- 1Expertise Unit Psychology, Technology & Society, Thomas More University of Applied Sciences, Antwerp, Belgium
- 2Centre for the Psychology of Learning and Experimental Psychopathology, KU Leuven, Leuven, Belgium
- 3Mobilab & Care, Thomas More University of Applied Sciences, Geel, Belgium
New technologies, such as virtual reality (VR) and augmented reality (AR), can be used as an add-on to exposure therapy for common anxiety disorders. Although the benefits of VR for exposure therapy have already been demonstrated extensively in research, AR applications are only just becoming widely available. Evidence for the added value and effectiveness of AR exposure therapy (ARET) is still scarce. The current study aimed to explore whether a first markerless AR iOS app for specific phobia could induce fear for multiple animal species in a general population sample. In two experiments, participants made use of the PHOBOS AR app in a behavioral approach task (BAT), using animals for which they were anxious, but not phobic. Self-report data and physiological measures were recorded. In Experiment 1, 108 participants chose one of the seven available animal species and were allocated to either a smartphone or tablet condition. Results showed increasing levels of self-reported anxiety with increasing levels of BAT difficulty. However, this increase was smaller in individuals reporting low levels of perceived realism. No effects on heart rate (HR) could be established. In Experiment 2, 52 participants were exposed to virtual spiders. For both self-reported anxiety and the interaction with perceived realism, results were similar to those of Experiment 1. Skin conductance did increase significantly from baseline to the highest level of difficulty of the BAT, and the severity of fear of spiders also appeared to be related to the fear response in the BAT. In conclusion, the study shows that animals presented in AR through a mobile device can evoke anxiety, which is a pre-requisite for the implementation of ARET. However, further research should establish the effects of ARET in a clinical sample of people with specific phobias.
Introduction
Specific phobia is a highly prevalent mental illness for which exposure therapy is the first-choice treatment (Baus and Bouchard, 2014; Böhnlein et al., 2020). Specific phobia refers to a persistent unreasonable fear concerning a specific object or situation, which is accompanied by avoidance of the object or situation and results in clinically significant distress or impairment (Eaton et al., 2018). The review of Eaton et al. (2018) shows that specific phobia has a median lifetime prevalence of 7.2%, although large regional differences occur, and that fear of animals and heights are the most common subtypes. During exposure therapy, the client is confronted with stimuli and situations that elicit fear. Gradual, controlled, and hierarchical exposure to fearful stimuli can allow individuals to gain experiences that disprove anxiety beliefs and create new adaptive associations (Krijn et al., 2004; Baus and Bouchard, 2014; Craske et al., 2014). Exposure can be imaginal, in vivo, and more recently also occur in virtual reality (VR) and augmented reality (AR). Although the benefits of VR for exposure therapy have already been shown extensively in research (Krijn et al., 2004; Juan et al., 2005; Powers and Emmelkamp, 2008; Baus and Bouchard, 2014; Botella et al., 2017; Carl et al., 2019), AR applications are only just becoming widely available, and evidence for their added value and effectiveness is still scarce.
AR merges virtual elements into the physical world. This can be useful for exposure therapy in specific phobia, because it allows therapists and clients to project and control a virtual object (often an animal) in the therapist's office. AR exposure therapy (ARET) is an add-on to or type of exposure therapy in which clients can interact with a virtual representation of an object or situation in the actual world to help them reduce fear or avoidance. The client is not immersed in an entire virtual environment through a head-mounted display (HMD) as is the case in VR exposure therapy (VRET), but a virtual object (e.g., a spider) is added to the current surroundings. In ARET, clients can also use HMDs, but this technology additionally allows to make use of projection displays or ubiquitous handheld devices like smartphones or tablets. To effectively visualize an object in the real world, AR can use markers (i.e., stylized real elements on which the software overlays a virtual element) or use a markerless approach where the content is placed relative to the environment, GPS position, and compass of the device. Markerless AR can potentially lead to a higher sense of presence and fear for the virtual element (Juan and Joele, 2011). The level of realism of the virtual elements and the level of reality (or authentic experience) contribute to the user experience in both VR and AR (Baus and Bouchard, 2014). Dünser et al. (2011) go even further and propose an adaptive and interactive AR system where virtual animals respond to the patient's behavior, which could further contribute to the authenticity of the experience.
For virtual animals to be useful for exposure therapy, they should evoke some level of discomfort and anxiety. According to traditional approaches of exposure therapy, such as emotional processing theory (EPT), high anxiety levels are a pre-requisite for effective exposure (Foa and Kozak, 1986). In addition, more recent approaches of exposure therapy based on violating expectancies (e.g., inhibitory learning theory; Craske et al., 2014) emphasize the importance of (sustained) high levels of anxiety and arousal during exposure (e.g., Culver et al., 2012) and learning to tolerate anxiety (Jacoby and Abramowitz, 2016). The activation of a fear structure can potentially be observed in an individual's behavior (e.g., refusing to move closer to the animal), self-reported level of anxiety and distress, and physiology. Anxiety is associated with an increase in skin conductance (SC) and heart rate (HR). HR activity is seen as a contributor to exposure success (Böhnlein et al., 2020).
Previous research has shown that virtual animals can elicit anxiety and be used in ARET, but studies mostly used small sample sizes and were generally limited to the use of HMDs, the use of markers, and the target of cockroaches and spiders (Chicchi Giglioli et al., 2015). The case study of Botella et al. (2005) and the study of Juan et al. (2005) were the first to suggest that ARET, using an HMD, was able to reduce anxious feelings and change behavior, that is, reduce avoidance and make participants able to interact with and kill cockroaches and spiders. Later studies show that the effects of ARET can be maintained up to 1 year follow-up (Botella et al., 2010) and that ARET can achieve similar outcomes as compared with in vivo exposure (Wrzesien et al., 2011). The first larger randomized controlled trial, using an HMD, also showed that both in vivo and AR exposure were effective in individuals with spider or cockroach phobia (Botella et al., 2016). HMDs can limit visual awareness and contact with the therapist; therefore, Wrzesien et al. (2013) developed projection-based AR and showed that exposure to projected animals could reduce anxiety for cockroaches in a non-clinical sample. The first smartphone-based AR application for phobia was a serious game for cockroach exposure. Botella et al. (2011) designed a puzzle game with virtual cockroaches, which could be implemented as homework between AR exposure sessions. The first results of this case study are promising. More flexible use of AR for exposure seems possible by relying on ubiquitous handheld devices (with a much lower cost than HMDs or projection displays).
Suso-Ribera et al. (2019) performed an analysis on aggregated data, which suggests that VRET, ARET, and in vivo exposure are equally effective and that outcomes are independent of patient characteristics. However, advantages of VRET and ARET over exposure in vivo include practical benefits, potential for repetition, and acceptability, including a lower refusal rate (Garcia-Palacios et al., 2007; Baus and Bouchard, 2014). The most important strength of ARET and VRET is the increased control you can have over a virtual environment, as opposed to a real-life context. Therapists can easily manipulate the precise circumstances and switch from a small animal to its bigger version, from a non-threatening stance to a more aggressive posture in an animal, or from a stationary to a moving animal. A large study in individuals with spider phobia showed that the locomotion pattern was the primary fear association of spiders (Lindner et al., 2019). Additionally, moving, as opposed to stationary, animals are associated with increased fear and effectiveness of ARET (Bretón-López et al., 2010). ARET can also facilitate executing exposure in multiple contexts, which could attenuate the risk of renewal of fear (Bandarian-Balooch et al., 2015).
The current study aims to explore the potential of an AR smartphone application to elicit fear of virtual animals. This application is a first markerless AR app for a handheld device that includes specimens of multiple different species (insects, reptiles, birds, and mammals). In line with EPT, virtual animals need to evoke some level of discomfort and anxiety to be useful for exposure therapy. Therefore, the current study evaluates whether projecting virtual animals in the real environment through AR is associated with anxiety in a non-clinical sample. Although the main focus is on self-reported anxiety, physiological data are included in exploratory analyses. The current study also investigates the effects of experienced realism and means of delivery (screen size) on self-reported anxiety. The study consists of two experiments that rely on behavioral approach tasks (BATs). Whereas the first experiment uses a broad array of animals and explores the effect of screen size, the follow-up experiment is tailored to exposure to spiders.
Experiment 1
Participants selected one animal for which they experienced non-phobic discomfort and executed a BAT. The goals of this experiment were to investigate to what extent different virtual animals could evoke feelings of discomfort or anxiety and assess the impact of screen size and realism for AR exposure.
Materials and Methods
Sample
First-year applied psychology students at Thomas More University of Applied Sciences were invited for participation in the study through e-mail. After receiving information about the study in the informed consent and providing their agreement for participation, they were asked to “choose an animal that you feel somewhat uncomfortable with, but for which you do not consider yourself phobic” and to which they wanted to be exposed in AR. To be eligible, their self-reported discomfort or fear should not exceed phobic levels in the Severity Measure for Specific Phobia-Adult (SMSP-A) (Craske et al., 2013). A total of 108 students participated in the experiment (Figure 1). The current study opted to limit the scope to a healthy sample because the implemented app has not yet been tested or validated in research, nor have other similar smartphone approaches for ARET (to our knowledge). In line with the cautionary principle, we did not want to induce undue distress in patients with anxiety disorders at this stage if the research questions could be answered using a healthy population. All participants received course credits for participation and provided informed consent. The study was approved by the Ethical Committee of Thomas More University of Applied Sciences.
Virtual Rendering of Animals
The commercially available iOS PHOBOS AR app (Tarnogol, 2018) was used to add virtual animals to the real world. This markerless AR app contains virtual rats, pigeons, snakes, cockroaches, spiders, frogs, and dogs. Visualizations can range from schematic to realistic. Animals can be placed on a preferred location in the users' environment and can perform certain actions, such as moving or making noise. In the current study, the PHOBOS AR app was used on both a smartphone (iPhone 8; 5.5-inch display) and a tablet (iPad; 9.7-inch display) to assess whether screen size influenced the effects.
Behavioral Approach Task
A BAT was used to assess to what extent exposure to animals through AR elicited anxiety. The outcome of interest was reported anxiety during each of the steps. Because participants were non-phobic, all participants completed all steps of the BAT. Therefore, the number of steps is not included as an outcome measure. Participants selected an animal for the BAT that evoked some subjective discomfort and they wanted to be exposed to. Nevertheless, participants were only exposed to the animal of their choice, when their anxiety scores were considered equal or below mild (≤10) on the SMSP-A (Craske et al., 2013). When the scores of participants exceeded the cutoff for mild anxiety (N = 37), they were requested to choose a different animal. This process was repeated until an animal and a corresponding fear were found to be meet this criterion. Twenty-seven participants were given their second choice and 10 participants their third.
Three subtasks with three increasing levels of difficulty were used. In line with Bretón-López et al. (2010), moving animals had a higher difficulty level than stationary animals. Participants could run through each phase at their own pace and were asked to report their anxiety level in Subjective Units of Distress (SUDs; Tanner, 2012) on a scale of 0 (“free of anxiety or tension”) to 10 (“extremely anxious or tense”) immediately at the end of each step. Depending on the nature of the animal, three phases with each three levels of increasing difficulty were designed. For example, the BAT for a dog consisted of first placing the animal 6 m in front of them (level 1), the participant moving 2 m closer to the animal (level 2), and coming to 2 m distance of the animal (level 3). Crosses of red tape on the gray concrete floor indicated the different levels of the BATs. The second phase consisted of the same three levels, but this time, the dog was also barking at the participant. In the third phase, participants had to make the animal walk toward them across all three levels. BATs with smaller animals were presented on a table setup, while participants were seated and moved their hand closer to the animal. The BAT for a spider, for example, consisted of the following three phases. First, a spider was projected at three decreasing distances on the table (level 1), which was followed by projecting a spider at a fixed position and letting participants move their hand increasingly closer (level 2) and, finally, letting a spider gradually move toward them (level 3). The spider used in the current experiment is Spider 3 of Experiment 2. All steps of the BAT were executed using virtual animals in AR, and no real animals were presented to participants.
Physiological Measurement
The naturalistic and flexible approach of the AR paradigm required mobile and non-invasive physiological measurement, which can be achieved through wearable monitoring. Electrocardiogram (ECG) measurement has shown higher accuracy as compared to wrist-worn devices (e.g., Etiwy et al., 2019), so Byteflies sensor dots (Byteflies NV, Belgium) were used to capture HR through ECG measurement because they provide a qualitative ECG signal with an adequate sampling frequency of 250 Hz. ECG was measured using a two-lead wired ECG patch and disposable electrodes. One patch was placed on the top of the sternum, whereas the other was placed below the left clavicle (from the participants' perspective). HR is calculated based on the detection of the rising edge of the R-peak regarding the QRS complex. As the ECG signal is of good quality, we are able to use simple signal processing steps in order to obtain a reliable HR. This processing consists of a median filter (a fifth-order one-dimensional filter) to remove spikes. Afterwards, a difference equation operation is applied on the signal in order to ease the detection of the most prominent peaks, which are the R-waves. This method was described by Yeh and Wang (2008) as part of the difference operation method. These local maxima are then determined based on the minimal peak height, the minimal peak distance, and the minimal peak prominence. Out of these local maxima, the HR is calculated using a sliding window of 60 s and a step count of 250 samples, which corresponds to 1 s. Finally, the calculated HR (beats/min) is smoothened by a moving average filter (filter length 10) to reduce artifacts.
Questionnaires
Severity measure for specific phobia—adult
After participants selected an animal, they completed the SMSP-A (Craske et al., 2013). In this questionnaire, participants rate the severity of phobic symptoms toward the animal during the past seven days. Ten items are answered on a scale of 0 (“never”) to 4 (“all of the time”). Participants were only exposed to the animal if their anxiety scores were equal or below mild (≤10) on the SMSP-A. Cronbach's alpha (α) in the current experiment was high (0.85).
Unified theory of acceptance and use of technology questionnaire
Participants' attitude toward e-mental health was measured with a questionnaire based on Ebert et al. (2015) and the unified theory of acceptance and use of technology (UTAUT) model (Venkatesh et al., 2003). The Dutch qualitative questionnaire contains the following scales: performance expectancy (Cronbach's α = 0.86), effort expectancy (Cronbach's α = 0.56), social influence (Cronbach's α = 0.56), facilitating conditions (Cronbach's α = 0.62), fear, trust in data security, and knowledge about internet interventions (De Witte and Van Daele, 2017). Scores range from 1 (“do not agree at all”) to 5 (“completely agree”), and means are calculated per scale. Higher scores reflect positive attitudes, except for the fear scale, where they reflect increased fear. Reliability was calculated in the current sample, but coefficients of subscales with two items were not calculated because they are an underestimation of true reliability (Eisinga et al., 2013).
The profile of mood states
Participants were asked to report their current mood in the Profile of Mood States (POMS) questionnaire (McNair et al., 1992). The Dutch 32-item questionnaire contains five scales, that is, tension–anxiety, depression, anger, vigor, and fatigue (Wald and Mellenbergh, 1990). The current study only reports the tension–anxiety subscale (Cronbach's α of 0.86 in the current sample).
The Igroup presence questionnaire
The Igroup Presence Questionnaire (IPQ; Regenbrecht and Schubert, 2002) contains information on general presence, spatial presence, involvement, and experienced realism. Because presence might not be as important for AR as compared to VR, whereas realism is still very important (Baus and Bouchard, 2014), the current study focused only on realism (Cronbach's α = 0.64).
Procedure
Students of applied psychology collected the data, under supervision of a clinical psychologist (TVD), in the context of their bachelor dissertations. Prior to the testing day, participants had to fill in an online survey, consisting of the UTAUT questionnaire, Big Five Inventory (BFI), and SMSP-A. This survey could be completed at home, whereas the remainder of the experiment took place on the campus of Thomas More University of Applied Sciences. On the day of testing, participants were allocated at random to either the iPhone (N = 55) or iPad (N = 56) condition. Approximately half of the sample in each group was randomly assigned to be fitted with the Byteflies ECG sensors (see also Figure 1). Immediately before the BAT, participants completed the POMS. After participants executed the BAT, participants completed the POMS again and filled in the IPQ. Participants were subsequently allowed to have a further look at the PHOBOS AR app. A debriefing was given through a collective oral presentation in which the general concepts, purpose, and results of the study were explained.
Data Reduction and Statistical Analysis
A one-way analysis of variance (ANOVA) assessed whether realism scores differed between the different animals. To assess whether the increasing levels of difficulty in the BAT were associated with increased self-reported anxiety in the SUDs, three repeated measures ANOVAs were executed (one for each phase). Changes from pre-test to post-test in POMS and HR were also analyzed using repeated measures ANOVAs. The selected animals, screen size, and IPQ realism were consistently added as between-subjects factors. Low, medium, and high perceived realism categories were defined by subtracting and adding half a standard deviation to the mean IPQ realism score. A paired samples t-test was used to establish whether reported anxiety during the highest difficulty level (BAT phase 3 level 3) was significantly different from reported anxiety upon first confrontation with the virtual animal (BAT phase 1 level 1).
Results
Descriptive Statistics
Of the 108 participants who participated, 25 were male, 82 were female, and 1 person identified as non-binary. Age ranged between 17 and 40, with a mean of 19.02 (SD = 3.10). Table 1 provides an overview of the POMS, experienced realism, and UTAUT scores of the sample. Participants generally had the means and support to use e-mental health applications (as indicated by the facilitating conditions scale); other average UTAUT scores were mediocre. Most participants selected the spider (N = 34), followed by the snake (N = 25), the cockroach (N = 18), the mouse (N = 12), the dog (N = 9), the frog (N = 5), and the pigeon (N = 5). A one-way ANOVA shows that perceived realism does not differ significantly between selected animals, F(6, 97) = 1.22, p = 0.31.
Results of the Behavioral Approach Task
SUDs increased significantly in the three BAT phases. A repeated measures ANOVA with a Greenhouse–Geisser correction determined that SUDs differed significantly between the difficulty levels of the first, F(1.34, 93.51) = 80.9803, p < 0.001, second, F(1.37, 94.73) = 99.99, p < 0.001, and third, F(1.40, 96.33) = 83.42, p < 0.001, phases of the BAT. However, there is also a significant interaction with perceived realism in phase 1, F(2.67, 93.51) = 4.77, p = 0.005, phase 2, F(2.75, 94.73) = 3.60, p = 0.02, and phase 3, F(2.79, 96.33) = 3.98, p = 0.01. Figure 2 shows the evolution in BAT scores throughout the different phases and the effect of realism. Individuals who reported lower realism show lower SUDs and a less pronounced increase in SUDs throughout the BATs. There was no interaction between SUDs scores and screen size or choice of animal in any of the BAT phases. A paired samples t-test shows that SUDs are significantly higher during the most difficult level (SUDs phase 3 level 3; M = 4.50, SD = 2.87) as compared with during initial exposure (SUDs phase 1 level 1; M = 0.95, SD = 1.28), t(107) = 16.07, p < 0.001. A repeated measures ANOVA showed that the POMS anxiety score slightly decreased from pre-test to post-test, F(1, 69) = 7.39, p = 0.008, but there was an interaction with the selected animal, F(1, 69) = 2.27, p = 0.05. For most animals, no difference or a small increase in POMS scores was found, except for participants exposed to mice (N = 12) and frogs (N = 5), for whom POMS scores on average decreased with 2.42 and 3.88, respectively. Given the small sample sizes in these subgroups, no formal follow-up analyses were performed. HR did not change from pre-test to post-test, F(1, 12) = 0.01, p = 0.93.
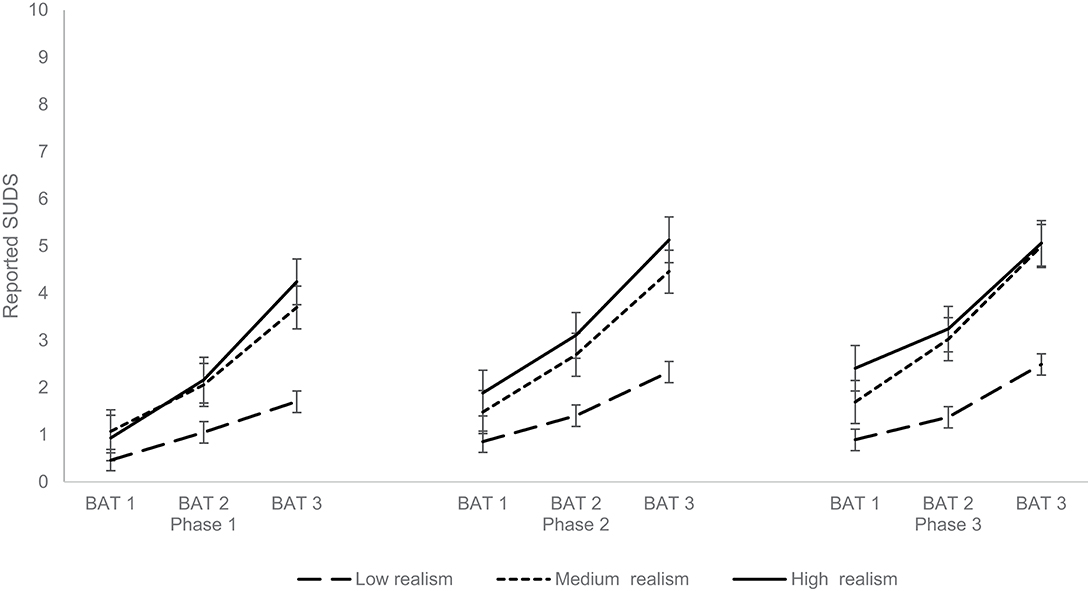
Figure 2. Results of the three main ANOVAs of Experiment 1. Mean reported Subjective Units of Distress during each of the three difficulty levels of each of the three phases of the behavioral approach task. The different lines represent low (N = 32), medium (N = 42), and high (N = 30) perceived realism.
Experiment 2
The second experiment applied a more standardized exposure paradigm with fixed exposure times and continuously increasing levels of difficulty, specifically tailored to spiders (which were the most commonly selected animal in Experiment 1). Experiment 2 also investigated the association between severity of spider anxiety and BAT outcomes. The exploratory physiological measurement was extended to include SC.
Materials and Methods
Sample
Fifty-two first-year applied psychology students at Thomas More University of Applied Sciences participated in this experiment after being invited through e-mail (Figure 3). Inclusion criteria consisted of reported discomfort, but not phobic levels of fear, to spiders in the SMSP-A (Craske et al., 2013). Eleven participants surpassing a moderate level of anxiety for spiders (≥25) in this questionnaire were excluded. All participants received course credits for participation and provided informed consent. The study was approved by the Ethical Committee of Thomas More University of Applied Sciences.
Virtual Rendering of Animals
Because screen size did not influence the results of Experiment 1, all individuals used the iOS PHOBOS AR app on an iPhone 8. The Materials and Methods section of Experiment 1 provides a description of this app.
Behavioral Approach Task
As opposed to the nine-step BAT with three separate phases of Experiment 1, Experiment 2 opted for a consistent buildup of anxiety in a six-step continuous BAT for spiders. Different types and visualizations of spiders were used (Figure 4). Consecutive difficulty levels in the BAT were based on both the hypothesized fearfulness of a spider and the exposure circumstances (e.g., distance and movement). The following six steps were included in the BAT: (1) participants were standing in front of a table, and Spider 1 was placed stationary on that table at 1 m distance from the table edge; (2) participants placed their hand at 25 cm distance from the table edge, and Spider 1 was placed stationary at 75 cm distance from table edge; (3) participants placed their hand at 50 cm distance from table edge, and Spider 2 was placed stationary at 75 cm distance from the table edge; (4) participants were standing in front of a table, and Spider 2 crawled from 1 m to 50 cm distance from the table edge; (5) participants were standing in front of a table, and Spider 3 crawled from 50 to 15 cm distance from the table edge; (6) participants placed their hand at 25 cm distance from the table edge, and Spider 4 crawled from 1 m distance from the table edge onto the hand of the participant. Each step took 30 s, followed by a 30 s break. A fixed duration was used because some participants went through the different steps of Experiment 1 very quickly, which could reflect avoidance or reduce the impact of exposure to an animal. During each step, participants reported SUDs on a scale from 0 (“free of anxiety or tension”) to 10 (“extremely anxious or tense”). All steps of the BAT were executed using virtual animals in AR, and no real animals were presented to participants. Participants were asked to rank the spiders according to evoked fear after the BAT.
Physiological Measurement
The Empatica E4 was worn on the wrist and calculated both HR and SC. Whereas HR measured at the wrist is highly similar to HR measured using stationary devices, SC measurement appears to show limited agreement between wearable and stationary monitoring, possibly owing to electrode placement, sampling frequency, and the presence of fewer sweat glands resulting in lower responsiveness of the signal (Menghini et al., 2019; Konstantinou et al., 2020). However, despite low correlation between SC signals from Empatica E4 and stationary measurement, the wearable SC signal is found to be usable at parameter level and even shows good sensitivity to emotional stress (Ollander et al., 2017; Menghini et al., 2019; van Lier et al., 2019). The Empatica E4 device measures the blood volume pulse (BVP) signal using green and red LED technologies at a sampling rate of 64 Hz. SC is measured using two silver (Ag) electrodes at the inner wrist and sampled at 4 Hz. Data were measured continuously after a settling in period of 5 min. For the exploratory physiological analyses, a subsample of 42 individuals could be included. SC was filtered using a low pass filter with a cutoff frequency of 0.4 Hz (Sano et al., 2018). For BVP, a bandpass filter with cutoff frequencies of 15 and 240 bpm were used, followed by a median filter (filter length 7) and a mean filter (filter length 5) to remove noise. HR was subsequently calculated based on this filtered signal. Finally, both signals were analyzed using a 60 s sliding window.
Participants needed to wear the wearable device for at least 5 min before the task to exclude effects relating to settling in (which is especially relevant for SC). A comparison was made between SC and HR during the 60 s before the start of the task and the last 60 s of step 6, which is the moment with the highest level of difficulty.
Questionnaires
Similar to Experiment 1, the SMSP-A, UTAUT questionnaire, BFI, POMS, and IPQ were used. The following questionnaires were added.
Fear of spiders questionnaire
The Fear of Spiders Questionnaire (FSQ; Szymanski and Donohue, 1995) measures self-reported fear of spiders. The Dutch translation was used (Muris and Merckelbach, 1996) and showed high reliability (Cronbach's α = 0.96).
Circumscribed fear measure
The Dutch version of the Circumscribed Fear Measure (CFM_NL) is a flexible trans-stimulus fear measure, based on five components: risk analysis, physiological symptoms, fear/anxiety, escape/avoidance, and control (McCraw and Valentiner, 2015). Participants were asked to complete the CFM_NL for spiders. Reliability in the current sample was high (Cronbach's α = 0.95).
Procedure
Students of applied psychology collected the data, under supervision of a clinical psychologist (TVD), in the context of their bachelor dissertations. Prior to the testing day, participants had to complete the informed consent and fill in an online survey, consisting of the UTAUT questionnaire, BFI, CFM_NL, FSQ, and SMSP-A. This survey could be completed at home whereas the remainder of the experiment took place on campus. On the day of testing, participants were first given the Empatica E4 wearable so that there was enough time to settle in and physiological habituation effects did not influence the measurements during the BAT. Subsequently, participants completed the POMS and started the BAT. After participants executed the six-step BAT and reported the SUD scores, participants completed the POMS again, filled in the IPQ, and provided some demographic information. As a next step, participants were asked to rank the four spiders they were presented with in the task, according to how much fear they evoked. After all data were collected, a debriefing was given through an oral presentation in which the general concepts, purpose, and results of the experiment were explained.
Data Reduction and Statistical Analysis
A repeated measures ANOVA was used to assess whether the increasing levels of difficulty in the BAT were associated with increased self-reported discomfort in the SUDs. Changes from pre-test to post-test in POMS, SC, and HR were also analyzed using repeated measures ANOVAs. Perceived realism was consistently added as between-subjects factors. The ranking of the spiders was analyzed using a Friedman's ANOVA. Two-tailed Pearson correlations were used to investigate the association between the total scores of the CFM_NL and FSQ and the BAT outcomes.
Results
Descriptive Statistics
A sample of 40 women and 12 men were recruited with a mean age of 18.63 (SD = 1.05). Table 2 provides an overview of descriptive statistics. Participants generally had the means and support to use e-mental health applications (as indicated by the facilitating conditions scale); other average UTAUT scores are within a mediocre range.
Results of the Behavioral Approach Task
A repeated measures ANOVA (with Greenhouse–Geisser correction) showed that there was a significant increase in SUDs throughout the different steps of the BAT, F(2.07, 101.51) = 74.69, p < 0.001. The contrast between BAT 6 and BAT 1 was significant, F(1, 49) = 112.08, p < 0.001. Similar to Experiment 1, reported SUDs interacted significantly with perceived realism, F(4.14, 101.51) = 5.39, p < 0.001. The reported SUDs and different trajectories depending on perceived realism are shown in Figure 5. Although there was no main effect in the repeated measures ANOVA on the POMS anxiety score, F(1, 48) = 1.21, p = 0.28, there was a crossover interaction, F(2, 48) = 5.01, p = 0.01. Visual inspection of the data showed that while participants who perceived high realism showed a small increase in POMS score, the other two groups showed a decrease. However, one should be careful with interpreting these effects, given the small sample sizes in the three groups (N = 17, N = 13, and N = 22). Although HR did not change, F(1, 38) = 0.21, p = 0.65, SC did significantly increase from pre-BAT to post-BAT, F(1, 38) = 8.65, p = 0.006. Perceived realism did not interact with psychophysiology.
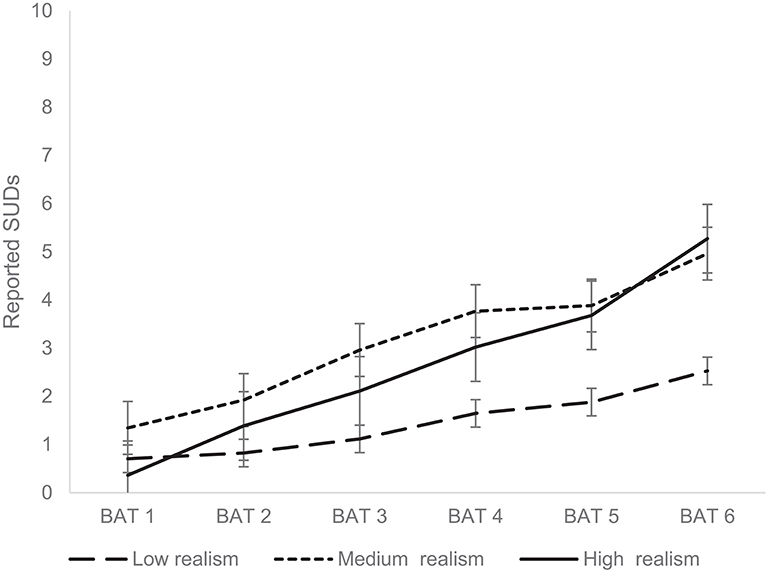
Figure 5. Results of the main repeated measures ANOVA of Experiment 2. Mean reported Subjective Units of Distress during the subsequent difficulty levels of the behavioral approach task. The different lines represent low (N = 17), medium (N = 13), and high (N = 22) perceived realism.
Spider Ranking
Spiders were ranked according to self-reported fear with significant differences reported across spiders, χ2(52) = 44.03, p < 0.001. Spider 1 was considered the least fear inducing (M = 1.65, SD = 0.79), followed by Spider 4 (M = 2.23, SD = 1.21). Spider 2 (M = 3.04, SD = 0.99) and Spider 3 were ranked as the most fear inducing (M = 3.08, SD = 0.76).
Associations Between Spider Anxiety and Behavioral Approach Task Outcomes
Both CFM_NL and FSQ correlated significantly with initial self-reported anxiety toward the virtual animal, anxiety during peak exposure, and change in anxiety during the BAT (Table 3).
General Discussion
The current study aimed to explore the potential of an AR app for inducing fear for animals in a general sample because the elicitation of fear of virtual animals is an important pre-requisite for the effectiveness of ARET. Two experiments showed that increasing levels of difficulty of exposure to various virtual animals in a BAT were associated with increases in self-reported anxiety. While screen size did not influence results, individual differences such as fear of spiders and experienced realism could influence the level of evoked discomfort. BAT effects were stronger in individuals who report higher levels of perceived realism. The elicitation of fear by the BAT was supported by the observed increase in SC from baseline to peak exposure. However, no increase in HR was recorded.
Increasing levels of difficulty of the BAT were associated with increasing levels of self-reported anxiety, and this was the case for different animal species. Moving closer toward virtual animals or experiencing a change in stance and behavior of an animal did evoke anxiety. In line with Bretón-López et al. (2010), virtual animals in locomotion elicited more anxiety than stationary animals. In line with the assumptions of Baus and Bouchard (2014), perceived realism had an important influence on the effect of AR. Individuals who experienced a relatively low level of realism of the animal did not increase in anxiety to the same extent as the other participants in both experiments. The current study did not observe significant differences in perceived realism between different virtual animals. This suggests that how realistic a certain virtual animal is not dependent on the type of the animal but lies in the eye of the beholder. Nevertheless, a more schematic representation of a spider was ranked lower in terms of fear than a realistic version of the same spider. Although the general sample of both experiments showed an increase in anxiety throughout the task, Experiment 2 showed highly significant correlations between spider anxiety (assessed with a questionnaire) and an individual's initial fear response to a virtual spider, fear at the most difficult exposure level, and the increase in anxiety during exposure.
The elicitation of anxiety by the virtual elements was also partially supported by the exploratory physiological analyses. It is important to note that two lines of inquiry are present in the discourse on physiological correlates of exposure therapy (e.g., Craske et al., 2008; Diemer et al., 2014): (1) whether exposure can effectively pose an immediate challenge to the fear system and (2) whether exposure results in habituation and according reductions in anxious arousal over time owing to treatment success. The current study investigates increases in anxiety and physiological activation, in accordance with the first research line. The review of Diemer et al. (2014) shows that exposure in VR implicates a physiological challenge, with increased SC, in both clinical patients and healthy controls, and suggests that SC is more sensitive than HR to the effects of exposure. Although only one (out of 39) of the included studies of this review focused on animal phobia, the current study corroborates these findings by showing that virtual spiders in AR challenged the stress system as shown by an increase in SC from baseline to peak exposure. No differences in HR were documented in the experiments. This could be explained by the fact that SC is strongly rooted in the sympathetic nervous system, which is responsible for fear-based arousal. On the other hand, HR is influenced by an interaction between many different biological pathways. The role of changes in HR during exposure therapy, both in the context of initial fear activation and within-session habituation, has been investigated by multiple studies with varying results (Craske et al., 2008). Overall, it seems that clinical improvements can occur independent of HR changes.
In line with EPT (Foa and Kozak, 1986), the current mobile AR application shows potential to be used for exposure therapy. Nevertheless, further research should establish whether the current effects translate to a clinical sample and whether ARET using mobile devices could potentially be a useful add-on treatment option for simple phobia, next to VRET and in vivo exposure therapy. Using ubiquitous mobile devices, which should have sufficient memory capacity and graphic capabilities, could allow for easy implementation in clinical practice. It is also relevant in this respect that screen size, whether a smartphone or tablet was used, did not influence the results. Mobile devices (especially markerless ones) also allow the therapist to perform exposure in different naturalistic contexts or use homework assignments, which can contribute to fear elicitation and reduce the risk of renewal after treatment (Juan and Joele, 2011; Bandarian-Balooch et al., 2015). The current study points to several potentially useful features of applications for ARET. Being able to offer multiple animals within one application leads to a substantially bigger target population. Providing several tailoring options for each animal, that is, presenting them in a schematic and realistic representation, static or in locomotion, or performing certain behaviors that may be experienced as threatening (e.g., barking), allow therapists to offer ARET in a very controlled and flexible way that permits a gradual buildup of anxiety.
The current study has several limitations. Findings in the general population cannot be directly transferred to phobic individuals and the exclusion of participants with elevated anxiety results in a more restrictive range of affective responses. Participants were not screened for depressive or anxiety symptoms, other than specific phobia in relation to the selected animal. Because research on AR exposure to fearful stimuli is limited, it is important to first monitor the effects in a population with low levels of fear (cf. precautionary principle), but future research should explore the effects of ARET in a clinical sample. The current study also did not include a comparison with in vivo exposure. Future research should ascertain whether ARET is sufficiently effective as a stand-alone intervention or should be combined with in vivo exposure to achieve the desired effects. The current markerless app scanned the surroundings before a virtual animal was presented. This could sometimes take time and incorrect placement did sometimes still ensue, with, for example, animals walking in the wrong direction. These factors contributed to lower realism and anxiety scores. The sample sizes of the different realism categories were also very small in Experiment 2.
In conclusion, animals presented in AR through a mobile device with increasing levels of difficulty can evoke anxiety in a non-clinical sample. Whereas the general sample of the current study showed increasing levels of anxiety with increasingly levels of difficulty in the BAT (irrespective of animal species), anxiety was lower in individuals who experienced low realism of animals. There were also significant associations between the severity of anxiety, as measured by stimulus-specific questionnaires and the experienced distress in the task. The potential of virtual animals to evoke a fear response is a pre-requisite for the implementation of ARET. However, further research should establish the effects of ARET in a clinical sample.
Data Availability Statement
The raw data supporting the conclusions of this article will be made available by the authors to any qualified researcher, without undue reservation.
Ethics Statement
The studies involving human participants were reviewed and approved by the Ethical Committee of Thomas More University of Applied Sciences. The participants provided their written informed consent to participate in this study.
Author Contributions
TV and ND designed the study, conducted the experiments, and analyzed the results. ND wrote the first draft of the paper. TV and SS also contributed to the writing. SS and DH reviewed the study design and the manuscript. RS and GD analyzed the physiological parameters and reviewed the manuscript. All authors contributed to the article and approved the submitted version.
Funding
This research was partially funded by KU Leuven project C16/19/02. Funding for open access fees was received from Thomas More University of Applied Sciences and KU Leuven.
Conflict of Interest
The authors declare that the research was conducted in the absence of any commercial or financial relationships that could be construed as a potential conflict of interest.
References
Bandarian-Balooch, S., Neumann, D. L., and Boschen, M. J. (2015). Exposure treatment in multiple contexts attenuates return of fear via renewal in high spider fearful individuals. J. Behav. Ther. Exp. Psychiatry 47, 138–144. doi: 10.1016/j.jbtep.2014.12.006
Baus, O., and Bouchard, S. (2014). Moving from virtual reality exposure-based therapy to augmented reality exposure-based therapy: a review. Front. Hum. Neurosci. 8:112. doi: 10.3389/fnhum.2014.00112
Böhnlein, J., Altegoer, L., Muck, N. K., Roesmann, K., Redlich, R., Dannlowski, U., et al. (2020). Factors influencing the success of exposure therapy for specific phobia: a systematic review. Neurosci. Biobehav. Rev. 108, 796–820. doi: 10.1016/j.neubiorev.2019.12.009
Botella, C., Bretón-López, J., Quero, S., Baños, R., and García-Palacios, A. (2010). Treating cockroach phobia with augmented reality. Behav. Ther. 41, 401–413. doi: 10.1016/j.beth.2009.07.002
Botella, C., Breton-López, J., Quero, S., Baños, R. M., García-Palacios, A., Zaragoza, I., et al. (2011). Treating cockroach phobia using a serious game on a mobile phone and augmented reality exposure: a single case study. Comput. Human Behav. 27, 217–227. doi: 10.1016/j.chb.2010.07.043
Botella, C., Fernández-Álvarez, J., Guillén, V., García-Palacios, A., and Baños, R. (2017). Recent progress in virtual reality exposure therapy for phobias: a systematic review. Curr. Psychiatry Rep. 19:42. doi: 10.1007/s11920-017-0788-4
Botella, C., Pérez-Ara, M. Á., Bretón-López, J., Quero, S., García-Palacios, A., and Baños, R. M. (2016). In vivo versus augmented reality exposure in the treatment of small animal phobia: a randomized controlled trial. PLoS ONE 11:e0148237. doi: 10.1371/journal.pone.0148237
Botella, C. M., Juan, M. C., Baños, R. M., Alcañiz, M., Guillén, V., and Rey, B. (2005). Mixing realities? An application of augmented reality for the treatment of cockroach phobia. Cyberpsychol. Behav. 8, 162–171. doi: 10.1089/cpb.2005.8.162
Bretón-López, J., Quero, S., Botella, C., García-Palacios, A., Baños, R. M., and Alcañiz, M. (2010). An augmented reality system validation for the treatment of cockroach phobia. Cyberpsychol. Behav. Soc. Netw. 13, 705–710. doi: 10.1089/cyber.2009.0170
Carl, E., Stein, A. T., Levihn-Coon, A., Pogue, J. R., Rothbaum, B., Emmelkamp, P., et al. (2019). Virtual reality exposure therapy for anxiety and related disorders: a meta-analysis of randomized controlled trials. J. Anxiety Disord. 61, 27–36. doi: 10.1016/j.janxdis.2018.08.003
Chicchi Giglioli, I. A., Pallavicini, F., Pedroli, E., Serino, S., and Riva, G. (2015). Augmented reality: a brand new challenge for the assessment and treatment of psychological disorders. Comput. Math. Methods Med. 2015:862942. doi: 10.1155/2015/862942
Craske, M., Treanor, M., Conway, C., Zbozinek, T., and Vervliet, B. (2014). Maximizing exposure therapy: an inhibitory learning approach. Behav. Res. Ther. 58, 10–23. doi: 10.1016/j.brat.2014.04.006
Craske, M., Wittchen, U., Stein, M., Andrews, G., and Lebeu, R. (2013). Severity Measure for Specific Phobia—Adult. Washington, DC: American Psychiatric Association.
Craske, M. G., Kircanski, K., Zelikowsky, M., Mystkowski, J., Chowdhury, N., and Baker, A. (2008). Optimizing inhibitory learning during exposure therapy. Behav. Res. Ther. 46, 5–27. doi: 10.1016/j.brat.2007.10.003
Culver, N. C., Stoyanova, M., and Craske, M. G. (2012). Emotional variability and sustained arousal during exposure. J. Behav. Ther. Exp. Psychiatry 43, 787–793. doi: 10.1016/j.jbtep.2011.10.009
De Witte, N. A. J., and Van Daele, T. (2017). Vlaamse UTAUT-Vragenlijsten [Flemish UTAUT-Questionnaires]. Antwerp: Applied Psychology, Thomas More University of Applied Sciences.
Diemer, J., Mühlberger, A., Pauli, P., and Zwanzger, P. (2014). Virtual reality exposure in anxiety disorders: impact on psychophysiological reactivity. World J. Biol. Psychiatry 15, 427–442. doi: 10.3109/15622975.2014.892632
Dünser, A., Grasset, R., and Farrant, H. (2011). “Towards immersive and adaptive augmented reality exposure treatment,” in Annual Review of Cybertherapy and Telemedicine 2011, eds B. K. Wiederhold, S. Bouchard, and S. Riva (San Diego, CA: Interactive Media Institute), 31–34.
Eaton, W. W., Bienvenu, O. J., and Miloyan, B. (2018). Specific phobias. Lancet Psychiatry 5, 678–686. doi: 10.1016/S2215-0366(18)30169-X
Ebert, D. D., Berking, M., Cuijpers, P., Lehr, D., Pörtner, M., and Baumeister, H. (2015). Increasing the acceptance of internet-based mental health interventions in primary care patients with depressive symptoms. A randomized controlled trial. J. Affect. Disord. 176, 9–17. doi: 10.1016/j.jad.2015.01.056
Eisinga, R., te Grotenhuis, M., and Pelzer, B. (2013). The reliability of a two-item scale: Pearson, Cronbach, or Spearman-Brown? Int. J. Public Health 58, 637–642. doi: 10.1007/s00038-012-0416-3
Etiwy, M., Akhrass, Z., Gillinov, L., Alashi, A., Wang, R., Blackburn, G., et al. (2019). Accuracy of wearable heart rate monitors in cardiac rehabilitation. Cardiovasc. Diagn. Ther. 9, 262–271. doi: 10.21037/cdt.2019.04.08
Foa, E. B., and Kozak, M. J. (1986). Emotional processing of fear. Exposure to corrective information. Psychol. Bull. 99, 20–35. doi: 10.1037/0033-2909.99.1.20
Garcia-Palacios, A., Botella, C., Hoffman, H., and Fabregat, S. (2007). Comparing acceptance and refusal rates of virtual reality exposure vs. in vivo exposure by patients with specific phobias. Cyberpsychol. Behav. 10, 722–724. doi: 10.1089/cpb.2007.9962
Jacoby, R. J., and Abramowitz, J. S. (2016). Inhibitory learning approaches to exposure therapy: a critical review and translation to obsessive-compulsive disorder. Clin. Psychol. Rev. 49, 28–40. doi: 10.1016/j.cpr.2016.07.001
Juan, M. C., Alcañiz, M., Monserrat, C., Botella, C., Baños, R. M., and Guerrero, B. (2005). Using augmented reality to treat phobias. IEEE Comput. Graph. Appl. 25, 31–37. doi: 10.1109/MCG.2005.143
Juan, M. C., and Joele, D. (2011). A comparative study of the sense of presence and anxiety in an invisible marker versus a marker augmented reality system for the treatment of phobia towards small animals. Int. J. Hum. Comput. Stud. 69, 440–453. doi: 10.1016/j.ijhcs.2011.03.002
Konstantinou, P., Trigeorgi, A., Georgiou, C., Gloster, A. T., Panayiotou, G., and Karekla, M. (2020). Comparing apples and oranges or different types of citrus fruits? Using wearable versus stationary devices to analyze psychophysiological data. Psychophysiology 57, 1–21. doi: 10.1111/psyp.13551
Krijn, M., Emmelkamp, P. M. G., Olafsson, R. P., and Biemond, R. (2004). Virtual reality exposure therapy of anxiety disorders: a review. Clin. Psychol. Rev. 24, 259–281. doi: 10.1016/j.cpr.2004.04.001
Lindner, P., Miloff, A., Reuterskiöld, L., Andersson, G., and Carlbring, P. (2019). What is so frightening about spiders? Self-rated and self-disclosed impact of different characteristics and associations with phobia symptoms. Scand. J. Psychol. 60, 1–6. doi: 10.1111/sjop.12508
McCraw, K. S., and Valentiner, D. P. (2015). The circumscribed fear measure : development and initial validation of a trans-stimulus phobia measure. Psychol. Assess. 27, 403–414. doi: 10.1037/pas0000046
McNair, D. M., Droppleman, L. F., and Lorr, M. (1992). Edits Manual for the Profile of Mood States: POMS. San Diego, CA: Edits.
Menghini, L., Gianfranchi, E., Cellini, N., Patron, E., Tagliabue, M., and Sarlo, M. (2019). Stressing the accuracy: wrist-worn wearable sensor validation over different conditions. Psychophysiology 56, 1–15. doi: 10.1111/psyp.13441
Muris, P., and Merckelbach, H. (1996). A comparison of two spider fear questionnaires. J. Behav. Ther. Exp. Psychiatry 27, 241–244. doi: 10.1016/S0005-7916(96)00022-5
Ollander, S., Godin, C., Campagne, A., and Charbonnier, S. (2017). “A comparison of wearable and stationary sensors for stress detection,” in Proceedings of the 2016 IEEE International Conference on Systems, Man, and Cybernetics (SMC) (Budapest), 4362–4366. doi: 10.1109/SMC.2016.7844917
Powers, M. B., and Emmelkamp, P. M. G. (2008). Virtual reality exposure therapy for anxiety disorders: a meta-analysis. J. Anxiety Disord. 22, 561–569. doi: 10.1016/j.janxdis.2007.04.006
Regenbrecht, H., and Schubert, T. (2002). Real and illusory interactions enhance presence in virtual environments. Presence Teleoper. Virtual. Environ. 11, 425–434. doi: 10.1162/105474602760204318
Sano, A., Taylor, S., McHill, A. W., Phillips, A. J. K., Barger, L. K., Klerman, E., et al. (2018). Identifying objective physiological markers and modifiable behaviors for self-reported stress and mental health status using wearable sensors and mobile phones: observational study. J. Med. Internet Res. 20:e210. doi: 10.2196/jmir.9410
Suso-Ribera, C., Fernández-Álvarez, J., García-Palacios, A., Hoffman, H. G., Bretón-López, J., Baños, R. M., et al. (2019). Virtual reality, augmented reality, and in vivo exposure therapy: a preliminary comparison of treatment efficacy in small animal phobia. Cyberpsychol. Behav. Soc. Netw. 22, 31–38. doi: 10.1089/cyber.2017.0672
Szymanski, J., and Donohue, W. O. (1995). Fear of spiders questionnaire. J. Behav. Ther. Exp. Psychiatry 26, 31–34. doi: 10.1016/0005-7916(94)00072-T
Tanner, B. A. (2012). Validity of global physical and emotional SUDS. Appl. Psychophysiol. Biofeedback 37, 31–34. doi: 10.1007/s10484-011-9174-x
Tarnogol, F. M. (2018). Phobos AR (Version 1.3.2) [Mobile Application Software]. Retrieved from: http://itunes.apple.com (accessed November 14, 2019).
van Lier, H. G., Pieterse, M. E., Garde, A., Postel, M. G., de Haan, H. A., Vollenbroek-Hutten, M. M. R., et al. (2019). A standardized validity assessment protocol for physiological signals from wearable technology: methodological underpinnings and an application to the E4 biosensor. Behav. Res. Methods 52, 607–629. doi: 10.3758/s13428-019-01263-9
Venkatesh, V., Morris, M. G., Davis, G. B., and Davis, F. D. (2003). User acceptance of information technology: toward a unified view. Mis Q. 27, 425–478. doi: 10.2307/30036540
Wald, F. D. M., and Mellenbergh, G. J. (1990). De verkorte versie van de Nederlandse vertaling van de Profile of Mood States (POMS) [The shortened version of the Dutch translation of the Profile of Mood States (POMS)]. Ned. Tijdschr. Psychol. 45, 86–90.
Wrzesien, M., Alcañiz, M., Botella, C., Burkhardt, J. M., Bretón-López, J., Ortega, M., et al. (2013). The therapeutic lamp: treating small-animal phobias. IEEE Comput. Graph. Appl. 33, 80–86. doi: 10.1109/MCG.2013.12
Wrzesien, M., Burkhardt, J.-M., Alcañiz, M., and Botella, C. (2011). “How technology influences the therapeutic process: a comparative field evaluation of augmented reality and in vivo exposure therapy for phobia of small animals,” in Proceedings of the Human-Computer Interaction - INTERACT 2011 - 13th IFIP TC 13 International Conference (Vol. 6946) (Lisbon). doi: 10.1007/978-3-642-23774-4_43
Keywords: augmented reality exposure therapy, mixed reality, anxiety disorders, specific phobia, psychophysiology, skin conductance, e-mental health
Citation: De Witte NAJ, Scheveneels S, Sels R, Debard G, Hermans D and Van Daele T (2020) Augmenting Exposure Therapy: Mobile Augmented Reality for Specific Phobia. Front. Virtual Real. 1:8. doi: 10.3389/frvir.2020.00008
Received: 17 April 2020; Accepted: 09 July 2020;
Published: 25 August 2020.
Edited by:
Belinda Lange, Flinders University, AustraliaReviewed by:
Sheila A. M. Rauch, Emory University, United StatesKelsey Sprang Jones, Emory University, United States
Copyright © 2020 De Witte, Scheveneels, Sels, Debard, Hermans and Van Daele. This is an open-access article distributed under the terms of the Creative Commons Attribution License (CC BY). The use, distribution or reproduction in other forums is permitted, provided the original author(s) and the copyright owner(s) are credited and that the original publication in this journal is cited, in accordance with accepted academic practice. No use, distribution or reproduction is permitted which does not comply with these terms.
*Correspondence: Nele A. J. De Witte, bmVsZS5kd0B0aG9tYXNtb3JlLmJl