- 1School of Microbiology, University College Cork, Cork, Ireland
- 2Synthesis and Solid State Pharmaceutical Centre, University College Cork, Cork, Ireland
- 3School of Applied Psychology, University College Cork, Cork, Ireland
- 4Centre for the Integration of Research Teaching and Learning, University College Cork, Cork, Ireland
- 5APC Microbiome Ireland, Cork, Ireland
- 6School of Computer Science and Information Technology, University College Cork, Cork, Ireland
- 7MAVRIC Research Lab, School of Computer Science and Information Technology, University College Cork, Cork, Ireland
The rapidly expanding biotechnology sector horizon is expected to create a surge in demand for expertise underpinning cell and gene therapies, which are recognized as the next generation of medicines. New and innovative approaches to implement active and performative learning in the Molecular Life Sciences are required to support this and to address limitations associated with traditional “front of class” lectern delivery of challenging, three dimensional molecular concepts. Therefore, an immediate need exists for the development and implementation of immersive learning approaches in Virology, Cellular Sciences and Molecular Biology to underpin sustainable development of graduate students for academic and industrial research careers. The Covid-19 pandemic has led to significant changes in the delivery of education globally, with online engagement and accelerated uptake of novel teaching and assessment modalities into majority practice within institutions. This development has been driven by externally imposed necessity and it remains to be seen what form teaching and learning will take post-Covid. Irrespective of the pandemic, technologies are available which can serve intrinsically motivated, discipline specific shifts toward enhanced learner experiences and learning outcomes. Immersive virtual reality offers one such approach to open new entry points for student learning of abstract molecular concepts, which will be just as relevant upon our return to face-to-face teaching. Key to delivering this will be engagement and collaboration by disciplinary and technical experts. Here, we discuss global advances in the area of VR and Molecular Science education and assess potential paths forward for teaching and learning impact and innovative education.
Introduction
There has been a long history of anecdotal evidence for the challenges around teaching and learning in molecular biology (Tibell and Rundgren, 2010). The Teaching for Understanding (TfU) framework aims to take generative topics, knowledge that is central to a discipline, and allow teachers to better understand what students know, for example through structured tasks known as performances of understanding (Blythe and Perkins, 1998; Wiske, 1998). The content of molecular life sciences is inherently complex, highly abstract, and deeply rooted in diverse disciplines ranging from biology and math, to medicine and agriculture, through to philosophy and ethics (Tibell and Rundgren, 2010). Therefore, new approaches have been needed for some time to engage students in this important discipline, and to design new entry points for the students to explore and engage in deep learning for these challenging concepts. There is an emerging viewpoint in cognition and learning that suggests the body and the mind are intrinsically linked with respect to learning. It follows therefore that the inability of students to directly experience the molecular world may explain many of the documented learning difficulties in molecular sciences e.g. various aspects of genetics, cell structure and function, and macromolecular structure, as well as issues related to size and scale (Bell, 2001; Tibell and Rundgren, 2010).
Apart from the abstract nature of molecular biology, and the lack of a tactile spatially oriented reference point for students, there is also a large gap between what is known by practicing molecular life scientists and what is taught about the domain in secondary schools and universities (Howitt et al., 2008). This gap is closing as research-led and research-based teaching find their way into the curriculum in many Universities, and yet the technologies underpinning molecular and cellular biology continue to advance apace. Also changing is the dynamic between lecturer and student, underpinned by the realization that the centuries old practice of the professor at the lectern cannot be sustained, nor was it ever ideal. A rich tapestry of investigative studies has revealed important insights into the learning experience that have guided new approaches to knowledge construction and the facilitation of deep learning (Sanchez-Sepulveda et al., 2019).
Virtual Reality Technologies and the Student Experience
Before addressing the utility of VR in education, it is first important to define the terms used in the industry and to acknowledge the variety of means through which one can engage in a VR experience. Arriving at a concise definition of VR’s key characteristics is challenging due to the rapidly evolving nature of the technology. In the broad sense, VR can be categorized as desktop (D-VR, also referred to as a 2D simulation) and immersive VR (I-VR, which is typically multi-modal by nature and provides a headset experience in 360°).
While there is some evidence to suggest that the specifications of the headsets are not paramount, with low-end headsets producing comparable learning outcomes to higher-end equipment (Selzer et al., 2019), there are important considerations in matching the technology requirements with the learning goals. Essentially, the level of immersion is constrained by the physical properties of the system (Slater, 2009), and a more immersive experience can lend itself to a higher degree of implicit learning (Slater and Wilbur, 1997). The Oculus Quest offers an immersive experience, and its compact self-contained form-factor enables users to operate independent of a fixed space. The HTC Vive Pro Eye and Vive Focus Plus headsets work through a desktop computer system (as with the Oculus Rift) and as such requires a dedicated space to be developed for its use. This becomes challenging where the group dynamic is favored, with self-paced learning more difficult to deliver in this setting, and the requirement for space being a significant issue. In contrast, the HoloLens offers an Augmented Reality (AR) experience and has very clear benefits for use in the exploration of molecular constructs.
Factors that have been described to influence the user experience with VR have included age, gender, sensation-seeking tendency, personal innovativeness, and geographical location (Suh and Prophet, 2018). Coxon and colleagues reported that younger users are more likely to be engaged with an AR experience than older users (Coxon et al., 2016), while Plechatá and co-workers also described how older users were more likely to engage with a non-immersive experience (Plechatá et al., 2019). In contrast, other studies have reported no age-related difference with respect to the immersive VR experience (Alelis et al., 2015). Age dependent differences in the teaching and learning experience with immersive technologies have also been reported in primary school children (Fransson et al., 2020). Research on this important aspect of the user experience is ongoing (McGlynn et al., 2018). From the perspective of gender, Tan and Waugh investigated the use of VR in molecular biology in secondary schools in Singapore and found a positive impact on male students with respect to achievement (Tan and Waugh, 2013). The issue of gender divergence with respect to learning through the VR modality will be an important research area in the development of VR immersive experiences, particularly from an academic accessibility perspective (Tan, 2014). It is important not to extrapolate this work however, merely to be aware of the potential bias that may exist in the design of the VR experiences. It is clear however that gender is a significant factor in the design and universality of VR immersive experiences. A recent study highlighted how males performed better when the avatar was a drone, rendered as a futuristic robot. On the other hand, females fared better when the avatar was a female scientist (Makransky et al., 2019). This highlights the importance of social considerations in addition to pedagogical and technological barriers when seeking to optimize user experience and engagement. Another study reported a greater propensity to cybersickness among females (Stanney et al., 2020).
It should be noted that the virtual experience of students in their learning can occur without the need for headsets. Non-immersive 2D virtual simulations may prove more suitable to some students. Therefore, it is important to stress that this approach does not suggest a “one size fits all” solution. Rather, the aim is to integrate the various virtual approaches into a complete package of student education that reaches all individuals within the class, rather than designing for “the average student”, who simply does not exist. Consultation with students during the design phase is key to achieving flexibility in design and operation that will result in an educational experience that is as inclusive as possible. As a simple example, perhaps students could choose the gender of their avatar before they start.
Immersive Learning of Challenging Molecular Concepts Through Virtual Reality
Apart from the interactive nature of VR, and the ability for the immersed learner to engage with a visual object in real time, there is also the added value of being able to interact with multiple frames of reference (Leung et al., 2018; Alalwan et al., 2020). This could be of value in the teaching of molecular and cellular biology where concepts are difficult to comprehend since they are abstract, three-dimensional, and lack real life referents to which learners can anchor their understanding. Concepts related to recombinant DNA technology and heterologous expression e.g., the functional components of plasmids and protein production/secretion, can be difficult to engage with in a 2-dimensional frame. Student paced exploration of these and other molecular aspects such as viral and cellular structures in 3D could offer new entry points to learners, providing a spatial context that cannot be achieved from a computer screen (Johnston et al., 2018). Immersive visualization may also have benefits in research led learning, with Knote and colleagues reporting the benefits of its use in the study of multi-cellular tumor spheroids (Knote et al., 2019) and crystallographic diffraction data (Knote et al., 2020). This has been recognized in other disciplines, whereby the use of VR demonstrated significant benefits to the student learning experience addressing challenging concepts in affine transformations through gamification (Oberdörfer and Latoschik, 2019a).
Early studies exploring the relationship between VR and learning reported that, on average, 66% of students in groups exposed to virtual simulations (ranging from pre-school to higher education) outperformed those in their respective control groups (Lee, 1999). Of course, the technology has advanced significantly since then and there has been an increase in studies that address the use of mixed reality and virtual systems in teaching and learning in recent years (Kamińska et al., 2018; Cook et al., 2019). In general, these studies have reported better understanding and higher motivation, among other benefits (Hernández-de-Menéndez et al.). Spatial design or otherwise dangerous environments have become a principal focus for the learning context in Higher Education (Dede and Richards, 2017; Cook et al., 2019). Many of these studies focus on the enhanced engagement linked to the two profound affordances of VR: 1) the sense of presence, and 2) the embodied affordances of gesture and manipulation in the third dimension (Johnson-Glenberg, 2018). Presence [sometimes referred to as place illusion or the sense of being there (Slater 2009)], is a behavioral trait that reflects the extent to which the user considers the VR experience to be a place visited rather than a set of images seen (Slater and Wilbur, 1997). Achieving presence has been shown to impact directly on the degree to which learning is achieved (Stevens and Kincaid, 2015). It should be noted that defining presence can be a difficult task, and while there have been efforts to develop objective correlates of presence, the challenge remains to be addressed (Skarbez et al., 2017). Another illusion, that of embodiment, describes how the form of the virtual body or avatar can result in implicit changes in attitudes, perception and cognition, and changes in behavior (Slater, 2017). Embodiment and the provision of an embodied agent can facilitate implicit learning, an important trait in the educational experience (Slater, 2017). It will be fascinating to see how both presence and embodiment impact on the learner experience with respect to construction or exploration of molecular structures and how immersion in complex, dynamic, and three-dimensional structures and relationships via sensory aids such as haptics will enhance the depth of learning achieved.
Tang and colleagues reported an improved performance in spatial design in students that adopted a mixed reality approach to learning when compared to the control cohort (Tang et al., 2020). Practical guides have been presented for the use of VR in teaching of structural biology, with particular emphasis on protein modelling (Garcia-Bonete et al., 2018; Cassidy et al., 2020). An interesting feature of VR engagement is the need for, and positive outcome, of pre-training, which has been shown to increase knowledge, transfer, and self-efficacy only in VR (Meyer et al., 2019) (Table 1). Medical education in particular has been an early adopter of these technologies (Pottle, 2019), highlighted through the Medicine Meets VR (MMVR) conference series and an extensive body of research work. Zhao and colleagues reporting increased post-intervention scores in the teaching of anatomy when compared with other teaching modalities (Zhao et al., 2020). Fairén and colleagues reported similar outcomes when they presented their VR4Health platform (Fairén et al., 2020). However, there are significant limitations to the data currently available in the literature. Most of these studies only featured a single institution and data reported is typically an objective measurement of learning, i.e., test score or completion times. It is interesting to note the absence of self-assessment in these studies, perhaps a gap in the literature that can be addressed in the current proposed research project. Peer reviewed studies in the use of VR for teaching of virology, cell culture and molecular biology are rare in the literature, but some recent reports have begun to address that (Bennett and Saunders, 2019; Coan et al., 2020).
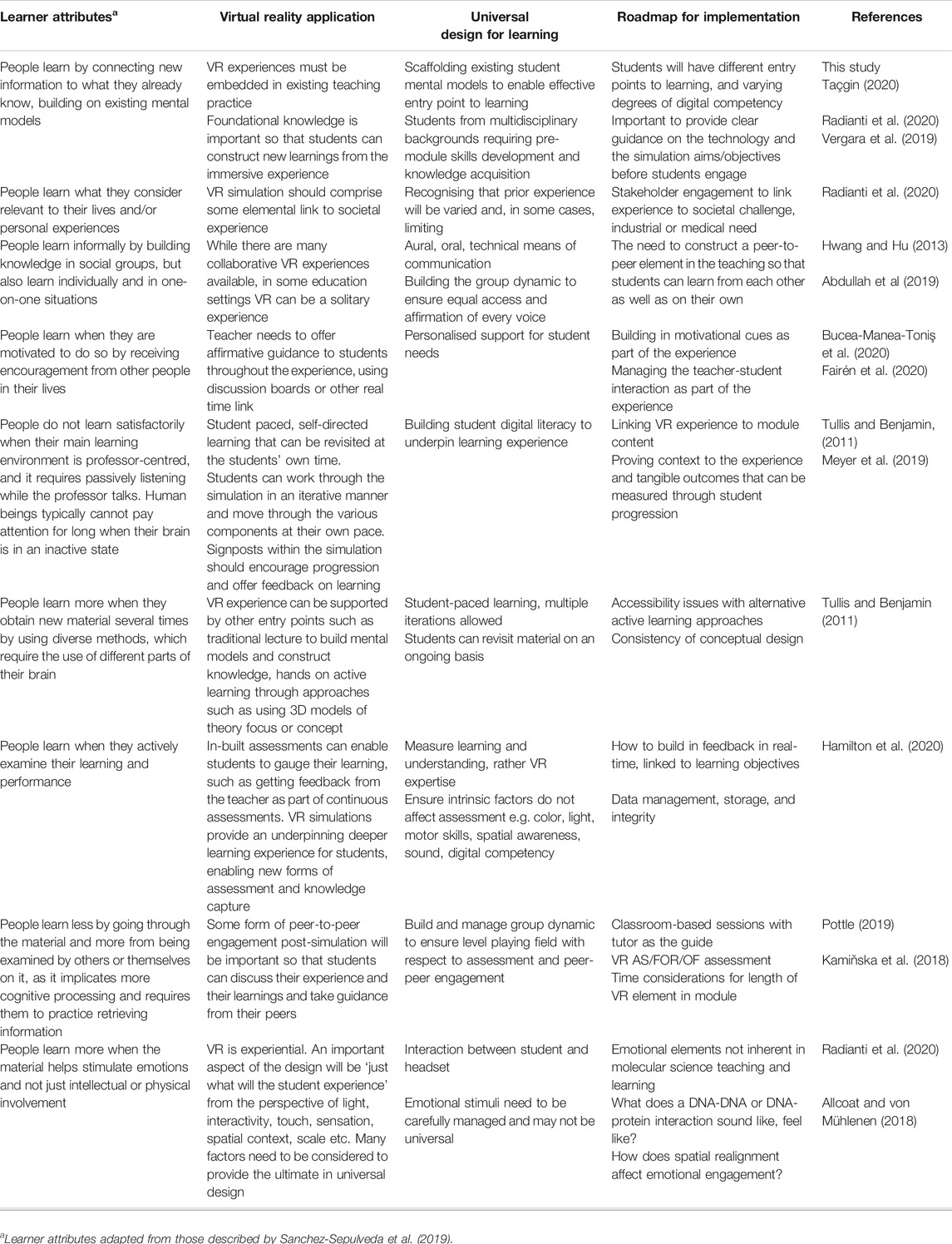
TABLE 1. Summary of VR applications and the challenges faced in implementing them to address keystone learner attributes.
A key challenge to the implementation of VR in the study of abstract molecular concepts is understanding how it could enhance the student’s capacity to achieve a deep understanding of the learning outcomes (Table 1). Indeed, research focusing on learning outcomes, intervention characteristics, and assessment measures associated with VR use has been limited thus far (Hamilton et al., 2020). It is clear from studies that a step-by-step protocol system whereby each stage is explained and worked through with the students is key to successfully achieving long term retention of knowledge (Vergara et al., 2019) (Table 1). It is equally clear that challenges exist in the adoption of this technology with issues around safe handling and operation (Potkonjak et al., 2016) and the requirement for updating of software and content by the lecturer or design team (Vergara et al., 2020). Notwithstanding the benefits, or perhaps because of the challenges, the adoption of immersive VR learning approaches in teaching and learning in STEM remains low (Stains et al., 2018).
Virtual Reality and Learning Theory
The active learning situational experience provided by VR lends itself to several learning theory elements, principally constructivism, cognitivism, and behaviorism. Constructivist philosophy favors the learning-by-doing approach and is based on the theory that knowledge is constructed through an individual’s interaction with the environment. It is not just the interaction with the environment but the integration of knowledge (new and old) via exploration of the environment, e.g., knowledge is constructed via the interaction of prior knowledge, the exploration of ideas within a contextualised environment, and that facilitates the integration of new knowledge (Piaget, 1971; Tobias and Duffy, 2009). According to the learning theory proposed by Lave (Lave and Wenger, 1991), learning is situated in a specific context or physical environment. This goes against the conventional thinking of the time where learning was equated with abstract knowledge in a classroom, a time when learning was equated with a transmission model of teaching, in which the student passively received knowledge from the 'expert' teacher. The VR experience can be designed to embed learning within the activity and context in which it occurs (Table 1). Furthermore, it can scaffold the situational learning experience in an authentic framework through which the student can grow experientially through the learning process. This can be supported by the provision of an agent which can compensate for the lack of a real person and create an illusion of perspective in the experience (Slater, 2017; Bucher et al., 2018).
Embodied cognition holds that the mind alone does not construct knowledge, but rather it is mind-body axis around movement and thought in a particular environment that delivers the knowledge construct to the learner. Perhaps this can explain, in part, one of the issues faced in teaching and learning theoretical aspects of virology, cellular sciences and molecular biology. The abstract nature of the topic gives no reference point to the learner, no hands-on experiential understanding with which to engage the topic. It is thought by some that the inability of the learner to directly experience the molecular world may explain many of the documented learning difficulties in molecular sciences (Schönborn and Anderson, 2010; Tibell and Rundgren, 2010). VR can address this imbalance and enable the learner to experience visually and interactively the molecular content, in a spatial immersive context within which they can “see”’ the otherwise invisible parts. Visualization has long been considered key to unlocking the complexity of molecular structures (Kozma and Russell, 2005). While the benefits of VR in embodied cognition theory might be inherently obvious, one might argue the impact on social cognitive theory might be less apparent. Social cognitive theory is based on interactive work, peer-to-peer learning, the idea that people learn from observing others. While collaborative experiences are possible in VR, the VR experience can be individualistic, based on users occupying a solitary space where they can engage with the simulation. As such, one of the challenges in VR design for academic contexts is incorporating some level of peer-to-peer engagement so that learning can be enhanced. However, VR simulations have been used to increase social awareness in users, particularly where learners might require such interventions. Didehbani and colleagues showed how VR could be used to enhance social skills, social cognition, and social functioning in young adults with autism spectrum disorder (Didehbani et al., 2016). Often, lecturers can be unaware of the personal histories of their students and designing accessible and compatible VR simulations is an important consideration.
While the VR experience is primarily best represented by constructivist and cognitive theories, the educational requirements for VR design also lend to situations of behaviorism theory (Chen, 2009). This is true where discrete short modules of experience are built to enable the student to revisit concepts or theories over a period of time. Behaviorism holds to the theory of knowledge reproduction and this approach will be another important aspect of VR design in the education sector. VR may also provide an opportunity for academics to explore non-disciplinary models in their scholarship of teaching and learning. For example, the clarify, ideate, develop, implement creative design model espoused by David Goligorsky can be facilitated through VR, particularly where students become co-designers of their learning process (Goligorsky, 2012).
Research Methodologies and Immersive Virtual Reality
Capturing the true impact of VR on the teaching and learning landscape has been challenging, and novel approaches to research methodology may be required for this experiential technology. Ambrose states that learning is a process and not a product (Ambrose et al., 2010), and it follows that one must understand each element of that process before one can understand its true impact. Many research studies have focused primarily on pre- and post-testing of students; however, there are constraints to this approach within an academic institution where ethical constraints pervade. One must also consider that VR by its very nature challenges the conceptual definition of a learning environment insofar as one could never envisage a lecture room in the shape of a complex protein structure. In assessing the pedagogical utility of VR in teaching and learning, one must seek to capture data on age, task difficulty, applications, experience, engagement, broader learning capacity, follow on application of new knowledge and ability to extend learning to related theory, amongst other aspects. This complexity raises the issue of the pre-existing data and the importance of understanding the limitations in how the studies that populate the literature were designed. Hamilton and colleagues reported that 69% of studies they evaluated featured random allocation between control and experimental groups, meaning 31% could only be described as quasi-experimental (Hamilton et al., 2020). The authors considered the greatest weakness in the studies presented to date to be the validity of the evaluation instrumentation used to assess the learning outcomes. Methodological descriptions on the design of quizzes, tests, or questionnaires were limited, and validation of these approaches was absent in 66% of the studies (Hamilton et al., 2020). Therefore, careful consideration of the research design and, in particular, how success of these approaches can be assessed is needed to address the central question of how VR can best be integrated within the curriculum for virology and molecular/cellular biology in higher education (Figure 1).
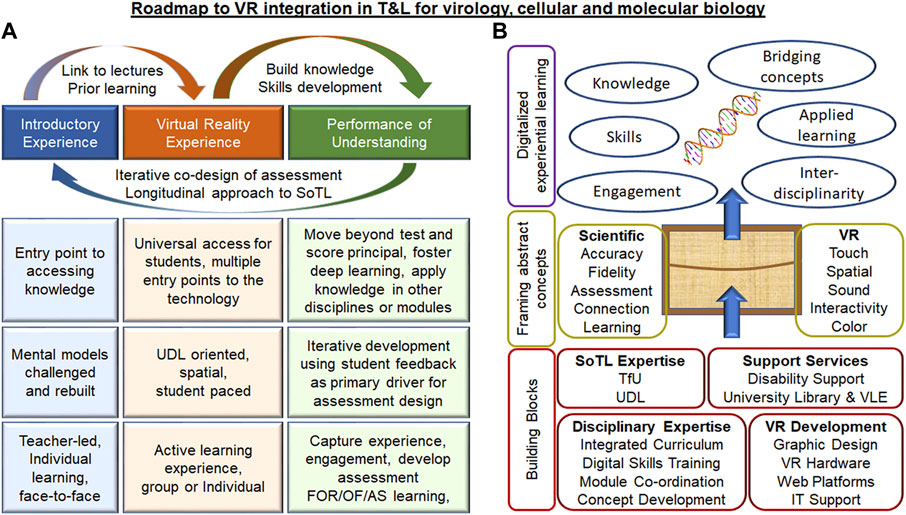
FIGURE 1. Overview of the key considerations underpinning the effective integration and implementation of immersive learning technologies in teaching and learning of challenging molecular concepts. 1) Modular roadmap bridging the introductory experience with a performance of understanding, whereby a VR experience acts as a throughline upon which to scaffold the overall learning experience. 2) The collaborative expertise required to successfully design and integrate a VR experience within modular content highlights the true potential of this multi-disciplinary approach to T&L in higher education.
Discussion
If this literature review was written at the end of 2019 how different the context would have been. Twelve months ago may well be seen as a utterly distinct teaching landscape before Covid-19 (Rospigliosi, 2020). All appears changed but one wonders whether the switch to online and blended learning will be retained given the external motivator. Xie and colleagues previously proposed that institutional change requires a sustained, threshold commitment of a 10% minority (Xie et al., 2011). Perhaps with the unprecedented challenge of Covid-19 has come the parallel opportunity to herald a new dawn for interactive learning, where the student becomes the focus, and the independent learner is fostered and encouraged. One can only hope.
In summarizing and providing a context for the work to follow, there are two elements to be considered: 1) the literature from the perspective of scholarship of teaching and learning i.e. what the research tells us about VR and immersive learning and 2) how common mixed reality and virtual systems are in the teaching and learning of challenging scientific concepts. In both elements, it is clear that while VR and immersive education will not replace classical teaching modalities, they offer a real opportunity for the enhancement of same, and the opening of new horizons. It is equally clear that there is a strong bias in the literature towards the use of VR for practical lab teaching. The use of VR for teaching of challenging theoretical concepts remains largely unexplored. This has huge potential to support and underpin the move towards multi-disciplinary research and could cross conventional disciplinary boundaries in teaching and learning. Knowledge gaps between computer science experts and expert biologists can be seen as challenges to collaboration. An ideal platform for bridging this gap is working together to create immersive VR learning experiences for the students we teach; learning from each other as we go. The anticipated surge in global demand for Advanced Therapy Medicinal Products is likely to be paralleled by increased digitalization of production processes. Collaboration between Computational Scientists and Life/Biological Scientists in Higher Education, particularly in the areas of Virology, Cellular Sciences and Molecular Biology is needed now to prepare both disciplines for the road ahead.
Research at University College Cork in Ireland is currently ongoing into the application of virtual simulations for the teaching and learning of challenging concepts in the molecular sciences. Enhanced Active LEarning in Virology, cell culture and moleculAr biotechnology (ELEVATE) seeks to develop a roadmap for integration of virtual and active learning approaches into existing and new curricula. ELEVATE represents an interdisciplinary team comprised of experts in Microbiology, Virology, Computer Science, Applied Psychology, and the Centre for the Integration of Research, Teaching, and Learning at UCC, who together have a shared vision for how VR can enable students to explore the spatial and structural complexity of e.g., viruses and next generation molecular systems. Funded through the National Forum for the Enhancement of Teaching and Learning in Higher Education in Ireland, the team have developed a series of bespoke desktop and immersive VR simulations for the teaching of virus structure, heterologous expression, recombinant plasmids and green chemistry solutions for the bioeconomy. The team adopt a co-design approach in partnership with students and disciplinary experts to create pedagogically robust learning experiences with embedded assessments. A pilot survey (n = 22) completed by third year students taking a microbiology module at UCC prior to the co-design of the bespoke VR simulations showed that 88% of respondents could see potential in the use of digital technologies. This was further supported by 79% of respondents who indicated that they learn well through active learning that involves visual engagement. Somewhat surprising was the finding that less than 15% of respondents declared competency or expertise in the use of VR technologies. Together, these data highlight the huge potential for VR integration into curricula, but also the need for strong support systems to underpin student learning through this medium (Table 1). These and other initiatives in this space will deliver a new entry point for learning for students, and new horizons of teaching approaches for academics to adopt as part of their practice. Developments in the field of molecular sciences are fast paced, leading to the introduction of new concepts into the curriculum on an ongoing basis. Keeping pace with these developments will require an equally agile and progressive approach to VR development in order to sustain the adoption of these exciting technologies in Higher Education.
Data Availability Statement
The original contributions presented in the study are included in the article/Supplementary Material, further inquiries can be directed to the corresponding author.
Ethics Statement
The studies involving human participants were reviewed and approved by Social Research Ethics Committee (SREC) University College Cork, Cork, Ireland. The participants provided their written informed consent to participate in this study.
Author Contributions
Conceptualization: FR, OJ, BM, JM, DM, NO’L, MS. Funding: FR, OJ, JM, NO’L, MS. FR wrote the first draft of this manuscript. All authors contributed to the writing and editing of the final manuscript.
Funding
The ELEVATE team acknowledge the support of the National Forum for the Enhancement of Teaching and Learning in Higher Education (TL19UCC1481/02). FR also acknowledges the support of Science Foundation Ireland (SSPC-3, 12/RC/2275_2), the Health Research Board (HRB-ILP-POR-2019-004), and the Health Research Board/Irish Thoracic Society (MRCG-2018-16).
Conflict of Interest
The authors declare that the research was conducted in the absence of any commercial or financial relationships that could be construed as a potential conflict of interest.
Acknowledgments
The authors thank the National Forum for the Enhancement of Teaching and Learning in Higher Education and the Centre for the Integration of Research Teaching and Learning at University College Cork for continuing support and engagement.
References
Abdullah, J., Mohd-Isa, W. N., and Samsudin, M. A. (2019). Virtual Reality to Improve Group Work Skill and Self-Directed Learning in Problem-Based Learning Narratives. Virtual Reality 23, 461–471. doi:10.1007/s10055-019-00381-1
Alalwan, N., Cheng, L., Al-Samarraie, H., Yousef, R., Ibrahim Alzahrani, A., and Sarsam, S. M. (2020). Challenges and Prospects of Virtual Reality and Augmented Reality Utilization Among Primary School Teachers: A Developing Country Perspective. Stud. Educ. Eval. 66, 100876. doi:10.1016/j.stueduc.2020.100876
Alelis, G., Bobrowicz, A., and Ang, C. S. (2015). Comparison of Engagement and Emotional Responses of Older and Younger Adults Interacting with 3D Cultural Heritage Artefacts on Personal Devices. Behav. Inf. Tech. 34 (11), 1064–1078. doi:10.1080/0144929X.2015.1056548
Allcoat, D., and von Mühlenen, A. (2018). Learning in Virtual Reality: Effects on Performance, Emotion and Engagement. Res. Learn. Technol. 26. doi:10.25304/rlt.v26.2140
Ambrose, S. A., Bridges, M. W., DiPietro, M., Lovett, M. C., and Norman, M. K. (2010). How Learning Works. Hoboken, NJ, USA: John Wiley & Sons.
Bell, E. (2001). The Future of Education in the Molecular Life Sciences. Nat. Rev. Mol. Cel. Biol. 2, 221–225. doi:10.1038/35056610
Bennett, J. A., and Saunders, C. P. (2019). A Virtual Tour of the Cell: Impact of Virtual Reality on Student Learning and Engagement in the STEM Classroom. J. Microbiol. Biol. Educ. 20 (2), 20. doi:10.1128/jmbe.v20i2.1658
Blythe, T., and Perkins, D. (1998). “Understanding Understanding,” in The Teaching for Understanding Guide. Editor T. Blythe (San Francisco: Jossey-Bass), 9–16.
Bucea-Manea-Toniş, R., Bucea-Manea-Toniş, R., Simion, V. E., Ilic, D., Braicu, C., and Manea, N. (2020). Sustainability in Higher Education: The Relationship between Work-Life Balance and XR E-Learning Facilities. Sustainability 12 (14), 5872. doi:10.3390/su12145872
Bucher, K., Blome, T., Rudolph, S., and von Mammen, S. (2018). Vreanimate Ii: Training First Aid and Reanimation in Virtual Reality. J. Comput. Educ. 6, 53–78. doi:10.1007/s40692-018-0121-1
Cassidy, K. C., Šefčík, J., Raghav, Y., Chang, A., and Durrant, J. D. (2020). ProteinVR: Web-Based Molecular Visualization in Virtual Reality. Plos Comput. Biol. 16 (3), e1007747. doi:10.1371/journal.pcbi.1007747
Chen, C. (2009). Theoretical Bases for Using Virtual Reality in Education. Themes Sci. Technol. Educ. 2, 71–90.
Coan, H. A., Goehle, G., and Youker, R. T. (2020). Teaching Biochemistry and Molecular Biology with Virtual Reality — Lesson Creation and Student Response. J. Teach. Learn. –Special Issue Digit. Learn. High. Educat. 14 (1), 71–92. doi:10.22329/jtl.v14i1.6234
Cook, M., Lischer-Katz, Z., Hall, N., Hardesty, J., Johnson, J., McDonald, R., et al. (2019). Challenges and Strategies for Educational Virtual Reality. Ital 38 (4), 25–48. doi:10.6017/ital.v38i4.11075
Coxon, M., Kelly, N., and Page, S. (2016). Individual Differences in Virtual Reality: Are Spatial Presence and Spatial Ability Linked?. Virtual Reality 20, 203–212. doi:10.1007/s10055-016-0292-x
Dede, C., and Richards, J. (2017). “Glossary of Realities' Terms,” in Virtual, Augmented, and Mixed Realities in Education. Editors D. Liu, C. Dede, and J. Richards (Berlin: Springer-Verlag), 5–11.
Didehbani, N., Allen, T., Kandalaft, M., Krawczyk, D., and Chapman, S. (2016). Virtual Reality Social Cognition Training for Children with High Functioning Autism. Comput. Hum. Behav. 62, 703–711. doi:10.1016/j.chb.2016.04.033
Fairén, M., Moyés, J., and Insa, E. (2020). VR4Health: Personalized Teaching and Learning Anatomy Using VR. J. Med. Syst. 44 (5), 94. doi:10.1007/s10916-020-01550-5
Fransson, G., Holmberg, J., and Westelius, C. (2020). The Challenges of Using Head Mounted Virtual Reality in K-12 Schools From a Teacher Perspective. Educ. Inf. Technol. 25, 3383–3404. doi:10.1007/s10639-020-10119-1
Garcia-Bonete, M.-J., Jensen, M., and Katona, G. (2018). A Practical Guide to Developing Virtual and Augmented Reality Exercises for Teaching Structural Biology. Biochem. Mol. Biol. Educ. 47 (1), 16–24. doi:10.1002/bmb.21188
Hamilton, D., McKechnie, J., Edgerton, E., and Wilson, C. (2020). Immersive Virtual Reality as a Pedagogical Tool in Education: a Systematic Literature Review of Quantitative Learning Outcomes and Experimental Design. J. Comput. Educ. 8, 1–32. doi:10.1007/s40692-020-00169-2
Hernández-de-Menéndez, M., Vallejo Guevara, A., and Morales-Menendez, R. (2019). Virtual Reality Laboratories: A Review of Experiences. Int. J. Interact. Des. Manuf. 13, 947–966. doi:10.1007/s12008-019-00558-7
Howitt, S., Anderson, T., Costa, M., Hamilton, S., and Wright, T. (2008). A Concept Inventory for Molecular Life Sciences: How Will it Help Your Teaching Practice?. Aust. Biochemist 39 (3), 14–17.
Hwang, W.-Y., and Hu, S.-S. (2013). Analysis of Peer Learning Behaviors Using Multiple Representations in Virtual Reality and Their Impacts on Geometry Problem Solving. Comput. Edu. 62, 308–319. doi:10.1016/j.compedu.2012.10.005
Johnson-Glenberg, M. C. (2018). Immersive VR and Education: Embodied Design Principles that Include Gesture and Hand Controls. Front. Robot. AI 5, 81. doi:10.3389/frobt.2018.00081
Johnston, A. P. R., Rae, J., Ariotti, N., Bailey, B., Lilja, A., Webb, R., et al. (2018). Journey to the centre of the Cell: Virtual Reality Immersion into Scientific Data. Traffic 19 (2), 105–110. doi:10.1111/tra.12538
Kamińska, M. S., Miller, A., Rotter, I., Szylińska, A., and Grochans, E. (2018). The Effectiveness of Virtual Reality Training in Reducing the Risk of Falls Among Elderly People. Clin. Interv. Aging 13, 2329–2338. doi:10.2147/CIA.S183502
Knote, A., Fischer, S. C., Cussat-Blanc, S., Niebling, F., Bernard, D., Cogoni, F., et al. (2019). “Immersive Analysis of 3D Multi-Cellular In-Vitro and In-Silico Cell Cultures,” in 2019 IEEE International Conference on Artificial Intelligence and Virtual Reality (AIVR), 82–827. doi:10.1109/AIVR46125.2019.00021
Knote, A., von Mammen, S., Gao, Y. Y., and Thorn, A. (2020). “Immersive Analysis of Crystallographic Diffraction Data,” in 26th ACM Symposium on Virtual Reality Software and Technology (VRST'20), Virtual Event, Canada, November 1–4, 2020. New York, NY: ACM. doi:10.1145/3385956.3422097
Kozma, R., and Russell, J. (2005). “Students Becoming Chemists: Developing Representationl Competence,” in Visualization in Science Education. Models and Modeling in Science Education. Editor J. K. Gilbert (Dordrecht: Springer), 121–145. doi:10.1007/1-4020-3613-2_8
Lave, J., and Wenger, E. (1991). Situated Learning: Legitimate Peripheral Participation. Cambridge, UK: Cambridge University Press. doi:10.1017/cbo9780511815355
Lee, J. (1999). Effectiveness of Computer-Based Instructional Simulation: A Meta Analysis. Int. J. Instruct. Med. 26, 71–86.
Leung, T., Zulkernine, F., and Isah, H. (2018). The Use of Virtual Reality in Enhancing Interdisciplinary Research and Education. arXiv: 1809.08585v1.
Makransky, G., Wismer, P., and Mayer, R. E. (2019). A Gender Matching Effect in Learning with Pedagogical Agents in an Immersive Virtual Reality Science Simulation. J. Comput. Assist. Learn. 35 (3), 349–358. doi:10.1111/jcal.12335
McGlynn, S. A., Sundaresan, R. M., and Rogers, W. A. (2018). Investigating Age-Related Differences in Spatial Presence in Virtual Reality. Proc. Hum. Factors Ergon. Soc. Annu. Meet. 62 (1), 1782–1786. doi:10.1177/1541931218621404
Meyer, O. A., Omdahl, M. K., and Makransky, G. (2019). Investigating the Effect of Pre-training when Learning through Immersive Virtual Reality and Video: a media and Methods experiment. Comput. Edu. 140, 103603. doi:10.1016/j.compedu.2019.103603
Oberdörfer, S., and Latoschik, M. E. (2019a). Knowledge Encoding in Game Mechanics: Transfer-Oriented Knowledge Learning in desktop-3D and VR. Int. J. Comp. Games Tech. 2019, 1–17. doi:10.1155/2019/7626349
Piaget, J. (1971). “The Theory of Stages in Cognitive Development,” in GB Flamer, Measurement and Piaget. Editors DR. Green, and MP. Ford (McGraw-Hill).
Plechatá, A., Sahula, V., Fayette, D., and Fajnerová, I. (2019). Age-Related Differences with Immersive and Non-immersive Virtual Reality in Memory Assessment. Front. Psychol. 10, 1330. doi:10.3389/fpsyg.2019.01330
Potkonjak, V., Gardner, M., Callaghan, V., Mattila, P., Guetl, C., Petrović, V. M., et al. (2016). Virtual Laboratories for Education in Science, Technology, and Engineering: A Review. Comput. Edu. 95, 309–327. doi:10.1016/j.compedu.2016.02.002
Pottle, J. (2019). Virtual Reality and the Transformation of Medical Education. Future Healthc. J. 6 (3), 181–185. doi:10.7861/fhj.2019-0036
Rospigliosi, P. a. (2020). How the Coronavirus Pandemic May Be the Discontinuity Which Makes the Difference in the Digital Transformation of Teaching and Learning. Interactive Learn. Environments 28 (4), 383–384. doi:10.1080/10494820.2020.1766753
Radianti, J., Majchrzak, T. A., Fromm, J., and Wohlgenannt, I. (2020). A Systematic Review of Immersive Virtual Reality Applications for Higher Education: Design Elements, Lessons Learned, and Research Agenda. Comput. Educ. 147, 103778. doi:10.1016/j.compedu.2019.103778
Sanchez-Sepulveda, M. V., Torres-Kompen, R., Fonseca, D., and Franquesa-Sanchez, J. (2019). Methodologies of Learning Served by Virtual Reality: A Case Study in Urban Interventions. Appl. Sci. 9 (23), 5161. doi:10.3390/app9235161
Schönborn, K. J., and Anderson, T. R. (2010). Bridging the Educational Research-Teaching Practice gap. Biochem. Mol. Biol. Educ. 38 (5), 347–354. doi:10.1002/bmb.20436
Selzer, M. N., Gazcon, N. F., and Larrea, M. L. (2019). Effects of Virtual Presence and Learning Outcome Using Low-End Virtual Reality Systems. Displays 59, 9–15. doi:10.1016/j.displa.2019.04.002
Skarbez, R., Brooks, Jr., F. P., and Whitton, M. C. (2018). A Survey of Presence and Related Concepts. ACM Comput. Surv. 50, 1–39. doi:10.1145/3134301
Slater, M. (2017). “Implicit Learning through Embodiment in Immersive Virtual Reality,” in Virtual, Augmented, and Mixed Realities in Education. Editors D. Liu, C. J. Dede, R. Huang, and J. Richards (Singapoore: Springer, Smart Computing and Intelligence).
Slater, M. (2009). Place Illusion and Plausibility Can lead to Realistic Behaviour in Immersive Virtual Environments. Phil. Trans. R. Soc. B 364, 3549–3557. doi:10.1098/rstb.2009.0138
Slater, M., and Wilbur, S. (1997). A Framework for Immersive Virtual Environments (Five): Speculations on the Role of Presence in Virtual Environments. Presence: Teleoperators & Virtual Environments 6, 603–616. doi:10.1162/pres.1997.6.6.603
Stains, M., Harshman, J., Barker, M. K., Chasteen, S. V., Cole, R., DeChenne-Peters, S. E., et al. (2018). Anatomy of STEM Teaching in North American Universities. Science 359, 1468–1470. doi:10.1126/science.aap8892
Stanney, K., Fidopiastis, C., and Foster, L. (2020). Virtual Reality Is Sexist: But it Does Not Have to Be. Front. Robot. AI 7, 4. doi:10.3389/frobt.2020.00004
Stevens, J. A., and Kincaid, J. P. (2015). The Relationship between Presence and Performance in Virtual Simulation Training. OJMSi 03, 41–48. doi:10.4236/ojmsi.2015.32005
Suh, A., and Prophet, J. (2018). The State of Immersive Technology Research: A Literature Analysis. Comput. Hum. Behav. 86, 77–90. doi:10.1016/j.chb.2018.04.019
Tan, S. (2014). “Gender Differences in Learning Molecular Biology Using Virtual Learning Environments,” in Simulations, Serious Games and Their Applications. Editors Yiyu Cai,, and Sui Lin Goei (Singapore: Springer). doi:10.4135/978144627305013514692
Tan, S., and Waugh, R. (2013). “Use of Virtual-Reality in Teaching and Learning Molecular Biology,” in Use of Virtual-Reality in Teaching and Learning Molecular Biology (Springer), 17–43. doi:10.1007/978-981-4021-90-6_2
Tang, Y. M., Au, K. M., Lau, H. C. W., Ho, G. T. S., and Wu, C. H. (2020). Evaluating the Effectiveness of Learning Design with Mixed Reality (MR) in Higher Education. Virtual Reality 24, 797–807. doi:10.1007/s10055-020-00427-9
Taçgın, Z. (2020). The Perceived Effectiveness Regarding Immersive Virtual Reality Learning Environments Changes by the Prior Knowledge of Learners. Educ. Inf. Technol. 25, 2791–2809. doi:10.1007/s10639-019-10088-0
Tibell, L. A. E., and Rundgren, C.-J. (2010). Educational Challenges of Molecular Life Science: Characteristics and Implications for Education and Research. Lse 9 (1), 25–33. doi:10.1187/cbe.08-09-0055
Tobias, S., and Duffy, T. M. (2009). Constructivist Instruction: Success or Failure?. Routledge/Taylor & Francis Group.
Tullis, J. G., and Benjamin, A. S. (2011). On the Effectiveness of Self-Paced Learning. J. Mem. Lang. 64 (2), 109–118. doi:10.1016/j.jml.2010.11.002
Vergara, D., Extremera, J., Rubio, M. P., and Dávila, L. P. (2019). Meaningful Learning through Virtual Reality Learning Environments: A Case Study in Materials Engineering. Appl. Sci. 9, 4625. doi:10.3390/app9214625
Vergara, D., Extremera, J., Rubio, M. P., and Dávila, L. P. (2020). The Technological Obsolescence of Virtual Reality Learning Environments. Appl. Sci. 10 (3), 915. doi:10.3390/app10030915
Wiske, M. S. (1998). Teaching for Understanding: Linking Research with Practice. San Francisco, CA: Jossey-Bass.
Xie, J., Sreenivasan, S., Korniss, G., Zhang, W., Lim, C., and Szymanski, B. K. (2011). Social Consensus through the Influence of Committed Minorities. Phys. Rev. E 84 (1), 011130. doi:10.1103/PhysRevE.84.011130
Keywords: virtual reality, molecular biology, virology, cellular biology, immersive learning
Citation: Reen FJ, Jump O, McSharry BP, Morgan J, Murphy D, O’Leary N, O’Mahony B, Scallan M and Supple B (2021) The Use of Virtual Reality in the Teaching of Challenging Concepts in Virology, Cell Culture and Molecular Biology. Front. Virtual Real. 2:670909. doi: 10.3389/frvir.2021.670909
Received: 22 February 2021; Accepted: 07 May 2021;
Published: 21 May 2021.
Edited by:
Benjamin Weyers, University of Trier, GermanyReviewed by:
Rajiv Khadka, Idaho National Laboratory (DOE), United StatesSebastian Oberdörfer, Julius Maximilian University of Würzburg, Germany
Copyright © 2021 Reen, Jump, McSharry, Morgan, Murphy, O’Leary, O’Mahony, Scallan and Supple. This is an open-access article distributed under the terms of the Creative Commons Attribution License (CC BY). The use, distribution or reproduction in other forums is permitted, provided the original author(s) and the copyright owner(s) are credited and that the original publication in this journal is cited, in accordance with accepted academic practice. No use, distribution or reproduction is permitted which does not comply with these terms.
*Correspondence: F. Jerry Reen, ai5yZWVuQHVjYy5pZQ==