- 1Department of Physical Therapy, Rehabilitation Science and Athletic Training, University of Kansas Medical Center, Kansas City, KS, United States
- 2Institute for Rehabilitation Science and Engineering, Madonna Rehabilitation Hospitals, Lincoln, NE, United States
- 3Department of Health and Rehabilitation Sciences, University of Nebraska Medical Center, Omaha, NE, United States
Virtual reality (VR) gaming is promising in sustaining children’s participation during intensive physical rehabilitation. This study investigated how integration of a custom active serious gaming with a robot-motorized elliptical impacted children’s perception of engagement (Intrinsic Motivation Inventory), physiologic effort (i.e., exercise speed, heart rate, lower extremity muscle activation), and joint kinematics while overriding the motor’s assistance. Compared to Non-VR condition, during the VR-enhanced condition participants’ perceived engagement was 23% greater (p = 0.01), self-selected speed was 10% faster (p = 0.02), heart rate was 7% higher (p = 0.08) and muscle demands increased. Sagittal plane kinematics demonstrated only a small change at the knee. This study demonstrated that VR plays an essential role in promoting greater engagement and physiologic effort in children performing a cyclic locomotor rehabilitation task, without causing any adverse events or substantial disruption in lower extremity joint kinematics. The outcomes of this study provide a foundation for understanding the role of future VR-enhanced interventions and research studies that weigh/balance the need to physiologically challenge a child during training with the value of promoting task-related training to help promote recovery of walking.
Introduction
Following a severe neurologic injury or illness, intensive task-specific practice is often integrated therapeutically to improve walking and/or fitness in children with disabilities (Richards et al., 1993; Katz-Leurer et al., 2003; Hubbard et al., 2009; Salem et al., 2012). The Intelligently Controlled Assistive Rehabilitation Elliptical (ICARE, SportsArt, Taiwan) is a motorized elliptical that enables training of gait-like movements for individuals with disabilities and chronic conditions (Burnfield et al., 2011; Nelson et al., 2011; Irons et al., 2015). The motor assistance provided by this device helps guide leg movements as users train at speeds up to 65 cycles per minute. Training faster than the speed set on the console causes the motor to disengage and promotes greater physiologic challenge for lower extremity muscles and the heart (Burnfield et al., 2017; Burnfield et al., 2019). The device has recently been adapted to train children as young as three years of age with physical disabilities and special healthcare needs (Nelson et al., 2015; Burnfield et al., 2017; Burnfield et al. 2018; Burnfield et al. 2019; Burnfield et al. 2021). However, one practical challenge is encouraging children to exert the greater effort required to override the motor’s assistance without external cueing from a clinician, educator, or caregiver.
Serious gaming or virtual reality (VR) offers a promising approach for promoting exercise engagement (Salem et al., 2012; Abdulsatar et al., 2013; Salem and Elokda 2014; Deutsch and Westcott McCoy 2017). VR can immerse participants in scenarios full of vivid visual stimulation and provides valuable, functionally relevant sensory input such as perceptions of visual/optic flow during treadmill walking (Walker et al., 2010). Although VR would be expected to improve children’s engagement (Kim, 2011; Schuler et al., 2011), its overall impact on physiologic variables that clinicians often seek to challenge during training (e.g., heart rate, HR, or muscle demands/electromyography, EMG) is not well documented and remains controversial. For instance, a 30-min interactive cycling video game demonstrated a significant increase in healthy adults’ energy expenditure and enjoyment (Monedero et al., 2015) while after a 10-min active game of bicycling, heart rate reserve did not show significant changes between children with cerebral palsy and the age-matched typically developing controls (Robert et al., 2013). Unknown is whether physiologic responses during VR-enhanced ICARE training would exceed responses generated simply by asking children to override the motor’s assistance. Additionally, it is not clear how integration of non-immersive VR gaming with ICARE would influence lower extremity joint kinematics during ICARE exercise.
The purpose of this study was to identify how integration of non-immersive VR gaming with the pediatric-modified ICARE impacts children’s perception of engagement, physiologic effort and joint kinematics while overriding the motor’s assistance. We hypothesized that when overriding ICARE’s motor-assistance, participants’ perceived engagement, self-selected training speed, EMG and HR demands would be greater during the VR versus Non-VR condition due to children exerting more effort to capture VR rewards. Despite exerting greater effort, we hypothesized that participants’ sagittal plane joint kinematics would not differ significantly between the VR and Non-VR conditions due to the end-effector guidance provided by the pedals. Understanding the impact of VR on children’s engagement (motivational and physiologic effort) and the impact on joint motions is critical to guiding clinical practice approaches that may weigh/balance the need to physiologically challenge a child during training with the value of promoting task-related training to help promote recovery of walking.
Methods
Participants
Ten typically developing children between 7 and 12 years of age and free from any diagnosed disability were recruited through convenience sampling from regional schools and neighborhoods. Participants and their guardians were provided with a detailed explanation of the study and signed assent and consent forms approved by the Institutional Review Board of Madonna Rehabilitation Hospitals prior to participation.
Procedures
During the initial visit, each participant used the modified ICARE for three minutes with the motor’s assistance. Self-selected comfortable speed and stride length were determined for each child (Burnfield et al., 2017). After determining comfortable settings, children used the ICARE for three minutes with VR activated and were instructed to over-ride the device’s motor assistance. Participants were instructed to hold the ICARE’s stationary triangular handle throughout the session to control for variability that might arise if using different hand holds available on the device.
During the second visit, wireless surface EMG electrodes were affixed over seven lower extremity muscles of the dominant leg. Then, participants completed isometric manual muscle testing for each muscle against the resistance of a researcher (Hislop and Montgomery 2007). Testing procedures for the gastrocnemius were modified to include a single unilateral heel raise against downward pressure exerted on the participant’s shoulders. Reflective markers were affixed over the iliac crest, posterior superior iliac spine, anterior superior iliac spine, greater trochanter, medial and lateral femoral condyles, medial and lateral malleoli, posterior heel, first metatarsal, between the distal second and third metatarsals, distal lateral fifth metatarsal and the lateral border of the midfoot. Additional tracking marker cluster plates were secured on the thigh and lower leg (Burnfield et al., 2010; Burnfield et al., 2017). Then, participants performed five 10-m walks across a level surface at their self-selected comfortable speed and five trials at their self-selected fast speed. Next, participants performed two ICARE trials which differed based on the presence of a VR immersive environment. Instructions to participants preceding the Non-VR trial were to “Push as fast as you can” and prior to the VR-enhanced trial to “Push as fast as you can to collect as many carrots as possible.” Each condition lasted three minutes. The order of ICARE trial performance was randomized using a random numbers generator. Participants were provided an opportunity to rest between trials and hydrate as desired. To quantify participants’ perceived engagement, the Intrinsic Motivation Inventory (IMI) questionnaire was administered immediately after the Non-VR and VR-enhanced trials.
Instrumentation and Outcome Measures
The ICARE was modified to increase usability by children as young as three years of age including decreasing the lower limit for stride length and integrating height adjustable pedals so children could interact at eye level with the console (Nelson et al., 2015; Burnfield et al., 2019). The ICARE was used throughout the study, including the Non-VR and VR-enhanced conditions.
Figure 1A illustrates the framework that combines a serious video game development, asset creation through 3D modeling, embedded systems, and computer networking. In specific, the current system utilizes a speed sensor to detect the revolutions per minute (RPM) of the flywheel on ICARE. The speed sensor is wired to a GPIO board on a Raspberry Pi 3 (Raspberry Pi Foundation, United Kingdom) based on the signals received. The calculated RPM was sent via Bluetooth communication to the computer system which ran the serious game.
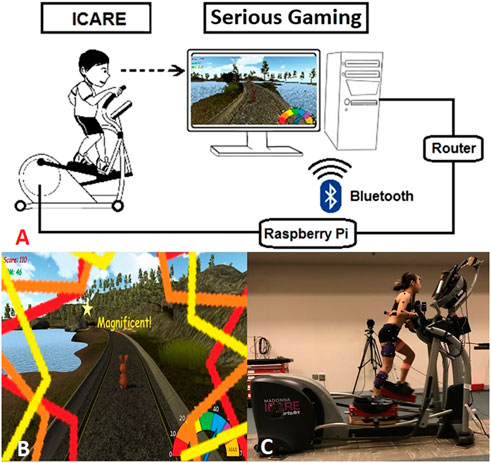
FIGURE 1. (A) The conceptual framework of non-immersive VR-ICARE (top). (B) The monitor positioned at eye-level in front of the ICARE console delivered positive visual and auditory feedback in the VR-enhanced gaming environment while a bunny collected carrots (bottom left). (C) Kinematic and electromyographic data were recorded simultaneously as participant used a modified ICARE during the VR-enhance'd' condition (bottom right).
To encourage mass practice, engagement and effort during exercise on the ICARE, a non-immersive VR gaming interface that consists of a virtual bunny moving along an outdoor trail and collecting carrots was developed using Unity Technology (San Francisco, CA) and integrated with ICARE (Figure 1B). The objective of the non-immersive VR serious gaming was to obtain as many carrots as possible within three minutes by controlling the virtual bunny’s speed. The bunny’s moving speed was again synchronized with ICARE’s flywheel speed (RPM) via Bluetooth communication. If a child pushed the pedals faster than their self-selected comfortable speed, then the bunny’s speed accelerated while the bunny’s speed decelerated if a child slowed their cycling rate. Based on inputs from clinicians, children, parents and researchers prior to initiation of the study, elements were added to the VR gaming environment to facilitate children’s engagement (e.g., presenting overall score on the VR console, real-time visual/auditory feedback when a carrot was collected, and a colorful speedometer reflecting the bunny’s current movement speed). Exercise HR was detected using a Polar heart rate monitor (Lake Success, NY) and automatically recorded every 20-s through a custom data logger (Pfeifer et al., 2019). Wireless EMG electrodes (Delsys, Natick, MA) recorded seven targeted muscles’ activation at 2000 Hz (i.e., gluteus maximus, gluteus medius, lateral hamstrings, medial hamstrings, vastus lateralis, gastrocnemius, and tibialis anterior; Figure 1C). Lower extremity kinematics during overground walking and ICARE conditions were recorded using 12 Oqus 300 series cameras (120 Hz; Qualisys Motion Analysis System, Sweden).
The Intrinsic Motivation Inventory (IMI) used a 7-point Likert scale to rate participants’ level of agreement with a series of questions related to the Non-VR and VR-enhanced trials (e.g., enjoyment and interest) (Schuler et al., 2011).
Data reduction and analysis
Ten representative strides from the self-selected fast walking and 10 from the final minute of each ICARE condition were used to calculate lower extremity joint kinematics and muscle demands. Foot center of velocity defined gait cycle timing during walking (O'Connor et al., 2007) while pedal marker trajectories defined cycle phasing during ICARE training (i.e., pedal’s most anterior position defined the start of each successive cycle).
Kinematic marker trajectories were processed in Visual 3D software (C-Motion, Inc. Germantown, MD). Marker trajectories were filtered (fourth-order Butterworth low-pass filter, 6 Hz cutoff frequency) and thigh (thigh relative to vertical), hip, knee and ankle joint angles were calculated. Ensemble averages of each condition’s joint angles were created from the time normalized gait cycles. The coefficient of multiple correlations (CMC) was used to assess the similarity between ICARE and fast walking ensemble averaged hip, thigh, knee and ankle kinematic profiles. A CMC value that approaches one suggests a strong level of similarity between ICARE’s and fast walking’s movement profiles, while a value approaching zero reflects less similarity (Kadaba et al., 1989; Burnfield et al., 2017).
EMG signals were also processed in Visual 3D software. First, the signals were adjusted for DC bias, filtered (60 Hz notch filter and 10–350 Hz band pass filter), full wave rectified, and integrated (0.01 s intervals). Then, muscle activity was normalized to the maximum 0.5 s moving window of the manual muscle test (% MMT). Onsets and cessation of muscle activity were calculated for envelopes of EMG that exceeded 5% amplitude of the MMT and 5% duration of the movement cycle. When envelopes of EMG were separated by less than 5% of the movement cycle, they were combined for calculating duration and expressed as a % of the movement cycle (% cycle). EMG peaks (maximum 0.03 s moving window average) and means (average of all data meeting onset criteria above) were averaged from 10 representative strides of the data for each participant and condition.
Total IMI scores for each condition were calculated. Seven represented the lowest total score, while 49 represented the highest level of perceived engagement/enjoyment.
Statistical analysis
If data met assumptions of normality (Shapiro-Wilk test), then paired t-tests were used to compare IMI, exercise speed (i.e., RPM), HR, EMG, and relative similarity of joint kinematics to normal gait between Non-VR and VR-enhanced conditions. For data not meeting the assumption of normality, the Wilcoxon signed-rank test was adopted. Statistical analyses were conducted using SigmaPlot 11.0 (Systat Software Inc., Palo Alto, CA), and all the significance levels were determined as α = 0.05.
Results
All recruited children (mean age = 9.8 ± 1.1 years; height = 145.3 ± 8.3 cm; mass = 37.1 ± 5.9 kg; six girls) completed the trials without any documented adverse events.
Compared to Non-VR condition, during the VR-enhanced condition participants’ perceived engagement (IMI) was 23% greater (p = 0.01), self-selected speed while overriding the motor’s assistance was 10% faster (p = 0.02), and HR was 7% higher (p = 0.08; Table 1). Muscle demands (EMG peak, mean and duration) were higher during VR compared to the Non-VR condition in 17 of 21 comparisons (Table 2), however only 4 of these comparisons achieved statistical significance (gluteus medius duration, lateral hamstrings duration, vastus lateralis mean and duration; p ≤ 0.04). CMC values (Figure 2) were similar between the Non-VR and VR-enhanced conditions at the hip [Non-VR = 0.89 (SD = 0.05) vs. VR = 0.88 (SD = 0.04); p = 0.56], thigh [Non-VR = 0.93 (IQR = 0.90-0.96) vs. VR = 0.94 (IQR = 0.93–0.95); p = 0.46], and ankle [Non-VR = 0.61 (SD = 0.11) vs. VR = 0.61 (SD = 0.12); p = 0.97]. However, small CMC differences at the knee were significant between conditions [Non-VR = 0.89 (SD = 0.04) vs. VR = 0.87 (SD = 0.04); p = 0.02].

TABLE 1. Comparison of impact of exercising without virtual reality (Non-VR) and with virtual reality (VR) on participants’ perceived engagement (IMI), self-selected exercise training speed (RPM), and heart rate (beats/minute) while overriding ICARE’s motor assistance.
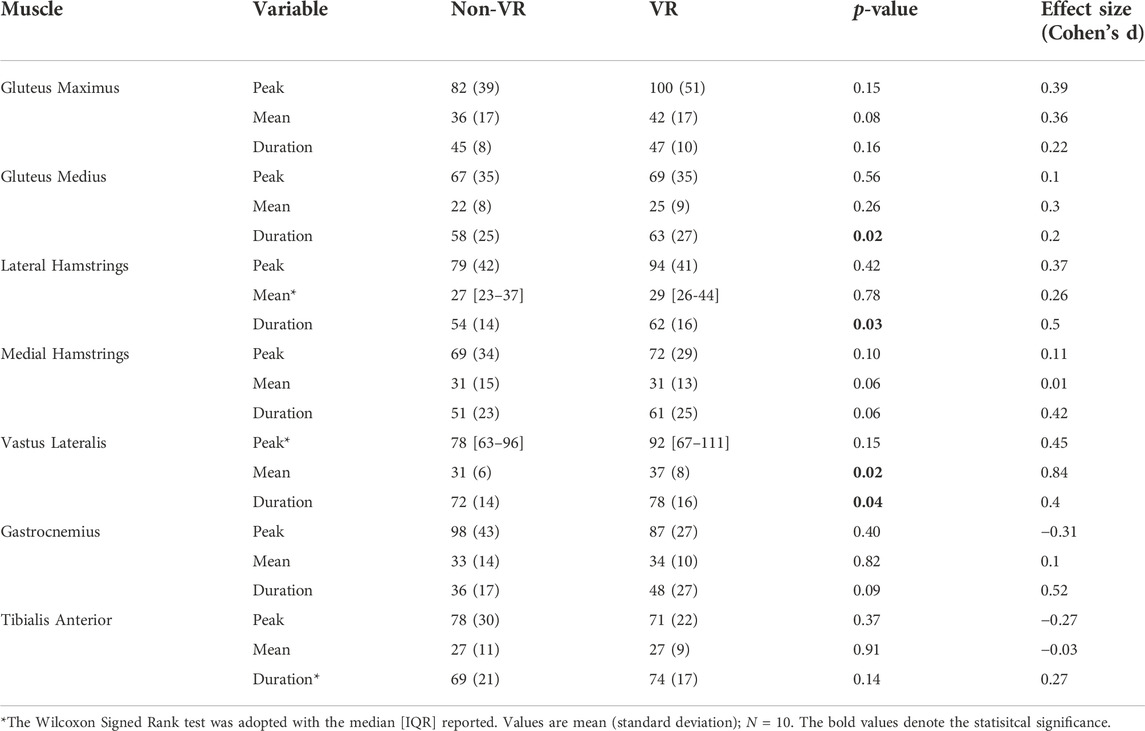
TABLE 2. Comparisons of peak (% MMT), mean (% MMT), and duration (% cycle) of muscle activity recorded during ICARE exercise while overriding ICARE’s motor assistance with and without virtual reality (VR).
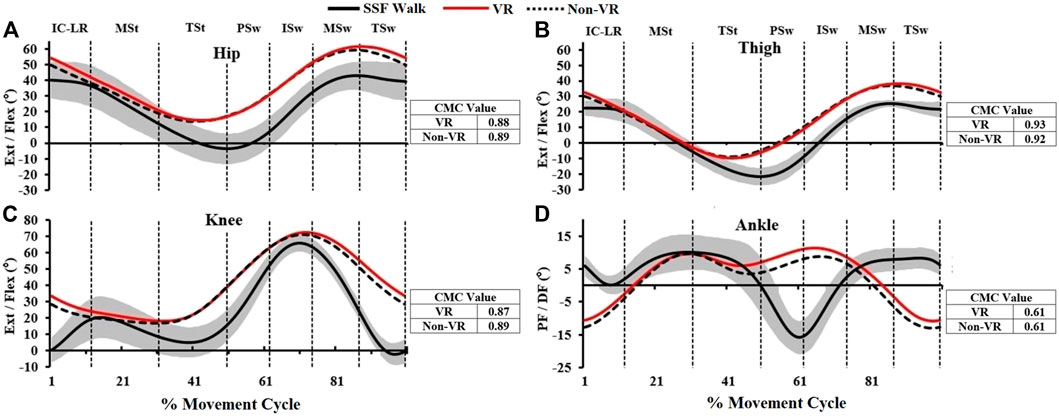
FIGURE 2. The ensemble sagittal plane joint kinematics (degrees) of hip, thigh, knee, and ankle (A–D) of this study which include 1) fast overground walking (SSF Walk; black line = mean; gray band = ± one standard deviation), 2) exercise while overriding motor’s assistance with VR (solid red line) and without VR gaming (dashed line). The coefficient of multiple correlation (CMC) represents joint kinematic similarity between VR/Non-VR vs. SSF Walk, respectively (IC: Initial Contact, LR: Loading Response, MSt: Mid Stance, TSt: Terminal Stance, PSw: Pre-Swing, ISw: Initial Swing, MSw: Mid Swing, TSw: Terminal Swing).
Discussion
While VR gaming offers an exciting tool for enhancing a patient’s level of interaction, immersion, motivation and effort during therapy (You et al., 2005; Deutsch and Westcott McCoy 2017; Deutsch et al., 2021), the practicalities of implementing VR gaming across different age groups and with varying therapeutic devices is not well understood. This pilot study suggests that a non-immersive VR gaming can play a role in promoting greater engagement and physiologic effort in children performing a cyclic locomotor rehabilitation task guided only by end-effectors, without causing substantial disruption in sagittal plane joint kinematics.
The impact of virtual reality gaming on intrinsic motivation inventory, revolutions per minute, HR and EMG
Consistent with our first set of hypotheses, children were more engaged and selected to train faster during VR-enhanced ICARE training compared to the Non-VR condition. Often times, clinicians and/or caregivers are tasked with maintaining children’s engagement in therapeutic exercise activities, using tools such as verbal encouragement and/or rewards for successful performance. We found that VR-enhanced ICARE training resulted in greater physiologic effort (significantly greater EMG, trend towards higher HR) compared to when VR was not employed. Unknown is whether incorporation of verbal encouragement from clinicians and/or caregivers could lead to even greater physiologic challenge. Previous research suggests there may be a potentiating effect of combining a clinician’s verbal encouragement with VR on lower extremity muscle activation during robot-assisted gait training in children with and without walking impairments (Schuler et al., 2011).
Self-selected training speed when overriding the motor’s assistance was 10% faster during the VR-enhanced compared to the Non-VR condition, a finding expected to have important clinical implications. Research from the neurosciences has pointed to the importance of performing many step-like movements each day to not only improve walking, but also promote lasting neuroplastic change (Hirsch et al., 2016; Shimada et al., 2017). In the current study, the 10% faster training speed during VR-enhanced ICARE training equated to a similar increase in number of step-like movements compared to the Non-VR condition. Further research is required to determine if children are able to sustain higher training speeds during longer sessions and whether increases in steps within and across sessions enhances recovery of walking.
Participants were able to train faster during VR-enhanced ICARE training owing, in part, to an increase in lower extremity muscle effort that helped advance the pedals more rapidly. While most muscles evaluated except the tibialis anterior displayed a trend towards greater activity during the VR-enhanced compared to the Non-VR condition, only three muscles displayed statistically significant differences between conditions. The greater mean activity and duration of vastus lateralis arising during VR compared to Non-VR training suggests that integration of VR might provide clinicians with a means of further challenging a muscle critical for stability and shock absorption during weight acceptance of gait. Likewise, the longer duration of gluteus medius activity during the VR compared to Non-VR condition, suggests enhanced capacity during the VR condition to build strength in a muscle that plays an important role in maintaining frontal plane stability during the stance period of gait. Finally, the longer duration activity of the lateral hamstrings during the VR condition might suggest an opportunity to build capacity needed to control/restrain thigh advancement during late swing (Perry and Burnfield 2010). Further ICARE and VR research should integrate a larger sample size to determine if trends identified in muscle activation patterns achieve statistical significance in children with and without disabilities.
The heart plays a critical role supporting sustained muscle effort. It is likely that the 7% faster heart rate observed during the VR-enhanced compared to the Non-VR condition arose, in part, to support increases in muscle demands during VR-enhanced training. Collectively, findings from our work reinforce the potential for VR-enhanced locomotor training to promote the greater physiologic challenge (Deutsch and Westcott McCoy 2017) that is often encouraged to improve health and prevent secondary medical conditions in children of all abilities. Our findings align with previous research reporting that children with neurological disorders increased their HR and muscle activation (i.e., rectus femoris) when training with VR-enhanced robot-assisted gait training compared to robot-assisted gait training alone (Labruyere et al., 2013). However, further ICARE and VR research should integrate a larger sample size as a post hoc analysis revealed that 85 children without disabilities would have been required to ensure adequate power to detect significant differences in heart rate between VR-enhanced and Non-VR ICARE conditions.
Together, these findings suggest that VR can serve as a beneficial tool for helping sustain children’s interest and physiologic effort during training. Unknown is whether a VR-enhanced ICARE training program could lead to clinically meaningful changes in function, cardiorespiratory fitness and strength in children with disabilities compared to a Non-VR assisted program. Potential differences in processing sensory and motor information in children with neurologic diseases could contribute to challenges interpreting/interacting with visual and auditory information (Wuang and Su 2011; Liu 2013). Additionally, some children with weakness and movement control challenges may lack the strength required to override the motor’s assistance. While previous research has demonstrated that speed can be used to manipulate lower extremity muscle demands (Burnfield et al., 2017) and cardiorespiratory challenge (Burnfield et al., 2018; Burnfield et al., 2019; Cesar et al., 2020), yet to be determined is whether these parameters can be fine-tuned sufficiently during VR-enhanced training to sustain appropriate engagement and physiologic challenge.
The impact of virtual reality gaming on joint kinematics
Greater physiologic effort during VR-enhanced vs. Non-VR ICARE training was accompanied by only minimal kinematic differences. In particular, both conditions displayed a strong similarity to fast overground gait at the thigh as evidenced by CMC values that exceeded 0.93, and moderately strong similarities at the hip (CMCs ≥0.88) and knee (CMCs ≥0.87). The significantly smaller CMC value identified at the knee during the VR-enhanced condition compared to the Non-VR condition suggests that knee motion was slightly less similar to gait during the VR condition, with subtle differences (<6°) identified between the two ICARE conditions at the beginning and end of the movement cycles. Across both VR conditions, the ankle motion pattern was least similar to fast gait and points to the need for further design modifications if normal ankle gait kinematics are desired during training. Collectively, these data suggest that the VR-enhanced condition did not contribute to notably aberrant joint kinematics during the more vigorous exercise compared to the Non-VR condition. However, if the clinical goal is focused solely on mass-repetition of a gait-like activity, then the Non-VR condition may allow for a minimally closer emulation of gait.
Limitations and future work
There are several limitations in this study. First, this preliminary work used a small sample size and within-subject comparison research design. However, this pilot work provides critical data that could be used to guide sample size calculations required to adequately power future studies that also integrate a control group. A larger sample size and integration of a control group would help discern the impact of the VR on key outcome metrics. The current study focused on a single non-immersive VR environment. We elected to integrate a non-immersive VR environment given ease of integration into the rehabilitation environment (e.g., reduced infection control issues that might arise when applying devices to the faces of multiple patients and the added weight to the head/face region and reduced capacity to interact visually with the clinician when wearing head mounted devices). However, it remains unknown whether customization of VR environments such as adopting a head-mounted device as a more immersive approach to the unique interests of a child may lead to greater effort during training, and importantly whether a more engaged child would lessen the need for clinician verbal encouragement during training. Also, the trials were relatively short (3-min), thus it is not clear whether the non-immersive VR environment that was provided would be sufficient to maintain children’s engagement across a longer training session. Future work could also include creating other VR scenarios and tasks, enabling multiplayer or network gaming capabilities, and adding leaderboards and custom avatars to potentially increase children’s engagement and motivation. Lastly, future longitudinal studies aimed at refining VR’s utility in clinical practice for various rehabilitation populations (e.g., cerebral palsy traumatic brain injury, and stroke) with a broader age range are warranted given expected variations in motor, sensory and cognitive capabilities across children.
Conclusion
This study incorporated non-immersive VR gaming (as the contextual environmental factor) into a locomotor training activity and presented direct evidence of its positive impact on children’s engagement and physiologic effort during exercise (increased exercise speed, heart rate, and selected muscle activation). The outcomes of this study can help guide design of future VR-enhanced interventions and research studies (Fritz et al., 2007).
Data availability statement
Given Institutional Review Board disclosures provided in informed consent and assent forms, sharing of raw data with external parties is not possible.
Ethics statement
The studies involving human participants were reviewed and approved by the Institutional Review Board of Madonna Rehabilitation Hospitals. Written informed consent to participate in this study was provided by the participants’ legal guardian/next of kin. Written informed consent was obtained from the individual(s), and minor(s)’ legal guardian/next of kin, for the publication of any potentially identifiable images or data included in this article.
Author contributions
Study Design (CH, KS, and JB), Recruitment (CH), Data Collection (CH and TB), Data Analysis (CH, JB, and TB), Manuscript Writing (JB, CH, TB, and KS).
Funding
The contents of this work were developed, in part, under a grant initially received from the National Institute on Disability and Rehabilitation Research, Department of Education (H133G130274; Principal Investigator: Burnfield) and subsequently funded through a grant from the National Institute on Disability, Independent Living, and Rehabilitation Research, Administration for Community Living (90IF0060; Principal Investigator: Burnfield). The contents of the article do not necessarily represent the policy of the Department of Education or the Administration for Community Living, and endorsement by the federal government should not be assumed. Additional support was provided by Joseph R. and Barbara A. Gard Family Foundation.
Acknowledgments
We gratefully acknowledged all the participating children of this study and Jonathan Hautzinger for his dedication developing the virtual reality gaming.
Conflict of interest
The co-authors (JB and TB) are the inventors of the patented motor-assisted elliptical technology. The patented technology has been licensed to SportsArt for commercial distribution. The inventors receive royalties.
The remaining authors declare that the research was conducted in the absence of any commercial or financial relationships that could be construed as a potential conflict of interest.
Publisher’s note
All claims expressed in this article are solely those of the authors and do not necessarily represent those of their affiliated organizations, or those of the publisher, the editors and the reviewers. Any product that may be evaluated in this article, or claim that may be made by its manufacturer, is not guaranteed or endorsed by the publisher.
References
Abdulsatar, F., Walker, R. G., Timmons, B. W., and Choong, K. (2013). Wii-hab" in critically ill children: A pilot trial. J. Pediatr. Rehabil. Med. 6 (4), 193–202. doi:10.3233/PRM-130260
Burnfield, J. M., Buster, T. W., Pfeifer, C. M., Irons, S. L., Cesar, G. M., and Nelson, C. A. (2019b). Adapted motor-assisted elliptical for rehabilitation of children with physical disabilities. J. Med. Device. 13 (1), 9. doi:10.1115/1.4041588
Burnfield, J. M., Cesar, G. M., and Buster, T. W. (2021). Feasibility of motor-assisted elliptical to improve walking, fitness and balance following pediatric acquired brain injury: A case series. J. Pediatr. Rehabil. Med. 14 (3), 539–551. doi:10.3233/PRM-200717
Burnfield, J. M., Cesar, G. M., Buster, T. W., Irons, S. L., and Nelson, C. A. (2017). Kinematic and muscle demand similarities between motor-assisted elliptical training and walking: Implications for pediatric gait rehabilitation. Gait Posture 51, 194–200. doi:10.1016/j.gaitpost.2016.10.018
Burnfield, J. M., Cesar, G. M., Buster, T. W., Irons, S. L., and Pfeifer, C. M. (2018). Walking and fitness improvements in a child with diplegic cerebral palsy following motor-assisted elliptical intervention. Pediatr. Phys. Ther. 30 (4), E1–E7. doi:10.1097/PEP.0000000000000541
Burnfield, J. M., Pfeifer, C. M., Kwapiszeski, S. J., Irons, S. L., Buster, T. W., and Cesar, G. M. (2019a). Impact of ICARE training speed and motor assistance on cardiovascular response. Cardiopulm. Phys. Ther. J. 30 (3), 115–122. doi:10.1097/cpt.0000000000000098
Burnfield, J. M., Shu, Y., Buster, T., and Taylor, A. (2010). Similarity of joint kinematics and muscle demands between elliptical training and walking: Implications for practice. Phys. Ther. 90 (2), 289–305. doi:10.2522/ptj.20090033
Burnfield, J. M., Shu, Y., Buster, T. W., Taylor, A. P., and Nelson, C. A. (2011). Impact of elliptical trainer ergonomic modifications on perceptions of safety, comfort, workout, and usability for people with physical disabilities and chronic conditions. Phys. Ther. 91 (11), 1604–1617. doi:10.2522/ptj.20100332
Cesar, G. M., Buster, T. W., and Burnfield, J. M. (2020). Cardiorespiratory fitness, balance and walking improvements in an adolescent with cerebral palsy (GMFCS II) and autism after motor-assisted elliptical training. Eur. J. Physiother. 22 (3), 124–132. doi:10.1080/21679169.2018.1536764
Deutsch, J. E., James-Palmer, A., Damodaran, H., and Puh, U. (2021). Comparison of neuromuscular and cardiovascular exercise intensity and enjoyment between standard of care, off-the-shelf and custom active video games for promotion of physical activity of persons post-stroke. J. Neuroeng. Rehabil. 18 (1), 63. doi:10.1186/s12984-021-00850-2
Deutsch, J. E., and Westcott McCoy, S. (2017). Virtual reality and serious games in neurorehabilitation of children and adults: Prevention, plasticity, and participation. Pediatr. Phys. Ther. 29 (3), S23–S36. doi:10.1097/PEP.0000000000000387
Fritz, S. L., Pittman, A. L., Robinson, A. C., Orton, S. C., and Rivers, E. D. (2007). An intense intervention for improving gait, balance, and mobility for individuals with chronic stroke: A pilot study. J. Neurol. Phys. Ther. 31 (2), 71–76. doi:10.1097/NPT.0b013e3180674a3c
Hirsch, M. A., Iyer, S. S., and Sanjak, M. (2016). Exercise-induced neuroplasticity in human Parkinson's disease: What is the evidence telling us? Park. Relat. Disord. 22 (1), S78–S81. doi:10.1016/j.parkreldis.2015.09.030
Hubbard, I. J., Parsons, M. W., Neilson, C., and Carey, L. M. (2009). Task-specific training: Evidence for and translation to clinical practice. Occup. Ther. Int. 16 (3-4), 175–189. doi:10.1002/oti.275
Irons, S. L., Brusola, G. A., Buster, T. W., and Burnfield, J. M. (2015). Novel motor-assisted elliptical training intervention improves 6-minute walk test and oxygen cost for an individual with progressive supranuclear palsy. Cardiopulm. Phys. Ther. J. 26 (2), 36–41. doi:10.1097/cpt.0000000000000007
Kadaba, M. P., Ramakrishnan, H. K., Wootten, M. E., Gainey, J., Gorton, G., and Cochran, G. V. (1989). Repeatability of kinematic, kinetic, and electromyographic data in normal adult gait. J. Orthop. Res. 7 (6), 849–860. doi:10.1002/jor.1100070611
Katz-Leurer, M., Carmeli, E., and Shochina, M. (2003). The effect of early aerobic training on independence six months post stroke. Clin. Rehabil. 17 (7), 735–741. doi:10.1191/0269215503cr671oa
Labruyere, R., Gerber, C. N., Birrer-Brutsch, K., Meyer-Heim, A., and van Hedel, H. J. (2013). Requirements for and impact of a serious game for neuro-pediatric robot-assisted gait training. Res. Dev. Disabil. 34 (11), 3906–3915. doi:10.1016/j.ridd.2013.07.031
Liu, T. (2013). Sensory processing and motor skill performance in elementary school children with autism spectrum disorder. Percept. Mot. Ski. 116 (1), 197–209. doi:10.2466/10.25.PMS.116.1.197-209
Monedero, J., Lyons, E. J., and O'Gorman, D. J. (2015). Interactive video game cycling leads to higher energy expenditure and is more enjoyable than conventional exercise in adults. PLoS One 10 (3), e0118470. doi:10.1371/journal.pone.0118470
Nelson, C. A., Burnfield, J. M., Shu, Y., Buster, T. W., Taylor, A. P., and Graham, A. (2011). Modified elliptical machine motor-drive design for assistive gait rehabilitation. J. Med. Devices 5 (2), 7. doi:10.1115/1.4003693
Nelson, C. A., Stolle, C. J., Burnfield, J. M., and Buster, T. W. (2015). Modification of the ICARE system for pediatric therapy. J. Med. Device. 9 (4), 6. doi:10.1115/1.4030276
O'Connor, C. M., Thorpe, S. K., O'Malley, M. J., and Vaughan, C. L. (2007). Automatic detection of gait events using kinematic data. Gait Posture 25 (3), 469–474. doi:10.1016/j.gaitpost.2006.05.016
Perry, J., and Burnfield, J. M. (2010). Gait analysis: Normal and pathological function.Thorofare, NJ SLACK Incorporated.
Pfeifer, C. M., Rowen, D. A., Buster, T. W., Cesar, G. M., Irons, S. L., and Burnfield, J. M. (2019). Design and validation of a heart rate and speed monitoring device with intelligently controlled assistive rehabilitation elliptical. J. Med. Device. 13 (1), 4. doi:10.1115/1.4041337
Richards, C. L., Malouin, F., Wood-Dauphinee, S., Williams, J. I., Bouchard, J. P., and Brunet, D. (1993). Task-specific physical therapy for optimization of gait recovery in acute stroke patients. Arch. Phys. Med. Rehabil. 74 (6), 612–620. doi:10.1016/0003-9993(93)90159-8
Robert, M., Ballaz, L., Hart, R., and Lemay, M. (2013). Exercise intensity levels in children with cerebral palsy while playing with an active video game console. Phys. Ther. 93 (8), 1084–1091. doi:10.2522/ptj.20120204
Salem, Y., and Elokda, A. (2014). Use of virtual reality gaming systems for children who are critically ill. J. Pediatr. Rehabil. Med. 7 (3), 273–276. doi:10.3233/PRM-140296
Salem, Y., Gropack, S. J., Coffin, D., and Godwin, E. M. (2012). Effectiveness of a low-cost virtual reality system for children with developmental delay: A preliminary randomised single-blind controlled trial. Physiotherapy 98 (3), 189–195. doi:10.1016/j.physio.2012.06.003
Schuler, T., Brutsch, K., Muller, R., van Hedel, H. J., and Meyer-Heim, A. (2011). Virtual realities as motivational tools for robotic assisted gait training in children: A surface electromyography study. NeuroRehabilitation 28 (4), 401–411. doi:10.3233/NRE-2011-0670
Shimada, H., Ishii, K., Makizako, H., Ishiwata, K., Oda, K., and Suzukawa, M. (2017). Effects of exercise on brain activity during walking in older adults: A randomized controlled trial. J. Neuroeng. Rehabil. 14 (1), 50. doi:10.1186/s12984-017-0263-9
Walker, M. L., Ringleb, S. I., Maihafer, G. C., Walker, R., Crouch, J. R., Van Lunen, B., et al. (2010). Virtual reality-enhanced partial body weight-supported treadmill training poststroke: Feasibility and effectiveness in 6 subjects. Arch. Phys. Med. Rehabil. 91 (1), 115–122. doi:10.1016/j.apmr.2009.09.009
Wuang, Y. P., and Su, C. Y. (2011). Correlations of sensory processing and visual organization ability with participation in school-aged children with Down syndrome. Res. Dev. Disabil. 32 (6), 2398–2407. doi:10.1016/j.ridd.2011.07.020
Keywords: virtual reality, serious gaming, engagement, electromyography, heart rate, joint kinematics
Citation: Huang C-K, Buster TW, Siu K-C and Burnfield JM (2022) Combining a non-immersive virtual reality gaming with motor-assisted elliptical exercise increases engagement and physiologic effort in children. Front. Virtual Real. 3:1063187. doi: 10.3389/frvir.2022.1063187
Received: 06 October 2022; Accepted: 18 November 2022;
Published: 07 December 2022.
Edited by:
Valentino Megale, Softcare Studios, ItalyReviewed by:
Błażej Cieślik, Jan Długosz University, PolandVangelis Lympouridis, University of Southern California, United States
Copyright © 2022 Huang, Buster, Siu and Burnfield. This is an open-access article distributed under the terms of the Creative Commons Attribution License (CC BY). The use, distribution or reproduction in other forums is permitted, provided the original author(s) and the copyright owner(s) are credited and that the original publication in this journal is cited, in accordance with accepted academic practice. No use, distribution or reproduction is permitted which does not comply with these terms.
*Correspondence: Chun-Kai Huang, Y2h1YW5nN0BrdW1jLmVkdQ==; Judith M. Burnfield, amJ1cm5maWVsZEBtYWRvbm5hLm9yZw==