- 1School of Integrated Chinese and Western Medicine, Hunan University of Chinese Medicine, Changsha, China
- 2Hunan Province Key Laboratory of Cerebrovascular Disease Prevention and Treatment of Integrated Traditional Chinese and Western Medicine, Hunan University of Chinese Medicine, Changsha, China
- 3School of Traditional Chinese Medicine, Hunan University of Chinese Medicine, Changsha, China
The incidence of ischemic stroke has been increasing annually with an unfavorable prognosis. Cerebral ischemia reperfusion injury can exacerbate nerve damage. Effective mitochondrial quality control including mitochondrial fission, fusion and autophagy, is crucial for maintaining cellular homeostasis. Several studies have revealed the critical role of mitophagy in Cerebral ischemia reperfusion injury. Cerebral ischemia and hypoxia induce mitophagy, and mitophagy exhibits positive and negative effects in cerebral ischemia reperfusion injury. Studies have shown that Chinese herbal medicine can alleviate Cerebral ischemia reperfusion injury and serve as a neuroprotective agent by inhibiting or promoting mitophagy-mediated pathways. This review focuses on the mitochondrial dynamics and mitophagy-related pathways, as well as the role of mitophagy in ischemia reperfusion injury. Additionally, it discusses the therapeutic potential and benefits of Chinese herbal monomers and decoctions in the treatment of ischemic stroke.
1 Introduction
Stroke is the most common serious manifestation of cerebrovascular disease (Feske, 2021). Ischemic stroke accounts for the largest proportion of all types of stroke and has high mortality and severe disability rates worldwide (Kim et al., 2020; Thayabaranathan et al., 2022; Tsao et al., 2023). The main lesion of ischemic stroke is cerebral infarction, which causes clinical classic symptoms such as facial droop, weakness or numbness in arm, difficulty speaking, confusion, loss of vision and poor balance (Walter, 2022). The brain is the most sensitive of all human organs to ischemia. Insufficient blood supply to cerebral tissue, combined with hypoxia and glucose deprivation, results in irreversible impairment of neuronal function and tissue integrity (Feske, 2021). The ultimate goal of therapeutic intervention for ischemic stroke is to restore sufficient blood flow to the damaged tissue. The common modern treatments include surgery, thrombolysis and thrombectomy (Herpich and Rincon, 2020).
The process of restoring blood flow after cerebral ischemia and inducing tissue reperfusion injury is commonly known as cerebral ischemia/reperfusion (I/R) injury (Kalogeris et al., 2016). The pathological mechanisms of cerebral I/R injury are complex, including oxidative stress, ion balance disturbance, inflammation responses, mitochondrial energy metabolism disturbance, mitophagy, apoptosis, blood-brain barrier destruction and aggravation of capillary no-reflow (Kalogeris et al., 2016; Jiang et al., 2018; Wang et al., 2018; Wu et al., 2018; Malone et al., 2019). Mitochondrial autophagy, also referred to as mitophagy, is a type of autophagy that continually monitors the quality of mitochondria and eliminates damaged mitochondria through lysosomal targeting via various pathways (Ashrafi and Schwarz, 2013). The role of mitophagy in cerebral I/R remains controversial. Inadequate removal of damaged mitochondria or excessive degradation of essential mitochondria both lead to cell death (De et al., 2021). The prevailing opinion is that the activation of autophagy mitigates cerebral I/R injury through multiple pathways, such as reducing damage to neurons, glia, and endothelial cells (Papadakis et al., 2013). Additionally, mitophagy provides neuroprotection by inhibiting inflammasome activation (Zhou et al., 2011; Zhong et al., 2016a).
Chinese herbal medicine (CHM) has been used for centuries to treat ischemic stroke, with proven clinical efficacy (Li et al., 2022). Animal and cellular experiments have further demonstrated that CHM monomers and decoctions can improve ischemic stroke symptoms and provide neuroprotection by attenuating cerebral I/R injury (Ding et al., 2019; Jiao-Yan et al., 2021; Tang et al., 2021; Ni et al., 2022; Liao et al., 2023). These studies have found that many Chinese medicines, including monomers and decoctions, can attenuate cerebral I/R injury by modulating mitophagy (Zhang et al., 2020a; Han et al., 2022a; Ji et al., 2022a; Huang et al., 2023a). Interestingly, some of these medicines work by inhibiting mitophagy, while others work by activating it. The ameliorative effects of these herbs on ischemic stroke are consistent, regardless of whether they inhibit or activate mitophagy. Furthermore, some of these herbs are clinically used together in one herbal formula to treat ischemic stroke (Ji et al., 2022a). There is limited literature on this interesting phenomenon, and the exact reasons for it are unclear. However, it demonstrates the potential and advantages of herbal medicine in treating ischemic stroke by mediating mitophagy.
The current study briefly introduced the physiological functions of mitochondria and the molecular mechanism of mitophagy, further explaining the complex pathological mechanism of mitophagy involved in cerebral I/R. We summarised the existing evidence on Chinese herbal medicine targeting mitochondrial autophagy to improve cerebral ischemic reperfusion. We also discussed the potential therapeutic value of Chinese herbal medicine for cerebral ischemic stroke. This may provide new directions for future research.
2 Mitochondria and mitophagy
2.1 Mitochondrial dynamics and mitochondrial quality control
Mitochondria are crucial organelles for energy metabolism in the human body. They play a vital role in ATP production, phospholipid biosynthesis and transport, calcium signaling and iron homeostasis (Friedman and Nunnari, 2014). Mitochondrial dysfunction can disrupt cell homeostasis, which is the pathological basis of many diseases, including cardiovascular diseases, neurodegenerative diseases and cancers. Mitochondria maintain their quality control and dynamic balance through continuous fission, fusion, motility and autophagy, which are regulated by multiple mechanisms.
Mitochondrial fission is primarily mediated by dynamin-related protein 1 (Drp1). Drp1 is a large GTPase, and also belongs to the mitochondrial dynamin family. Drp1 is located in the cytoplasm and can be recruited to the outer mitochondrial membrane to interact with the four fission proteins involved in this mechanism (Westermann, 2010): mitochondrial fission factor (Mff) (Otera et al., 2010), mitochondrial dynamics protein of 49 kDa (Mid49) (Palmer et al., 2011), Mid51 (Palmer et al., 2011) and fission1 (Fis1) (Zhang and Hu, 2008). The combination of Drp1 and fission proteins constricts mitochondria, resulting in the division of mitochondria into separate entities (Figure 1A). An increasing number of studies suggest that post-translational modifications (PTMs) of Drp1 and fission proteins, such as phosphorylation, dephosphorylation, ubiquitination, and polymerization, are crucial in regulating mitochondrial fission (Yamada et al., 2018; Yu et al., 2020; Kleele et al., 2021; Yu et al., 2021). Mitochondria that have undergone mitochondrial fission become smaller and more susceptible to mitophagy.
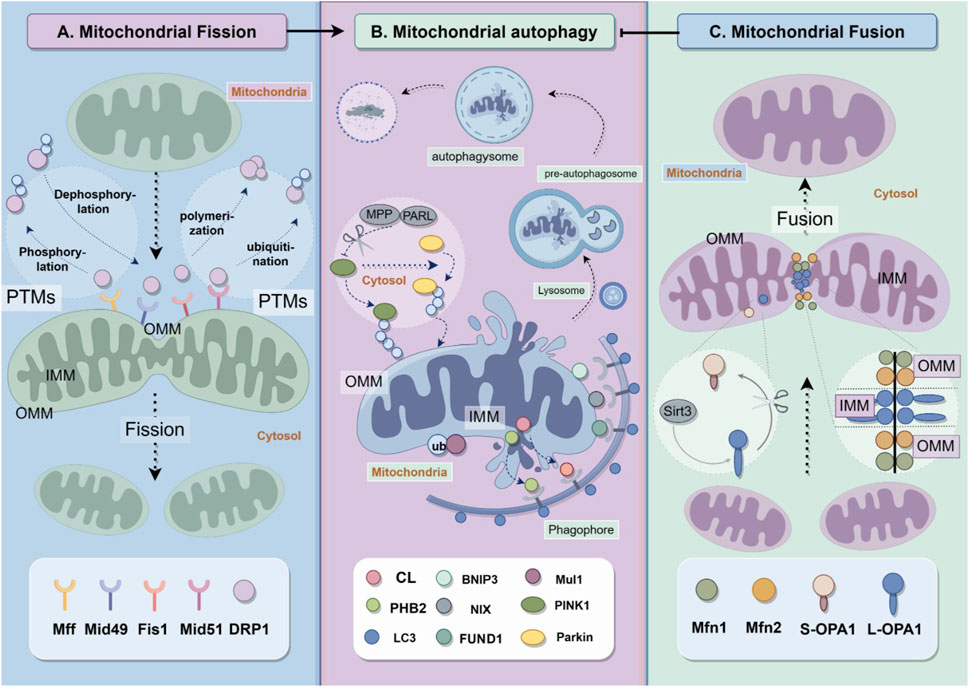
Figure 1. Mitochondrial quality control includes fission, fusion and autophagy. (A) Mitochondrial fission depends on the recruitment of Drp1 from cytosol to mitochondria to react with Drp1 receptor proteins Mff, Mid49, Fis1 and Mid51. (B) Mitochondrial autphagy requires various pathways, including PINK1/Parkin, Mul1/Mfn2 pathways and receptor dependent pathways. The proteins on outer mitochondrial membranes and inner mitochondrial membranes play vital role in the formation of autophagysomes. (C) mitochondrial fusion is regulated by dynamin-related proteins including Mfn1, Mfn2 and OPA1.
Mitochondrial fusion is also primarily regulated by dynamin-related proteins, as shown in Figure 1C. Mitochondria are organelles with double membranes, consisting of outer and inner membranes. Mitofusin1 (Mfn1) and mitofusin2 (Mfn2) are proteins located in the outer mitochondrial membrane (OMM) that mediate OMM fusion, while Optic atrophy 1 (OPA1) is a protein located in the inner mitochondrial membrane (IMM) that mediates IMM fusion. Downregulation of mitochondrial fusion improves mitophagy (Archer, 2013).
Mitochondrial motility is linked to mitochondrial dynamics. Mitochondria are in constant motion along cytoskeletal tracks that contain motility proteins, dynein and kinesin. The OMM protein Miro combined motility proteins through milton. Research has shown that the phosphorylation and degradation of Miro can arrest mitochondrial motility (Wang et al., 2011).
Both mitochondrial fission and fusion are important quality control mechanisms that regulate mitophagy and ensure mitochondrial function. Fission separates depolarized mitochondria from the network, and a coordinated downregulation of fusion mediators prevents the re-integration of these mitochondria, which then triggers mitophagy. Additionally, the motility of mitochondria is also involved in mitophagy.
Mitophagy is a type of autophagy that selectively eliminates mitochondria. Cellular autophagy, also known as type II programmed cell death, refers to the self-digestive process in which cells use lysosomes to degrade their own damaged, denatured, or aged macromolecules and organelles under the influence of external environmental factors (Dikic, 2017). Autophagy is initiated in response to stimuli such as hypoxia, starvation, and calcium overload. This process involves the formation of a double-membrane cup-like dividing membrane around the material to be degraded, which then forms a pre-autophagosome. The divided membrane gradually extends until the degraded material is completely enclosed, forming the autophagosome. Autophagosomes merge with lysosomes to form autophagic lysosomes, which are eventually fully degraded and absorbed (Palikaras et al., 2018) (Figure 1B).
2.2 Signaling pathways
2.2.1 Receptor-independent mitophagy
2.2.1.1 PINK1/parkin
Since the ubiquitin-proteasome system plays an important role in mitophagy by degrading mitochondrial outer membrane proteins, the synergistic interaction between the Ser/Thr kinase PINK1 and the E3 ubiquitin ligase Parkin is one of the most important signaling pathways for mitophagy and has been the most studied (Wen et al., 2021). In normal cells, PINK1 remains inactive in the cytosol due to the coordination between the mitochondrial processing peptidase (MPP) and the rhomboid-like serine protease PARL (Deas et al., 2011; Greene et al., 2012). Mitochondria dysfunctions, such as the dissipation of mitochondrial membrane potential (MMP), inhibit the MPP/PARL-mediated process and promote the homodimerization and autophosphorylation of PINK1. This, in turn, activate PINK1 and facilitates the translocation of Parkin to mitochondria (Okatsu et al., 2012; Aerts et al., 2015). PINK1 phosphorylates Parkin S65 in the UBL domain and also phosphorylates mitochondrial ubiquitin chains to promote Parkin activation for mitophagy both directly and indirectly (Koyano et al., 2014). The ubiquitination and phosphorylation of multiple OMM proteins lead to a feed-forward signaling for mitophagy adapter proteins which link the polyubiquitin signal with LC-3, resulting in the formation of autophagosomes for mitochondria.
Recent studies have shown that deubiquitinases, such as USP8, USP15 and USP30, play a crucial role in PINK1/Parkin-mediated mitophagy. While USP8 promotes mitophagy (Durcan et al., 2014), USP15 and USP30 remove the ubiquitin chains from mitochondrial proteins, thereby inhibiting mitophagy (Bingol et al., 2014; Cornelissen et al., 2014).
2.2.1.2 Mul1/Mfn2
Mitochondrial ubiquitin ligase 1 (Mul1) is a protein located in mitochondrial membrane that triggers trigger mitophagy by promoting the ubiquitination and degradation of the mitochondrial fusion protein mitifusion1 (Mfn2). In skeletal muscles and myoblast cultures, upregulation of Mul1 promoted mitophagy by inhibiting Mfn2 (Lokireddy et al., 2012). Mul1 deficiency increased Mfn2 activity and suppressed neuronal mitophagy (Puri et al., 2020). The Mul/Mfn2 pathway that regulates mitophagy is thought to be Parkin-independent.
2.2.2 Receptor-dependent mitophagy
Several mitophagy receptor proteins, such as FUNDC1, BNIP3, NIX and CL, are located on the mitochondrial membrane. These proteins, including inner and outer membrane receptor proteins can directly interact with LC-3B and promote mitochondrial autophagy by recruiting autophagosomes to damaged mitochondria (Lampert et al., 2019).
2.2.2.1 Outer membrane protein receptors
BCL2 and adenovirus E1B 19-kDa-interacting protein3 (BNIP3) and BNIP3-like (BNIP3L), also known as NIX, are integral outer mitochondrial membrane proteins. During hypoxia, BNIP3 is upregulated, homodimerizes and binds to LC3 to induce mitophagy. BNIP3L shares 53%–56% amino acid sequence homology with BNIP3 (Zhang and Ney, 2009). Under hypoxic conditions, BNIP3 and NIX are activated at the mRNA level in an HIF-1α-dependent manner. BNIP3 binds and inhibits the activity of Rheb, a mammalian Sirolimus target protein activator critical for mTORC1 activation, which negatively regulates bulk autophagy and mitophagy. Therefore, BNIP3 can activate mitophagy (Novak et al., 2010). FUNDC1 is also an integral OMM protein that functions as a receptor for mitophagy through its dephosphorylation (Liu et al., 2012). Dephosphorylated FUNDC1 has greater binding affinity for LC3 than NIX. Bcl-2-like protein 13 (BCL2L13) is a single-pass membrane protein anchored to the outer mitochondrial membrane. Its function is to regulate mitochondrial morphology and interacts with ULK1 to localize the mitophagy initiation complex to mitochondria (Murakawa et al., 2015; Murakawa et al., 2019).
2.2.2.2 Inner membrane protein receptors
Cardiolipin (CL) is a phospholipid dimer synthesized in the inner mitochondrial membrane (IMM). It externalises to the mitochondrial outer surface to interact with mitophagy proteins and initiate mitophagy in response to mitochondrial injury and depolarisation (Chu et al., 2013).
PHB2 is an IMM mitophagy receptor. Proteasomal-dependent OMM rupture is necessary for PHB2 before it binds to LC3 II. The LIR Domain of PHB2 is required for Parkin-mediated mitophagy (Wei et al., 2017b). The mechanisms of mitophagy is shown in Figure 1B.
3 The role of mitophagy in the pathological mechanism of cerebral I/R injury
3.1 Ischemia and hypoxia lead to mitochondrial dysfunction and induce mitophagy
The brain requires a significant amount of ATP to maintain its function in the human body. Mitochondria are the primary source of ATP production, resulting in higher mitochondrial content in brain tissue than in other tissues (Tang et al., 2016). However, since the energy storage in brain tissue is lower compared to other organs in the body, even a transient ischemia-hypoxia state can cause severe and potentially irreversible damage to brain tissue cells (Tierradentro-García et al., 2023). When brain tissue cells transition from normoxia to ischemia-hypoxia due to arterial blood flow obstruction, cellular anaerobic metabolism is induced. This impairs mitochondrial ATP synthesis, causes dysfunction in the mitochondrial electron transport chain, and significantly dissipates the mitochondrial membrane potential. These changes affect the operation of cellular ion exchange channels. As a result, Ca2+, sodium and hydrogen ions accumulate in the cell, leading to hyperosmolarity and causing the cell to swell to a certain degree. Additionally, cytoplasmic enzyme activity is impaired (Hofmeijer and van Putten, 2012; Dharmasaroja, 2016). The mitochondria must take up a large amount of Ca2+, which causes them to swell and become impaired. To maintain cellular homeostasis, the cell must engulf the damaged mitochondria (Lampert et al., 2019). Furthermore, during ischemic hypoxia, intracellular antioxidant levels decrease, leading to an overproduction of reactive oxygen species (ROS) and promoting oxidative stress (Yingze et al., 2022). mitophagy is induced as a means to reduce ROS levels (Shadel and Horvath, 2015). In summary, ischemia and hypoxia result in mitochondrial dysfunction and induce mitophagy through various mechanisms.
3.2 Mitophagy has dual roles in cerebral I/R injury
Mitophagy plays a dual role in cerebral ischemia reperfusion injury, and the mechanism remains complex and controversial.
During the ischemic phase, pathological factors induce mitochondrial dysfunction. The mitochondrial damage is a significant contributor to the production of oxidative stress and can lead to cell death (Granger and Kvietys, 2015). Restoring the blood flow during the reperfusion stage brings fresh oxygen but also leads to a high production of ROS. Mitophagy is a form of selective autophagy that eliminates damaged mitochondria, attenuating cerebral I/R injury (Baek et al., 2014a). Appropriate mitophagy promotes the restoration of intracellular homeostasis to a certain extent, thus providing neuroprotection. Promoting mitophagy during the rapid phase of reperfusion favours neuronal survival (Zhang, 2013; Liu et al., 2014; Yuan et al., 2017). It has been found that activation of PINK1/Parkin-dependent mitophagy ameliorates neuronal damage in the cortex and hippocampal CA1 region after cerebral ischemia and removes damaged mitochondria (Wang and Zhou, 2020; Wu et al., 2021). The neuroprotective effect of tPA on CIRI can be achieved by promoting FUNDC1-dependent mitophagy, which improves mitochondrial function and inhibits apoptosis (Cai et al., 2021). It has been found that promotion of mitochondrial autophagy through PINK/PARKIN-mediated ubiquitination of PA2G4/EBP1 contributes to neuroprotection after CIRI (Hwang et al., 2024).
However, if the stress caused by reperfusion is too high and autophagy fails to relieve it, then cellular damage will still occur (Qin et al., 2016). Excessive mitophagy can also induce cell death and aggravate brain injury. The reperfusion of ischemic cerebral tissues changes dynamically. Recent studies have shown that mitophagy may have varying effects on neurons in response to ischemia and during different pathological stages of reperfusion. In the early stage of CIRI, mitophagy can engulf a large number of functionally impaired mitochondria, recycle the useful substances in them and synthesize new mitochondria. The activation of mitophagy facilitates the restoration of the balance of energy metabolism in the brain. In the middle and late stages of CIRI, excessive mitophagy will lead to a shortage of mitochondria, which is not conducive to the recovery of brain injury (Baek et al., 2014b; Zhong et al., 2016b; Sulkshane et al., 2021). However, the reasons for this change have not been clearly investigated.
4 Regulation for management of cerebral I/R injury
CHM are one of the common therapies used clinically for the treatment of ischemic stroke in China. Natural compounds have also gained the attention of many researchers in recent years. By summarising some current in vitro and in vivo studies of CHM, we explored the mechanism by which these herbs act on mitophagy and thus alleviate CIRI. Moreover, CHM could regulate some important pathological aspects of CIRI, such as calcium overload, inflammation, apoptosis and oxidative stress through mitophagy. These interesting phenomena may bring some inspiration for subsequent studies.
4.1 Monomers/decoctions promoting mitophagy for cerebral I/R injury
Ligustilide (LIG) is a natural monomer isolated from Ligusticum chuanxiong hort and Angelica sinensis (Oliv.) diels. Both of them have the effect of activating blood circulation and removing blood stasis in Chinese medicine. Recent studies have shown that LIG protects hippocampal neurons from CIRI both in vivo and in vitro (Gan et al., 2020; Sun et al., 2021). The research found that LIG reduced hippocampal neuron injury in both middle cerebral artery occlusion and reperfusion (MCAO/R) Sprague-Dawley (SD) rat models and oxygen-glucose deprivation and reoxygenation (OGD/R) HT-22 cell models. This was achieved by significantly elevating the protein expressions of PINK and Parkin (Mao et al., 2022), which enhance mitophagy. The neuroprotective effects of LIG were counteracted by midivi-1, a mitochondrial-autophagy inhibitor. Furthermore, the silencing of PINK1 partially impeded the effect of LIG on mitophagy. Therefore, it is possible that LIG can mitigate the ischemia/reperfusion injury by activating mitophagy through the PINK1/Parkin pathway.
Panax notoginseng Saponins (PNS) is the main active ingredient extracted from the rhizome of P. notoginseng (Burkill), a traditional Chinese medicine. P. notoginseng is widely used clinically to treat cardiovascular and cerebrovascular diseases (Tong et al., 2019). Mitophagy inhibits the activation of the NLRP3 inflammasome in cerebral ischemia (Shao et al., 2018; Mai et al., 2019; Ren et al., 2019; Xu et al., 2019). The inflammasome is central to the inflammatory response and mediates many neurodegenerative diseases (Prabhakaran et al., 2015; Mangan et al., 2018). PNS restrained NLRP3 inflammasome activation and activated mitochondrial autophagy via the PINK1/Parkin pathway in cerebral I/R injury rat brains (Xiao et al., 2022). Xuesaitong (XST), a Chinese medicinal preparation containing PNS, is widely used in the treatment of ischemic stroke. A study showed that it combined with dexmedetomidine I attenuated I/R injury by activating Keap1/Nrf2 signaling and mitophagy (Han et al., 2022b).
Jionoside A1 is a native compound derived from Rehmannia glutinosa (Di-huang), a Chinese medicine that is commonly used to treat brain diseases, including stroke. Rehmannia glutinosa has been found to be neuroprotective against acute cerebral ischemia and to improve cognitive dysfunction (Fu et al., 2022; Wang et al., 2022). Although the study did not show significant alterations in Parkin and FUNDC1 expression, it did reveal that Jionoside A1 significantly enhanced mitochondrial content and promoted mitophagy in OGD/R and MCAO/R models by upregulating the expression of NIX protein in ischemic stroke I/R injury (Fu et al., 2022). Furthermore, Jionoside A1 was found to increase the cellular ATP levels and decrease the release of LDH, which is an indicator of neuronal cytotoxicity in the OGD/R model. Additionally, the knockdown of NIX inhibited the aforementioned effects of Jionoside A1 (Yu et al., 2023).
Baicalein 7-O-β-D-glucuronide (Baicalin), a natural flavonoid isolated from Scutellaria baicalensis Georgi (Huang-qin or Chinese skullcap), has been shown to provide neuron protection from cerebral ischemia by inhibiting inflammation, reducing apoptosis and regulating mitochondrial functions (Zhang et al., 2017; Hao et al., 2023). In the MCAO/R model, Baicalin decreased infarction volume and attenuated neurobehaviors (Li et al., 2017). Baicalin treatment inhibited the expression of Drp1 and promoted the synthesis of Mfn2, regulating mitochondrial fission and fusion. Additionally, it suppressed the production of ROS and elevated MMP in OGD/R PC12 cells through the activation of protein kinase by adenosine monophosphate (AMPK), resulting in suppressing cell apoptosis and enhancement of mitophagy (Li et al., 2017).
Resveratrol (Res) is a natural extract from Rhizoma Polygoni Cuspidati (Hu-zhang), a traditional Chinese medicine. It has an overall neuroprotective role in ischemic stroke (Hou et al., 2018; Ashafaq et al., 2021). Res activates autophagy by increasing the level of phosphorylated AMPK in cerebral ischemia rats and attenuates the mitochondrial failure in neuronal cultures (Pineda-Ramírez et al., 2020). According to a study, Res controlled the PINK1/Parkin-mediated mitophagy in OGD/R-injured neuron cells, increasing cell viability and suppressing apoptosis (Ye et al., 2021). The neuroprotective benefits of Res treatment were diminished due to the inhibition of mitophagy caused by the downregulation of PINK1 or Parkin.
Taohong Siwu Decoction (THSWD) is a compounded Chinese medicinal preparation used to treat blood stasis diseases, including stroke. The formula comprises six herbs: Tao-Ren [(Prunus persica (L.) Batsch], Dang-Gui [A. sinensis (Oliv.) Diels], Shu-Di-Huang [R. glutinosa (Gaertn.) DC.], Hong-Hua [Carthamus tinctorius L.], Chuan-Xiong [Conioselinum anthriscoides] and Bai-Shao [Paeonia lactiflora Pall]. THSWD has been found to alleviate cerebral I/R injury by suppressing cell pyroptosis (Wang et al., 2020), necrosis and neuroinflammation (Wang et al., 2021). It increased the expression of autophagy-related proteins (LC3 and Beclin1) and mitophagy marker proteins (PINK1 and Parkin), and reduced reactive oxygen species (ROS), the NLRP3 inflammasome and pro-inflammatory cytokines in OGD/R-induced P12 cells (Ji et al., 2022b; Shi et al., 2023). Furthermore, the formula also significantly reduced neurological deficit scores and cerebral infarct volume in MCAO/R rats (Shi et al., 2023). The effects of THSWD above were blocked with the use of Mdivi-1 both in vivo and in vitro.
Dengzhan Xixin Injection (DX) is a widely prescribed Chinese medicine injection derived mainly from Erigeron breviscapus (Vaniot) Hand.-Mazz. (National Pharmacopoeia Commission, 2020). It has been widely prescribed for years to treat cerebral ischemic stroke (Hu et al., 2020). The six components of DX, including scutellarin, 3,5-OdiCQA, 4,5-O-diCQA, 3,4-O-diCQA, caffeic acid and 5-O-CQA were determined using high performance liquid chromatography (HPLC). Scutellarin was the major representative compound with the highest concentration. DX administration attenuated cerebral infarction volumes and neuronal loss in rats and alleviated cerebral I/R injury by activating mitophagy. This was achieved via stimulation the protein expression level of LC3, PINK1 and Parkin (Yang et al., 2022). DX reduced ROS and MDA levels and increased SOD levels. DX intervention reversed the imbalance of ATP and MMP and maintained mitochondrial ultra structure by increasing the number of autophagic lysosomes. Interestingly, DX also decreased the expressions pf apoptosis-related proteins including Bax, Cyto-c and cleaved Caspase-3 and increased Bcl-2 level (Yang et al., 2022).
The table below summarises the studies on the promotion of mitophagy in cerebral I/R injury by traditional Chinese medicine (Table 1).
Figure 2 generally demonstrates the improvement of CHM for cerebral ischemia reperfusion injury by inhibiting or promoting mitophagy through different signaling pathways.
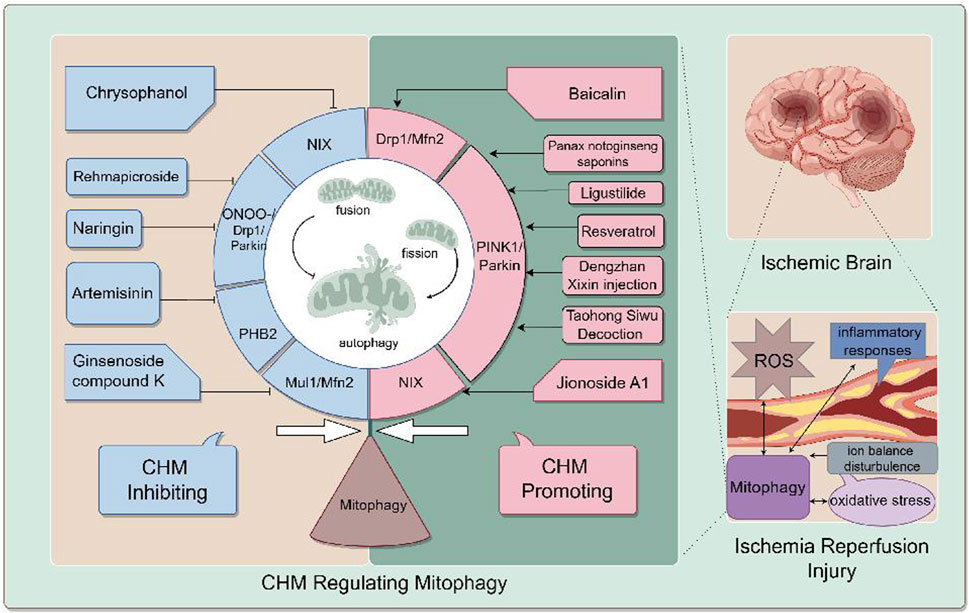
Figure 2. the mechanism of CIRI is complicated, including oxidative stress, ion balance disturbance, inflammation responses, blood-brain barrier destruction and mitophagy. CHM promoting mitophagy for cerebral IR injury include Ligustilide (LIG), Panax notoginseng saponins (PNS), Jionoside A1, Baicalin, Resveratrol (Res), Taohong Siwu Decoction (THSWD) and Dengzhan Xixin injection. CHM inhibiting mitophagy for cerebral IR injury include Ginsenoside compound K (CK), Rehmapicroside (Reh), Naringin, Artemisinin (ART), Chrysophanol (Chry), Xiao-Xu-Ming Decoction (XXMD). CHM regulates mitophagy to alleviate cerebral I/R inury through various pathways. For example, Ligustilide promotes mitophagy through the PINK1/Parkin pathway.
4.2 Monomers/decoctions inhibiting mitophagy for cerebral I/R injury
Panax ginseng is a Chinese herbal medicine that has been used as a tonic medicine for centuries in China. Ginsenoside compound K(CK), an important saponin-like component of ginseng, augmented mitochondrial fusion and attenuated mitophagy in cerebral IR injury through the Mul1/Mfn2 pathway (Huang et al., 2023b). The study found that Ginsenoside CK inhibited mitochondrial dynamics imbalance and damage by reducing the binding affinity of Mul1 and Mfn2, which in turn inhibited the ubiquitination of Mfn2 by Mul1. Additionally, in OGD/R-induced PC12 cells, Ginsenoside CK was found to rescue mitochondrial dysfunction by promoting mitochondrial oxygen consumption. In the cerebral I/R injury rat model, the infarct volume of the pretreatment group was significantly smaller than that of control group. Ginsenoside CK reduced mitochondrial fission and mitophagy, counteracting I/R-induced neurological impairment and mitochondrial dysfunction. The mitigation effect of ginsenoside CK on mitophagy was markedly abrogated by Mfn2 knockdown (Huang et al., 2023b).
Radix Rehmanniae, a medicinal plant, is commonly used in Chinese medicine formulas for cerebral diseases (Zhou et al., 2016; Fei et al., 2018). Rehmapicroside (Reh), a natural compound found in Radix Rehmanniae, has neuroprotective effects against cerebral IR injury by inhibiting peroxynitrite (ONOO−)-mediated mitophagy activation (Zhang et al., 2020b). During cerebral ischemia reperfusion injury, a large amount of NO and superoxide anion (O2−) contemporaneously form ONOO−. ONOO− is a cytotoxic factor that exacerbate neuronal damage by causing both oxidative and nitrosative stress, leading to cerebral impairments in I/R (Chen X. M. et al., 2013; Lourenco et al., 2017). It promotes DRP1 nitration and the recruitment of DRP one to impaired mitochondria, causing over-activation of mitophagy (Feng et al., 2017; Feng et al., 2018a; Li et al., 2019). The study found that Reh reacted with ONOO−, resulting in a decrease of ONOO−. Additionally, Reh downregulated PINK1, Parkin, p62 and the ratio of LC3-II to LC3-I in the OGD/RO-treated PC12 cells. Reh attenuated infarct size and improved neurological deficit scores in the MCAO rats by preventing the translocations of PINK1, Parkin and Drp1 into mitochondria for mitophagy. Furthermore, NADPH oxidases and iNOS expression were also suppressed by Reh (Zhang et al., 2020b).
Naringin (4′,5,7-trihydroxy-flavonone-7-rhamnoglucoside) is a bioflavonoid extracted from grapefruit and related citrus species, and has also been found in many herbal medicines for various diseases (Chen Y. et al., 2013; Li et al., 2014; Sharma et al., 2015). Recently, Naringin has shown potential in protecting cerebral neurons in different animal models including traumatic brain injury (Cui et al., 2014), subarachnoid hemorrhage (Han et al., 2016) and cerebral I/R injury (Gaur et al., 2009). Naringin reduced excessive mitophagy activation in the ischemic brains during cerebral I/R injury by scavenging ONOO− (Feng et al., 2018b). It also inhibited the production of superoxide and nitric oxide in SH-SY5Y cells under OGD/RO condition. Additionally, Naringin inhibited the expression of NADPH oxidase subunits and iNOS in rat brains with cerebral I/R injury. Naringin decreased the formation of 3-nitrotyrosine formation, inhibited the translocation of Parkin to the mitochondria and reduced the ratio of LC3-II to LC3-I in the mitochondrial fraction (Feng et al., 2018b).
Artemisinin (ART) is a bioactive compound derived from the plant Artemisia annua, known for its effectiveness in treating malaria (Tu, 2016). Studies showed that ART inhibited autophagy to alleviate apoptosis and oxidative stress in MPP(+)-induced SH-SY5Y cells (Yan et al., 2021), and it possessed the ability to reverse the oxidative stress damage aggravated by OGD/R treatment in the human neuroblastoma SH-SY5Y cells (Jiang et al., 2022). The antioxidative stress effect of ART is related to PHB2-mediated autophagy prohibitin 2 (PHB2). Unlike other autophagy receptors that target outer mitochondrial membrane (Lazarou et al., 2015), PHB2 is a mitophagy receptor on mitochondrial inner membrane that binds to LC3 and leads to mitopagy (Wei et al., 2017a). ART impaired mitophagy by inhibiting PHB2, which decreased the conversions of LC3I to LC3II. Silencing PHB2 eliminated the protective effect of ART against OGD/R-induced oxidative stress damage (Jiang et al., 2022). Other studies showed that ART also reduced the inflammation by activating Nrf2 and ROS-dependent p38 MAPK against cerebral ischemia reperfusion injury (Lu et al., 2018).
Chrysophanol (Chry) is the main active ingredient isolated from the rhizome of Da-Huang (rhubarb), a traditional Chinese medicine. Chry alleviated cerebral I/R injury in mice though inhibiting neuroinflammation and nitrosative/oxidative stress (Liu et al., 2022a; Liu et al., 2022b). A study found that Chry reduced mitophagy of hippocampus through inhibiting the expression of LC3B and NIX in bilateral common carotid arteries occlusion and reperfusion (CCAO/R)-induced Kunming (KM) mice (Cui et al., 2022). The alleviation of cerebral I/R injury after Chry treatment may function in inhibiting NIX-mediated mitophagy.
Xiao-Xu-Ming Decoction (XXMD) is a classical chinese herbal medicine containing twelve herbs, including Huang-Qin (S. baicalensis Georgi), Fang-Feng (Saposhnikovia divaricata (Turcz. ex Ledeb.) Schischk.), Fang-Ji (Stephania tetrandra S. Moore), Ren-Shen (P. ginseng C.A.Mey.), Gui-Zhi (Cinnamomum cassia (L.) J. Presl), Xing-Ren (Prunus armeniaca L.), Ma- Huang (Ephedra sinica Stapf), Chuan-Xiong (C. anthriscoides “Chuanxiong”), Fu-Zi (Aconitum carmichaeli Debeaux), Shao-Yao (P. lactiflora Pall.), Gan-Cao (Glycyrrhiza uralensis Fisch. ex DC.) and Sheng-Jiang (Zingiber officinale Roscoe). XXMD has been widely used to treat stroke for thousand years in china. Studies showed its improvement of cerebral injury after ischemia and protection for neuron cells (Wu et al., 2022). Ferulic acid, zingerone, and vanillic acid in XXMD were tested to be protective for OGD/R-induced cells (Chen et al., 2022). XXMD preserved mitochondrial integrity and function via inhibiting mitophagy and reduced apoptosis via the mitochondrial p53 pathway in cerebral I/R injured rats (Lan et al., 2014; Lan et al., 2018).
The table below summarises the studies on the inhibition of mitophagy involved in cerebral I/R injury by traditional Chinese medicine (Table 2).
5 Discussion
Due to the limited therapeutic options available for treating ischemic stroke, researchers have increasingly focused on mitophagy as a potential target. The studies mentioned above have shown that regulating mitophagy can significally alleviate cerebral ischemia/reperfusion injury, providing in vitro and in vivo evidence for mitophagy as a new treatment option for ischemic stroke. mitochondrial autophagy, as well as mitochondrial fission, fusion and other processes are involved in adjusting the quantity and quality of cellular mitochondria. This further regulates the processes of cellular oxidative stress, inflammation, apoptosis, and pyroptosis, achieving a therapeutic effect and significantly alleviating neurological damage.
Currently, there are few drugs that modulate mitophagy for the treatment of cerebral ischemia/reperfusion injury. However, traditional Chinese medicine (TCM) has shown promising results as a complementary treatment for ischemic stroke in China. Furthermore, there is a substantial body of in vivo and in vitro evidence indicating that TCM can mitigate cerebral ischemia/reperfusion injury. In recent years, there has been a gradual increase in studies examining the regulation of TCM monomers and combinations.
This paper summarises in vivo and in vitro studies of Chinese herbal medicines that modulate mitophagy for the treatment of I/R mechanism. It was found that different Chinese herbal medicines can regulate mitophagy positively or negatively. When the organism has an excess of damaged mitochondria in situations such as ischemia and hypoxia, herbal medicines like LIG and PNS can activate mitophagy to maintain cellular homeostasis. On the other hand, when excessive mitophagy leads to a shortage of mitochondria in the organism, herbal medicines such as Reh and ART can inhibit excessive mitophagy and delay cell death. Therefore, both types of herbal medicines play a significant role in ameliorating I/R. In a clinical herbal combination for the treatment of ischemic stroke, different herbs have opposite effects on mitophagy. However, the overall therapeutic effect is clear and consistent, and may even be superior to that of the herbs alone.
Chinese medicine has a long history in China, and despite being around for thousands of years, it is still widely used due to its good clinical efficacy. Numerous in vivo and in vitro studies have provided evidence of the mechanisms through which CHM monomers work to treat diseases, but the complexity of TCM formulas has limited research to date. We think that TCM formulas have more research potential because the mechanisms of action between their various components are thought to be more complex than those of TCM monomers.
In addition, we discovered that traditional Chinese medicine regulates mitophagy to alleviate cerebral I/R inury through various pathways. The promotion of mitophagy is dominated by the PINK1/Parkin pathway, while the inhibition of mitophagy is achieved through mitochondrial fission and fusion proteins, ubiquitin-independent receptor proteins, and other pathways. After filtering, the number of studies included in this paper is limited. While the conclusion is not entirely rigorous, but it does offer some ideas for subsequent experimental validation based on the previous hypotheses.
This paper provides a brief description of the mechanisms of mitochondria and mitophagy and their roles in cerebral I/R injury. It summarises in vivo and in vitro evidence that different TCMs modulate mitophagy through different pathways, both positively and negatively, thereby alleviating I/R. The next step is to investigate how various active ingredients in the classic TCM formula for ischemic stroke work together to improve cerebral ischemia/reperfusion by maintaining mitochondrial homeostasis. This will provide new insights for the clinical development of drugs.
Author contributions
YC: Writing–review and editing, Writing–original draft, Investigation, Conceptualization. YZ: Writing–review and editing, Methodology. QW: Writing–review and editing, Methodology, Conceptualization. JC: Writing–review and editing. YD: Writing–review and editing, Supervision, Project administration, Methodology, Funding acquisition.
Funding
The author(s) declare financial support was received for the research, authorship, and/or publication of this article. This work was supported by grants from the Science and Technology Innovation Team Project of Hunan Province (2020RC4050), Hunan Province Traditional Chinese Medicine Research Program Project (E2022010).
Acknowledgments
Figure 1 and Figure 2 were assisted by Figdraw (https://www.figdraw.com).
Conflict of interest
The authors declare that the research was conducted in the absence of any commercial or financial relationships that could be construed as a potential conflict of interest.
Publisher’s note
All claims expressed in this article are solely those of the authors and do not necessarily represent those of their affiliated organizations, or those of the publisher, the editors and the reviewers. Any product that may be evaluated in this article, or claim that may be made by its manufacturer, is not guaranteed or endorsed by the publisher.
References
Aerts, L., Craessaerts, K., De Strooper, B., and Morais, V. A. (2015). PINK1 kinase catalytic activity is regulated by phosphorylation on serines 228 and 402. J. Biol. Chem. 290 (5), 2798–2811. doi:10.1074/jbc.M114.620906
Archer, S. L. (2013). Mitochondrial dynamics--mitochondrial fission and fusion in human diseases. N. Engl. J. Med. 369 (23), 2236–2251. doi:10.1056/NEJMra1215233
Ashafaq, M., Intakhab Alam, M., Khan, A., Islam, F., Khuwaja, G., Hussain, S., et al. (2021). Nanoparticles of resveratrol attenuates oxidative stress and inflammation after ischemic stroke in rats. Int. Immunopharmacol. 94, 107494. doi:10.1016/j.intimp.2021.107494
Ashrafi, G., and Schwarz, T. L. (2013). The pathways of mitophagy for quality control and clearance of mitochondria. Cell. Death Differ. 20 (1), 31–42. doi:10.1038/cdd.2012.81
Baek, S. H., Noh, A. R., Kim, K. A., Akram, M., Shin, Y. J., Kim, E. S., et al. (2014a). Modulation of mitochondrial function and autophagy mediates carnosine neuroprotection against ischemic brain damage. Stroke 45 (8), 2438–2443. doi:10.1161/STROKEAHA.114.005183
Baek, S. H., Noh, A. R., Kim, K. A., Akram, M., Shin, Y. J., Kim, E. S., et al. (2014b). Modulation of mitochondrial function and autophagy mediates carnosine neuroprotection against ischemic brain damage. Stroke 45 (8), 2438–2443. doi:10.1161/STROKEAHA.114.005183
Bingol, B., Tea, J. S., Phu, L., Reichelt, M., Bakalarski, C. E., Song, Q., et al. (2014). The mitochondrial deubiquitinase USP30 opposes parkin-mediated mitophagy. Nature 510 (7505), 370–375. doi:10.1038/nature13418
Cai, Y., Yang, E., Yao, X., Zhang, X., Wang, Q., Wang, Y., et al. (2021). FUNDC1-dependent mitophagy induced by tPA protects neurons against cerebral ischemia-reperfusion injury. Redox Biol. 38, 101792. doi:10.1016/j.redox.2020.101792
Chen, X. M., Chen, H. S., Xu, M. J., and Shen, J. G. (2013a). Targeting reactive nitrogen species: a promising therapeutic strategy for cerebral ischemia-reperfusion injury. Acta Pharmacol. Sin. 34 (1), 67–77. doi:10.1038/aps.2012.82
Chen, Y., Nie, Y. C., Luo, Y. L., Lin, F., Zheng, Y. F., Cheng, G. H., et al. (2013b). Protective effects of naringin against paraquat-induced acute lung injury and pulmonary fibrosis in mice. Food Chem. Toxicol. 58, 133–140. doi:10.1016/j.fct.2013.04.024
Chen, Y. P., Wang, K. X., Cai, J. Q., Li, Y., Yu, H. L., Wu, Q., et al. (2022). Detecting key functional components group and speculating the potential mechanism of xiao-xu-ming decoction in treating stroke. Front. Cell. Dev. Biol. 10, 753425. doi:10.3389/fcell.2022.753425
Chu, C. T., Ji, J., Dagda, R. K., Jiang, J. F., Tyurina, Y. Y., Kapralov, A. A., et al. (2013). Cardiolipin externalization to the outer mitochondrial membrane acts as an elimination signal for mitophagy in neuronal cells. Nat. Cell. Biol. 15 (10), 1197–1205. doi:10.1038/ncb2837
Cornelissen, T., Haddad, D., Wauters, F., Van Humbeeck, C., Mandemakers, W., Koentjoro, B., et al. (2014). The deubiquitinase USP15 antagonizes Parkin-mediated mitochondrial ubiquitination and mitophagy. Hum. Mol. Genet. 23 (19), 5227–5242. doi:10.1093/hmg/ddu244
Cui, Q. J., Wang, L. Y., Wei, Z. X., and Qu, W. S. (2014). Continual naringin treatment benefits the recovery of traumatic brain injury in rats through reducing oxidative and inflammatory alterations. Neurochem. Res. 39 (7), 1254–1262. doi:10.1007/s11064-014-1306-2
Cui, W. H., Zhang, H. H., Qu, Z. M., Wang, Z., Zhang, D. J., and Wang, S. (2022). Effects of chrysophanol on hippocampal damage and mitochondrial autophagy in mice with cerebral ischemia reperfusion. Int. J. Neurosci. 132 (6), 613–620. doi:10.1080/00207454.2020.1830085
De, R., Mazumder, S., and Bandyopadhyay, U. (2021). Mediators of mitophagy that regulate mitochondrial quality control play crucial role in diverse pathophysiology. Cell. Biol. Toxicol. 37 (3), 333–366. doi:10.1007/s10565-020-09561-1
Deas, E., Plun-Favreau, H., Gandhi, S., Desmond, H., Kjaer, S., Loh, S. H., et al. (2011). PINK1 cleavage at position A103 by the mitochondrial protease PARL. Hum. Mol. Genet. 20 (5), 867–879. doi:10.1093/hmg/ddq526
Dharmasaroja, P. A. (2016). Fluid intake related to brain edema in acute middle cerebral artery infarction. Transl. Stroke Res. 7 (1), 49–53. doi:10.1007/s12975-015-0439-1
Dikic, I. (2017). Proteasomal and autophagic degradation systems. Annu. Rev. Biochem. 86, 193–224. doi:10.1146/annurev-biochem-061516-044908
Ding, Y., Du, J., Cui, F., Chen, L., and Li, K. (2019). The protective effect of ligustrazine on rats with cerebral ischemia-reperfusion injury via activating PI3K/Akt pathway. Hum. Exp. Toxicol. 38 (10), 1168–1177. doi:10.1177/0960327119851260
Durcan, T. M., Tang, M. Y., Pérusse, J. R., Dashti, E. A., Aguileta, M. A., McLelland, G. L., et al. (2014). USP8 regulates mitophagy by removing K6-linked ubiquitin conjugates from parkin. EMBO J. 33 (21), 2473–2491. doi:10.15252/embj.201489729
Fei, X., Zhang, X., Wang, Q., Li, J., Shen, H., Wang, X., et al. (2018). Xijiao dihuang decoction alleviates ischemic brain injury in MCAO rats by regulating inflammation, neurogenesis, and angiogenesis. Evid. Based Complement. Altern. Med. 2018, 5945128. doi:10.1155/2018/5945128
Feng, J., Chen, X., Guan, B., Li, C., Qiu, J., and Shen, J. (2018a). Inhibition of peroxynitrite-induced mitophagy activation attenuates cerebral ischemia-reperfusion injury. Mol. Neurobiol. 55 (8), 6369–6386. doi:10.1007/s12035-017-0859-x
Feng, J., Chen, X., Lu, S., Li, W., Yang, D., Su, W., et al. (2018b). Naringin attenuates cerebral ischemia-reperfusion injury through inhibiting peroxynitrite-mediated mitophagy activation. Mol. Neurobiol. 55 (12), 9029–9042. doi:10.1007/s12035-018-1027-7
Feng, J. H., Chen, X. M., and Shen, J. G. (2017). Reactive nitrogen species as therapeutic targets for autophagy: implication for ischemic stroke. Expert Opin. Ther. Targets 21 (3), 305–317. doi:10.1080/14728222.2017.1281250
Feske, S. K. (2021). Ischemic stroke. Am. J. Med. 134 (12), 1457–1464. doi:10.1016/j.amjmed.2021.07.027
Friedman, J. R., and Nunnari, J. (2014). Mitochondrial form and function. Nature 505 (7483), 335–343. doi:10.1038/nature12985
Fu, C., Wu, Y., Liu, S., Luo, C., Lu, Y., Liu, M., et al. (2022). Rehmannioside A improves cognitive impairment and alleviates ferroptosis via activating PI3K/AKT/Nrf2 and SLC7A11/GPX4 signaling pathway after ischemia. J. Ethnopharmacol. 289, 115021. doi:10.1016/j.jep.2022.115021
Gan, Y. M., Liu, D. L., Chen, C., Duan, W., Yang, Y. X., and Du, J. R. (2020). Phthalide derivative CD21 alleviates cerebral ischemia-induced neuroinflammation: involvement of microglial M2 polarization via AMPK activation. Eur. J. Pharmacol. 886, 173552. doi:10.1016/j.ejphar.2020.173552
Gaur, V., Aggarwal, A., and Kumar, A. (2009). Protective effect of naringinagainst ischemic reperfusion cerebral injury: possible neurobehavioral, biochemical and cellular alterations in rat brain. Eur. J. Pharmacol. 616 (1–3), 147–154. doi:10.1016/j.ejphar.2009.06.056
Granger, D. N., and Kvietys, P. R. (2015). Reperfusion injury and reactive oxygen species: the evolution of a concept. Redox Biol. 6, 524–551. doi:10.1016/j.redox.2015.08.020
Greene, A. W., Grenier, K., Aguileta, M. A., Muise, S., Farazifard, R., Haque, M. E., et al. (2012). Mitochondrial processing peptidase regulates PINK1 processing, import and Parkin recruitment. EMBO Rep. 13 (4), 378–385. doi:10.1038/embor.2012.14
Han, G. J., Min, X. Z., Ma, S. S., Ding, C., and Wang, X. Q. (2022a). Xuesaitong combined with dexmedetomidine improves cerebral ischemia-reperfusion injury in rats by activating keap1/nrf2 signaling and mitophagy in hippocampal tissue. Oxid. Med. Cell. Longev. 7, 5126042. doi:10.1155/2022/5126042
Han, G. J., Min, X. Z., Ma, S. S., Ding, C., and Wang, X. Q. (2022b). Xuesaitong combined with dexmedetomidine improves cerebral ischemia-reperfusion injury in rats by activating keap1/nrf2 signaling and mitophagy in hippocampal tissue. Oxid. Med. Cell. Longev. 7, 5126042. doi:10.1155/2022/5126042
Han, Y., Su, J., Liu, X., Zhao, Y., Wang, C., and Li, X. (2016). Naringin alleviates early brain injury after experimental subarachnoid hemorrhage by reducing oxidative stress and inhibiting apoptosis. Brain Res. Bull. 133, 42–50. doi:10.1016/j.brainresbull.2016.12.008
Hao, Z., Zhang, Z., Zhao, Y., and Wang, D. (2023). Baicalin reduces immune cell infiltration by inhibiting inflammation and protecting tight junctions in ischemic stroke injury. Am. J. Chin. Med. 51 (2), 355–372. doi:10.1142/S0192415X23500180
Herpich, F., and Rincon, F. (2020). Management of acute ischemic stroke. Crit. Care Med. 48 (11), 1654–1663. doi:10.1097/CCM.0000000000004597
Hofmeijer, J., and van Putten, M. J. (2012). Ischemic cerebral damage: an appraisal of synaptic failure. Stroke 43 (2), 607–615. doi:10.1161/STROKEAHA.111.632943
Hou, Y., Wang, K., Wan, W., Cheng, Y., Pu, X., and Ye, X. (2018). Resveratrol provides neuroprotection by regulating the JAK2/STAT3/PI3K/AKT/mTOR pathway after stroke in rats. Genes. Dis. 5 (3), 245–255. doi:10.1016/j.gendis.2018.06.001
Hu, M. Z., Zhou, Z. Y., Zhou, Z. Y., Lu, H., Gao, M., Liu, L. M., et al. (2020). Effect and safety of hydroxysafflor yellow A for injection in patients with acute ischemic stroke of blood stasis syndrome: a phase II, multicenter, randomized, double-blind, multiple-dose, active-controlled clinical trial. Chin. J. Integr. Med. 26 (6), 420–427. doi:10.1007/s11655-020-3094-7
Huang, Q., Li, J., Chen, J., Zhang, Z., Xu, P., Qi, H., et al. (2023a). Ginsenoside compound K protects against cerebral ischemia/reperfusion injury via Mul1/Mfn2-mediated mitochondrial dynamics and bioenergy. J. Ginseng Res. 47 (3), 408–419. doi:10.1016/j.jgr.2022.10.004
Huang, Q., Li, J., Chen, J., Zhang, Z., Xu, P., Qi, H., et al. (2023b). Ginsenoside compound K protects against cerebral ischemia/reperfusion injury via Mul1/Mfn2-mediated mitochondrial dynamics and bioenergy. J. Ginseng Res. 47 (3), 408–419. doi:10.1016/j.jgr.2022.10.004
Hwang, I., Kim, B. S., Lee, H. Y., Cho, S. W., Lee, S. E., and Ahn, J. Y. (2024). PA2G4/EBP1 ubiquitination by PRKN/PARKIN promotes mitophagy protecting neuron death in cerebral ischemia. Autophagy 20 (2), 365–379. doi:10.1080/15548627.2023.2259215
Ji, Z. J., Shi, Y., Li, X., Hou, R., Yang, Y., Liu, Z. Q., et al. (2022a). Neuroprotective effect of Taohong Siwu decoction on cerebral ischemia/reperfusion injury via mitophagy-NLRP3 inflammasome pathway. Front. Pharmacol. 13, 910217. doi:10.3389/fphar.2022.910217
Ji, Z. J., Shi, Y., Li, X., Hou, R., Yang, Y., Liu, Z. Q., et al. (2022b). Neuroprotective effect of Taohong Siwu decoction on cerebral ischemia/reperfusion injury via mitophagy-NLRP3 inflammasome pathway. Front. Pharmacol. 13, 910217. doi:10.3389/fphar.2022.910217
Jiang, M., Lai, X., Zhang, Y., Shen, M., Ma, H., Liu, A., et al. (2022). Artemisinin alleviates cerebral ischemia/reperfusion-induced oxidative damage via regulating PHB2-mediated autophagy in the human neuroblastoma SH-SY5Y cell line. Oxid. Med. Cell. Longev. 15, 6568748. doi:10.1155/2022/6568748
Jiang, X., Andjelkovic, A. V., Zhu, L., Yang, T., Bennett, M. V. L., Chen, J., et al. (2018). Blood-brain barrier dysfunction and recovery after ischemic stroke. Prog. Neurobiol. 163-164, 144–171. doi:10.1016/j.pneurobio.2017.10.001
Jiao-Yan, Y., Qing-Qing, L., Xi, L., Mei, Z., Ting, S., Na, H., et al. (2021). Oxymatrine improves blood-brain barrier integrity after cerebral ischemia-reperfusion injury by downregulating CAV1 and MMP9 expression. Phytomedicine 84, 153505. doi:10.1016/j.phymed.2021.153505
Kalogeris, T., Baines, C. P., Krenz, M., and Korthuis, R. J. (2016). Ischemia/reperfusion. Compr. Physiol. 7 (1), 113–170. doi:10.1002/cphy.c160006
Kim, J., Thayabaranathan, T., Donnan, G. A., Howard, G., Howard, V. J., Rothwell, P. M., et al. (2020). Global stroke statistics 2019. Int. J. Stroke 15 (8), 819–838. doi:10.1177/1747493020909545
Kleele, T., Rey, T., Winter, J., Zaganelli, S., Mahecic, D., Perreten Lambert, H., et al. (2021). Distinct fission signatures predict mitochondrial degradation or biogenesis. Nature 593 (7859), 435–439. doi:10.1038/s41586-021-03510-6
Koyano, F., Okatsu, K., Kosako, H., Tamura, Y., Go, E., Kimura, M., et al. (2014). Ubiquitin is phosphorylated by PINK1 to activate parkin. Nature 510 (7503), 162–166. doi:10.1038/nature13392
Lampert, M. A., Orogo, A. M., Najor, R. H., Hammerling, B. C., Leon, L. J., Wang, B. J., et al. (2019). BNIP3L/NIX and FUNDC1-mediated mitophagy is required for mitochondrial network remodeling during cardiac progenitor cell differentiation. Autophagy 15 (7), 1182–1198. doi:10.1080/15548627.2019.1580095
Lan, R., Zhang, Y., Wu, T., Ma, Y. Z., Wang, B. Q., Zheng, H. Z., et al. (2018). Xiao-xu-ming decoction reduced mitophagy activation and improved mitochondrial function in cerebral ischemia and reperfusion injury. Behav. Neurol. 19, 4147502. doi:10.1155/2018/4147502
Lan, R., Zhang, Y., Xiang, J., Zhang, W., Wang, G. H., Li, W. W., et al. (2014). Xiao-Xu-Ming decoction preserves mitochondrial integrity and reduces apoptosis after focal cerebral ischemia and reperfusion via the mitochondrial p53 pathway. J. Ethnopharmacol. 151 (1), 307–316. doi:10.1016/j.jep.2013.10.042
Lazarou, M., Sliter, D. A., Kane, L. A., Sarraf, S. A., Wang, C., Burman, J. L., et al. (2015). The ubiquitin kinase PINK1 recruits autophagy receptors to induce mitophagy. Nature 524 (7565), 309–314. doi:10.1038/nature14893
Li, P., Wang, S., Guan, X., Cen, X., Hu, C., Peng, W., et al. (2014). Six months chronic toxicological evaluation of naringin in Sprague-Dawley rats. Food Chem. Toxicol. 66, 65–75. doi:10.1016/j.fct.2014.01.023
Li, S., Sun, X., Xu, L., Sun, R., Ma, Z., Deng, X., et al. (2017). Baicalin attenuates in vivo and in vitro hyperglycemia-exacerbated ischemia/reperfusion injury by regulating mitochondrial function in a manner dependent on AMPK. Eur. J. Pharmacol. 815, 118–126. doi:10.1016/j.ejphar.2017.07.041
Li, W. T., Feng, J. H., Gao, C., Wu, M. L., Du, Q. H., Tsoi, B., et al. (2019). Nitration of Drp1 provokes mitophagy activation mediating neuronal injury in experimental autoimmune encephalomyelitis. Free Radic. Biol. Med. 143, 70–83. doi:10.1016/j.freeradbiomed.2019.07.037
Li, X. H., Yin, F. T., Zhou, X. H., Zhang, A. H., Sun, H., Yan, G. L., et al. (2022). The signaling pathways and targets of natural compounds from traditional Chinese medicine in treating ischemic stroke. Molecules 27 (10), 3099. doi:10.3390/molecules27103099
Liao, J., Wei, M., Wang, J., Zeng, J., Liu, D., Du, Q., et al. (2023). Naotaifang formula attenuates OGD/R-induced inflammation and ferroptosis by regulating microglial M1/M2 polarization through BMP6/SMADs signaling pathway. Biomed. Pharmacother. 167, 115465. doi:10.1016/j.biopha.2023.115465
Liu, L., Feng, D., Chen, G., Chen, M., Zheng, Q., Song, P., et al. (2012). Mitochondrial outer-membrane protein FUNDC1 mediates hypoxia-induced mitophagy in mammalian cells. Nat. Cell. Biol. 14 (2), 177–185. doi:10.1038/ncb2422
Liu, L., Sakakibara, K., Chen, Q., and Okamoto, K. (2014). Receptor-mediated mitophagy in yeast and mammalian systems. Cell. Res. 24, 787–795. doi:10.1038/cr.2014.75
Liu, X., Zhang, X., Chen, J., Song, D., Zhang, C., Chen, R., et al. (2022a). Chrysophanol facilitates long-term neurological recovery through limiting microglia-mediated neuroinflammation after ischemic stroke in mice. Int. Immunopharmacol. 112, 109220. doi:10.1016/j.intimp.2022.109220
Liu, X., Zhang, X., Chen, J., Song, D., Zhang, C., Chen, R., et al. (2022b). Chrysophanol facilitates long-term neurological recovery through limiting microglia-mediated neuroinflammation after ischemic stroke in mice. Int. Immunopharmacol. 112, 109220. doi:10.1016/j.intimp.2022.109220
Lokireddy, S., Wijesoma, I. W., Teng, S., Bonala, S., Gluckman, P. D., McFarlane, C., et al. (2012). The ubiquitin ligase Mul1 induces mitophagy in skeletal muscle in response to muscle-wasting stimuli. Cell. Metab. 16 (5), 613–624. doi:10.1016/j.cmet.2012.10.005
Lourenco, C. F., Ledo, A., Barbosa, R. M., and Laranjinha, J. (2017). Neurovascular-neuroenergetic coupling axis in the brain: master regulation by nitric oxide and consequences in aging and neurodegeneration. Free Radic. Biol. Med. 108, 668–682. doi:10.1016/j.freeradbiomed.2017.04.026
Lu, H., Wang, B., Cui, N., and Zhang, Y. (2018). Artesunate suppresses oxidative and inflammatory processes by activating Nrf2 and ROS-dependent p38 MAPK and protects against cerebral ischemia-reperfusion injury. Mol. Med. Rep. 17 (5), 6639–6646. doi:10.3892/mmr.2018.8666
Mai, C., Wu, M., Wang, C., Su, Z., Cheng, Y., and Zhang, X. (2019). Palmatine attenuated dextran sulfate sodium (DSS)-induced colitis via promoting mitophagy-mediated NLRP3 inflammasome inactivation. Mol. Immunol. 105, 76–85. doi:10.1016/j.molimm.2018.10.015
Malone, K., Amu, S., Moore, A. C., and Waeber, C. (2019). The immune system and stroke: from current targets to future therapy. Immunol. Cell. Biol. 97, 5–16. doi:10.1111/imcb.12191
Mangan, M. S. J., Olhava, E. J., Roush, W. R., Seidel, H. M., Glick, G. D., and Latz, E. (2018). Targeting the NLRP3 inflammasome in inflammatory diseases. Nat. Rev. Drug Discov. 17, 688–606. doi:10.1038/nrd.2018.149
Mao, Z., Tian, L., Liu, J., Wu, Q., Wang, N., Wang, G., et al. (2022). Ligustilide ameliorates hippocampal neuronal injury after cerebral ischemia reperfusion through activating PINK1/Parkin-dependent mitophagy. Phytomedicine 101, 154111. doi:10.1016/j.phymed.2022.154111
Murakawa, T., Okamoto, K., Omiya, S., Taneike, M., Yamaguchi, O., and Otsu, K. (2019). A mammalian mitophagy receptor, bcl2-L-13, recruits the ULK1 complex to induce mitophagy. Cell. Rep. 26 (2), 338–345. doi:10.1016/j.celrep.2018.12.050
Murakawa, T., Yamaguchi, O., Hashimoto, A., Hikoso, S., Takeda, T., Oka, T., et al. (2015). Bcl-2-like protein 13 is a mammalian Atg32 homologue that mediates mitophagy and mitochondrial fragmentation. Nat. Commun. 6, 7527. doi:10.1038/ncomms8527
National Pharmacopoeia Commission (2020) Chinese Pharmacopoeia, Part 1. Beijing: China Medical Science and Technology Press, 923–924.
Ni, H., Li, J., Zheng, J., and Zhou, B. (2022). Cardamonin attenuates cerebral ischemia/reperfusion injury by activating the HIF-1α/VEGFA pathway. Phytother. Res. 36 (4), 1736–1747. doi:10.1002/ptr.7409
Novak, I., Kirkin, V., McEwan, D. G., Zhang, J., Wild, P., Rozenknop, A., et al. (2010). Nix is a selective autophagy receptor for mitochondrial clearance. EMBO Rep. 11 (1), 45–51. doi:10.1038/embor.2009.256
Okatsu, K., Oka, T., Iguchi, M., Imamura, K., Kosako, H., Tani, N., et al. (2012). PINK1 autophosphorylation upon membrane potential dissipation is essential for Parkin recruitment to damaged mitochondria. Nat. Commun. 3, 1016. doi:10.1038/ncomms2016
Otera, H., Wang, C., Cleland, M. M., Setoguchi, K., Yokota, S., Youle, R. J., et al. (2010). Mff is an essential factor for mitochondrial recruitment of Drp1 during mitochondrial fission in mammalian cells. J. Cell. Biol. 191 (6), 1141–1158. doi:10.1083/jcb.201007152
Palikaras, K., Lionaki, E., and Tavernarakis, N. (2018). Mechanisms of mitophagy in cellular homeostasis, physiology and pathology. Nat. Cell. Biol. 20 (9), 1013–1022. doi:10.1038/s41556-018-0176-2
Palmer, C. S., Osellame, L. D., Laine, D., Koutsopoulos, O. S., Frazier, A. E., and Ryan, M. T. (2011). MiD49 and MiD51, new components of the mitochondrial fission machinery. EMBO Rep. 12 (6), 565–573. doi:10.1038/embor.2011.54
Papadakis, M., Hadley, G., Xilouri, M., Hoyte, L. C., Nagel, S., McMenamin, M. M., et al. (2013). Tsc1 (hamartin) confers neuroprotection against ischemia by inducing autophagy. Nat. Med. 19 (3), 351–357. doi:10.1038/nm.3097
Pineda-Ramírez, N., Alquisiras-Burgos, I., Ortiz-Plata, A., Ruiz-Tachiquín, M. E., Espinoza-Rojo, M., and Aguilera, P. (2020). Resveratrol activates neuronal autophagy through AMPK in the ischemic brain. Mol. Neurobiol. 57 (2), 1055–1069. doi:10.1007/s12035-019-01803-6
Prabhakaran, S., Ruff, I., and Bernstein, R. A. (2015). Acute stroke intervention: a systematic review. JAMA 313, 1451–1462. doi:10.1001/jama.2015.3058
Puri, R., Cheng, X. T., Lin, M. Y., Huang, N., and Sheng, Z. H. (2020). Defending stressed mitochondria: uncovering the role of MUL1 in suppressing neuronal mitophagy. Autophagy 16 (1), 176–178. doi:10.1080/15548627.2019.1687216
Qin, J., Zhou, J., Dai, X., Zhou, H., Pan, X., Wang, X., et al. (2016). Short-term starvation attenuates liver ischemia-reperfusion injury (IRI) by Sirt1-autophagy signaling in mice. Am. J. Transl. Res. 8 (8), 3364–3375.
Ren, J., Pei, Z., Chen, X., Berg, M. J., Matrougui, K., Zhang, Q., et al. (2019). Inhibition of CYP2E1 attenuates myocardial dysfunction in a murine model of insulin resistance through NLRP3-mediated regulation of mitophagy. Biochimica Biophysica Acta (BBA)- Mol. Basis Dis. 1865, 206–217. doi:10.1016/j.bbadis.2018.08.017
Shadel, G. S., and Horvath, T. L. (2015). Mitochondrial ROS signaling in organismal homeostasis. Cell. 163 (3), 560–569. doi:10.1016/j.cell.2015.10.001
Shao, N., Yu, X., Ma, X., Lin, W., Hao, M., and Kuang, H. (2018). ExenatideDelays the progression of nonalcoholic fatty liver disease in C57bl/6 mice, which may involve inhibition of the NLRP3 inflammasome through the mitophagy pathway. Gastroenterology Res. Pract. 2018, 1864307–1864309. doi:10.1155/2018/1864307
Sharma, M., Akhtar, N., Sambhav, K., Shete, G., Bansal, A. K., and Sharma, S. S. (2015). Emerging potential of citrus flavanones as an antioxidant in diabetes and its complications. Curr. Top. Med. Chem. 15 (2), 187–195. doi:10.2174/1568026615666141209163013
Shi, Y., Liu, Q., Chen, W., Wang, R., Wang, L., Liu, Z. Q., et al. (2023). Protection of Taohong Siwu Decoction on PC12 cells injured by oxygen glucose deprivation/reperfusion via mitophagy-NLRP3 inflammasome pathway in vitro. J. Ethnopharmacol. 301, 115784. doi:10.1016/j.jep.2022.115784
Sulkshane, P., Ram, J., Thakur, A., Reis, N., Kleifeld, O., and Glickman, M. H. (2021). Ubiquitination and receptor-mediated mitophagy converge to eliminate oxidation-damaged mitochondria during hypoxia. Redox Biol. 45, 102047. doi:10.1016/j.redox.2021.102047
Sun, J., Zhou, X., Wu, J., Xiao, R., Chen, Y., Lu, Y., et al. (2021). Ligustilide enhances hippocampal neural stem cells activation to restore cognitive function in the context of postoperative cognitive dysfunction. Eur. J. Neurosci. 54 (3), 5000–5015. doi:10.1111/ejn.15363
Tang, C., Hong, J., Hu, C., Huang, C., Gao, J., Huang, J., et al. (2021). Palmatine protects against cerebral ischemia/reperfusion injury by activation of the AMPK/Nrf2 pathway. Oxid. Med. Cell. Longev. 11, 6660193. doi:10.1155/2021/6660193
Tang, Y. C., Tian, H. X., Yi, T., and Chen, H. B. (2016). The critical roles of mitophagy in cerebral ischemia. Protein Cell. 7 (10), 699–713. doi:10.1007/s13238-016-0307-0
Thayabaranathan, T., Kim, J., Cadilhac, D. A., Thrift, A. G., Donnan, G. A., Howard, G., et al. (2022). Global stroke statistics 2022. Int. J. Stroke 17 (9), 946–956. doi:10.1177/17474930221123175
Tierradentro-García, L. O., Saade-Lemus, S., Freeman, C., Kirschen, M., Huang, H., Vossough, A., et al. (2023). Cerebral blood flow of the neonatal brain after hypoxic-ischemic injury. Am. J. Perinatol. 40 (5), 475–488. doi:10.1055/s-0041-1731278
Tong, Q., Zhu, P. C., Zhuang, Z., Deng, L. H., Wang, Z. H., Zeng, H., et al. (2019). Notoginsenoside R1 for organs ischemia/reperfusion injury: a preclinical systematic review. Front. Pharmacol. 10, 1204. doi:10.3389/fphar.2019.01204
Tsao, C. W., Aday, A. W., Almarzooq, Z. I., Anderson, C. A. M., Arora, P., Avery, C. L., et al. (2023). Heart disease and stroke statistics-2023 update: a report from the American heart association. Circulation 147 (8), e93–e621. doi:10.1161/CIR.0000000000001123
Tu, Y. (2016). Artemisinin—a gift from traditional Chinese medicine to the world (nobel lecture). Angew. Chem. Int. Ed. 55 (35), 10210–10226. doi:10.1002/anie.201601967
Wang, J., Zhang, Y., Zhang, M., Sun, S., Zhong, Y., Han, L., et al. (2022). Feasibility of catalpol intranasal administration and its protective effect on acute cerebral ischemia in rats via anti-oxidative and anti-apoptotic mechanisms. Drug Des. Devel Ther. 16, 279–296. doi:10.2147/DDDT.S343928
Wang, J., and Zhou, H. (2020). Mitochondrial quality control mechanisms as molecular targets in cardiac ischemia–reperfusion injury. Acta Pharm. Sin. B 10, 1866–1879. doi:10.1016/j.apsb.2020.03.004
Wang, M., Liu, Z., Hu, S., Duan, X., Zhang, Y., Peng, C., et al. (2020). Taohong Siwu decoction ameliorates ischemic stroke injury via suppressing pyroptosis. Front. Pharmacol. 11, 590453. doi:10.3389/fphar.2020.590453
Wang, N., Fei, C., Chu, F., Huang, S., Pan, L., Peng, D., et al. (2021). Taohong Siwu decoction regulates cell necrosis and neuroinflammation in the rat middle cerebral artery occlusion model. Front. Pharmacol. 12, 732358. doi:10.3389/fphar.2021.732358
Wang, P., Shao, B., Deng, Z., Chen, S., Yue, Z., and Miao, C. (2018). Autophagy in ischemic stroke. Prog. Neurobiol. 163-164, 98–117. doi:10.1016/j.pneurobio.2018.01.001
Wang, X., Winter, D., Ashrafi, G., Schlehe, J., Wong, Y. L., Selkoe, D., et al. (2011). PINK1 and Parkin target Miro for phosphorylation and degradation to arrest mitochondrial motility. Cell. 147 (4), 893–906. doi:10.1016/j.cell.2011.10.018
Wei, Y., Chiang, W. C., Sumpter, R., Mishra, P., and Levine, B. (2017a). Prohibitin 2 is an inner mitochondrial membrane mitophagy receptor. Cell. 168 (1-2), 224–238. doi:10.1016/j.cell.2016.11.042
Wei, Y., Chiang, W. C., Sumpter, R., Mishra, P., and Levine, B. (2017b). Prohibitin 2 is an inner mitochondrial membrane mitophagy receptor. Cell. 168 (1-2), 224–238. doi:10.1016/j.cell.2016.11.042
Wen, H., Li, L., Zhan, L., Zuo, Y., Li, K., Qiu, M., et al. (2021). Hypoxic postconditioning promotes mitophagy against transient global cerebral ischemia via PINK1/Parkin-induced mitochondrial ubiquitination in adult rats. Cell. Death Dis. 12 (7), 630. doi:10.1038/s41419-021-03900-8
Westermann, B. (2010). Mitochondrial fusion and fission in cell life and death. Nat. Rev. Mol. Cell. Biol. 11 (12), 872–884. doi:10.1038/nrm3013
Wu, M., Yiang, G., Liao, W., Tsai, A., Cheng, Y., Cheng, P., et al. (2018). Current mechanistic concepts in ischemia and reperfusion injury. Cell. Physiology Biochem. 46, 1650–1667. doi:10.1159/000489241
Wu, Y., Liu, H., and Wang, X. (2021). Cardioprotection of pharmacological postconditioning on myocardial ischemia/reperfusion injury. Life Sci. 264, 118628. doi:10.1016/j.lfs.2020.118628
Wu, Z., Qian, S., Zhao, L., Zhang, Z., Song, C., Chen, L., et al. (2022). Metabolomics-based study of the potential interventional effects of Xiao-Xu-Ming Decoction on cerebral ischemia/reperfusion rats. J. Ethnopharmacol. 295, 115379. doi:10.1016/j.jep.2022.115379
Xiao, Q., Kang, Z., Liu, C., and Tang, B. (2022). Panax notoginseng saponins attenuate cerebral ischemia-reperfusion injury via mitophagy-induced inhibition of NLRP3 inflammasome in rats. Front. Biosci. Landmark Ed. 27 (11), 300. doi:10.31083/j.fbl2711300
Xu, Y., Wang, J., Xu, W., Ding, F., and Ding, W. (2019). Prohibitin 2-mediated mitophagy attenuates renal tubular epithelial cells injury by regulating mitochondrial dysfunction and NLRP3 inflammasome activation. Am. J. Physiology-Renal Physiology 316, F396–F407. doi:10.1152/ajprenal.00420.2018
Yamada, T., Murata, D., Adachi, Y., Itoh, K., Kameoka, S., Igarashi, A., et al. (2018). Mitochondrial stasis reveals p62-mediated ubiquitination in parkin-independent mitophagy and mitigates nonalcoholic fatty liver disease. Cell. Metab. 28 (4), 588–604. doi:10.1016/j.cmet.2018.06.014
Yan, J., Ma, H., Lai, X., Wu, J., Liu, A., Huang, J., et al. (2021). Artemisinin attenuated oxidativestress and apoptosis by inhibiting autophagy in MPP+-treated SH-SY5Y cells. J. Biol. Res. 28, article6. doi:10.1186/s40709-021-00137-6
Yang, L., Tao, Y., Luo, L., Zhang, Y., Wang, X., and Meng, X. (2022). Dengzhan Xixin injection derived from a traditional Chinese herb Erigeron breviscapus ameliorates cerebral ischemia/reperfusion injury in rats via modulation of mitophagy and mitochondrial apoptosis. J. Ethnopharmacol. 288, 114988. doi:10.1016/j.jep.2022.114988
Ye, M., Wu, H., and Li, S. (2021). Resveratrol alleviates oxygen/glucose deprivation/reoxygenation-induced neuronal damage through induction of mitophagy. Mol. Med. Rep. 23 (1), 73. doi:10.3892/mmr.2020.11711
Yingze, Y., Zhihong, J., Tong, J., Yina, L., Zhi, Z., Xu, Z., et al. (2022). NOX2-mediated reactive oxygen species are double-edged swords in focal cerebral ischemia in mice. J. Neuroinflammation 19 (1), 184. doi:10.1186/s12974-022-02551-6
Yu, B., Ma, J., Li, J., Wang, D., Wang, Z., and Wang, S. (2020). Mitochondrial phosphatase PGAM5 modulates cellular senescence by regulating mitochondrial dynamics. Nat. Commun. 11 (1), 2549. doi:10.1038/s41467-020-16312-7
Yu, X., Liu, X., Mi, X., Luo, X., Lian, Z., Tang, J., et al. (2023). Jionoside A1 alleviates ischemic stroke ischemia/reperfusion injury by promoting Nix-mediated mitophagy. Cell. Mol. Biol. (Noisy-le-grand) 69 (8), 237–245. doi:10.14715/cmb/2023.69.8.37
Yu, Y., Peng, X. D., Qian, X. J., Zhang, K. M., Huang, X., Chen, Y. H., et al. (2021). Fis1 phosphorylation by Met promotes mitochondrial fission and hepatocellular carcinoma metastasis. Signal Transduct. Target Ther. 6 (1), 401. doi:10.1038/s41392-021-00790-2
Yuan, Y., Zheng, Y., Zhang, X., Chen, Y., Wu, X., Wu, J., et al. (2017). BNIP3L/NIX-mediated mitophagy protects against ischemic brain injury independent of PARK2. Autophagy 13, 1754–1766. doi:10.1080/15548627.2017.1357792
Zhang, J. (2013). Autophagy and mitophagy in cellular damage control. Redox Biol. 1, 19–23. doi:10.1016/j.redox.2012.11.008
Zhang, J., and Ney, P. A. (2009). Role of BNIP3 and NIX in cell death, autophagy, and mitophagy. Cell. Death Differ. 16 (7), 939–946. doi:10.1038/cdd.2009.16
Zhang, Q., Fu, X., Wang, J., Yang, M., and Kong, L. (2017). Treatment effects of ischemic stroke by berberine, Baicalin, and jasminoidin from huang-lian-jie-du-decoction (HLJDD) explored by an integrated metabolomics approach. Oxid. Med. Cell. Longev. 2017, 9848594. doi:10.1155/2017/9848594
Zhang, X. C., and Hu, J. P. (2008). FISSION1A and FISSION1B proteins mediate the fission of peroxisomes and mitochondria in Arabidopsis. Mol. Plant 1 (6), 1036–1047. doi:10.1093/mp/ssn056
Zhang, Y., He, Y., Wu, M., Chen, H., Zhang, L., Yang, D., et al. (2020a). Rehmapicroside ameliorates cerebral ischemia-reperfusion injury via attenuating peroxynitrite-mediated mitophagy activation. Free Radic. Biol. Med. 160, 526–539. doi:10.1016/j.freeradbiomed.2020.06.034
Zhang, Y., He, Y., Wu, M., Chen, H., Zhang, L., Yang, D., et al. (2020b). Rehmapicroside ameliorates cerebral ischemia-reperfusion injury via attenuating peroxynitrite-mediated mitophagy activation. Free Radic. Biol. Med. 160, 526–539. doi:10.1016/j.freeradbiomed.2020.06.034
Zhong, Z., Umemura, A., Sanchez-Lopez, E., Liang, S., Shalapour, S., Wong, J., et al. (2016a). NF-κB restricts inflammasome activation via elimination of damaged mitochondria. Cell. 164 (5), 896–910. doi:10.1016/j.cell.2015.12.057
Zhong, Z., Umemura, A., Sanchez-Lopez, E., Liang, S., Shalapour, S., Wong, J., et al. (2016b). NF-κB restricts inflammasome activation via elimination of damaged mitochondria. Cell. 164 (5), 896–910. doi:10.1016/j.cell.2015.12.057
Zhou, R., Yazdi, A. S., Menu, P., and Tschopp, J. (2011). A role for mitochondria in NLRP3 inflammasome activation. Nature 469 (7329), 221–225. doi:10.1038/nature09663
Keywords: mitophagy, Chinese herbal medicine, cerebral ischemia reperfusion injury, neuroprotective effect, mitochondrial dynamics
Citation: Chen Y, Zhang Y, Wu Q, Chen J and Deng Y (2024) The neuroprotective effect of Chinese herbal medicine for cerebral ischemia reperfusion injury through regulating mitophagy. Front. Pharmacol. 15:1378358. doi: 10.3389/fphar.2024.1378358
Received: 29 January 2024; Accepted: 14 May 2024;
Published: 04 June 2024.
Edited by:
Jiahong Lu, University of Macau, ChinaReviewed by:
Ai-Jun Liu, Second Military Medical University, ChinaSivareddy Challa, University of Illinois at Peoria, United States
Copyright © 2024 Chen, Zhang, Wu, Chen and Deng. This is an open-access article distributed under the terms of the Creative Commons Attribution License (CC BY). The use, distribution or reproduction in other forums is permitted, provided the original author(s) and the copyright owner(s) are credited and that the original publication in this journal is cited, in accordance with accepted academic practice. No use, distribution or reproduction is permitted which does not comply with these terms.
*Correspondence: Yihui Deng, dengyihui@hnucm.edu.cn