- 1Department of Brain Sciences, Imperial College London, London, United Kingdom
- 2Department of Bioengineering, Imperial College London, London, United Kingdom
Hearing loss is an important risk factor for the development of dementia, particularly Alzheimer's disease (AD). Mid-life hearing loss increases the risk of developing dementia by double any other single factor. However, given this strong connection between hearing loss and AD, the mechanisms responsible for this link are still unknown. Data from observational studies relating hearing loss and cognitive impairment, measured with standardized questionnaires, has shown a strong relationship between them. Similar findings have emerged from animal studies, showing that the induction of hearing loss via prolonged loud sound exposure or ear canal blocking, can impair cognitive abilities. Interestingly, patients with age-related hearing impairment exhibit increased phosphorylated tau in the cerebrospinal fluid, but no such relationship has been identified for amyloid-β. In addition, hearing loss predisposes to social isolation precipitating the development of dementia through a supposed reduction in cognitive load and processing requirements. Given this link between hearing loss and dementia, the question arises whether the restoration of hearing might mitigate against the onset or progress of AD. Indeed, there is a growing body of research that suggests that those who wear hearing aids for age-related hearing problems maintain better cognitive function over time than those who do not. These are compelling findings, as they suggest the use of hearing aids has the potential to be a cost-effective treatment for those with hearing loss both prior (for those at high risk for AD) and after the development of symptoms. This review aims to summarize the current theories that relate hearing loss and cognitive decline, present the key findings of animal studies, observational studies and summarize the gaps and limitations that need to be addressed in this topic. Through this, we suggest directions for future studies to tackle the lack of adequately randomized control trials in the field. This omission is responsible for the inability to provide a conclusive verdict on whether to use hearing interventions to target hearing-loss related cognitive decline.
1. Introduction
Dementia poses a global burden—a 2022 report estimated that in 2019, 55 million people were living with a diagnosis of dementia (Gauthier et al., 2022). Future projections suggest that dementia will continue to increase in prevalence, approaching 140 million individuals by 2050 (Gauthier et al., 2022). To slow this exponential growth, novel interventions, either improving management of those already diagnosed, or via the prevention of those at an increased risk of developing it, are imperative.
Logically, risk factors for impaired cognition precede those for dementia, due to its clinical progression. Notably, age is distinguished as an important risk factor—the natural aging process leads to an inherent risk of cognitive decline, independent of dementia. Indeed, aging itself is simultaneously associated with a 70% increased incidence of dementia (Juan and Adlard, 2019). In addition, it is well established that loneliness and social isolation are contributing factors in poor cognitive performance, and are associated with an increased rate of decline (Cacioppo and Hawkley, 2009; Dominguez et al., 2021). Further to this, research has shown that individuals categorized as socially isolated have a 50% increased relative risk of developing dementia (Evans et al., 2018; Dominguez et al., 2021). Interestingly, a recent Lancet review (Livingston et al., 2020) focusing on various modifiable risk factors for the development of dementia, showed the importance of risk reduction, suggesting that modifying such risk factors has the potential to reduce the likelihood of dementia by 40% (LaPlume et al., 2022). Among different risks factors, including traumatic brain injury, hypertension, depression, and diabetes mellitus, the review highlights hearing loss (HL) as one of the potential factors that, when excluded, reduced the risk of dementia by 8%. Mild, moderate or severe HL particularly in the middle-life (specified as between the ages of 45 and 65) has been associated with an increase of 2, 3 and 5 times respectively in the risk of reduced cognition and dementia (Lin et al., 2011; Livingston et al., 2020). Furthermore, observational studies suggest that the severity of hearing impairment (HI) is associated with a risk of accelerated cognitive decline (LaPlume et al., 2022), and one report even demonstrated that the dementia risk increased linearly with the severity of baseline HL (1.27 per 10 dB loss) (Ford et al., 2018). However, limitations remain regarding confounding factors, which may influence the generalizability of these findings (Ford et al., 2018).
In this review, we explore HL as a risk factor for the development of cognitive impairment and dementia, focusing on molecular mechanisms. Through this, we summarize the research surrounding clinical manifestations of HL in dementia, proposed mechanisms to this relationship, and the role of interventions, including the use of hearing aid (HA) devices to minimize the effects of HL in the progression of cognitive impairment to dementia. We highlight the importance of further understanding the relationships between the proposed mechanisms and cognitive impairment, with the eventual aim of providing effective novel interventions to mitigate the risk of HL in the development of dementia.
2. Causes of hearing loss and their link to dementia
Hearing loss affects currently 466 million people Worldwide (World Health Organisation, 2021). Individuals suffering from disabling HL account for over 5% of the global population (World Health Organisation, 2021) and it is predicted that by 2050, nearly 1 in every 10 people will require hearing rehabilitation. Causative factors of hearing loss include genetic factors, ear infections, cerumen impaction (impacted ear wax), trauma to the ear or head, loud noise/loud sounds (NHL), ototoxic medicines and others.
HL mechanisms implicated in dementia predominantly surround sensorineural hearing changes, in which there is dysfunction of the cochlea. Most often, it is seen as age-related hearing loss (ARHL) or presbycusis, which affects around 40% of individuals over the age of 65 (Gates and Mills, 2005). Causes of HL including presbycusis, noise-induced hearing loss (NIHL) and ototoxicity, all precipitate permanent HL, and subsequently, result in limited management options (Lee and Bance, 2019).
ARHL is highly polygenic, with over 100 genes known to underlie human non-syndromic hearing impairment (Lewis et al., 2018; Van Camp and Smith, 2023), and of these many genes, possibly each makes small contributions to create an estimated heritability of 36–70% (Nagtegaal, 2019). Most cases of genetic deafness imply alterations of the cochlea, the auditory sensory organ; for instance, the OTOF gene encodes the protein otoferlin, which allows synaptic vesicles to fuse to the plasma membrane in the ribbon synapse. Thus, mutations in this gene can lead to a deficiency in exocytosis in the inner hair cells, which interrupts auditory signal transmission and can cause prelingual deafness (Vona et al., 2020). However, some forms of genetic hearing loss can involve failures in the central auditory system. Genome wide association studies (GWAS) that have focused on the genes associated with ARHL, although showing some overlap, do not show strong agreement with one another (Nagtegaal, 2019; Wells et al., 2019; Liu et al., 2021; Lewis et al., 2022). When considering a genetic relationship between hearing loss and AD, no GWAS have found a direct causal link between ARHL and AD. However, a recent study using UK biobank data (Brenowitz et al., 2020) demonstrated that a genetic risk for AD also influences speech-in-noise hearing. In addition, in another report (Mitchell et al., 2020), individuals with higher polygenic risk score (PRS) for AD were more likely to experience hearing difficulty than those with lower PRS.
Sudden sensorineural hearing loss (SSHL) has also been identified as a risk factor for the development of dementia. This form of HL is characterized as an otological emergency, defined by HL of at least 30 dB over 72 h, affecting at least three different auditory frequencies, often with a viral, vascular, or autoimmune etiology (Lee and Bance, 2019). In a retrospective cohort study comparing the incidence of dementia in individuals with and without a previous diagnosis of SSHL, it was established that the occurrence of SSHL was associated with a 1.39 times higher likelihood in the incidence of all-cause dementia (Tai et al., 2021).
However, specifically within presbycusis, a variety of other mechanisms have also been implicated, including metabolic factors (for instance, mitochondrial dysfunction), oxidative stress (including changes in reactive oxygen species and Superoxide dismutase deficiency), neurotransmitter imbalance (GABA deficiency), among others (Jafari et al., 2021) (Figure 1). Further insight into the underlying mechanisms and how these different classes of ARHL may herald dementia, is not yet completely understood (Bowl and Dawson, 2019).
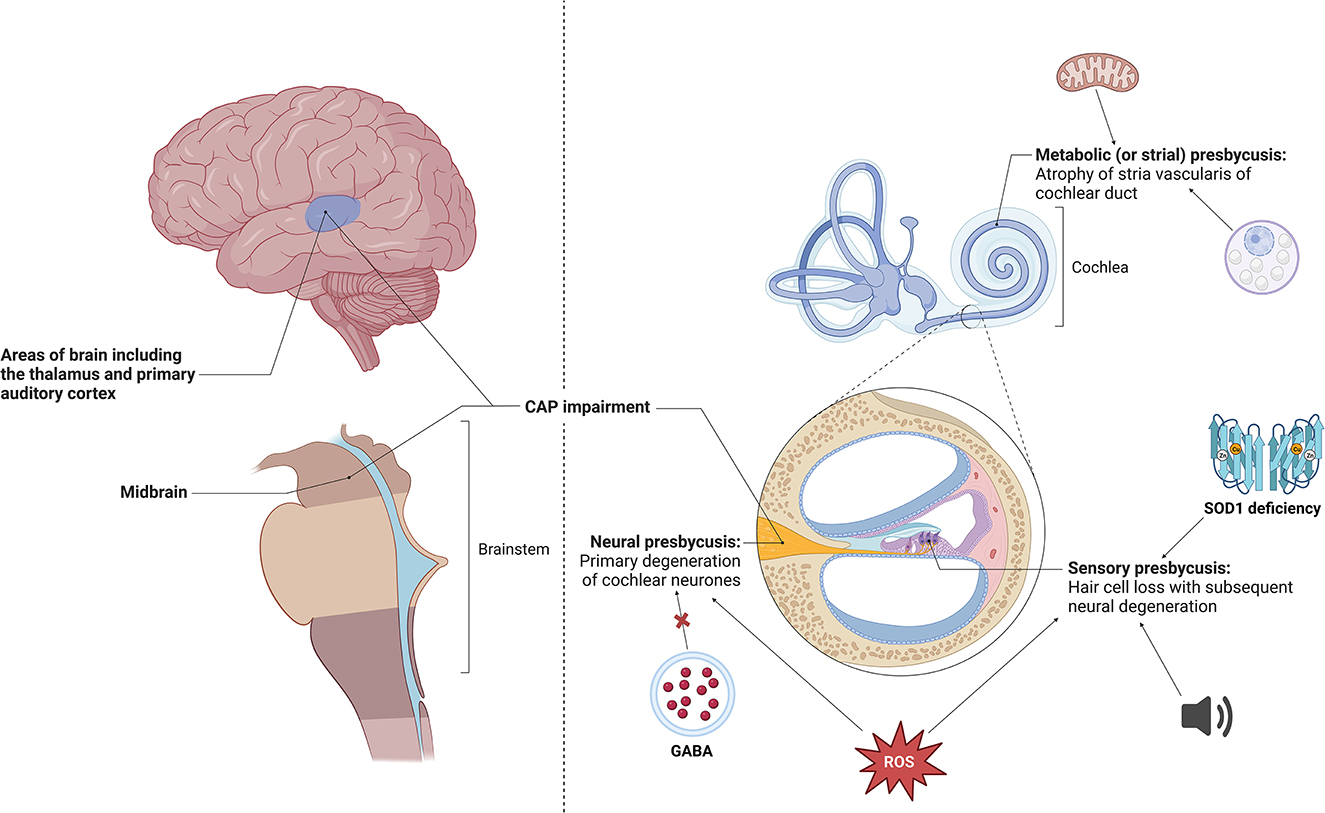
Figure 1. Different types of age-related hearing impairment, including atrophy of the stria vascularis, hair cell loss and primary cochlear neuron degeneration (Quaranta et al., 2015; Fortunato et al., 2016). Specific changes seen in stria vascularis include age-related morphological changes such as numerous cytoplasmic vacuoles, enlargement of intracellular spaces and irregularity of mitochondria and its constituents, most notably disorganization of cristae (Lyu et al., 2020). These changes have been shown to be precipitated by oxidative damage and downregulation of TMEM16A, a calcium-activated chloride channel (Spicer and Schulte, 2005; Zhou et al., 2019). Cochlear disease or trauma have been shown to give rise to hair cell loss, with potential causative factors including continuous industrial noise exposure, reactive oxygen species and Superoxide dismutase (SOD1) deficiency (McFadden et al., 1999a,b, 2001; Emmerich et al., 2000; Huang et al., 2000; Vlajkovic and Thorne, 2021). Primary neural degeneration via disconnecting of auditory neurones from their hair cell targets during aging has been shown to trigger the loss of hair cells (Viana et al., 2015; Wu et al., 2019). Studies have identified that loss of GABA in the central nucleus of the inferior colliculus and the build-up of reactive oxygen species has led to neural presbycusis (Caspary et al., 1990, 2013; Huang et al., 2000). Made using BioRender software. CAP, central auditory processing; ROS, reactive oxygen species.
3. Current understanding of the association between dementia and hearing loss
Poorer scores on the Mini Mental State Examination (MMSE) have been shown to strongly correlate with deficits in audiological testing (Quaranta et al., 2015; Golub et al., 2019; Saji, 2021; Mohammed et al., 2022; Huang et al., 2023). This link is supported by the demonstration of a relationship between HL and MRI brain atrophy (Jafari et al., 2021). Despite a recent study suggesting otherwise (Marinelli et al., 2022), meta-analyses pooling observational studies have further strengthened evidence of an association between ARHL and cognitive decline (Loughrey et al., 2017; Mamo et al., 2018; Liang et al., 2021). Similar correlations between age-associated hearing loss and cognitive decline have been reported in mice (Dong et al., 2018). However, this observational data should be interpreted with caution, as there is a subsequent inability to draw causal links. Additionally, potential confounders that are often seen in the elderly, such as nutritional issues, visual impairment, vascular risk factors, frailty, bad physical health, depression, and other mental illnesses, could contribute to the trend seen (Hirose et al., 2014; Gill et al., 2020). Among those, the weakening of the vascular system is an interesting link, since it is involved in both deafness and neurodegeneration. Vascular networks of the cochlea (Kurata et al., 2016; Nyberg et al., 2019) and the auditory cortex might be impaired in genetic forms of hearing loss, although neural activity by itself can also affect the remodeling of the vascular system (Lacoste et al., 2014; Whiteus et al., 2014).
Interestingly, central auditory pathway disorder in the studied cohorts correlated more with cognitive changes than non-central HL. Why this occurs is not completely understood, but it is hypothesized that the dementia-related neurodegeneration and healthy aging could affect auditory areas of the brain, and lead to subsequent HI (Bidelman et al., 2014; Johnson et al., 2021). Age-related hearing problems are common among people with dementia and are associated with poor cognitive function and reduced quality of life (Maharani et al., 2018a), suggesting that sensory markers could be useful to detect and target cognitive aging.
Contrastingly, there could be an indirect mechanism, as HI predisposes individuals to social isolation and subsequent dementia risk (Sardone et al., 2020). Both dementia and HL present highly heterogeneous conditions, and therefore the investigation of various subtypes within each could be valuable to help further the field of research exploring their interrelationship. Different forms of both dementia and hearing loss may have different trajectories and studies to elucidate this would allow more effective treatments, as shown in frailty (Segaux et al., 2019).
Mirroring human studies, investigations with animal models of AD, including 5XFAD, APP/PS1 and 3XTg transgenic mice have demonstrated both significant HL and degeneration of spiral ganglion cells in the cochlea (Wang and Wu, 2015, 2021; O'Leary et al., 2017; Liu et al., 2020; Weible et al., 2020). Additionally, 5XFAD mice subjected to surgical deafness through tympanic membrane resection i.e., conductive hearing loss (CHL), showed greater cognitive impairment than those with no HI (Kim et al., 2020). This could imply a reciprocal relationship—that AD can lead to auditory deficits and auditory deficits can influence cognition. Whether these AD models can be applied to dementia generally, is not yet known.
4. Mechanisms underlying hearing loss, cognitive function, and AD pathology in animal models
Animal models attempting to elucidate the relationship between dementia and HL center around rodents with induced hearing deficits. Two main paradigms are currently utilized to induce these deficits. Most common methods applied expose rodents to high-volume background noise for several days, inducing permanent trauma (Wang and Wu, 2015; Zhuang et al., 2020; Kurioka et al., 2021a; Li et al., 2021; Paciello et al., 2021), or by the bilateral cochlear ablation. Alternatively, HI is mechanically modeled through insertion of a silicone mold into the ear canal, followed by permanent suturing to close the canal (Paciello et al., 2021). Additionally, administration of ototoxic substances such as furosemide and kanamycin can be employed to induce sensorineural HL, but this is not used often (Shen et al., 2021). These methods inflict physical trauma and stress and subsequently, could influence cognition via mechanisms independent of HI, confounding results. Following HL induction, mice undergo acoustic brainstem response testing, a quantitative method of measuring inner ear and hearing pathway responses to sound (Zhuang et al., 2020; Li et al., 2021). Animal studies more specifically looking at brain region histology conduct further posthumous histology of the animal brain (Li et al., 2021).
One of the key mechanisms explored through animal models and human cases is how, following HL, subsequent decline in hippocampal neurogenesis can lead to cognitive deterioration (Kurioka et al., 2021b). The hippocampus, primarily involved in memory and auditory information processing, is one of the key areas affected in dementia. Interestingly, studies in a rat model with hearing loss and/or Aβ administration demonstrated that the group with lower cognitive abilities was the one with both amyloid-beta (Aβ) and SSHL (Chang et al., 2019). In this report, it was demonstrated a significant decrease in hippocampal synaptic proteins in the Aβ-deaf group, implying that HL influences synaptic plasticity, and that there must be a connection between the central auditory cortex and the hippocampus (Chang et al., 2019).
Another potential link between hearing loss and cognitive impairment in animals implies changes in neuroinflammatory markers. Two animal model studies examining NIHL demonstrated alterations in microglia and the presence of irregularly shaped somas when compared to the control mice (Zhuang et al., 2020; Li et al., 2021). Similar results were reported in a conductive hearing loss (CHL) animal model, showing morphological microglial changes specifically in the dentate gyrus and subgranular zone (Kurioka et al., 2021b). Therefore, it appears that microglial activation does occur following HL and could cause a consequent impairment of hippocampal neurogenesis. However, recent studies seem to contradict these findings, showing that there is no correlation between spatial learning ability and the level of hearing loss or altered microglial density in the hippocampus following noise exposure in rats, suggesting that other mechanisms are involved in the hippocampal-dependent cognitive dysfunction due to noise exposure (Patel et al., 2022). Besides changes in glial cell activation, other studies implementing hearing loss by the use of toxins in mouse models of neurodegeneration have shown increases in inflammatory cytokines such as IL-1β and TNF-α (Ren et al., 2011; Shen et al., 2021).
There are also evidences from human and preclinical studies that AD pathological hallmarks, including hyperphosphorylated Tau (p-Tau) and Aβ, could be affected by HL. A recent report in C57Bl6 in mice exposed to noise induced hearing loss have shown reduced cognition and p-tau and lipofuscin in the hippocampus (Park et al., 2018). Another study in rats also demonstrated that chronic noise exposure (CNE) was associated with tau hyperphosphorylation in the hippocampus and the prefrontal cortex (Cui et al., 2012). In agreement with this, human studies have associated ARHL with higher CSF levels (and accelerated rates of elevation) of p-Tau, but have demonstrated no association between ARHL and the levels of Aβ42 in CSF (Xu et al., 2019; Sarant et al., 2022). In line with this, a cross-sectional cohort study utilizing positron emission tomography scans to investigate the presence of Aβ, also found no association between HL and Aβ load (Sarant et al., 2022). In contrast, studies in rats have shown that chronic exposure to noise resulted in increased generation of endogenous Aβ levels in hippocampus (Cui et al., 2015).
In line with reports showing alterations in hippocampal function, HL has been involved in changes on neurotransmitter expression as well. ARHL mouse models have shown changes in NMDA receptor expression within the hippocampus, which can take place after 4 months of natural HL (Cui et al., 2009). Additionally, GABAa and GABAb receptors were altered during this time period. The change in these neurotransmitter receptors is implicated in hippocampal synaptic plasticity, affecting hippocampal learning, and resulting in cognitive decline (Guo et al., 2021).
An emerging hypothesis links social memory impairment and HL—partially due to limitations of social interaction faced by those with a HI (Beckmann et al., 2020). A study using a mouse model of congenital deafness with OTOF gene knockout, attempted to investigate this through eliminating hearing input from the cochlea to the brain, resulting in decreased social memory. If this hypothesis is correct, it would help explain why associated brain regions' functionality, for example, the hippocampus, is affected following HL (Glick and Sharma, 2020). Furthermore, there is the possibility of a compensatory mechanism, whereby the brain may offset certain neural networks to promote better hearing. In line with this, a human MRI study of HI individuals demonstrated that auditory brain regions displayed an increase in activity, whilst other areas showed a consequential decrease (Rigters et al., 2017). Therefore, this could indicate that this activity decrease is linked to the reduction in cognitive function seen in HL.
5. Clinical interventions and their limitations
As discussed, observational studies have established an association between HI and an increased risk of accelerated cognitive decline. The emphasis placed on HL as a modifiable risk factor for dementia (LaPlume et al., 2022) has led to the reasonable hypothesis that audibility restoration could potentially alleviate cognitive decline, by counteracting the postulated mechanisms which underlie this process and by reducing social isolation, loneliness, and depressed mood (Jiang et al., 2023). It is hypothesized that hearing restoration, may reverse cognitive changes of cortical reallocation of sensory processing patterns, leading to consequent cognitive gains (Glick and Sharma, 2020).
In two short-term rodent studies modeling CHL through use of ear plugs, significant cochlear degeneration (Kurioka et al., 2021b) and changes in the neurotransmitter expression within auditory neurons (Kurioka et al., 2020) was demonstrated following reduced auditory inputs. Interestingly, removal of the ear plugs led to recovery of almost all these anatomical changes, suggesting that similar treatment with either HAs or cochlear implantation in humans could reverse such changes, and perhaps cognitive processing.
Overall, many observational, prospective and retrospective cohort, cross-sectional and longitudinal studies have linked HA use to lower rates of cognitive decline (Maharani et al., 2018b; Glick and Sharma, 2020; Cuoco et al., 2021; Fernandes and Mastroianni Kirsztajn, 2021; Sugiura et al., 2021, 2022; Bucholc et al., 2022; Dillard et al., 2022; Vella Azzopardi et al., 2023) and dementia (Maharani et al., 2018b; Mahmoudi et al., 2019; Byun et al., 2022; Dillard et al., 2022; Naylor et al., 2022). Recent data from the UK biobank support these findings, showing no increased risk of dementia in people with hearing loss using hearing aids (Jiang et al., 2023). Strikingly, one of the studies demonstrated that the diagnosis of dementia is associated with a 54% subsequent reduction in HA usage compared to those without dementia (Naylor et al., 2022). The importance is thus placed on not only increasing HA usage amongst older individuals at higher risk of both HL and dementia, but also working to maximize adherence in this community.
On the other hand, two prospective longitudinal studies found no statistical significance between cognitive scores pre- and post- HA fittings (Sarant et al., 2020; Kawade et al., 2022). Furthermore, the only double-blind, randomized controlled trial evaluating the cognitive benefit of HAs in patients with AD also found no statistically significant relationship (Nguyen et al., 2017). There is a clear lack of consensus of the current evidence, but this could be explained by the various limitations and differences across all the study designs.
Generally, studies investigating cognition pre- vs. post- cochlear implantation reported either no change (Sonnet et al., 2017; Kramer et al., 2018; Sarant et al., 2019), an improvement (Jayakody et al., 2017; Claes et al., 2018; Mosnier et al., 2018; Völter et al., 2021, 2022a,b; Calvino et al., 2022), or a mixed picture across the various subtests (Sorrentino et al., 2020; Huber et al., 2021). Cochlear implantation is one phase of a complex rehabilitation process involving multiple appointments and training programmes following surgery (British Cochlear Implant Group, 2023). These all pose opportunities for increased engagement in cognitive stimulation, known to reduce risk of cognitive decline. Whether the cognitive improvement seen across these studies is due to restored hearing itself or a confounding influence of rehabilitation, would be difficult to determine (Völter et al., 2022b).
Much of the heterogeneity across both the hearing aids and cochlear implantation literature exists due to the variety of tests used to measure cognitive function. Some papers choose to use standardized screening tests like the MMSE (Sonnet et al., 2017; Sorrentino et al., 2020; Herzog et al., 2022; Vella Azzopardi et al., 2023), or the MoCA (Cuoco et al., 2021; Fernandes and Mastroianni Kirsztajn, 2021) as an assessment of global cognitive function, whilst other studies focus on assessing subdomains of cognition through use of investigations like the ALAcog (Sarant et al., 2019; Völter et al., 2021; Calvino et al., 2022), ADAScog (Nguyen et al., 2017), Cogstate (Sarant et al., 2019, 2020), RBANS-H (Claes et al., 2018; Brewster et al., 2020; Calvino et al., 2022) and various other subtests (Jayakody et al., 2017; Mosnier et al., 2018; Füllgrabe, 2020; Kurioka et al., 2020). Using tests like the MMSE, which utilize auditory assessments, leaves HI individuals at a direct disadvantage. Cognition prior to rehabilitation could be underestimated, and treatment may simply allow the participant to perform better due to increased ability to understand the task presented (Füllgrabe, 2020). A ceiling effect is also observed through use of MMSE (Sonnet et al., 2017)—those with a normal cognition pre-intervention are unable to show any further improvement. The introduction of a standardized neurocognitive battery, which assesses all necessary subdomains and is adapted for the HI, needs to be implemented to allow comparison across the literature.
Further sources of heterogeneity across results could be due to the varying durations of studies. Undoubtedly, measures of cognitive function are susceptible to time due to the cognitive decline observed with aging. Some studies examined cognitive outcomes over longer periods, ranging from 3.5 to 18 years (Maharani et al., 2018b; Naylor et al., 2022; Sugiura et al., 2022), whilst others observed significantly shorter periods; for example, one report (Glick and Sharma, 2020) studied participants over 6 months, whilst another (Fernandes and Mastroianni Kirsztajn, 2021) over only 12 weeks. Shorter trials should be interpreted with additional caution—interventions may not have significant time to influence a change, nor do they consider the normal cognitive decline represented over time.
6. Conclusions
Overall, a clear trend between HL and dementia is demonstrated across the literature. However, evidence is largely composed of observational studies and there remains a lack of interventional studies relating HL to dementia. This is due to a clinical dilemma- that it would be unethical to deny treatment to those with HL, especially when considering the ramifications of untreated HL, not just in relation to dementia. The introduction of open label trials, recruiting from surgical candidates for cochlear implantation, could circumvent this. These trials could build on previous limitations by including a standardized neurocognitive battery. Furthermore, despite a multitude of animal models demonstrating potential underlying mechanisms linking HI and cognitive decline, further research, particularly with longer follow-up periods and invasive recording technology is required to help interrogate the mechanisms of action.
Uncertainties also remain in the implementation of HAs and CIs as interventions against cognitive decline. As a result, algorithms and guidelines may need to be synthesized from future interventional studies to best improve patient outcomes. There should be a focus on maximizing early HL diagnoses and swift implementation of auditory treatment to mitigate the risks of cognitive decline. Models calculating dementia risk would allow the stratification of the geriatric population and perhaps help clinicians decide risk and subsequent necessary auditory treatment. Additionally, technology such as smart phone connected HAs could target the current limitations encompassing HA usage in those with dementia.
Author contributions
AA wrote the current understanding of the association between dementia and hearing loss. AJ wrote the introduction and the most of the section of causes of hearing loss and dementia and made the figure. BK wrote the clinical interventions and their limitations. IS wrote the mechanisms underlying hearing loss, cognitive function, and AD Pathology in animal models. MB-H and MS designed, edited the manuscript and organized the references. All authors contributed to the article and approved the submitted version.
Conflict of interest
MB-H and MS declared that they were an editorial board member of Frontiers, at the time of submission. This had no impact on the peer review process and the final decision.
The remaining authors declare that the research was conducted in the absence of any commercial or financial relationships that could be construed as a potential conflict of interest.
Publisher's note
All claims expressed in this article are solely those of the authors and do not necessarily represent those of their affiliated organizations, or those of the publisher, the editors and the reviewers. Any product that may be evaluated in this article, or claim that may be made by its manufacturer, is not guaranteed or endorsed by the publisher.
References
Beckmann, D., Feldmann, M., Shchyglo, O., and Manahan-Vaughan, D. (2020). Hippocampal synaptic plasticity, spatial memory, and neurotransmitter receptor expression are profoundly altered by gradual loss of hearing ability. Cereb. Cort. 30, 4581–4596. doi: 10.1093/cercor/bhaa061
Bidelman, G. M., Villafuerte, J. W., Moreno, S., and Alain, C. (2014). Age-related changes in the subcortical-cortical encoding and categorical perception of speech. Neurobiol. Aging 35, 2526–40. doi: 10.1016/j.neurobiolaging.2014.05.006
Bowl, M. R., and Dawson, S. J. (2019). Age-Related Hearing Loss. Cold Spring Harbor Perspect. Biol. 9, a033217. doi: 10.1101/cshperspect.a033217
Brenowitz, W. D., Filshtein, T. J., Yaffe, K., Walter, S., Ackley, S. F., Hoffmann, T. J., et al. (2020). Association of genetic risk for Alzheimer disease and hearing impairment. Neurology 95, e2225. doi: 10.1212/WNL.0000000000010709
Brewster, K. K., Pavlicova, M., Stein, A., Chen, M., Chen, C., Brown, P. J., et al. (2020). A pilot randomized controlled trial of hearing aids to improve mood and cognition in older adults. Int. J. Geriatric Psychiatry. 35, 842–850. doi: 10.1002/gps.5311
British Cochlear Implant Group (2023). Rehabilitation. Available online at: https://www.bcig.org.uk/assessed/rehabilitation/ (accessed 2023).
Bucholc, M., Bauermeister, S., Kaur, D., McClean, P. L., and Todd, S. (2022). The impact of hearing impairment and hearing aid use on progression to mild cognitive impairment in cognitively healthy adults: an observational cohort study. Alzheimer's Dement. 8, e12248. doi: 10.1002/trc2.12248
Byun, H., Chung, J. H., Lee, S. H., Kim, E. M., and Kim, I. (2022). Dementia in a hearing-impaired population according to hearing aid use: a nationwide population-based study in Korea. Ear Hear. 43, 1661–1668. doi: 10.1097/AUD.0000000000001249
Cacioppo, J. T., and Hawkley, L. C. (2009). Perceived social isolation and cognition. Trends Cogn. Sci. 13, 447–454. doi: 10.1016/j.tics.2009.06.005
Calvino, M., Sánchez-Cuadrado, I., Gavilán, J., Gutiérrez-Revilla, M. A., Polo., R., and Lassaletta, L. (2022). Effect of cochlear implantation on cognitive decline and quality of life in younger and older adults with severe-to-profound hearing loss. Eur. Arch. Otorhinolaryngol. 279,4745–4759. doi: 10.1007/s00405-022-07253-6
Caspary, D. M., Hughes, L. F., and Hughes, L. L. (2013). Age-related GABAA receptor changes in rat auditory cortex. Neurobiol. Aging 34, 1486–1496. doi: 10.1016/j.neurobiolaging.2012.11.009
Caspary, D. M., Raza, A., Lawhorn, Armour, B. A., Pippin, J., and Arneric, S. P. (1990). Immunocytochemical and neurochemical evidence for age-related loss of GABA in the inferior colliculus: implications for neural presbycusis. J. Neurosci. 10, 2363–2372. doi: 10.1523/JNEUROSCI.10-07-02363.1990
Chang, M., Kim, H. J., Mook-Jung, I., and Oh, S. (2019). Hearing loss as a risk factor for cognitive impairment and loss of synapses in the hippocampus. Behav. Brain Res. 372, 112069. doi: 10.1016/j.bbr.2019.112069
Claes, A., Van de Heyning, P., Gilles, A., Van Rompaey, V., and Mertens, G. (2018). Cognitive performance of severely hearing-impaired older adults before and after cochlear implantation: preliminary results of a prospective, longitudinal cohort study using the RBANS-H. Otol. Neurotol. 39, e765–e773. doi: 10.1097/MAO.0000000000001936
Cui, B., Li, K., Gai, Z., She, X., Zhang, N., Xu, C., et al. (2015). Chronic noise exposure acts cumulatively to exacerbate Alzheimer's disease-like Amyloid-β pathology and neuroinflammation in the rat hippocampus. Sci Rep. 5,12943. doi: 10.1038/srep12943
Cui, B., Wu, M., and She, X. (2009). Effects of chronic noise exposure on spatial learning and memory of rats in relation to neurotransmitters and NMDAR2B alteration in the hippocampus. J. Occup. Health 51,152–8. doi: 10.1539/joh.L8084
Cui, B., Zhu, L., She, X., Wu, M., Ma, Q., Wang, T., et al. (2012). Chronic noise exposure causes persistence of tau hyperphosphorylation and formation of NFT tau in the rat hippocampus and prefrontal cortex. Exp Neurol. 238, 122–9. doi: 10.1016/j.expneurol.2012.08.028
Cuoco, S., Cappiello, A., Scarpa, A., Troisi, D., Autuori, M., Ponticorvo, S., et al. (2021). Neuropsychological profile of hearing-impaired patients and the effect of hearing aid on cognitive functions: an exploratory study. Sci. Rep. 11, 9384. doi: 10.1038/s41598-021-88487-y
Dillard, L. K., Pinto, A., Mueller, K. D., Schubert, C. R., Paulsen, A. J., Merten, N., et al. (2022). Associations of hearing loss and hearing aid use with cognition, health-related quality of life, and depressive symptoms. J. Aging Health. doi: 10.1177/08982643221138162
Dominguez, L. J., Veronese, N., Vernuccio, L, Catanese, G., Inzerillo, F., Salemi, G., et al. (2021). Nutrition, physical activity, and other lifestyle factors in the prevention of cognitive decline and dementia. Nutrients 13, 4080. doi: 10.3390/nu13114080
Dong, Y., Guo, C., Chen, D., Chen, S., Peng, Y., Song, H., et al. (2018). Association between age-related hearing loss and cognitive decline in C57BL/6J mice. Mol. Med. Rep. 18, 1726–1732. doi: 10.3892/mmr.2018.9118
Emmerich, E. C., Richter, F. G., Reinhold, U., Linss, V., and Linss, W. (2000). Effects of industrial noise exposure on distortion product otoacoustic emissions (DPOAEs) and hair cell loss of the cochlea – long term experiments in awake guinea pigs. Hear. Res. 148, 9–17. doi: 10.1016/S0378-5955(00)00101-5
Evans, I. E. M., Llewellyn, D. J., Matthews, F. E., Woods, R. T., Brayne, C., and Clare, L. (2018). Social isolation, cognitive reserve, and cognition in healthy older people. PLoS ONE 13, e0201008. doi: 10.1371/journal.pone.0201008
Fernandes, D. E., and Mastroianni Kirsztajn, G. Almeida, K. (2021). Effect of hearing aids on attention, memory, and auditory evoked potentials: a pragmatic, single-blinded, and randomised pilot clinical trial. Int. J. Clin. Pract. 75, e13953. doi: 10.1111/ijcp.13953
Ford, A. H., Hankey, G. J., Yeap, B. B., Golledge, J., Flicker, L., and Almeida, O.P. (2018). Hearing loss and the risk of dementia in later life. Maturitas 112, 1–11. doi: 10.1016/j.maturitas.2018.03.004
Fortunato, S., Forli, F., Guglielmi, V., De Corso, E., Paludetti, G., Berrettini, S., et al. (2016). A review of new insights on the association between hearing loss and cognitive decline in ageing. Acta Otorhinolaryngol. Italica. 36, 155–166. doi: 10.14639/0392-100X-993
Füllgrabe, C. (2020). On the possible overestimation of cognitive decline: the impact of age-related hearing loss on cognitive-test performance. Front. Neurosci. 14, 454. doi: 10.3389/fnins.2020.00454
Gates, G. A., and Mills, J.H. (2005). Presbycusis. Lancet 366, 1111–1120. doi: 10.1016/S0140-6736(05)67423-5
Gauthier, S., Webster, C., Servaes, S., Morais, J. A., and Rosa-Neto, P. (2022). World Alzheimer Report 2022: Life After Diagnosis: Navigating Treatment, Care and Support. London: Alzheimer's Disease International.
Gill, T. M., Han, L., Gahbauer, E. A., Leo-Summers, L., and Murphy, T. E. (2020). Risk factors and precipitants of severe disability among community-living older persons. JAMA Network Open 3, e206021. doi: 10.1001/jamanetworkopen.2020.6021
Glick, H. A., and Sharma, A. (2020). Cortical neuroplasticity and cognitive function in early-stage, mild-moderate hearing loss: evidence of neurocognitive benefit from hearing aid use. Front. Neurosci.14, 93. doi: 10.3389/fnins.2020.00093
Golub, J. S., Brickman, A. M., Ciarleglio, A. J., Schupf, N., and Luchsinger, J.A. (2019). Association of subclinical hearing loss with cognitive performance. Arch. Otolaryngol. Head Neck Surg. 146, 57–67. doi: 10.1001/jamaoto.2019.3375
Guo, R., Li, Y., Liu, J., Gong, S., and Liu, K. (2021). Complete elimination of peripheral auditory input before onset of hearing causes long-lasting impaired social memory in mice. Front. Neurosci. 15, 723658. doi: 10.3389/fnins.2021.723658
Herzog, J. A., Buchman, C. A., Kallogjeri, D., Chen, S., Wick, C., Durakovic, N., et al. (2022). Cognitive assessment in elderly cochlear implant recipients: long-term analysis. Laryngoscope. doi: 10.1002/lary.30466
Hirose, T., Hasegawa, J., Izawa, S., Enoki, H., Suzuki, Y., and Kuzuya, M. (2014). Accumulation of geriatric conditions is associated with poor nutritional status in dependent older people living in the community and in nursing homes. Geriat. Gerontol. Int. 14, 198–205. doi: 10.1111/ggi.12079
Huang, A. R., Jiang, K., Lin, F. R., Deal, J. A., and Reed, N. S. (2023). Hearing loss and dementia prevalence in older adults in the US. JAMA 329, 171–173. doi: 10.1001/jama.2022.20954
Huang, T., Cheng, A. G., Stupak, H., Liu, W., Kim, A., Staecker, H., et al. (2000). Oxidative stress-induced apoptosis of cochlear sensory cells: otoprotective strategies. Int. J. Dev. Neurosci. 18, 259–270. doi: 10.1016/S0736-5748(99)00094-5
Huber, M., Roesch, S., Pletzer, B., Lukaschyk, J., Lesinski-Schiedat, A., and Illg, A. (2021). Can cochlear implantation in older adults reverse cognitive decline due to hearing loss? Ear Hear. 42, 1560–1576. doi: 10.1097/AUD.0000000000001049
Jafari, Z., Kolb, B. E., and Mohajerani, M. H. (2021). Age-related hearing loss and cognitive decline: MRI and cellular evidence. Ann. N.Y. Acad. Sci 1500, 17–33. doi: 10.1111/nyas.14617
Jayakody, D. M., Friedland, P., Nel, E., Martins, R., Atlas, M., and Sohrabi, H. (2017). Impact of cochlear implantation on cognitive functions of older adults: pilot test results. Otol. Neurotol. 38, e289–e295. doi: 10.1097/MAO.0000000000001502
Jiang F. Mishra S. R. Shrestha N. Ozaki A. Virani S. S. Bright T. (2023) Association between hearing aid use all-cause cause-specific dementia: an analysis of the UK Biobank cohort. Lancet Public Health 8, e329. 10.1016/S2468-2667(23)00048-8 .
Johnson, J. C. S., Marshall, C. R., Weil, R. S., Bamiou, D., Hardy, C. J. D., and Warren, J.D. (2021). Hearing and dementia: from ears to brain. Brain 144, 391–401. doi: 10.1093/brain/awaa429
Juan, S. M. A., and Adlard, P. A. (2019). Ageing and cognition biochemistry and cell biology of ageing: part II clinical science. J. Robin Harris, Viktor I. Korolchuk. Springer 91, 107–122. doi: 10.1007/978-981-13-3681-2_5
Kawade, Y., Uchida, Y., Sugiura, S., Suzuki, H., Shimono, M., Ito, E., et al. (2022). Relationship between cognitive domains and hearing ability in memory clinic patients: how did the relationship change after 6 months of introducing a hearing aid? Auris Nasus Larynx. 50, 343–350. doi: 10.1016/j.anl.2022.09.005
Kim, J. S., Lee, H., Lee, S., Lee, H. S., Jeong, Y. J., Son, Y., et al. (2020). Conductive hearing loss aggravates memory decline in Alzheimer model mice. Front Neurosci. 14, 843. doi: 10.3389/fnins.2020.00843
Kramer, S., Vasil, K. J., Adunka, O. F., Pisoni, D. B., and Moberly, A. C. (2018). Cognitive functions in adult cochlear implant users, cochlear implant candidates, and normal-hearing listeners. Laryngoscope Invest. Otolaryngol. 3, 304–310. doi: 10.1002/lio2.172
Kurata, N., Schachern, P. A., Paparella, M. M., and Cureoglu, S. (2016). Histopathologic evaluation of vascular findings in the cochlea in patients with presbycusis. JAMA Otolaryngol. Head Neck Surg. 142, 173–178. doi: 10.1001/jamaoto.2015.3163
Kurioka, T., Mogi, S., Tanaka, M., and Yamashita, T. (2021a). Activity-dependent neurodegeneration and neuroplasticity of auditory neurons following conductive hearing loss in adult mice. Cell. Mol. Neurobiol. 41, 31–42. doi: 10.1007/s10571-020-00829-y
Kurioka, T., Mogi, S., and Yamashita, T. (2021b). Decreasing auditory input induces neurogenesis impairment in the hippocampus. Sci. Rep. 11, 423. doi: 10.1038/s41598-020-80218-z
Kurioka, T., Mogi, S., and Yamashita, T. (2020). Transient conductive hearing loss regulates cross-modal VGLUT expression in the cochlear nucleus of C57BL/6 mice. Brain Sci. 10, 260. doi: 10.3390/brainsci10050260
Lacoste, B., Comin, C. H., Ben-Zvi, A., Kaeser, P. S., Xu, X., Costa Lda, F., et al. (2014). Sensory-related neural activity regulates the structure of vascular networks in the cerebral cortex. Neuron 83, 1117–30. doi: 10.1016/j.neuron.2014.07.034
LaPlume, A. A., McKetton, L., Levine, B., and Troyer, A.K. (2022). The adverse effect of modifiable dementia risk factors on cognition amplifies across the adult lifespan. Alzheimer's Dementia. 14, e12337. doi: 10.1002/dad2.12337
Lee, J. W., and Bance, M.L. (2019). Hearing loss. Pract. Neurol. 19, 28–35. doi: 10.1136/practneurol-2018-001926
Lewis, M. A., Nolan, L. S., Cadge, B. A., Matthews, L. J., Schulte, B. A., Dubno, J. R., et al. (2018). Whole exome sequencing in adult-onset hearing loss reveals a high load of predicted pathogenic variants in known deafness-associated genes and identifies new candidate genes. BMC Med. Genom. 11, 77. doi: 10.1186/s12920-018-0395-1
Lewis, M. A., Schulte, B. A., Dubno, J. R., and Steel, K. P. (2022). Investigating the characteristics of genes and variants associated with self-reported hearing difficulty in older adults in the UK Biobank. BMC Biol. 20, 150. doi: 10.1186/s12915-022-01349-5
Li, Q., Li, H., Yao, X., Wang, C., Liu, H., Xu, D., et al. (2021). Stress response and hearing loss differentially contribute to dynamic alterations in hippocampal neurogenesis and microglial reactivity in mice exposed to acute noise exposure. Front. Neurosci. 15, 749925. doi: 10.3389/fnins.2021.749925
Liang, Z., Li, A., Xu, Y., Qian, X., and Gao, X. (2021). Hearing loss and dementia: a meta-analysis of prospective cohort studies. Front. Aging Neurosci. 13, 695117. doi: 10.3389/fnagi.2021.695117
Lin, F. R., Metter, E. J., O'Brien, R. J., Resnick, S. M., Zonderman, A. B., and Ferrucci, L. (2011). Hearing loss and incident dementia. Arch. Neurol. 6, 214–220. doi: 10.1001/archneurol.2010.362
Liu, W., Johansson, Å., Rask-Andersen, H., and Rask-Andersen, M. (2021). A combined genome-wide association and molecular study of age-related hearing loss in H. sapiens. BMC Med. 19, 302. doi: 10.1186/s12916-021-02169-0
Liu, Y., Fang, S., Liu, L., Zhu, Y., Li, C., Chen, K., et al. (2020). Hearing loss is an early biomarker in APP/PS1 Alzheimer's disease mice. Neurosci. Letters 717, 134705. doi: 10.1016/j.neulet.2019.134705
Livingston, G., Huntley, J., Sommerlad, A., Ames, D., Ballard, C., Banerjee, S., et al. (2020). Dementia prevention, intervention, and care: 2020 report of the lancet commission. Lancet 396, 413–446. doi: 10.1016/S0140-6736(20)30367-6
Loughrey, D. G., Kelly, M. E., Kelley, G. A., Brennan, S., and Lawlor, B. A. (2017). Association of age-related hearing loss with cognitive function, cognitive impairment, and dementia: a systematic review and meta-analysis. JAMA Otolaryngol. 144, 115–126. doi: 10.1001/jamaoto.2017.2513
Lyu, A., Kim, T. H., Park, S. J., Shin, S., Jeong, S., Yu, Y. N., et al. (2020). Mitochondrial damage and necroptosis in aging cochlea. Int. J. Mol. Sci. 21, 2505. doi: 10.3390/ijms21072505
Maharani, A., Dawes, P., Nazroo, J., Tampubolon, G., and Pendleton, N.; Sense-Cog WP1 group (2018a). Visual and hearing impairments are associated with cognitive decline in older people. Age Ageing 47, 575–581. doi: 10.1093/ageing/afy061
Maharani, A., Tampubolon, G., Dawes, P., Nazroo, J., and Pendleton, N. (2018b). Hearing aid use and cognitive function in older adults. J. Am. Ger. Soc. 66, 1130–1136. doi: 10.1111/jgs.15363
Mahmoudi, E., Basu, T., Langa, K., McKee, M. M., Zazove, P., Alexander, N, et al. (2019). Can hearing aids delay time to diagnosis of dementia, depression, or falls in older adults? J. Am. Ger. Soc 67, 2362–2369. doi: 10.1111/jgs.16109
Mamo, S. K., Reed, N. S., Price, C., Occhipinti, D., Pletnikova, A., Lin, F. R., et al. (2018). Hearing loss treatment in older adults with cognitive impairment: a systematic review. J Speech Lang Hear Res. 61, 2589–2603. doi: 10.1044/2018_JSLHR-H-18-0077
Marinelli, J. P., Lohse, C. M., Fussell, W. L., Petersen, R. C., Reed, N. S., Machulda, M. M., et al. (2022). Association between hearing loss and development of dementia using formal behavioural audiometric testing within the Mayo Clinic Study of Aging (MCSA): a prospective population-based study. Lancet 3, e817–e824. doi: 10.1016/S2666-7568(22)00241-0
McFadden, S. L., Ding, D., Burkard, R. F., Jiang, H., Reaume, A. G., Flood, D. G., et al. (1999b). Cu/Zn SOD deficiency potentiates hearing loss and cochlear pathology in aged 129, CD-1 mice. J. Comp. Neurol. 413, 101–112.
McFadden, S. L., Ding, D., Reaume, A. G., Flood, D. G., and Salvi, R. J. (1999a). Age-related cochlear hair cell loss is enhanced in mice lacking copper/zinc superoxide dismutase. Neurobiol. Aging. 20, 1–8. doi: 10.1016/S0197-4580(99)00018-4
McFadden, S. L., Ding, D., and Salvi, R. (2001). Anatomical, metabolic and genetic aspects of age-related hearing loss in mice. Audiology 40, 313–321. doi: 10.3109/00206090109073128
Mitchell, B. L., Thorp, J. G., Evans, D. M., Nyholt, D. R., Martin, N. G., and Lupton, M. K. (2020). Exploring the genetic relationship between hearing impairment and Alzheimer's disease. Alzheimer's Dement. 12, e12108. doi: 10.1002/dad2.12108
Mohammed, A., Gibbons, L. E., Gates, G., Anderson, M.L., McCurry, S. M., McCormick, W., et al. (2022). Association of performance on dichotic auditory tests with risk for incident dementia and Alzheimer dementia. Arch. Otolaryngol Head Neck Surg. 148, 20–27. doi: 10.1001/jamaoto.2021.2716
Mosnier, I., Vanier, A., Bonnard, D., Lina-Granade, G., Truy, E., Bordure, P., et al. (2018). Long-term cognitive prognosis of profoundly deaf older adults after hearing rehabilitation using cochlear implants. J. Am. Ger. Soc. 66, 1553–1561. doi: 10.1111/jgs.15445
Nagtegaal, AP, Broer L, Zilhao NR, Jakobsdottir J, Bishop CE. (2019). Genome-wide association meta-analysis identifies five novel loci for age-related hearing impairment. Sci. Rep. 9, 15192. doi: 10.1038/s41598-019-51630-x
Naylor, G., Dillard, L., Orrell, M., Stephan, B. C. M., Zobay, O., and Saunders, G. H. (2022). Dementia and hearing-aid use: a two-way street. Age Ageing 51, afac266. doi: 10.1093/ageing/afac266
Nguyen, M., Bonnefoy, M., Adrait, A., Gueugnon, M., Petitot, C., Collet, L., et al. (2017). Efficacy of hearing aids on the cognitive status of patients with Alzheimer's disease and hearing loss: a multicenter controlled randomized trial. J. Alz. Dis. 58, 123–137. doi: 10.3233/JAD-160793
Nyberg, S., Abbott, N. J., Shi, X., Steyger, P. S., and Dabdoub, A. (2019). Delivery of therapeutics to the inner ear: The challenge of the blood-labyrinth barrier. Sci. Transl. Med. 11, eaao0935. doi: 10.1126/scitranslmed.aao0935
O'Leary, T. P., Shin, S., Fertan, E., Dingle, R. N., Almuklass, A., Gunn, R. K., et al. (2017). Reduced acoustic startle response and peripheral hearing loss in the 5xFAD mouse model of Alzheimer's disease. Genes Brain Behav. 16, 554–563. doi: 10.1111/gbb.12370
Paciello, F., Rinaudo, M., Longo, V., Cocco, S., Conforto, G., Pisani, A., et al. (2021). Auditory sensory deprivation induced by noise exposure exacerbates cognitive decline in a mouse model of Alzheimer's disease. eLife 10, e70908. doi: 10.7554/eLife.70908.sa2
Park, S. Y., Kim, M. J., Kim, H. L., Kim, D. K., Yeo, S. W., and Park, S. N. (2018). Cognitive decline and increased hippocampal p-tau expression in mice with hearing loss. Beh. Brain Res. 342, 19–26. doi: 10.1016/j.bbr.2018.01.003
Patel, S. V., DeCarlo, C. M., Book, S. A., Schormans, A. L., Whitehead, S. N., Allman, B. L., et al. (2022). Noise exposure in early adulthood causes age-dependent and brain region-specific impairments in cognitive function. Front Neurosci. 16, 1001686. doi: 10.3389/fnins.2022.1001686
Quaranta, N., Coppola, F., Casulli, M., Barulli, O., Lanza, F., Tortelli, R., et al. (2015). The Prevalence of peripheral and central hearing impairment and its relation to cognition in older adults. Audiol. Neurotol. 19, 10–14. doi: 10.1159/000371597
Ren, W. J., Liu, Y., Zhou, L. J., Li, W., Zhong, Y., Pang, R. P., et al. (2011). Peripheral nerve injury leads to working memory deficits and dysfunction of the hippocampus by upregulation of TNF-α in rodents. Neuropsychopharmacology 36, 979–992. doi: 10.1038/npp.2010.236
Rigters, S. C., Bos, D., Metselaar, M., Roshchupkin, G. V., Baatenburg de Jong, R. J., Ikram, M. A., et al. (2017). Hearing impairment is associated with smaller brain volume in aging. Front. Aging Neurosci. 9, 2. doi: 10.3389/fnagi.2017.00002
Saji, N. (2021). Association between hearing impairment and cognitive decline: the protective role of hearing aids. Brain Nerve. 73, 907–912. doi: 10.11477/mf.1416201860
Sarant, J., Harris, D., Busby, P., Maruff, P., Schembri, A., Dowell, R., et al. (2019). The effect of cochlear implants on cognitive function in older adults: initial baseline and 18-month follow up results for a prospective international longitudinal study. Front. Neurosci. 13, 789. doi: 10.3389/fnins.2019.00789
Sarant, J., Harris, D., Busby, P., Maruff, P., Schembri, A., Lemke, U., et al. (2020). The effect of hearing aid use on cognition in older adults: can we delay decline or even improve cognitive function? J. Clin. Med. 9, 254. doi: 10.3390/jcm9010254
Sarant, J. Z., Harris, D. C., Busby, P. A., Fowler, C., Fripp, J., Masters, C. L., et al. (2022). No influence of age-related hearing loss on brain Amyloid-β. J. Alz. Dis. 85, 359–367. doi: 10.3233/JAD-215121
Sardone, R., Battista, P., Donghia, R., Lozupone, M., Tortelli, R., Guerra, V., et al. (2020). Age-related central auditory processing disorder, MCI, and dementia in an older population of Southern Italy. Otolaryngol Head Neck. Surg. 163, 348–355. doi: 10.1177/0194599820913635
Segaux, L., Oubaya, N., Broussier, A., Baude, M., Canouï-Poitrine, F., Naga, H., et al. (2019). Identification of five frailty profiles in community-dwelling individuals aged 50–75: a latent class analysis of the SUCCEED survey data. Maturitas 127, 1–11. doi: 10.1016/j.maturitas.2019.05.007
Shen, Y., Hu, H., Fan, C., Wang, Q., Zou, T., Ye, B., et al. (2021). Sensorineural hearing loss may lead to dementia-related pathological changes in hippocampal neurons. Neurobiol. Dis 156, 105408. doi: 10.1016/j.nbd.2021.105408
Sonnet, M., Montaut-Verient, B., Niemier, J., Hoen, M., Ribeyre, L., and Parietti-Winkler, C. (2017). Cognitive abilities and quality of life after cochlear implantation in the elderly. Otol. Neurotol. 38, e296–e301. doi: 10.1097/MAO.0000000000001503
Sorrentino, T., Donati, G., Nassif, N., Pasini, S., and Redaelli de Zinis, L.O. (2020). Cognitive function and quality of life in older adult patients with cochlear implants. Int. J. Audiol. 59, 316–322. doi: 10.1080/14992027.2019.1696993
Spicer, S. S., and Schulte, B. A. (2005). Pathologic changes of presbycusis begin in secondary processes and spread to primary processes of strial marginal cells. Hearing Res. 205, 225. doi: 10.1016/j.heares.2005.03.022
Sugiura, S., Nishita, Y., Uchida, Y., Shimono, M., Suzuki, H., Teranishi, M., et al. (2021). Longitudinal associations between hearing aid usage and cognition in community-dwelling Japanese older adults with moderate hearing loss. PLoS ONE 16, e0258520. doi: 10.1371/journal.pone.0258520
Sugiura, S., Uchida, Y., Nishita, Y., Teranishi, M., Shimono, M., Suzuki, H., et al. (2022). Prevalence of usage of hearing aids and its association with cognitive impairment in Japanese community-dwelling elders with hearing loss. Auris Nasus Larynx. 49, 18–25. doi: 10.1016/j.anl.2021.03.017
Tai, S., Shen, C., Wang, L., and Chien, C. (2021). Association of sudden sensorineural hearing loss with dementia: a nationwide cohort study. BMC Neurol. 21, 88. doi: 10.1186/s12883-021-02106-x
Van Camp, G., and Smith, R.J.H. (2023). Hereditary Hearing Loss Homepage. Available online at: https://hereditaryhearingloss.org (accessed 2023).
Vella Azzopardi, R., Beyer, I., De Raedemaeker, K., Foulon, I., Vermeiren, S., Petrovic, M., et al. (2023). Hearing aid use and gender differences in the auditory-cognitive cascade in the oldest old. Aging Ment. Health 27, 184–192. doi: 10.1080/13607863.2021.2007355
Viana, L. M., O'Malley, J. T., Burgess, B. J., Jones, D. D., Oliveira, C. A. C. P., Santos, F., et al. (2015). Cochlear neuropathy in human presbycusis: Confocal analysis of hidden hearing loss in post-mortem tissue. Hear. Res. 327, 78–88. doi: 10.1016/j.heares.2015.04.014
Vlajkovic, S. M., and Thorne, P. R. (2021). Molecular mechanisms of sensorineural hearing loss and development of inner ear therapeutics. Int. J. Mol. Sci. 22, 5647. doi: 10.3390/ijms22115647
Völter, C., Götze, L, Haubitz, I., Müther, J., Dazert, S., and Thomas, J.P. (2021). Impact of cochlear implantation on neurocognitive subdomains in adult cochlear implant recipients. Audiol. Neurotol. 26, 236–245. doi: 10.1159/000510855
Völter, C., Götze, L., Bajewski, M., Dazert, S., and Thomas, J.P. (2022a). Cognition and cognitive reserve in cochlear implant recipients. Front Aging Neurosci. 14, 838214. doi: 10.3389/fnagi.2022.838214
Völter, C., Götze, L., Kamin, S. T., Haubitz, I., Dazert, S., and Thomas, J.P. (2022b). Can cochlear implantation prevent cognitive decline in the long-term follow-up? Front. Neurol. 13, 1009087. doi: 10.3389/fneur.2022.1009087
Vona, B., Rad, A., and Reisinger, E. (2020). The many faces of DFNB9: relating OTOF variants to hearing impairment. Genes 11, 1411. doi: 10.3390/genes11121411
Wang, S., and Wu, C. (2015). Physiological and histological evaluations of the Cochlea between 3xTg-AD mouse model of Alzheimer's diseases and R6/2 mouse model of Huntington's diseases. Chin. J.Physiol. 58, 359–366. doi: 10.4077/CJP.2015.BAD334
Wang, S., and Wu, C. (2021). Tau phosphorylation and cochlear apoptosis cause hearing loss in 3xTg-AD mouse model of Alzheimer's disease. Chin. J. Physiol. 64, 61. doi: 10.4103/CJP.CJP_79_20
Weible, A. P., Stebritz, A. J., and Wehr, M. (2020). 5XFAD mice show early-onset gap encoding deficits in the auditory cortex. Neurobiol. Aging 94, 101–110. doi: 10.1016/j.neurobiolaging.2020.05.013
Wells, H. R. R., Freidin, M. B., Zainul Abidin, F. N., Payton, A., Dawes, P., Munro, K. J., et al. (2019). GWAS identifies 44 independent associated genomic loci for self-reported adult hearing difficulty in UK Biobank. Am. J. Hum. Genet. 105, 788–802. doi: 10.1016/j.ajhg.2019.09.008
Whiteus, C., Freitas, C., and Grutzendler, J. (2014). Perturbed neural activity disrupts cerebral angiogenesis during a postnatal critical period. Nature 505, 407–11. doi: 10.1038/nature12821
World Health Organisation (2021). Deafness and Hearing Loss. Available online at: https://www.who.int/news-room/fact-sheets/detail/deafness-and-hearing-loss (accessed 2023).
Wu, P. Z., Liberman, L. D., Bennett, K., de Gruttola, V., O'Malley, J. T., and Liberman, M. C. (2019). Primary neural degeneration in the human cochlea: evidence for hidden hearing loss in the aging ear. Neuroscience 407, 8–20. doi: 10.1016/j.neuroscience.2018.07.053
Xu, W., Zhang, C., Li, J., Tan, C., Cao, X., Tan, L, et al. (2019). Age-related hearing loss accelerates cerebrospinal fluid tau levels and brain atrophy: a longitudinal study. Aging 11, 3156. doi: 10.18632/aging.101971
Zhou, Y., Song, J., Wang, Y., Zhang, A., Tan, C., Liu, Y., et al. (2019). Age associated variation in the expression and function of TMEM16A calcium activated chloride channels in the cochlear stria vascularis of guinea pigs. Mol. Med. Rep. 20, 1593–1604. doi: 10.3892/mmr.2019.10423
Keywords: hearing loss, dementia, Alzheimer's disease, hearing aid, cochlear implant, models of hearing loss, cognitive impairment
Citation: Azeem A, Julleekeea A, Knight B, Sohail I, Bruyns-Haylett M and Sastre M (2023) Hearing loss and its link to cognitive impairment and dementia. Front. Dement. 2:1199319. doi: 10.3389/frdem.2023.1199319
Received: 03 April 2023; Accepted: 26 May 2023;
Published: 15 June 2023.
Edited by:
Sabina Tahirovic, Deutsches Zentrum für Neurodegenerative Erkrankungen e. V. (DZNE), GermanyReviewed by:
Christof Haffner, Technical University of Munich, GermanyDavid Geldmacher, University of Alabama at Birmingham, United States
Copyright © 2023 Azeem, Julleekeea, Knight, Sohail, Bruyns-Haylett and Sastre. This is an open-access article distributed under the terms of the Creative Commons Attribution License (CC BY). The use, distribution or reproduction in other forums is permitted, provided the original author(s) and the copyright owner(s) are credited and that the original publication in this journal is cited, in accordance with accepted academic practice. No use, distribution or reproduction is permitted which does not comply with these terms.
*Correspondence: Magdalena Sastre, bS5zYXN0cmVAaW1wZXJpYWwuYWMudWs=
†These authors have contributed equally to this work