primarily used as food or dietary supplements, while wasted aerial parts have the potential to be used as phytodrug, animal feed, or biofuel (Fernández et al., 2006; Gostin and Waisundara, 2019; Barbosa et al., 2020). The artichoke is often added to the hepatoprotective proprietary blends along with MT. Chicory is also best known as food (e.g., coffee substitute) (Wu and Cadwallader, 2019) or dietary supplement (inulin, a prebiotic driven from chicory root) (Shoaib et al., 2016) and less known as a phytodrug. The chicory wasted aerial part is occasionally a part of the phytodrug and can be used as animal feed (Niness, 1999; Githiori et al., 2006; Kandeler and Ullrich, 2009; Himalaya Wellness Company., 2021b). Chicory is a part of a multi-component phytodrug developed by the Himalaya Drug Company (Himalaya Wellness Company., 2021a). Unlike the other two plants, milk thistle fruits/seeds are mainly used as phytodrugs or dietary supplements, but the remaining plant parts can also be used as food additives for animals and biofuel (Chabaev et al., 2011; Andrzejewska et al., 2015; Ataei Nukabadi et al., 2021). Several MT-based phytodrugs were marketed [e.g., Carsil (SoPharma, Bulgaria); and Legalon (Flordis, Australia)]. Currently, MT-based dietary supplements are in the top-40 best-selling herbal supplements (Smith et al., 2017).
Distinct parts of these plants have different compositions of nutrients and phytochemicals. For example, the bioactive constituents responsible for the hepatoprotective properties of MT are present in the highest concentration in plant fruits and seeds (Baginskaya et al., 2000; Vargas-Mendoza et al., 2014). The hepatoprotective phytochemicals are present in roots and aerial part of chicory (Thabrew et al., 1982; Huseini et al., 2005; Abenavoli et al., 2010; Cha et al., 2010; Abd El-Mageed, 2011).
The demand for phytodrugs and dietary supplements with hepatoprotective properties will grow. This may increase the exhaustion of wild-grown resources and waste parts of plants not used for drug/supplement preparation. This article will show the potential use of distinct parts of MT, artichoke, and chicory as food, phytodrugs, dietary supplements, functional food, and animal feed.
Methods
This extensive review (Munn et al., 2018) of existing literature and evaluation of the existing literature found numerous gaps in our knowledge related to the use of distinct parts of MT, chicory, and artichoke in food and pharmaceutical industries animal husbandry. The authors conducted a comprehensive search in six electronic databases (PubMed, Embase, Scopus, ScienceDirect, Web Science, and elibrary.ru), the All-Russian Institute of Aromatic and Medicinal Plants (VILAR) medical library, and other relevant medicinal herbs websites for literature published between January 1976 and May 2021. Searches were conducted using the keywords: artichoke, chicory, Silybum marianum, Cichorium intybus, phytodrugs, drugs, dietary supplements, food, functional food, and animal feed.
Milk Thistle
Milk thistle (MT, Silybum marianum L. Gaertn.,), a member of the Asteraceae family, is native to Southern Europe and Asia and widely naturalized in Europe, North America, South America, Australia, and New Zealand (Missouri Botanical Garden., 2019). It is not used actively in agriculture and is considered to be an invasive weed in North America, Australia, New Zealand, and South Africa (King County, 2018).
MT is best known for its hepatoprotective and antioxidant properties (Siegel and Stebbing, 2013) (Table 1). Bioactive compounds isolated from MT, specifically a bioactive flavonolignan, silymarin, have antioxidant properties, protect against damage by the free radicals generated from the metabolism of ethanol, acetaminophen, and carbon tetrachloride ingested in excessive amounts (Krepkova et al., 2008; Vargas-Mendoza et al., 2014). In addition, MT has anti-inflammatory, anti-cancer, antiviral, and immunomodulatory properties (Deak et al., 1990; Krepkova et al., 2008; Vargas-Mendoza et al., 2014) (Table 2). Carsil, Legalon, and Silimar are popular phytodrugs prepared from the fruit of MT (Brailski et al., 1986; Feher et al., 1989; Soleimani et al., 2019; Gillessen and Schmidt, 2020). Now, MT and plant extracts are immensely popular as dietary supplements (Table 1).
However, the use of MT as food, functional food for humans and animals is not well studied. Several publications describe the usefulness of bread or pastry fortification with MT fruits and oil and the supplementation to animal feed with bioactive compounds from the plant. It appears that plant stems and foliage are not used following phytodrugs and dietary supplements preparations and have the potential to be wasted.
Milk Thistle Bioactive Constituents and Their Biological Activity
Silymarin is the main bioactive constituent found in the MT fruit oilseed cake (Vargas-Mendoza et al., 2014) (Table 2). It is a complex flavonolignan responsible for the plant’s hepatoprotective properties. Silymarin is a complex flavonolignan comprising silybin A and B, isosilybin A and B, silydianin, silychristin, isosilychristin, and taxifolin, a flavonoid precursor (Pradhan and Girish, 2006; Vargas-Mendoza et al., 2014). The silibinin, a mixture of silybins A and B, is also named silibinin. The ratio of flavonolignan isomers may differ depending on the region where MT was grown (Lee et al., 2007; Poppe and Petersen, 2016). Flavonolignan’s concentrations can also be influenced by oil extraction technology from MT fruits. The cold-pressed oil extraction preserves a unique combination and activity of bioactive constituents present in the plant fruits (Hou et al., 2010; Lee et al., 2013). The MT oilseed cake or seeds are the primary raw material used by the pharmaceutical and dietary supplement companies to prepare phytodrugs and dietary supplements.
The hepatoprotective properties of silibinin include blocking stabilizing liver cell membranes and blocking phosphodiesterase (Romanova and Krasavtsev, 2007). In addition, silibinin can bind radicals due to its phenolic structure, resulting in a significant increase in the reduced glutathione content in the liver. This reduction in glutathione increases the organ’s protection from oxidative stress, maintaining its normal detoxification function.
Silymarin reduces inflammation via downregulation of the expression of transcription factors [nuclear factor-kappa B (NF-kB) and signal transducer and activator of transcription 1 (STAT-1)] and inflammation-associated proteins. The downregulation of transcription factors led to a significant reduction of interleukin 1 alpha (IL1α) and tumor necrosis factor-alpha (TNFα) production (Lee et al., 2013) (Table 2). It has also been reported that silibinin may suppress melanoma cell growth by reducing the phosphorylation of extracellular signal-regulated kinase (ERK)-1/2. The reduction of ERK-1/2 resulted in downregulation of the MEK1/2 and reduced activity of NF-κB and STAT-3, followed by the cell-cycle arrest in the G1 (Lee et al., 2013). Interestingly, silymarin, but not silibinin, could attenuate ischemia-reperfusion-induced brain injury (Hou et al., 2010). Silibinin also reduced chemotherapeutic drug resistance in human bladder cancer cell lines via NF-kB dependent and independent pathways.
Milk Thistle Hepatoprotective Properties
Bioactive flavonoids have been used to treat hepatobiliary system diseases (Flora et al., 1998; Pradhan and Girish, 2006; Abenavoli et al., 2010) (Table 1). Flavonolignans isolated from the fruit of MT prevail in this group of drugs. Studies have shown that the hepatoprotective effect of these phytodrugs are due to the presence of three flavonolignans: silibinin, silidianin, and silicristin (Abenavoli et al., 2010). Numerous preclinical studies were published demonstrating hepatoprotective properties of MT or MT extracts in animal models of liver injury. Only the PubMed database holds 311 articles, including review articles, published between 1989 and 2021. Several animal models of hepatotoxicity were used by investigators, including acetaminophen, alcohol, carbon tetrachloride, phenylhydrazine, and thioacetamide (Abenavoli et al., 2010; Kantah et al., 2011; Ahmed et al., 2020).
It has been reported that liver fibrosis in rats induced by the 8-weeks intragastric administration of carbon tetrachloride (CCl4) was successfully treated with a standardized extract of the MT (Tsai et al., 2008). The extract was administered at a 200 mg/kg dose four times per week for 3 weeks. The treatment has significantly decreased aspartate aminotransferase (AST), alanine aminotransferase (ALT), and alkaline phosphatase (ALP) in the serum of experimental animals.
A study confirming the hepatoprotective activity of Silimar, an original phytodrug developed by the VILAR, was conducted on a model of experimental hepatitis in rats induced by a single subcutaneous administration of oil-based CCl4 (Baginskaya et al., 2000; Sokol’skaya, 2000). Silimar at a dose of 100 mg/kg was administered 5 days before CCl4 administration and continued 21 days after liver damage. The administration of CCl4 resulted in a significant elevation of the γ-glutamyl transferase (GGT), ALP, ALT, and AST compared with healthy controls (Baginskaya et al., 2000). Following chronic administration of Silimar resulted in significantly reduced levels of GGT, ALT, AST. In an in vitro experiment, Silimar suppressed induced lipid peroxidation, evidenced by reducing the amount of malondialdehyde by 15–41% compared to the control (Baginskaya et al., 2000).
It appears that MT extracts have low bioavailability (El-Gazayerly et al., 2014; Mukhtar et al., 2021). The treatment of rats receiving CCl4 with silymarin incorporated into phytosomes significantly decreased superoxide dismutase activities and glutamic pyruvic transaminase compared to the plant extract free of phytosomes (El-Gazayerly et al., 2014). Additionally, the treatment with encapsulated silymarin inhibited liver fibrosis induced in rats by administering 2 mg/kg acetaminophen for 2 weeks (Mukhtar et al., 2021). The treatment significantly decreased levels of ALT, AST, and ALP.
Several investigators have studied the pharmacokinetics of MT in healthy volunteers and patients with various liver diseases. The study published by Calani et al. investigated the bioavailability and metabolism of flavonolignans after a single administration of the water-soluble MT extract (Calani et al., 2012). After overnight fasting, the healthy volunteers consumed 8 g of the extract dissolved in the water in this study. The study showed that MT flavonolignans have low bioavailability (0.45%). Urinary excretion was studied following 48 h. Thirty-one metabolites of flavonolignans have been found in the urine. The most common metabolites were monoglucuronides followed by sulfate–glucuronides and diglucuronides. Zhu et al. investigated the pharmacokinetics of individual flavonolignans in humans following single or chronic administration of MT extract (Zhu et al., 2013). The authors determined that all investigated flavonolignans were rapidly absorbed and eliminated by the organism. Flavonolignan exposure was dose-dependent. The most prevalent flavonolignans detected in volunteers were silybin A and silybin B, followed by isosilybin B and isosilybin A. The pharmacokinetics of MT flavonolignans was significantly altered in patients infected with Hepatitis C virus and patients with non-alcoholic fatty liver disease (Abenavoli et al., 2018). High exposure to bioactive flavonolignans was observed in patients with live cirrhosis.
Several MT phytodrugs are marketed under different brand names (e.g., Silymarin, Legalon, Carsil, and Silibor). Legalon is a standardized phytodrug containing 70–140 mg silymarin (calculated as silibinin) in 86–186 mg of dry extract of the fruit (European OTC medicines and devices., 2020). The phytodrug is intended to treat acute and chronic hepatitis and cirrhosis. Legalon at a 25 mg/kg dose reduced lipid peroxidation and increased superoxide dismutase activity in hepatic tissues (Batakov, 2001). It has been reported that workers chronically exposed to toluene and/or xylene vapors receiving oral Legalon for 30 days had significant improvements in liver function and platelet counts compared with workers that did not receive this treatment (Szilard et al., 1988). Legalon-SIL® has been used for intravenous treatment of patients infected with hepatitis C virus (HCV) and not responding to pegylated interferon/ribavirin treatment (Rutter et al., 2011). Most of the patients (85%) had undetectable HCV ribonucleic acid (RNA) after a 14-days course of Legalon-SIL®.
Many clinical trials were conducted to find the efficacy of MT extracts in humans with various liver diseases. The first trial that we were able to find was conducted in 1980 and investigated the effectiveness of the MT derivatives for the treatment of chronic hepatopathies (De Martiis et al., 1980). The first randomized placebo-controlled trial was performed in 1989 and investigated the efficacy of silymarin in patients with liver cirrhosis (Ferenci et al., 1989). The patients in this trial received 140 mg of silymarin three times a day for an extended period. It has been determined that the 4-years survival rate in the treatment group was significantly higher compared with patients receiving placebo. Another double-blind placebo-controlled clinical trial proved the efficacy of milk thistle for treating patients with acute hepatitis (El-Kamary et al., 2009). The therapy reduced symptoms of biliary retention such as jaundice and scleral icterus. Interestingly, unlike in animal experiments, the reduction of ALT and AST in human subjects was not significant (El-Kamary et al., 2009). The evaluation of the efficacy of silymarin for treatment of non-alcoholic steatohepatitis has shown that patients receiving 700 mg of silymarin for 48-weeks had comparable to control non-alcoholic fatty liver disease activity scores; however, silymarin-treated patients had significantly lower liver fibrosis (Wah Kheong et al., 2017).
A more detailed analysis of available clinical data confirming hepatoprotective properties of MT is available in the recently published articles by Abenavoli et al. (Abenavoli et al., 2018) and Marmouzi et al. (Marmouzi et al., 2021). The literature analysis led us to conclude that MT standardized extracts can improve liver function following several hepatic disorders, including chronic exposure to toxic chemicals, viral hepatitis, liver cirrhosis, and non-alcoholic liver steatosis. However, this treatment cannot cure the disease. In our opinion, this treatment can be combined with the standard of care therapies, especially when there is no improvement following conventional treatment.
Milk Thistle as Food and MT-Based Functional Food
MT oil, oilseed cake, or seeds could fortify bakery products (Semenkina, 2010; Ataei Nukabadi et al., 2021) (Table 1). MT fruits contain a complex of biologically active substances (vitamins, minerals, flavonoids, a significant amount of dietary fiber, and amino acids) (Semenkina, 2010). The MT seed oil is enriched with omega-6 and omega-3 fatty acids, tocopherols, and carotenoids. The addition of MT in bakery products may increase the nutritional value by supplying extra proteins, linoleic acid, vitamin E, and calcium. The presence of flavonolignans in the MT oilseed cake could supply additional support for liver function. MT flavonolignans may help increase bone calcium absorption when added to bakery products combined with fat-free milk (Semenkina, 2010; Nekrasova and Popov, 2020). The addition of nettle leaf and MT seed powder to sponge cakes could reduce blood sugar levels (Ataei Nukabadi et al., 2021) and improve liver functions.
Andrzejewska with colleague suggested that whole MT plant can be used as food and cosmetics (Andrzejewska et al., 2015). According to the Edible Wild Food resource, “the young stalks, leaves, roots and flowers can be eaten” (Edible Wild Food., 2021). Mediterranean communities have been using MT young stems as food for centuries (Andrzejewska et al., 2015). Spanish natives have been adding aerial parts to salads or eating them cooked (Andrzejewska et al., 2015). Unfortunately, the reports on the use of MT as food or functional food for humans are limited and primarily focused on the use of plant fruit, not aerial parts, potentially due to the presence of spikes on the leaves. MT may be an active ingredient of the cream intended to treat melasma (Nofal et al., 2019).
Milk Thistle as Animal Feed
The MT can also be used as a part of functional food for farm animals (Table 1). MT oilseed cake is enriched in crude proteins, dietary fiber, and fat (Stastnik et al., 2020). The seed cake contains ∼4% of flavonolignans (Stastnik et al., 2020). It has been reported that the addition of MT oilseed cake in the diet of lactating dairy cows in the amount of 25% of the required digestible protein increased the protein and fat content in the milk, improved its amino acid composition, and augmented the average daily milk yield (Chabaev et al., 2011). The administration of MT oilseed cake at a dose of 44 mg/kg/day to Ayrshire heifers during the first twenty-first months of their lives reduced the number of diseases caused by impaired liver function and metabolic processes by ∼35% and prevented mortality of the livestock from the first month of cattle life to the end first lactation (Kravainis et al., 2014). The supplementation of the sow diet with MT at the dose 100 mg/kg/day starting from 88 days of gestation to farrowing positively affected the fetal formation and the weight of newborn piglets (Kolesnyk and Bankovska, 2008). The addition of MT to the sow fodders prevented suckling pig’s mortality and increased the average daily gain by ∼19% (Kolesnyk et al., 2009). Chickens receiving MT extract at doses of 0.1; 1.0; 1.5 and 2.0 mg/kg had the average daily body weight gain during the rearing period by 1.1–5.3%, the European productivity index increased 2.4–10.2%, and feed consumption per 1 kg of the weight gain increased 1.6–4.8% compared to the control group (Bagno et al., 2020). Feeding MT oilseed cake in combination with ascorbic acid to broiler chickens reduced the concentration of lead and cadmium in meat by 2.72 and 2.08 times and increased its biological value (Mildzikhov et al., 2013). Adding oilseed cake to the hens’ feed increased egg production and egg size (Stastnik et al., 2020). Some studies reported no effect on chicken wellbeing (Stastnik et al., 2020). As shown above, the fortification of farm animals’ fodder with the MT oilseed cake has significantly improved animals’ survival.
It has been hypothesized that the aerial part of the plant can also be used to fortify farm animal feed (Andrzejewska et al., 2015). It has been reported that MT can be used as supplemental food in cattle and chicken (Bagno et al., 2020; Shemuranova and Garifullina, 2020). Unfortunately, the nutritional value of the MT areal part is lower than barley (Stastnik et al., 2020).
Artichoke
Cynara cardunculus L. is a member of the Asteraceae family. There are three taxonomic variants of the Cynara cardunculus species: Cynara cardunculus L., var. scolymus (L.) Fiori (globe artichoke), Cynara cardunculus L., var. altilis DC. (cultivating cardoon) and Cynara cardunculus L., var. sylvestris (Lamk), Fiori (wild cardoon) (Gostin and Waisundara, 2019). The plant is native to the Mediterranean area, and nowadays, it is cultivated in other countries (Gostin and Waisundara, 2019). Artichoke prefers hot and dry climates and can live in an adverse environment. The artichoke immature flower heads have a bitter-sweet flavor and are part of the diet in many countries.
Artichoke bioactive constituents have antibacterial, anti-malarian, antiviral (Hep C virus), anti-inflammatory, antioxidative, hepatoprotective, and metabolic effects (Ben Salem et al., 2015; Elsebai et al., 2016; Villarini et al., 2021) (Table 3). Constituents isolated from artichoke (e.g., cynaropicrin) are involved in the regulation of the NF-κB pathway and downregulate inflammatory cytokines (Elsebai et al., 2016). An extract of the artichoke leaves has anti-hypercholesterolemic and antioxidative properties in an in vivo model of rats with high-fat diet-induced obesity (Ben Salem et al., 2019). Prophylactic use of artichoke leaf and flower head extracts may also protect against cancer development (Abdel-Moneim et al., 2021). The preparations from artichoke can be added to cosmetics intended for prophylaxis of skin photoaging (D'Antuono et al., 2018).
Numerous companies market artichoke to improve liver function dietary supplement (Zeaiter et al., 2019; GistGear, 2021) (Table 1). The artichoke is often used in combination with MT and dandelion (GistGear, 2021). The immature flower is the most used part of the plant in the food industry. As a result, >80% of biomass can be wasted (Barbosa et al., 2020). The use of wasted artichoke biomass could produce phytodrugs, dietary supplements, biofuel, biodiesel, cellulose, and animal feed.
Artichoke Bioactive Constituents and Their Biological Activity
The artichoke plant has significant levels of polyphenols, sesquiterpene lactones, terpenoids, carotenoids, and chlorophylls (Turkiewicz et al., 2019; Zeaiter et al., 2019; Rocchetti et al., 2020) (Table 3). The artichoke is an excellent source of inulin, minerals (e.g., potassium and phosphorus), and vitamins (vitamins group B and vitamin C) (Bundy et al., 2008; Zeaiter et al., 2019). Levels of phytochemicals can vary significantly depending on the taxonomic variant, cultivar, or hybrid plant (Turkiewicz et al., 2019). The artichoke bracts have elevated levels of inulin and polyphenols (Turkiewicz et al., 2019). The analysis of phytochemicals in the seed-propagated artichoke hybrids revealed that leaves of artichoke contain >90 polyphenols and >120 sesquiterpene lactones (Rocchetti et al., 2020). Seven cultivars were investigated by Rocchetti and others (Rocchetti et al., 2020). The authors reported significant differences in the composition of bioactive compounds present in these cultivars. As proved by Turkiewicz and others, the health-promoting benefits of artichoke depended on the levels of bioactive phytochemicals present in cultivars and hybrids (Turkiewicz et al., 2019).
The preclinical studies investigating pharmacological properties of the artichoke leaf compounds have found that the hepatoprotective properties of the phenolic compounds (e.g., luteolin, chlorogenic and caffeic acids, cynarin and luteolin 7-O-glucoside) are associated with their ability to reduce plasma levels of malondialdehyde induced by t-BHP and as a result decrease of free radicals and hepatocyte damage (Ben Salem et al., 2015) (Table 3). The phenolic leaf compounds also prevent lipid peroxidation. Additionally, phenolic compounds may exhibit hypocholesterolemic, anti-cholestatic, and antimicrobial effects (Ben Salem et al., 2015). The treatment of volunteers with total plasma cholesterol levels 6.0–8.0 mmol/l with artichoke leaf extract (ALE) significantly decreased total cholesterol levels, but not low-density lipoprotein and high-density lipoprotein (Bundy et al., 2008). An in vitro study demonstrated that treatment of HT-29 and RKO tumor cells with cynaropicrin, caffeoylquinic acids, and chlorogenic acid-induced cell apoptosis suggests that ALEs could be potentially used as an effective tool chemotherapeutic agent (Villarini et al., 2021) (Table 3).
Artichoke Hepatoprotective Properties
The hepatoprotective properties of the artichoke were actively studied in animal models of acute and chronic liver injury (Tables 1, 3). In 1987, Adzed and others showed that cynarine and caffeic acid possess cytoprotective properties in vitro experiments (Adzet et al., 1987). The ethanolic ALE was given to experimental rats for 60 days, and a high-fat diet was significantly reduced by high-fat diet levels of ALT, AST, and ALP (Ben Salem et al., 2019). Another study reported that the ALE treatment of rats with diazinon-induced liver injury reduced levels of ALT, AST, ALT, and TNFα gene expression in hepatocytes (Ahmadi et al., 2019). The treatment of rats with ALE for 2 weeks improved ALT and AST levels after CCl4 hepatic injury and decreased DNA fragmentation and caspase 3 levels in liver tissues of CCl4-exposed rats (Colak et al., 2016). While most of the studies evaluating the hepatoprotective properties of the artichoke were done using leaf extracts. Sumer and others investigated the hepatoprotective properties of artichoke stems and bracts in an animal model of acetaminophen-induced liver injury in rats (Sumer et al., 2020). They found that stem and bract extract also effectively reduced ALT and AST levels but not ALP. It appears that the intensity of hepatoprotective effects of artichoke extracts is associated with the levels of phenolic compounds present in the extract (Speroni et al., 2003).
Artichoke extracts are marketed as phytodrugs for the treatment of liver disorders (Lattanzio et al., 2009). A double-blind clinical trial in patients with non-alcoholic liver injury confirmed the results of preclinical studies. Patients receiving ALE 600 mg daily for 2 months had an increased hepatic vein flow, reduced portal vein diameter, and improved lipid and hepatic enzyme profiles (Panahi et al., 2018). Another trial investigated the efficacy of a standardized ALE in patients with chronic Hep C infection (Huber et al., 2009). Unfortunately, the treatment of patients for 12 weeks with ALE 3,200 mg/day did not improve hepatic enzymes levels while reducing the patient’s fatigue and joint problems.
The reviewed literature confirmed that the artichoke extracts from leaves and other parts of the plant exhibit strong hepatoprotective properties (Table 1). More clinical trials are needed to verify the hepatoprotective properties of artichoke extracts in humans.
Artichoke Use as Food or Functional Food
The artichoke was used as food from ancient times in Egypt, Greece, and Rome. Immature flowers and stems were used to prepare dishes, while mature flowers coagulate milk (Gostin and Waisundara, 2019) (Table 1). Modern-day artichoke flowers are exceedingly popular worldwide as a part of the everyday diet or delicatessen food. Immature flowers can be used fresh and canned or frozen for future use (Gostin and Waisundara, 2019). The artichoke was traditionally used in Mediterranean cuisine to prepare salads, dips, or soups (Barbosa et al., 2020). It can also be roasted with garlic and olive oil. The artichoke flowers and stigmas are used as a vegetable alternative to animal-driven rennet (Barbosa et al., 2020). The ability to clot milk is attributed to two enzymes mature artichoke flowers, cardosins A and B. The artichoke seed oil is enriched by linoleic, oleic, palmitic, and stearic fatty acids (Barbosa et al., 2020). The oil obtained from artichoke seeds is similar in composition to sunflower oil and can be used for human consumption and as a dietary supplement.
Artichoke is a rich source of non-digestible inulin, a heterogenous fructose polymer (Niness, 1999; Nwafor et al., 2017) and oligofructose, a shorter-chain oligomer, belonging to the inulin subgroup, which can be used as a part of dietary supplement blends or can be added to food to enhance its health benefits (Lattanzio et al., 2009). Inulin is well-known prebiotic that can support grows of a healthy intestinal microbiome. Antioxidant properties of phenolic compounds can be used in a functional food to support immune, endocrine, and cardiovascular systems (Table 3).
The biomass that is not used by the food industry could be a source of animal feed or feed supplement for farm animals, especially in areas of extensive artichoke cultivation. Silage artichoke (e.g., artichoke bracts and plants) has been investigated as an animal feed with generally positive results for animals and animal products (Meneses et al., 2007; Monllor et al., 2020) (Table 1). The analysis of artichoke foliage revealed that it is safe to use as animal feed.
Artichoke By-Products Use
A significant part of the artichoke is wasted after the food industry uses artichoke flowers. The alternative use of the artichoke aerial part is presented in Figure 1. It has been proposed to use remaining lignocellulosic biomass as solid biofuel for heating houses or energy generation (Fernández et al., 2006; Barbosa et al., 2020). Several studies evaluated the heating value and combustion of artichoke biomass (Fernández et al., 2006; Oliveira et al., 2012; Gominho et al., 2018). Artichoke biomass and oil can be used for biodiesel production (Fernández et al., 2006). A bioplastic prepared from a plant’s leaves can potentially replace slowly degrading plastic (Mirpoor et al., 2022). Gominho et al. suggested using artichoke stalks as a source for paper production (Gominho et al., 2001). It appears that the artichoke has good potential to be used from stem-to-stern in food, pharmaceutical, and bioenergetic industries. The use of waste by food industry by-products could have a significant impact on the environment.
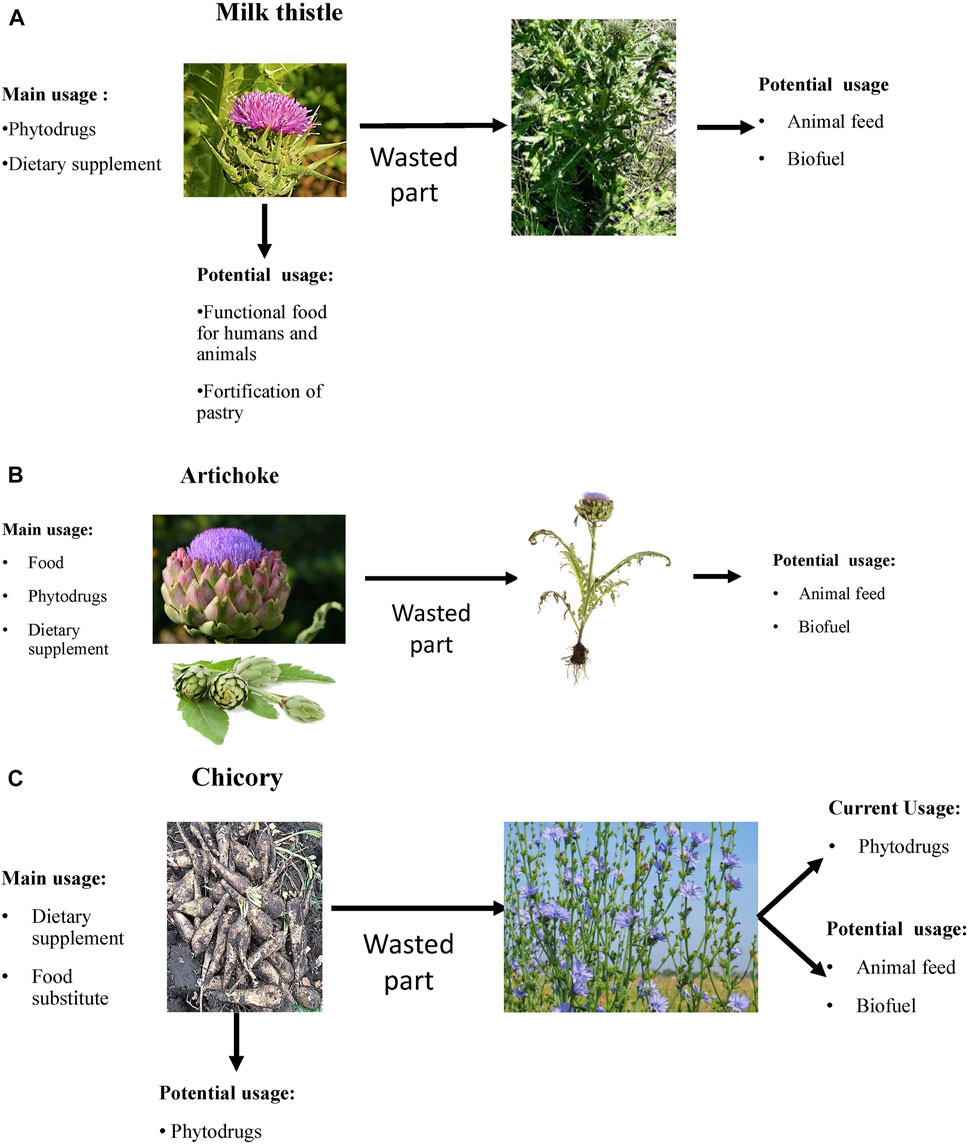
FIGURE 1. The rational use of plants with hepatoprotective properties. (A) Milk thistle; (B) Artichoke; (C) Chicory.
Chicory
Chicory, Cichorium intybus L., a member of the Asteraceae family, is a well-known plant cultivated in Europe, Asia, Canada, the eastern part of the United States, northern Africa, and Australia (Kandeler and Ullrich, 2009). Practically, all plant parts are used as a source of food, phytodrugs, and dietary supplements (Perovic et al., 2021) (Table 1). In the past, fresh plant roots and young leaves were used as vegetables (Kandeler and Ullrich, 2009; Al-Snafi, 2016; Perovic et al., 2021). The plant was used to treat pulmonary and reproductive systems diseases, the biliary tract, hepatic disorders, diarrhea, and cancer (Al-Snafi, 2016). The hepatoprotective activities of chicory have been established in animals and humans (Sama et al., 1976; Saxena and Garg, 1979; Thabrew et al., 1982; Cimen et al., 2020). Inulin, a chicory root constituent, is extremely popular among consumers as a prebiotic and source of dietary fiber (Shoaib et al., 2016). It is sold as a dietary supplement worldwide. Additionally, chicory root and root bioactive constituent, inulin, are often used in the food industry as a coffee substitute or substitute for fat and sugar in pastry and ice cream (Kandeler and Ullrich, 2009; Shoaib et al., 2016; Wu and Cadwallader, 2019).
Chicory Bioactive Constituents and Their Biological Activity
The chicory plant contains numerous biologically active constituents, including inulin, sesquiterpene lactones (e.g., chicoroisides B and C, sonchuside C), flavonoids, alkaloids, caffeic acid derivatives (e.g., chicoric acid, chlorogenic acid), vitamins E, β-carotene, and minerals (calcium, phosphorus, magnesium, and potassium) (Al-Snafi, 2016; Perovic et al., 2021) (Table 4). The distinct parts of the plant may contain different amounts of bioactive constituents. Plant roots are enriched in inulin and tannins but contain low amounts of phenolic acids (Al-Snafi, 2016). Inulin comprises ∼70% of constituents present in the fresh chicory root (Nwafor et al., 2017). The leaves and seeds contain high levels of phenolic compounds and flavonoids and low levels of inulin (Al-Snafi, 2016). Both wild and cultivated chicory is used in the food and dietary supplement industries. The aerial part of the plant may be used as a raw material for hepatoprotective phytodrugs, while the food and dietary supplement industries use roots.
Chicory is reported to have several pharmacological properties, including antimicrobial, anthelmintic, antimalarial, anti-inflammatory, antioxidant, gastroprotective, and hepatoprotective (Street et al., 2013; Al-Snafi, 2016; Janda et al., 2021; Perovic et al., 2021) (Table 1). In addition, chicoric acid has hypoglycemic activity and antiviral effects (Zhang et al., 2014; Ferrare et al., 2018). Aqueous extracts from cultivated chicory possess antioxidant properties that were confirmed in animal models (Saybel', 2020). Hepatoprotective properties were reported for flavonoids isolated from Cichorium glandulosum Boiss. et Huet (Tong et al., 2015) (Table 4). Phenolic flavonoids extracted from leaves of chicory and artichoke also demonstrated hepatoprotective properties (Mohafrash and Mossa, 2020). The constituents present in the chicory extract are oxycoumarins: esculetin and cichoriin, hydroxycinnamic acids: chicory, chlorogenic, caftaric acids (Petropoulos et al., 2017; Saybel', 2020) (Table 4). These constituents have antioxidant and hepatoprotective properties. It is suggested that the complex biologically active constituents such as polyfructose, hydroxycinnamic acids, coumarins, and flavonoids, responsible for the plant’s hepatoprotective properties (Domitrovic and Potocnjak, 2016).
A double-blind, randomized controlled trial conducted in patients with chronic periodontitis has determined that treatment with 1 g of the chicory leaf extract decreases serum levels of total cholesterol, low-density lipoprotein cholesterol, and triglycerides, while increased serum levels of high-density lipoprotein cholesterol, uric acid, and total antioxidant capacity (Babaei et al., 2018). Additionally, the authors reported that the treatment decreased periodontal pocket depth.
Several clinical trials were conducted to evaluate inulin or chicory coffee’s effects on various human organ and system functions (e.g., lipid profile, bowel movement, and platelet aggregation Schumacher et al., 2011; Grela et al., 2014; Buddington et al., 2017). One of these studies evaluated whether chicory coffee has any beneficial effects on the cardiovascular system (Schumacher et al., 2011). The consumption of chicory coffee for 1 week resulted in a significant decrease in whole blood, red blood cell deformability, plasma viscosity, and macrophage migration inhibitory factors. A more recent randomized crossover trial has determined that supplementation of diets with chicory inulin contacting snack bars of people with low fiber intake led to an increase of beneficial members of the human intestinal microbiome, Bifidobacterium genus (Reimer et al., 2020).
Chicory Hepatoprotective Properties
Cichorium intybus is a part of a phytodrug (Liv-52) introduced to the pharmaceutical market by the Himalaya Drug Company in 1955 (Himalaya Wellness Company., 2021b). Liv-52 includes Capparis spinosa, Cichorium intybus, Solanum nigrum, Cassia occidentalis, Terminalia arjuna, Achillea millefolium, and Tamarix gallica (Huseini et al., 2005; Himalaya Wellness Company., 2021b). Currently, Liv-52 and its modifications are widely used as dietary supplements in the United States and European countries. Liv-52 prevents the loss of functional integrity of the cell membrane, maintains cytochrome P-450 activity, and promotes hepatocellular regeneration (Himalaya Wellness Company., 2021b). The high hepatoprotective properties of this phytodrug were confirmed in patients with viral hepatitis, alcoholic and fatty liver, cirrhosis, anorexia (Sama et al., 1976; Huseini et al., 2005). Patients receiving Liv-52 for six consecutive months in a double-blind placebo-controlled trial had decreased ascites and significantly lower ALT and AST levels (Huseini et al., 2005). Huseini et al. attributed the hepatoprotective properties of the phytodrug to its antioxidant, anti-inflammatory, and immunomodulatory properties (Huseini et al., 2005). Recently, it has been proven that Liv-52 could treat ischemia reperfusion-induced liver damage in rats (Cimen et al., 2020).
Another clinical trial reported that supplementation of the diets of patients with non-alcoholic fatty liver disease for 12 weeks with a mixture of turmeric and chicory seeds resulted in a significant decrease of participants’ BMI and waist circumference and levels of serum alkaline phosphatase (Ghaffari et al., 2019).
We recently presented data demonstrating hepatoprotective properties of standardized dry extract obtained from the aerial part of wild chicory (Cichorium intybus L., DEC) (Bortnikova et al., 2021). DEC was developed at the VILAR. The analysis of the chemical composition revealed that the primary constituents present in DEC are phenol carboxylic acids [e.g., esters of caffeic, ferulic, coumaric acids with organic acids (quinic and tartaric)], flavonoids (isoquercetin, astragalin, rutin, luteolin, and kaempferol), and oxycoumarins (esculetin, cichoriin) (Saybel', 2020). The DEC contains 9.20 ± 0.43% of phenolic constituents calculated as chicoric acid.
DEC has significant hepatoprotective properties confirmed in a model of acute toxic hepatitis induced by a single subcutaneous injection of mercuric chloride (HgCl2). Mercuric chloride is a potent thiol poison leading to protein structure damage and inhibition of thiol-containing antioxidants and liver damage caused by the development of oxidative stress (Deng et al., 2012).
Mercuric chloride intoxication led to increased relative liver weight in the HgCl2 treated group (Bortnikova et al., 2021). Rats treated with HgCl2 had a statistically significant increase in total protein, glucose, total cholesterol, total bilirubin, triglycerides, and the GGT, ALP, AST, and AST activities. The histological liver analysis showed the presence of hepatocyte dystrophy, characterized by hyaline droplet dystrophy. Treatment of rats with DEC in doses of 100 and 500 mg/kg for 3 weeks prevented a sharp decrease in the animals’ body weight and physical activity. The relative liver weight was lower than in animals treated with HgCl2 (Bortnikova et al., 2021). The administration of the DEC resulted in a decrease in the activity of several liver enzymes characterizing the functional state of the liver and contributed to experimental rats’ fast recovery (Bortnikova et al., 2021). These changes were more noticeable in animals receiving the maximal DEC dose. Lipid, protein, glucose, and bilirubin levels decreased significantly in the DEC groups compared to the HgCl2 group. The dystrophic changes in hepatocytes of rats treated with DEC 500 mg/kg were not visible. The comparison of hepatoprotective activity of DEC and Silimar indicates that the hepatoprotective effect of DEC at the dose 500 mg/kg was comparable with the effects observed in the group receiving 100 mg/kg of Silimar (Bortnikova et al., 2021). Preclinical data demonstrating DEC hepatoprotective properties in rats need to be confirmed in clinical studies.
Additionally, we have reported that DEC has immunomodulatory properties (Saybel', 2020). Oral administration of DEC to immunosuppressed mice at a 50 mg/kg dose for five consecutive days resulted in upregulation of innate, humoral, and cell-mediated immune responses. Similar upregulations were not observed in animals with normal immune responsiveness.
Chicory-Based Functional Food and Supplements
The food and dietary supplement industries actively use chicory roots (e.g., inulin and oligofructose) as a food substitute, dietary, functional, or food supplements (Wu and Cadwallader, 2019) (Table 1). Inulin is widely used as a prebiotic supporting the intestinal microbiome, specifically stimulating the development of the bifidobacteria in the colon (Niness, 1999). Additionally, inulin acts as dietary fiber (Niness, 1999). Therefore, inulin consumption may increase intestinal peristalsis and may help people experiencing constipation.
Overconsumption of fatty and sugary food has contributed to the disproportional increase in the number of people who are obese or have diabetes. Therefore, reducing fat and sugar in food products could help reduce this trend. Moreover, many people are interested in a healthy lifestyle and healthy eating habits. When dissolved in the water or milk, inulin forms a creamy texture and gives the food a fatty feel (Niness, 1999). Oligofructose has a sweet taste and can be used as a sugar substitute (Niness, 1999). Replacement of high-fat milk and sugar with inulin and oligofructose in milk products (e.g., ice cream) may significantly reduce fat and sugar consumption. At the same time, it will not compromise product taste. Significantly, inulin and oligofructose do not influence glucose levels and insulin secretion (Niness, 1999).
Animal Feed
Lastly, the aerial part and root of chicory can be used as fodder and complementary treatment of livestock (Nwafor et al., 2017) (Table 1). It appears that chicory extracts or phytochemicals like inulin and volatile oils can eliminate or suppress the growth of intestinal worms and other parasites found in animals (Githiori et al., 2006). Therefore, fodder fortified by chicory foliage can promote overall livestock health and prevent intestinal parasite development. Adding 0.1% of chicory powder to the broiler’s diet increased body weight but significantly lower abdominal fat compared to control birds (Khoobani et al., 2020). The same study reported that adding probiotics or chicory to the diet improved the broiler’s ileal microbiome. When chicory is given in excessive amounts to farm animals, it can negatively affect the growth and performance of the livestock.
Discussion
This review article has focused on the complex use of distinct parts of MT, artichoke, and chicory for medicinal purposes, food for humans and animals, and potentially biofuel. As presented in the preceding sections, all parts of these plants can potentially be used as phytodrugs, dietary supplements, components of functional food, and food for humans and animals. It is important to note that usually, only one part of these plants is actively used by the food or drug/dietary supplement industry, and as a result, dozens of articles focus on the biological properties of this part of the plant and constituents responsible for its activity. There are very few articles analyzing real-world aspects of by-product use. Review articles summating available literature on by-product use cite 1–2 articles or state that based on the known biological activity of by-product, it can be used as a dietary supplement, animal feed, or biofuel.
The actively used part of MT is plant fruit (e.g., whole fruit, seeds, oilseed cake, or oil) (Figure 1). It is actively used for medicinal purposes to produce phytodrugs and dietary supplements but can also be added to bakery products. The fortification of the bread with MT oil and oilseed cake improves the bread quality and may help support proper liver function and provide an additional source of amino acids, minerals, and vitamins. Several reports demonstrate the benefits of oilseed cake as a supplement in improving the wellbeing and survival of farm animals (see Section 3.4). The question then becomes what happens to the remaining parts of the plant. Are they wasted? MT leaves are occasionally used to prepare salads. The addition of the aerial part of MT to animal feed may have nutritional benefits, helping to improve the weight gain and survival of farm animals (Andrzejewska et al., 2015; Bagno et al., 2020). It is doubtful that the aerial part of the plant will be used as the main feed for animals due to its low nutritional value (Stastnik et al., 2020). The aerial part represents 70–80% of the whole plant and can be potentially used as a biofuel source.
The food industry uses flowers of artichoke (Figure 1). It appears that 80% of the plant is wasted. Artichoke leaves demonstrate many biological activities, including hepatoprotective. Therefore, they can be used for medicinal purposes as a phytodrug or dietary supplement. It has been proposed that the aerial part of artichoke can be used as animal feed and biofuel.
The most used part of chicory is the root (Figure 1). Roots from cultivated chicory are inulin’s core sources, actively used as dietary supplements and substitutes for fat and sugar. The aerial part of the plant is usually wasted. Bioactive constituents present in the aerial part of the plant possess hepatoprotective properties. The aerial part of the cultivated plant can serve as a raw material for the pharmaceutical or dietary supplement industry, and potentially, as biofuel.
As another example of a plant with the predominant use of roots is licorice (Glycyrrhiza glabra). It is a cough remedy (Kamei et al., 2003; Nosalova et al., 2013; Ruetzler et al., 2013; Kuang et al., 2018) and a gum flavor for candies and food (Kwon et al., 2020). It can treat digestive problems, atopic dermatitis, bacterial and viral infections (Kwon et al., 2020). The licorice roots also possess hepatoprotective properties (Li et al., 2019). It has been reported that licorice ethanolic extract prepared in the leaves possesses antimicrobial activity against Gram-positive bacteria, which was higher than in extract prepared from roots (Irani et al., 2010). Compounds isolated from the leaves of G. uralensis demonstrated anti-inflammatory properties in vitro (Wang et al., 2019).
One of the best examples is the stem-to-stern use of pumpkin (Cucurbita pepo) and corn (Zea mays). Pumpkin is a well-known source of food for humans and farm animals. It is highly nutritional (Klyuchnikova et al., 2011; Brennan, 2020). Pumpkin is rich in proteins, carbohydrates, dietary fiber, vitamin A, vitamin C, magnesium, and potassium (Brennan, 2020). All parts of the pumpkin are edible, including seeds, seed oil, and even leaves. Pumpkins can be given to domestic animals to support their digestive system (Lans, 2019). Elevated levels of α-carotene, β-carotene and β-cryptoxanthin, well-known antioxidants, and vitamin C could help to improve immune system functions and protect against infections, reduce risk of cancer development (Ben-Amotz and Fishier, 1998; Zhou et al., 2016).
Corn is high in dietary fiber, regulates bowel movement, prevents constipation, and decreases cholesterol and glucose levels (Cooper et al., 2017). Various parts of corn are used as a human food source (ear of corn, corn flour, cornmeal, and high-fructose corn syrup), food for farm animal food (plant), a source for fuel, ethanol, and plastic (Fowler, 2012; Gwirtz and Garcia-Casal, 2014). Corn constituents are also used as dietary supplements. For example, corn contains inositol hexaphosphate (IP6), a phosphorylated carbohydrate that has anti-tumor properties and modulatory effects on macrophages (Wee et al., 2021).
The potential of the whole plant use for MT, artichoke, chicory, and other plants is evident. Unfortunately, different industries use distinct parts of the plant (Figure 1). Other parts of the plant are likely wasted. Considering the growing human population, responsible management of plant resources could provide sufficient medicine and food supplies for humans and animals.
The whole plant use should be a priority for businesses and the government. For example, when chicory plant is collected at the field, roots can be sold to the food industry and aerial part to pharmaceutical/dietary supplement companies. Alternatively, dietary supplement companies producing inulin from roots can use the aerial part of the plant to produce supplements supporting liver function.
We feel that the research community needs to analyze and summarize the potential use of distinct parts of plants. This includes estimating the economic judiciousness and proposing a complex and rational use plan for plants to pharmaceutical/dietary supplements, food industries, and country government. The joint efforts with the government must stimulate companies to utilize by-products coming from their technological processes by providing, for example, tax credits.
Many plants used by pharmaceutical and dietary supplement companies are wild plants. About 20 North American medicinal plants are at risk of endangerment due to overharvesting and decreased natural habitats (United Plant Savers, 2021). The development of new plant cultivation techniques could help overcome the overharvesting of precious plants.
Conclusion
Milk thistle, artichoke, and chicory are highly versatile and valuable plants that can be potentially used as phytodrugs, dietary supplements, functional food, food for humans and animals, or biofuel. Distinct parts of these plants are used by pharmaceutical/dietary supplement and food industries. Other parts of plants are possibly wasted. These are only three examples of the potential use of the whole plant. There are many other plants, some parts of which are used, and others are wasted. A plan for the rational stem-to-stern use of the whole plant needs to be developed for most, if not all, plants actively used by food or drug/supplement industries. This should include measures to prevent wild plant overharvesting and the active introduction of cultivation techniques utilizing all plant parts.
Author Contributions
LK, EE, and CS contributed to the conception, design, and preparation of the manuscript. EE, LK, AB, OS, IL, OK, KJ, and CS contributed to the literature search and interpretation of published data. EE, LK, AB, OS, IL, OK, KJ, and CS made substantial contributions to draft the manuscript and revise it critically for valuable intellectual content. All authors have read and approved the final version of the manuscript.
Conflict of Interest
The authors declare that the research was conducted in the absence of any commercial or financial relationships that could be construed as a potential conflict of interest.
Publisher’s Note
All claims expressed in this article are solely those of the authors and do not necessarily represent those of their affiliated organizations, or those of the publisher, the editors and the reviewers. Any product that may be evaluated in this article, or claim that may be made by its manufacturer, is not guaranteed or endorsed by the publisher.
Acknowledgments
The authors would like to thank the Senior Research Scientist of the laboratory of natural resources, Fadeev N., for supplying the photographs of the milk thistle and chicory. We want to acknowledge leopictures for the photo of an artichoke flower, Tom Viggars for the photo of the whole Artichoke plant, Iryna Denysova for the photo of the fresh artichoke with a stalk and leaves, Purple artichoke Elisa Galceran Garcia, LifeCollectionPhotography for the photo of a field of blue Chicory. Licenses for these images have been purchased from https://www.shutterstock.com/.
Abbreviations
ALT, alanine aminotransferase; ALP, alkaline phosphatase; AST, aspartate aminotransferase; CCl4, carbon tetrachloride; DEC, dry extract obtained from wild chicory; DSHEA, dietary supplement health and education act; GGT, γ-glutamyl transferase; ERK1/2, extracellular signal-regulated kinase 1/2; HCV, hepatitis C virus; HgCl2, mercury chloride; IL1α, interleukin 1 alpha; IP6, inositol hexaphosphate; MT, Milk Thistle; NF-kB, nuclear factor kappa B; RNA, ribonucleic acid; STAT-1, signal transducer and activator of transcription 1; STAT-3, signal transducer and activator of transcription 3; TNFα, tumor necrosis factor-alpha; US FDA, the United States food and drug administration; VILAR, All-Russian research institute of medicinal and aromatic plants.
References
Abd El-Mageed, N. M. (2011). Hepatoprotective Effect of Feeding Celery Leaves Mixed with Chicory Leaves and Barley Grains to Hypercholesterolemic Rats. Pharmacogn Mag. 7 (26), 151–156. doi:10.4103/0973-1296.80675
Abdel-Moneim, A., Ahmed, O. M., Abd El-Twab, S. M., Zaky, M. Y., and Bakry, L. N. (2021). Prophylactic Effects of Cynara Scolymus L. Leaf and Flower Hydroethanolic Extracts against Diethylnitrosamine/acetylaminoflourene-Induced Lung Cancer in Wistar Rats. Environ. Sci. Pollut. Res. Int. 28 (32), 43515–43527. doi:10.1007/s11356-021-13391-x
Abenavoli, L., Capasso, R., Milic, N., and Capasso, F. (2010). Milk Thistle in Liver Diseases: Past, Present, Future. Phytother Res. 24 (10), 1423–1432. doi:10.1002/ptr.3207
Abenavoli, L., Izzo, A. A., Milić, N., Cicala, C., Santini, A., and Capasso, R. (2018). Milk Thistle (Silybum marianum): A Concise Overview on its Chemistry, Pharmacological, and Nutraceutical Uses in Liver Diseases. Phytother Res. 32 (11), 2202–2213. doi:10.1002/ptr.6171
Achufusi, T. G. O., and Patel, R. K. (2020). Milk Thistle. in StatPearls [Internet]. Treasure Island (FL: StatPearls Publishing.
Adzet, T., Camarasa, J., and Laguna, J. C. (1987). Hepatoprotective Activity of Polyphenolic Compounds from Cynara Scolymus against CCl4 Toxicity in Isolated Rat Hepatocytes. J. Nat. Prod. 50 (4), 612–617. doi:10.1021/np50052a004
Ahmadi, A., Heidarian, E., and Ghatreh-Samani, K. (2019). Modulatory Effects of Artichoke (Cynara Scolymus L.) Leaf Extract against Oxidative Stress and Hepatic TNF-α Gene Expression in Acute Diazinon-Induced Liver Injury in Rats. J. Basic Clin. Physiol. Pharmacol. 30 (5). doi:10.1515/jbcpp-2018-0180
Ahmed, H. S., Mohamed, W. R., Moawad, A. S., Owis, A. I., Ahmed, R. R., and AbouZid, S. F. (2020). Cytotoxic, Hepatoprotective and Antioxidant Activities of Silybum marianum Variety Albiflorum Growing in Egypt. Nat. Prod. Res. 34 (24), 3540–3544. doi:10.1080/14786419.2019.1582039
Al-Snafi, A. E. (2016). Medical Importance of Cichorium Intybus – A Review. J. Pharm. Pharmacol. 6 (3), 41–56.
Andrzejewska, J., Martinelli, T., and Sadowska, K. (2015). Silybum marianum: Non-medical Exploitation of the Species. Ann. Appl. Biol. 167 (3), 285–297. doi:10.1111/aab.12232
Asrani, S. K., Devarbhavi, H., Eaton, J., and Kamath, P. S. (2019). Burden of Liver Diseases in the World. J. Hepatol. 70 (1), 151–171. doi:10.1016/j.jhep.2018.09.014
Ataei Nukabadi, F., Hojjatoleslamy, M., and Abbasi, H. (2021). Optimization of Fortified Sponge Cake by Nettle Leaves and Milk Thistle Seed Powder Using Mixture Design Approach. Food Sci. Nutr. 9 (2), 757–771. doi:10.1002/fsn3.2041
Babaei, H., Forouzandeh, F., Maghsoumi-Norouzabad, L., Yousefimanesh, H. A., Ravanbakhsh, M., and Zare Javid, A. (2018). Effects of Chicory Leaf Extract on Serum Oxidative Stress Markers, Lipid Profile and Periodontal Status in Patients with Chronic Periodontitis. J. Am. Coll. Nutr. 37 (6), 479–486. doi:10.1080/07315724.2018.1437371
Baginskaya, A. I., Kolchir, V. K., Shkarenkov, A. A., Gorodniuk, T. I., Glazova, N. G., Leskova, T. E., et al. (2000). MIlk Thistle Based Medicines. Ann. scientific works VILAR: Chem. Technol. Med., 282–291.
Bagno, O. A., Shevchenko, S. A., Fedorov, Y. N., Shevchenko, A. I., Prokhorov, O. N., and Shentseva, A. V. (2020). The Influence of Milk Thistle Extract on Productive Qualities and Survivial of Broiler Chickens. Vestnik Altai State. Agric. Univ. 5 (187), 84–91.
Barbosa, C. H., Andrade, M. A., Vilarinho, F., Castanheira, I., Fernando, A. L., Loizzo, M. R., et al. (2020). A New Insight on Cardoon: Exploring New Uses besides Cheese Making with a View to Zero Waste. Foods 9 (5), 564. doi:10.3390/foods9050564
Bashir, A., Hoilat, G. J., Sarwal, P., and Mehta, D. (2021). Liver Toxicity. in StatPearls. Treasure Island FL: StatPearls Publishing.
Batakov, E. A. (2001). Effect of Silybum marianum Oil and Legalon on Lipid Peroxidation and Liver Antioxidant Systems in Rats Intoxicated with Carbon Tetrachloride. Eksp Klin Farmakol 64 (4), 53–55.
Ben Salem, M., Affes, H., Ksouda, K., Dhouibi, R., Sahnoun, Z., Hammami, S., et al. (2015). Pharmacological Studies of Artichoke Leaf Extract and Their Health Benefits. Plant Foods Hum. Nutr. 70 (4), 441–453. doi:10.1007/s11130-015-0503-8
Ben Salem, M., Ksouda, K., Dhouibi, R., Charfi, S., Turki, M., Hammami, S., et al. (2019). LC-MS/MS Analysis and Hepatoprotective Activity of Artichoke (Cynara Scolymus L.) Leaves Extract against High Fat Diet-Induced Obesity in Rats. Biomed. Res. Int. 2019, 4851279. doi:10.1155/2019/4851279
Ben-Amotz, A., and Fishier, R. (1998). Analysis of Carotenoids with Emphasis on 9-cis β-carotene in Vegetables and Fruits Commonly Consumed in Israel. Food Chem. 62 (4), 515–520. doi:10.1016/S0308-8146(97)00196-9
Bortnikova, V. V., Krepkova, L. V., Babenko, A. N., Saybel, O. L., Borovkova, M. V., Kuzina, O. S., et al. (2021). “A Perspective Botanical Drug: Hepatoprotective Activity of Dry Extract Prepared from the Aerial Part of Chicory Plant (Cichorium Intybus L.),” in American College Of Clinical Pharmacology (ACCP)), 100.
Brailski, Kh., Chernev, K., Etŭrska, M., Donov, M., and Velev, G. (1986). Effect of Carsil and Legalon in Treating Chronic Liver Diseases. Vutr Boles 25 (3), 43–49.
Brennan, D. (2020). Health Benefits Of Pumpkin. WebMD Medical Reference. Available: https://www.webmd.com/diet/health-benefits-pumpkin#2 (Accessed May 12, 2021).
Britannica Encyclopaedia (2020). Food. Available: https://www.britannica.com/topic/food (Accessed May 12, 2021).
Buddington, R. K., Kapadia, C., Neumer, F., and Theis, S. (2017). Oligofructose Provides Laxation for Irregularity Associated with Low Fiber Intake. Nutrients 9 (12). doi:10.3390/nu9121372
Bundy, R., Walker, A. F., Middleton, R. W., Wallis, C., and Simpson, H. C. (2008). Artichoke Leaf Extract (Cynara Scolymus) Reduces Plasma Cholesterol in Otherwise Healthy Hypercholesterolemic Adults: a Randomized, Double Blind Placebo Controlled Trial. Phytomedicine 15 (9), 668–675. doi:10.1016/j.phymed.2008.03.001
Calani, L., Brighenti, F., Bruni, R., and Del Rio, D. (2012). Absorption and Metabolism of Milk Thistle Flavanolignans in Humans. Phytomedicine 20 (1), 40–46. doi:10.1016/j.phymed.2012.09.004
Cha, J.-Y., Park, C.-K., and Cho, Y.-S. (2010). Hepatoprotective Effect of Chicory (Chicorium Intybus) Root Extract against Orotic Acid-Induced Fatty Liver in Rats. Food Sci. Biotechnol. 19 (4), 865–871. doi:10.1007/s10068-010-0123-x
Chabaev, M. G., Ryijkov, I. V., Nikolaichenko, N. V., and Khabibulina, V. A. (2011). Productivity and Metabolism in Lactating Cows when by Feeding Milk Thistle Meal. Zootechnika (6), 8–10.
Cimen, O., Eken, H., Keskin Cimen, F., Cekic, A. B., Kurt, N., Ozbek Bilgin, A., et al. (2020). The Effect of Liv-52 on Liver Ischemia Reperfusion Damage in Rats. BMC Pharmacol. Toxicol. 21 (1), 2. doi:10.1186/s40360-019-0380-0
Colak, E., Ustuner, M. C., Tekin, N., Colak, E., Burukoglu, D., Degirmenci, I., et al. (2016). The Hepatocurative Effects of Cynara Scolymus L. Leaf Extract on Carbon Tetrachloride-Induced Oxidative Stress and Hepatic Injury in Rats. Springerplus 5, 216. doi:10.1186/s40064-016-1894-1
Cooper, D. N., Kable, M. E., Marco, M. L., De Leon, A., Rust, B., Baker, J. E., et al. (2017). The Effects of Moderate Whole Grain Consumption on Fasting Glucose and Lipids, Gastrointestinal Symptoms, and Microbiota. Nutrients 9 (2). doi:10.3390/nu9020173
D'Antuono, I., Carola, A., Sena, L. M., Linsalata, V., Cardinali, A., Logrieco, A. F., et al. (2018). Artichoke Polyphenols Produce Skin Anti-age Effects by Improving Endothelial Cell Integrity and Functionality. Molecules 23 (11). doi:10.3390/molecules23112729
De Martiis, M., Fontana, M., Assogna, G., D'Ottavi, R., and D'Ottavi, O. (1980). Milk Thistle (Silybum marianum) Derivatives in the Therapy of Chronic Hepatopathies. Clin. Ter 94 (3), 283–315.
Deák, G., Müzes, G., Láng, I., Niederland, V., Nékám, K., Gonzalez-Cabello, R., et al. (1990). Immunomodulator Effect of Silymarin Therapy in Chronic Alcoholic Liver Diseases. Orv Hetil 131 (24), 12911295–12961296.
Deng, Y., Xu, Z., Liu, W., Yang, H., Xu, B., and Wei, Y. (2012). Effects of Lycopene and Proanthocyanidins on Hepatotoxicity Induced by Mercuric Chloride in Rats. Biol. Trace Elem. Res. 146 (2), 213–223. doi:10.1007/s12011-011-9242-3
Domitrović, R., and Potočnjak, I. (2016). A Comprehensive Overview of Hepatoprotective Natural Compounds: Mechanism of Action and Clinical Perspectives. Arch. Toxicol. 90 (1), 39–79. doi:10.1007/s00204-015-1580-z
Edible Wild Food (2021). Milk Thistle. Available: https://www.ediblewildfood.com/milk-thistle.aspx (Accessed May 12, 2021).
Ekor, M. (2014). The Growing Use of Herbal Medicines: Issues Relating to Adverse Reactions and Challenges in Monitoring Safety. Front. Pharmacol. 4, 177. doi:10.3389/fphar.2013.00177
El-Gazayerly, O. N., Makhlouf, A. I., Soelm, A. M., and Mohmoud, M. A. (2014). Antioxidant and Hepatoprotective Effects of Silymarin Phytosomes Compared to Milk Thistle Extract in CCl4 Induced Hepatotoxicity in Rats. J. Microencapsul 31 (1), 23–30. doi:10.3109/02652048.2013.805836
El-Kamary, S. S., Shardell, M. D., Abdel-Hamid, M., Ismail, S., El-Ateek, M., Metwally, M., et al. (2009). A Randomized Controlled Trial to Assess the Safety and Efficacy of Silymarin on Symptoms, Signs and Biomarkers of Acute Hepatitis. Phytomedicine 16 (5), 391–400. doi:10.1016/j.phymed.2009.02.002
Elsebai, M. F., Mocan, A., and Atanasov, A. G. (2016). Cynaropicrin: A Comprehensive Research Review and Therapeutic Potential as an Anti-hepatitis C Virus Agent. Front. Pharmacol. 7, 472. doi:10.3389/fphar.2016.00472
Enioutina, E. Y., Job, K. M., Krepkova, L. V., Reed, M. D., and Sherwin, C. M. (2020). How Can We Improve the Safe Use of Herbal Medicine and Other Natural Products? A Clinical Pharmacologist mission. Expert Rev. Clin. Pharmacol. 13 (9), 935–944. doi:10.1080/17512433.2020.1803739
European OTC medicines and devices (2020). Carsil 22,5 Mg Silymarinum N80 Tabs. Stabilizes Liver Cell Membrane. Available: https://bbeautybureau.com/carsil-225mg-silymarinum-n80-liver-cell-membrane/(Accessed May 12, 2021).
Fehér, J., Deák, G., Müzes, G., Láng, I., Niederland, V., Nékám, K., et al. (1989). Liver-protective Action of Silymarin Therapy in Chronic Alcoholic Liver Diseases. Orv Hetil 130 (51), 2723–2727.
Fenclova, M., Novakova, A., Viktorova, J., Jonatova, P., Dzuman, Z., Ruml, T., et al. (2019). Poor Chemical and Microbiological Quality of the Commercial Milk Thistle-Based Dietary Supplements May Account for Their Reported Unsatisfactory and Non-reproducible Clinical Outcomes. Sci. Rep. 9 (1), 11118. doi:10.1038/s41598-019-47250-0
Ferenci, P., Dragosics, B., Dittrich, H., Frank, H., Benda, L., Lochs, H., et al. (1989). Randomized Controlled Trial of Silymarin Treatment in Patients with Cirrhosis of the Liver. J. Hepatol. 9 (1), 105–113. doi:10.1016/0168-8278(89)90083-4
Fernández, J., Curt, M. D., and Aguado, P. L. (2006). Industrial Applications of Cynara Cardunculus L. For Energy and Other Uses. Ind. Crops Prod. 24 (3), 222–229. doi:10.1016/j.indcrop.2006.06.010
Ferrare, K., Bidel, L. P. R., Awwad, A., Poucheret, P., Cazals, G., Lazennec, F., et al. (2018). Increase in Insulin Sensitivity by the Association of Chicoric Acid and Chlorogenic Acid Contained in a Natural Chicoric Acid Extract (NCRAE) of Chicory (Cichorium Intybus L.) for an Antidiabetic Effect. J. Ethnopharmacol 215, 241–248. doi:10.1016/j.jep.2017.12.035
Flora, K., Hahn, M., Rosen, H., and Benner, K. (1998). Milk Thistle (Silybum marianum) for the Therapy of Liver Disease. Am. J. Gastroenterol. 93 (2), 139–143. doi:10.1111/j.1572-0241.1998.00139.x
Fowler, J. (2012). FarmProgress. 13 Ways Corn Is Used in Our Everyday Lives. Available: https://www.farmprogress.com/vegetables/13-ways-corn-used-our-everyday-lives (Accessed May 12, 2021).
Ghaffari, A., Rafraf, M., Navekar, R., Sepehri, B., Asghari-Jafarabadi, M., and Ghavami, S. M. (2019). Turmeric and Chicory Seed Have Beneficial Effects on Obesity Markers and Lipid Profile in Non-alcoholic Fatty Liver Disease (NAFLD). Int. J. Vitam Nutr. Res. 89 (5-6), 293–302. doi:10.1024/0300-9831/a000568
Gillessen, A., and Schmidt, H. H. (2020). Silymarin as Supportive Treatment in Liver Diseases: A Narrative Review. Adv. Ther. 37 (4), 1279–1301. doi:10.1007/s12325-020-01251-y
GistGear (2021). Best Artichoke Herbal Supplements - Buying Guide. Available: https://gistgear.com/health-personal-care/nutrition-dietary/artichoke-herbal-supplements (Accessed August 31, 2021).
Githiori, J. B., Athanasiadou, S., and Thamsborg, S. M. (2006). Use of Plants in Novel Approaches for Control of Gastrointestinal Helminths in Livestock with Emphasis on Small Ruminants. Vet. Parasitol. 139 (4), 308–320. doi:10.1016/j.vetpar.2006.04.021
Gominho, J., Curt, M. D., Lourenço, A., Fernández, J., and Pereira, H. (2018). Cynara Cardunculus L. As a Biomass and Multi-Purpose Crop: A Review of 30 Years of Research. Biomass and Bioenergy 109, 257–275. doi:10.1016/j.biombioe.2018.01.001
Gominho, J., Fernandez, J., and Pereira, H. (2001). Cynara Cardunculus L. - a New Fibre Crop for Pulp and Paper Production. Ind. Crops Prod. 13 (1), 1–10. doi:10.1016/S0926-6690(00)00044-3
Gostin, A.-I., and Waisundara, V. Y. (2019). Edible Flowers as Functional Food: A Review on Artichoke (Cynara Cardunculus L.). Trends Food Sci. Technology 86, 381–391. doi:10.1016/j.tifs.2019.02.015
Gottlieb, S. (2019). “Statement from FDA Commissioner on the agency’s New Efforts to Strengthen Regulation of Dietary Supplements by Modernizing and Reforming FDA’s Oversight,” in The U.S. Food and Drug Administration (Washington, D.C.: U.S. Department of Health and Human Services.).
Grela, E. R., Sobolewska, S., and Roziński, T. (2014). Effect of Inulin Extracts or Inulin-Containing Plant Supplement on Blood Lipid Indices and Fatty Acid Profile in Fattener Tissues. Pol. J. Vet. Sci. 17 (1), 93–98. doi:10.2478/pjvs-2014-0012
Gwirtz, J. A., and Garcia-Casal, M. N. (2014). Processing maize Flour and Corn Meal Food Products. Ann. N. Y Acad. Sci. 1312, 66–75. doi:10.1111/nyas.12299
Himalaya Wellness Company (2021a). Himalaya, Our story [Online]. Makali, Bengaluru: Himalaya Wellness Company. Available: https://himalayawellness.in/pages/our-story (Accessed September 11, 2021).
Himalaya Wellness Company (2021b). Liv.52. Description. Available: https://himalayawellness.in/products/liv-52 (Accessed June 1, 2021).
Hou, Y. C., Liou, K. T., Chern, C. M., Wang, Y. H., Liao, J. F., Chang, S., et al. (2010). Preventive Effect of Silymarin in Cerebral Ischemia-Reperfusion-Induced Brain Injury in Rats Possibly through Impairing NF-Κb and STAT-1 Activation. Phytomedicine 17 (12), 963–973. doi:10.1016/j.phymed.2010.03.012
Huber, R., Müller, M., Naumann, J., Schenk, T., and Lüdtke, R. (2009). Artichoke Leave Extract for Chronic Hepatitis C - a Pilot Study. Phytomedicine 16 (9), 801–804. doi:10.1016/j.phymed.2009.03.007
Hughes, S. R., Qureshi, N., López-Núñez, J. C., Jones, M. A., Jarodsky, J. M., Galindo-Leva, L. Á., et al. (2017). Utilization of Inulin-Containing Waste in Industrial Fermentations to Produce Biofuels and Bio-Based Chemicals. World J. Microbiol. Biotechnol. 33 (4), 78. doi:10.1007/s11274-017-2241-6
Huseini, H. F., Alavian, S. M., Heshmat, R., Heydari, M. R., and Abolmaali, K. (2005). The Efficacy of Liv-52 on Liver Cirrhotic Patients: a Randomized, Double-Blind, Placebo-Controlled First Approach. Phytomedicine 12 (9), 619–624. doi:10.1016/j.phymed.2004.10.003
Irani, M., Sarmadi, M., Bernard, F., Ebrahimi Pour, G. H., and Shaker Bazarnov, H. (2010). Leaves Antimicrobial Activity of Glycyrrhiza Glabra L. Iran J. Pharm. Res. 9 (4), 425–428.
Janda, K., Gutowska, I., Geszke-Moritz, M., and Jakubczyk, K. (2021). The Common Cichory (Cichorium Intybus L.) as a Source of Extracts with Health-Promoting Properties-A Review. Molecules 26 (6), 1814. doi:10.3390/molecules26061814
Kamei, J., Nakamura, R., Ichiki, H., and Kubo, M. (2003). Antitussive Principles of Glycyrrhizae Radix, a Main Component of the Kampo Preparations Bakumondo-To (Mai-Men-Dong-Tang). Eur. J. Pharmacol. 469 (1-3), 159–163. doi:10.1016/s0014-2999(03)01728-x
Kandeler, R., and Ullrich, W. R. (2009). Symbolism of Plants: Examples from European-Mediterranean Culture Presented with Biology and History of Art: NOVEMBER: Chicory. J. Exp. Bot. 60 (14), 3973–3974. doi:10.1093/jxb/erp248
Kantah, M. K., Kobayashi, R., Sollano, J., Naito, Y., Solimene, U., Jains, S., et al. (2011). Hepatoprotective Activity of a Phytotherapeutic Formula on Thioacetamide-Iinduced Liver Fibrosis Model. Acta Biomed. 82 (1), 82–89.
Khoobani, M., Hasheminezhad, S. H., Javandel, F., Nosrati, M., Seidavi, A., Kadim, I. T., et al. (2020). Effects of Dietary Chicory (Chicorium Intybus L.) and Probiotic Blend as Natural Feed Additives on Performance Traits, Blood Biochemistry, and Gut Microbiota of Broiler Chickens. Antibiotics (Basel) 9 (1), 5. doi:10.3390/antibiotics9010005
King County, W. (2018). King County, Washington (2018). Milk Thistle – May 2018 Weed of the Month. King County, Washington. Available at: https://kingcountyweeds.com/2018/05/14/milk-thistle-may-2018-weed-of-the-month/#:∼:text=Left%20unchecked%2C%20they%20produce%20purple (Accessed May 12, 2021).
Klyuchnikova, O. V., Skogoreva, E. A., Kozhevnikova, N. P., and Slobodyanik, V. S. (2011). “Vegetable Raw Materials in the Creation of Functional Meat Products,” in Successes in Modern Natural Sciences.
Kolesnyk, M. D., and Bankovska, I. (2008). Application of Milk Thistle to Spotted Piglets. Livestock of Ukraine (2), 32–34.
Kolesnyk, M. D., Bankovska, I. B., and Kostenko, O. I. (2009). Components of the Effectiveness of the Use Milk Thistle. Vestnyl Poltava State. Adgicultural Acad. 1, 76–77.
Kravainis, Y. Y., Tanifa, V. V., and Kravaine, R. S. (2014). Application of Milk Thistle Cake in Cattle Breading. Vestnik Russ. Acad. Argicultural Sceince (4), 44–46.
Krepkova, L. V., Shkarenkov, A. A., and Sokol'skaya, T. A. (2008). Experimental and Clinical Study of Herbal Remedies from Milk Thistle. Probl. Biol. Med. Pharm. Chem. (4), 3–6.
Krepkova, L. V., and Sokol'skaya, T. A. (2007). Preclinical Investigation of the Safety of the Hepatoprotective Drug Silimar. Khimiko-Farmatsevticheskii Zhurnal 41 (9), 32–34. doi:10.1007/s11094-007-0108-0
Kuang, Y., Li, B., Fan, J., Qiao, X., and Ye, M. (2018). Antitussive and Expectorant Activities of Licorice and its Major Compounds. Bioorg. Med. Chem. 26 (1), 278–284. doi:10.1016/j.bmc.2017.11.046
Kwon, Y. J., Son, D. H., Chung, T. H., and Lee, Y. J. (2020). A Review of the Pharmacological Efficacy and Safety of Licorice Root from Corroborative Clinical Trial Findings. J. Med. Food 23 (1), 12–20. doi:10.1089/jmf.2019.4459
Lans, C. (2019). Do recent Research Studies Validate the Medicinal Plants Used in British Columbia, Canada for Pet Diseases and Wild Animals Taken into Temporary Care? J. Ethnopharmacol 236, 366–392. doi:10.1016/j.jep.2019.02.030
Lattanzio, V., Kroon, P. A., Linsalata, V., and Cardinali, A. (2009). Globe Artichoke: A Functional Food and Source of Nutraceutical Ingredients. J. Funct. Foods 1 (2), 131–144. doi:10.1016/j.jff.2009.01.002
Lee, J. I., Narayan, M., and Barrett, J. S. (2007). Analysis and Comparison of Active Constituents in Commercial Standardized Silymarin Extracts by Liquid Chromatography-Electrospray Ionization Mass Spectrometry. J. Chromatogr. B Analyt Technol. Biomed. Life Sci. 845 (1), 95–103. doi:10.1016/j.jchromb.2006.07.063
Lee, M. H., Huang, Z., Kim, D. J., Kim, S. H., Kim, M. O., Lee, S. Y., et al. (2013). Direct Targeting of MEK1/2 and RSK2 by Silybin Induces Cell-Cycle Arrest and Inhibits Melanoma Cell Growth. Cancer Prev. Res. (Phila) 6 (5), 455–465. doi:10.1158/1940-6207.CAPR-12-0425
Li, G. Y., Gao, H. Y., Huang, J., Lu, J., Gu, J. K., and Wang, J. H. (2014). Hepatoprotective Effect of Cichorium Intybus L., a Traditional Uighur Medicine, against Carbon Tetrachloride-Induced Hepatic Fibrosis in Rats. World J. Gastroenterol. 20 (16), 4753–4760. doi:10.3748/wjg.v20.i16.4753
Li, X., Sun, R., and Liu, R. (2019). Natural Products in Licorice for the Therapy of Liver Diseases: Progress and Future Opportunities. Pharmacol. Res. 144, 210–226. doi:10.1016/j.phrs.2019.04.025
Marmouzi, I., Bouyahya, A., Ezzat, S. M., El Jemli, M., and Kharbach, M. (2021). The Food Plant Silybum marianum (L.) Gaertn.: Phytochemistry, Ethnopharmacology and Clinical Evidence. J. Ethnopharmacol 265, 113303. doi:10.1016/j.jep.2020.113303
Martirosyan, D. M., and Singh, J. (2015). A New Definition of Functional Food by FFC: what Makes a New Definition Unique? Ffhd 5 (66), 209209–223223. doi:10.31989/ffhd.v5i6.183
Meneses, M., Megías, M. D., Madrid, J., Martínez-Teruel, A., Hernández, F., and Oliva, J. (2007). Evaluation of the Phytosanitary, Fermentative and Nutritive Characteristics of the Silage Made from Crude Artichoke (Cynara Scolymus L.) By-Product Feeding for Ruminants. Small Ruminant Res. 70 (2-3), 292–296. doi:10.1016/j.smallrumres.2006.05.008
Mildzikhov, T. Z., Temiraev, R. B., Khadikova, M. A., and Malieva, E. V. (2013). Quality of Broiler Meat in Heavy Metal Detoxication. Meat Industry (12), 55–57.
Mirpoor, S. F., Varriale, S., Porta, R., Naviglio, D., Spennato, M., Gardossi, L., et al. (2022). A Biorefinery Approach for the Conversion of Cynara Cardunculus Biomass to Active Films. Food Hydrocolloids 122, 107099. doi:10.1016/j.foodhyd.2021.107099
Missouri Botanical Garden (2019). Plant Finder Database: Silybum Marianum. St. Louis, MO. Available: https://www.missouribotanicalgarden.org/PlantFinder/PlantFinderDetails.aspx?taxonid=277472&isprofile=0& (Accessed May 12, 2021).
Mohafrash, S. M. M., and Mossa, A. H. (2020). Herbal Syrup from Chicory and Artichoke Leaves Ameliorate Liver Damage Induced by Deltamethrin in Weanling Male Rats. Environ. Sci. Pollut. Res. Int. 27 (7), 7672–7682. doi:10.1007/s11356-019-07434-7
Monllor, P., Romero, G., Sendra, E., Atzori, A. S., and Díaz, J. R. (2020). Short-Term Effect of the Inclusion of Silage Artichoke By-Products in Diets of Dairy Goats on Milk Quality. Animals (Basel) 10 (2), 339. doi:10.3390/ani10020339
Mukhtar, S., Xiaoxiong, Z., Qamer, S., Saad, M., Mubarik, M. S., Mahmoud, A. H., et al. (2021). Hepatoprotective Activity of Silymarin Encapsulation against Hepatic Damage in Albino Rats. Saudi J. Biol. Sci. 28 (1), 717–723. doi:10.1016/j.sjbs.2020.10.063
Munn, Z., Peters, M. D. J., Stern, C., Tufanaru, C., McArthur, A., and Aromataris, E. (2018). Systematic Review or Scoping Review? Guidance for Authors when Choosing between a Systematic or Scoping Review Approach. BMC Med. Res. Methodol. 18 (1), 143. doi:10.1186/s12874-018-0611-x
Nekrasova, K. L., and Popov, V. G. (2020). Scientific Approach to the Production of Functional Food Ingredients Based on Unconventional Plant Materials. Vestn. Voronež. Gos. Univ. Inž. Tehnol. 82 (2), 77–82. doi:10.20914/2310-1202-2020-2-77-82
Niness, K. R. (1999). Inulin and Oligofructose: what Are They? J. Nutr. 129, 1402S–6S. doi:10.1093/jn/129.7.1402S
Nofal, A., Ibrahim, A. M., Nofal, E., Gamal, N., and Osman, S. (2019). Topical Silymarin versus Hydroquinone in the Treatment of Melasma: A Comparative Study. J. Cosmet. Dermatol. 18 (1), 263–270. doi:10.1111/jocd.12769
Nosalova, G., Fleskova, D., Jurecek, L., Sadlonova, V., and Ray, B. (2013). Herbal Polysaccharides and Cough Reflex. Respir. Physiol. Neurobiol. 187 (1), 47–51. doi:10.1016/j.resp.2013.03.015
Nwafor, I. C., Shale, K., and Achilonu, M. C. (2017). Chemical Composition and Nutritive Benefits of Chicory (Cichorium Intybus) as an Ideal Complementary And/or Alternative Livestock Feed Supplement. ScientificWorldJournal 2017, 7343928. doi:10.1155/2017/7343928
Oliveira, I., Gominho, J., Diberardino, S., and Duarte, E. (2012). Characterization of Cynara Cardunculus L. Stalks and Their Suitability for Biogas Production. Ind. Crops Prod. 40, 318–323. doi:10.1016/j.indcrop.2012.03.029
Panahi, Y., Kianpour, P., Mohtashami, R., Atkin, S. L., Butler, A. E., Jafari, R., et al. (2018). Efficacy of Artichoke Leaf Extract in Non-alcoholic Fatty Liver Disease: A Pilot Double-Blind Randomized Controlled Trial. Phytother Res. 32 (7), 1382–1387. doi:10.1002/ptr.6073
Perović, J., Tumbas Šaponjac, V., Kojić, J., Krulj, J., Moreno, D. A., García-Viguera, C., et al. (2021). Chicory (Cichorium Intybus L.) as a Food Ingredient - Nutritional Composition, Bioactivity, Safety, and Health Claims: A Review. Food Chem. 336, 127676. doi:10.1016/j.foodchem.2020.127676
Petropoulos, S. A., Levizou, E., Ntatsi, G., Fernandes, Â., Petrotos, K., Akoumianakis, K., et al. (2017). Salinity Effect on Nutritional Value, Chemical Composition and Bioactive Compounds Content of Cichorium Spinosum L. Food Chem. 214, 129–136. doi:10.1016/j.foodchem.2016.07.080
Poppe, L., and Petersen, M. (2016). Variation in the Flavonolignan Composition of Fruits from Different Silybum marianum Chemotypes and Suspension Cultures Derived Therefrom. Phytochemistry 131, 68–75. doi:10.1016/j.phytochem.2016.09.003
Pradhan, S. C., and Girish, C. (2006). Hepatoprotective Herbal Drug, Silymarin from Experimental Pharmacology to Clinical Medicine. Indian J. Med. Res. 124 (5), 491–504.
Rahman, I. U., Afzal, A., Iqbal, Z., Ijaz, F., Ali, N., Shah, M., et al. (2019). Historical Perspectives of Ethnobotany. Clin. Dermatol. 37 (4), 382–388. doi:10.1016/j.clindermatol.2018.03.018
Rehm, J., Samokhvalov, A. V., and Shield, K. D. (2013). Global burden of Alcoholic Liver Diseases. J. Hepatol. 59 (1), 160–168. doi:10.1016/j.jhep.2013.03.007
Reimer, R. A., Soto-Vaca, A., Nicolucci, A. C., Mayengbam, S., Park, H., Madsen, K. L., et al. (2020). Effect of Chicory Inulin-type Fructan-Containing Snack Bars on the Human Gut Microbiota in Low Dietary Fiber Consumers in a Randomized Crossover Trial. Am. J. Clin. Nutr. 111 (6), 1286–1296. doi:10.1093/ajcn/nqaa074
Rocchetti, G., Lucini, L., Corrado, G., Colla, G., Cardarelli, M., Pascale, S., et al. (2020). Phytochemical Profile, Mineral Content, and Bioactive Compounds in Leaves of Seed-Propagated Artichoke Hybrid Cultivars. Molecules 25 (17), 3795. doi:10.3390/molecules25173795
Romanova, E. I., and Krasavtsev, E. L. (2007). Modern Aspects of Hepatoprotective Therapy. Health Ecol. Issues 12 (12), 42–50.
Ruetzler, K., Fleck, M., Nabecker, S., Pinter, K., Landskron, G., Lassnigg, A., et al. (2013). A Randomized, Double-Blind Comparison of Licorice versus Sugar-Water Gargle for Prevention of Postoperative Sore Throat and Postextubation Coughing. Anesth. Analg 117 (3), 614–621. doi:10.1213/ANE.0b013e318299a650
Rutter, K., Scherzer, T. M., Beinhardt, S., Kerschner, H., Stättermayer, A. F., Hofer, H., et al. (2011). Intravenous Silibinin as 'rescue Treatment' for On-Treatment Non-responders to Pegylated Interferon/ribavirin Combination Therapy. Antivir. Ther. 16 (8), 1327–1333. doi:10.3851/IMP1942
Sama, S. K., Krishnamurthy, L., Ramachandran, K., and Lal, K. (1976). Efficacy of an Indigenous Compound Preparation (Liv-52) in Acute Viral Hepatitis-A Double Blind Study. Indian J. Med. Res. 64 (5), 738–742.
Saxena, A., and Garg, N. K. (1979). Effect of Liv-52 on Hepatic Enzymes. Indian J. Exp. Biol. 17 (7), 662–664.
Saybel, O. L., Radimich, A. I., Adamov, G. V., and Dargaeva, T. D. (2020). Comparative Phytochemical Study of the Aerial Parts of the Wild-Growing and Cultivat-Ed Chicory Ordinary (Cichorium Intybus L.). Jcprm (3), 187–195. doi:10.14258/jcprm.2020037386
Schmidt, B. M. (2012). Responsible Use of Medicinal Plants for Cosmetics. horts 47 (8), 985–991. doi:10.21273/hortsci.47.8.985
Schumacher, E., Vigh, E., Molnár, V., Kenyeres, P., Fehér, G., Késmárky, G., et al. (2011). Thrombosis Preventive Potential of Chicory Coffee Consumption: a Clinical Study. Phytother Res. 25 (5), 744–748. doi:10.1002/ptr.3481
Semenkina, N. G. (2010). Using Milk Thistle as a Source of Functional Ingredients. Food Industry (5), 40–41.
Shemuranova, N. A., and Garifullina, N. A. (2020). Plants as the Basis for the Development of Environmentally Friendly Highly Functional Bioadditives for Animals (Review). Аграрная наука Евро-Северо-Востока 21 (5), 483–502. doi:10.30766/2072-9081.2020.21.5.483-502
Shoaib, M., Shehzad, A., Omar, M., Rakha, A., Raza, H., Sharif, H. R., et al. (2016). Inulin: Properties, Health Benefits and Food Applications. Carbohydr. Polym. 147, 444–454. doi:10.1016/j.carbpol.2016.04.020
Siegel, A. B., and Stebbing, J. (2013). Milk Thistle: Early Seeds of Potential. Lancet Oncol. 14 (10), 929–930. doi:10.1016/S1470-2045(13)70414-5
Smith, T., Kawa, K., Eckl, V., Morton, C., and Stredney, R. (2017). Herbal Supplement Sales in US Increase 7.7% in 2016 Consumer Preferences Shifting toward Ingredients with General Wellness Benefits, Driving Growth of Adaptogens and Digestive Health Products. HerbalGram 115, 56–65.
Sokol'skaya, T. A. (2000). Complex Processing of Milk Thistle Fruits and Production of the Drug "Silimar" on its Basis. Khimiko-Farmatsevticheskii Zhurnal 34 (9), 227–230.
Soleimani, V., Delghandi, P. S., Moallem, S. A., and Karimi, G. (2019). Safety and Toxicity of Silymarin, the Major Constituent of Milk Thistle Extract: An Updated Review. Phytother Res. 33 (6), 1627–1638. doi:10.1002/ptr.6361
Song, X. Y., Li, R. H., Liu, W. W., Hayashi, T., Mizuno, K., Hattori, S., et al. (2021). Effect of Silibinin on Ethanol- or Acetaldehyde-Induced Damge of Mouse Primary Hepatocytes In Vitro. Toxicol. Vitro 70, 105047. doi:10.1016/j.tiv.2020.105047
Speroni, E., Cervellati, R., Govoni, P., Guizzardi, S., Renzulli, C., and Guerra, M. C. (2003). Efficacy of Different Cynara Scolymus Preparations on Liver Complaints. J. Ethnopharmacol 86 (2-3), 203–211. doi:10.1016/s0378-8741(03)00076-x
Stastnik, O., Pavlata, L., and Mrkvicova, E. (2020). The Milk Thistle Seed Cakes and Hempseed Cakes Are Potential Feed for Poultry. Animals (Basel) 10 (8), 1384. doi:10.3390/ani10081384
Street, R. A., Sidana, J., and Prinsloo, G. (2013). Cichorium Intybus: Traditional Uses, Phytochemistry, Pharmacology, and Toxicology. Evid. Based Complement. Alternat Med. 2013, 579319. doi:10.1155/2013/579319
Sümer, E., Senturk, G. E., Demirel, Ö. U., and Yesilada, E. (2020). Comparative Biochemical and Histopathological Evaluations Proved that Receptacle Is the Most Effective Part of Cynara Scolymus against Liver and Kidney Damages. J. Ethnopharmacol 249, 112458. doi:10.1016/j.jep.2019.112458
Szilárd, S., Szentgyörgyi, D., and Demeter, I. (1988). Protective Effect of Legalon in Workers Exposed to Organic Solvents. Acta Med. Hung 45 (2), 249–256.
Thabrew, M. I., Emerole, G. O., and Subbarao, V. V. (1982). Effect of Liv-52 on Carbon Tetrachloride-Induced Changes in Hepatic Microsomal Drug-Metabolizing Enzymes of the Rat. Toxicol. Lett. 14 (3-4), 183–188. doi:10.1016/0378-4274(82)90049-2
Thomas, P. R., and Earl, R. (1994). Institute of Medicine; Committee On Opportunities In the Nutrition And Food Sciences: Research Challenges And the Next Generation Of Investigators [Online]. Washington, DC: The National Academies Press. doi:10.17226/2133
Tong, J., Yao, X., Zeng, H., Zhou, G., Chen, Y., Ma, B., et al. (2015). Hepatoprotective Activity of Flavonoids from Cichorium Glandulosum Seeds In Vitro and In Vivo Carbon Tetrachloride-Induced Hepatotoxicity. J. Ethnopharmacol 174, 355–363. doi:10.1016/j.jep.2015.08.045
Tsai, J. H., Liu, J. Y., Wu, T. T., Ho, P. C., Huang, C. Y., Shyu, J. C., et al. (2008). Effects of Silymarin on the Resolution of Liver Fibrosis Induced by Carbon Tetrachloride in Rats. J. Viral Hepat. 15 (7), 508–514. doi:10.1111/j.1365-2893.2008.00971.x
Turkiewicz, I. P., Wojdyło, A., Tkacz, K., Nowicka, P., and Hernández, F. (2019). Antidiabetic, Anticholinesterase and Antioxidant Activity vs. Terpenoids and Phenolic Compounds in Selected New Cultivars and Hybrids of Artichoke Cynara Scolymus L. Molecules 24 (7), 1222. doi:10.3390/molecules24071222
Ullah, K., Ahmad, M., Sofia, , , and Qiu, F. (2015). Assessing the Experimental Investigation of Milk Thistle Oil for Biodiesel Production Using Base Catalyzed Transesterification. Energy 89, 887–895. doi:10.1016/j.energy.2015.06.028
United Plant Savers (2021). Species at Risk. Available at: https://unitedplantsavers.org/species-at-risk/(Accessed Sep, 2021).
Vargas-Mendoza, N., Madrigal-Santillán, E., Morales-González, A., Esquivel-Soto, J., Esquivel-Chirino, C., García-Luna Y González-Rubio, M., et al. (2014). Hepatoprotective Effect of Silymarin. World J. Hepatol. 6 (3), 144–149. doi:10.4254/wjh.v6.i3.144
Villarini, M., Acito, M., di Vito, R., Vannini, S., Dominici, L., Fatigoni, C., et al. (2021). Pro-Apoptotic Activity of Artichoke Leaf Extracts in Human HT-29 and RKO Colon Cancer Cells. Int. J. Environ. Res. Public Health 18 (8). doi:10.3390/ijerph18084166
Wah Kheong, C., Nik Mustapha, N. R., and Mahadeva, S. (2017). A Randomized Trial of Silymarin for the Treatment of Nonalcoholic Steatohepatitis. Clin. Gastroenterol. Hepatol. 15 (12), 1940–e8. doi:10.1016/j.cgh.2017.04.016
Wang, L., Zhang, K., Han, S., Zhang, L., Bai, H., Bao, F., et al. (2019). Constituents Isolated from the Leaves of Glycyrrhiza Uralansis and Their Anti-inflammatory Activities on LPS-Induced RAW264.7 Cells. Molecules 24 (10). doi:10.3390/molecules24101923
Wee, Y., Yang, C. H., Chen, S. K., Yen, Y. C., and Wang, C. S. (2021). Inositol Hexaphosphate Modulates the Behavior of Macrophages through Alteration of Gene Expression Involved in Pathways of Pro- and Anti-inflammatory Responses, and Resolution of Inflammation Pathways. Food Sci. Nutr. 9 (6), 3240–3249. doi:10.1002/fsn3.2286
Wu, T., and Cadwallader, K. R. (2019). Identification of Characterizing Aroma Components of Roasted Chicory "Coffee" Brews. J. Agric. Food Chem. 67 (50), 13848–13859. doi:10.1021/acs.jafc.9b00776
Zeaiter, Z., Regonesi, M. E., Cavini, S., Labra, M., Sello, G., and Di Gennaro, P. (2019). Extraction and Characterization of Inulin-type Fructans from Artichoke Wastes and Their Effect on the Growth of Intestinal Bacteria Associated with Health. Biomed. Res. Int. 2019, 1083952. doi:10.1155/2019/1083952
Zhang, H. L., Dai, L. H., Wu, Y. H., Yu, X. P., Zhang, Y. Y., Guan, R. F., et al. (2014). Evaluation of Hepatocyteprotective and Anti-hepatitis B Virus Properties of Cichoric Acid from Cichorium Intybus Leaves in Cell Culture. Biol. Pharm. Bull. 37 (7), 1214–1220. doi:10.1248/bpb.b14-00137
Zhou, Y., Wang, T., Meng, Q., and Zhai, S. (2016). Association of Carotenoids with Risk of Gastric Cancer: A Meta-Analysis. Clin. Nutr. 35 (1), 109–116. doi:10.1016/j.clnu.2015.02.003
Zhu, H. J., Brinda, B. J., Chavin, K. D., Bernstein, H. J., Patrick, K. S., and Markowitz, J. S. (2013). An Assessment of Pharmacokinetics and Antioxidant Activity of Free Silymarin Flavonolignans in Healthy Volunteers: a Dose Escalation Study. Drug Metab. Dispos 41 (9), 1679–1685. doi:10.1124/dmd.113.052423
Keywords: Milk Thistle, Chicory, Artichoke, rational use, food, phytodrug, functional food, animal feed
Citation: Krepkova LV, Babenko AN, Saybel’ OL, Lupanova IA, Kuzina OS, Job KM, Sherwin CM and Enioutina EY (2021) Valuable Hepatoprotective Plants - How Can We Optimize Waste Free Uses of Such Highly Versatile Resources?. Front. Pharmacol. 12:738504. doi: 10.3389/fphar.2021.738504
Received: 08 July 2021; Accepted: 27 October 2021;
Published: 18 November 2021.
Edited by:
Alessandra Durazzo, Council for Agricultural Research and Economics, ItalyReviewed by:
Jadwiga Andrzejewska, University of Technology and Life Sciences in Bydgoszcz, PolandBilal Haider Abbasi, Quaid-i-Azam University, Pakistan
Kunjbihari Sulakhiya, Indira Gandhi National Tribal University, India
Copyright © 2021 Krepkova, Babenko, Saybel’, Lupanova, Kuzina, Job, Sherwin and Enioutina. This is an open-access article distributed under the terms of the Creative Commons Attribution License (CC BY). The use, distribution or reproduction in other forums is permitted, provided the original author(s) and the copyright owner(s) are credited and that the original publication in this journal is cited, in accordance with accepted academic practice. No use, distribution or reproduction is permitted which does not comply with these terms.
*Correspondence: Elena Y. Enioutina, elena.enioutina@hsc.utah.edu
†These authors have contributed equally to this work and share senior authorship