- 1Department of Psychology, University of Fribourg, Fribourg, Switzerland
- 2Business School, Institute for New Work, Bern University of Applied Sciences, Bern, Switzerland
The highly immersive Virtual reality (VR) headset is gaining popularity in multiple application domains. In the context of learning, it has been proposed to be beneficial by increasing presence and attention in noisy and distracting environments, both factors that are considered important for learning. Despite intensified research efforts in recent years, empirical knowledge of experimental research addressing the link between presence and learning in specific environmental contexts is still rather scarce. In this study following an experimental mixed-method approach, the link between presence and memorization as a particular form of learning is addressed by comparing memorization with a highly immersive VR headset to a less immersive system (desktop screen) in noisy and calm learning environments. Using a 2 (learning location) x 2 (learning device) between-subjects design, 63 participants interacted with one of the two devices in either of the two environments. As expected, VR headset users reported higher presence levels. While participants subjectively evaluated the VR headset as a better device for learning, the memorization test scores were higher for desktop screen users in both calm and noisy environments. Learning location did not show significant effects. Attention distraction and context-dependent learning are discussed with regard to the unexpected results, while implications for practice and future research are discussed.
1 Introduction
In the past few years, a new trend has emerged in line with the commercialization of a new device, allowing for complete immersion in a virtual world (Lombard, 2016; Skarredghost, 2017). Virtual reality (VR) technology is opening up a plethora of new opportunities. It is being used in exposure therapy (Price and Anderson, 2007; Parsons and Rizzo, 2008), treatment of addiction, and post-traumatic stress disorder (Beck et al., 2007; Baños et al., 2011), military training (Rizzo et al., 2011), medical training (Piedra et al., 2016; Rahm et al., 2016), museums (Sylaiou et al., 2010), tourism (Huang et al., 2016; Potdevin et al., 2021), and other domains (Merchant et al., 2014). Another promising application domain for VR technology is the field of education and training (Pan et al., 2006; Hayes et al., 2013; Stevens and Kincaid, 2015; Cryer et al., 2019). In this context, the question arises as to whether the use of VR technology has a beneficial influence on learning outcomes. Although this question has been addressed in previous research, the number of methodologically sound studies assessing learning outcomes objectively are rather scarce (Radianti et al., 2020). In addition, still very little is known regarding the underlying processes influencing the link between VR use and learning. In this regard, experience of presence is often referred to as explanation for a positive link between VR use and learning. However, the empirical findings are inconclusive, with studies indicating positive effects of presence on student learning while others reported the opposite effect (Jensen and Konradsen, 2018; Radianti et al., 2020). Several factors have been put forward that might have an influence on the interplay between presence and learning such as the experience of cybersickness, type of content to be learned and the environment or context in which such learning takes place (Jensen and Konradsen, 2018; Radianti et al., 2020). In this respect, this study sets a focus on the interplay between the experience of presence as a consequence of VR use, learning environment, cybersickness and learning outcomes in the context of cognitive skills acquisition.
1.1 Immersion, Presence and Virtual Reality
VR is a computer technology composed of hardware and software that simulates a physical presence of a user in an environment that is virtually created (Biocca and Delaney, 1995; Radianti et al., 2020). While different definitions of VR have been put forward, a classification often referred to differentiates between low and high immersive VR (Biocca and Delaney, 1995; Lee and Wong, 2014). Low immersive VR relates to technological devices such as a desktop computer screen while high immersion VR is most commonly associated with a head-mounted system (i.e., VR headset).
VR systems will meet a user’s sensory expectations to different degrees (Alhalabi, 2016). The more the system meets these expectations, the more a person will be able to forget that she or he is using a VR system and will be able to appreciate its content (Burdea et al., 1996; Slater and Wilbur, 1997; Bowman and McMahan, 2007). The quality of the experience in virtual environments is generally referred to as presence or immersion. Although slightly different in terms of scope and definition, the two concepts are closely linked: if immersion is high, presence will be high and vice versa (Bowman and McMahan, 2007).
There is some disagreement about the definition of immersion (Slater, 2003; Mütterlein, 2018). Some researchers consider immersion as a psychological state and define it as the feeling of being absorbed in the virtual world (Freina and Canessa, 2015; Mütterlein, 2018). Other researchers, us included, define immersion as an objective measure that reflects the level of sensory fidelity a VR system affords (Slater and Wilbur, 1997; Bowman and McMahan, 2007). It represents an objective indicator which depends on the hardware and/or software of the VR environment (e.g., the display size and resolution, the refresh rate, the realism of lighting, the type of position tracker, the quality of the visual, audio, and haptic feedback Burdea et al. (1996)).
Presence, on the other hand, is the subjective psychological response to a VR experience, reflecting the extent to which users actually feel part of the (virtual) world they are experiencing (Slater and Wilbur, 1997; Bowman and McMahan, 2007). Presence is defined as the successful experience of being somewhere else (Heeter, 1992; Butler, 1997). In other words, the level of immersion with a specific VR system should be the same for every user as it is an objective measure that depends on the software and hardware of the device. In contrast, the individual experience of presence is very subjective and depends heavily on specific individual appraisal processes (Bowman and McMahan, 2007).
Several studies have indicated that presence may have a considerable influence on emotional reactions (Riva et al., 2007), motivation (Vogel et al., 2006), pain management (Sharar et al., 2008), exposure therapy (Parsons and Rizzo, 2008), and training (Stevens and Kincaid, 2015). Several authors also suggested that presence might be linked to learning. Psotka (1995) and Makransky et al. (2019) suggest that the feeling of presence might increase students’ motivation to learn which leads to better learning. Witmer and Singer (1998) argued that the positive link between presence and learning can be explained based on the reduction of distraction and increasing focus. In addition, they suggest that VR is beneficial for learning due to the increased level of immersion it offers (as compared to a desktop computer).
1.2 Presence and Learning
Defined as “the relatively permanent change in a person’s knowledge or behaviour due to experience or practice” (Mayer, 1982), learning can be categorized into various levels of difficulty, with memorization (e.g., being able to recall facts and concepts) being the simplest and creating (e.g., producing new or original work) the most complex level (Anderson et al., 2001). In addition, various types of learning can be differentiated. In this regard, the distinction between the acquisition of cognitive, psychomotor and affective skills has been suggested (Jensen and Konradsen, 2018).
Potential positive benefits of presence for learning were already discussed before highly immersive devices were available on the consumer market (Psotka, 1995; Salzman et al., 1995; Mikropoulos and Natsis, 2011). Few studies however addressed this link in low immersion environments. One study reported that presence felt by participants using a desktop computer did have an effect on learning (Ai-Lim Lee et al., 2010). Regarding highly immersive VR, quite a few studies have been conducted in the context of learning. Most of these studies, however, were addressing issues of usability of educational software, in which neither presence nor learning was measured (Schofield, 2012; Hupont et al., 2015; Dolezal et al., 2017; dela Cruz and Mendoza, 2018; Veronez et al., 2018; Radianti et al., 2020). While the usability of a virtual learning environment is necessary for both successful learning and presence, it does not guarantee a sense of presence or successful learning.
To our knowledge, very few studies have assessed both presence and learning in low and highly immersive environments. Some studies only measured presence and others only measured learning. Hupont et al. (2015) for example showed that presence was higher in a highly immersive VR educational environment (e.g., head-mounted headset) than in a low immersive desktop computer environment without assessing learning. In a similar vein a different study measured presence, immersion and self-efficacy in a low and highly immersive VR environment and reported that while presence and immersion was significantly higher, self-efficacy did not significantly vary between devices (Shu et al., 2019). The consequences on learning however have not been assessed in that work.
In other studies, learning was assessed in different immersive environments, but not presence. Alhalabi (2016) for example, compared the learning performance of students using four different devices (e.g., high immersive VR headset with head tracking, high immersive corner cave system, low immersive VR headset without head tracking, and low immersive computer screens). While students performed best in the most immersive system (which made the authors conclude that immersion was positively influencing learning), presence was not measured. In a similar study comparing learning with three devices (i.e., desktop computer, cave system and VR headset), VR users performed the worst. While the paper suggest that the novelty of immersion might have led to lower score, presence was also not measured. In another study (Kozhevnikov et al., 2013), participants learned relative motion concepts either on a desktop computer or with a VR headset. VR headset users had a higher learning score, which was interpreted by the authors to be a consequence—among others—of the higher immersion of VR devices, which however was not assessed. Learning was also shown to be higher for VR users in a study comparing learning on the topic of vocational training either with a google carboard VR headset or in a conventional classroom setting (Ray and Deb, 2016). Another study using google carboard VR headset, users were reported to specifically do better on questions regarding spatial awareness (Rasheed et al., 2015). Lastly since the start of the COVID-19 pandemic some studies have also looked into the possibility of teaching medical skills thanks to VR, with mixed results in terms of learning (Lohre et al., 2020; Birrenbach et al., 2021; Clarke, 2021; Pears and Konstantinidis, 2021). However, in none of these studies presence was measured. Therefore, is not clear what processes led to differences in learning performance comparing the different learning environments.
Astonishingly, two studies that did asses presence and learning in low and highly immersive learning environments (Moreno and Mayer, 2004; Makransky et al., 2019) showed that while presence was higher in the highly immersive VR device, learning scores were lower. The authors assumed that these results were due to a higher cognitive workload that was linked to the novelty of the VR headset. In a similar vein, findings of other studies indicated that while presence was higher in a more immersive environment (cave system vs interactive workbench and PC vs head mounted display), learning (referred to as memorization) was not affected by immersiveness of device, with no significant link between presence and memorization (Sutcliffe et al., 2005; Buttussi and Chittaro, 2018). In contrast to this, Cadet and Chainay (2020) reported that both presence as well as memorization were significantly higher for participants using the more immersive device (VR-headset vs computer scren; Cadet and Chainay, 2020). In a similar vein, Stevens and Kincaid (2015) reported a moderate positive correlation between the degree of presence and learning performance (Stevens and Kincaid, 2015). Interestingly another more recent study found that while device (screen vs VR headset) showed no effect on learning performance, there was a link between presence and performance, with participants subjectively reporting higher levels of presence also obtaining higher scores on a performance test (Grassini et al., 2020).
Hence, it can be summarized that the state of knowledge regarding the relation between presence and learning is inconclusive. In theory, presence has been argued to be beneficial for learning, suggesting VR to be a useful technology in this context. Studies indicating a positive relation between VR and learning hence often refer to increased presence as explanation for the effect, without explicitly assessing it. In contrast to this, presence did not show a positive relation with learning in several studies assessing both, learning and presence empirically. Therefore, additional research addressing the link between presence and learning is needed, taking into consideration other potential influencing factors such as the learning environment or negative consequences of technology use such as cybersickness (Psotka, 1995; Witmer and Singer, 1998; Salzman et al., 1999; Alhalabi, 2016; Jensen and Konradsen, 2018).
1.3 Additional Factors Influencing Learning in Virtual Reality
1.3.1 Learning Environment
With regard to training and education, an advantage to VR environments is the control they provide over the contextual learning environment. This is because contextual elements such as temperature (Hutchinson, 2003), noise (Haines et al., 2001; Cassidy and MacDonald, 2007), lighting (Haines et al., 2001), air quality (Wyon, 2004), furniture setting, and visual cues (Davis, 1984) have been shown to have significant effects on learning.
Although it is not yet possible to control all elements of the learning environment in a VR application (e.g., temperature or air quality are difficult to influence), aspects such as lighting, colour, noise, and visual cues can be designed in order to create an ideal environment for learning. Especially with regard to disturbances due to visual cues, VR head mounted systems might come with an extra benefit for learning. In highly-immersive VR learning environments, students wear a headset, which forces them to focus on the content provided in the VR-environment. Since they cannot see anything else, it can be expected that they are less prone to distractions compared to a classical learning environment (Salzman et al., 1999; Bowman and McMahan, 2007). Because any form of distraction in the environment (e.g., visual, physical or auditory) has been shown to create short interruptions in attention that impinge on individual learning outcomes (Altmann et al., 2014), this isolation from the exterior world in a VR environment can be a decisive advantage over conventional learning environments. This is particularly the case taking into consideration the fact that nowadays, most people receive pop up messages on their phone or computer that distract them and entice them to multitask, which has been shown to negatively influence learning (Spira and Feintuch, 2005; Winter et al., 2010).
In current VR environments, the user is fully immersed in the environment he or she is learning in (Salzman et al., 1995; Fried, 2008; Winter et al., 2010; Fisher et al., 2014). Although the isolation from external disturbing cues seems to be an obvious advantage of highly-immersive VR systems, very limited evidence of empirical research is available addressing this question by comparing high and low immersive technology while varying disturbance potential of the learning environment.
1.3.2 Cybersickness
One potential influencing factor regarding the link between increased presence as consequence of a highly immersive virtual environments and learning is cybersickness. Cybersickness, also referred to as virtual reality induced symptoms and effects (VRISE, e.g., Nichols et al., 1997) or negative effects (e.g., Lessiter et al., 2001), is a subtype of motion sickness that is used to describe symptoms of discomfort and illness caused by VR (Mazloumi Gavgani et al., 2018). Possible manifestations of cybersickness are nausea, vomiting, fainting, dizziness, a sensation of spinning, sweating, feeling hot, tiredness, annoyance, and blurred vision (e.g., LaViola, 2000).
The sensory mismatch theory has been put forward in order to explain both cybersickness and motion sickness (Mazloumi Gavgani et al., 2018). The theory posits that motion sickness occurs when conflicting signals are received from the spatial orientation senses (e.g., the vestibular system, the eyes, and the non-vestibular proprioceptors; Bles et al., 1998). Cybersickness is most likely caused by a mismatch between visual stimuli and the appropriate vestibular or proprioceptive feedback (Mazloumi Gavgani et al., 2018).
Some factors are known to increase the likelihood of experiencing cybersickness (Mousavia et al., 2013). Some of these are related to technical issues such as flickers, lags, and position tracking errors. While others are related to individual difference such as gender, age, and illness (e.g., Sharples et al., 2008; Davis et al., 2014). Children from the age of 2–12, women and individuals experiencing some form of illness are more likely to be affected by cybersickness. Cybersickness has been shown to negatively influence presence (Lessiter et al., 2001; Polcar and Horejsi, 2015; Weech et al., 2019), and might hence play an important role in the context of VR and learning. However a previous study comparing two different VR headset devices has shown that cybersickness had no effect on learning (Moro et al., 2017).
1.4 The Present Study
VR allows for the simulation of real-life scenarios and create immersive and captivating experiences. This is why this technical environment has often been considered to be the future of teaching and training (Abulrub et al., 2011; Fisher et al., 2014). While there seems to be evidence for an increased use of VR-technology in teaching and education (e.g., Lessiter et al., 2001; Carrozzino and Bergamasco, 2010; Radianti et al., 2020) it is still not clear how the use of VR is related to learning performance and to what extent presence as underlying mechanism plays a role in this relation. In addition, only very little is known so far regarding the usefulness of VR in different learning environments. While learners in a classical learning environment using a desktop computer may be distracted by visual and auditory cues of their environment, VR isolates learners from contextual distractors. As distraction has been negatively linked with learning performance in previous research, VR users are expected to show better performances in a learning task compared to computer users.
In order to address these open questions, an experiment was conducted in which participants either used VR or a desktop computer screen for a learning task. Presence and cybersickness were assessed in order to better understand the underlying processes linked with the use of VR for learning. In order to reduce a potential novelty effect, participants were given time to familiarize themselves with the virtual environment. In addition, the level of contextual distraction was manipulated experimentally. Half of the participants completed the learning task in a calm and quiet room with no visual and auditory distractions. The other half learned in the entry hall of the university cafeteria, a noisy space with a lot of activity going on. The experiment being quite short we conceptualized learning as the simplest category of Bloom’s taxonomy of educational objectives: participants were only expected to recall facts and basic concepts (Bloom et al., 1984). We therefore tested their learning performance with a memory test on information participants had seen in the virtual environment. It was expected that learning performance would be highest for VR users in the lab, followed by VR-users in the cafeteria and computer users in the lab, while computer users in the cafeteria were expected to show the weakest learning performance.
2 Method
2.1 Participants
Sixty-five participants aged 18 to 26 (M = 21.27, SD = 1.64) took part in the study (Nfemale = 50). In order to obtain a homogeneous sample, only university students from the University of Fribourg were recruited for the study. Participants could receive course credits in exchange for participation. Data of two participants were removed; one, due to technical problems during data collection, the other because the language of the experiment was not the mother tongue of the participant (which was a prerequisite for participation in the study). An additional exclusion criterion, participants had to meet was not to be dyslexic.
We also controlled participants' level of exposure to media devices (TV, Virtual reality headset, video games) that could influence their experience with VR. Of the 63 participants, 19 had already used a VR headset. From these, none had used one more than four times. Participants reported their level of expertise with a VR headset on a five-point Likert scale (1 = novice and 5 = expert) with a mean of 3.27 (SD = 0.77). On average, participants, watched 6.27 h of TV a week (SD = 0.679). Only 4.8% of the participants reported playing video games daily while 57.1% reported never playing any video games.
2.2 Experimental Design
The experiment followed a 2 by 2 between-subjects design, with location and immersion as independent factors. The participants either did the experiment in the university cafeteria (N = 32) or a lab (N = 31). They either completed the memorization tasks with a desktop computer screen (N = 30) or with a VR headset (N = 33). Figure 1A shows an actor representing a participant working with a VR headset while in Figure 1B, the same actor can be seen working in the desktop computer condition.
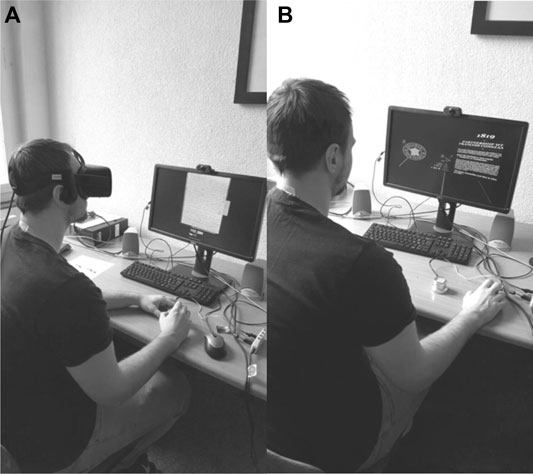
FIGURE 1. Example participant (actor) in (A) the VR headset condition, (B) the desktop computer condition.
2.3 Material
2.3.1 Hardware
A desktop system (Apple Mac Pro A1481) was used with either an immersive VR system (Oculus Rift CV1) or a 17 computer screen (DELL monitor E916H, resolution 1366 x 768 pixels). Participants using the VR only used a controller (Griffin™ PowerMate Programmable Multimedia Controller). It allowed them to select documents, advance on the timeline and zoom into documents. Participants using the virtual environment on a desktop computer screen also had to use a mouse (Microsoft L2 Comfort Mouse 4500) to change visual fields. Figure 2 depicts both the controller and mouse.
In addition, loudspeakers were used to play the pre-recorded instructions for the memorization tasks. A decibel meter (Voltcraft SL-100) was used to measure the sound level in all experimental conditions.
2.3.2 The Virtual Environment
The environment the participants used was a virtual archive for historical documents of a traditional watch-manufacturer (Figure 3). The documents appeared on the sides of a timeline in chronological order. The participants could travel the timeline in both directions and select documents. The documents and the timeline were represented in an abstract black three-dimensional space.
The documents were organized by country of origin of the document. There was a main timeline with documents originating from Switzerland. From this timeline, it was possible to get to timelines from other countries (e.g., French timeline contained only documents that originated from France). The content of the documents were letters, pictures, contracts, flyers, and newspaper articles.
2.4 Measures
2.4.1 Virtual Reality Experience and Media Usage
We asked a few questions in order to control for eventual differences of VR effects. We asked participants to estimate how much television they watched (“Please estimate your daily television consumption (hours/minutes).”). Participants were as well asked to rate their frequency of video game playing (“How frequently do you play video games?”) on a Likert scale 1 (never) to 5 (every day). They were also asked to rate on a Likert scale ranging from 1 (none) to 5 (expert), their experience with computers (“Please rate your level of experience with computers”) as well as their knowledge on VR (“How would you rate your level of knowledge on VR? (e.g., how it functions).”). Lastly, participants were asked if they had ever used a VR headset before (“Have you already used a VR headset before?”) and if so, how often (“If yes, how often have you used it?”)
2.4.2 Presence
To measure spatial presence we used an adapted version of the ITC-Sense of Presence Inventory (ITC-SOPI) (Lessiter et al., 2001). In contrast to most other presence questionnaires, the ITC-SOPI was created to measure presence independently from the type of device and environment that is used (TV, computer, VR headset, etc.). The original questionnaire is composed of 44 items that can be divided into four factors: spatial presence, ecological validity, engagement and negative effects (i.e., cybersickness). The questionnaire uses a five-point Likert scale (ranging from completely disagree to strongly agree). In this study we only used negative effects (i.e., cybersickness) and spatial presence which together encompassed 25 items.
Spatial Presence
The spatial presence dimension refers to the participant’s sensation of “being there”. Thirteen items of the spatial presence scale were removed because they were irrelevant for this study. Example of reasons for exclusion are referring to non-existing characters (e.g., “I had the sensation that characters were aware of me.”) or non-existing smells (e.g., I could almost smell different features of the displayed environment). The items that were selected for spatial presence can be found in Table 1. The deletion of the inappropriate items left a scale of five items. The items could be answered on a Likert scale ranging from 1 (strongly disagree) to 5 (strongly agree). The reliability for this shortened Spatial Presence scale (5 Items) was 0.76 (Cronbach’s alpha).
Cybersickness
The subscale “negative effects” of the ITC-SOPI is referred to in this study as cybersickness and measures adverse physiological reactions while using a VR headset (e.g., dizziness, headache). The scale is composed of six items. Each item could be answered on a Likert scale ranging from (strongly disagree) to (strongly agree). The reliability for the for cybersickness scale was 0.9 (Cronbach’s alpha).
2.4.3 Memorization Test
A memorization test was developed on the basis of the information presented in the digitalized archive. The tasks the participants completed (cf. procedure section) ensured that participants read all the respective text in the archive to be able to answer the test. The test was composed of 21 multiple choice questions with an option of four answers, with only one answer being correct. Six questions asked about the date and locations of the documents (i.e., when and where something happened). Five questions were asked about the number of documents represented in a specific area in the VR environment. Four questions were about the type of document which was viewed. Finally, seven questions were asked about the content of the diverse documents (i.e., what was said). The average item difficulty was M = 0.58, SD = 0.2, with the most difficult item having a difficulty level of 0.3 and the easiest being at 1.
2.4.4 Disturbance due to Location
A four-item scale was created to measure the possible level of experienced disturbance by the participants depending on the location. Participants could answer on a Likert scale ranging from 1 (not at all) to 5 (a lot). Two items asked questions regarding the sound level (‘was there any noise during the experiment?’ “Were you disturbed by the noise?”) and two other items were linked to the presence of others (“were you disturbed by the presence of others during the experiment?” “Did you feel uncomfortable during the experiment?”)
2.4.5 Comparative Questions on the Two Devices
At the end of the experiment, after participants had interacted with the other device (e.g., VR for those who learned with the computer, cf. procedure section), a final questionnaire was administered containing five questions comparing the two devices (e.g., “Which of the two devices was easier to use?”). The five questions can be found in Table 3. A semantic differential scale ranging from one (computer screen) to five (VR) was used. In addition, after each of the five questions, participants were asked to justify their rating by entering a short comment as free text.
2.5 Procedure
Participants were either received in the lab or the cafeteria. They were first asked to sign the consent form and were informed about the procedure of the experiment. They then filled out a questionnaire containing the demographic and control questions. After this, they were instructed in detail on how to use the device they were going to use (desktop computer screen or virtual reality headset). This was done so that users wouldn’t encounter usability issues during the experiment. In a warm-up phase, participants were asked to familiarize themselves with the device for 3 minutes. We did this to avoid concerns put forward by Moreno and Mayer (2004) and Makransky et al. (2019) about participants not performing well in VR due to excitement and distraction in the new and unfamiliar virtual environment, to which we refer to as the “wow-effect”.
After the warm-up phase, participants received instructions for the following tasks. They were informed that questions would be asked later about their task. In addition, they were reminded to stay concentrated throughout the whole experiment. Then, the facilitator left the laboratory or the cafeteria and went to a neighbouring room, from where she could see and hear what the participant was doing by means of a screen-mirroring application (TeamViewer). Participants received all information regarding the different tasks via pre-recorded audio-instructions. If needed, participants could ask to have the task instructions repeated by saying “repeat”.
After task completion, participants were asked to answer a series of questionnaires including the spatial presence and negative effects (i.e., cybersickness) dimensions from the ITC-SOPI, the test on the tasks, the questions on annoyance and finally the ones on satisfaction.
After this, participants changed the device (e.g., computer screen for participants who completed the memorization tasks in the VR environment) and interacted with the alternative device for 3 minutes. They then replied to the questions comparing the two devices.
2.6 Data Analysis and Manipulation Check
Data was analysed using a two-factorial analysis of variance (ANOVA). For some variables, the Kolmogorov Smirnoff test indicated a non-normal distribution and the assumption of homogeneity of variance was not met (short presence 2, disturbance, satisfaction and learning). Since the QQ plots showed for most of these variables a normal distribution and since the absolute size of the skewness and kurtosis relative to their standard error was always below 2, it was assumed that the ANOVA can be considered robust (Khan and Rayner, 2003).
Results of the manipulation check regarding location can be found in Table 2. Manipulation check indicated that the physical sound measured (in decibels) was louder in the cafeteria (M = 55,59, SD = 11.31) than in the lab (M = 30,14, SD = 10,48) indicating a significant manipulation of noise F (1,58) = 81.51, p < 0.05, η2 = 0.58. There was also a positive correlation between the level of sound and the perceived level of sound r = 0.60, N = 60, p < 0.05, as well as between the level of sound and the level of disturbance through sound r = 0.51, N = 60, p < 0.05.
The level of disturbance was not affected by the device used by the participant F (1, 53) = 2,1 p > 0.05, η2 = 0.04. Participants using VR headset (M = 1.9, SD = 1.23) reported a similar level of disturbance to desktop computer screen users (M = 1.51, SD = 0.74).
3 Results
3.1 Memorization Test
Results in the memorization test showed, in contrast to our expectations, that participants in the desktop computer screen condition performed better (M = 12.57, SD = 1.87) than participants in the VR headset condition (M = 10.73, SD = 2.71; F (1, 58) = 9.51 p < 0.05, η2 = 0.14). In order to control for the influence of cybersickness, the same analysis was run with cybersickness as a co-variate. Results showed that desktop users still showed a higher memorization score than the VR headset users, but the size of this effect was considerably smaller F (1, 57) = 5.38 p < 0.05, η2 = 0.09.
Location had no significant effect on learning F (1, 59) = 0.21, p > 0.05, η2 = 0.01. Participants in the cafeteria (M = 11.5, SD = 2.99) showed similar learning scores compared to the ones in the lab (M = 11.71, SD = 1.92). There was no significant interaction of device and location on the memorization score F (1, 59) = 0.79, p > 0.05, η2 = 0.01.
3.2 Presence
3.2.1 Spatial Presence
Results showed that there was a significant main effect of device indicating higher spatial presence scores in the VR condition (M = 3.54, SD = 0.6) compared to the computer condition (M = 3.16, SD = 0.72; F (1, 59) = 5.76, p < 0.05, η2 = 0.09). Location did not show a significant main effect F (1, 59) = 2.05, p > 0.05, η2 = 0.034. There was also no significant interaction between the two factors F (1, 59) = 0.003 p > 0.05, η2 = 0.00.
3.2.2 Cybersickness
Cybersickness was higher in VR headset users (M = 3, SD = 1.16) compared to desktop users (M = 1.93, SD = 0.76; F (1, 59) = 18.37 p < 0.05, η2 = 0.87). There was no significant difference between participants in the cafeteria (M = 2.52, SD = 1.2) and in the lab condition (M = 2.46, SD = 1.04; F (1, 59) = 0.11, p > 0.05, η2 = 0.00). There was also no significant interaction between the two factors F (1, 59) = 3.37, p > 0.05, η2 = 0.05. An analysis of the above-reported triple interaction on spatial presence using cybersickness as co-variate did not result in any changes with regard to the presented results.
3.3 Correlation of Main Variables
Analysis of correlations between our main variables (memorization test, spatial presence, and cybersickness) revealed a significant negative link between cybersickness and the score on the memorization test (cf. Table 3). Spatial presence, however, was not significantly linked to memorization or cybersickness. As can be seen in Table 3, when running the analysis for each of the two immersion conditions separately, there was no significant link between the different variables.
3.4 Comparative Evaluation of the Two Devices
Participants interacted with both devices (cf. method section). This made it possible to ask participants, at the end of the study, to reply to items addressing their beliefs on the benefits and disadvantages of these two devices for learning and studying. In addition to the comparative semantic differentials, written comments were screened and categorized by one of the authors. Two coders then coded participants’ answers into the categories defined by the author. In case of disaccord, the statement was discussed by the two raters in order to obtain a consistent assessment.
As can be seen in Table 4, a majority of participants preferred the VR headset to the desktop computer. Reasons for preferring VR for learning given by participants were being more immersed with VR (mentioned by 46.03% of participants), feeling more implicated (14.28%), finding VR more interesting (12.69%) and interactive (11.11%). In terms of device preferences for work, the results are more nuanced. Participants that reported preferring VR for work explained that they felt more immersed with VR (17.46%), that VR was easier to use (11.11%) and that they would be more concentrated with VR (11.11%). Participants that though they would work better with the computer listed the absence of cybersickness symptoms (12.7%) and their previous knowledge with computers (12.7%) as reasons. Cybersickness symptoms were also listed as an explanation for believing that they would be more focused on a desktop computer (12.69%). Additionally, some participants expressed the belief that VR was for fun and not for work (15.87%). Participants who believe that they would be most concentrated with the VR headset listed immersion (31.74%) and being physically cut off from the outside world (23.8%) as reasons.
In terms of ease of use (cf. Table 4), participants preferring VR explained that VR felt more intuitive and that it was easier to orient oneself. Several participants (15.85%) mentioned that the desktop computer was easier to use because of previous experience.
There was no clear preference with regard to the question about the device’s usefulness for information retention (cf. Table 4). However, only VR users gave explanations about their reasoning, mentioning higher immersion (17.46%), implication (17.46%) and focus (19.05%) as reasons for why they prefer VR over desktop computers regarding information retention.
4 Discussion
The primary hypothesis of this study was that students using a VR headset would feel more present and hence learn better compared to participants using a desktop computer. The difference between the groups was expected to be even stronger if the participants took part in the experiment in the noisy and distracting cafeteria instead of the calm and quite lab.
As expected, students reported higher values of spatial presence when using a VR headset compared to the desktop screen users. Contrary to our expectations, however, this had no beneficiary influence on participants’ memorization performance, since data of the memorization test showed that participants learned better when using a computer screen. Interestingly, this also contradicts participants’ subjective evaluation of the usefulness of the two technological devices for learning; 65% of the participants reported that they expected to learn better using a VR headset compared to the desktop computer. These findings are is in stark contrast to the widely accepted assumption that presence positively influences learning (Psotka, 1995; Salzman et al., 1995; Mikropoulos and Natsis, 2011). Interestingly, this is in line with results of other studies that have addressed presence and learning in highly immersive environments (Moreno and Mayer, 2004; Makransky et al., 2019). Several potential explanations can be put forward in this regard.
A first potential explanation for this unexpected result might be found in the data on cybersickness and disturbances. VR headset users reported increased disturbance levels (e.g., felt uncomfortable) and experienced cybersickness (e.g., dizziness, feeling sick, headaches). Also, in their qualitative feedback, participants mentioned cybersickness as main reason for preferring the desktop computer screen to the VR headset for working and memorization (cf. Table 4). However, presence seems to be affected by cybersickness only to a very limited extent, as the correlation between these two variables was rather small.
However, factors such as lag (delay between user action and system response), flicker, calibration (interpupillary adaptation), and general ergonomics (e.g., heavy and poor fitting headsets) have also been shown to have a considerable influence on the prevalence of negative consequences of VR use (e.g, McCauley and Sharkey, 1992; LaViola, 2000; Sharples et al., 2008). Previous research has shown that the occurrence of cybersickness was linked with lower attitudes towards the technology, lower learning outcomes and lower presence (Polcar and Horejsi, 2015; Weech et al., 2019). With regard to learning and VR, future research needs to address the question of how the improvement of hardware and software can help to reduce the occurrence of negative consequences in VR use, as this aspect seems to have an important influence on learning performance. Although such negative effects seem to impinge on learning with the VR headset (cf. the negative correlation between the two measures in this study), analysis of co-variance showed that when controlling for cybersickness, participants still perform better in the desktop computer screen condition—however with a reduced effect size. Therefore, it can be assumed that cybersickness linked with the use of the VR headset is not the only explanation for the reported differences in learning performance.
A second assumption explaining this unexpected effect might be found in differences in previous experience of the participants with their respective device. All 65 participants had used a desktop computer screen before and had considerable experience using it. On the contrary, only nineteen participants reported previous experiences of using a VR headset, and no one had used it more often than five times. Participants using the desktop computer screen did not need to adapt and learn how to use the device, while VR headset users did. Although we provided participants with clear explanations on how to use both devices and gave them 3 minutes to practice and familiarize with the virtual environment, it is possible that this was not enough time. VR headset users might have needed to spend cognitive effort on the handling of the VR device during the memorization task that the computer users did not need. The verbal comments of the test participants (cf. Table 4) indicate that the lack of experience with a VR headset device is one reason why the environment was considered less suitable for learning. This suggestion is in line with Makransky et al. (2019) and Polcar and Horejsi (2015) who also reported better learning performances for less immersive environments. Both suggested that lower learning might be linked to the lack of experience with the device. Makransky et al. (2019) explained that a lack of experience might lead to a higher cognitive load for participants using a VR environment.
A third explanation could be what we refer to as the “wow-effect.” Using the VR headset was a new and impressive experience for most of the participants. For many of them, it was the first time they used such a high immersive device. Therefore, it can be assumed that they were distracted by the immersive experience and were, in consequence, less able to concentrate on the content provided in the virtual environment that they were supposed to memorize. For desktop users, however, this wow-effect was less pronounced as the device is less immersive and well known among university students. A similar argument was put forward by Makransky et al. (2019). They suggested that the strong hedonic component of the VR experience might lead participants to view the environment as playful and hence distract from the learning task. We were aware of the risk of a “wow-effect” and the potential impact it could have on learning. Therefore, participants in this study were asked to familiarize with the environment in a warm-up phase, what was expected to reduce initial excitement when experiencing for the first time such an immersive environment. It might be assumed however that the 3-min session was too short for the participants in our study. As VR becomes common and participants are expected to become more and more accustomed to its use, it could be speculated that the wow-effect as well the cognitive load due to lack of experience will be of lesser importance in the near future. Nonetheless, it might be interesting for this field of research to address questions regarding the wow-effect (e.g., does it wane over time, and how long does it last?) and the influence of extended usage experience (e.g., is cognitive load reduced in highly experienced VR users?), and their link to learning in future studies.
Finally, context-dependent memory might play a role in the unexpected effects reported in this piece of research. The essence of this well-established research topic (Godden and Baddeley, 1975; Herz, 1997; Smith and Vela, 2001) is that information is better recalled in the environment the information was learned in comparison to a new and unfamiliar context. Godden and Baddeley (1975) for example, found that divers learning underwater recalled the information better underwater than above. In the present study, both VR headset and desktop computer screen users answered the test (i.e., information recall) on the computer. As a consequence, computer users filled in the test on the same device they had been using while learning. In contrast, VR users answered on a different device, which might lead to reduced learning performances due to context-dependent memory. Although not explicitly mentioned in their article, Makransky et al. (2019) also asked their participants to answer the learning test on a desktop computer, regardless of which device they used for learning (personal communication). As they reported similar results to ours, context-dependent memory might have had an impact on the reduced learning performance of VR headset users in both studies. Future studies comparing learning with a VR headset with less immersive environments (e.g., desktop screen) should control for this potential error variable. This could be done either by presenting the assessment or test in the same technological environment as the learning took place (e.g., participants learning in VR take the test in VR as well) or by choosing an alternative medium (e.g., participants using high and low immersive devices complete the test with paper and pencil).
Overall, it is not possible to conclude with certainty that presence has no influence on learning based on the findings presented in this piece of research and the similar previous studies. It could be that that presence has a positive effect on learning, but this positive effect is outweighed by the negative consequences of using a highly immersive VR headset (i.e., cybersickness, increased cognitive workload, wow-effect or context dependent memory). In this regard, additional research is needed in which the confounding effect of these different influencing variables for learning can be controlled. This is important since the use of highly immersive VR headsets used to increase presence in this research context is always directly linked with an increase in the other influencing variables (e.g., increased cognitive workload). Possibilities to handle these issues have been discussed above: new VR systems need to be developed that reduce the prevalence of cybersickness, the recruitment of experienced participants might reduce the wow-effect, knowledge tests should be conducted in the same environment as the learning has taken place and cognitive workload needs to be held constant. One possibility to overcome these issues in experimental research might be to refrain from comparing VR systems with computer screen environments but to manipulate a VR environment which induces different levels of presence while keeping cognitive demand, cybersickness and other aspects constant.
With regard to learning, it was rather astonishing that the location did not show any influence. The manipulation check showed that the noise level in the cafeteria was considerably above the one in the lab (56 dB compared to 30 dB). Subjective ratings of participants’ distraction and disturbance level also revealed significant differences between the two experimental conditions with the cafeteria being rated more disturbing and distracting than the lab. Since previous research has shown that noise (e.g., Cassidy and MacDonald, 2007) and interruptions (e.g., Altmann et al., 2014) have a negative effect on learning performance, it is intriguing that the location had no influence on learning in the present study. A possible explanation for this nil effect could be the sample of this study. Participants were students who are generally used to learn in noisy environments such as cafeterias and libraries. Accustomed to these places, their learning performance therefore might not have been influenced strongly enough. In addition, it could be speculated that the nil effects are a consequence of a participant behaviour particular in experimental studies. Although much effort has been put into making the experimental setup as natural as possible, participants were aware of the fact that they take part in a scientific study. This might have kept their concentration and focus on the presented content, while in a real learning situation, they might be more inclined to let themselves be distracted by the environment. This represents an extremely difficult challenge for future studies addressing the influence of the learning context experimentally. A possible approach could be a longitudinal field experiment, in which students’ learning success with different systems is compared over a longer period of time–if possible, in a natural environment as implemented in this study.
Additional limitations relate to the generalisability of the results. It should be noted that only students were considered for this study. A generalisation across this population is therefore not appropriate. But as students are an important group of potential users of VR systems for learning, these results seem to be of practical relevance for an important part of the population. However, it would be of considerable interest for future studies to evaluate whether similar results (especially with regard to noisy environments) could be obtained with different user groups (e.g., pupils, adult trainees etc.). A second limitation concerns the controls used in the experiment. The controls in the VR headset were slightly easier to use compared to the ones for the desktop computer. VR headset users only had to use one controller (PowerMate) while desktop computer users had to deal with two (mouse and PowerMate). Four participants reported this in the comment section of the questionnaire as a factor that made them prefer the VR headset. This is particularly important since usability has been shown to be an important factor influencing the suitability of VR for learning (Hupont et al., 2015; Fernandes et al., 2016; Dolezal et al., 2017; Bryan et al., 2018; dela Cruz and Mendoza, 2018; Veronez et al., 2018). Although it would have been preferable to have used the same interaction mode for both experimental conditions in this study, this was technically not feasible since navigation via head movement was not applicable for the desktop computer condition. We would, however, expect the higher usability of the VR system to have a positive effect on learning performance and hence would expect an increase in the learning performance in the VR condition compared to the desktop computer condition—which was not the case. Another possible issue might have been the chosen time to adjust and practice to the novel device. Three minutes is possibly not enough time to familiarize participants with a novel device like a VR headset or at least not enough to eliminate a preference for the desktop computer due to experience. Extra time could have also reduced a possible wow-effect. Participants should have been given more time with the VR headset to overcome the wow and experience effect, although it remains unclear and subject for future research how much additional interaction time should be accorded to novice participants in order to overcome those two effects. Another way to control for the wow-effect would be running the experiment with experienced VR headset users. Lastly, while this study chose to conceptualize learning as memorization, other forms of learning (i.e., psychomotor or embodied learning) might be of interest for future research (Seo et al., 2018; Alvarez-Lopez et al., 2020). In addition, the VR environment in this study was simulating a place illusion in a pointandclick manner which is highly similar to a traditional screen usage, while plausibility illusion or agency in the VR world has not been assessed but might be of interest for future research (Slater, 2017; Gruber and Kaplan-Rakowski, 2020; Hurault et al., 2021).
5 Conclusion
Evidence of this study indicates that highly immersive VR may not always be beneficial for memorization, despite the higher level of perceived presence experienced by students using the VR headset and despite the fact that students are isolated from noisy and distracting environments. These results suggest that the link between presence and memorization with highly immersive devices is not as strong as often expected. Although performance was lower with the VR headset, users subjectively preferred the VR headset compared to a low-immersive desktop system. This might suggest that the VR headset may be a useful device for learning, nevertheless. This might especially be the case taking into consideration that feelings of presence increase students’ motivation to learn (Psotka, 1995), suggesting that learning and presence might be positively correlated in the long run. Future studies should address the possible reasons for the reduced learning performance in VR (e.g., cybersickness, experience effect, wow effect, context-dependent memory) adopting a longitudinal research approach. This would provide in-depth knowledge of the VR learning processes and procedures that are necessary to create meaningful and useful learning environments for the future.
Data Availability Statement
The raw data supporting the conclusion of this article will be made available by the authors, without undue reservation.
Ethics Statement
The studies involving human participants were reviewed and approved by Internal Review Board of the Department of Psychology University of Fribourg. The patients/participants provided their written informed consent to participate in this study.
Author Contributions
CO: Conceptualization, Methodology, Investigation, Formal analysis, Writing- Original draft preparation, Reviewing and Editing. AS: Conceptualization, Methodology, Writing- Reviewing and Editing.
Conflict of Interest
The authors declare that the research was conducted in the absence of any commercial or financial relationships that could be construed as a potential conflict of interest.
Publisher’s Note
All claims expressed in this article are solely those of the authors and do not necessarily represent those of their affiliated organizations, or those of the publisher, the editors and the reviewers. Any product that may be evaluated in this article, or claim that may be made by its manufacturer, is not guaranteed or endorsed by the publisher.
Acknowledgments
We want to thank Gabriela Lidia Hofmann, and Patrizia Baccarella, for their help with testing, as well as Huntley Thatcher for her many proofreads, and lastly, Kaspar Burger for his feedback on an earlier version of the manuscript.
References
Abulrub, A. H. G., Attridge, A. N., and Williams, M. A. (2011). “Virtual Reality in Engineering Education: The Future of Creative Learning,” in IEEE Global Engineeering Education Conference, Amman, Jordan, April 4–6, 2011 (EDUCON), 751–257. doi:10.1109/educon.2011.5773223
Ai-Lim Lee, E., Wong, K. W., and Fung, C. C. (2010). How Does Desktop Virtual Reality Enhance Learning Outcomes? A Structural Equation Modeling Approach. Comput. Educ. 55, 1424–1442. doi:10.1016/j.compedu.2010.06.006
Alhalabi, W. (2016). Virtual Reality Systems Enhance Students' Achievements in Engineering Education. Behav. Inf. Techn. 35, 919–925. doi:10.1080/0144929X.2016.1212931
Altmann, E. M., Trafton, J. G., and Hambrick, D. Z. (2014). Momentary Interruptions Can Derail the Train of Thought. J. Exp. Psychol. Gen. 143, 215–226. doi:10.1037/a0030986
Alvarez-Lopez, F., Maina, M. F., and Saigí-Rubió, F. (2020). Use of a Low-Cost Portable 3D Virtual Reality Gesture-Mediated Simulator for Training and Learning Basic Psychomotor Skills in Minimally Invasive Surgery: Development and Content Validity Study. J. Med. Internet Res. 22, e17491. doi:10.2196/17491
Alves Fernandes, L. M., Cruz Matos, G., Azevedo, D., Rodrigues Nunes, R., Paredes, H., Morgado, L., et al. (2016). Exploring Educational Immersive Videogames: an Empirical Study with a 3D Multimodal Interaction Prototype. Behav. Inf. Techn. 35, 907–918. doi:10.1080/0144929X.2016.1232754
Anderson, L. W., Krathwohl, D. R., and Bloom, B. S. (2001). A Taxonomy for Learning, Teaching, and Assessing: A Revision of Bloom’s Taxonomy of Educational Objectives. New York: Longman.
Baños, R. M., Guillen, V., Quero, S., García-Palacios, A., Alcaniz, M., and Botella, C. (2011). A Virtual Reality System for the Treatment of Stress-Related Disorders: A Preliminary Analysis of Efficacy Compared to a Standard Cognitive Behavioral Program. Int. J. Human Comput. Stud. 69, 602–613. doi:10.1016/j.ijhcs.2011.06.002
Beck, J. G., Palyo, S. A., Winer, E. H., Schwagler, B. E., and Ang, E. J. (2007). Virtual Reality Exposure Therapy for PTSD Symptoms after a Road Accident: An Uncontrolled Case Series. Behav. Ther. 38, 39–48. doi:10.1016/j.beth.2006.04.001
Biocca, F., and Delaney, B. (1995). “Communication in the Age of Virtual Reality,” in Virtual Reality as a Communication System (Hillsdale, NJ, US: Lawrence Erlbaum Associates), 57–124.
Birrenbach, T., Zbinden, J., Papagiannakis, G., Exadaktylos, A. K., Müller, M., Hautz, W. E., et al. (2021). Effectiveness and Utility of Virtual Reality Simulation as an Educational Tool for Safe Performance of COVID-19 Diagnostics: Prospective, Randomized Pilot Trial. JMIR Serious Games 9, e29586. doi:10.2196/29586
Bles, W., Bos, J. E., de Graaf, B., Groen, E., and Wertheim, A. H. (1998). Motion Sickness: Only One Provocative Conflict? Brain Res. Bull. 47, 481–487. doi:10.1016/S0361-9230(98)00115-4
Bloom, B. S., Krathwohl, D. R., and Masia, B. B. (1984). Bloom Taxonomy of Educational Objectives. Copyright (c) by Pearson Education. Boston, MA: Allyn & Bacon. Available at: http://www.coun.uvic.ca/learn/program/hndouts/bloom.html.
Bowman, D. A., and McMahan, R. P. (2007). Virtual Reality: How Much Immersion Is Enough? Computer 40, 36–38. doi:10.1109/mc.2007.257
Bryan, S. J., Campbell, A., and Mangina, E. (2018). “Scenic Spheres - An AR/VR Educational Game,” in 2018 IEEE Games, Entertainment, Media Conference (GEM), Galway, Ireland, August 15–17, 2018, 1–9. doi:10.1109/GEM.2018.8516456
Burdea, G., Richard, P., and Coiffet, P. (1996). Multimodal Virtual Reality : Input-Output Devices, System Integration, and Human Factors. Int. J. Human Comput. Interaction, 5–24.
Buttussi, F., and Chittaro, L. (2018). Effects of Different Types of Virtual Reality Display on Presence and Learning in a Safety Training Scenario. IEEE Trans. Vis. Comput. Graph. 24, 1063–1076. doi:10.1109/TVCG.2017.2653117
Cadet, L. B., and Chainay, H. (2020). Memory of Virtual Experiences: Role of Immersion, Emotion and Sense of Presence. Int. J. Human Comput. Stud. 144, 102506. doi:10.1016/j.ijhcs.2020.102506
Carrozzino, M., and Bergamasco, M. (2010). Beyond Virtual Museums: Experiencing Immersive Virtual Reality in Real Museums. J. Cult. Heritage 11, 452–458. doi:10.1016/j.culher.2010.04.001
Cassidy, G., and MacDonald, R. A. R. (2007). The Effect of Background Music and Background Noise on the Task Performance of Introverts and Extraverts. Psychol. Music 35, 517–537. doi:10.1177/0305735607076444
Clarke, E. (2021). Virtual Reality Simulation-The Future of Orthopaedic Training? A Systematic Review and Narrative Analysis. Adv. Simul 6, 2. doi:10.1186/s41077-020-00153-x
Cryer, A., Kapellmann-Zafra, G., Abrego-Hernandez, S., Marin-Reyes, H., and French, R. (2019). “Advantages of Virtual Reality in the Teaching and Training of Radiation Protection during Interventions in Harsh Environments,” in 2019 24th IEEE International Conference on Emerging Technologies and Factory Automation (ETFA), Zaragoza, Spain, September 10-13, 2019 784–789. doi:10.1109/ETFA.2019.8869433
Davis, S., Nesbitt, K., and Nalivaiko, E. (2014). “A Systematic Review of Cybersickness,” in Proceedings of the 2014 Conference on Interactive Entertainment, IE2014, Newcastle NSW, Australia, December 2-3, 2014 (New York, NY, USA: ACM), 8:1–8:9. doi:10.1145/2677758.2677780
Davis, T. R. V. (1984). The Influence of the Physical Environment in Offices. AMR 9, 271–283. doi:10.2307/25844010.5465/amr.1984.4277654
dela Cruz, D. R., and Mendoza, D. M. M. (2018). “Design and Development of Virtual Laboratory: A Solution to the Problem of Laboratory Setup and Management of Pneumatic Courses in Bulacan State University College of Engineering,” in 2018 IEEE Games, Entertainment, Media Conference (GEM), Galway, Ireland, August 15-17, 2018 1–23. doi:10.1109/GEM.2018.8516467
Dolezal, M., Chmelik, J., and Liarokapis, F. (2017). “An Immersive Virtual Environment for Collaborative Geovisualization,” in 2017 9th International Conference on Virtual Worlds and Games for Serious Applications (VS-Games), Athens, Greece, October 05, 2017 (Athens, Greece: IEEE), 272–275. doi:10.1109/VS-GAMES.2017.8056613
Fisher, A. V., Godwin, K. E., and Seltman, H. (2014). Visual Environment, Attention Allocation, and Learning in Young Children. Psychol. Sci. 25, 1362–1370. doi:10.1177/0956797614533801
Freina, L., and Canessa, A. (2015). “Immersive vs Desktop Virtual Reality in Game Based Learning,” in The European Conference on Games Based Learning (Academic Conference International Limited), 195.
Fried, C. B. (2008). In-class Laptop Use and its Effects on Student Learning. Comput. Educ. 50, 906–914. doi:10.1016/j.compedu.2006.09.006
Godden, D. R., and Baddeley, A. D. (1975). Context-dependent Memory in Two Natural Environments: on Land and Underwater. Br. J. Psychol. 66 (3), 325–331. doi:10.1111/j.2044-8295.1975.tb01468.x
Grassini, S., Laumann, K., and Rasmussen Skogstad, M. (2020). The Use of Virtual Reality Alone Does Not Promote Training Performance (But Sense of Presence Does). Front. Psychol. 11. doi:10.3389/fpsyg.2020.01743
Gruber, A., and Kaplan-Rakowski, R. (2020). “User Experience of Public Speaking Practice in Virtual Reality,” in Cognitive and Affective Perspectives on Immersive Technology, 235–249. doi:10.4018/978-1-7998-3250-8.ch012
Haines, M. M., Stansfeld, S. A., Brentnall, S., Head, J., Berry, B., Jiggins, M., et al. (2001). The West London Schools Study: the Effects of Chronic Aircraft Noise Exposure on Child Health. Psychol. Med. 31, 1385–1396. doi:10.1017/S003329170100469X
Hayes, A. T., Straub, C. L., Dieker, L. A., Hughes, C. E., and Hynes, M. C. (2013). Ludic Learning. IJGCMS 5, 20–33. doi:10.4018/jgcms.2013040102
Heeter, C. (1992). Being There: The Subjective Experience of Presence. Presence Teleop. Virtual Environ. 1, 262–271. doi:10.1162/pres.1992.1.2.262
Herz, R. S. (1997). The Effects of Cue Distinctiveness on Odor-Based Context-dependent Memory. Mem. Cogn. 25, 375–380. doi:10.3758/bf03211293
Huang, Y. C., Backman, K. F., Backman, S. J., and Chang, L. L. (2016). Exploring the Implications of Virtual Reality Technology in Tourism Marketing: An Integrated Research Framework. Int. J. Tourism Res. 18, 116–128. doi:10.1002/jtr.2038
Hupont, I., Gracia, J., Sanagustin, L., and Gracia, M. A. (2015). “How Do New Visual Immersive Systems Influence Gaming QoE? A Use Case of Serious Gaming with Oculus Rift,” in 2015 Seventh International Workshop on Quality of Multimedia Experience (QoMEX), Pilos, Greece, May 26-29, 2015 1–6. doi:10.1109/QoMEX.2015.7148110
Hurault, J.-C., Tedesco, A., and Brunel, L. (2021). " I Know what You Mean »: Investigating the Sense of agency in Learning an Abstract Mathematical Knowledge Using a Constructivist Method in Virtual Reality. Lannee Psychol. 121, 443–487. doi:10.3917/anpsy1.214.0443
Hutchinson, L. (2003). ABC of Learning and Teaching: Educational Environment. BMJ: Br. Med. J. 326, 810–812. doi:10.1136/bmj.326.7393.810
Jensen, L., and Konradsen, F. (2018). A Review of the Use of Virtual Reality Head-Mounted Displays in Education and Training. Educ. Inf. Technol. 23, 1515–1529. doi:10.1007/s10639-017-9676-0
Khan, A., and Rayner, G. D. (2003). Robustness to Non-normality of Common Tests for the many-sample Location Problem. J. Appl. Maths. Decis. Sci. 7, 187–206. doi:10.1155/s1173912603000178
Kozhevnikov, M., Gurlitt, J., and Kozhevnikov, M. (2013). Learning Relative Motion Concepts in Immersive and Non-immersive Virtual Environments. J. Sci. Educ. Technol. 22, 952–962. doi:10.1007/s10956-013-9441-0
LaViola, J. J. (2000). A Discussion of Cybersickness in Virtual Environments. SIGCHI Bull. 32, 47–56. doi:10.1145/333329.333344
Lee, E. A.-L., and Wong, K. W. (2014). Learning with Desktop Virtual Reality: Low Spatial Ability Learners Are More Positively Affected. Comput. Educ. 79, 49–58. doi:10.1016/j.compedu.2014.07.010
Lessiter, J., Freeman, J., Keogh, E., and Davidoff, J. (2001). A Cross-media Presence Questionnaire: The ITC-Sense of Presence Inventory. Presence Teleop. Virtual Environ. 10, 282–297. doi:10.1162/105474601300343612
Lohre, R., Bois, A. J., Athwal, G. S., and Goel, D. P. (2020). Improved Complex Skill Acquisition by Immersive Virtual Reality Training: A Randomized Controlled Trial. JBJS 102, e26. doi:10.2106/JBJS.19.00982
Lombard, M. (2016). A New Age of VR Involving All Five Senses [WWW Document]. International Society for Presence Research. Available at: https://ispr.info/2016/08/02/a-new-age-of-vr-involving-all-five-senses/ (Accessed 6 3, 2018).
Makransky, G., Terkildsen, T. S., and Mayer, R. E. (2019). Adding Immersive Virtual Reality to a Science Lab Simulation Causes More Presence but Less Learning. Learn. Instruction 60, 225–236. doi:10.1016/j.learninstruc.2017.12.007
Mayer, R. E. (1982). “Learning,” in Encyclopedia of Education Research. Editor H. E. Mitzel (New York: Free Press), 1040–1058.
Mazloumi Gavgani, A., Walker, F. R., Hodgson, D. M., and Nalivaiko, E. (2018). A Comparative Study of Cybersickness during Exposure to Virtual Reality and “Classic” Motion Sickness: Are They Different? J. Appl. Physiol. 125, 1670–1680. doi:10.1152/japplphysiol.00338.2018
McCauley, M. E., and Sharkey, T. J. (1992). Cybersickness: Perception of Self-Motion in Virtual Environments. Presence Teleop. Virtual Environ. 1, 311–318. doi:10.1162/pres.1992.1.3.311
Merchant, Z., Goetz, E. T., Cifuentes, L., Keeney-Kennicutt, W., and Davis, T. J. (2014). Effectiveness of Virtual Reality-Based Instruction on Students' Learning Outcomes in K-12 and Higher Education: A Meta-Analysis. Comput. Educ. 70, 29–40. doi:10.1016/j.compedu.2013.07.033
Mikropoulos, T. A., and Natsis, A. (2011). Educational Virtual Environments: A Ten-Year Review of Empirical Research (1999-2009). Comput. Educ. 56, 769–780. doi:10.1016/j.compedu.2010.10.020
Moreno, R., and Mayer, R. E. (2004). Personalized Messages that Promote Science Learning in Virtual Environments. J. Educ. Psychol. 96, 165–173. doi:10.1037/0022-0663.96.1.165
Moro, C., Štromberga, Z., and Stirling, A. (2017). Virtualisation Devices for Student Learning: Comparison between Desktop-Based (Oculus Rift) and mobile-based (Gear VR) Virtual Reality in Medical and Health Science Education. Australas. J. Educ. Tec 33. doi:10.14742/ajet.3840
Mousavia, M., Jenb, Y. H., Nurmaya, S., and Musac, B. (2013). A Review on Cybersickness and Usability in Virtual Environments. Adv. Eng. Forum 10, 34. doi:10.4028/www.scientific.net/AEF.10.34
Mütterlein, J. (2018). “The Three Pillars of Virtual Reality? Investigating the Roles of Immersion, Presence, and Interactivity,” in 51st Hawaii International Conference on System Sciences (HICSS). doi:10.24251/HICSS.2018.174
Nichols, S., Cobb, S., and Wilson, J. R. (1997). Health and Safety Implications of Virtual Environments: Measurement Issues. Presence Teleop. Virtual Environ. 6, 667–675. doi:10.1162/pres.1997.6.6.667
Pan, Z., Cheok, A. D., Yang, H., Zhu, J., and Shi, J. (2006). Virtual Reality and Mixed Reality for Virtual Learning Environments. Comput. Graph. 30, 20–28. doi:10.1016/j.cag.2005.10.004
Parsons, T. D., and Rizzo, A. A. (2008). Affective Outcomes of Virtual Reality Exposure Therapy for Anxiety and Specific Phobias: A Meta-Analysis. J. Behav. Ther. Exp. Psychiatry 39, 250–261. doi:10.1016/j.jbtep.2007.07.007
Pears, M., and Konstantinidis, S. (2021). The Future of Immersive Technology in Global Surgery Education. Indian J. Surg., 1–5. doi:10.1007/s12262-021-02998-6
Piedra, J. A., Ojeda-Castelo, J. J., Quero-Valenzuela, F., and Piedra-Fdez, I. (2016). “Virtual Environment for the Training of the Hands in Minimally Invasive Thoracic Surgery,” in 2016 8th International Conference on Games and Virtual Worlds for Serious Applications (VS-GAMES), 1–4. doi:10.1109/VS-GAMES.2016.7590375
Polcar, J., and Horejsi, P. (2015). Knowledge Acquisition and Cyber Sickness: a Comparison of Vr Devices in Virtual Tours. MM Sci. J. 2015, 613–616. doi:10.17973/MMSJ.2015_06_201516
Potdevin, D., Clavel, C., and Sabouret, N. (2021). A Virtual Tourist Counselor Expressing Intimacy Behaviors: A New Perspective to Create Emotion in Visitors and Offer Them a Better User Experience? Int. J. Human Comput. Stud. 150, 102612. doi:10.1016/j.ijhcs.2021.102612
Price, M., and Anderson, P. (2007). The Role of Presence in Virtual Reality Exposure Therapy. J. Anxiety Disord. 21, 742–751. doi:10.1016/j.janxdis.2006.11.002
Psotka, J. (1995). Immersive Training Systems: Virtual Reality and Education and Training. Instr. Sci. 23, 405–431. doi:10.1007/bf00896880
Radianti, J., Majchrzak, T. A., Fromm, J., and Wohlgenannt, I. (2020). A Systematic Review of Immersive Virtual Reality Applications for Higher Education: Design Elements, Lessons Learned, and Research Agenda. Comput. Educ. 147, 103778. doi:10.1016/j.compedu.2019.103778
Rahm, S., Wieser, K., Wicki, I., Holenstein, L., Fucentese, S. F., and Gerber, C. (2016). Performance of Medical Students on a Virtual Reality Simulator for Knee Arthroscopy: an Analysis of Learning Curves and Predictors of Performance. BMC Surg. 16, 14. doi:10.1186/s12893-016-0129-2
Rasheed, F., Onkar, P., and Narula, M. (2015). “Immersive Virtual Reality to Enhance the Spatial Awareness of Students,” in Proceedings of the 7th International Conference on HCI, IndiaHCI 2015, Guiwahati, India, December 17-19, 2015 (New York, NY, USA: Association for Computing Machinery), 154–160. doi:10.1145/2835966.2836288
Ray, A. B., and Deb, S. (2016). “Smartphone Based Virtual Reality Systems in Classroom Teaching - A Study on the Effects of Learning Outcome,” in 2016 IEEE Eighth International Conference on Technology for Education (T4E), Mumbai, India, January 16, 2017 68–71. doi:10.1109/T4E.2016.022
Riva, G., Mantovani, F., Capideville, C. S., Preziosa, A., Morganti, F., Villani, D., et al. (2007). Affective Interactions Using Virtual Reality: The Link between Presence and Emotions. Cyberpsychol. Behav. 10, 45–56. doi:10.1089/cpb.2006.9993
Rizzo, A., Parsons, T. D., Lange, B., Kenny, P., Buckwalter, J. G., Rothbaum, B., et al. (2011). Virtual Reality Goes to War: A Brief Review of the Future of Military Behavioral Healthcare. J. Clin. Psychol. Med. Settings 18, 176–187. doi:10.1007/s10880-011-9247-2
Salzman, M. C., Dede, C., Loftin, R. B., and Chen, J. (1995). The Design and Evaluation of Virtual Reality-Based Learning Environments. Presence Teleop. Virtual Environ., 293–316. (special issue on education).
Salzman, M. C., Dede, C., Loftin, R. B., and Chen, J. (1999). A Model for Understanding How Virtual Reality Aids Complex Conceptual Learning. Presence Teleop. Virtual Environ. 8, 293–316. doi:10.1162/105474699566242
Schofield, D. (2012). “Mass Effect: A Chemical Engineering Education Application of Virtual Reality Simulator Technology. 8 (1), 17
Seo, J. H., Smith, B. M., Cook, M., Malone, E., Pine, M., Leal, S., et al. (2018). “Anatomy Builder VR: Applying a Constructive Learning Method in the Virtual Reality Canine Skeletal System,” in Advances in Human Factors in Training, Education, and Learning Sciences, Advances in Intelligent Systems and Computing. Editor T. Andre (Cham: Springer International Publishing), 245–252. doi:10.1007/978-3-319-60018-5_24
Sharar, S. R., Miller, W., Teeley, A., Soltani, M., Hoffman, H. G., Jensen, M. P., et al. (2008). Applications of Virtual Reality for Pain Management in Burn-Injured Patients. Expert Rev. Neurotherapeutics 8, 1667–1674. doi:10.1586/14737175.8.11.1667
Sharples, S., Cobb, S., Moody, A., and Wilson, J. R. (2008). Virtual Reality Induced Symptoms and Effects (VRISE): Comparison of Head Mounted Display (HMD), Desktop and Projection Display Systems. Displays 29, 58–69. doi:10.1016/j.displa.2007.09.005
Shu, Y., Huang, Y.-Z., Chang, S.-H., and Chen, M.-Y. (2019). Do virtual Reality Head-Mounted Displays Make a Difference? A Comparison of Presence and Self-Efficacy between Head-Mounted Displays and Desktop Computer-Facilitated Virtual Environments. Virtual Reality 23, 437–446. doi:10.1007/s10055-018-0376-x
Skarredghost (2017). The Sense of Taste in Virtual Reality. The Ghost Howls. Available at: https://skarredghost.com/2017/07/07/sense-taste-virtual-reality/ (Accessed 6 3, 2018).
Slater, M., and Wilbur, S. (1997). A Framework for Immersive Virtual Environments (FIVE): Speculations on the Role of Presence in Virtual Environments. Presence Teleop. Virtual Environ. 6, 603–616. doi:10.1162/pres.1997.6.6.603
Slater, M. (2017). “Implicit Learning through Embodiment in Immersive Virtual Reality,” in Virtual, Augmented, and Mixed Realities in Education, Smart Computing and Intelligence. Editors D. Liu, C. Dede, R. Huang, and J. Richards (Singapore: Springer), 19–33. doi:10.1007/978-981-10-5490-7_2
Smith, S. M., and Vela, E. (2001). Environmental Context-dependent Memory: A Review and Meta-Analysis. Psychon. Bull. Rev. 8, 203–220. doi:10.3758/bf03196157
Spira, J. B., and Feintuch, J. B. (2005). The Cost of Not Paying Attention: How Interruptions Impact Knowledge Worker Productivity. New York, NY: Report from Basex.
Stevens, J. A., and Kincaid, J. P. (2015). The Relationship between Presence and Performance in Virtual Simulation Training. OJMSi 03, 41–48. doi:10.4236/ojmsi.2015.32005
Sutcliffe, A., Gault, B., and Shin, J.-E. (2005). Presence, Memory and Interaction in Virtual Environments. Int. J. Human Comput. Stud. 62, 307–327. doi:10.1016/j.ijhcs.2004.11.010
Sylaiou, S., Mania, K., Karoulis, A., and White, M. (2010). Exploring the Relationship between Presence and Enjoyment in a Virtual Museum. Int. J. Human Comput. Stud. 68, 243–253. doi:10.1016/j.ijhcs.2009.11.002
Veronez, M. R., Gonzaga, L., Bordin, F., Kupssinsku, L., Kannenberg, G. L., Duarte, T., et al. (2018). “RIDERS: Road Inspection & Driver Simulation,” in 2018 IEEE Conference on Virtual Reality and 3D User Interfaces (VR), Tuebingen/Reutlingen, Germany, August 18-22, 2018 715–716. doi:10.1109/VR.2018.8446207
Vogel, J. J., Greenwood-Ericksen, A., Cannon-Bowers, J., and Bowers, C. A. (2006). Using Virtual Reality with and without Gaming Attributes for Academic Achievement. J. Res. Techn. Educ. 39, 105–118. doi:10.1080/15391523.2006.10782475
Weech, S., Kenny, S., and Barnett-Cowan, M. (2019). Presence and Cybersickness in Virtual Reality Are Negatively Related: A Review. Front. Psychol. 10, 158. doi:10.3389/fpsyg.2019.00158
Winter, J., Cotton, D., Gavin, J., and Yorke, J. D. (2010). Effective E-Learning? Multi-Tasking, Distractions and Boundary Management by Graduate Students in an Online Environment. ALT-J 18, 71–83. doi:10.1080/09687761003657598
Witmer, B. G., and Singer, M. J. (1998). Measuring Presence in Virtual Environments: A Presence Questionnaire. Presence 7, 225–240. doi:10.1162/105474698565686
Keywords: presence, memorization, learning, virtual reality, immersion, environment, noise
Citation: Ochs C and Sonderegger A (2022) The Interplay Between Presence and Learning. Front. Virtual Real. 3:742509. doi: 10.3389/frvir.2022.742509
Received: 16 July 2021; Accepted: 14 January 2022;
Published: 18 February 2022.
Edited by:
Richard Skarbez, La Trobe University, AustraliaReviewed by:
Aleshia Hayes, University of North Texas, United StatesGeorge Papagiannakis, University of Crete, Greece
Copyright © 2022 Ochs and Sonderegger. This is an open-access article distributed under the terms of the Creative Commons Attribution License (CC BY). The use, distribution or reproduction in other forums is permitted, provided the original author(s) and the copyright owner(s) are credited and that the original publication in this journal is cited, in accordance with accepted academic practice. No use, distribution or reproduction is permitted which does not comply with these terms.
*Correspondence: Andreas Sonderegger, andreas.sonderegger@bfh.ch