- 1Department of Physical Education, National Kaohsiung Normal University, Kaohsiung, Taiwan
- 2School of Kinesiology, University of Minnesota, Minneapolis, MI, United States
- 3Institute of Physical Education, Health and Leisure Studies, National Cheng Kung University, Tainan, Taiwan
- 4CIPER, Faculty of Human Kinetics, University of Lisbon, Lisbon, Portugal
Previous research has shown that motion sickness associated with virtual vehicles is more common among passengers than among drivers. Separately, other studies have shown that postural precursors of motion sickness during virtual driving differ as a function of prior experience driving physical vehicles. We investigated the intersection of those prior effects: We asked whether decades of physical driving experience 1) would influence motion sickness among passengers in a virtual vehicle, and 2) would influence postural precursors of motion sickness among passengers in a virtual vehicle. In our study, middle-aged adults were exposed to a virtual vehicle as passengers. Some participants (Physical Drivers) had decades of experience driving physical automobiles, while others (Physical Non-Drivers) had rarely or never driven a physical vehicle. First, we measured head and torso movement as standing participants performed simple visual tasks. Then, each participant watched a recording of the motion of a virtual vehicle, which induced motion sickness in some participants. Afterward, neither the incidence nor the severity of motion sickness differed between Physical Drivers and Physical Non-Drivers. Our analysis of pre-exposure standing body sway revealed postural precursors of motion sickness in measures of the spatial magnitude and temporal dynamics of movement. In statistically significant interactions, these precursors (Well vs. Sick) differed as a function of physical driving experience (Physical Drivers vs. Physical Non-Drivers). Overall, our results indicate that, among virtual passengers, long-term real-world driving experience influenced the postural precursors of motion sickness, but not the incidence or severity of motion sickness. We discuss these results in terms of relationships between perception and motor control in theories of motion sickness etiology.
1 Introduction
The driver-passenger effect (Curry et al., 2020c; Chang et al., 2021) describes the finding that drivers, that is, people who control physical (Rolnick and Lubow, 1991) or virtual vehicles (Dong et al., 2011), are less likely to experience motion sickness than passengers, or, people who are exposed to vehicle motion without the ability to control it. Typically, studies of the driver-passenger effect are conducted using yoked-control designs, in which passengers and drivers experience identical stimulus motions. The use of yoked-control designs reveals that the driver-passenger effect cannot be caused by the environment (i.e., imposed motion stimuli), and must lie in the individuals, themselves (Rolnick and Lubow, 1991).
Typically, experimental studies of the driver-passenger effect have focused on the roles of driver and passenger as they existed during data collection in the laboratory. However, the existence of the driver-passenger effect raises questions relating to people’s experiences outside the laboratory; in particular, their prior experience in physical vehicles. In the general literature on motion sickness, it is widely recognized that prior experience can have a significant impact on one’s susceptibility to motion sickness. Consider the fact that motion sickness tends to decrease with prolonged stimulus exposure, that is, with (recent) prior experience with a given motion stimulus. The classical example is seasickness, which generally subsides after several days at sea, despite the ongoing motion of the ship (Stevens and Parsons, 2002). This effect does not occur due to a change in ship motion, but rather a change in the individual’s response to the continuing motion. The role of experience has special relevance for etiological theories. The sensory conflict theory (Reason, 1978; Oman, 1982) claims that our perceptual-motor interactions with our environment create stored inferential models of multisensory stimulation, and that these experience-based models provide the standard for evaluation of the multisensory consequences of current behavior. In the sensory conflict theory, discrepancies between current stimulation and those hypothetical experience-based models are interpreted as sensory conflict, enough of which should cause motion sickness. An advantage of sensory conflict theory is its ability to account for the existence of individual differences in motion sickness susceptibility: Everyone has a unique perceptual-motor history and, therefore, unique hypothetical internal models. Thus, in any given motion situation, the level of sensory conflict (and, therefore, the motion sickness risk) will differ between individuals. Within this model, more extensive experience should lead to more detailed and/or robust internal models. Accordingly, an implication of sensory conflict theory is that the effects of experience on motion sickness susceptibility should increase with increasing experience.
While travelling by boat is not necessarily a common experience, the vast majority of people have significant experience with terrestrial travel. All of us have been passengers in road vehicles, often from infancy. However, actually controlling the vehicle is less universal: not everyone has driven a car. In Taiwan, many adults do not have a driver’s license and have never driven a physical vehicle. For example, in May 2023, 29.13% of residents over 18 years of age did not have a driver’s license (Dept. of Household Registration, M. O. I Taiwan, 2023; Ministry of Transportation and Communications, Taiwan, 2023). Recent research has taken advantage of this demographic fact to evaluate the influence of an individual’s past experience driving automobiles upon their risk of motion sickness while controlling a virtual vehicle (Stoffregen et al., 2017a; Chang et al., 2017), or while passively viewing the motion of a virtual vehicle (Chang et al., 2021).
In a virtual vehicle, Chang, et al. (2017) and Stoffregen et al. (2017b) compared adults with physical driving experience (Physical Drivers) to adults without such experience (Physical Non-Drivers). In the experiment, all participants drove a virtual vehicle. Chang et al. (2017), investigated young adults (mean age = 24 years), while Stoffregen et al. (2017a), used middle-aged adults (mean age = 50 years). In each study, motion sickness incidence was determined with a forced-choice, Yes/No question, Are you motion sick? Individuals answering yes were assigned to the sick group; all others were assigned to the well group. Symptom severity was assessed separately. Neither study found a difference between Physical Drivers and Physical Non-drivers in terms of incidence or severity of motion sickness; the incidence of motion sickness when driving a virtual vehicle did not differ between participants with and without physical driving experience.
As noted above, in the studies of both Chang et al. (2017) and Stoffregen et al. (2017b) participants were exposed to a virtual vehicle as drivers; that is, they drove the car in a driving video game. Accordingly, those studies provided information about how experience driving a physical vehicle affected motion sickness during driving. Given that motion sickness routinely differs between drivers and passengers, it cannot be assumed that studies in which participants were exposed to a virtual vehicle as drivers would generalize to situations in which participants were exposed to a virtual vehicle as passengers. This distinction is especially salient given that, in the classical driver-passenger effect passengers are more likely than drivers to become sick. For this reason, Chang et al. (2021) investigated motion sickness that occurred when participants with versus without physical driving experience were exposed to a virtual vehicle as passengers. Their participants were young adults, such that the mean duration of physical driving experience was 4.6 years. Chang et al. (2021) again found that physical driving experience had no effect on either the incidence or severity of motion sickness. Taken together, the studies of Chang et al. (2017); Chang et al. (2021) and Stoffregen et al. (2017a) provide no evidence that physical driving experience (Physical Drivers vs. Physical Non-Drivers) influenced motion sickness either when controlling a virtual vehicle (i.e., as a virtual driver) or passively watching a virtual vehicle (i.e., as a virtual passenger). In the present study, one of our aims was to ask whether the incidence or severity of motion sickness would be affected by decades of physical driving experience (cf. Stoffregen et al., 2017b).
The postural instability theory of motion sickness (Riccio and Stoffregen, 1991) claims that there should exist postural precursors of motion sickness. Specifically, the theory claims that the quantitative kinematics of postural activity should differ between individuals who experience motion sickness and those who do not, and that those differences should exist (and be measurable) before any participants experience motion sickness. This general prediction has been verified in many contexts. For example, Stoffregen et al. (2013) measured standing body sway 24 h before the beginning of a sea voyage. During the voyage, the collected data on seasickness among individuals, and they used those data to classify individuals into three groups in terms of the severity of seasickness: None, Mild, or Moderate/Severe. Using those categories, they then analyzed the postural data that had been collected 24 h before the voyage. This analysis revealed that standing body sway differed between the three seasickness groups, and that those differences in sway existed 24 h before the onset of the seasickness stimulus. Other studies have documented similar effects in the context of virtual environments. For example, Munafo et al. (2017) measured standing body sway before participants were exposed to self-controlled ambulation in a head-mounted virtual environment. Following exposure to the virtual environment, participants were classified into Well and Sick groups, which revealed (across two experiments) that the overall incidence of motion sickness was 39%. In addition, the Well/Sick grouping was used to analyze the data on postural sway that had been collected pre-exposure. The results revealed that postural precursors of motion sickness existed before participants were exposed to the head-mounted virtual environment. Similarly, Arcioni et al. (2019) measured standing body sway before participants were exposed to a head-mounted display. Following HMD exposure, participants were classified into Well and Sick groups, revealing an overall motion sickness incidence of 42.5%. That grouping was used to analyze data on pre-exposure postural sway, revealing that postural precursors of motion sickness existed before participants donned the HMD. Finally, Curry et al. (2020b) measured standing body sway before participants were exposed to a virtual vehicle presented via a head-mounted display. In a yoked-control design, some participants drove the virtual vehicle (Drivers), while others passively watched a recording of vehicle motion (Passengers). After exposure to the virtual vehicle participants were classified into Well and Sick groups, revealing the overall incidence of motion sickness to be 43%. The Well/Sick groupings were then used to analyze pre-exposure body sway, revealing the existence of pre-exposure postural precursors of motion sickness. In addition, Curry et al. (2020b) found (in statistically significant interactions) that postural precursors of motion sickness differed between participants in the Driver and Passenger groups. In the present study, we extended previous research by asking whether pre-exposure postural precursors of motion sickness might differ among virtual passengers with versus without decades of physical driving experience.
The sensory conflict theory of motion sickness etiology claims that past interactions with the world lead to internal expectations about current sensory stimulation. Sensory expectations or internal models are claimed to affect susceptibility to motion sickness (Reason, 1978; Oman, 1982). In addition, it is claimed that these hypothetical internal models guide perceptual-motor interactions with the environment (Reason, 1978; Oman, 1982). That is, an individual’s experience influences their hypothetical expectations, which then influence both their motor control and their likelihood of suffering motion sickness. In this context, the finding that postural precursors of motion sickness differed between Physical Drivers and Physical Non-Drivers can be interpreted as having theoretical significance. Physical driving experience appeared to yield postural control skills that affected motion sickness susceptibility but, simultaneously, were independent of hypothetical internal models claimed to be relevant to the etiology of motion sickness (given the lack of differences between Drivers and Non-Drivers in Chang et al. (2017); Chang et al. (2021) and Stoffregen et al. (2017a). Considered together, these findings challenge the parsimony of the theory of intersensory conflict.
In daily life, postural sway is influenced by the performance of non-postural tasks. As one example, standing body sway during performance of a reading task differs from sway in the same individuals during performance of a visual inspection task in which participants merely look at a blank sheet of paper (e.g., Stoffregen, et al., 2000). This relationship between postural sway and visual tasks has been documented in many contexts, including the elderly (e.g., Prado et al., 2007), and adolescents (e.g., Izquierdo-Herrera et al., 2018). Several studies of motion sickness have incorporated this variation in visual tasks during assessments of postural active before participants were exposed to potentially nauseogenic stimuli. These studies have revealed that simple variations in visual tasks can influence pre-exposure postural precursors of motion sickness (e.g., Munafo et al., 2017; Curry et al., 2020b). To increase the comparability of our results with this existing research, in the present study we evaluated pre-exposure standing body sway while participants performed two different visual tasks.
In the present study, we compared participants with versus without decades of physical driving experience. We examined standing postural sway before seated participants were exposed to a recording of virtual driving that was presented on a desktop monitor. In our pre-exposure assessment, we measured standing body sway while participants performed two simple visual tasks. Our study was designed to address two questions. First, we asked whether decades of driving experience would influence the incidence or severity of motion sickness when participants were exposed to a virtual vehicle as passengers. Second, we predicted that pre-exposure postural activity would exhibit postural precursors of motion sickness, and that these precursors would differ as a function of decades of physical driving experience. That is, we expected pre-exposure postural sway to exhibit a statistically significant interaction between Sickness groups (Well vs. Sick) and Physical Driving Experience groups (Physical Drivers vs. Physical Non-Drivers). While we predicted the existence of such interactions, we did not predict their direction. For this reason, we do not include post-hoc comparisons on statistically significant interactions.
2 Methods
2.1 Participants
Twenty individuals (mean age = 54.1 ± 5.33 years; mean height = 165.25 ± 8.92 cm; mean weight = 64.49 ± 10.95 kg; 10 men and 10 women) were identified for the Physical Driver group. Each Driver held a current, valid Driver’s license. Members of the Physical Driver group had been licensed drivers for an average of 22.00 years (SD = 2.77 years). Each Physical Driver reported driving at least once per week over the preceding 2 months, and average driving time was, approximately 3.69 h (SD = 3.13 h) per week. Twenty additional individuals (mean age = 54.27 ± 5.70 years; mean height = 165.03 ± 10.82 cm; mean weight = 69.19 ± 13.42 kg; 10 men and 10 women) were identified as Physical Non-Drivers. Seventeen Physical Non-Drivers had never held a Driver’s license nor driven an automobile. Three male Physical Non-Drivers had previously obtained a Driver’s license, one at the age of 28 and two others at 30. However, all three of them affirmed that they had not driven any automobile for more than 15 years. All participants had normal or corrected-to-normal vision, and reported no history of disease or malfunction of the vestibular apparatus, recurrent dizziness, or falls. Informed consent was obtained from the participants. The experimental protocol was approved by the Research Ethics Committee for Human Behavioral Sciences of National Cheng Kung University, Taiwan and all methods were performed in accordance with the relevant guidelines and regulations.
2.2 Procedure
As part of the informed consent process, participants were warned that they might become motion sick, and were instructed to discontinue their participation immediately if they experienced symptoms of motion sickness, however mild. Participants also were instructed that they were free to discontinue their participation at any time, for any reason. The fact that participants were free to discontinue at any time, for any reason minimized the risk that any participant would falsely report motion sickness as a way to end participation (Stoffregen and Smart, 1998).
We assessed the incidence of motion sickness and the severity of symptoms separately. Symptom severity was assessed via the Simulator Sickness Questionnaire (SSQ); (Kennedy et al., 1993). The SSQ includes 16 items with a 4-point scale. To assess the incidence of motion sickness participants answered a forced-choice, yes/no question, Are you motion sick? The forced-choice question and the SSQ questions were translated into Chinese and presented on a single form. Those responding yes to the forced-choice, yes/no question were assigned to the Sick group; all others were assigned to the Well group. The incidence of motion sickness and the severity of symptoms were assessed twice; before standing body sway assessment, and then again after exposure to the virtual environment. After finishing the informed consent procedure, each participant responded to the initial SSQ (SSQ-1) and answered the forced-choice question. Participants were instructed (both verbally and on the consent form) that they should discontinue their participation in the experiment immediately if they experienced any motion sickness symptoms, however mild. After exposure to the virtual environment, participants completed the second SSQ (SSQ-2) and again responded to the forced-choice question.
Before being exposed to the virtual vehicle, we assessed standing body sway during two simple visual tasks. Each participant completed one trial of an inspection task and one of a search task (Stoffregen et al., 2000). For those trials, the participant stood with their toes on a line on the floor. Targets were affixed to a stand that was 40 cm in front of the toes. The stand was adjustable, and targets were placed at each participant’s eye height. Targets were sheets of white paper 13.5 cm in length and 17 cm in width affixed to rigid cardboard. For the inspection task, the target was blank, and participants were asked to keep their gaze within the target’s outline. For the search task, the target was a paragraph of Arabic numerals in a 12-point Calibri font. Numerals were arranged in groups of five, with 13 groups in each line, and 13 lines of numerals. The participant was tasked with counting the number of times a specific numeral (0, 2, 6, 8, or 9) appeared. After 70 s, each participant reported the number of numerals counted, along with their position on the target. The order of the two tasks was counterbalanced across participants. During both tasks, we monitored head and torso movement with a magnetic tracking system (Flock of Birds, Ascension Technologies, Inc., Burlington, VT). One receiver was attached to a bicycle helmet, while a second receiver was taped to the skin at the level of the 7th cervical vertebra. The transmitter was located 50 cm behind each participant’s head. Six degree-of-freedom position data were collected from each receiver at 60 Hz and stored for later analysis.
After completing the pre-exposure postural assessment, participants were seated 105 cm in front of an LED monitor, which measured 139.67 cm diagonally (122 cm × 68 cm), such that the visual angle of the screen was approximately 60° horizontal by 36° vertical. The visual motion stimulus and corresponding audio soundtrack, a 40-min recording of the virtual driving performance of an individual playing Forza Motosport 3, was created using an X-Box 360 gaming system. The viewpoint was set at the Driver’s seat, yielding a first-person perspective. All participants were exposed to the same recording. Participants were reminded that they were free to discontinue their participation at any time, for any reason, and that they should discontinue their participation immediately if they experienced symptoms of motion sickness, however mild. Participants were told to continuously watch the recording. At the end of the recording, or when the participant chose to discontinue (whichever came first), the participant immediately completed the SSQ (SSQ-2). Using a magnetic tracking system, we monitored head and torso movement during seated exposure to the virtual vehicle; however, those data will be reported elsewhere.
2.3 Data analysis
We used χ2 statistics to analyze the motion sickness incidence data. For the SSQ data, we used the Total Severity Score, computed in the manner recommended by Kennedy et al. (1993). We evaluated SSQ scores using the Mann-Whitney and the Wilcoxon signed ranks tests.
In analyzing data on pre-exposure postural sway, we evaluated spatial magnitude and the temporal dynamics of movement separately. For movement magnitude, we analyzed positional variability, operationalized as the standard deviation of position. For temporal dynamics, we analyzed α, the scaling exponent of detrended fluctuation analysis (DFA). DFA describes the relationship between the magnitude of fluctuations in postural motion and the time scale over which those fluctuations are measured (Chen et al., 2002). DFA has been used in many studies of the control of stance (e.g., Lin et al., 2008), and in research on motion sickness (e.g., Bonnet et al., 2006; Arcioni et al., 2019). We conducted inferential tests on α, the scaling exponent of DFA. The scaling exponent is an index of long-range autocorrelation in the data, that is, the extent to which the data are self-similar over different time scales. White noise, which is uncorrelated, yields α = .5. The presence of long-range autocorrelation is indicated by α > .5. Pink noise (also known as 1/f noise) is indicated when α = 1.0. Values of α > 1.0 indicate nonstationary activity that resembles a random walk, while α > 1.5 indicates Brownian noise. Quiet stance in healthy adults tends to be nonstationary, typically yielding 1.0 > α > 1.5 (e.g., Riley et al., 1999). We did not integrate the time series before conducting DFA. Each dependent variable was computed separately for the head and torso. Separate ANOVAs for head and torso movement were conducted in the AP and ML axes, using 2 (Sickness Groups: Well vs. Sick) × 2 (Driving Experience: Physical Drivers vs. Physical Non-Drivers) × 2 (Tasks: Inspection, Search) ANOVAs with the last factor as the repeated measure. In our ANOVAs, we estimated the effect size using the partial η2 statistic. According to Cohen. (1988), values of partial η2 > 0.14 indicate a large effect, and values of partial η2 > .06 indicate a medium effect.
3 Results
3.1 Motion sickness incidence
Prior to virtual vehicle exposure, each participant expressed that they were not experiencing motion sickness. Post-exposure, the overall incidence of motion sickness was 35% (14/40). Of the 14 individuals assigned to the sick group, there were nine Physical Drivers (45.00%; 4 men and 5 women) and five Physical Non-Drivers (25.00%; 1 man and 4 women). The percentages did not differ between Physical Drivers and Physical Non-Drivers, χ2 (1) = 1.76, p = .185.
The 35% overall motion sickness incidence was significantly lower than the 62.5% found by Chang et al. (2021) for young adults who had viewed the same video recording, χ2 (1) = 6.054, p = .014; that is, using the identical visual stimulus, middle-aged adults (in the present study) were less likely to become sick than young adults (Chang et al., 2021).
3.2 Discontinuation
Each participant in the Well group watched the entire 40-min recording. All nine Physical Drivers in the Sick group discontinued. Among these nine participants, the mean exposure time to recorded virtual vehicle was 12.69 ± 6.37 min. One sick Physical Non-Driver completed the 40-min viewing, while the other four sick Physical Non-Drivers discontinued. Across the five Sick Physical Non-Drivers, the mean time of exposure was 20.88 ± 11.92 min. The mean exposure times were consistent between Physical Drivers (27.75 ± 14.57 min) and Physical Non-Drivers (35.27 ± 10.13 min), t (33.89) = −1.897, p = .066, and sick Physical Drivers and sick Physical Non-Drivers, t (12) = −1.702, p = .115.
3.3 Symptom severity
Symptom severity data are summarized in Figure 1. Before exposure, SSQ-1 scores did not differ between well and sick Physical Drivers, Mann-Whitney U = 37.50, p = .370, or between well and sick Physical Non-Drivers, Mann-Whitney U = 29.00, p = .497. These results indicate that the groups had no difference in symptom severity prior to beginning their exposure.
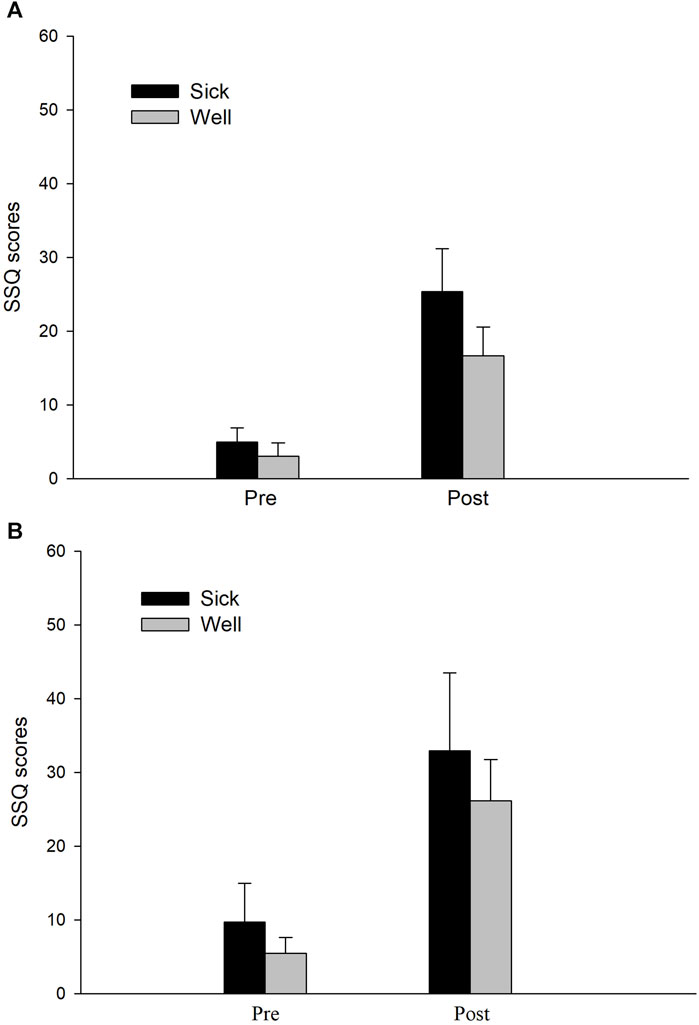
FIGURE 1. Symptom severity (SSQ Total Severity Scores) for the Well and Sick groups. Pre: Pre-exposure (SSQ-1). Post: Post-exposure (SSQ-2). (A). Drivers. (B). Non-Drivers. The error bars represent the standard error of the mean.
Among sick Physical Drivers, post-exposure scores (SSQ-2) exceeded pre-exposure scores, Z = −2.533, p = .011. Similarly, sick Physical Non-Drivers had higher post-exposure scores (SSQ-2) than pre-exposure scores, Z = −2.023, p = .043. However, SSQ scores also differed between post-exposure and pre-exposure for both well Physical Drivers, Z = −2.405, p = .016, and well Physical Non-Drivers, Z = −3.418, p = .001. No difference was found between post-exposure SSQ scores (SSQ-2) for the sick group and the well group for Physical Drivers, Mann-Whitney U = 34.00, p = .261, or for Physical Non-Drivers, Mann-Whitney U = 26.000, p = .349. These scores indicate that virtual vehicle exposure increased symptom severity among all groups of participants.
Post-exposure scores (SSQ-2) did not differ between sick Drivers and sick Non-Drivers, Mann-Whitney U = 17.000, p = .518, or between well Physical Drivers and well Physical Non-Drivers, Mann-Whitney U = 64.000, p = .357. Across sickness groups, there was no differences in post-exposure SSQ scores between Physical Drivers and Physical Non-Drivers, Mann-Whitney U = 167.500, p = .383. In summary, there was no evidence that physical driving experience (even over decades) influenced the severity of post-exposure symptoms.
3.4 Pre-exposure postural activity
Due to an equipment failure, pre-exposure postural data for one member of the Physical Driver group were lost. Accordingly, for the Physical Driver group the sample size in our analyses of pre-exposure postural activity was 19.
3.4.1 Movement magnitude
For positional variability, ANOVA revealed no significant effects for head or torso movement in the AP axes. In the ML axis for head movement, the main effect of Tasks was significant, F (1, 35) = 7.47, p = .010, partial η2 = .18, post-hoc power = 0.81. During the search task, positional variability of the head in the ML axis (1.14 ± 0.75 cm) was greater than during the inspection task (0.83 ± 0.53 cm). Also for ML axis head movement, the main effect of Sickness Groups was significant, F (1, 35) = 4.28, p = .046, partial η2 = .11, post-hoc power = 0.57. Positional variability in the Well group was greater (1.14 ± 0.74 cm) than for the Sick group 0.74 ± 0.43 cm). For ML axis torso movement, the main effect of Sickness Groups was significant, F (1, 35) = 5.60, p = .024, partial η2 = .14, post-hoc power = 0.69. Positional variability was higher among members of the Well group (0.78 ± 0.53 cm) than among the Sick group (0.49 ± 0.21 cm).
3.4.2 Temporal dynamics
For temporal dynamics, ANOVA revealed that, for head movement in the AP axis, the main effect of Tasks was significant, F (1, 35) = 14.39, p < .001, partial η2 = .29, post-hoc power = 0.97. The value of α during the inspection task (0.83 ± 0.06) was greater than during the search task (0.79 ± 0.07). In addition, the Physical Driving Experience × Tasks interaction was significant, F (1, 35) = 10.43, p = .003, partial η2 = .23, post-hoc power = 0.91 (Figure 2).
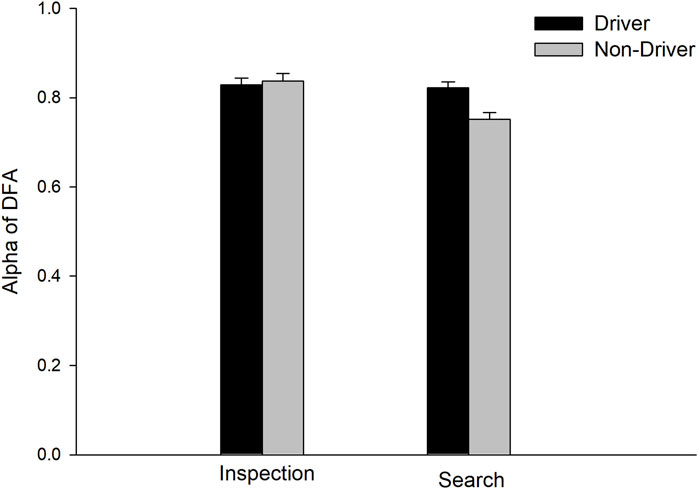
FIGURE 2. Temporal dynamics (α, the scaling exponent of DFA) for head movement in the AP axis, illustrating statistically significant interactions between Driving Experience and Tasks. The error bars represent the standard error of the mean.
For ML axis head movement, the main effect of Tasks was significant, F (1, 35) = 10.72, p = .002, partial η2 = .23, post-hoc power = 0.91. The value of α during the search task (0.79 ± 0.12) was greater than during the inspection task (0.72 ± 0.13). In addition, the Sickness Groups × Physical Driving Experience × Tasks interaction was significant, F (1, 35) = 4.79, p = .035, partial η2 = .12, post-hoc power = 0.61 (Figure 3).
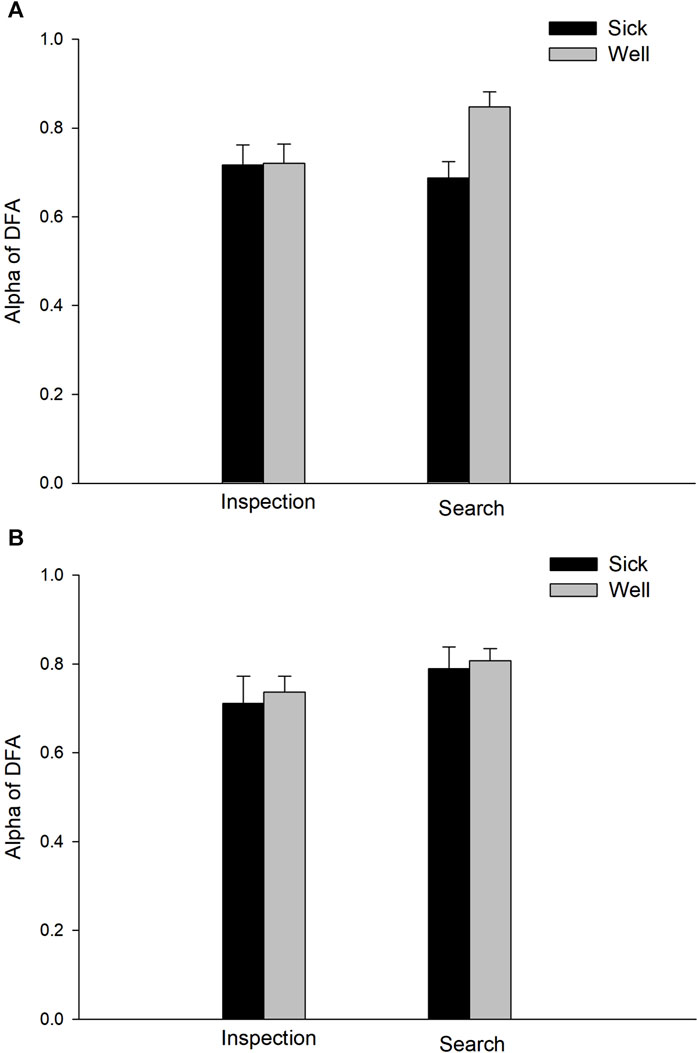
FIGURE 3. Temporal dynamics (α, the scaling exponent of DFA) for head movement in the ML axis, illustrating statistically significant Sickness Groups, Driving Experience and Tasks interaction effect. (A). Drivers. (B). Non-Drivers. The error bars represent the standard error of the mean.
For AP axis torso movement, the main effect of Tasks was significant, F (1, 35) = 10.82, p = .002, partial η2 = .24, post-hoc power = 0.93. The value of α during the inspection task (0.81 ± 0.06) was greater than during the search task (0.78 ± 0.07).
For torso movement in the ML axis, the main effect of Sickness Groups was significant, F (1, 35) = 4.60, p = .039, partial η2 = .12, post-hoc power = 0.61. The value of α was higher for the Well group (0.71 ± 0.14) than for the Sick group (0.62 ± 0.10). In addition, the Sickness Groups × Tasks interaction was significant, F (1, 35) = 6.02, p = .019, partial η2 = .15, post-hoc power = 0.72. However, both of these effects were subsumed in a significant 3-way interaction among Physical Driving Experience, Tasks, and Sickness Groups, F (1, 35) = 5.56, p = .031, partial η2 = .13, post-hoc power = 0.65 (Figure 4).
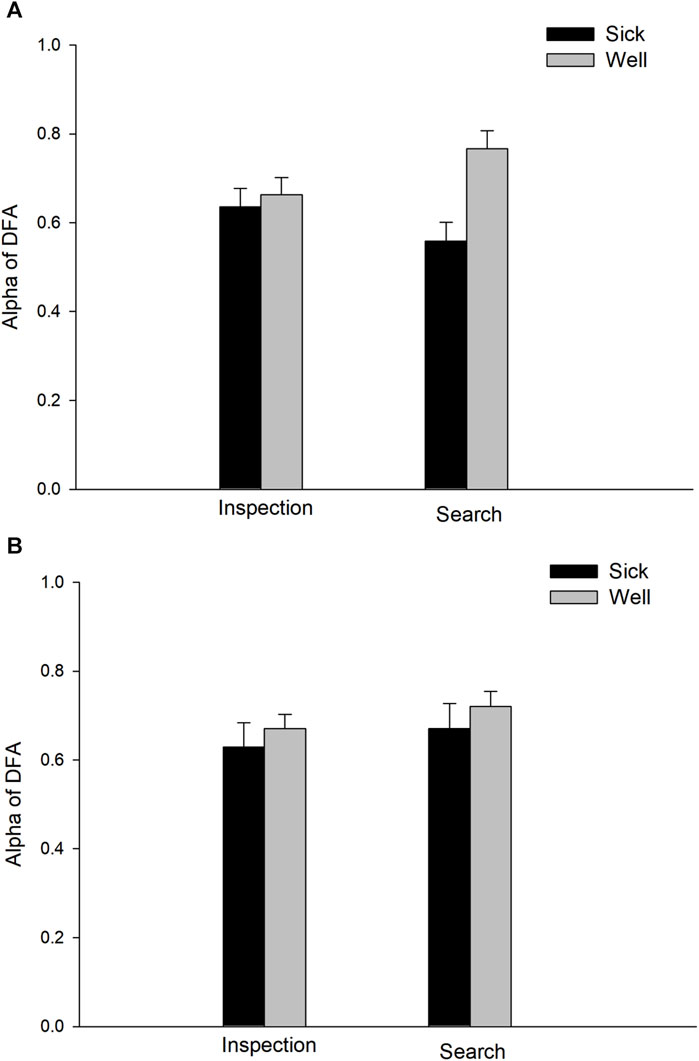
FIGURE 4. Temporal dynamics (α, the scaling exponent of DFA) for torso movement in the ML axis, illustrating statistically significant Sickness Groups, Driving Experience and Tasks interaction effect. (A). Drivers. (B). Non-Drivers. The error bars represent the standard error of the mean.
4 Discussion
In the current study, seated middle-aged adults experienced a virtual vehicle as passengers; that is, they viewed the motion of the vehicle without being able to control it. Drivers had decades of experience driving physical vehicles, while non-drivers had none. Before exposure to the virtual vehicle, participants performed simple visual tasks while standing. We evaluated pre-exposure standing body sway relative to the incidence of motion sickness associated with virtual vehicle exposure. We found no significant difference in motion sickness incidence between the Physical Driver and Physical Non-Driver groups. Among those who indicated motion sickness, symptom severity increased significantly with exposure, but this was also true for participants who stated that they were not motion sick. Prior to virtual vehicle exposure, standing postural activity differed between the visual tasks, between the Physical Driver and Physical Non-Driver groups, and between the Well and Sick groups. Finally, confirming our prediction, statistically significant interactions revealed that postural precursors of motion sickness differed between participants with and without decades of physical driving experience.
4.1 The role of physical driving experience in motion sickness
Following virtual vehicle exposure as passengers, we compared Drivers versus Non-Drivers in terms of the incidence of motion sickness, the likelihood and timing of voluntary discontinuation, and symptom severity. Across these measures, we found no evidence of any influence of decades of real-world driving experience. These non-effects echo the study of Stoffregen et al. (2017b), in which middle-aged Physical Drivers and Physical Non-Drivers drove a virtual vehicle. That is, the current study extends Stoffregen et al.‘s findings to middle-aged virtual passengers. Taken together, the null results of these two studies fail to provide evidence for the idea that motion sickness risk is related to internal models or expectations that are derived from past experience.
4.2 Motion sickness in relation to decades of physical driving experience
We found that motion sickness among our middle-aged participants (35%) occurred at lower rate than among the young adult participants (62.5%) in Chang et al. (2021). This result differs from studies in which participants have controlled the virtual vehicle. For example, Brooks et al. (2010), reported that, while driving an automobile simulator, older adults were more likely than younger adults to become sick. It may be that effects of age on motion sickness susceptibility differ between drivers and passengers. Our results parallel those of Turner and Griffin. (1999), who studied motion sickness among passengers in physical vehicles (bus travel), and found that motion sickness was less likely and less severe among older adults than among teens and young adults. Thus, our results provide motivation for new research on driving-related motion sickness in older virtual vehicle passengers.
A comparison of motion sickness incidence between this study and that of Chang et al. (2021) has implications for the role of experience-based expectations in the etiology of motion sickness. The current study used the same virtual vehicle recording used by Chang et al. (2021). The participants in the two studies differed only in age. In the present study, participants had decades of experience as occupants of physical vehicles. Half of our sample had been only passengers, while half had been both passengers and drivers. If experience traveling (as a passenger or driver) in vehicles generates expectations about multisensory stimulation which are relevant to the occurrence of motion sickness, then the hypothetical expectations should have been very strong for our middle-aged participants. Multisensory stimulation differs substantially between physical and virtual vehicles (e.g., the presence vs. absence of inertial displacement). For this reason, it would appear that the sensory conflict theory should predict an increase in the risk of motion sickness in virtual vehicles related to physical driving experience. Accordingly, the fact that we found just the opposite effect raises questions for the sensory conflict theory.
4.3 Symptom severity in relation to motion sickness incidence
For individuals who indicated motion sickness (i.e., the Sick group), symptom severity after exposure was significantly greater than before exposure, replicating a central finding of the motion sickness literature. However, the same was true for those not experiencing motion sickness (i.e., the Well group). This latter result replicates a common finding that virtual environment exposure corresponds with increased symptoms among participants who expressly deny feeling ill (Stanney et al., 1998; Munafo et al., 2017; Li et al., 2018; Walter et al., 2019; Curry et al., 2020c). Thus, a significant increase in symptoms at post-exposure (compared to pre-exposure) cannot be taken as sufficient evidence for the occurrence of motion sickness.
4.4 Postural precursors of motion sickness: Main effects
Our analysis of pre-exposure postural activity revealed the existence of postural precursors of motion sickness. For ML axis movement, positional variability of both the head and torso was higher among the Well group than the Sick group. The direction of this effect echoes Dennison and D’Zmura. (2017), who exposed standing participants to a head-mounted display and found the spatial magnitude of body sway to be significantly larger for “more comfortable” participants than for “less comfortable” participants. Both these effects are consistent with the postural instability theory of motion sickness. The theory predicts differences in postural activity between people who become sick and those who do not, but does not predict the direction of the difference (Riccio and Stoffregen, 1991). Contrary to traditional assumptions (Dennison & D’Zmura, 2017), “more movement” need not indicate “less stability” (Lin et al., 2008; Weech et al., 2018). In this regard it is helpful to note that we also found a postural precursor of motion sickness in the temporal dynamics of the torso in the ML axis. Because temporal dynamics of movement are orthogonal to their spatial magnitude, this effect confirms that postural precursors of motion sickness cannot be defined exclusively in terms of the spatial magnitude of movement. In fact, these precursors have been found in several different parameters of movement orthogonal to their spatial magnitude (Stanney et al., 2020).
4.5 Postural precursors of motion sickness: Interactions
When exposed as passengers, we predicted that postural precursors of motion sickness would differ based on participants’ driving experience. A statistically significant 2-way interaction between Sickness Groups and Physical Driving Experience Groups confirmed this prediction (Figure 2). It was further supported by the statistically significant 3-way interactions involving Sickness Groups, Physical Driving Experience, and Visual Tasks (Figures 3, 4). The existence of task-specific postural precursors of motion sickness is consistent with previous research (Koslucher et al., 2016; Munafo et al., 2017; Curry et al., 2020b). Similarly, higher-order interactions involving sickness groups, visual tasks, and other factors have been reported for unperturbed standing body sway (Curry et al., 2020a; Curry et al., 2020b). However, the effects illustrated in Figures 3, 4 are novel in one important respect: They reveal that these precursors can be modulated simultaneously by both long-term variations in perceptual-motor experience and by short-term variations in visual tasks (Inspection vs. Search).
In this study, we found that postural precursors of motion sickness were influenced by physical driving experience, despite the fact that, for these same participants, motion sickness incidence and severity were not related to physical driving experience. This independence of motor control from motion sickness is incompatible with claims that hypothetical models of expected multisensory stimulation (alleged to cause motion sickness) are used in the organization and control of movement (Reason, 1978; Oman, 1982). Thus, when considered with the results of Stoffregen et al. (2017a), our research indicates that these hypothetical internal models appear to have no other function than to cause motion sickness. In other words, the sensory conflict theory is not parsimonious (Stoffregen and Riccio, 1991). This logical problem has not been addressed by more recent presentations of the sensory conflict theory and its variants (Stanney et al., 2020). Our results re-affirm this problem, and may provide additional motivation for proponents of sensory conflict theory to address the issue of theoretical parsimony.
The present study adds to the growing body of literature in which postural precursors of motion sickness have been found in movement that was measured before participant exposure to a variety of motion stimuli (Stoffregen and Smart, 1998; Stoffregen et al., 2013; Munafo et al., 2017; Palmisano et al., 2018; Arcioni et al., 2019; Risi and Palmisano, 2019; Curry et al., 2020b) and, for the first time, extends this finding to the domain of middle-aged adults. The sensory conflict theory attributes motion sickness to “conflicts between expected sensory cues established through previous experiences and those currently being experienced in a novel environment” (Stanney et al., 2020, p. 1788). Presumably, then, sensory conflict should not exist (or should be minimal and irrelevant to motion sickness), prior to exposure to nauseogenic virtual environments. For this reason, the repeated demonstration that these precursors do exist before exposure does not disprove or refute the sensory conflict theory but does pose a challenge for it. The existence of this effect has not been addressed (or explained) by proponents of any version of sensory conflict theory (Dennison and D’Zmura, 2017; Keshavarz et al., 2014; Stanney et al., 2020) and, thus, remains a challenge.
4.6 Etiological theory and observability
In the present study, the motion stimulus was the same for each participant. Scientists do not have access to comprehensive data on the sensory-motor experience of any individual. Therefore, individual differences in motion sickness cannot be explained in terms of any objective measure of stimulus motion, or of the level of hypothetical sensory conflict. In research inspired by the sensory conflict theory, it is common to infer the presence and/or magnitude of hypothetical sensory conflict from manipulations of other factors, such as the temporal lag between a participant’s head movement and the display of the optical consequences of that movement in a head-mounted display system. As one example, Irmak et al. (2022), manipulated the acceleration of fore-aft physical oscillation of the body in a hydraulic motion device. Their dependent variables were self-reports of motion sickness. Irmak et al. (p. 4) did not directly measure hypothetical sensory conflict; rather, “sensory conflict was … assumed to be proportional to the acceleration stimulus”. Absent actual measurements this relation can be only hypothetical and is not empirical. The study of Irmak et al. is but one example of a common practice in research motivated by versions of the sensory conflict theory. In such research, the existence and magnitude of sensory conflict are inferred from objective data that bear only a hypothetical relationship to actual sensory conflict. We know of no published research in which sensory conflict (defined as the difference between actual and expected patterns of sensory stimulation) has been directly manipulated or quantitatively measured by scientists. It is sometimes argued that sensory conflict can be computed objectively by measuring discrepancies between the stimulation of different perceptual systems (e.g., for a review, see Stoffregen and Riccio, 1991). We agree that such measurements can be computed, and that such computations are objective. We acknowledge that such measurements sometimes have an empirical relation to observed motion sickness (e.g., Kim et al., 2022). However, the interpretation of any discrepancies in terms of sensory conflict is not mandatory. It is hypothetical, as underscored by the fact that other interpretations exist in the literature (e.g., Gibson, 1966; Stoffregen and Riccio, 1988; Stoffregen and Bardy, 2001; Stoffregen et al., 2017b). An interpretation in terms of sensory conflict requires the scientist to assume some level of discrepancy that corresponds to “zero conflict”. It might be claimed that “zero discrepancy” constitutes zero conflict, but such an interpretation is not consistent with the fact that motion sickness naturally fades with prolonged exposure. On a ship at sea (for example,), motion sickness fades after a few hours or days, even though ship motion continues. This effect cannot be explained based on objective measurements of observable ship motion.
By contrast, the postural instability theory makes predictions about observable data, that is, about patterns of physical movement that can be directly observed by scientists. To be sure, the kinematics of movement can be measured in a variety of ways (e.g., the center of pressure, or direct measures of the position, velocity, or acceleration of body segments), and those data can be analyzed in a wide variety of ways (e.g., the spatial dynamics, temporal dynamics, or multifractality of movement). Yet debates about the nature of postural instability are debates about objective, observable data on human movement. In part, this is because there is not a unique, 1:1 relation between patterns of postural activity and patterns of neural activity. Postural kinematics are influenced by neural activity, but also by factors outside the nervous system; this is the phenomena known as context-conditioned variability (e.g., Bongaardt and Meijer, 2000; Profeta and Turvey, 2018). For this reason, the quantitative kinematics of posture cannot be determined solely from observations of neural activity. In this fundamental sense, postural instability can be a stimulus for motion sickness that is both observable and logically distinct from neural activity. Thus, in the postural instability theory, it is not necessary to infer the existence of the hypothesized cause of motion sickness; rather, that cause can be observed. This qualitative difference between the sensory conflict theory and the postural instability theory in the observability of theoretically implicated causative factors has not been addressed by supporters of the sensory conflict theory.
5 Conclusion
Our sample comprised middle aged adults with versus without decades of physical driving experience. We measured the standing body sway of participants, after which seated participants were exposed to a recording of virtual vehicle motion. Following exposure, 35% of participants stated that they were motion sick. There was no difference in the incidence of motion sickness between participants with versus without decades of physical driving experience. By contrast, analysis of pre-exposure standing body sway revealed postural precursors of motion sickness; that is, measures of postural activity taken before participants were exposed to the virtual vehicle differed between participants in the Well and Sick groups. In addition, in statistically significant interactions, postural precursors of motion sickness differed between participants with versus without decades of physical driving experience. Taken together, the findings are consistent with the postural instability theory of motion sickness (Riccio and Stoffregen, 1991), but provide no support for sensory conflict theory (Stanney et al., 2020).
Data availability statement
The original contributions presented in the study are included in the article/Supplementary material, further inquiries can be directed to the corresponding author.
Ethics statement
The studies involving humans were approved by The Research Ethics Committee for Human Behavioral Sciences of National Cheng Kung University, Taiwan. The studies were conducted in accordance with the local legislation and institutional requirements. The participants provided their written informed consent to participate in this study.
Author contributions
C-HC: Conceptualization, Formal Analysis, Funding acquisition, Methodology, Writing–original draft. TS: Conceptualization, Funding acquisition, Methodology, Writing–review and editing. ML: Data curation, Formal Analysis, Writing–review and editing. KC: Data curation, Writing–review and editing. C-CL: Formal Analysis, Methodology, Project administration, Writing–review and editing.
Funding
The author(s) declare financial support was received for the research, authorship, and/or publication of this article. C-HC was supported by the Ministry of Science and Technology, Taiwan (MOST 107-2410-H-017-026-). TS was supported by National Science Foundation, U.S.A., NSF-1901423, C-HS: Medium: Prediction, Early Detection, and Mitigation of Virtual Reality Simulator Sickness.
Acknowledgments
Portions of the data were presented at the virtual conference of 2020 North American Society for the Psychology of Sport and Physical Activity, Vancouver, British Columbia, Canada. We thank Yu-Sian Su and Ting-Yi Wu, who assisted with data collection.
Conflict of interest
The authors declare that the research was conducted in the absence of any commercial or financial relationships that could be construed as a potential conflict of interest.
Publisher’s note
All claims expressed in this article are solely those of the authors and do not necessarily represent those of their affiliated organizations, or those of the publisher, the editors and the reviewers. Any product that may be evaluated in this article, or claim that may be made by its manufacturer, is not guaranteed or endorsed by the publisher.
References
Arcioni, B., Palmisano, S., Apthorp, D., and Kim, J. (2019). Postural stability predicts the likelihood of cybersickness in active HMD-based virtual reality. Displays 58, 3–11. doi:10.1016/j.displa.2018.07.001
Bongaardt, R., and Meijer, O. G. (2000). Bernstein's theory of movement behavior: historical development and contemporary relevance. J. Mot. Behav. 32, 57–71. doi:10.1080/00222890009601360
Bonnet, C. T., Faugloire, E. M., Riley, M. A., Bardy, B. G., and Stoffregen, T. A. (2006). Motion sickness preceded by unstable displacements of the center of pressure. Hum. Mov. Sci. 25, 800–820. doi:10.1016/j.humov.2006.03.001
Brooks, J. O., Goodenough, R. R., Crisler, M. C., Klein, N. D., Alley, R. L., Koon, B. L., et al. (2010). Simulator sickness during driving simulation studies. Accid. Anal. Prev. 42, 788–796. doi:10.1016/j.aap.2009.04.013
Chang, C. H., Chen, F. C., Kung, W.-C., and Stoffregen, T. A. (2017). Effects of physical driving experience on body movement and motion sickness during virtual driving. Aerosp. Med. Hum. Perf. 88, 985–992. doi:10.3357/AMHP.4893.2017
Chang, C.-H., Stoffregen, T. A., Cheng, K. B., Lei, M. K., and Li, C. C. (2021). Effects of physical driving experience on body movement and motion sickness among passengers in a virtual vehicle. Exp. Brain Res. 239, 491–500. doi:10.1007/s00221-020-05940-6
Chen, Z., Ivanov, P. C., Hu, K., and Stanley, H. E. (2002). Effect of nonstationarities on detrended fluctuation analysis. Phys. Rev. E 65, 041107–041114. doi:10.1103/PhysRevE.65.041107
Cohen, J. (1988). Statistical power analysis for the behavioral sciences. 2nd ed. New York: Erlbaum.
Curry, C., Li, R., Peterson, N., and Stoffregen, T. A. (2020c). Cybersickness in virtual reality head-mounted displays: examining the influence of sex differences and vehicle control. Int. J. Hum–Comput Int. 36, 1161–1167. doi:10.1080/10447318.2020.1726108
Curry, C., Peterson, N., Li, R., and Stoffregen, T. A. (2020a). Postural activity during use of a head-mounted display: sex differences in the “Driver–Passenger” effect. Front. Virtual Real. 1 (38). doi:10.3389/frvir.2020.581132
Curry, C., Peterson, N., Li, R., and Stoffregen, T. A. (2020b). Postural precursors of motion sickness in head-mounted displays: drivers and passengers, women and men. Ergonomics 63 (12), 1502–1511. doi:10.1080/00140139.2020.1808713
Dennison, M. S., and D’Zmura, M. (2017). Cybersickness without the wobble: experimental results speak against postural instability theory. Appl. Ergon. 58, 215–223. doi:10.1016/j.apergo.2016.06.014
Dept. of Household Registration, M. O. I., Taiwan (2023). Population by city and age (9701). Avaliable at: https://www.ris.gov.tw/info-popudata/app/awFastDownload/toMain_panel.
Dong, X., Yoshida, K., and Stoffregen, T. A. (2011). Control of a virtual vehicle influences postural activity and motion sickness. J. Exp. Psychol. Appl. 17, 128–138. doi:10.1037/a0024097
Irmak, T., Kotian, V., Happee, R., de Winkel, K. N., and Pool, D. M. (2022). Amplitude and temporal dynamics of motion sickness. Front. Syst. Neurosci. 16, 866503. doi:10.3389/fnsys.2022.866503
Izquierdo-Herrera, R., García-Massó, X., González, L.-M., Wade, M. G., and Stoffregen, T. A. (2018). Visual tasks and stance width influence the spatial magnitude and temporal dynamics of standing body sway in 6- to 12-year old children. Hum. Mov. Sci. 59, 56–65. doi:10.1016/j.humov.2018.03.017
Kennedy, R. S., Lane, N. E., Berbaum, K. S., and Lilienthal, M. G. (1993). Simulator sickness questionnaire: an enhanced method for quantifying simulator sickness. Int. J. Aviat. Psychol. 3, 203–220. doi:10.1207/s15327108ijap0303_3
Keshavarz, B., Hecht, H., and Lawson, B. D. (2014). “Visually-induced motion sickness: causes, characteristics, and countermeasures,” in Handbook of virtual environments: design, implementation, and applications. Editors K. S. Hale, and K. M. Stanney (Boca Raton, FL: CRC Press), 647–698.
Kim, J., Charbel-Salloum, A., Perry, S., and Palmisano, S. (2022). Effects of display lag on vection and presence in the Oculus Rift HMD. Virtual Real. 26, 425–436. doi:10.1007/s10055-021-00570-x
Koslucher, F. C., Haaland, E., and Stoffregen, T. A. (2016). Sex differences in visual performance and postural sway precede sex differences in visually induced motion sickness. Exp. Brain Res. 234 (1), 313–322. doi:10.1007/s00221-015-4462-y
Li, R., Peterson, N., Walter, H. J., Rath, R., Curry, C., and Stoffregen, T. A. (2018). Real-time visual feedback about postural activity increases postural instability and visually induced motion sickness. Gait Posture 65, 251–255. doi:10.1016/j.gaitpost.2018.08.005
Lin, D., Seol, H., Nussbaum, M. A., and Madigan, M. L. (2008). Reliability of COP-based postural sway measures and age-related differences. Gait Posture 28 (2), 337–342. doi:10.1016/j.gaitpost.2008.01.005
Ministry of Transportation and Communications, Taiwan (2023). Number of driver licenses by age and gender. Avaliable at: https://stat.motc.gov.tw/mocdb/stmain.jsp?sys=210&funid=b330203.
Munafo, J., Diedrick, M., and Stoffregen, T. A. (2017). The virtual reality head-mounted display Oculus Rift induces motion sickness and is sexist in its effects. Exp. Brain Res. 235, 889–901. doi:10.1007/s00221-016-4846-7
Oman, C. M. (1982). A heuristic mathematical model for the dynamics of sensory conflict and motion sickness. Acta Oto-Laryngol, Suppl. 392, 4–44. doi:10.3109/00016488209108197
Palmisano, S., Arcioni, B., and Stapley, P. J. (2018). Predicting vection and visually induced motion sickness based on spontaneous postural activity. Exp. Brain Res. 236 (1), 315–329. doi:10.1007/s00221-017-5130-1
Prado, J. M., Stoffregen, T. A., and Duarte, M. (2007). Postural sway during dual tasks in young and elderly adults. Gerontology 53, 274–281. doi:10.1159/000102938
Profeta, V. L. S., and Turvey, M. T. (2018). Bernstein’s levels of movement construction: a contemporary perspective. Hum. Mov. Sci. 57, 111–133. doi:10.1016/j.humov.2017.11.013
Reason, J. T. (1978). Motion sickness adaptation: a neural mismatch model. J. R. Soc. Med. 71, 819–829. doi:10.1177/014107687807101109
Riccio, G. E., and Stoffregen, T. A. (1991). An ecological theory of motion sickness and postural instability. Ecol. Psychol. 3, 195–240. doi:10.1207/s15326969eco0303_2
Riley, M., Balasubramaniam, R., and Turvey, M. (1999). Recurrence quantification analysis of postural fluctuations. Gait Posture 9, 65–78. doi:10.1016/S0966-6362(98)00044-7
Risi, D., and Palmisano, S. (2019). Effects of postural stability, active control, exposure duration and repeated exposures on HMD induced cybersickness. Displays 60, 9–17. doi:10.1016/j.displa.2019.08.003
Rolnick, A., and Lubow, R. E. (1991). Why is the driver rarely motion sick? The role of controllability in motion sickness. Ergonomics 34, 867–879. doi:10.1080/00140139108964831
Stanney, K., Lawson, B. D., Bokers, B., Dennison, M., Fidopiastis, C., Stoffregen, T. A., et al. (2020). Identifying causes of and solutions for cybersickness in immersive technology: reformulation of a research and development agenda. Int J Hum-Comput Int 36, 1783–1803. doi:10.1080/10447318.2020.1828535
Stanney, K., Salvendy, G., Deisinger, J., DiZio, P., Ellis, S., Ellison, J., et al. (1998). Aftereffects and sense of presence in virtual environments: formulation of a research and development agenda. Int. J. Hum–Comput Int. 10, 135–187. doi:10.1207/s15327590ijhc1002_3
Stevens, S. C., and Parsons, M. G. (2002). Effects of motion at sea on crew performance: a survey. Mar. Technol. 39 (1), 29–47. doi:10.5957/mt1.2002.39.1.29
Stoffregen, T. A., and Bardy, B. G. (2001). On specification and the senses. Behav. Brain Sci. 24, 195–213. doi:10.1017/S0140525X01003946
Stoffregen, T. A., Chang, C.-H., Chen, F.-C., and Zeng, W.-J. (2017a). Effects of decades of physical driving on body movement and motion sickness during virtual driving. PLOS ONE 12 (11), e0187120. doi:10.1371/journal.pone.0187120
Stoffregen, T. A., Chen, F.-C., Varlet, M., Alcantara, C., and Bardy, B. G. (2013). Getting your sea legs. Plos One 8 (6), e66949. doi:10.1371/journal.pone.0066949
Stoffregen, T. A., Mantel, B., and Bardy, B. G. (2017b). The senses considered as one perceptual system. Ecol. Psychol. 29, 165–197. doi:10.1080/10407413.2017.1331116
Stoffregen, T. A., Pagulayan, R. J., Bardy, B. G., and Hettinger, L. J. (2000). Modulating postural control to facilitate visual performance. Hum. Mov. Sci. 19, 203–220. doi:10.1016/S0167-9457(00)00009-9
Stoffregen, T. A., and Riccio, G. E. (1988). An ecological theory of orientation and the vestibular system. Psychol. Rev. 95, 3–14. doi:10.1037/0033-295X.95.1.3
Stoffregen, T. A., and Riccio, G. E. (1991). An ecological critique of the sensory conflict theory of motion sickness. Ecol. Psychol. 3, 159–194. doi:10.1207/s15326969eco0303_1
Stoffregen, T. A., and Smart, L. J. (1998). Postural instability precedes motion sickness. Brain Res. Bull. 47, 437–448. doi:10.1016/S0361-9230(98)00102-6
Turner, M., and Griffin, M. J. (1999). Motion sickness in public road transport: passenger behaviour and susceptibility. Ergonomics 42, 444–461. doi:10.1080/001401399185586
Walter, H. J., Li, R., Munafo, J., Curry, C., Peterson, N., and Stoffregen, T. A. (2019). Unstable coupling of body sway with imposed motion precedes visually induced motion sickness. Hum. Mov. Sci. 64, 389–397. doi:10.1016/j.humov.2019.03.006
Keywords: visual tasks, sway, vehicle control, postural instability, motion sickness
Citation: Chang C-H, Stoffregen TA, Lei MK, Cheng KB and Li C-C (2024) Effects of decades of physical driving experience on pre-exposure postural precursors of motion sickness among virtual passengers. Front. Virtual Real. 5:1258548. doi: 10.3389/frvir.2024.1258548
Received: 14 July 2023; Accepted: 19 January 2024;
Published: 02 February 2024.
Edited by:
Jaehyun Park, Incheon National University, Republic of KoreaCopyright © 2024 Chang, Stoffregen, Lei, Cheng and Li. This is an open-access article distributed under the terms of the Creative Commons Attribution License (CC BY). The use, distribution or reproduction in other forums is permitted, provided the original author(s) and the copyright owner(s) are credited and that the original publication in this journal is cited, in accordance with accepted academic practice. No use, distribution or reproduction is permitted which does not comply with these terms.
*Correspondence: Chih-Hui Chang, Y2hpaHVpQG1haWwubmtudS5lZHUudHc=