- 1Laboratoire de recherche en imagerie et orthopédie (LIO), Ecole de technologie supérieure (ETS), Montreal, QC, Canada
- 2CHUM Research Center, Université de Montréal, Montreal, QC, Canada
- 3Laboratoire de pathokinésiologie de Montréal, Institut universitaire sur la réadaptation en déficience physique de Montréal-Centre de recherche interdisciplinaire en réadaptation du Montréal métropolitain, Université de Montréal, Montreal, QC, Canada
When immersed in virtual reality, users who view their body as a co-located virtual avatar that reflects their movements, generally develop a sense of embodiment whereby they perceive the virtual body to be their own. One aspect of the sense of embodiment is the feeling of agency over the avatar, i.e., the feeling that one is producing the movements of the avatar. In contexts such as physical rehabilitation, telepresence and gaming, it may be useful to induce a strong sense of agency in users who cannot produce movements or for whom it is not practical to do so. Being able to feel agency over a walking avatar without having to produce walking movements could be especially valuable. Muscle vibrations have been shown to produce the proprioceptive perception of movements, without any movement on the part of the user. The objectives of the current study were to: 1-determine if the addition of lower-limb muscle-vibrations with gait-like patterns to a walking avatar can increase the illusory perception of walking in healthy individuals who are standing still; 2-compare the effects of the complexity of the vibration patterns and of their synchronicity on the sense of agency and on the illusory perception of walking. Thirty participants viewed a walking avatar from a first-person perspective, either without muscle vibrations or with one of four different patterns of vibrations. These five conditions were presented pairwise in a two-alternative forced choice paradigm and individually presented, after which participants answered an embodiment questionnaire. The displacement of center of pressure of the participants was measured throughout the experiment. The results show that all patterns of proprioceptive stimulation increased the sense of agency to a similar degree. However, the condition in which the proprioceptive feedback was realistic and temporally aligned with the avatar’s leg movements led to significantly larger anteroposterior sway of the center of pressure. The frequency of this sway matched the cadence of the avatar’s gait. Thus, congruent and realistic proprioceptive stimulation increases the feeling of agency, the illusory perception of walking and the motor responses of the participants when viewing a walking avatar from a first-person perspective.
Introduction
In immersive virtual reality (VR), viewed through a head-mounted display (HMD), a user’s body can be represented using a co-located virtual avatar. Different motion capture technologies can be used to animate the avatar in real-time so that when users move their limbs, they see their virtual limbs moving accordingly (Spanlang et al., 2014). As the brain receives sensory inputs from the body and the outside world, it is constantly updating the mental representation it makes of the body (Holmes and Spence, 2004; Berti, 2013). Therefore, when an avatar that is viewed from a first-person perspective (1PP) reflects the user’s movements in real-time with a very short delay (i.e., when visuomotor synchronicity is high), the virtual body is integrated into the user’s mental representation of their body’s configuration and position (Slater et al., 2010; Won et al., 2015). This results in a subjective feeling of embodiment in the avatar whereby the users feel the avatar as if it was their actual physical body (Slater et al., 2010; Kokkinara and Slater, 2014). The sense of embodiment can be induced through visuomotor (as just described) or visuotactile synchronous stimulation (the users are touched and simultaneously see their avatar being touched at the same anatomical location) but the former contributes the most to the attainment of the illusion (Kokkinara and Slater, 2014).
The sense of embodiment is a concept generally regarded as having three underlying subcomponents: ownership, agency, and self-location (Kilteni et al., 2012a). Ownership refers to the feeling that this body is the source of the experienced sensations. Agency refers to a feeling of global motor control where one feels they have the ability to control the movements of the body or that they are the author of observed movements of the body. Discrepancy between an action and visual feedback of the action negatively affects the feeling of agency (Franck et al., 2001). The sense of self-location refers to one’s feeling of being spatially inside of the virtual body.
The sense of embodiment can be a particularly strong illusion whose psychological effects have been studied extensively in recent years. It has been shown that one can be embodied in the body of a child (Banakou et al., 2013; Tajadura-Jiménez et al., 2017) or a body of different gender (Lopez et al., 2019; Schulze et al., 2019), ethnicity (Maister et al., 2013, 2015) or body shape (Normand et al., 2011; Kilteni et al., 2012b), for example. Embodiment has been shown to have an effect on motor control (Burin et al., 2019; Gonzalez-Franco et al., 2020) and, specifically, on gait rehabilitation (Liu et al., 2020; Willaert et al., 2020; Hamzeheinejad et al., 2021; Keshner and Lamontagne, 2021).
In addition to feeling agency over a virtual avatar that is mimicking one’s movements, some studies have shown users can feel a sense of agency over the movements of their avatar even though they are independent of their own movements. That is to say that, despite producing no physical movement, they have a feeling that they are actually producing the movements of the avatar. One example even shows illusory agency over the voice of an avatar: in the study by Banakou and Slater (2014), participants embodied in a virtual avatar that spontaneously began to speak reported a feeling of having spoken the words themselves.
In some contexts, it may be useful to induce a strong feeling of agency in users who cannot produce voluntary movements or for whom it isn’t practical to do so. One such example is in early phases of motor rehabilitation where patients may be unable to produce certain specific movements. Other examples include immersive gaming and telepresence applications where navigation is often achieved via controllers, in an unnatural manner. Different solutions have been proposed to allow for more natural navigation in immersive environments (e.g., sliding shoes, treadmills, walking in place, etc.), which could lead to embodied experiences, but these usually require cumbersome equipment and/or unnecessary physical effort (Whitton and Razzaque, 2008; Iwata, 2013; Nilsson et al., 2018). Being able to feel agency over a walking avatar without having to physically produce walking movements would be valuable in such contexts.
Some studies have shown that it is possible to induce the illusion of agency over the walking of a virtual avatar without any movement on the part of the user. For example, in the study of Kokkinara et al. (2016), participants were seated and observing a walking avatar from first—and third-person perspectives. In 1PP, the VE was displayed with and without head-sway. Through a subjective questionnaire, participants reported a strong feeling that they were walking and that the leg movements of the avatar were caused by their movements, when viewing the avatar in 1 PP. Head sway reduced this level of agency. Physiological responses (skin conductance, heart rate, and respiration rate) showed an increased arousal when the avatar walked up a hill. Matsuda et al. (2020) have recently shown that seated participants felt an increased sensation of walking in a VE when they observed a walking avatar from a 1PP (vs. vection without an avatar or with only virtual hands and feet). They also showed that combining the avatar with foot vibrations that were synchronized with foot strikes improved the sensation of walking and the illusion of agency over the leg movements (vs. randomized vibrations). The vibrations used in this study were to mimic contact of the body (foot) with an element of the VE (the floor), essentially creating visuotactile synchronicity.
Despite these results showing the possibility of agency without any movement from the user, a strong sense of agency is generally thought to require visuomotor synchronicity (Tsakiris et al., 2006; Kilteni et al., 2012a). However, several studies have shown that the feeling of embodiment of a surrogate body (physical or virtual) can be induced through synchronicity between the surrogate body and the movements of the user, even if these movements are passively produced (Tsakiris et al., 2006; Dummer et al., 2009; Walsh et al., 2011; Kalckert and Ehrsson, 2012). The finding that passive movements lead to embodiment indicates that proprioceptive sensory afference plays an important role in embodiment, in addition to motor control. In fact, Tsakiris and Haggard (2005) studied the role of agency and sensory afferences on body-awareness and showed that proprioception, action and touch all contribute.
Proprioceptive sensory afference can also be elicited without any movement (passive or active) by applying muscle vibrations (Goodwin et al., 1972; Roll and Vedel, 1982; Taylor et al., 2017). Indeed, perception of one’s own movement can be generated when applying vibration on muscle groups. Muscle vibration is known to activate sensory endings of neuromuscular spindles, particularly the primary endings Ia. This sensory activity is interpreted by the central nervous system as the lengthening of the vibrated muscle. When vibrations are applied on one muscle group, such as the quadriceps, the individual describes a perception of knee flexion, which is normally associated with lengthening of the quadriceps, despite the absence of actual movement. The perceived velocity of the illusory movements increases progressively with vibration frequencies from 10–70–80 Hz, then decreases from 80–120 Hz. In addition, the perception of more complex movements can be generated when several muscle groups are vibrated with the appropriate sequence. For example, applying vibrations to six elbow and shoulder muscle groups, Thyrion and Roll (2010) generated a perception of drawing letters and geometrical forms. More recently, the application of vibrations on the flexor and extensor muscle groups at the hips, knees, and ankles, with a sequence determined from lower-limb movements during gait, generated a strong illusory perception of gait movements in healthy individuals standing quietly (Tapin et al., 2019). Leonardis et al. (2014) have used such muscle vibrations to enhance embodiment of a walking avatar, although they were only applied to the knee flexors. Moreover, the vibrations were used in combination with a chair that was mounted on a mobile platform that generated body accelerations and rotations to provide vestibular sensory feedback. The addition of proprioceptive and vestibular feedback to the visual stimulation was found to significantly increase embodiment, respiration rate, and skin conductance, but not the feeling of walking.
The objectives of the current study were twofold. The first was to determine if the addition of lower-limb muscle-vibrations with gait-like patterns to a walking avatar can increase the feeling of agency and the illusory perception of walking in healthy individuals that are standing still. The second objective was to compare the effects of the complexity of the vibration patterns and of their synchronicity on the feeling of agency and on the illusory perception of walking. It was hypothesized that: (1) the addition of proprioceptive stimulation, through the use of muscle vibrations, would increase the level of agency and the illusory perception of walking; (2) complex vibrations patterns in which the proprioceptive stimulation applied on the hip, knee, and ankle joints of the participant matched the flexion/extension motion of the corresponding joint on the avatar would yield the highest level of agency and highest illusory perception of walking.
Methods
Virtual Environment and Avatar
A virtual replica of a corridor at our research center was developed using version 2017.4 of Unity game engine (www.unity.com). The virtual corridor has a width of 2.35 m and is designed to replicate itself every 28 m as a user navigates along its length, and create the perception that it is of infinite length. The walls forming the corridor both comprise a series of office doors and windows and are painted gray with brightly colored vertical stripes (Figure 1B). These stripes contribute to the user’s optical flow as their avatar progresses along the corridor, hence adding to the perception of motion.
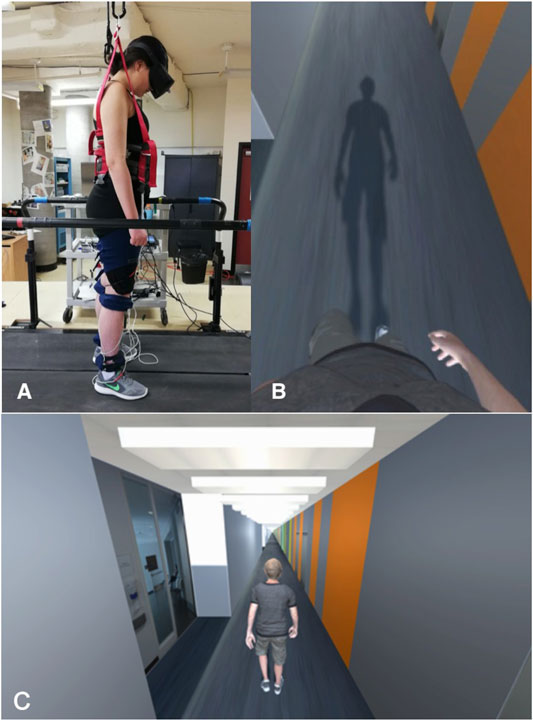
FIGURE 1. Experimental set-up, with head-mounted display, vibrators strapped on the ankles, knees, and hips, and security harness (A); the visual scene displayed in the head-mounted display from a 1 PP (B); the virtual environment shown from a 3 PP (C).
A rigged generic male virtual avatar (Remy character from www.mixamo.com) was animated, walking down the virtual corridor (Figure 1C). The avatar had a height of 1.7 m and an average build. The avatar was not gender matched to the user as the avatar was viewed from a first-person perspective and only visible from approximately the abdomen on down. However, the shadow of the full avatar was visible on the floor in front of the user (Figure 1B).
The avatar was animated from a motion capture (mocap) acquisition of a single participant (26 year old male, height of 1.8 m, weight of 75 kg) walking on a treadmill at a self-selected comfortable pace on a force-sensing split-belt treadmill (AMTI—www.amti.biz). The participant walked at a pace of 1.0 m/s during which a 60-s recording was taken, to be used for the animation of the avatar. The average cadence over this recording was 100.4 steps per minute. Gait kinematics were acquired using a 12-camera Vicon T20S system and Vicon Nexus software. The participant wore 15 marker clusters, each composed of four reflective mocap markers fixed to a rigid object. These marker clusters were placed on the head, hands, forearms, arms, feet, shanks, thighs, upper back (over T1 vertebrae), and lower back (over L3 vertebrae). Single markers were placed over the femoral epicondyles and malleoli. A functional calibration method, consisting of a series of joint rotations, was then performed to establish joint centers and rotation axes (Dallaire-côté et al., 2016). Upper—and lower-limb joint rotation angles were computed from the raw kinematic data using the method first described by Grood and Suntay (1983). The avatar was animated by mapping the positions and orientations of the upper back and lower back marker clusters to the rig of the avatar. The rotation angles of each of the upper and lower limb joints were applied to the joints of the avatar’s rig. MotionBuilder (Autodesk) was used to manually improve the visual fluidity of the animation.
During experimentations, the virtual environment (VE) was viewed from the first-person perspective of the avatar through an Oculus Rift CV1 HMD (110° field of view, 1,080 × 1,200 pixels per eye, 90 Hz refresh rate). The head of the avatar, and therefore the visual perspective of the participants, was animated in real-time from the position and orientation of the HMD, obtained using the Oculus internal inertial sensors (orientation) and an Oculus Sensor (position). Thus, the participants could look around the VE and down at their virtual avatar as it walked in the corridor. The overhead lighting of the virtual corridor produced a shadow of the avatar on the floor in front of it so the participants could see the whole body movements of the avatar (Figure 1B).
Proprioceptive Stimulation
The proprioceptive stimulation system was composed of 12 vibrators (VB115, Techno Concept, France), held in place on the lower limbs using a neoprene band tightened with Velcro (Figure 2). The vibrators were placed on the flexor and extensor muscles at the hip, knee, and ankle, bilaterally. The vibrators were controlled using a custom-made LabVIEW interface (National Instruments; Austin, Texas). The sequence of activation of the vibrators was determined based on the kinematics of the lower-limbs of the avatar. As vibrations reproduce sensory activity associated with muscle lengthening, vibrators on the flexor muscles were activated at 70 Hz when the joint extended on the visually presented avatar, and reciprocally for extensor muscles, as described by Duclos et al. (2014). The activation and deactivation sequences for all vibrators were produced offline from the gait kinematics used to animate the avatar. Figure 3 illustrates these patterns for each of the vibrators on an averaged gait cycle. Only one train of vibration was applied per gait cycle due to mechanical limitations of the vibrators to change vibration frequency fast enough (Ivanenko et al., 2000; Duclos et al., 2014). Synchronization between the vibrators and the VE was achieved by sending a TTL signal from the rendering system to the computer running Labview. The delay between the signal and the onset of vibrations was previously found to be 22.4 ± 22.9 ms (Duclos et al., 2014).
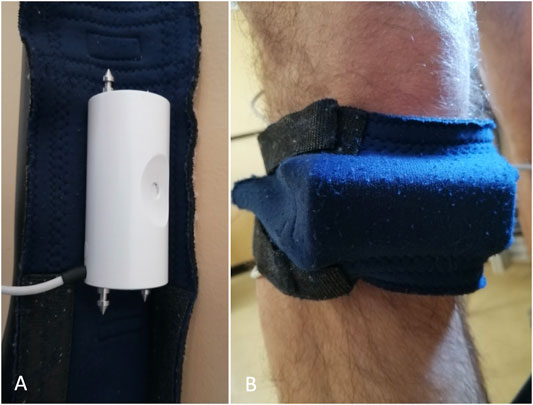
FIGURE 2. A vibrator used to apply vibrations to the muscle groups of the lower limbs (A); a vibrator held in place on over the extensor muscles of the knee, using a neoprene band tightened with Velcro (B).
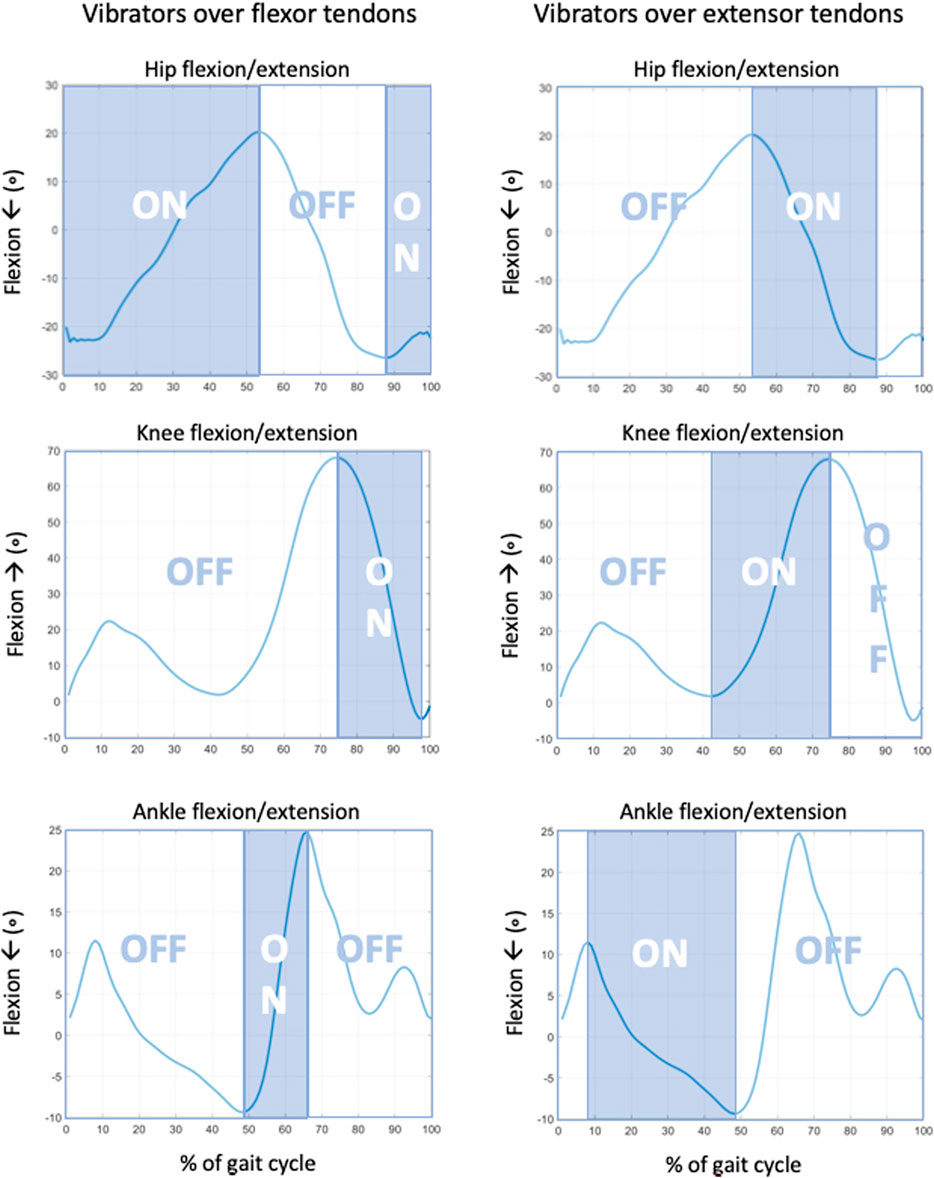
FIGURE 3. Kinematic data of the hips (top), knees (middle), and ankles (bottom) used to animate the avatar and to trigger the vibrators on the flexor muscles (left column) and on the extensor muscles (right column). Vibrators were turned on (ON) on flexor muscles (left column) when joint moved toward extension and on extensor muscles (left column) when joint moved toward flexion, to mimic muscle sensory activity associated with muscle lengthening. Vibrators were off (OFF) the rest of the time.
Procedures
Thirty healthy participants (16 male, 14 female; 24.8 ± 3.0 years old; 1.75 ± 0.10 m; 70.6 ± 12.8 kg) volunteered to take part in this study. Inclusion criteria were to have no pathology, medical history or medication that could affect gait, balance, motor function or sensory perception. Participants were recruited amongst students and research assistants from the authors’ research centers and universities. The experiment was approved by the Research Ethics Committees of Universite de Montreal Hospital Center (CHUM), Université de Montreal and of Ecole de technologie supérieure (ETS), project MP-02-2019-7977. All participants gave informed written consent before the beginning of the experimentation.
For the duration of the experiment, participants were asked to wear sports shorts to facilitate the placement of the vibrators and to avoid attenuating the perception of vibrations by having the vibrator placed over a layer of clothing. They also wore a medical treadmill harness to support their weight in the event of a fall due to loss of balance while immersed in the VE. They stood on a treadmill that was instrumented with embedded force plates (Bertec Fully Instrumented Treadmill) and were allowed to hold onto the handrailing on either side of the treadmill during the setup of the equipment (Figure 1A). The treadmill was never activated during the experimentations and was only used to record ground-reaction forces.
Once the vibrators were in place, there was a 1-min familiarization period, where the participants had gait-pattern vibrations applied to their lower limbs, without wearing the HMD or viewing the VE. This was done to familiarize participants to the sensation of the vibrations and to ensure that the vibrators had been placed correctly and were not slipping. Following this familiarization, the Oculus HMD was positioned on the participant’s head. They were instructed to stand upright, with both feet side by side, and knees fully extended but not locked back. They were asked to look down at their lower limbs for the duration of the experimentation, occasionally looking up ahead in the VE and back down, if that improved their comfort. They were also asked not to hold on the railing while they were immersed in the VE. They were instructed to inform the experimenter immediately if they felt dizziness, nausea or discomfort so that the experiment could be halted.
Five different conditions were presented in two separate phases of the experimentation. These conditions differed in the concordance between the visual stimulation (the avatar’s lower-limb kinematics) and the proprioceptive stimulation (the sequence of muscle vibrations). For all conditions, the visual simulation that was presented in the HMD was exactly the same. Only the vibration patterns differed between the different conditions, which were the following:
Visual only (VIS) No proprioceptive stimulation was presented. In other words, the participants viewed their avatar walking down the virtual corridor, but the vibrators were never activated.
Congruent (CON) The participants viewed their avatar walking in the virtual corridor and the vibrator activations were synchronized and congruent with the movements of the avatar.
Delayed (DEL) The vibrators were activated as for the congruent condition but a constant 100 ms delay was added between the movements of the avatar and the onset of the vibrations, for the entirety of the condition.
Out of phase (OOP) The vibrators were activated as for the congruent condition but on the contralateral limb, resulting in proprioceptive stimulation of a gait pattern that is out of phase with the visual feedback. In other words, the proprioceptive stimulation matching the movement of the left limb of the avatar was applied to the right limb of the participant and the stimulation matching the avatars right limb was applied to the left limb of the participant.
Lateralized (LAT) The six vibrators on a limb were activated simultaneously during the stance phase of gait only. In other words, the vibrators of one limb were activated continuously while the foot of that limb was visually in contact with the ground (heel-strike to toe-off), ignoring the individual joint kinematics of the avatar.
Pairwise Comparison of Conditions
In this first phase, conditions were presented in pairs to the participants and they were asked to choose the condition that gave them the strongest feeling of walking in a two-alternative forced choice (2AFC) paradigm. Each condition was compared to all other conditions once, i.e., they were not presented in both possible orders. This resulted in a total of 10 pairs of conditions which were presented in randomized order. The first condition presented within each pair was also randomized. Each pair of conditions was presented as follows:
• The participant viewed a 60-s trial of the avatar walking with vibration congruence according to the first condition.
• The avatar stopped walking and stood still with vibrations disabled, for a 10-s period.
• The participant viewed a 60-s trial of the avatar walking with vibration congruence according to the second condition.
• The participant was asked to verbally answer the following question: “In which of the two presented conditions did you have the strongest feeling that you were walking.”
Data Acquisition
In the second phase, each of the five conditions were presented in randomized order. The participants observed their virtual avatar walking for a period of 60 s in each condition. At the end of each condition, they removed the HMD and answered a 9-question questionnaire. They were asked to read the questions once before the beginning of the first condition, so that they would know what they would be asked to evaluate. This was done to prevent bias in the first condition because in the following conditions, participants would have already answered the questionnaire and would know what to pay attention to. This questionnaire was composed of a subset of 8 questions from the Gonzalez-Franco Peck (2018) questionnaire on avatar embodiment. The subset of questions was obtained by taking all questions from the 3 components of embodiments and removing the questions that could not be applied to our study. The removed questions were questions 3 through 5 (body ownership) which only apply when there is a virtual mirror present, as well as question 8 (agency) which does not apply to a context where the user is static. The participants answered each question on an electronic tablet, using a 7-point Likert-scale with: strongly disagree (1), disagree (2), somewhat disagree (3), neither agree nor disagree (4), somewhat agree (5), agree (6), strongly agree (7). The resulting questionnaire was the following:
Body Ownership
Q1: “I felt as if the virtual body was my body”Q2: “It seemed as if I might have more than one body”
Agency
Q3: “It felt like I could control the virtual body as if it was my own body”Q4: “The movements of the virtual body were caused by my movements”Q5: “I felt as if the virtual body was moving by itself”
Location of the Body
Q6: “I felt as if my body was located where I saw the virtual body”Q7: “I felt out of my body”Q8: “I felt as if my (real) body were drifting toward the virtual body or as if the virtual body were drifting toward my (real) body”.
Participants were also asked to answer the following question (hereinafter referred to as the “Perception of walking” question), specific to our study: “I felt like I was walking”, with the same scale Likert scale.
For the duration of all of the trials of both phases, ground-reaction forces were recorded at a 1000 Hz sampling-rate. These forces were collected as an additional measure of the strength of the perceptual illusion of walking that was induced, given previous studies have shown that muscle vibrations can trigger actual movements of small amplitude (Duclos et al., 2014) and that embodiment of an avatar can lead the user to follow the avatars position (Asai, 2014) or movements (Burin et al., 2019; Gonzalez-Franco et al., 2020). Raw ground-reaction force were smoothed using a 2nd order, 499 point Savitzky–Golay filter. The center of pressure (COP) across time (the barycenter of the COP of each foot) was computed from the smoothed ground reaction forces for the middle 50 s of each trial. The first and last 5 s of each trial were excluded because the avatar’s gait was in the acceleration or deceleration phase. Additionally, to remove outliers, data points that were beyond three standard deviations from the mean of the trial were excluded.
Data Analysis
In the 2AFC phase, the percentage of comparisons where each condition was chosen over the other presented conditions (regardless of what the other condition was) was calculated. The answers to questions Q1 through Q8 were analyzed by category (body ownership score = Q1−Q2; agency score = Q3+Q4−Q5; location of the body score = Q6−Q7+Q8). One-way repeated-measures ANOVA was performed on responses to the Perception of walking question and on the three categories of the questionnaire to determine if the pattern of proprioceptive stimulation has a significant effect the subjective scores (Carifio and Perla, 2008; Norman, 2010; Sullivan and Artino, 2013), with a Bonferroni-adjusted significance level set at 0.013. The normality assumption was verified by applying the D’Agostino-Pearson normality test to the residuals of each ANOVA, at 95% confidence level. For the Perception of walking question and agency, the normality assumption was confirmed so post-hoc pairwise comparisons were conducted using Tukey’s HSD test (α = 0.05). For body ownership and body location, the normality assumption was rejected. Therefore, a non-parametric Friedman test (repeated measures) was performed with a Bonferroni-adjusted significance level set at 0.013 to determine if vibration patterns had a significant effect on these scores. Post-hoc pairwise comparisons were conducted using Dunn’s test (α = 0.05), corrected for multiple comparisons.
For each trial, the area (convex hull) covered by the displacement of the COP during the trial was computed. For each trial of a given condition, the visual and proprioceptive signals occurred at the exact same times within the recordings. Therefore, a mean COP displacement was computed for each condition. To compute this mean COP, the mean position of the COP (in x and y) of each trial was subtracted from all COP position values in order to align the different trials. Then, for each trial of a given condition, a mean x and y position was calculated for each timeframe, across both phases of the study and all participants. A spectral analysis by Fourier transform was performed on the resulting data to compare their frequencies in each condition. The mean amplitude of anteroposterior (AP) oscillation, at the dominant frequency, was calculated for each condition. The normality assumption for a one-way repeated-measures ANOVA was rejected by applying the D’Agostino-Pearson normality test to the residuals of the COP area and COP amplitude of oscillation. Therefore, a non-parametric Friedman test (repeated measures) was performed to determine if the pattern of proprioceptive stimulation has a significant effect on the COP area or oscillation amplitude, with a Bonferroni-adjusted significance level set at 0.025. Post-hoc pairwise comparisons were conducted using Dunn’s test (α = 0.05), corrected for multiple comparisons.
Results
All thirty participants that were recruited completed the study. None of them reported any dizziness, nausea or other discomfort associated with simulator sickness.
Two-Alternative Forced Choice
In one-to-one comparisons, the lateralized (LAT) condition was the most often preferred, having been chosen 64% of the time. The delayed (DEL) and congruent (CON) conditions were also preferred in a majority of matchups, with 60 and 57%, respectively. The out of phase (OOP) condition was preferred in 49% of comparisons, while the visual-only (VIS) condition, with no proprioceptive stimulation was preferred only 20% of the time. Figure 4 shows the percentage of preference of each condition across all trials and all participants, regardless of which condition it was being compared to.
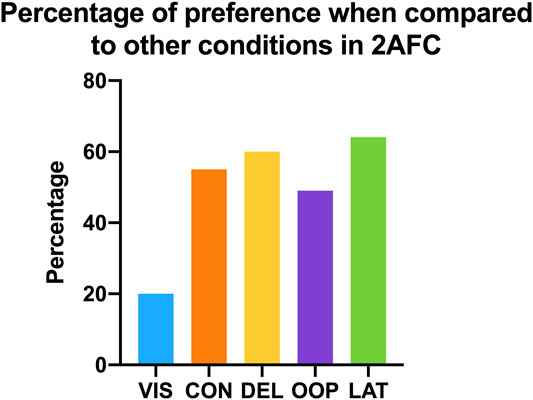
FIGURE 4. Percentage of choice for each condition in the ten pairs of one-to-one comparisons in the two-alternative forced choice phase (30 participants for each ten comparisons). VIS, Visual-only condition (no vibration); CON, congruent condition; DEL, delayed condition; OOP, out-of-phase condition; LAT, lateralized condition.
Subjective Questionnaire
Statistical analysis using one-way ANOVA revealed that the pattern of proprioceptive stimulation had a significant effect on the participant’s illusory perception that they were actually walking (Perception of walking question), (F(4,116) = 12.02, p < 0.001, R2 = 0.328). Pairwise comparisons revealed significant differences between the visual-only condition and every other condition (CON: diff. = −1.73, 95% CI = −2.74:−0.72, p < 0.001; DEL: diff. = −1.87, 95% CI = −2.88:−0.86 p < 0.001; OOP: diff. = −1.77, 95% CI = −2.78:−0.76, p < 0.001; LAT: diff. = −2.33, 95% CI = −3.34:−1.32, p < 0.001). Differences between conditions with proprioceptive stimulation were not significant. Figure 5 shows the mean score and 95% confidence interval for each condition.
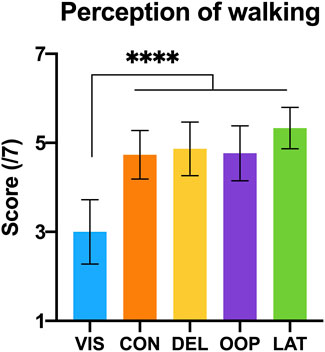
FIGURE 5. Mean score (N = 30 participants) and 95% interval limits obtained in the second phase of the experiment, for the Perception of walking question (/7) for each condition. VIS, Visual-only condition (no vibration); CON, congruent condition; DEL, delayed condition; OOP, out-of-phase condition; LAT, lateralized condition. ****Indicates statistical significance, p < 0.0001.
When grouping questions into the 3 components of embodiment, the proprioceptive stimulation patterns had no significant effect on body ownership ( χ2 = 1.972, p = 0.741) or body location (χ2 = 6.336, p = 0.175). It did however have a significant effect on agency (F(4,116) = 3.94, p < 0.005, R2 = 0.120). Pairwise comparisons revealed significant differences between the visual-only condition and every other condition (CON: diff. = −2.53, 95% CI = −4.96:−0.11, p = 0.036; DEL: diff. = −2.77, 95% CI = −5.19:−0.34, p = 0.017; OOP: diff. = −2.83, 95% CI = −5.26:−0.41, p = 0.013; LAT: diff. = −2.80, 95% CI = −5.22:−0.38, p = 0.015). No other differences between conditions were significant. Figure 6 shows the mean score and 95% confidence value for each question, grouped by embodiment component.
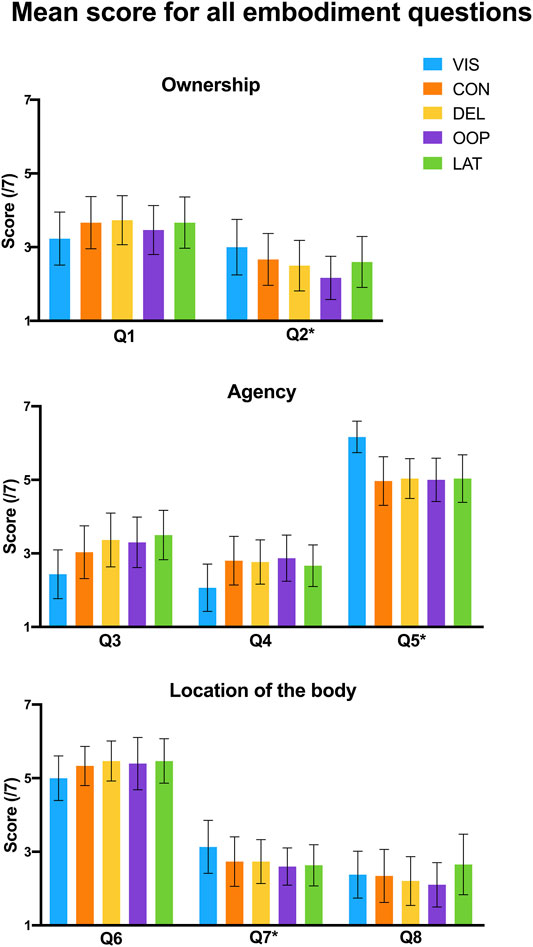
FIGURE 6. Mean score (N = 30 participants) and 95% interval limits obtained in the second phase of the experiment, for all questions of the embodiment questionnaire, grouped by embodiment component. VIS, Visual-only condition (no vibration); CON, congruent condition; DEL, delayed condition; OOP, out-of-phase condition; LAT, lateralized condition. *Indicates a question that is scored negatively (a lower value is indicative of a stronger sensation in the related embodiment component).
Center of Pressure
A non-parametric Friedman test of differences among repeated measures was conducted and showed that the condition of proprioceptive stimulation had a significant effect on the area covered by the COP (χ2 = 32.18, p < 0.001). Pairwise comparisons revealed significant differences between the visual-only condition and every other condition (CON: Z = 4.70, rank sum diff. = −57.50, p < 0.001; DEL: Z = 4.37, rank sum diff. = −53.50, p < 0.001; OOP: Z = 3.35, rank sum diff. = −41.00, p = 0.008; LAT: Z = 4.74, rank sum diff. = −58.00, p < 0.001). Differences between the conditions with proprioceptive stimulation were not significant. The average area (convex hull) covered by the COP displacement was 3.8 ± 2.3 cm2 for the visual-only condition and 6.9 ± 5.4 cm2 for all the conditions with proprioceptive stimulation, combined.
Figure 7 presents a 13-s segment (for visual clarity, although the entire duration was analyzed) of the mean AP displacement of the COP, across all participants. The pattern of proprioceptive stimulation had a statistically significant effect on the mean amplitude of AP oscillation at 0.84 Hz (χ2 = 106.0, p < 0.001). In pairwise comparisons, the mean AP displacement of the COP in the visual-only conditions was statistically smaller than in each of the vibration conditions (CON: Z = 9.72, rank sum diff. = −119.0, p < 0.001; DEL: Z = 6.78, rank sum diff. = −83.0, p < 0.001; OOP: Z = 4.57, rank sum diff. = −56.0, p < 0.001; LAT: Z = 3.43, rank sum diff. = −42.0, p = 0.006). Moreover, the mean AP displacement of the COP in the congruent condition was significantly larger than in each of the other vibration conditions (DEL: Z = 2.94, rank sum diff. = 36.0, p = 0.033; OOP: Z = 5.14, rank sum diff. = 63.0, p < 0.001; LAT: Z = 6.29, rank sum diff. = 77.0, p < 0.001). Finally, the mean AP displacement of the COP in the DEL condition was significantly larger than it was in the LAT condition (Z = 3.35, rank sum diff. = 41.0, p = 0.008). There was no significant difference between DEL and OOP or between OOP and LAT. Figure 8 shows the mean value and 95% confidence interval for each condition. Fourier analysis revealed a main frequency of 0.84 Hz for all four conditions with proprioceptive stimulation, which corresponds to a cadence of 100.8 “steps” per minute.
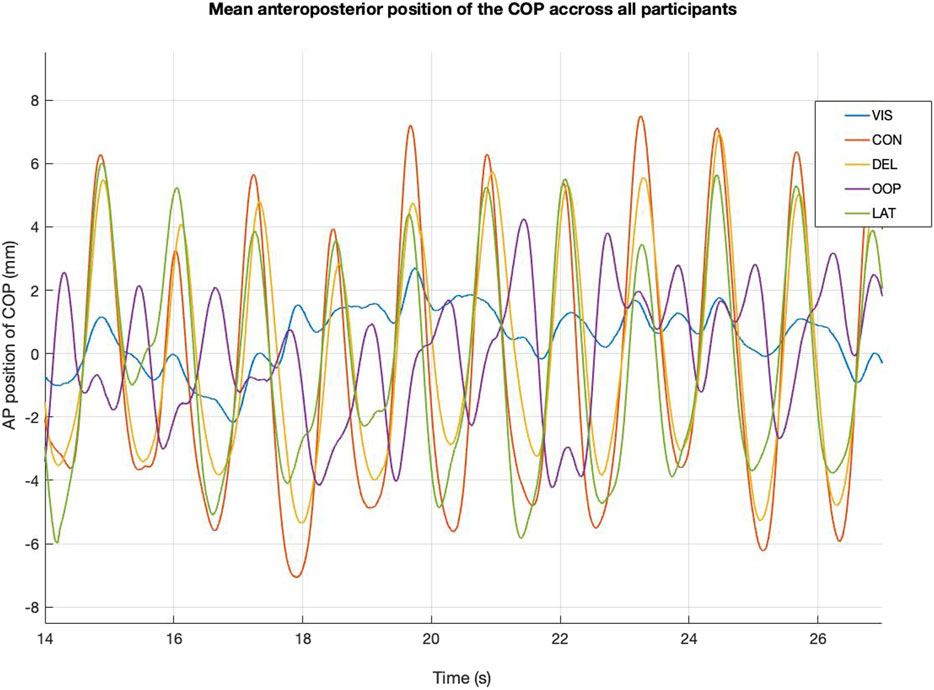
FIGURE 7. Averaged (N = 30 participants) anteroposterior instant position of the centre of pressure, presented over 13 s. VIS, Visual-only condition (no vibration); CON, congruent condition; DEL, delayed condition; OOP, out-of-phase condition; LAT, lateralized condition.
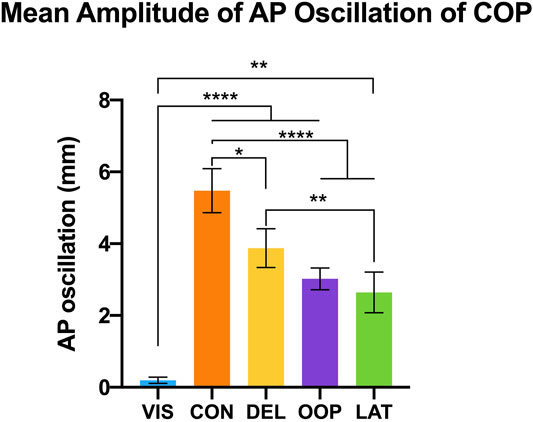
FIGURE 8. Mean (N = 30 participants), and 95% interval limits, amplitude of anteroposterior displacements of the centre of pressure for each condition. VIS, Visual-only condition (no vibration); CON, congruent condition; DEL, delayed condition; OOP, out-of-phase condition; LAT, lateralized condition. *Indicates statistical significance with p < 0.05, **indicates statistical significance with p < 0.01 and **** indicates statistical significance with p < 0.0001.
Discussion
This study investigated whether the addition of proprioceptive feedback to a walking avatar, viewed in 1 PP, increases agency and illusory perception of walking in static healthy participants. The results of the subjective evaluations (questionnaires and 2AFC) support this hypothesis. We had further hypothesized that vibration patterns that were more complex and synchronized with the movements of the avatar, therefore simulating the proprioception of walking in a more realistic manner, would result in higher levels of agency and of illusory perception of walking. This hypothesis was not supported by the subjective results. However, analysis of COP showed a higher AP sway, at the frequency of the avatar’s gait pattern, with the more complex and synchronized vibration pattern (congruent condition).
Proprioceptive stimulation, irrespective of the vibration pattern, had a significant effect on the agency score of the questionnaire but not on the ownership or self-localisation scores. Tsakiris et al. (2006) found that a purely proprioceptive sense of body ownership, induced through passive movements, is localised and fragmented, contrary to a sense of ownership induced through active movements. They suggested that action plays an important role in the sense of self, over and above that of proprioception. The absence of movement on the part of the participants of our study may have indeed prevented the illusion of ownership from developing. In contrast, the “cue integration” theory of sense of agency stipulates that it is based on various different sources of information, such as visual and sensorimotor, according to their relative perceived reliability (Moore, 2016). In our study, visual information alone was sufficient for participants to agency, and the addition of proprioceptive stimulation augmented that illusory feeling. As for the self-localisation, it is not a surprise that it was not affected as this component refers to the feeling of one’s body being spatially aligned with one’s virtual body. In this study, the virtual avatar was collocated with the participant’s actual body.
The participants had some degree of illusory perception that they were walking with only visual feedback (VIS condition), although this perception was significantly lower than with proprioceptive stimulation. Indeed, they reported a mean of 3.0/7 to the Perception of walking question, without any vibrations. Other studies have also reported some level of illusory perception of walking from purely visual feedback in the form of an avatar walking in a VE, viewed from a 1PP. In a study by Leonardis et al. (2014), participants subjectively reported a median of 3.5/7 in such a condition, similar to our findings. Matsuda et al. (2020) conducted a study where participants viewed a walking avatar, accompanied by vibrations under their feet that were either synchronous with foot strike or randomized. In the condition where vibrations were not synchronous, participants reported a mean perception of walking of approximately 35/100. In contrast to these results, a study by Kokkinara et al. (2016) reported a strong perception of walking from simply viewing a walking avatar (median 6/7). Many factors can impact subjective evaluations between different studies (e.g., quality of graphics, realism of the avatar, and of its gait, etc.). However, one factor that may have contributed to the higher perception of walking from viewing a walking avatar in a 1PP in the study of Kokkinara et al. (2016) is that participants also viewed the scene from a 3 PP, in another condition. Therefore, the 1PP condition was comparatively better in terms of embodiment and agency. In our study, the visual-only (VIS) condition represented the worst scenario. In the first phase of our study, participants were placed in each condition several times, before subjectively evaluating them in phase 2. Therefore, they had the conditions with proprioceptive stimulation as points of comparison. By comparison, the perception of walking in the visual only condition may have been lower.
In the 2AFC paradigm, the VIS condition was preferred in 20% of trials when compared to any of the proprioceptive stimulation conditions. The addition of proprioceptive stimulation to the visual stimulation led to a significant increase in the illusory perception of walking and of the feeling of agency. Indeed, illusory perception of walking increased from 3/7 to a mean of 4.9/7 (or 70/100) across all conditions with proprioceptive stimulation (CON, DEL, OOP, and LAT). In the enriched condition of Leonardis et al. (2014), where vibrations over the knee flexor muscles were combined with vestibular feedback from a chair mounted on a mobile platform, perception of walking was increased from 3.5/7 to 5/7, which was not statistically significant. Our results, using more complex vibration patterns but without vestibular feedback, show a larger increase in the illusory perception of walking than was found in the study of Leonardis et al.(2014). Matsuda et al. (2020) used foot vibrations as a tactile feedback to simulate the feeling of the foot contacting the ground. When these vibrations were synchronized with foot strikes of the avatar, participants in the study reported a significant increase from 35/100 (with randomized vibrations) to 65/100 in perception of walking. The tactile simulation therefore led to a perception of walking that was only slightly lower than what was found in the current study.
The results of the subjective questionnaire showed no significant differences between the different patterns of proprioceptive stimulation, which refutes our second hypothesis. All of the vibration patterns in this study were rhythmic at a frequency that matched the frequency of the gait cycle of the avatar (100.4 steps per min or 0.84 Hz). Therefore, even if the proprioceptive feedback provided to the participants was not congruent with the avatar’s movements, and thus not stimulating the proprioception of gait, the vibrations were providing a vibrotactile feedback that was synchronous with the visual feedback. As discussed above, foot vibrations synchronized with the foot strikes of a walking avatar have been shown to be sufficient to significantly improve the illusory perception of gait (Matsuda et al., 2020). A condition where vibrations were randomized or not of the same frequency as the gait of the avatar could presumably have led to lower embodiment and perception of walking scores, and it is a limitation of this study that such a condition was not included. Moreover, several studies have shown that the addition of synchronous vibrotactile feedback in a VE significantly increases the perceived level of presence (Dinh et al., 1999; Meehan et al., 2002) and embodiment (Slater et al., 2008; Kokkinara and Slater, 2014) even though this feedback is generally of low fidelity (simple vibrations that do not attempt to simulate actual tactile sensations). Therefore, in our study, it is conceivable that the vibrations were consciously interpreted more as vibrotactile feedback, synchronized with the gait of the avatar even though they were not replicating contact with elements of the VE.
Taken alone, the results of the 2AFC do not indicate that the illusory perception of walking was induced in any of the conditions as participants could presumably have been forced to choose between two conditions in which they did not feel like they were walking. However, considering them in conjunction with the questionnaire scores that show a high level of illusory perception of walking and of agency for all conditions with vibrations, some trends are observable. The lateralized condition was chosen 64% of the time overall despite being the least realistic in terms of proprioception. This may support the idea that the vibrations were consciously interpreted as synchronous tactile feedback. Additionally, some participants shared after the experimentation that the more complex patterns were more difficult to interpret and they preferred the simple, alternating vibrations in the LAT condition. The out-of-phase (OOP) condition was the least often chosen proprioceptive condition. This also supports the role of vibrotactile synchronicity in the participants’ perception because, in the OOP condition, vibrations are switched between the limbs so that synchronicity with the avatar’s movements is diminished. Given the 100.8 steps per minute cadence of the virtual avatar, the vibrations in the out-of-phase condition are similar to what would be obtained be adding a delay of 595 ms (the average duration of a single step). Conditions CON and DEL were chosen at a similar rate, 60 and 57% respectively. The introduction of a delay decreases visuotactile synchronicity but it is possible the delay we introduced (100 ms) in the DEL condition was insufficient for this decrease to be perceived. Previous studies have shown that users in VR begin to notice latency at values as low as 10–15 ms in some contexts (Jerald and Whitton, 2009). However, in a study by Samaraweera et al. (2013), 12 of 14 participants failed to notice a latency of 225 ms applied to the movements of their avatar during gait, even though this created a visuotactile asynchronicity between their foot strikes and the foot strikes of their avatar. Moreover, Maselli et al. (2016) showed that inducing body ownership increases the just noticeable difference (JND) between visual and tactile stimuli and mean JND values were larger than 100 ms, with or without ownership.
The area covered by the displacement of the COP during the experiment was significantly higher for all proprioceptive conditions, compared to the visual-only condition. The area was 81.5% larger, for all proprioceptive conditions, combined. Taken alone, this result could simply be reflecting the fact that vibrations applied to the lower limb, and especially to the ankle, can impair postural control (Hay et al., 1996; Vuillerme et al., 2002). However, when we analyze anteroposterior COP displacement averaged across all participants (Figure 7), we observe a rhythmic, forward and back, sway. Moreover, the frequency of this sway (0.84 Hz or 100.8 steps per min) matches the frequency of the avatar’s gait for all proprioceptive conditions. In the visual-only condition, no such rhythmic sway of the COP is observed. This result suggests that the participants have a motor response to the vibration patterns, either through gait-like movements and/or postural adjustments, but not to the visual perception of the avatar alone. However, the amplitudes of oscillations at 0.84 Hz reveal a strong interaction between vibration patterns and the visual perception of the virtual body. Indeed, in CON, OOP, and DEL conditions, the vibration patterns that are applied to the participants lower limbs and the visual feedback (the walking avatar) are exactly the same. All that distinguishes them is the temporal alignment between the vibrations and the gait of the avatar. If the proprioceptive stimulation alone were responsible for the AP sway, we could expect them to be similar across these three conditions but they are all significantly different from one another. The CON condition, where proprioceptive stimulation was congruent with visual feedback, lead to the largest sway. The DEL condition had the next closest alignment between visual and proprioceptive feedback and produced the second highest AP sway of the COP, significantly lower than in the CON condition although participants did not seem to perceive this delay in subjective evaluations. OOP condition, where the proprioceptive stimulation is applied to the wrong limb, and LAT condition, where vibrations produce rhythmic feedback but no realistic proprioceptive stimulation, led to the least amount of sway. This important finding suggests that while the different vibration patterns were not subjectively perceived differently with regards to their ability to induce the illusory perception of walking, the motor response was larger when the proprioceptive stimulation matched the visual stimulation.
The use of an unvalidated questionnaire to measure the different components of embodiment is a limitation of this study. Indeed, when this experiment took place, there didn’t exist any validated embodiment questionnaire. We therefore used a questionnaire that had been suggested in the literature, but had not yet been validated (Gonzalez-Franco and Peck, 2018). In addition, as suggested by the authors of this questionnaire, we removed the questions that did not apply to our context. Therefore, although we grouped the subjective questions into embodiment components, the resulting scores cannot be considered to be validated measures of these components. Therefore, only the Perception of walking question results are directly compared to the results of previous studies, as they also included such a question. Recently, two validated questionnaires have been proposed (Roth and Latoschik, 2020; Peck and Gonzalez-Franco, 2021).
Another limitation is the use of a generic male avatar without matching the morphology of the participants. If participants perceived their virtual bodies to be very different from their own in terms of height and weight, this could have had an impact on the position of their COP during perceived gait. Nonetheless, the results that are reported compare the different conditions (repeated measures) where the avatar is always the same so the effects of morphology differences on the results are believed to be limited. Despite the fact that the participants could only see their lower limbs and their shadow, it is possible that some female participants noticed the gender-mismatch. This could have had an impact on the strength of their embodiment in the avatar although previous studies have shown that participants can successfully embody gender mismatched avatars (Petkova and Ehrsson, 2008; Lopez et al., 2019; Schulze et al., 2019).
Overall, the results show that muscle vibrations can be used to improve the illusory perception of walking and agency obtained through a virtual avatar in a VE, even in the absence of actual movement of the user. Though the level of realism of the proprioceptive stimulation does not seem to affect the subjective experience of the user, realistic patterns may increase the motor facilitation or response associated with the level of embodiment, and more so when these patterns are precisely synchronised with the visual feedback. Thus, congruent and synchronous multisensory stimulation may be particularly important in situations of motor learning or physical rehabilitation, where sensory stimulation could be used to improve motor output. In addition to these applications, the proposed method allows a more realistic navigation of VEs without the space requirements or physical effort required by other methods. Moreover, although no measurement of simulator sickness was taken, the fact that no participant reported any associated symptoms throughout the experimental sessions seems to indicate that the method can be comfortably used for prolonged periods.
Conclusion
In conclusion, this study showed that proprioceptive stimulation increases the feeling of agency and the illusory perception of walking when viewing a walking avatar. Patterns of vibrations that differed in their complexity and synchronicity with the avatar’s movements did not significantly impact either of these subjective measures. However, motor behavior as measured by AP sway of the COP, differed significantly between vibration patterns. Indeed, sway was increased when the visual and proprioceptive information were congruent. Future work will include combining the proprioceptive stimulation with a brain-computer interface (BCI) that controls the gait of the avatar (Alchalabi et al., 2021), in an effort to produce a stronger sense of agency and test its application for motor rehabilitation.
Data Availability Statement
The raw data supporting the conclusions of this article will be made available by the authors, without undue reservation.
Ethics Statement
The studies involving human participants were reviewed and approved by Comité d’éthique de la recherche du CHUM, Comité d’éthique de la recherche en santé de l’Université de Montreal and Comité d’éthique de la recherche de l’ETS. The patients/participants provided their written informed consent to participate in this study.
Author Contributions
DL, KK, and CD conceived and designed the experiment with input from SN and RA. KK implemented the virtual avatar, its control or proprioceptive stimulations. KK carried out the experiment and collected and compiled the data. KK, DL, and CD carried out the analysis of results. DL wrote the paper, with the help of all of the authors. The manuscript was reviewed by all of the authors.
Funding
Funding for this research was provided by a Team Research Grant of the Fonds de recherche du Quebec—Nature et technologies (FRQNT), Project Number 2018-PR-206224.
Conflict of Interest
The authors declare that the research was conducted in the absence of any commercial or financial relationships that could be construed as a potential conflict of interest.
Acknowledgments
The authors would like to thank Michel Goyette for his technical assistance in developing the proprioceptive stimulation system and its integration with the virtual environment.
References
Alchalabi, B., Faubert, J., and Labbé, D. R. (2021). A Multi-Modal Modified Feedback Self-Paced BCI to Control the Gait of an Avatar. J. Neural Eng. 18, 056005. doi:10.1088/1741-2552/abee51
Asai, T. (2014). Illusory Body-Ownership Entails Automatic Compensative Movement: for the Unified Representation between Body and Action. Exp. Brain Res. 233, 777–785. doi:10.1007/s00221-014-4153-0
Banakou, D., Groten, R., and Slater, M. (2013). Illusory Ownership of a Virtual Child Body Causes Overestimation of Object Sizes and Implicit Attitude Changes. Proc. Natl. Acad. Sci. 110, 12846–12851. doi:10.1073/pnas.1306779110
Banakou, D., and Slater, M. (2014). Body Ownership Causes Illusory Self-Attribution of Speaking and Influences Subsequent Real Speaking. Proc. Natl. Acad. Sci. USA 111, 17678–17683. doi:10.1073/pnas.1414936111
Berti, A. (2013). This Limb Is Mine but I Do Not Want it: From Anatomy to Body Ownership. Brain 136, 11–13. doi:10.1093/brain/aws346
Burin, D., Kilteni, K., Rabuffetti, M., Slater, M., and Id, L. P. (2019). Body Ownership Increases the Interference between Observed and Executed Movements. PLoS One 14, 1–16. doi:10.1371/journal.pone.0209899
Carifio, J., and Perla, R. (2008). Resolving the 50-year Debate Around Using and Misusing Likert Scales. Med. Educ. 42, 1150–1152. doi:10.1111/j.1365-2923.2008.03172.x
Dallaire-côté, M., Charbonneau, P., Côté, S. S.-P., Aissaoui, R., and Labbe, D. R. (2016). “Animated Self-Avatars for Motor Rehabilitation Applications that Are Biomechanically Accurate, Low-Latency and Easy to Use,” in IEEE Virtual Reality (South Carolina: Greenville), 167–168. doi:10.1109/VR.2016.7504706
Dinh, H. Q., Walker, N., Hodges, L. F., Chang Song, C., and Kobayashi, A. (1999). “Evaluating the Importance of Multi-Sensory Input on Memory and the Sense of Presence in Virtual Environments,” in Proceedings Virtual Real (Annu. Int. Symp.), 222–228. doi:10.1109/vr.1999.756955
Duclos, C., Kemlin, C., Lazert, D., Gagnon, D., Dyer, J.-O., and Forget, R. (2014). Complex Muscle Vibration Patterns to Induce Gait-like Lower-Limb Movements: Proof of Concept. J. Rehabil. Res. Dev. 51, 245–252. doi:10.1682/JRRD.2013.04.0079
Dummer, T., Picot-Annand, A., Neal, T., and Moore, C. (2009). Movement and the Rubber Hand Illusion. Perception 38, 271–280. doi:10.1068/p5921
Franck, N., Farrer, C., Georgieff, N., Marie-Cardine, M., Daléry, J., d’Amato, T., et al. (2001). Defective Recognition of One's Own Actions in Patients with Schizophrenia. Am. J. Physchitary. 158, 454–459. doi:10.1176/appi.ajp.158.3.454
Gonzalez-Franco, M., Cohn, B., Ofek, E., Burin, D., and Maselli, A. (2020). “The Self-Avatar Follower Effect in Virtual Reality,” in Proceedings—2020 IEEE Conference on Virtual Reality and 3D User Interfaces, 18–25. doi:10.1109/VR46266.2020.1580500165557
Gonzalez-Franco, M., and Peck, T. C. (2018). Avatar Embodiment. Towards a Standardized Questionnaire. Front. Robot. AI 5, 74. doi:10.3389/FROBT.2018.00074
Goodwin, G. M., Mccloskey, D. I., and Matthews, P. B. C. (1972). Proprioceptive Illusions Induced by Muscle Vibration: Contribution by Muscle Spindles to Perception? Science 175, 1382–1384. doi:10.1126/science.175.4028.1382
Grood, E. S., and Suntay, W. J. (1983). A Joint Coordinate System for the Clinical Description of Three-Dimensional Motions: Application to the Knee. J. Biomech. Eng. 105, 136–144. doi:10.1115/1.3138397
Hamzeheinejad, N., Roth, D., Monty, S., Breuer, J., Rodenberg, A., and Latoschik, M. E. (2021). “The Impact of Implicit and Explicit Feedback on Performance and Experience during VR-Supported Motor Rehabilitation,” in Proceedings of the 28th IEEE Virtual Reality Conference Lisbon, Portugal. doi:10.1109/vr50410.2021.00061
Hay, L., Bard, C., Fleury, M., and Teasdale, N. (1996). Availability of Visual and Proprioceptive Afferent Messages and Postural Control in Elderly Adults. Exp. Brain Res. 108, 121. doi:10.1007/BF00242910
Holmes, N. P., and Spence, C. (2004). The Body Schema and Multisensory Representation(s) of Peripersonal Space. Cogn. Process. 5, 94–105. doi:10.1007/s10339-004-0013-3
Ivanenko, Y. P., Grasso, R., and Lacquaniti, F. (2000). Influence of Leg Muscle Vibration on Human Walking. J. Neurophysiol. 84, 1737–1747. doi:10.1152/jn.2000.84.4.1737
Iwata, H. (2013). “Locomotion Interfaces,” in Human Walking in Virtual Environments: Perception, Technology, and Applications, Berlin: Springer, 199–219. doi:10.1007/978-1-4419-8432-6_9
Jerald, J., and Whitton, M. (2009). “Relating Scene-Motion Thresholds to Latency Thresholds for Head-Mounted Displays,” in Proceedings IEEE Virtual Real, 211–218. doi:10.1109/VR.2009.4811025
Kalckert, A., and Ehrsson, H. H. (2012). Moving a Rubber Hand that Feels like Your Own: A Dissociation of Ownership and Agency. Front. Hum. Neurosci. 6, 40. doi:10.3389/fnhum.2012.00040
Keshner, E. A., and Lamontagne, A. (2021). The Untapped Potential of Virtual Reality in Rehabilitation of Balance and Gait in Neurological Disorders. Front. Virtual Real. 2, 114. doi:10.3389/frvir.2021.641650
Kilteni, K., Groten, R., and Slater, M. (2012a). The Sense of Embodiment in Virtual Reality. Presence: Teleoperators and Virtual Environments 21, 373–387. doi:10.1162/pres_a_00124
Kilteni, K., Normand, J.-M., Sanchez-vives, M. V., and Slater, M. (2012b). Extending Body Space in Immersive Virtual Reality: A Very Long Arm Illusion. PLoS ONE 7, e40867. doi:10.1371/journal.pone.0040867
Kokkinara, E., Kilteni, K., Blom, K. J., and Slater, M. (2016). First Person Perspective of Seated Participants over a Walking Virtual Body Leads to Illusory Agency over the Walking. Sci. Rep. 6, 28879. doi:10.1038/srep28879
Kokkinara, E., and Slater, M. (2014). Measuring the Effects through Time of the Influence of Visuomotor and Visuotactile Synchronous Stimulation on a Virtual Body Ownership Illusion. Perception 43, 43–58. doi:10.1068/p7545
Leonardis, D., Frisoli, A., Barsotti, M., Carrozzino, M., and Bergamasco, M. (2014). Multisensory Feedback Can Enhance Embodiment within an Enriched Virtual Walking Scenario. Presence: Teleoperators and Virtual Environments 23, 253–266. doi:10.1162/PRES_a_00190
Liu, L. Y., Sangani, S., Patterson, K. K., Fung, J., and Lamontagne, A. (2020). Real-Time Avatar-Based Feedback to Enhance the Symmetry of Spatiotemporal Parameters after Stroke: Instantaneous Effects of Different Avatar Views. IEEE Trans. Neural Syst. Rehabil. Eng. 28, 878–887. doi:10.1109/TNSRE.2020.2979830
Lopez, S., Yang, Y., Beltran, K., Kim, S. J., Cruz Hernandez, J., Simran, C., Yang, B., and Yuksel, B. F. (2019). “Investigating Implicit Gender Bias and Embodiment of white Males in Virtual Reality with Full Body Visuomotor Synchrony,” in Conference. Human. Factors Comput. System. Proceedings, 1–12. doi:10.1145/3290605.3300787
Maister, L., Sebanz, N., Knoblich, G., and Tsakiris, M. (2013). Experiencing Ownership over a Dark-Skinned Body Reduces Implicit Racial Bias. Cognition 128, 170–177. doi:10.1016/j.cognition.2013.04.002
Maister, L., Slater, M., Sanchez-Vives, M. V., and Tsakiris, M. (2015). Changing Bodies Changes Minds: Owning Another Body Affects Social Cognition. Trends Cogn. Sci. 19, 6–12. doi:10.1016/j.tics.2014.11.001
Maselli, A., Kilteni, K., López-Moliner, J., and Slater, M. (2016). The Sense of Body Ownership Relaxes Temporal Constraints for Multisensory Integration. Sci. Rep. 6, 30628. doi:10.1038/srep30628
Matsuda, Y., Nakamura, J., Amemiya, T., Ikei, Y., and Kitazaki, M. (2020). “Perception of Walking Self-Body Avatar Enhances Virtual-Walking Sensation,” in 2020 IEEE Conference on Virtual Reality and 3D User Interfaces (Atlanta, Georgia, USA), 733–734. doi:10.1109/VRW50115.2020.00215
Meehan, M., Hill, U., Whitton, M. C., Hill, U., Brooks, F. P., and Hill, U. (2002). Effect of Latency on Presence in Stressful Virtual Environments, Berlin: Springer. doi:10.1145/566570.566630
Moore, J. W. (2016). What Is the Sense of agency and Why Does it Matter?. Front. Psychol. 7, 1–9. doi:10.3389/fpsyg.2016.01272
Nilsson, N. C., Serafin, S., Steinicke, F., and Nordahl, R. (2018). Natural Walking in Virtual Reality. Comput. Entertain. 16, 1–22. doi:10.1145/3180658
Norman, G. (2010). Likert Scales, Levels of Measurement and the “Laws” of Statistics. Adv. Health Sci. Educ. 15, 625–632. doi:10.1007/s10459-010-9222-y
Normand, J.-M., Giannopoulos, E., Spanlang, B., and Slater, M. (2011). Multisensory Stimulation Can Induce an Illusion of Larger Belly Size in Immersive Virtual Reality. PLoS One 6, e16128. doi:10.1371/journal.pone.0016128
Peck, T. C., and Gonzalez-Franco, M. (2021). Avatar Embodiment. A Standardized Questionnaire. Front. Virtual Real. 1, 1–12. doi:10.3389/frvir.2020.575943
Petkova, V. I., and Ehrsson, H. H. (2008). If I Were You: Perceptual Illusion of Body Swapping. PLoS One 3, e3832. doi:10.1371/journal.pone.0003832
Roll, J. P., and Vedel, J. P. (1982). Kinaesthetic Role of Muscle Afferents in Man, Studied by Tendon Vibration and Microneurography. Exp. Brain Res. 47, 177–190. doi:10.1007/BF00239377
Roth, D., and Latoschik, M. E. (2020). Construction of the Virtual Embodiment Questionnaire (VEQ). IEEE Trans. Vis. Comput. Graphics 26, 3546–3556. doi:10.1109/TVCG.2020.3023603
Samaraweera, G., Rongkai Guo, R., and Quarles, J. (2013). “Latency and Avatars in Virtual Environments and the Effects on Gait for Persons with Mobility Impairments,” in IEEE Symp. 3D User Interface 2013, 3DUI 2013—Proc, 23–30. doi:10.1109/3DUI.2013.6550192
Schulze, S., Pence, T., Irvine, N., and Guinn, C. (2019). “The Effects of Embodiment in Virtual Reality on Implicit Gender Bias,” in Lecture Notes in Computer Science Including Subseries Lecture Notes in Artificial Intelligence and Lecture Notes in Bioinformatics, 361–374. doi:10.1007/978-3-030-21607-8_28
Slater, M., Perez-Marcos, D., Ehrsson, H. H., and Sanchez-Vives, M. V. (2008). Towards a Digital Body: The Virtual Arm Illusion. Front. Hum. Neurosci. 2, 1–8. doi:10.3389/neuro.09.006.2008
Slater, M., Spanlang, B., Sanchez-Vives, M. V., and Blanke, O. (2010). First Person Experience of Body Transfer in Virtual Reality. PLoS One 5, e10564. doi:10.1371/journal.pone.0010564
Spanlang, B., Normand, J.-M., Borland, D., Kilteni, K., Giannopoulos, E., PomÃs, A. S., et al. (2014). How to Build an Embodiment Lab: Achieving Body Representation Illusions in Virtual Reality. Front. Robot. AI 1, 1–22. doi:10.3389/frobt.2014.00009
Sullivan, G. M., and Artino, A. R. (2013). Analyzing and Interpreting Data from Likert-type Scales. J. Grad. Med. Educ. 5, 541–542. doi:10.4300/jgme-5-4-18
Tajadura-Jiménez, A., Banakou, D., Bianchi-Berthouze, N., and Slater, M. (2017). Embodiment in a Child-like Talking Virtual Body Influences Object Size Perception, Self-Identification, and Subsequent Real Speaking. Sci. Rep. 7, 1–12. doi:10.1038/s41598-017-09497-3
Tapin, A., Duclos, N., and Duclos, C. (2019). “Perception of Gait Movements Using Gait-like Vibrations in Individuals with and without Sensorimotor Deficits,” in International Society of Posture and Gait Research (Scotland: Edimburgh)
Taylor, M. W., Taylor, J. L., and Seizova-Cajic, T. (2017). Muscle Vibration-Induced Illusions: Review of Contributing Factors, Taxonomy of Illusions and User’s Guide. Multisens. Res. 30, 25–63. doi:10.1163/22134808-00002544
Thyrion, C., and Roll, J.-P. (2010). Predicting Any Arm Movement Feedback to Induce Three-Dimensional Illusory Movements in Humans. J. Neurophysiol. 104, 949–959. doi:10.1152/jn.00025.2010
Tsakiris, M., and Haggard, P. (2005). The Rubber Hand Illusion Revisited: Visuotactile Integration and Self-Attribution. J. Exp. Psychol. Hum. Perception Perform. 31, 80–91. doi:10.1037/0096-1523.31.1.80
Tsakiris, M., Prabhu, G., and Haggard, P. (2006). Having a Body versus Moving Your Body: How agency Structures Body-Ownership. Conscious. Cogn. 15, 423–432. doi:10.1016/j.concog.2005.09.004
Vuillerme, N., Danion, F., Forestier, N., and Nougier, V. (2002). Postural Sway under Muscle Vibration and Muscle Fatigue in Humans. Neurosci. Lett. 333, 131–135. doi:10.1016/S0304-3940(02)00999-0
Walsh, L. D., Moseley, G. L., Taylor, J. L., and Gandevia, S. C. (2011). Proprioceptive Signals Contribute to the Sense of Body Ownership. J. Physiol. 589, 3009–3021. doi:10.1113/jphysiol.2011.204941
Whitton, M. C., and Razzaque, S. (2008). Locomotion Interfaces, 107, 146. doi:10.1016/B978-0-12-374017-5.00004-3
Willaert, I., Aissaoui, R., Nadeau, S., Duclos, C., and Labbe, D. R. (2020). “Modulating the Gait of a Real-Time Self-Avatar to Induce Changes in Stride Length during Treadmill Walking Mean Stride Length for a 10° Increase in Hip Flexion,” in 2020 IEEE Conference on Virtual Reality and 3D User Interfaces (IEEEVR), 719–720. doi:10.1109/VRW50115.2020.00208
Keywords: virtual reality, proprioception, embodiment, agency, gait, muscle vibration
Citation: Labbe DR, Kouakoua K, Aissaoui R, Nadeau S and Duclos C (2021) Proprioceptive Stimulation Added to a Walking Self-Avatar Enhances the Illusory Perception of Walking in Static Participants. Front. Virtual Real. 2:557783. doi: 10.3389/frvir.2021.557783
Received: 30 April 2020; Accepted: 18 May 2021;
Published: 02 June 2021.
Edited by:
David Swapp, University College London, United KingdomReviewed by:
Solène Neyret, ParisTech École Nationale Supérieure d’Arts et Métiers, FranceDaniel Roth, University of Erlangen Nuremberg, Germany
Copyright © 2021 Labbe, Kouakoua, Aissaoui, Nadeau and Duclos. This is an open-access article distributed under the terms of the Creative Commons Attribution License (CC BY). The use, distribution or reproduction in other forums is permitted, provided the original author(s) and the copyright owner(s) are credited and that the original publication in this journal is cited, in accordance with accepted academic practice. No use, distribution or reproduction is permitted which does not comply with these terms.
*Correspondence: David R. Labbe, ZGF2aWQubGFiYmVAZXRzbXRsLmNh