The integration of extended reality for student-developed games to support cross-curricular learning
- Faculty of Education, University of Canterbury, Christchurch, New Zealand
While eXtended Reality (XR) ha s been shown to provide rich promise, its adoption within the educational context for student created games is still limited. Recent advances in XR technology, especially in mobile XR tools, have made XR more accessible. These advances have also enabled the development of student-created XR experiences that provide opportunities for integrating learning with technology skills development. Through the integration of critical digital skills across the curriculum, students can demonstrate a range of learning outcomes across many different learning areas. In this study, we explore how the affordances of XR can be leveraged to enable new learning opportunities, specifically in enabling students to design their own XR learning experiences. We also explore how the added context of XR games provides additional benefits to engage and motivate learners. In this article, we identify and explore twelve affordances framed in the notions of engagement, authenticity and contextualisation. These affordances have been identified in the literature to highlight the benefits of XR and were explored in terms of how the added context of student-created games leveraged these affordances. Drawing on a wider research project, we identify three scenarios that were adopted by three teachers (Mathematics, Science and Language teacher) to teach a range of subjects that drew on XR student-created games. From this analysis, we conclude with six proposed design principles that could be adopted by other teachers to help guide them in applying a similar approach in their teaching.
Introduction
Digital technology (DT) has fundamentally changed how we engage with learning and, as technology continues to evolve, educators have sought new ways to bring technology into the classroom. Recent advances, especially in mobile technology, have meant new tools such as virtual (VR), mixed (MR), and augmented reality (AR), have become more viable as learning media in the classroom. The evolution of these technologies has also meant new terminology and concepts have arisen. The term extended reality (XR) has been recently coined to represent the different ways in which we can interact with virtual systems (Margrett et al., 2022). XR provides an umbrella term that brings together these different technologies under one banner to represent their abilities to go beyond a physical space and extend reality (Çöltekin et al., 2020). The immersive nature of XR provides the ability to draw in a user, where the digital sits over the physical space (AR) or draws the user fully into a fully digital environment (VR) or combines these approaches somewhere in between the two (MR). It is this immersive nature that holds great promise for education.
XR is increasingly used to provide immersive learning opportunities, providing significant benefits to the learner (Bacca et al., 2014; Di Natale et al., 2020). Though the adoption of XR is growing in schools, its application still tends to focus on students using ready-made experiences, where students are the consumers, not the creators, of the XR experience (Di Natale et al., 2020). The adoption of XR has generally been constrained by the cost of tools and software development as well as the fairly narrow applications of XR, which tend to focus on narrow learning outcomes within specific learning areas, such as within medical education (Parsons and MacCallum, 2021).
The development of new technologies, including low-cost mobile XR has, however, meant new opportunities for schools to integrate immersive virtual environments into their teaching in a more feasible manner, where the tools used to experience XR are now more readably available (Radianti et al., 2020). Enabling students to create their digital artefacts has been shown to provide greater engagement and outcomes for all students (Ananiadou and Claro, 2009). The potential for students to create their own immersive experiences also means that students move away from just being consumers of these experiences, and can become developers. This approach means learners can apply a wider range of skills and knowledge to develop virtual artefacts and environments and supports cross-curricular learning where students not only develop their coding skills but also engage with a range of subject-specific knowledge in the process. Learning, therefore, happens in the development of the XR experience, not just its use.
While the area of student-created XR artefacts is still evolving, the focus on student-created games is also growing. Supporting students to create their own games provides further opportunities to engage and motivate students as well as support the development of key technical and collaborative skills (Kafai and Burke, 2015). Games in learning (often referred to serious games) have the potential to make learning more student-centred, engrossing, enjoyable, interesting and thus, more effective and efficient (Anastasiadis et al., 2018).
In this article, we explore how the creation of XR games can enable learning that is cross-curricular and focuses on students developing artefacts rather than consuming digital technologies. Exploring this through an affordance lens, the article identifies the pivotal affordances of immersive technology and identifies design principles that can then be used by teachers to integrate XR gaming into schools, focused on student-created artefacts. As highlighted in Johnson-Glenberg (2018), there are few guidelines on how to make optimal educational content in VR, and the added contexts of AR, gaming and student creation provides additional complexity. Therefore in this article, we propose several concrete design principles aimed at extending the research in this area. Through the integration of student-created XR with gaming contexts we hope to leverage the benefits of both contexts to offer new and exciting ways to support student learning.
Drawing from a larger research project, the article examines how a group of teachers have integrated XR into their classes. The article focuses on three scenarios that drew on XR gaming approaches. Adopting a design-based approach, we explore how these three scenarios leverage the affordances of applied immersive gaming for cross-curricular learning to identify design principles that can be then used to support further development of these sorts of approaches. To address this, the article will focus on two research questions:
1. How can the affordances of XR be leveraged to support cross-curricular learning that focuses on gaming contexts?
2. From these affordances, what design principles can be proposed that can support the adoption of student-created XR gaming?
The article adopts a qualitative approach to examine these questions. The focus of this study is exploratory, the ideas identified were derived through examining the student artefacts and teacher lesson plans. Discussions with the teachers were then undertaken to explore how the technology was used in their classes and how this integration related to the relative affordances identified in the literature. As an exploratory study, it examines the approaches adopted along with the teacher perspectives, and the study explores how their approaches can be mapped to twelve affordances. The twelve affordances were identified from the literature however relate to either AR or VR contexts and not specifically gaming. Therefore the contribution of this study is to determine how well these affordances are situated in the broader concept of XR and within a gaming context. From these, the study then proposes six design principles that extend out of the affordances. These design principles are offered as a starting point for other teachers interested in adopting XR technology across different curriculum areas focused on students’ created experiences. The study highlights that adopting an affordance lens provides an effective approach to understand the influence of XR and how design principles can then be leveraged from this analysis. While the study provides a starting point to address the gap in the literature exploring how student-created XR artefacts can be used in different curriculum areas, it also addresses how the gaming context can fame and deepen the engagement in these contexts. While the study proposes both affordances and design principles these concepts require more research to validate.
Background
This article is developed out of a larger study undertaken over 2020–2021 (Author to add Reference after blind review). The study was developed to explore how the authentic integration of XR technology can support the meaningful integration and development of digital artefacts to support learning across the curriculum. The context of the study focused on exploring how two high schools in New Zealand were engaging with digital technologies to support learning across different subject areas. The emphasis of the study was exploring how XR could be used to move students beyond being just passive users of digital technologies to being active developers and designers of digital solutions.
The wider study was prompted by the recent changes within the NZ school curriculum (NZC). The changes to the curriculum were designed to support students to develop the digital literacies vital to engage in an increasingly digital society (Ministry of Education, 2017). The changes resulted in the strengthening of the digital technologies | hangarau matihiko (DT|HM) curriculum content with two areas being added to the Technology Strand of the curriculum: computational thinking (DTCT), and designing and developing digital outcomes (DDDO). Reorienting the use of technology away from consumption to creation has meant students need to be supported to become active creators of digital artefacts. The changes have been designed to be wide-reaching and have emphasised that digital technologies, as a subject, needs to be integrated from the first year of schooling, and the design and development of digital artifacts need to be conceptualized in authentic contexts across the curriculum (Ministry of Education, 2017). This influence has implications that the teaching of, and development of, digital technologies will need to be integrated across the curriculum and not taught in siloed subject that is not contextualised or applied across the curriculum.
Materials and methods
Research design
This larger study adopted a participatory action research (PAR) design to investigate and explore how XR could be framed within the changes to the NZC to support cross-curricular learning. PAR is a qualitative research methodology and is considered a subset of action research, which is the “systematic collection and analysis of data for the purpose of taking action and making change” by generating practical knowledge (Gillis and Jackson, 2002, p.264). Action research has been widely adopted within educational research, as it provides the opportunity to develop a cyclic process of research, reflection and action.
The participatory aspect of this research enabled the teachers to play an integral role in all phases of the project (MacDonald, 2012). The approach adopted a collective, self-reflective inquiry that researchers and participants undertake, so they can understand and improve upon the practices in which they take part and the situations in which they find themselves (Kemmis, 2011). Adopting PAR to examine how XR can be used in the classroom allows for a reflective process, led by teachers working with others in teams or as part of a “community of practice” to improve the way they address issues and solve problems (Stringer, 2013).
The study drew on two action cycles of interaction and reflection. It supported these cycles of action through workshops that provided teachers with technological, pedagogical, and content knowledge around key concepts within the digital technologies curriculum. The focus of these workshops was to provide the teachers with a foundational knowledge of the technologies that they could use in their classroom.
At the end of each action cycle, their approaches and perceptions were gathered through reflective diary entries, focus groups and interviews. Students’ work and teachers’ lessons were also collected to understand the approaches taken and were used to better understand how XR was used within each subject. This article examines XR was used to create different artefacts to demonstrate different ways that XR games could be integrated to support learning across the curriculum. The teachers’ perceptions, derived from the qualitative data, have also been used to inform the discussion. The focus of the study was not to test these ideas or to determine the learning benefit, rather the approach was to explore how XR could be integrated into different subjects. Data was only derived from analysing the approaches used by the teachers and ideas tested through the discussion with the teachers. No student data was collected.
The affordances of extended reality in education
To explore the affordances of XR, the study examined the different approaches taken by the teachers to support the cross-curriculum integration of different technologies aligned with the technical affordance of the technology. Affordances are potential actions and interactions that the environment offers (Greeno, 1994). Markus and Silver (2008) extend this definition by including what they call “functional affordances”, which is “defined as the possibilities for action afforded to specified user groups by technical objects” (Markus and Silver, 2008, p. 622). Adopting an affordance lens to analyse the different ways that the teachers adopted XR into their classes provides a level of abstraction that goes beyond the specific context of adoption. Affordances are relatively generalizable and constant across specific implementations, so are not tied to a particular approach. Analysing the different approaches adopted by the teachers enables them to be mapped against a set of affordances. While these approaches are contextual, the underlying affordances provide a more holistic view of the opportunities provided by XR and therefore provide a wider view, which helps teachers identify how XR could be reconceptualized in different contexts. As emphasized by a teacher involved in the study:
It’s important to try to work out what lends itself to what you’re trying to do and not just having it as an extra - ‘a just because’. Making it an important part of the learning is important… this will make the use beyond the gimmick and rather provide a solid learning component. (Mathematics Teacher)
In the literature, several studies have explored the affordances of different types of XR. For example, Johnson-Glenberg (2018) identified two “profound affordances” of VR, namely 1) the feeling of presence supported by the immersion in the digital environment, and 2) the embodiment and the subsequent agency associated with manipulating content. While Dalgarno and Lee (2010), offers five affordances that are driven by using three dimensional (3D) VR learning environments (VLE). On the other hand, Wu et al. (2013) identify five affordances of AR in the learning environment. Table 1 highlights these different affordances addressed by these different authors.
While each affordance conceptualized in the technology focuses on VR/VLE and AR, is some crossover. While on the MR continuum, where the blend exists between the two extremes of the users where learners are either immersed in the digital environment (VR) or where the physical location gives context and meaning to the digital (AR), many of the same affordances can be drawn together but interpreted in different ways under the banner of XR.
Notions of affordance
In order to make it easier to conceptualise and relate the different affordances identified in the literature we have adopted the approach of using notions to relate and group affordances. Adopting Wu et al. (2013)’s three notions of engagement, authenticity, and contextualization, we used these notions to map the additional affordances identified by Johnson-Glenberg (2018) (A1-2) and Dalgarno and Lee (2010) (A3-7). These notions, however, have significant cross-over so in some cases, affordances could map to more than one notion. However in this case we aligned it to the notion that was more dominate and related to Wu et al. (2013)’s original concept of these notions.
Engagement
According to Wu et al. (2013), AR offers specific approaches, such as simulations and role playing, that reinforce the roles that learners plays in an environment. By engaging them in the environment and providing them with roles they can play or simulations that they can explore, this could then enhance the learners sense of presence, immediacy, and immersion of the learner (A8). For example, role playing in AR allows students to feel being there and provides comprehensive, realistic experiences that can only be achieved in an extended reality. Whereas simulations can provide hands on experiences and bring the learners into new environments which allow them to experiment.
The notion of engagement is therefore captured by how the learning is driven through the use of the technology. The nature of the technology to capture the students’ interest can be a significant factor to drive higher engagement (Tsay et al., 2020). Engagement is a complex concept that is highly multidimensional, dynamic, fluctuating, context-dependent, and interactive (Tsay et al., 2020). However, engagement is typically explored as being either, cognitive, affective, or behavioural. Cognitive engagement relates to the learners’ efforts to understand and comprehend what is being taught. Affective engagement relates to the feelings concerning their learning experience, either positive or negative, and the connection they feel when engaging with their learning and with others. Behavioural engagement relates more to the observable behaviours that lead to academic success (Fredricks et al., 2016).
In general, the identified affordances tended to relate to emotional engagement—where students feel connected and motivated in their learning. For example, in VR, students feel a sense of presence through their immersion (A1). The physical act of bringing the learner into the virtual world enables a deeper sense of connection to their learning. While this engagement can bring a strong sense of presence, immediacy, and immersion. While this connection may be driven through the novel effect of the experience, the learning is still impacted. While novelty can often be dismissed as being temporary (Tsay et al., 2020) it can also be a vital component of intrinsic motivation (A5) (Benlahcene et al., 2021). Novelty is seen as important in eliciting emotions and memorability of an experience (Mitas and Bastiaansen, 2018) and positively related to behavioural, emotional and cognitive engagement (Benlahcene et al., 2021).
Authenticity
AR can facilitate more task based learning as it can support the conceptualising of complexity concepts (Wu et al., 2013). By presenting content, tasks, or problems from different perspectives, and in terms of objects in a 3D space (A9), learning can be made more visible and explicit (A11) but also where learning happens through the engagement and manipulation (A2). By providing learners with a way to reframe and better understand concepts can make learning more authentic.
In AR the authenticity is provided by being embedded in a location (Rosenbaum et al., 2007). However, authenticity can also happen in VR, where the virtual is substituted for the real. This is especially true where learners can fully engage and manipulate virtual objects. But also captures the concept that both AR and VR enable the visualisation of concepts, events or interact with objects that are invisible or would otherwise be impossible to do in the real world (A4). This engagement can often make learning more connected and drive a richer experience (A7).
Contextualization
The third notion identified by Wu et al. (2013) is contextualization. The ability for AR technology to support location-based learning approaches enable learners to engage with the physical environment. The ability to move learning outside the classroom means learning can be supported in formal and informal contexts (A12) where ubiquitous nature of the technology can support more collaborative and situated learning (A10).
Therefore in this study, contextualization can be defined as the “utilization of particular situations or events that occur outside of class or are of particular interest to students” (Rivet and Krajcik, 2008, p. 80). Contextualisation can also happen in VR where learning is driven by engaging in a virtual spaces. It is the engagement in these spaces that the context can take on additional affordances, such building into the experience spatial knowledge or awareness. A virtual experience can allow for the learner to move around and explore the environment (A3). In an AR experience the overlay of digital content can also provide for spatial knowledge helping user orientate and better engage with the physical space.
Contextualization can also be considered in terms of how the learning is transferred into real situations (A6). Virtual reality can provide the context to learn and apply new skills that can then be applied in the real context. Often these virtual experiences provide the ability for learning to happen that would otherwise be risky or difficult. VR experiences can provide levels of visual or sensory realism and interactivity consistent with the real world which can then be more readily recalled and applied within the real environment (Dalgarno and Lee, 2010). So while according to Dalgarno and Lee, (2010) VR can support learning that is situated in the virtual and then applied in real life, AR experience can also situate learning where the digital support the learning in the real.
The affordances of extended reality for student-created artefacts and gaming
The above affordances only focus on the properties of either AR or VR in education and not XR in general. It also did not recognise the specific context of this study of students creating these experiences or specifically related to the gaming context. These affordances focus on the adoption of XR as a tool and do not necessarily consider the context of how they are used. In particular, how do these XR affordances support students to create their own digital artefacts? Also, how does the context of XR games impact and drive these affordances? Therefore, in the next section, we start to explore how these affordances can be conceptualised through the examination of three scenarios. These scenarios were derived from the larger research project as they best captured the affordances of XR for student-created artefacts and gaming.
The next section starts with a brief description of the three different examples of how XR was integrated into the classroom and focused on students creating XR games. From this, we explore how these examples leveraged the affordance of XR and then propose a set of design principles that can be used to drive future adoption.
Three scenarios showcasing student-created extended reality games
The following three scenarios were selected to provide examples of how XR could be adapted to different contexts. While mathematics, science and languages were the basis for the three scenarios these subjects could also be substituted with other subjects using similar ideas. For example, the maths trail could have been adopted in the science class where students need to undertake an experiment at each location. The escape room could have been used to test any subject knowledge, Minecraft has been adopted to teach a range of subjects, from maths to science.
While these scenarios were not selected for their uniqueness or originality they were rather selected for their ease of portability into other subject areas. In addition, the scenarios were selected for their do ability. Rather than relying on sophisticated software (all software cloud-based so schools with limited technological capabilities could also adopt) and knowledge (where the expertise of the students could be scaffolded), these are examples that many teachers can implement in their own classes with limited experience.
Approach one: Augmented reality maths trail
AR extends the real environment, in this case students use the AR app to locate and solve set math problems. Maths trails, similar to scavenger hunts, have been adopted in the mathematics class to enable students to work collaboratively on mathematical tasks to solve situated problems. Math trails are a learning approach that requires students to engage with physical objects often situated in a school’s or city’s surroundings where students need to navigate around these locations to solve several problems (Buchholtz et al., 2020). In this scenario, students worked in groups to create different maths problems that would require learners to find a location and then engage with the objects in this environment to solve the problem, drawing on concepts they had learnt in the mathematics class. Each student group then combined their activity into a joint AR game. These games were then shared with their peers to test and extend their learning.
In this activity, students used a content creation tool, Metaverse (https://studio.gometa.io/), to design and develop an interactive game other students would then use to test their mathematical knowledge. Metaverse is an online platform that enables students to create interactive AR content. Metaverse provides a studio tool that enables students to develop sophisticated AR applications, including using features such as GPS coordinates, leader boards and interactive quizzes. The Metaverse Studio enabled students to apply simple coding concepts such as flowcharts and algorithmic thinking to design their application (Figure 1).
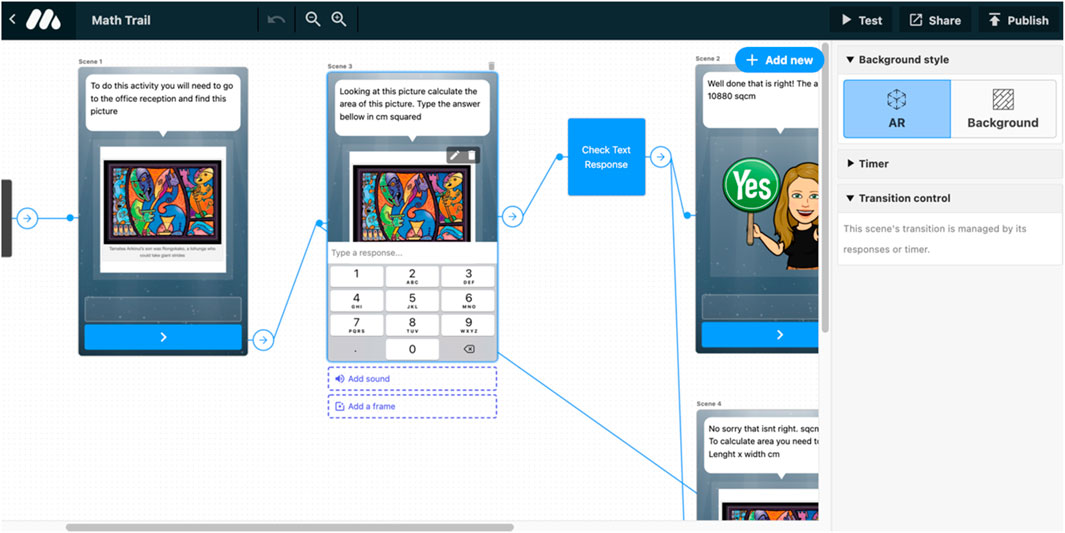
FIGURE 1. The Metaverse Studio that students used to create the AR application. The tool can be used to scaffold simple computational concepts such as flowcharts and algorithms.
The students created an AR app that would get the game player to navigate around the school using GPS coordinates, and at each location get the user to undertake a specific task, applying mathematical concepts they have learnt, to solve the maths problem. In this example, students combined gaming concepts of leader boards, time-based activities and interaction to create a resource that could be then shared with others. Figure 2 shows a sample question used in the maths trail where students need to find a specific picture hanging in the school and then calculate the area of the picture.
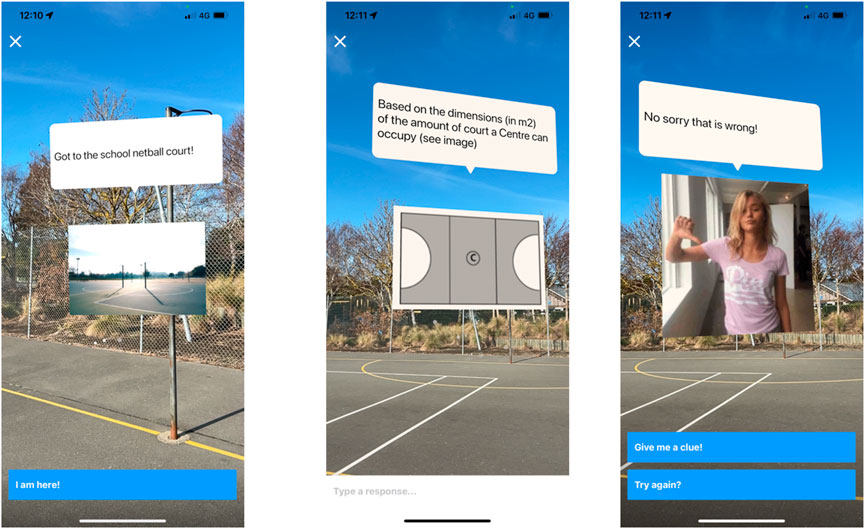
FIGURE 2. Sample question from the Maths Trail created in Metaverse. Students need to go the netball court and work out the area indicated in grey. Students needed to use their phones to measure the length of the court and then calculate the area.
Approach two: Virtual escape rooms
Escape rooms are a popular recreation game, where players are typically locked into a physical room and then need to solve a series of problems to escape the room. These activities are usually timed and require the player to apply logic and knowledge to solve a series of different problems. Due to the popularity of these games, digital escape rooms have also been created in a virtual environment where game players need to escape virtual rooms by solving problems, these can be in collaboration or individually.
In this scenario, students in their science class create their own virtual rooms with a series of questions that players needed to answer to be able to finish the game and exit the room. In this activity students were asked to come up with a series of questions about their topic (space) and then used an online platform, CoSpaces (https://cospaces.io/edu/), to develop and code their own escape room. Students were given the option to base their escape room on an existing template or they were able to make their own virtual escape room from scratch. Figure 3 shows an example based on the Space Escape template.
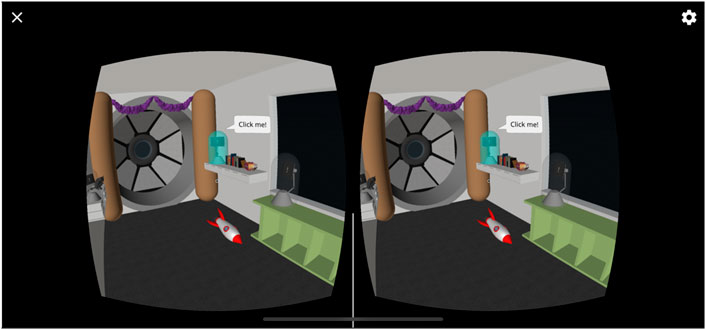
FIGURE 3. The remixed template Space Escape room viewed in Stereoscopic View using a mobile device sitting in an HMD.
CoSpaces is an online platform where students can create both AR and VR experiences. In this example, students created a virtual world that could then be engaged with either through their mobile phones placed in a head-mounted display (HMD) or on their laptops. CoSpaces enables the development of fairly sophisticated interactive environments through block-based or script coding (Figure 4 shows the block-based coding environment). The use of the CoSpaces enabled students to use a range of different features that enabled for development of a system that Turing-complete platform, which allows for a wide range of functions and interactions to be coded into these environments. CoSpaces also allowed students to create their own environments, while many chose to remix the existing template, students could choose to create their own and integrate a range of ready-made objects and import other 3D objects into this CoSpaces integrated development environment (IDE).
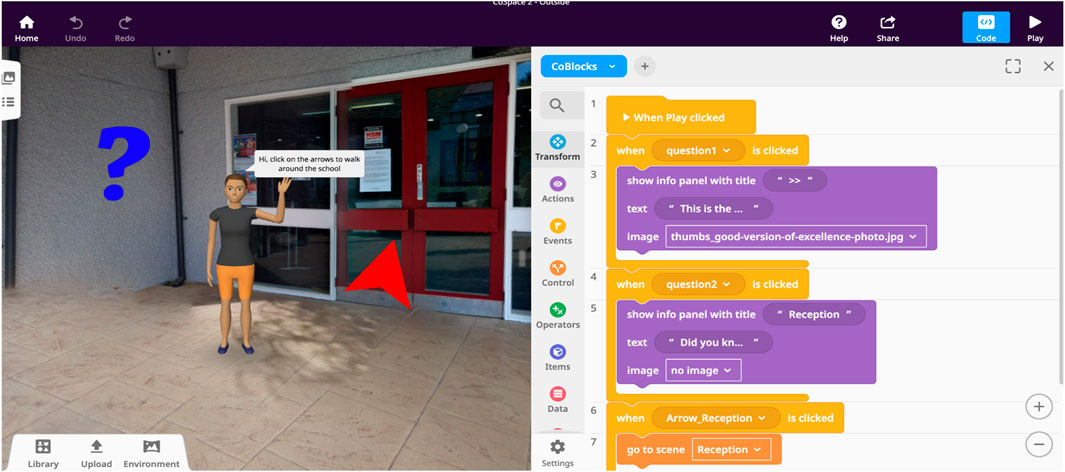
FIGURE 4. The CoSpaces studio environment where students create the interaction through block-based coding.
The approach provided the connecting of science with the creation of digital learning. As explained by the Science teacher the development enabled the students to;
… test their knowledge or research further, both to create it correctly and how to code it, in order to create what they want to create. It’s not just Science [content] it has also brought in the digital aspect as well, at the same time, without them ever really thinking about it they’re doing both together. So they’re actually learning both... The process of creation enabled them to apply their knowledge making sure that they actually understood what they had learnt but in a way that is applied and creative. They’re doing something they want to do. (Science Teacher)
The use of CoSpaces in this activity enabled students to apply their programming skills along with their knowledge from their science class to create an artefact that demonstrated their knowledge of their subject but also demonstrated their coding skills. Game-based activities such as score-keeping and leader boards helped to make the gaming aspects more engaging.
Approach three: Minecraft
Minecraft is an open-ended sandbox game, that was created to enable children to explore and create their own virtual worlds. While the original concept was to create a multiplayer sandbox building game (Ekaputra et al., 2013) the game has grown in complexity and now includes a Minecraft Edu Edition specially created to support learning. The design and interface of Minecraft is relatively simple, with the play centred around creating and engaging with block-based structures, either alone or collaboratively. Minecraft has also evolved into a fully immersive VR environment where users can interact via HMD. However, in the case of this scenario, the students engaged via a computer or tablet. This definition of VR was therefore more related more to Dalgarno and Lee’s (2010) concept of “immersive virtual environments” where “desktop virtual environments” can still provide a similar experience to one where students where HMD. So, while this was not true immersion in the technical sense, the students were cognitively immersed in a fully virtual world. In addition, the use of computers and tablets to engage with these virtual environments meant that students could more easily engage and talk with each other.
In this scenario, Minecraft was included into a Japanese class for students to practice and engage with their Japanese lessons. In this approach, the teacher used Minecraft in several ways, including students creating their own Japanese village (Figure 5) with each student creating a house in the village. Students were then asked to create a video tour through their house demonstrating their understanding of Japanese culture as well as their ability to communicate in Japanese (Figure 6). These houses were created into a virtual world where students could collaborate and apply their learning in a fun, engaging way.
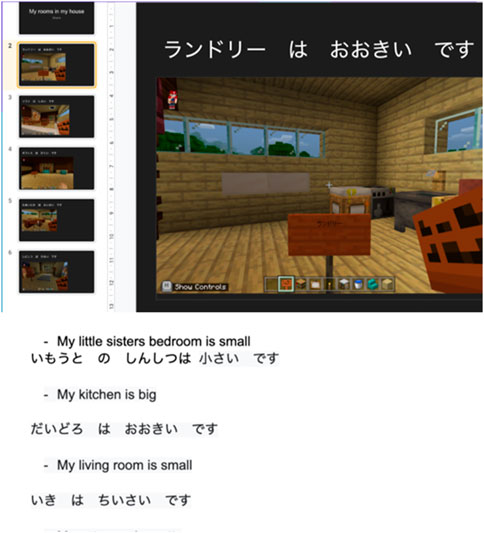
FIGURE 6. The PowerPoint slide containing the images and transcript a student used to create their walkthrough.
In this context, students were not using coding to engage with the virtual world; however, this could have been an option for opportunities to reinforce specific outcomes from DT. So, while this example did not explicitly adopt coding in its design students were still developing their own digital artefacts and therefore had links to designing and developing digital outcomes. Gaming concepts were emphasised through the collaborative nature of the activity. Students could cooperate and engage with each other in a fun manner that drew on a more open nature of gaming that is supported by Minecraft.
Exploring the different affordances in each scenario
In this section, we will start to explore how the three scenarios conceptualised the different affordances of XR and how the context helped to reinforce these affordances. To support the discussion of affordance the discussion will be grouped in the three notions of engagement, authenticity, and contextualization.
Engagement
The use of XR has highlighted the benefits of learners feeling more immersed (Wu et al., 2013; Johnson-Glenberg, 2018) and present (Johnson-Glenberg, 2018; Wu et al. (2013) which in turn support a higher level of motivation (Dalgarno and Lee, 2010).
VR immersion is supported by the learners’ feeling of being physically present in a fully digital world (Slater, 1999). Some authors (Jacobson, 2017; Johnson-Glenberg, 2018), however, have highlighted that the sense of immersion can be negatively impacted when only partial immersion is supported. Whereby, partial immersion is where the user still is connected to the real environment when engaging in the virtual, typically in mobile VR experiences or virtual worlds. In addition, other research has stated that learning is not as rich and may not be retained as effectively when a learner is only partially immersed (Johnson-Glenberg et al., 2016).
In the scenarios, full immersion is not sought. Due to the limitations of fully immersive technology, this level of immersion was simulated through mobile sitting in an HMD (in the escape room scenario) or via the laptop (Minecraft scenario). So while full immersion was not necessarily realised in these scenarios, the feeling of immersion can also be stimulated in other ways, typically developed through how the XR experience is designed (Hamari et al., 2016). In game theory, a users’ sense of immersion can be supported through the design and mechanics of the gameplay. Techniques such as the game design and storyline can draw a user into the game and create a sense of immersion. This immersion means a user lose connection with the real environment and become immersed in the game and storyline (Brown and Cairns, 2004). In this concept, immersion can be stimulated through the engagement of the user not through the VR technology.
Immersion can also happen in AR. As highlighted by Wu et al. (2013) immersion can also happen in AR experiences, where there is no pretence of being fully immersed in the digital. The concept of immersion is considered beyond just the use of technology but rather the user immersion in a physical location. As highlighted by Wu et al. (2013), “immersion could make possible the learning situated in real-world problems, issues and environments” (p44). The immersion relates to the learner’s perceptions that they are engaging in a realistic experience. In the maths trail scenario, the location situated in the real world, learners needed to enact their learning by applying this around the school to solve the maths problems.
In the context of the Minecraft scenario, students were able to walk around their simulated Japanese village. So, while students were not expected to feel that they were actually in Japan, they, however, could still develop a sense of presence and learning from their immersion into the artificial world. So, in the context of COVID-19, where students were unable to travel to Japan, it still provided a powerful approach to provide a simulated feeling of presence. The use of Minecraft gave the sense of being in the space with others where you could interact and learn from the other students in the virtual world. Whereas the Maths Trail provided the ability to fully engage in the locations. The game was location-based so students had to be in the location to complete the puzzles.
In Wu et al. (2013) presence is also considered in terms of how AR can mediate a space and connect them with others. The sense of presence is therefore deepened through the connection with others. In the maths trail students cooperated to solve the problems. However, this cooperation went deeper than just the use of the game. Students also collaborated to create these games. Therefore, the sense of presence is further enhanced beyond the use of technology, whereby presence can be considered in a cognitive, environmental or emotional sense (Baños et al., 2004).
As highlighted in Wu et al. (2013), the integration of AR experiences could lead to new forms of behavioral, emotional, and cognitive engagement that need to be documented and theorized in educational research. In education theory, both a sense of immersion and presence is said to be created through students being fully involved in the learning experience. In Csikszentmihalyi (1997), flow theory states that when students are fully involved in the flow of their learning it promotes deeper engagement and motivation for learning (Schmidt, 2010). In this context, flow refers to “the state of mind characterized by focused concentration and elevated enjoyment during intrinsically interesting activities” (Hamari et al., 2016 p171). In the scenarios, the teachers reported students having a high level of motivation. Where the novelty of created an opportunity to capture their attention.
They were fully engaged in the activities but especially with the virtual reality and especially with the [VR] Goggles. They were like, “Wow, look at this!”. In the current situation, with COVID, it means you could bring the outside into the classroom. COVID has meant we can’t really go out and do a lot at the moment. That was something the kids were really excited about. When students realised that they could actually develop something they were very motivated and this pushed some to create some really remarkable experiences, more than I would have expected (Science Teacher).
The sense of flow is often seen when students are engaging with hands-on activities and when activities that require high skills and challenge can result in deep concentration, absorption and immersion (Schmidt, 2010). In the study, students needed to develop their skills to create their own experience, this contributed to a higher sense of engagement, where students pushed themselves and found the process of creating the digital artefact to trigger a higher sense of motivation.
Some of the best ideas came out of some of those not as abled groups… [the students that were] challenged mathematically and challenged in terms of focus and motivation… found someone within their group who had a bit of a flare for [technology]. So they sort of apportioned the jobs for the project... They all contributed in some way, and through the use of technology, I think it gave an extra dimension to what potentially could have been not as exciting or an interesting project. (Maths Teacher)
… once the girls knew what to do they were away, and they were doing things that none of the teachers knew what to do. And so, it’s giving them enough knowledge and empowering them to realise that they’ve got the skills and the facilities at their fingertips, in terms of YouTubing it or looking up a video or whatever, that they can take it further so that they actually use the technology to their best of their ability, and they don’t just settle for, “oh yeah I’ve done that task, I’m bored now”. (Science teacher)
The development provided a sense of fun and engagement as students needed to work together to create and play the games. As reported in the study, the way that the games were developed and used provided opportunities for students to engage and learn together. Students were required to direct their own learning to solve problems, however, they could also lean on each other’s skills to help support the development. The inclusion of the development of the artefacts, as a collaborative effort, enabled further shifts in the learning, beyond just the use of the experience (Fluck et al., 2016). This social learning approach also provided new opportunities for students to take ownership over their learning;
Students have been incredibly engaged in the process and have found ways in which to use AR and VR to help them understand concepts that would have otherwise been less accessible. Students have been able to build experiences for each other to enrich their learning also; this reinforces a strong sense of mahi tahi or sharing learning. (Digital Technologies Teacher)
The approach also gave new opportunities for students to showcase different skills and learn from each other.
There were some, of course, that had different strengths in the group, and they’d help the others. That was a great thing about the teamwork. They’d each lean on each other if they needed to, and they’d lead at other times. And so, that was pretty cool, and they could also ask other groups in the classroom. (Maths Teacher)
As highlighted by one of the mathematics teachers involved in the study, they related an example of one of their students who was usually withdrawn and had significant learning difficulties. This student however shone in the class, “he really took the project on, and became an amazing support in my class ... [By given the opportunity to exhibit his technical abilities] he took a stronger role in the class and learnt how to use the tool much more than I ever did. He would then take groups of students and teach them to code different things”.
Therefore, XR technologies can support users to be more immersed and present supporting a higher level of motivation and engagement. The development of student-created games deepens these concepts beyond the actual affordance of the technology.
Authenticity
As highlighted in Johnson-Glenberg, (2018), the authenticity of VR is supported by the embodiment and the subsequent agency associated with manipulating content. While students were able to manipulate content and engage with context in a 3D space in both the escape room and in Minecraft the experiences were not immersive. In these scenarios, as students were not fully immersed in the virtual experience, but rather only experienced these scenarios through either an HMD or desktop, both concepts of embodiment and agency could not be explicitly explored in these scenarios. Therefore Johnson-Glenberg, (2018) affordance was only captured in a limited sense.
In VLEs, this authenticity can mean that learning tasks, that would be impractical or impossible, can be supported in the VR environment. Authenticity can also support a rich range of engagement where learning can be supported through the “sense of place” that is created in the virtual environment (Dalgarno and Lee, 2010). The learning can be driven through engagement with this environment.
In Minecraft, the authenticity of the tasks was heightened by the virtual nature of the learning. While the focus was on getting students to demonstrate their language ability, the development of the village made the learning more contextual. The buildings were used to facilitate the virtual learning experience, where students could walk around the village and experience the environment. The opportunity to walk around these houses enabled students to engage with their learning in a new way and experience the different layout and building styles typical in a traditional Japanese village. The added 3D engagement provided new depth to the learning which would have been difficult to experience with 2D materials.
As highlighted by the Japanese teacher,
[Using Minecraft was more] appealing for [the students], and being a 3D world of course... It is very motivating, and lots of them have played or still played Minecraft themselves and are quite skilful, and they’re sharing tips and tricks with each other about how to make furnishings for their house. Some looked like a concrete block prison, and some of them looked amazing. So that was an advantage. Some of the girls who are not so good at the language were much better at the gaming side of it, and were able to feel in control of helping out the smart kids for a change, who might be more academic and not play games at all. So yeah, it was a good mix.
In this example, students had a sense of place driven through the Japanese village. It became to focus to support their learning and provided an authentic context to test their learning. Therefore, the simulated village meant students could walk around the simulated version and practice their vocabulary more authentically. The engagement with others is further reinforced through this sense of authenticity.
Authenticity in AR is, however, supported by presenting learning content in three-dimensional space and making the invisible visible. AR can enhance learning experiences by supporting the visualisation of digital 3D objects. These objects can then be manipulated to enhance understanding. The visualisation of 3D models has been shown to give students a better understanding of concepts, as these models can be explored and manipulated (Yuen et al., 2011). Supporting students to manipulate digital objects can extend the learning as it can more effectively demonstrate the properties of these objects than 2D pictures or video animation (Kaufmann, 2003).
When students engage with these 3D models in a 3D space (either virtual/real) learning can be inferred from the context in which these models are placed. For example, in Petrucco and Agostini (2016), AR technology was used on heritage trails. Digital 3D models were created that could be then overlayed physical buildings to give visitors a better understanding of the sites on location. The digital overlay made learning more contextual and helped visitors to better understand the information provided.
In our AR example, 3D models were not used in the maths trail. Rather the process of overlaying the digital information (the problem) supported the learning in the physical space. In this example, the learning was supported in the context of the 3D physical environment. As students needed to interact with the real (3D) environment to solve the problems the three-dimensional space played a fundamental role in supporting their learning. The role of the location was fundamental to the learning process, where students need to pick locations, create an appropriate maths question and then build this question in the AR tool. The location provided the context for authentic application of their learning and brings a sense of authenticity to the learning (Wu et al., 2013). As highlighted in Rosenbaum et al. (2007), the students’ “reality” is grounded in the location. In the maths trail the location becomes part of the learning and can draw the students’ focus, as they engage in the design and answer the math problems.
While XR provides the ability to engage in more authentic learning, the context of student-developed artefacts also supported higher levels of authenticity. In escape rooms and the maths trail, the focus was to situate digital technologies in more authentic contexts, where students could apply their digital skills in a more authentic context (outside of the DT classroom). Therefore, the creation of the artefacts further supported a deeper sense of authenticity. The development of these experiences supported the student to apply their knowledge of coding but made the learning more situational and connected.
[The students] weren’t just the end-user of the technology they were using it for educational purposes to present their ideas to get other students to look at their [artefacts] and then to also use them. [students were not just] exploring something someone else had made, but they had to make their own and had to figure out how to do it, and what steps to go through, and it was trial and error… students need to persevere and learn debugging skills, and even just the logic of the connecting things together and applying their learning…. there’s a lot more self-thinking, collaborative learning, less learning for a test and forgetting it afterwards and working with real broad problems. (Science teacher)
Therefore, while XR technologies support a level of authenticity around the learning expanding the focus to include the creation of these experiences provides further opportunities for authenticity.
Contextualization
Context is developed in AR through the interaction with the locations, whereby the digital is overlaid and can be context-sensitive to the physical. In AR this ubiquitous link between digital and physical can make learning more situated and collaborative. It also enables a link between in-class and outside of class (Wu et al., 2013). In the AR scenario, students engaged with their mobile devices. Through this portable experience students’ learning was contextualized in their environment, however this also provided the opportunity for this experience can also be shared (Furió et al., 2013). In the maths trail, the location triggered the activity. This supported learning to be more connected to the environment so it became a fundamental part of the learning. The environments provided the context to support their learning in a more authentic and situated manner. The approach provided the opportunity to support learning outside the classroom, embedded in the context where the students need to interact, such as physically measuring, counting or finding objects in their environment to complete the task. While the scenario was purely focused on formal learning contexts this could have easily have extended into informal learning.
Alternatively in VR contexts, contextualization is supported within the digital space. According to Dalgarno and Lee (2010), VLE facilitates learning tasks that lead to the development of enhanced spatial knowledge representation of the explored domain as well as provide the ability to transfer knowledge and skills learnt in the virtual to the real. This connection between virtual and real was evident in the Minecraft scenario, where students practised in a safe space of the virtual community.
While the tool itself can provide a sense of contextualization, the way that the learning is designed can also reinforce this sense of contextualization. The game-based features intergrated into the maths trail and the escape room game provided further opportunities for connecting learning. For the maths trail they were connected to the physical locations of the environment—with the questions only able to be answered by using the locations. However, in the escape room the contextualisation was to the science problems they needed to answer to get through the room. In the maths trail the ability through the use of the mobile device to move throughout different real-world places provided the sense of connection. However, other features could be used to provide a sense of contextualization, such as the playing style, the speed of their movements, times, changes in the environment, acceleration, and the manipulation of objects, among many other things (Thomas et al., 2004). Enabling students to compete with each other to solve these problems adds a sense of competition and further supported learning (Admiraal et al., 2011).
As highlighted by Wu et al. (2013), contextualization can be supported through the portability of AR, however, this portability enables social interactivity either digitally or face-to-face. XR can support engagement and interaction between students who are collocated or situated remotely. In many XR experiences, the co-exploration of experiences enables learning to be more authentic and engaging, and therefore provide the sense of contextualization. While VR provides some limitations around collaborative engagement, some VR experiences, such as in the Minecraft example, allow for easier sharing. Collaboration is especially common in virtual worlds, where participants can join a shared virtual space. Nevertheless, AR tends to support better engagement as sharing of screens and experiences is easier (Morrison et al., 2011).
The sense of collaboration can, however, also be heightened by the integration of game features in the learning. The use of serious games has been shown to encourage high levels of participation in and motivation for learning (Sánchez and Olivares, 2011). While the social aspect can engage students more it can also enhance their problem-solving skills. When performed in groups working as a team, problem-solving is strengthened as a learning activity (O’Neil et al., 2004). In the escape room and maths trail traditional approaches to game-based learning was applied, where mechanics of scoreboards, timed activities sat alongside the learning collaboratively. The application of the digital game promoted the sense of collaboration, where students needed to share information and learn from each other.
Collaboration provides opportunities for peer-teaching, collaboration, and one-on-one teacher guidance (Cook, 2010; Kamarainen et al., 2018). It can also help bring learners together. In this study, social engagement happened during the use of the experiences but also through the development of the artefacts. The Maths Trail created by students required the students to create different experiences at each location, which were combined into one experience. While students were working on these locations in isolation the product was a collective artefact, therefore requiring students to coordinate and collaborate to achieve the group goals. In the Escape Room and the Minecraft scenario, the XR products were developed so others could use these and learn from each other. Therefore, while the use could be collaborative and shared, the development drew on a social process. Students would support each other in the development of their artefacts “... [Students] were happy to go to [other students to help or assist with problems], or they’d sit down with someone and ask them questions, ‘How did you do this?’ There was a lot of peer teaching” (Science teacher).
Contextualisation can therefore be provided through the mechanics of XR to draw a user and contextualise learning within a location. Or this contextualisation can lie with how the learning activity is designed, whereby gaming and creation can support interaction with peers and focus the learning within this engagement.
Design principles for extended reality student-created games
The above scenarios showed that the integration of XR within the context of student-created game-like activities enabled the wider application of learning. The use of the XR technologies enabled learning to be more centred into a location and this drew on specific affordances of those locations (either real or digital) and then situated them fully into the environment. The XR also provided the ability for learners to engage with each other more authentically and to co-construct their learning, which was situated within the location. The added context of student-development artefacts within a gaming context enabled a richer experience and leveraged these affordances more deeply.
While the exploration of these approaches from an affordance lens has helped to articulate the possibilities of XR used in this context, the next step is to extrapolate these affordances to design principles. The extrapolation of these affordances to propose design principles will provide more concrete guides for educators to integrate XR into their teaching. Design principles “can be used to guide the design and development of learning environments in higher education that are based on sound practical and theoretical principles,” (Herrington and Reeves, 2011, p. 594). In this study, we identify five design principles that would guide the integration of XR student-created games across different subject areas (Table 2).
Design principles form the foundation of Design-Based Research (DBR). DBR is defined as “the systematic study of designing, developing and evaluating educational interventions (such as programs, teaching-learning strategies and materials, products and systems) as solutions for complex problems in educational practice, which also aims at advancing our knowledge about the characteristics of these interventions and the processes of designing and developing them” (Plomp, 2007, p.13). Therefore, as an outcome of this research we propose six design principles that would be the starting point for further exploration and adoption of XR.
Implications and barriers to the adoption
Design principles can help teachers with a starting point to integrate XR into their own contexts, however, every context is different. Therefore, while the study only focused on how XR could be used, it is also important to acknowledge that the integration technology, as explored in this study, requires teachers to have a deeper technical, pedagogical and content knowledge (TPACK). In order for teachers to effectively integrate the technology in their different subjects, as shown in the study, teachers need to expand on each of the knowledge domains. The TPACK (Koehler et al., 2011) is a model that helps explain the different knowledge domains required by teachers to integrate technology. The development of knowledge of the intersections can support effective teaching with and engage students with technology. Therefore, for teachers to be successful integrating technology in their classrooms they need to consider the following intersections, 1) understand the best practices for teaching specific content to the students (PCK), 2) know how digital tools can enhance or transform the content and how it can be best delivered to students (TCK) and then 3) understand how to use digital tools can support the specific learning outcomes required (TPK).
In the study, however, the use of the XR went further that just using the XR experiences, rather students needed to create these experiences. This therefore required the teachers to develop a deeper technological knowledge. Teachers needed specific knowledge around how to introduce the design and development of these XR experiences within their subjects. This meant that teachers and students need to have some familiarity beyond just the use but rather how to code with these specific XR tools.
Therefore, in order for teachers to be able to adopt similar approaches as addressed in this study, they needed to be able to adopt appropriate pedagogical approaches that support the development of digital artefacts. The development of digital artefacts required new approaches which are more learner-centred (Fluck et al., 2016). These approaches helped students to have more ownership of the learning and allowed students to extend the use of these tools. For example, in the Escape Rooms Scenario, the students were given a general focus but they had the autonomy to create their own escape rooms in the manner they wished, with different models, backgrounds and features. While the approach meant students have more scope to expand on ideas, the teachers need to be able to support this differentiation and scaffold the students to be more self-directed, especially when solving issue with the code and trying out new features.
Teachers also need to be able to blend the creation of digital artefacts within their own subjects. In this study the math trail and Minecraft scenario did not consider the design and development of the artefact in the marking, the coding of the artefacts was not the focus, rather the development was just the mechanism to frame the different subject. However, in the escape room scenario the Science teacher worked with the Digital Technology teacher to support the design and development of the artefact. The assessment integration between the two subjects provided more complexity. This complexity therefore required the two teachers to better understand how each subject was framed within each other, whereby the digital technologies learning outcomes could be assessed inside the Science class. Therefore, teachers wanting to integrate subjects, where both learning is assesses require a clear understanding of how the different subjects intersect. Teachers therefore need to be able to effectively weave together the two subjects to enable students to use its core concepts and dispositions from the digital technologies subject to solve discipline-specific and interdisciplinary problems.
Conclusion
In this article we established that XR student-created games provide rich promise for education, especially to drive learning across the curriculum. By examining three scenarios that adopted different approaches we evaluated how XR technology was fundamental in driving these experiences. The ability of XR to connect learning to a specific context, either supporting then to engage in a location or bringing in a learning meant the learning became more powerful. However the scenarios also illustrated that the ability for student-created XR experience further emphasised the affordances of XR technologies. By leveraging on a range of affordances we explored how the three notions of education (engagement, authenticity and contextualisation) can better understand how these affordances play a role in the adoption of XR but also how the context of student-created games and game-like activities can further support these notions. The study concludes with six proposed design principles to guide others interested in integrating XR for student digital skills development through students- created XR games. While the study provides a starting point for this discussion these design principles need to be further evaluated and tested. However, this study highlights an important first step into exploring how XR could be more fully leveraged into education.
Data availability statement
The datasets presented in this article are not readily available because of ethical limitations. Requests to access the datasets should be directed to KM, Kathryn.maccallum@canterbury.ac.nz.
Ethics statement
The studies involving human participants were reviewed and approved by Dr. Patrick Shepherd Educational Research Human Ethics Committee. The patients/participants provided their written informed consent to participate in this study.
Author contributions
The author confirms being the sole contributor of this work and has approved it for publication.
Funding
This study forms part of a larger research project (Experiences and reflections of teachers on the use of mixed reality technologies to foster cross-curricular learning opportunities) funded by a grant from the New Zealand Teaching and Learning Research Initiative (TLRI) # ID9193.
Conflict of interest
The author declares that the research was conducted in the absence of any commercial or financial relationships that could be construed as a potential conflict of interest.
Publisher’s note
All claims expressed in this article are solely those of the authors and do not necessarily represent those of their affiliated organizations, or those of the publisher, the editors and the reviewers. Any product that may be evaluated in this article, or claim that may be made by its manufacturer, is not guaranteed or endorsed by the publisher.
Acknowledgments
The author would like to acknowledge the work of the teachers involved in this study. Without their dedication and efforts, this study would have not been possible. I would like to also acknowledge the Teaching and Learning Research Initiative (TLRI) for funding and supporting this research.
References
Admiraal, W., Huizenga, J., Akkerman, S., and Dam, G. t. (2011). The concept of flow in collaborative game-based learning. Comput. Hum. Behav. 27 (3), 1185–1194. doi:10.1016/j.chb.2010.12.013
Ananiadou, K., and Claro, M. (2009). 21st century skills and competences for new millennium learners in OECD countries Paris: OECD Education Working Papers, OECD Publishing. doi:10.1787/218525261154
Anastasiadis, T., Lampropoulos, G., and Siakas, K. (2018). Digital game-based learning and serious games in education. Int. J. Adv. Sci. Res. Eng. 4 (12), 139–144. doi:10.31695/IJASRE.2018.33016
Bacca, J., Baldiris, S., Fabregat, R., Graf, S., and Kinshuk, (2014). Augmented reality trends in education: A systematic review of research and applications. J. Educ. Technol. Soc. 17 (4), 133–149.
Baños, R. M., Botella, C., Alcañiz, M., Liaño, V., Guerrero, B., Rey, B., et al. (2004). Immersion and emotion: Their impact on the sense of presence. CyberPsychology Behav. 7 (6), 734–741. doi:10.1089/cpb.2004.7.734
Benlahcene, A., Kaur, A., and Awang-Hashim, R. (2021). Basic psychological needs satisfaction and student engagement: The importance of novelty satisfaction. J. Appl. Res. High. Educ. 13 (5), 1290–1304. doi:10.1108/JARHE-06-2020-0157
Brown, E., and Cairns, P. (2004). “A grounded investigation of game immersion,” in CHI '04 extended abstracts on human factors in computing systems (Vienna, Austria: Association for Computing Machinery).
Buchholtz, N., Orey, D. C., and Rosa, M. (2020). “Mobile learning of mathematical modelling with math trails in actionbound,” in Proceedings of the 2020 World Conference on Mobile and Contextual Learning.
Çöltekin, A., Lochhead, I., Madden, M., Christophe, S., Devaux, A., Pettit, C., et al. (2020). Extended reality in spatial sciences: A review of research challenges and future directions. ISPRS Int. J. Geo-Information 9 (7), 439. doi:10.3390/ijgi9070439
Cook, J. (2010). Mobile phones as mediating tools within augmented contexts for development. Int. J. Mob. Blended Learn. (IJMBL) 2 (3), 1–12. doi:10.4018/jmbl.2010070101
Csikszentmihalyi, M. (1997). Creativity: Flow and the psychology of discovery and invention New York: Harper Collins.
Dalgarno, B., and Lee, M. J. W. (2010). What are the learning affordances of 3-D virtual environments? Br. J. Educ. Technol. 41 (1), 10–32. doi:10.1111/j.1467-8535.2009.01038.x
Di Natale, A. F., Repetto, C., Riva, G., and Villani, D. (2020). Immersive virtual reality in K-12 and higher education: A 10-year systematic review of empirical research. Br. J. Educ. Technol. 51 (6), 2006–2033. doi:10.1111/bjet.13030
Ekaputra, G., Lim, C., and Eng, K. I. (2013). “Minecraft: A game as an education and scientific learning tool,” in Proceedings of the Information Systems International Conference (ISICO), Bali, Indonesia.
Fluck, A., Webb, M., Cox, M., Angeli, C., Malyn-Smith, J., Voogt, J., et al. (2016). Arguing for computer science in the school curriculum. Educ. Technol. Soc. 19 (3), 38–46.
Fredricks, J. A., Filsecker, M., and Lawson, M. A. (2016). Student engagement, context, and adjustment: Addressing definitional, measurement, and methodological issues. Learn. Instr. 43, 1–4. doi:10.1016/j.learninstruc.2016.02.002
Furió, D., González-Gancedo, S., Juan, M. C., Seguí, I., and Costa, M. (2013). The effects of the size and weight of a mobile device on an educational game. Comput. Educ. 64, 24–41. doi:10.1016/j.compedu.2012.12.015
Gillis, A., and Jackson, W. (2002). Research for nurses: Methods and interpretation. Philadelphia, PA: FA Davis.
Greeno, J. G. (1994). Gibson’s affordances. Psychol. Rev. 101 (2), 336–342. doi:10.1037/0033-295X.101.2.336
Hamari, J., Shernoff, D. J., Rowe, E., Coller, B., Asbell-Clarke, J., Edwards, T., et al. (2016). Challenging games help students learn: An empirical study on engagement, flow and immersion in game-based learning. Comput. Hum. Behav. 54, 170–179. doi:10.1016/j.chb.2015.07.045
Herrington, J., and Reeves, T. C. (2011). “Using design principles to improve pedagogical practice and promote student engagement,” in Changing demands, changing directions. Proceedings ascilite hobart 2011. Editors G. Williams, P. Statham, N. Brown, and B. Cleland, 594–601.
Jacobson, J. (2017). “Authenticity in immersive design for education,” in Virtual, augmented, and mixed realities in education. Smart computing and intelligence. Editors D. Liu, C. Dede, R. Huang, and J. Richards (Singapore: Springer). doi:10.1007/978-981-10-5490-7_3
Johnson-Glenberg, M. C. (2018). Immersive VR and education: Embodied design principles that include gesture and hand controls. Front. Robot. AI 5, 81. doi:10.3389/frobt.2018.00081
Johnson-Glenberg, M. C., Megowan-Romanowicz, C., Birchfield, D. A., and Savio-Ramos, C. (2016). Effects of embodied learning and digital platform on the retention of physics content: Centripetal force. Front. Psychol. 7, 1819. doi:10.3389/fpsyg.2016.01819
Kafai, Y. B., and Burke, Q. (2015). Constructionist gaming: Understanding the benefits of making games for learning. Educ. Psychol. 50 (4), 313–334. doi:10.1080/00461520.2015.1124022
Kamarainen, A., Reilly, J., Metcalf, S., Grotzer, T., and Dede, C. (2018). Using mobile location-based augmented reality to support outdoor learning in undergraduate ecology and environmental science courses. Bull. Ecol. Soc. Am. 99 (2), 259–276. doi:10.1002/bes2.1396
Kaufmann, H. (2003). “Collaborative augmented reality in education,” in Position paper for keynote speech at Imagina Conference.
Kemmis, S. (2011). “A self-reflective practitioner and a new definition of critical participatory action research,” in Rethinking educational practice through reflexive inquiry: Essays in honour of susan groundwater-smith. Editors N. Mockler, and J. Sachs (Dordrecht: Springer Netherlands), 11–29.
Koehler, M. J., Shin, T. S., and Mishra, P. (2011). “How do we measure TPACK? Let me count the ways,” in Educational technology, teacher knowledge, and classroom impact: A research handbook on frameworks and approaches. Editors R. N. Ronau, C. R. Rakes, and M. L. Niess (Hershey, PA: Information Science Reference), 16–31.
MacDonald, C. (2012). Understanding participatory action research: A qualitative research methodology option. Can. J. Action Res. 13 (2), 34–50. doi:10.33524/cjar.v13i2.37
Margrett, J. A., Ouverson, K. M., Gilbert, S. B., Phillips, L. A., and Charness, N. (2022). Older adults’ use of extended reality: A systematic review. Front. Virtual Real. 2. doi:10.3389/frvir.2021.760064
Markus, M. L., and Silver, M. (2008). A foundation for the study of it effects: A new look at DeSanctis and poole’s concepts of structural features and spirit. J. Assoc. Inf. Syst. 9 (10), 609–632. doi:10.17705/1jais.00176
Ministry of Education (2017). Digital technologies | Hangarau matihiko. Available at https://education.govt.nz/assets/Documents/Ministry/consultations/DT-consultation/DTCP1701-Digital-Technologies-Hangarau-Matihiko-ENG.pdf.
Mitas, O., and Bastiaansen, M. (2018). Novelty: A mechanism of tourists’ enjoyment. Ann. Tour. Res. 72, 98–108. doi:10.1016/j.annals.2018.07.002
Morrison, A., Mulloni, A., Lemmelä, S., Oulasvirta, A., Jacucci, G., Peltonen, P., et al. (2011). Collaborative use of mobile augmented reality with paper maps. Comput. Graph. 35 (4), 789–799. doi:10.1016/j.cag.2011.04.009
O’Neil, H., Chuang, S. H., and Chung, G. (2004). Issues in the computer-based assessment of collaborative problem solving. Los Angeles, CA: University of California.
Parsons, D., and MacCallum, K. (2021). Current perspectives on augmented reality in medical education: Applications, affordances and limitations. Adv. Med. Educ. Pract. 12, 77–91. doi:10.2147/amep.s249891
Petrucco, C., and Agostini, D. (2016). Teaching cultural heritage using mobile augmented reality. J. e-Learning Knowl. Soc. 12 (3).
Plomp, T. (2007). “Educational design research :an introduction an introduction to educational design research,” in Proceedings of the Seminar at the East China Normal University, Shanghai (PR China), November 23-26, 9–35.
Radianti, J., Majchrzak, T. A., Fromm, J., and Wohlgenannt, I. (2020). A systematic review of immersive virtual reality applications for higher education: Design elements, lessons learned, and research agenda. Comput. Educ. 147, 103778. doi:10.1016/j.compedu.2019.103778
Rivet, A. E., and Krajcik, J. S. (2008). Contextualizing instruction: Leveraging students' prior knowledge and experiences to foster understanding of middle school science. J. Res. Sci. Teach. 45 (1), 79–100. doi:10.1002/tea.20203
Rosenbaum, E., Klopfer, E., and Perry, J. (2007). On location learning: Authentic applied science with networked augmented realities. J. Sci. Educ. Technol. 16 (1), 31–45. doi:10.1007/s10956-006-9036-0
Sánchez, J., and Olivares, R. (2011). Problem solving and collaboration using mobile serious games. Comput. Educ. 57 (3), 1943–1952. doi:10.1016/j.compedu.2011.04.012
Schmidt, J. A. (2010). “Flow in education,” in International encyclopedia of education. Editors P. Peterson, E. Baker, and B. McGaw. Third Edition (Oxford: Elsevier), 605–611.
Slater, M. (1999). Measuring presence: A response to the witmer and singer presence questionnaire. Presence. (Camb). 8, 560–565. doi:10.1162/105474699566477
Thomas, S., Schott, G., and Kambouri, M. (2004). “Designing for learning or designing for fun? Setting usability guidelines for mobile educational games,” in Learning with mobile devices: A book of papers, 173–181.
Tsay, C. H.-H., Kofinas, A. K., Trivedi, S. K., and Yang, Y. (2020). Overcoming the novelty effect in online gamified learning systems: An empirical evaluation of student engagement and performance. J. Comput. Assist. Learn. 36 (2), 128–146. doi:10.1111/jcal.12385
Wu, H.-K., Lee, S. W.-Y., Chang, H.-Y., and Liang, J.-C. (2013). Current status, opportunities and challenges of augmented reality in education. Comput. Educ. 62, 41–49. doi:10.1016/j.compedu.2012.10.024
Keywords: cross-curriculum, affordance, technology-enhanced learning, digital technologies, extended reality, student-created artefacts
Citation: MacCallum K (2022) The integration of extended reality for student-developed games to support cross-curricular learning. Front. Virtual Real. 3:888689. doi: 10.3389/frvir.2022.888689
Received: 03 March 2022; Accepted: 28 June 2022;
Published: 15 July 2022.
Edited by:
Marcin Wardaszko, Kozminski University, PolandReviewed by:
Carlos Andújar, Universitat Politecnica de Catalunya, SpainIrene Gironacci, Agilent Technologies, Germany
Copyright © 2022 MacCallum. This is an open-access article distributed under the terms of the Creative Commons Attribution License (CC BY). The use, distribution or reproduction in other forums is permitted, provided the original author(s) and the copyright owner(s) are credited and that the original publication in this journal is cited, in accordance with accepted academic practice. No use, distribution or reproduction is permitted which does not comply with these terms.
*Correspondence: Kathryn MacCallum, Kathryn.maccallum@canterbury.ac.nz