- 1Department of Radiation Oncology, Cancer Center, West China Hospital, Sichuan University, Chengdu, China
- 2Department of Biotherapy, Cancer Center, West China Hospital, Sichuan University, Chengdu, China
- 3Hangzhou Linan Guorui Health Industry Investment Co., Ltd., Hangzhou, China
Background: The promise of immune checkpoint inhibitors (ICIs) therapy in cancer treatment is tempered by the occurrence of immune-related adverse events (irAEs). Many patients undergoing ICIs also take aspirin, but the association between aspirin and irAEs is not well understood.
Methods: This study analyzed adverse reaction data associated with the use of ICIs in the US Food and Drug Administration (FDA) Adverse Event Reporting System FDA Adverse Event Reporting System database, from the approval date of each drug until 1 October 2022. Multivariate logistic regression was employed to assess the association of aspirin use with irAEs in patients receiving ICIs.
Results: The results indicated that aspirin use was associated with an increased risk of irAEs in a pan-cancer analysis, with a more pronounced association in specific cancer types such as lung cancer, mesothelioma, and pancreatic cancer. However, in lymphoma, aspirin use was associated with a reduced risk of irAEs. Furthermore, aspirin use was associated with an increased risk of certain irAEs, such as anemia, colitis, myocarditis, myositis, pancreatitis, pericarditis, and pneumonia, while it was associated with a reduced risk of rash, Stevens-Johnson syndrome, and thyroiditis.
Conclusion: This study has unveiled an association between aspirin use and irAEs in cancer patients receiving ICIs therapy, emphasizing the need for individualized consideration of patients’ medication history when devising cancer treatment plans to enhance efficacy and reduce risks.
1 Introduction
ICIs therapy is a groundbreaking approach to treating tumors that leverages the immune system to combat malignancies. This approach enhances immune-mediated tumor clearance by blocking negative signals between cancer cells and immune cells (Waldman et al., 2020; Morad et al., 2021). To this end, ICIs that target programmed cell death-1 (PD-1)/programmed death ligand-1 (PD-L1) as well as cytotoxic T lymphocyte-associated protein 4 (CTLA-4) have been developed and employed by researchers in clinical practice (Waldman et al., 2020; Morad et al., 2021). As mounting evidence supports their efficacy and synergistic effects with other cancer treatments, ICIs are increasingly being utilized as a key component in the treatment of many types of cancer, such as melanoma, lung cancer and esophageal cancer (Gadgeel et al., 2020; Rudin et al., 2020; Doki et al., 2022; Livingstone et al., 2022). However, it is important to note that, to date, ICIs remains ineffective for several cancer types, for instance, pancreatic cancer (Bockorny et al., 2022). In some cases, ICIs have not yet attained the status of standard care, as seen in breast cancer (Debien et al., 2023).
However, a notable issue arising from the increasing use of ICIs in clinical practice is their uncontrolled additive impact on the immune system, resulting in irAEs. ICIs manifest unique patterns of toxicity distinct from conventional chemotherapy or other biological agents, often stemming from hyperactive immune reactions against normal organs. irAEs can affect any organ system, including the skin, gastrointestinal tract, cardiovascular system, and endocrine system, among others (Brahmer et al., 2018; Schneider et al., 2021). The frequency of irAEs ranged from 66.4% to 86.8% for all grades, and from 14.1% to 28.6% for grade 3 or higher (Xu et al., 2018). irAEs may be influenced by the patient’s genetic background and microbiome, as well as by treatment-related factors such as combination medication (Jelinic et al., 2018; Cortellini et al., 2020). The mechanism of irAEs is not fully understood but may be related to the overactivation of innate and adaptive immunity caused by the disruption of immune balance by immunotherapy (Pauken et al., 2019). Since the occurrence of irAEs restricts the use of ICIs, it is necessary to further understand the mechanism and influencing factors of irAEs.
Drug-drug interactions (DDI) are a significant focus in the field of systemic anti-cancer treatment. Previous studies have found that combination therapy has an important impact on the outcome of immunotherapy and irAEs. For example, the use of antibiotics and proton pump inhibitors (PPIs) has been associated with poorer outcomes in patients with ICIs (Kostine et al., 2021). Aspirin has become widely used in modern medicine, primarily due to its ability to inhibit the cyclooxygenase (COX) pathway and effectively treat inflammation, pain, and various cardiovascular diseases (Fijałkowski et al., 2022). In recent years, aspirin has also been found to have a well-documented role in the prevention and treatment of tumors (Algra and Rothwell, 2012; Rothwell et al., 2012), especially in colorectal cancer (Rothwell et al., 2010; Drew and Chan, 2021). With the innovation in the field of cancer treatment and the emergence of a new therapy, namely, immunotherapy, researchers have gradually paid attention to the relationship between aspirin and immunotherapy. Recent clinical studies have suggested that the combination of aspirin and ICIs is associated with better outcomes (Cortellini et al., 2020; Zhang et al., 2021). In addition, Aspirin use and its relationship to irAEs were rarely addressed in these studies. Given the widespread acceptance of ICIs into standard practice, it is crucial to gain a better understanding of the association between aspirin treatment and irAEs.
FAERS is a comprehensive drug adverse reaction database maintained by the FDA. Its advantages include broad coverage of adverse events from clinical trials to market use, timely updates, comprehensive drug information, large-scale data for analysis, and reliable reporting from healthcare professionals and consumers. It is a trusted resource for monitoring and reporting drug adverse reactions, and helps to inform better clinical practice and healthcare decision-making. To date, no systematic evaluation of the association of aspirin with irAEs has been published. Therefore, our aim was to determine the association between aspirin use and irAEs in patients receiving immunotherapy by analyzing the data in FAERS. Our research affirms that aspirin users exhibited a higher risk of irAEs when compared to non-aspirin users. Nonetheless, this association displayed variability across distinct cancer types, adverse events, and ICIs.
2 Methods
2.1 Data sources
The study utilized data from the FAERS database, a public repository that houses information on adverse events and medication errors reported to the FDA. This database is an essential tool for the FDA’s post-marketing safety surveillance program for drug and therapeutic biologic products. All data used for this analysis can be accessed at https://fis.fda.gov/sense/app/95239e26-e0be-42d9-a960-9a5f7f1c25ee/sheet/7a47a261-d58b-4203-a8aa-6d3021737452/state/analysis.
2.2 Data collection and screening
Adverse event (AE) reports from ICIs in the FAERS database were collected for this retrospective study. The analysis included every report from the date of each drug’s FDA approval until 1 October 2022. ICIs mainly consists of PD-1 inhibitors (Nivolumab, Pembrolizumab, Cemiplimab, Sintilimab, Camrelizumab, Tislelizumab, Toripalimab), PD-L1 inhibitors (Durvalumab, Atezolizumab, Avelumab), CTLA-4 inhibitors (Ipilimumab, Tremelimumab, Quavonlimab, Bms-986249), Lymphocyte-activation gene 3 (LAG-3) inhibitors (Opdualag, Relatlimab, Favezelimab, Fianlimab), PD-1/LAG-3 bispecific inhibitors (Nivolumab\Relatlimab-Rmbw, Tebotelimab). According to the patient’s medication, the treatment regimen were classified as monotherapy, dual immunotherapy, immunotherapy combined with targeted therapy, immunotherapy combined with chemotherapy, and immune combined antibody drug conjugates (ADC). We defined the use of aspirin during immunotherapy as aspirin users. irAEs were defined using AE terminology from the peer-reviewed immune-related adverse event (irAE) management guidelines (Martins et al., 2019). Patients with at least one irAE were categorized into the irAE group. The irAEs were sorted into primary system organ classes according to the Medical Dictionary for Regulatory Activities (Jing et al., 2022).
2.3 Statistical analysis
In this study, multivariable logistic regression was utilized to analyze adjusted odds ratios (OR) for evaluating the association between aspirin use and irAEs. The model included covariates such as age, sex, ICIs drugs, and treatment regimen. To account for multiple comparisons, Benjamini–Hochberg adjustment was performed using the “p.adjust” function in the “stats” R package. All comparisons are two tailed, and statistical significance was set at an FDR adjusted p < 0.05. The data were processed and analyzed using R statistical software version 4.2.1. On the overall population, we conducted multivariate regression analyses grouping by different tumor types, types of adverse reactions, and system organ classes (SOCs) to determine the impact of aspirin use on irAEs in patients treated with ICIs. Additionally, to further determine if different ICIs had an effect on the results, we performed multivariate logistic regression analyses in patients treated with PD-1 inhibitors, PD-L1 inhibitors, and CTLA-4 inhibitors, respectively.
3 Results
3.1 Baseline characteristics of patients
We collected information on 123,104 patients from FAERS and conducted a multivariate regression analysis (Table 1). Out of these patients, 70,655 were treated with PD-1 inhibitors, 21,315 were treated with PD-L1 inhibitors, 30,653 were treated with CTLA-4 inhibitors, 258 were treated with LAG-3 inhibitors, and 223 were treated with PD-1/LAG-3 bispecific inhibitors (Table 1). Moreover, 5,359 patients (4.4%) reported also taking aspirin (Table 1).
3.2 Association of aspirin treatment with irAEs in different cancer types
The multivariate logistic regression analysis results revealed that aspirin use was associated with an increased risk of irAEs in the pan-cancer analysis (odds ratio (OR) 1.18, 95% confidence interval (CI) 1.10–1.26, FDR adjusted p < 0.001) (Figure 1). After excluding cancer types with a sample size of less than 200, we included 24 cancer types for analysis (Table 1). The further analysis indicated that aspirin use was linked to a higher risk of irAEs in specific cancer types. Specifically, aspirin use was significantly associated with an increased risk of irAEs in lung cancer (OR 1.24, 95% CI 1.10–1.40, FDR adjusted p = 0.003) (Figure 1), mesothelioma (OR 2.90, 95% CI 1.75–4.82, FDR adjusted p < 0.001) (Figure 1), and pancreatic cancer (OR 2.51, 95% CI 1.79–3.51, FDR adjusted p < 0.001) (Figure 1). In contrast, aspirin use was linked to a lower risk of irAEs in lymphoma (OR 0.27, 95% CI 0.11–0.67, FDR adjusted p = 0.029) (Figure 1). However, no significant differences in irAEs were observed in the remaining cancer types (Figure 1).
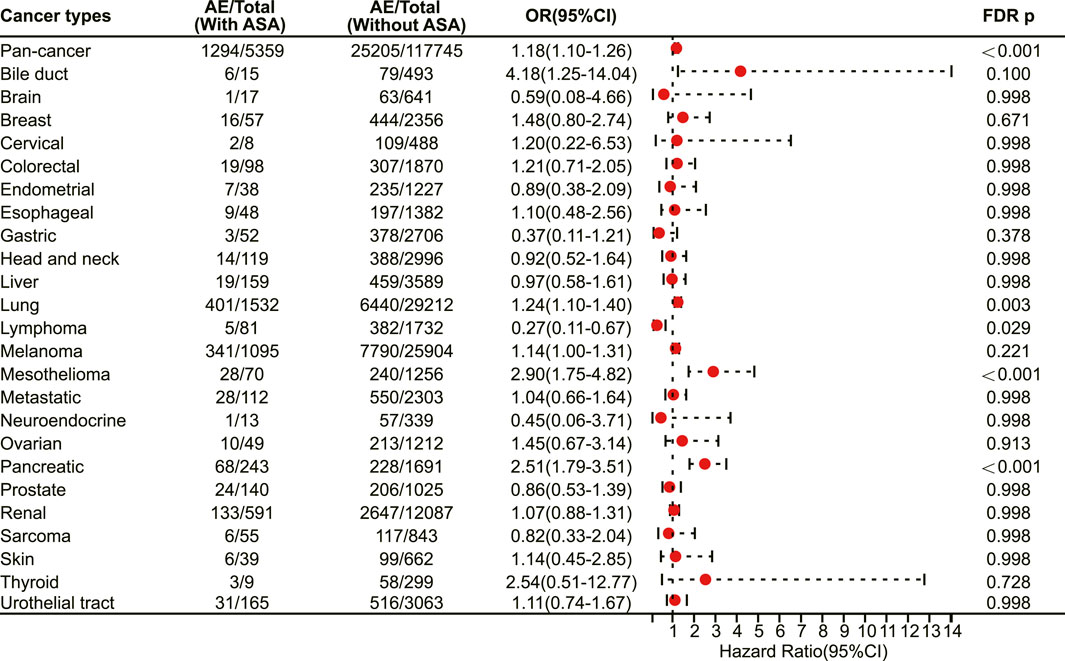
FIGURE 1. The forest plot showing the association between aspirin and irAEs in different cancer types among patients receiving immunotherapy. ASA: Aspirin.
3.3 Association of aspirin treatment with different irAEs
We conducted a survey to determine the association of aspirin with specific irAEs. Our results revealed that aspirin use was correlated with an elevated risk of several adverse reactions, including anaemia (OR 1.24, 95% CI 1.06–1.47, FDR adjusted p = 0.042) (Figure 2), colitis (OR 1.46, 95% CI 1.27–1.66, FDR adjusted p < 0.001) (Figure 2), myocarditis (OR 1.37, 95% CI 1.09–1.71, FDR adjusted p = 0.033) (Figure 2), myositis (OR 1.44, 95% CI 1.12–1.86, FDR adjusted p = 0.033) (Figure 2), pancreatitis (OR 1.69, 95% CI 1.23–2.32, FDR adjusted p = 0.015) (Figure 2), pericarditis (OR 1.93, 95% CI 1.20–3.11, FDR adjusted p = 0.033) (Figure 2) and pneumonitis (OR 1.60, 95% CI 1.39–1.84, FDR adjusted p < 0.001) (Figure 2). On the other hand, aspirin use was associated with a decreased risk of certain adverse reactions, such as rash (OR 0.68, 95% CI 0.56–0.82, FDR adjusted p = 0.001) (Figure 2), Stevens-Johnson syndrome (OR 0.18, 95% CI 0.06–0.56, FDR adjusted p = 0.027) (Figure 2), and thyroiditis (OR 0.48, 95% CI 0.28–0.81, FDR adjusted p = 0.033) (Figure 2).
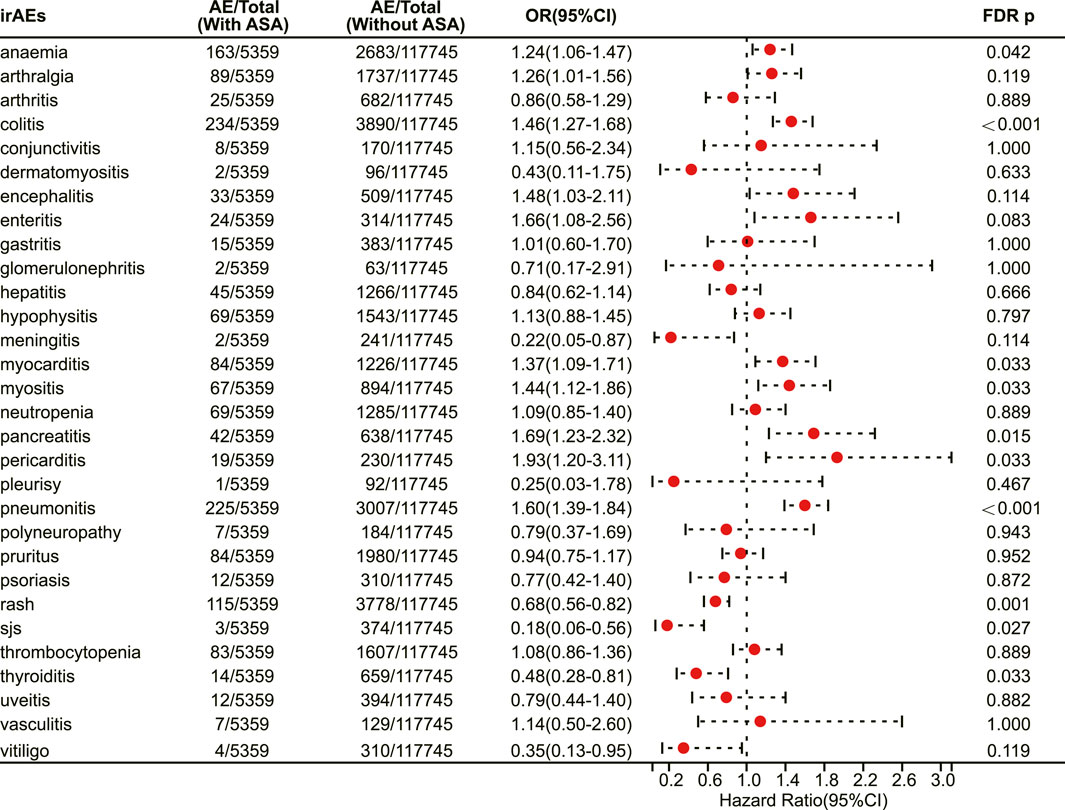
FIGURE 2. The forest plot showing the association between aspirin use and different irAEs among patients receiving immunotherapy. ASA: Aspirin; sjs: Stevens-Johnson syndrome.
3.4 Association of aspirin treatment with irAEs in different organs
Then, we mapped irAEs to their corresponding system organ classes, involving a total of 13 organ systems. Our results demonstrate that aspirin users have a higher risk of developing irAEs in the blood and lymphatic system disorders (OR 1.19, 95% CI 1.06–1.34, FDR adjusted p = 0.019) (Figure 3), cardiac disorders (OR 1.35, 95% CI 1.09–1.66, FDR adjusted p = 0.020) (Figure 3) and respiratory thoracic and mediastinal disorders (OR 1.30, 95% CI 1.12–1.51, FDR adjusted p = 0.004) (Figure 3), while having a lower risk of developing irAEs in the skin and subcutaneous tissue disorders (OR 0.74, 95% CI 0.64–0.86, FDR adjusted p = 0.001) (Figure 3).
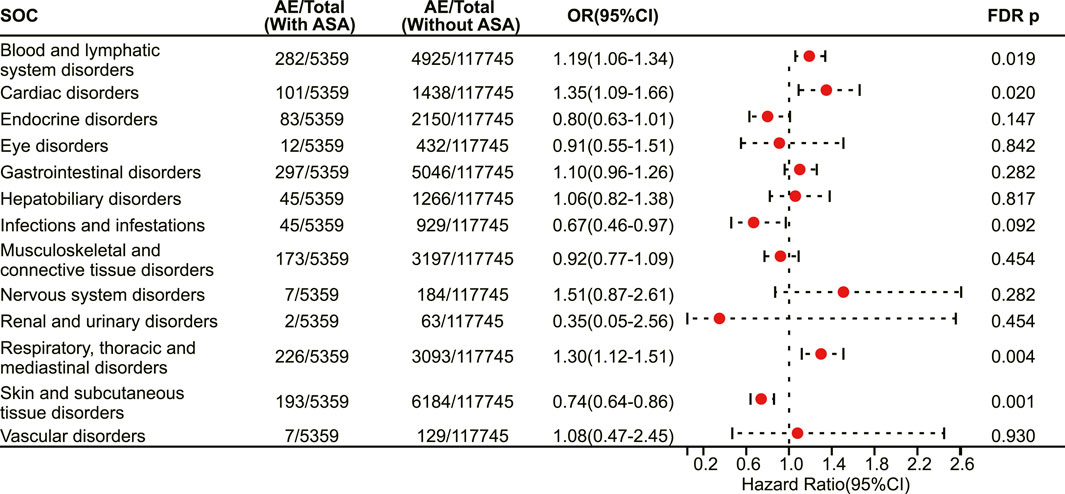
FIGURE 3. The forest plot showing the association between aspirin use and irAEs from different system organ classes (SOC) among patients receiving immunotherapy. ASA: Aspirin.
3.5 Association of aspirin use with irAEs among cancer patients treated with PD-1 inhibitors
We next investigated the association between aspirin use and irAE in patients using different ICIs. In a pan-cancer analysis of patients using PD-1 inhibitors, aspirin use was shown to be associated with a higher risk of irAEs (OR 1.20, 95% CI 1.09–1.31, FDR adjusted p = 0.002) (Figure 4). Further analysis revealed that aspirin use was associated with an increased risk of irAEs in lung cancer (OR 1.25, 95% CI 1.07–1.46, FDR adjusted p = 0.031) (Figure 4) and mesothelioma (OR 3.01, 95% CI 1.57–5.76, FDR adjusted p = 0.013) (Figure 4). In addition, for different adverse reactions, the risk of anaemia (OR 1.52, 95% CI 1.23–1.88, FDR adjusted p = 0.002) (Figure 5), enteritis (OR 2.16, 95% CI 1.29–3.63, FDR adjusted p = 0.040) (Figure 5), pneumonitis (OR 1.61, 95% CI 1.32–1.96, FDR adjusted p < 0.001) and pancreatitis (OR 2.18, 95% CI 1.46–3.25, FDR adjusted p = 0.002) (Figure 5) were higher in aspirin users.
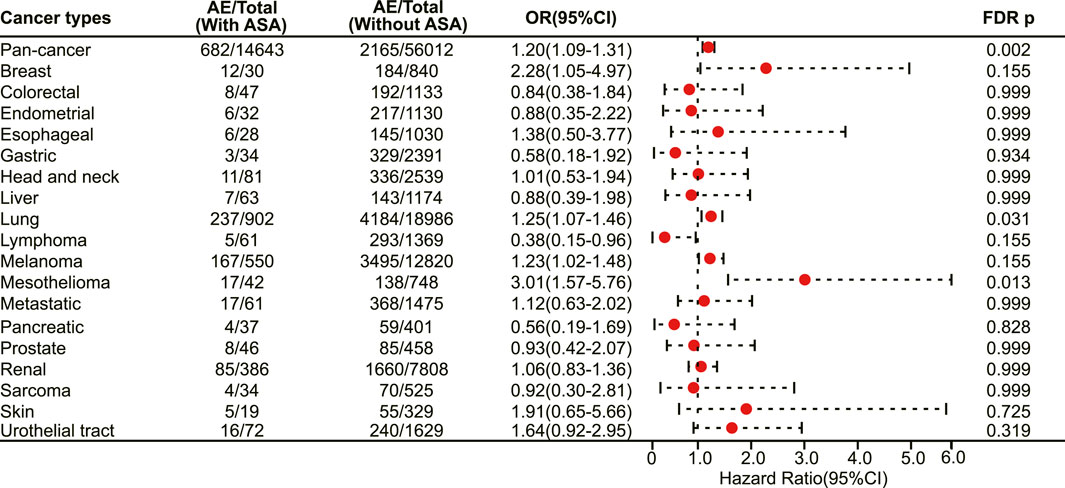
FIGURE 4. The forest plot showing the association between aspirin use and irAEs across different cancer types among patients using PD-1 inhibitors. ASA: Aspirin.
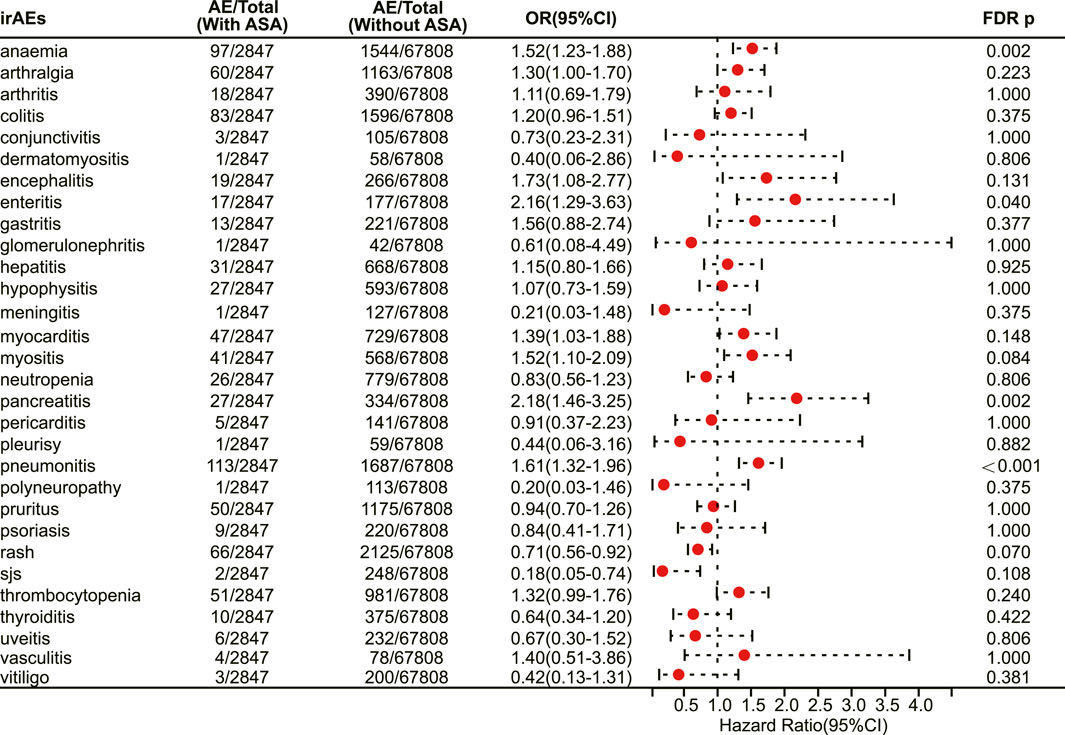
FIGURE 5. The forest plot showing the association between aspirin use and different irAEs among patients using PD-1 inhibitors. ASA: Aspirin; sjs: Stevens-Johnson syndrome.
3.6 Association of aspirin use with irAEs among cancer patients treated with PD-L1 inhibitors
In patients receiving PD-L1 inhibitors, the combination of aspirin demonstrated a tendency to increase adverse reactions in pan-cancer, but there was no statistically significant difference. However, aspirin increased the risk of irAEs in patients with pancreatic cancer (OR 3.48, 95% CI 2.07–5.86, FDR adjusted p < 0.001) (Figure 6). In addition, with respect to specific adverse reactions, the risk of colitis (OR 2.31, 95% CI 1.66–3.23, FDR adjusted p < 0.001) (Figure 7), pericarditis (OR 4.08, 95% CI 1.93–8.63, FDR adjusted p = 0.005) (Figure 7) and pneumonitis (OR 1.57, 95% CI 1.18–2.11, FDR adjusted p = 0.035) (Figure 7) were higher in aspirin users.
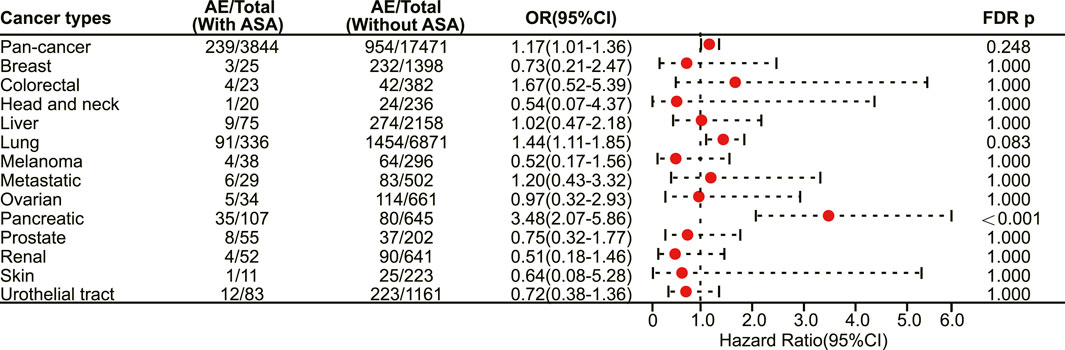
FIGURE 6. The forest plot showing the association between aspirin use and irAEs across different cancer types among patients using PD-L1 inhibitors. ASA: Aspirin.
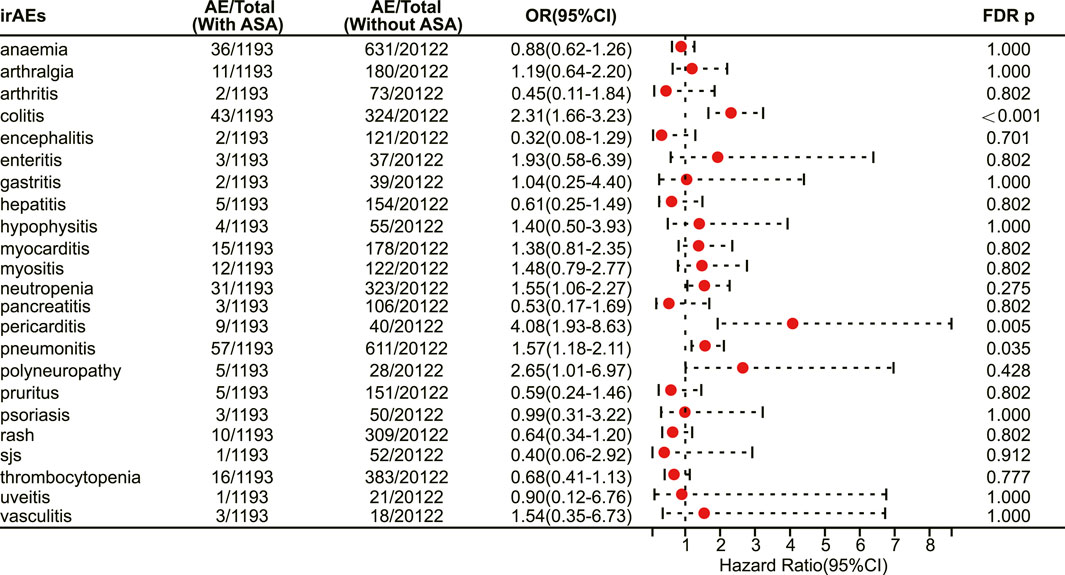
FIGURE 7. The forest plot showing the association between aspirin use and different irAEs among patients using PD-L1 inhibitors. ASA: Aspirin; sjs: Stevens-Johnson syndrome.
3.7 Association of aspirin use with irAEs among cancer patients treated with CTLA-4 inhibitors
In patients receiving CTLA-4 inhibitors, there is still a trend towards an increased risk of adverse reactions with the use of aspirin, but only with statistical significance in pancreatic cancer (OR 2.91, 95% CI 1.71–4.96, FDR adjusted p = 0.002) (Figure 8). No statistical difference was observed among different immune-related adverse events. Finally, subgroup analysis was not performed for patients receiving LAG-3 inhibitors and PD-1/LAG-3 inhibitors due to the small sample size.
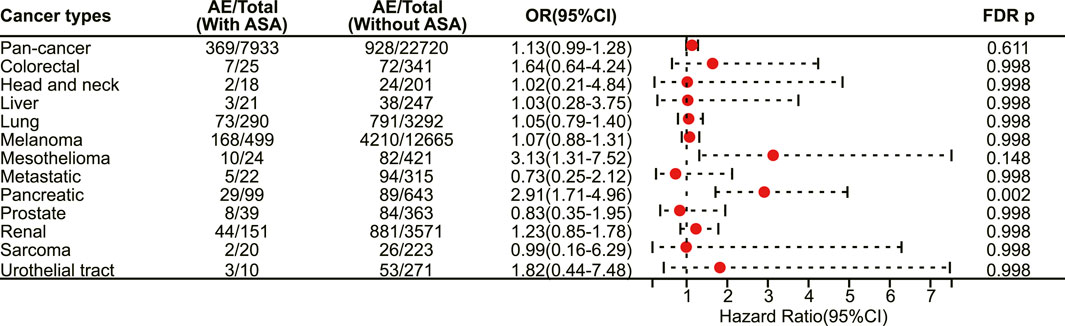
FIGURE 8. The forest plot showing the association between aspirin use and irAEs across different cancer types among patients using CTLA-4 inhibitors. ASA: Aspirin.
3.8 Hypothetical molecular mechanisms linking aspirin treatment to the risk of irAEs
Until now, the specific mechanisms underlying the association of aspirin with irAEs in cancer patients treated with ICIs remain unknown, but some studies have shown that aspirin plays an important role in immune regulation. Aspirin regulates T cells through COX-1 and COX-2 pathways (Zelenay et al., 2015; Rachidi et al., 2017), and activated T cells may lead to increased irAEs risk (Khan and Gerber, 2020). In addition, the modulation of gut microbiota by aspirin may also mediate the increased risk of irAEs (Chaput et al., 2017) (Figure 9).
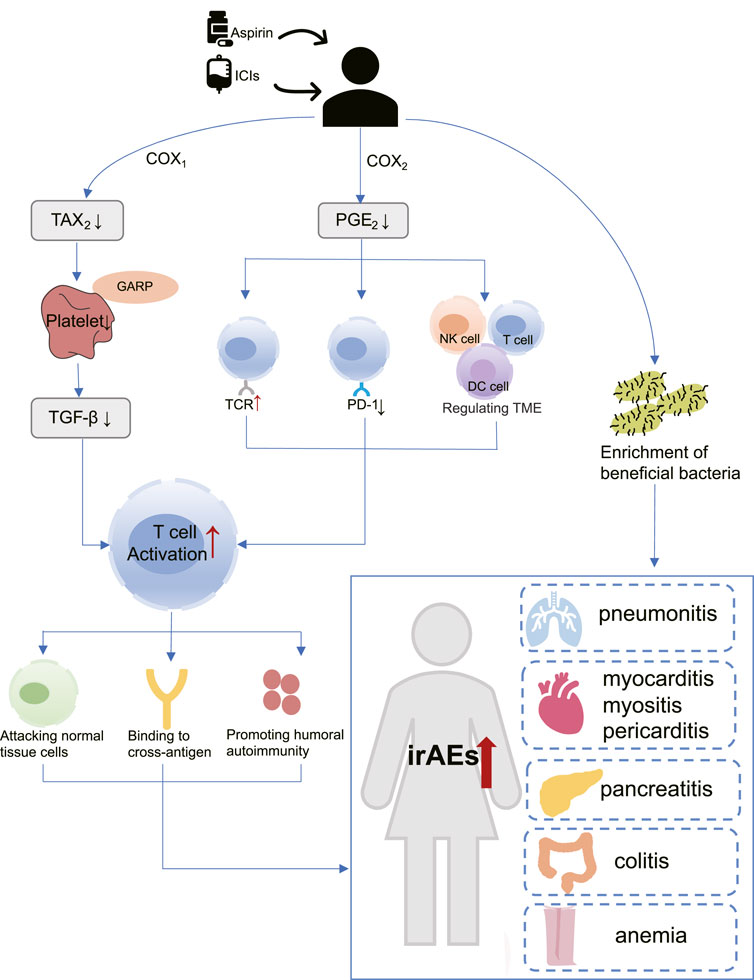
FIGURE 9. The hypothetical mechanism of increased risk of immune adverse reactions with aspirin use in patients receiving immunotherapy. COX-1: Cyclooxygenase-1; COX-2: Cyclooxygenase-2; DC cell: Dendritic cell; GARP: Glycoprotein A repetitions predominant; ICIs: Immune checkpoint inhibitors; irAEs: Immune-related adverse events; NK cell: Natural killer cell; PGE2: Prostaglandin E2; PD-1: programmed cell death-1; TAX2: Thromboxane2; TGF-β: Transforming growth factor-β; TCR: T-cell receptor; TME: tumor microenvironment.
4 Discussion
By understanding how the immune system interacts with tumor cells, scientists have established new therapies for cancer treatment that have brought noteworthy clinical benefits for cancer patients (Morad et al., 2021). However, many cancer patients have underlying diseases, and the presence of other drugs may affect the immunotherapy. ICIs leverage diverse mechanisms and pathways to harness the immune system’s ability to eradicate tumor cells. Consequently, potential interactions between concomitant medications and ICIs transcend the typical assessment of pharmacodynamic and pharmacokinetic interactions between drugs. Aspirin is currently one of the most widely used basic drugs, Previous studies reported that aspirin use is associated with better outcomes with immunotherapy, However, it is not clear whether aspirin use impacts irAEs. This is one of the first studies to analyze the association between aspirin use with irAEs using FEARS data with innovative and comprehensive benefits. Our study showed that aspirin exposure was associated with an increased risk of irAEs in all enrolled cancer patients treated with ICIs. However, it is important to note that the relationship between aspirin use and irAE risk varies across different tumor types, types of irAEs, and various ICIs. Our research findings highlight these distinctions.
As a well-known non-selective COX inhibitor, aspirin irreversibly acetylates the active sites of COX-1 and COX-2, thereby reducing their enzyme activity (Ornelas et al., 2017). COX-1 mainly mediates the formation of physiological prostaglandins, such as Thromboxane A2 (TXA2), which in turn promotes platelet aggregation (Menter and Bresalier, 2023). Aspirin inactivates COX-1 and prevents the production of TXA2, thus acting as an antiplatelet and preventing thrombosis. More importantly, previous studies have confirmed the role of platelets in promoting tumor growth and metastasis (Lichtenberger and Vijayan, 2019). Rachidi et al. (2017) found that a protein called Glycoprotein A repetitions predominant (GARP) exists on the surface of platelets, which traps and activates Transforming growth factor-β (TGF-β). TGF-β is an immunomodulatory molecule that suppresses CD4 and CD8 T cells, allowing tumors to evade the immune system. Riesenberg et al. (2019) confirmed through a mouse model that the antiplatelet effect of aspirin can inhibit TGF-β signaling, thereby enhancing T cell function, and synergistically exerting anti-tumor effects with PD-1 blocker.
COX-2 is an inducer of enzymes that promote the synthesis of inflammatory prostaglandins, such as Prostaglandin E2 (PGE2), which can cause inflammation (Jin et al., 2023). Interestingly, PGE2 has been shown to regulate the function of various immune cells within the tumor microenvironment (TME), including myeloid-derived suppressor cells (MDSCs), dendritic (DC) cells, natural killer (NK) cells, CD4 and CD8 T cells, resulting in immune evasion (Zelenay et al., 2015; Böttcher et al., 2018; Bonavita et al., 2020). Moreover, PGE2 is capable of upregulating PD-L1 expression (Goto et al., 2020) and inhibit T cell receptor activation (Newick et al., 2016). The above study suggests that aspirin may exert immunomodulatory effects and enhance T cell activation by inhibiting COX2/PGE2 pathway (Wei et al., 2022; Jin et al., 2023).
Aspirin has been found to aid ICIs in breaking immune tolerance and amplifying the immune response (Zelenay et al., 2015). Unfortunately, it is important to note that immune activation is not limited to tumor-specific responses. Some researchers have proposed that activated effector T cells also attack normal non-tumor tissues while increasing their anti-tumor activity (Khan and Gerber, 2020; Ronen et al., 2022). T-cell receptor (TCR) sequencing studies have provided evidence to support this theory (Porciello et al., 2022; Sanromán Á et al., 2023). In patients treated with Ipiliumumab, researchers detected greater CD4 and CD8 T cell diversity in irAEs patients compared with those who did not experience significant adverse reactions (Oh et al., 2017). A recent work from Luoma and others has demonstrated the presence of a large number of CD8 T cells with high cytotoxicity and proliferation ability in the colon of patients with colitis, and these CD8 T cells are mostly from tissue-resident populations (Luoma et al., 2020). Together, these studies support that irAEs may be caused by the mobilization of a large number of T cells (Ramos-Casals et al., 2020). Other studies have shown that the presence of cross-antigens can also influence T-cell responses. In a study by Berner et al., 73 patients with NSCLC who received anti-PD-1 treatment were included, and nine common T-cell antigens were identified between tumor tissues and skin. This indicates that ICIs target both non-small-cell lung cancer (NSCLC) cancer and skin, leading to immune-related dermal toxicity while treating tumors (Berner et al., 2019). On the other hand, self-antigens from dying cells are captured by antigen-presenting cells (APCs) during tumor cell killing. These APCs then migrate to lymph nodes and activate more reactive T and B cells, These novel T cell clones may initiate a distinct immuno-editing wave, leading to adverse reactions (Yost et al., 2019; Baumjohann and Brossart, 2021).
Multiple clinical studies have investigated the potential of aspirin in enhancing the immune response in immunotherapy. Cortellini et al. (2020) reported that concurrent use of aspirin can improve overall response rate (ORR) among patients with solid tumors receiving PD-1/PD-L1 checkpoint inhibitors. Another study have highlighted that aspirin can prolong overall survival (OS) (Kostine et al., 2021). Furthermore, a meta-analysis suggested a significant intensification in progression-free survival (PFS) with concurrent use of aspirin and ICIs (Zhang et al., 2021). The above statements have demonstrated the synergistic effect of aspirin in ICIs. Therefore, aspirin may have underestimated immunomodulatory effects can amplify immune activation induced by ICIs. However, coins always have two sides. Over-activated T cells lack tumor specificity, so we have to consider the impact of aspirin on irAEs. We propose that aspirin may enhances T cell activation through inhibition of PGE2 and platelets, contributing to the increased irAEs.
Moreover, it has been shown that microbiota composition was a key factor in maintaining immune homeostasis, and may affect the occurrence of irAEs (Dora et al., 2023). Chaput et al. (2017) demonstrated that protective bacteria in the gut led to positive outcomes for patients who receive ipilimumab therapy, but also with a higher incidence of ipilimumab-induced colitis. Mouse models have shown that aspirin modulates the gut microbiota by enrichment of probiotics (Zhao et al., 2020; Brennan et al., 2021). This may also be one of the reasons why aspirin is associated with an increased risk of irAEs occurring (Figure 9).
Our research has uncovered a connection between the use of aspirin and an increased susceptibility to irAEs in pan-cancer patients. Delving deeper into our findings, we have identified a notably increased risk of irAEs among patients afflicted with specific cancer types, including lung cancer, mesothelioma, and pancreatic cancer. Conversely, a perplexing reduction in irAE risk has emerged in lymphoma patients. Remarkably, these observations constitute a novel contribution to the field, as they have not been previously documented in existing literature.
In stark contrast to prior retrospective studies, our comprehensive analysis has demonstrated robust statistical significance in support of these findings (Gandhi et al., 2020; Sieber et al., 2022). We posit that aspirin’s influence on the occurrence of irAEs may be mediated through the COX pathway, thereby shedding light on a potential mechanistic explanation. Furthermore, the intriguing divergence observed within the lymphoma subgroup warrants further investigation. While our data show a diminished risk of irAEs in lymphoma patients, it is essential to acknowledge that this subgroup comprises a relatively small sample size, constituting only 1.5% of the overall study population. It is conceivable that this statistical anomaly may be attributed to the limited representation of lymphoma cases, or it may signify the existence of hitherto undiscovered mechanisms that demand further exploration and scrutiny. In addition, aspirin use, prescribing status, or combination of aspirin with these conditions. These circumstances will also have an impact on our results (Colard-Thomas et al., 2023).
Our in-depth analysis revealed a significant association between the use of aspirin and a range of irAEs. Specifically, we observed that aspirin use markedly increased the risk of patients experiencing irAEs such as pneumonia, myocarditis, myositis, pericarditis, pancreatitis, colitis, and anemia. In contrast, the risk of irAEs related to conditions like rash, Stevens-Johnson syndrome, and thyroiditis was notably reduced. To further support our conclusions, we conducted a comprehensive review of previously published articles, seeking evidence that aligns with the associations we identified. Prior studies may not have fully considered the relationship between aspirin and irAEs or may not have detected these associations due to differences in research methodologies. Nonetheless, our study fills this knowledge gap and provides healthcare professionals with a more comprehensive understanding of aspirin’s role in irAE risk.
In summary, these findings underscore the need for heightened vigilance among clinicians when treating patients with immunotherapy, especially in cases related to irAEs affecting organs or systems such as the gastrointestinal tract, lungs, pancreas, heart, and anemia. However, it is also essential to consider an additional factor, namely, the widespread use of aspirin in cardiovascular disease treatment (Byrne and Colleran, 2020), where a patient’s history of cardiovascular conditions may be one of the factors contributing to the heightened risk of irAEs (Yousif et al., 2023). Therefore, a comprehensive assessment of the patient’s overall health and treatment needs is crucial.
Despite some limitations in our study and a lack of supporting mechanistic research, our research still provides valuable pharmacological guidance to the greatest extent possible. For example, when using aspirin in patients receiving PD-1 inhibitors, it is advisable to pay closer attention to indicators related to anemia, enteritis, pneumonia, and pancreatitis. Similarly, for patients undergoing PD-L1 inhibitor treatment, increased attention should be directed towards indicators associated with colitis, pericarditis, and pneumonia. Furthermore, in patients receiving CTLA-4 inhibitors, no association has been observed between aspirin and irAEs, although further research is needed to confirm this, in order to offer clinicians more precise treatment guidelines.
Overall, our study highlights the potential risks associated with aspirin use in patients receiving immunotherapy, particularly with regards to irAEs. These findings could inform clinical decision-making and improve patient safety.
5 Study limitations
The FAERS database, as a voluntary, passive, and non-mandatory reporting system, faces inherent challenges. These include incompleteness, inaccuracy, inconsistency, and delay in reporting adverse events. These limitations stem from various factors, primarily the lack of detailed patient characteristics, drug exposure information, and outcome details, such as the dose and duration of aspirin use, as well as whether patients received other treatment regimens and the sequence of medication. These factors may influence the associations observed and the study outcomes. Therefore, it is essential to carefully consider these limitations, particularly when interpreting the research results.
Furthermore, our analysis is influenced by the uneven distribution of cases within the database, with a higher number of lung cancer patients but significantly fewer patients with other cancer types. This non-uniform case distribution may introduce bias and restrict the generalizability and applicability of our study findings.
To overcome these limitations and provide more robust insights, further prospective clinical studies are urgently needed. Additionally, the mechanisms underlying the association between aspirin use and irAEs remain unclear, underscoring the need for fundamental research to address these uncertainties and advance our understanding of immunotherapy.
6 Conclusion
This study has revealed a significant association between aspirin usage and irAEs in cancer patients undergoing ICIs. It is important to note that this association exhibits variations depending on the specific cancer type, the nature of adverse events, and the specific type of ICIs being utilized. These findings underscore the importance of assessing the effect of baseline drugs, including aspirin, on the safety and efficacy of ICIs in tumor treatment, and tailoring treatment plans accordingly on an individual basis.
Data availability statement
The original contributions presented in the study are included in the article/Supplementary Material, further inquiries can be directed to the corresponding author.
Author contributions
HY: Data curation, Formal Analysis, Investigation, Project administration, Resources, Validation, Visualization, Writing–original draft, Writing–review and editing. ZL: Investigation, Methodology, Writing–review and editing. RL: Methodology, Resources, Validation, Visualization, Writing–review and editing. RH: Data curation, Formal Analysis, Investigation, Resources, Software, Validation, Visualization, Writing–review and editing. XP: Conceptualization, Funding acquisition, Methodology, Supervision, Writing–review and editing.
Funding
The author(s) declare financial support was received for the research, authorship, and/or publication of this article. The work was supported by the National Key Research and Development Program of China (2021YFE0206600), National Natural Science Foundation of China (82172842 and 81672386), Sichuan Science and Technology Program (2022YFSY0012, 2021ZYCD011, 2021YFSY0008, 2021CDDZ-25, 2021CDZG-24), Chengdu International Science and Technology Cooperation Program (2022-GH03-00004-HZ), West China Nursing Discipline Development Special Fund Project (HXHL21008), the Post-Doctor Research Project, West China Hospital, Sichuan University (2020HXBH119), Translational medicine fund of West China Hospital (CGZH19002), the China Medical Board (Grant 22-482), and clinical research incubation project of West China Hospital (20HXFH037). The funders had no role in study design, data collection and analysis, decision to publish, or preparation of the manuscript.
Acknowledgments
This study was performed using the FDA Adverse Event Reporting System (FAERS) database that was provided by the FDA. The information, the results, or interpretation of the current study do not represent any opinion of the FDA.
Conflict of interest
Author RH was employed by Hangzhou Linan Guorui Health Industry Investment Co., Ltd.
The remaining authors declare that the research was conducted in the absence of any commercial or financial relationships that could be construed as a potential conflict of interest.
Publisher’s note
All claims expressed in this article are solely those of the authors and do not necessarily represent those of their affiliated organizations, or those of the publisher, the editors and the reviewers. Any product that may be evaluated in this article, or claim that may be made by its manufacturer, is not guaranteed or endorsed by the publisher.
References
Algra, A. M., and Rothwell, P. M. (2012). Effects of regular aspirin on long-term cancer incidence and metastasis: a systematic comparison of evidence from observational studies versus randomised trials. Lancet Oncol. 13, 518–527. doi:10.1016/S1470-2045(12)70112-2
Baumjohann, D., and Brossart, P. (2021). T follicular helper cells: linking cancer immunotherapy and immune-related adverse events. J. Immunother. Cancer 9, e002588. doi:10.1136/jitc-2021-002588
Berner, F., Bomze, D., Diem, S., Ali, O. H., FäSSLER, M., Ring, S., et al. (2019). Association of checkpoint inhibitor-induced toxic effects with shared cancer and tissue antigens in non-small cell lung cancer. JAMA Oncol. 5, 1043–1047. doi:10.1001/jamaoncol.2019.0402
Bockorny, B., Grossman, J. E., and Hidalgo, M. (2022). Facts and hopes in immunotherapy of pancreatic cancer. Clin. Cancer Res. 28, 4606–4617. doi:10.1158/1078-0432.CCR-21-3452
Bonavita, E., Bromley, C. P., Jonsson, G., Pelly, V. S., Sahoo, S., Walwyn-Brown, K., et al. (2020). Antagonistic inflammatory phenotypes dictate tumor fate and response to immune checkpoint blockade. Immunity 53, 1215–1229.e8. doi:10.1016/j.immuni.2020.10.020
BöTTCHER, J. P., Bonavita, E., Chakravarty, P., Blees, H., Cabeza-Cabrerizo, M., Sammicheli, S., et al. (2018). NK cells stimulate recruitment of cDC1 into the tumor microenvironment promoting cancer immune control. Cell. 172, 1022–1037. doi:10.1016/j.cell.2018.01.004
Brahmer, J. R., Lacchetti, C., Schneider, B. J., Atkins, M. B., Brassil, K. J., Caterino, J. M., et al. (2018). Management of immune-related adverse events in patients treated with immune checkpoint inhibitor therapy: American society of clinical oncology clinical practice guideline. J. Clin. Oncol. 36, 1714–1768. doi:10.1200/JCO.2017.77.6385
Brennan, C. A., Nakatsu, G., Gallini Comeau, C. A., Drew, D. A., Glickman, J. N., Schoen, R. E., et al. (2021). Aspirin modulation of the colorectal cancer-associated microbe fusobacterium nucleatum. mBio 12, e00547-21. doi:10.1128/mBio.00547-21
Byrne, R. A., and Colleran, R. (2020). Aspirin for secondary prevention of cardiovascular disease. Lancet 395, 1462–1463. doi:10.1016/S0140-6736(20)30799-6
Chaput, N., Lepage, P., Coutzac, C., Soularue, E., le Roux, K., Monot, C., et al. (2017). Baseline gut microbiota predicts clinical response and colitis in metastatic melanoma patients treated with ipilimumab. Ann. Oncol. 28, 1368–1379. doi:10.1093/annonc/mdx108
Colard-Thomas, J., Thomas, Q. D., and Viala, M. (2023). Comedications with immune checkpoint inhibitors: involvement of the microbiota, impact on efficacy and practical implications. Cancers (Basel) 15. doi:10.3390/cancers15082276
Cortellini, A., Tucci, M., Adamo, V., Stucci, L. S., Russo, A., Tanda, E. T., et al. (2020). Integrated analysis of concomitant medications and oncological outcomes from PD-1/PD-L1 checkpoint inhibitors in clinical practice. J. Immunother. Cancer 8, e001361. doi:10.1136/jitc-2020-001361
Debien, V., de Caluwé, A., Wang, X., Piccart-Gebhart, M., Tuohy, V. K., Romano, E., et al. (2023). Immunotherapy in breast cancer: an overview of current strategies and perspectives. NPJ Breast Cancer 9, 7. doi:10.1038/s41523-023-00508-3
Doki, Y., Ajani, J. A., Kato, K., Xu, J., Wyrwicz, L., Motoyama, S., et al. (2022). Nivolumab combination therapy in advanced esophageal squamous-cell carcinoma. N. Engl. J. Med. 386, 449–462. doi:10.1056/NEJMoa2111380
Dora, D., Bokhari, S. M. Z., Aloss, K., Takacs, P., Desnoix, J. Z., SzklenáRIK, G., et al. (2023). Implication of the gut microbiome and microbial-derived metabolites in immune-related adverse events: emergence of novel biomarkers for cancer immunotherapy. Int. J. Mol. Sci. 24, 2769. doi:10.3390/ijms24032769
Drew, D. A., and Chan, A. T. (2021). Aspirin in the prevention of colorectal neoplasia. Annu. Rev. Med. 72, 415–430. doi:10.1146/annurev-med-060319-120913
Fijałkowski, Ł., Skubiszewska, M., Grześk, G., Koech, F. K., and Nowaczyk, A. (2022). Acetylsalicylic acid-primus inter pares in Pharmacology. Molecules 27, 8412. doi:10.3390/molecules27238412
Gadgeel, S., RodríGUEZ-Abreu, D., Speranza, G., Esteban, E., Felip, E., DóMINE, M., et al. (2020). Updated analysis from KEYNOTE-189: Pembrolizumab or placebo plus pemetrexed and platinum for previously untreated metastatic nonsquamous non-small-cell lung cancer. J. Clin. Oncol. 38, 1505–1517. doi:10.1200/JCO.19.03136
Gandhi, S., Pandey, M., Ammannagari, N., Wang, C., Bucsek, M. J., Hamad, L., et al. (2020). Impact of concomitant medication use and immune-related adverse events on response to immune checkpoint inhibitors. Immunotherapy 12, 141–149. doi:10.2217/imt-2019-0064
Goto, S., Konnai, S., Hirano, Y., Kohara, J., Okagawa, T., Maekawa, N., et al. (2020). Upregulation of PD-L1 expression by prostaglandin E(2) and the enhancement of IFN-γ by anti-PD-L1 antibody combined with a COX-2 inhibitor in mycoplasma bovis infection. Front. Vet. Sci. 7, 12. doi:10.3389/fvets.2020.00012
Jelinic, P., Ricca, J., van Oudenhove, E., Olvera, N., Merghoub, T., Levine, D. A., et al. (2018). Immune-active microenvironment in small cell carcinoma of the ovary, hypercalcemic type: rationale for immune checkpoint blockade. J. Natl. Cancer Inst. 110, 787–790. doi:10.1093/jnci/djx277
Jing, Y., Chen, X., Li, K., Liu, Y., Zhang, Z., Chen, Y., et al. (2022). Association of antibiotic treatment with immune-related adverse events in patients with cancer receiving immunotherapy. J. Immunother. Cancer 10, e003779. doi:10.1136/jitc-2021-003779
Jin, K., Qian, C., Lin, J., and Liu, B. (2023). Cyclooxygenase-2-Prostaglandin E2 pathway: a key player in tumor-associated immune cells. Front. Oncol. 13, 1099811. doi:10.3389/fonc.2023.1099811
Khan, S., and Gerber, D. E. (2020). Autoimmunity, checkpoint inhibitor therapy and immune-related adverse events: a review. Semin. Cancer Biol. 64, 93–101. doi:10.1016/j.semcancer.2019.06.012
Kostine, M., Mauric, E., Tison, A., Barnetche, T., Barre, A., Nikolski, M., et al. (2021). Baseline co-medications may alter the anti-tumoural effect of checkpoint inhibitors as well as the risk of immune-related adverse events. Eur. J. Cancer 157, 474–484. doi:10.1016/j.ejca.2021.08.036
Lichtenberger, L. M., and Vijayan, K. V. (2019). Are platelets the primary target of aspirin's remarkable anticancer activity? Cancer Res. 79, 3820–3823. doi:10.1158/0008-5472.CAN-19-0762
Livingstone, E., Zimmer, L., Hassel, J. C., Fluck, M., Eigentler, T. K., Loquai, C., et al. (2022). Adjuvant nivolumab plus ipilimumab or nivolumab alone versus placebo in patients with resected stage IV melanoma with no evidence of disease (IMMUNED): final results of a randomised, double-blind, phase 2 trial. Lancet 400, 1117–1129. doi:10.1016/S0140-6736(22)01654-3
Luoma, A. M., Suo, S., Williams, H. L., Sharova, T., Sullivan, K., Manos, M., et al. (2020). Molecular pathways of colon inflammation induced by cancer immunotherapy. Cell. 182, 655–671. doi:10.1016/j.cell.2020.06.001
Martins, F., Sofiya, L., Sykiotis, G. P., Lamine, F., Maillard, M., Fraga, M., et al. (2019). Adverse effects of immune-checkpoint inhibitors: epidemiology, management and surveillance. Nat. Rev. Clin. Oncol. 16, 563–580. doi:10.1038/s41571-019-0218-0
Menter, D. G., and Bresalier, R. S. (2023). An aspirin a day: new pharmacological developments and cancer chemoprevention. Annu. Rev. Pharmacol. Toxicol. 63, 165–186. doi:10.1146/annurev-pharmtox-052020-023107
Morad, G., Helmink, B. A., Sharma, P., and Wargo, J. A. (2021). Hallmarks of response, resistance, and toxicity to immune checkpoint blockade. Cell. 184, 5309–5337. doi:10.1016/j.cell.2021.09.020
Newick, K., O'Brien, S., Sun, J., Kapoor, V., Maceyko, S., Lo, A., et al. (2016). Augmentation of car T-cell trafficking and antitumor efficacy by blocking protein kinase A localization. Cancer Immunol. Res. 4, 541–551. doi:10.1158/2326-6066.CIR-15-0263
Oh, D. Y., Cham, J., Zhang, L., Fong, G., Kwek, S. S., Klinger, M., et al. (2017). Immune toxicities elicted by CTLA-4 blockade in cancer patients are associated with early diversification of the T-cell repertoire. Cancer Res. 77, 1322–1330. doi:10.1158/0008-5472.CAN-16-2324
Ornelas, A., Zacharias-Millward, N., Menter, D. G., Davis, J. S., Lichtenberger, L., Hawke, D., et al. (2017). Beyond COX-1: the effects of aspirin on platelet biology and potential mechanisms of chemoprevention. Cancer Metastasis Rev. 36, 289–303. doi:10.1007/s10555-017-9675-z
Pauken, K. E., Dougan, M., Rose, N. R., Lichtman, A. H., and Sharpe, A. H. (2019). Adverse events following cancer immunotherapy: obstacles and opportunities. Trends Immunol. 40, 511–523. doi:10.1016/j.it.2019.04.002
Porciello, N., Franzese, O., D'Ambrosio, L., Palermo, B., and Nisticò, P. (2022). T-cell repertoire diversity: friend or foe for protective antitumor response? J. Exp. Clin. Cancer Res. 41, 356. doi:10.1186/s13046-022-02566-0
Rachidi, S., Metelli, A., Riesenberg, B., Wu, B. X., Nelson, M. H., Wallace, C., et al. (2017). Platelets subvert T cell immunity against cancer via GARP-TGFβ axis. Sci. Immunol. 2, eaai7911. doi:10.1126/sciimmunol.aai7911
Ramos-Casals, M., Brahmer, J. R., Callahan, M. K., Flores-CháVEZ, A., Keegan, N., Khamashta, M. A., et al. (2020). Immune-related adverse events of checkpoint inhibitors. Nat. Rev. Dis. Prim. 6, 38. doi:10.1038/s41572-020-0160-6
Riesenberg, B. P., Ansa-Addo, E. A., Gutierrez, J., Timmers, C. D., Liu, B., and Li, Z. (2019). Cutting edge: targeting thrombocytes to rewire anticancer immunity in the tumor microenvironment and potentiate efficacy of PD-1 blockade. J. Immunol. 203, 1105–1110. doi:10.4049/jimmunol.1900594
Ronen, D., Bsoul, A., Lotem, M., Abedat, S., Yarkoni, M., Amir, O., et al. (2022). Exploring the mechanisms underlying the cardiotoxic effects of immune checkpoint inhibitor therapies. Basel: Vaccines, 10.
Rothwell, P. M., Price, J. F., Fowkes, F. G., Zanchetti, A., Roncaglioni, M. C., Tognoni, G., et al. (2012). Short-term effects of daily aspirin on cancer incidence, mortality, and non-vascular death: analysis of the time course of risks and benefits in 51 randomised controlled trials. Lancet 379, 1602–1612. doi:10.1016/S0140-6736(11)61720-0
Rothwell, P. M., Wilson, M., Elwin, C. E., Norrving, B., Algra, A., Warlow, C. P., et al. (2010). Long-term effect of aspirin on colorectal cancer incidence and mortality: 20-year follow-up of five randomised trials. Lancet 376, 1741–1750. doi:10.1016/S0140-6736(10)61543-7
Rudin, C. M., Awad, M. M., Navarro, A., Gottfried, M., Peters, S., Csőszi, T., et al. (2020). Pembrolizumab or placebo plus etoposide and platinum as first-line therapy for extensive-stage small-cell lung cancer: randomized, double-blind, phase III KEYNOTE-604 study. J. Clin. Oncol. 38, 2369–2379. doi:10.1200/JCO.20.00793
SanromáN Á, F., Joshi, K., Au, L., Chain, B., and Turajlic, S. (2023). TCR sequencing: applications in immuno-oncology research. Immunooncol Technol. 17, 100373. doi:10.1016/j.iotech.2023.100373
Schneider, B. J., Naidoo, J., Santomasso, B. D., Lacchetti, C., Adkins, S., Anadkat, M., et al. (2021). Management of immune-related adverse events in patients treated with immune checkpoint inhibitor therapy: ASCO guideline update. J. Clin. Oncol. 39, 4073–4126. doi:10.1200/JCO.21.01440
Sieber, B., Strauss, J., Li, Z., and Gatti-Mays, M. E. (2022). Concomitant medication effects on immune checkpoint inhibitor efficacy and toxicity. Front. Oncol. 12, 836934. doi:10.3389/fonc.2022.836934
Waldman, A. D., Fritz, J. M., and Lenardo, M. J. (2020). A guide to cancer immunotherapy: from T cell basic science to clinical practice. Nat. Rev. Immunol. 20, 651–668. doi:10.1038/s41577-020-0306-5
Wei, J., Zhang, J., Wang, D., Cen, B., Lang, J. D., and Dubois, R. N. (2022). The COX-2-PGE2 pathway promotes tumor evasion in colorectal adenomas. Cancer Prev. Res. (Phila) 15, 285–296. doi:10.1158/1940-6207.CAPR-21-0572
Xu, C., Chen, Y. P., du, X. J., Liu, J. Q., Huang, C. L., Chen, L., et al. (2018). Comparative safety of immune checkpoint inhibitors in cancer: systematic review and network meta-analysis. Bmj 363, k4226. doi:10.1136/bmj.k4226
Yost, K. E., Satpathy, A. T., Wells, D. K., Qi, Y., Wang, C., Kageyama, R., et al. (2019). Clonal replacement of tumor-specific T cells following PD-1 blockade. Nat. Med. 25, 1251–1259. doi:10.1038/s41591-019-0522-3
Yousif, L. I., Screever, E. M., Versluis, D., Aboumsallem, J. P., Nierkens, S., Manintveld, O. C., et al. (2023). Risk factors for immune checkpoint inhibitor-mediated cardiovascular toxicities. Curr. Oncol. Rep. 25, 753–763. doi:10.1007/s11912-023-01414-4
Zelenay, S., van der Veen, A. G., BöTTCHER, J. P., Snelgrove, K. J., Rogers, N., Acton, S. E., et al. (2015). Cyclooxygenase-dependent tumor growth through evasion of immunity. Cell. 162, 1257–1270. doi:10.1016/j.cell.2015.08.015
Zhang, Y., Chen, H., Chen, S., Li, Z., Chen, J., and Li, W. (2021). The effect of concomitant use of statins, NSAIDs, low-dose aspirin, metformin and beta-blockers on outcomes in patients receiving immune checkpoint inhibitors: a systematic review and meta-analysis. Oncoimmunology 10, 1957605. doi:10.1080/2162402X.2021.1957605
Keywords: immune checkpoint Inhibitors (ICIs), aspirin, immune-related adverse events (irAEs), cancer, US food and drug administration adverse event reporting system (FAERS) database
Citation: Yang H, Liu Z, Li R, Huang R and Peng X (2023) The association between aspirin use and immune-related adverse events in specific cancer patients receiving ICIs therapy: analysis of the FAERS database. Front. Pharmacol. 14:1259628. doi: 10.3389/fphar.2023.1259628
Received: 16 July 2023; Accepted: 31 October 2023;
Published: 15 November 2023.
Edited by:
Elizabeth S. Fernandes, Pelé Pequeno Príncipe Research Institute, BrazilReviewed by:
Jian Zang, Fourth Military Medical University, ChinaElina Jerschow, Montefiore Medical Center, United States
Copyright © 2023 Yang, Liu, Li, Huang and Peng. This is an open-access article distributed under the terms of the Creative Commons Attribution License (CC BY). The use, distribution or reproduction in other forums is permitted, provided the original author(s) and the copyright owner(s) are credited and that the original publication in this journal is cited, in accordance with accepted academic practice. No use, distribution or reproduction is permitted which does not comply with these terms.
*Correspondence: Xingchen Peng, pxx2014@163.com
†These authors have contributed equally to this work and share first authorship