- 1Laboratório de Fisiologia de Plantas sob Estresse, Departamento de Botânica, Setor de Ciências Biológicas, Universidade Federal do Paraná, Curitiba, Paraná, Brazil
- 2Fundação Ezequiel Dias, Belo Horizonte, Minas Gerais, Brazil
- 3Laboratório de Micropropagação de Plantas, Departamento de Fitotecnia e Fitossanitaríssimo, Setor de Ciências Agrárias, Universidade Federal do Paraná, Curitiba, Paraná, Brazil
- 4Horto Botânico, Departamento de Agricultura, Escola de Ciências Agrárias, Universidade Federal de Lavras, Lavras, Minas Gerais, Brazil
- 5Ecotoxicology of Aquatic Microorganisms Laboratory, EcotoQ, GRIL, TOXEN, Department of Biological Sciences, Université du Québec à Montréal, Montréal, Montréal, QC, Canada
Enrofloxacin (Enro) is often detected in soil and animal manure used for crop production and phytotoxic responses have been observed in plants grown under antimicrobial presence. In the present paper, we investigated the effects of the presence of Enro in soils (1.9 mg kg-1) and manure (50.4 mg kg-1) in growth and flower production of calla lily plants (Zantedeschia aethiopica). We also reported the accumulation and distribution of Enro between plant tissues aiming to evaluate the safety of commercializing plants produced under Enro-contaminated conditions. The presence of Enro in soils and manure did not affect plant growth and flower production and did not induce any physiological effects in plants (as evaluated by photosynthetic pigment, hydrogen peroxide concentration, superoxide dismutase and catalase activity in leaves). Plants accumulated Enro mainly in their roots, restraining its translocation to shoots, which contributes to the safety of the commercialization of their flowers. However, when commercialized as pots, the amount of Enro carried by plants is a matter of concern, and therefore, selling pot plants must be avoided. Due to their tolerance and capacity to remove Enro (up to 14.76% of Enro from contaminated soil and/or manure), plants are indicated for phytoremediation programs.
Introduction
Ornamental plants are an important sector in agribusiness, moving billions of dollars a year around the world (1–3). In addition to generating income, ornamental plants play important environmental roles: they offer shelter for wild fauna, act as biological filters and help in environmental matrix decontamination, in addition to being used in the landscaping of parks and gardens (4–8).
Among ornamental plants, calla lily (Zantedeschia aethiopica (L.) Spreng.) is of growing commercial interest, showing high commercialization value (9–12). This monocotyledon belonging to the family Araceae is marketed as pot plants, and mainly as cut flowers for flower arrangements and bouquets. Although the production of calla lily has increased worldwide due to its good environmental adaptation (13), these plants require high levels of fertilization, which is often achieved by organic fertilization using manure (14).
Although manuring has several beneficial effects in agricultural practice, its harmful and neutral effects on the trinity formed by plants, soil and organisms are expected (15). One of the major problems caused by manuring is placing organic contaminants into soil and plants. Some contaminants, such as antimicrobials, largely used in animal production, are not fully metabolized as they are administered, and are excreted in animal feces and urine (16), resulting in the high observed concentrations of antimicrobials in manure (17, 18).
Once applied to soil, manure can release antimicrobials into soil solution, which become available for plant uptake (19). Some antimicrobials are phytotoxic causing delayed germination (20) reductions on photosynthesis (21, 22) and mitochondrial respiration (23), in addition to causing oxidative stress damage (24, 25).
Fluoroquinolones (FQ) are one of the main classes of antimicrobials occurring in livestock (26–30), and once present in agricultural systems, they can be leached to water bodies or adsorbed in the colloidal fraction of the soil, remaining for long periods until they are degraded by biodegradation and photooxidation processes (31). These processes depend on the antimicrobial physical-chemical properties and concentration (molecular structure, size, form, solubility, speciation, hydrophobicity, etc.), soil characteristics (pH, texture, organic matter, mineralogy, microbial activity, etc.), climatic conditions (luminosity and temperature), manure source (32) and the exposure time of the area (26).
In soils, Enrofloxacin (Enro), an FQ commonly used in veterinary medicine, has been detected at levels of 1.03 mg kg-1 (30) while in animal manure, its concentrations reach up to 46 mg kg-1 (29). This antimicrobial is of interest as its toxicity has been seen in crops, such as soybeans, decreasing plant yields (19). In this context, the use of Enro contaminated manure in calla lily fertilization could result in decreased yields if this antimicrobial threatens plant growth.
However, some ornamental plants have demonstrated great tolerance to organic contaminants such as antimicrobials (33, 34). In a previous study, the presence of Enro in water used for irrigation did not affect the growth and flower production of Zantedeschia rehmannii and Spathiphyllum wallisii (unpublished data). It is important to note that the antimicrobial tolerance of ornamental plants is often followed by the plant capacity to accumulate the antimicrobial in their tissues. In this scenario, although yields may not be reduced by the presence of antimicrobials, ornamental plants could constitute a vehicle of antimicrobial contamination, transporting these molecules in their biomasses upon their commercialization (35).
On the other hand, this capacity for antibiotic accumulation can be used for decontamination purposes such as the use of ornamental plants to mitigate water and/or soil contamination (phytoremediation). Indeed, ornamental plants have emerged as potential phytoremediator species due to their high stress tolerance and are not intended for animal or human food consumption – which avoids the introduction of contaminants into the food web (35). Moreover, in addition to improving the environments with aesthetic value, ornamental plants provide multiple ecosystem services and promote human well-being (35).
Therefore, for both, the production and safety of commercialization of ornamental plants grown under contaminated conditions as well as for phytoremediation purposes, it is important to investigate the accumulation and distribution of contaminants between the different plant organs (mainly in those that are marketable). We therefore investigated the effects of the presence of Enro in soils and manure used for the growth of calla lily on plant growth and flower production. Moreover, we evaluated the accumulation and distribution of Enro among the different plant organs aiming to predict the safety of commercializing plants and to evaluate the phytoremediation capacity of plants.
Material and methods
Soil preparation and seedling production
Propagative material of Z. aethiopica (L.) Spreng. was acquired from Horto Botânico, Departamento de Agricultura, Federal University of Lavras (Lavras, Brazil). Seedlings were produced by burying the tubers in pots (5 L capacity) filled with substrate composed of soil + sand (2:1). The pots were irrigated daily for 45 days with distilled water. Once emerged, seedlings of 10 ± 2 cm size, with 2 leaves were transplanted to pots containing their respective treatments as described below.
The soil used in the experiment was collected in Curitiba, Paraná, Brazil, in the 0 - 20 cm soil layer, in a non-agricultural area. Prior to its use, the soil was tested for the presence of Enro (as described below) and no Enro was observed. Then, soil was corrected with dolomitic limestone until pH 5.5 ± 0.5. The physical-chemical characteristics of soil was as follows: pH (CaCL2): 4.23; H+Al, Ca, Mg, K (cmolc dm-3): 12.1, 3.3, 1.6, 0.05, respectively; P (mg dm-3): 5.13; C (g dm-3): 23.88; sand, silt, clay (%): 31.3, 7.4, 61.3. The bovine manure used was collected in an area of organic production, free of antimicrobial use (Centro Paranaense de Referência em Agroecologia - CPRA) in Curitiba (Paraná, Brazil). As for the soil, the manure was checked for Enro presence, and no antimicrobial was found.
Soil and manure were contaminated with Enro by using analytical grade Enro (Vetranal, Sigama-Aldrich, Canada). A stock solution (1 mg mL-1) was firstly prepared in ultra-pure water and the correspondent aliquots used for the contamination of the matrices. Soil and manure were contaminated separately with 0 or 1.9 mg Enro kg-1 and 0 or 50 mg Enro kg-1, respectively. These concentrations were selected based on the environmental representative concentrations of Enro in soils (30) and manure (29).
The ultrapure water was added in the same volume as the Enro-solution to the treatments without contamination. After 24h, the manure was incorporated into the soil in a ratio of 3:1. After mixing, samples of the substrate were taken to evaluate the Enro concentrations and then, the substrates were packed in plastic pots with a capacity of 12 liters. One seedling was then transplanted in each pot and considered as an experimental unit. A total of 25 experimental units were prepared in a completely randomized design, with 5 replicates per treatment. The plants were irrigated twice a week with 300 mL of distilled water. In parallel, three pots without plants were prepared and exposed to the same conditions to determine the antimicrobial degradation (photolysis, hydrolysis, and biodegradation). Treatments were as follows: soil + manure (S + M), contaminated soil + manure (CS + M), soil + contaminated manure (S + CM), contaminated soil + contaminated manure (CS + CM).
Plant production and physiological evaluations
Evaluations were performed in plants after the emission of the first flower (about 60 days after the transplantation). A hand-help SPAD (CCM-200 plus; Opti-Sciences; USA) was used to estimate chlorophyll content. SPAD readings were taken on second fully expanded leaves (from apex to the base) in a total of three readings per leaf. Leavers used for SPAD readings were healthy, turgid, flat, and homogeneous in color and size. The leaves from each plant were counted and after that, plants were harvested, washed thoroughly with distilled water, gently dried with absorbent paper and segmented into flowers, leaves, roots, and rhizomes. Then, the fresh biomass of organs was measured, which was used to assess ornamental production of plants. Samples were then flash-frozen in liquid nitrogen and stored at -20 °C until being further evaluated.
Antioxidant enzymes and hydrogen peroxide (H2O2) concentrations (36) in leaves were determined using 0.1 g of plant tissues. Evaluations were performed in the same leaves used for SPAD readings. The enzymes were extracted in 1 mL of phosphate buffer containing 100 mM EDTA, 1 mL of L-ascorbic acid, and a 2% polyvinylpyrrolidone solution (PVP m/v) (37). The activities of superoxide dismutase (SOD) (38) and catalase (CAT) (39) were assessed after determining the total protein concentrations (40).
Chemical analyses
Enrofloxacin concentrations were investigated in dried samples (45 °C) using high performance liquid chromatography (HPLC). The antimicrobial was evaluated in substrates (soil and/or manure) at the beginning and at harvesting. For that purpose, at harvesting, substrate samples were collected at 10 cm depth, corresponding to the rhizosphere in pots with plants. Extraction was performed in 1 g substrate by adding 1 mL of 100 mM sodium phosphate buffer (pH 3) and 1 mL chloroform. After homogenization for 10 min in a rotating shaker (Firstlab, Brazil) at 25 rpm, samples were filtered in a syringe with cotton mesh and the organic phase (corresponding to chloroform) was then collected and dried in a SpeedVac machine (RC1010, Thermo).
For plant samples, dried organs were firstly homogenized, and the extraction of Enro was performed in three sub-replicates (1 g each) from each homogenate (with a total of three replicates/organs/pot), using 200 mg of plant material and 1.5 mL of acetonitrile containing 1% acetic acid, following Migliori et al. (41). After extraction, the samples were dried in a SpeedVac machine. For both, substrate and plant samples, the obtained residues after extraction were suspended in acidified water (pH 4.5). Samples were then filtered through C18 solid phase extraction cartridges previously conditioned with 15 mL of methanol and 5 mL of water (pH 3.0). The cartridges containing the samples were then washed with 1.6 mL of methanol:water (60:40, v/v), the eluate dried in a SpeedVac machine (RC1010, Thermo), and the residues resuspended in 0.4% aqueous triethylamine pH 3.0, acetonitrile and methanol (75:10:15 v/v/v) (42).
The concentrations of Enro were determined following Shi et al. (42) (LOD 0.19 ppb, LOQ 0.62 ppb) using high performance liquid chromatography (HLPC) and a fluorescence detector (Agilent 1290 Infinity II LC, Wilmington, DE, USA) after elution on a C18 column (Discovery® HS C18 column 250 x 4.6 mm, particle size 5 µm, Sigma-Aldrich). The FLD excitation/emission detection was 278/445 nm. Each batch of samples included three blanks, one standard, and one fortified sample (as a quality control). To determine recovery rates (88.5%), substrate and plant samples (without Enro treatment) were spiked with known concentrations of Enro, and then extracted and evaluated.
The Enro distribution among the different plant organs was analyzed by calculating the Translocation factor (TF) and Enro content (Enro concentration x organ DW) of the aboveground organs (shoots + flower) divided by the Enro content of the subterranean organs (roots + rhizomes) as follows:
Where DW: dry weight (weight of the sample after drying).
The natural degradation of Enro in the substrate and the plant removal efficiency of Enro (Removal Efficiency) was calculated as follows (43):
Where: Ci and Cf are the initial and final concentration of Enro in the substrates. To study the Enro bioconcentration behavior, the bioconcentration factor (BCF) was calculated by dividing the Enro-concentration in plant tissues (ppm) at harvesting by the initial Enro concentration in substrate.
Data analysis
Statistical analyses were performed using JMP 7.0 software (SAS Institute Inc.). The results were expressed as the average of five replicates. Data were tested for normality (Shapiro Wilk) and homoscedasticity (Bartlett), and then statistically evaluated. Data for biomass production, physiological parameters, Enro-degradation in substrates and plant removal efficiency were submitted to one-way analysis of variance (ANOVA) and data for Enro accumulation in plants and Enro concentration in substrates were submitted to two-way ANOVA. The interactions between treatments (S + M), CS + M, S + CM and CS + CM) and plant organs (leaves, flowers, rhizome, and roots), as well as treatments and substrate concentrations (C0, Cf -plants, Cf +plants) were evaluated using two-way ANOVA and the means were compared using the post hoc Tukey test (significance at P < 0.05).
Results
Initial concentration of Enro in substrates
No Enro was detected in substrate comprising soil and manure without artificial Enro contamination (S + M). The average concentration of Enro in contaminated soil was 1.9, 50.4, and 51.5 mg kg-1 for contaminated soil + manure, (CS + M), soil + contaminated manure (S + CM) and contaminated soil + contaminated manure (CS + CM), respectively.
Plant production and physiological responses
The number of flowers and leaves, the times until flowering, as well as the fresh biomass were not affected by manure addition or the presence of Enro in the substrate (Table 1; P>0.05). Similarly, SPAD readings, SOD and CAT activity, as well as H2O2 concentrations were not affected by treatments (Table 1). Moreover, no visual symptoms of toxicity and good flower and foliage conditions (i.e., flower strength and color, absence of chlorotic or necrotic points and physical defects) were observed in plants grown in the presence of the antimicrobial (Figure 1).
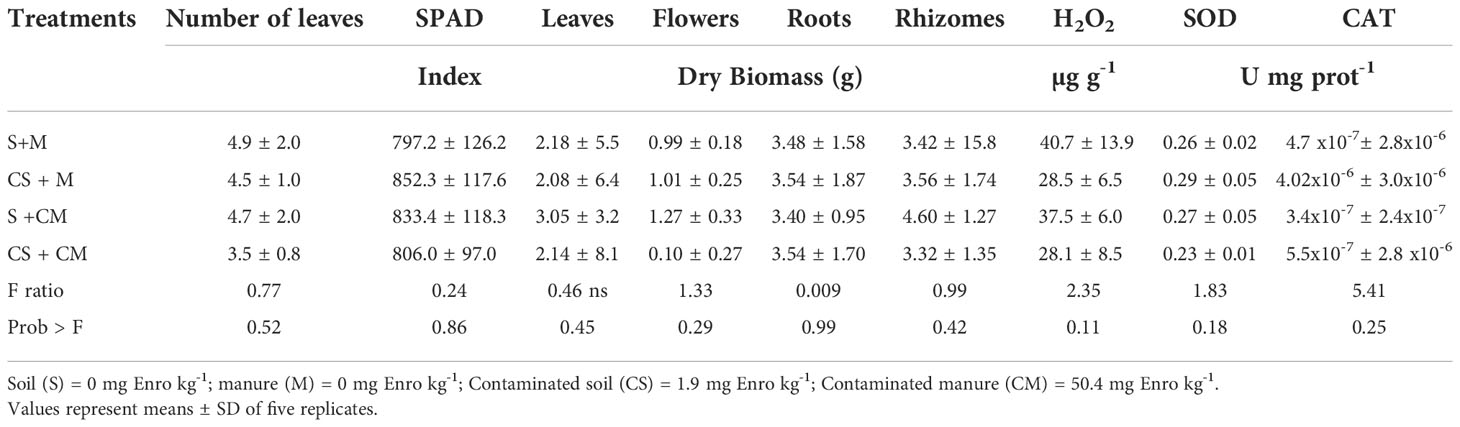
Table 1 Number of leaves, SPAD index, dry biomass of distinct organs, H2O2 concentration and activities of Superoxide Dismutase (SOD) and Catalase (CAT) enzymes in calla lily plants grown in substrates with or without enrofloxacin addition.
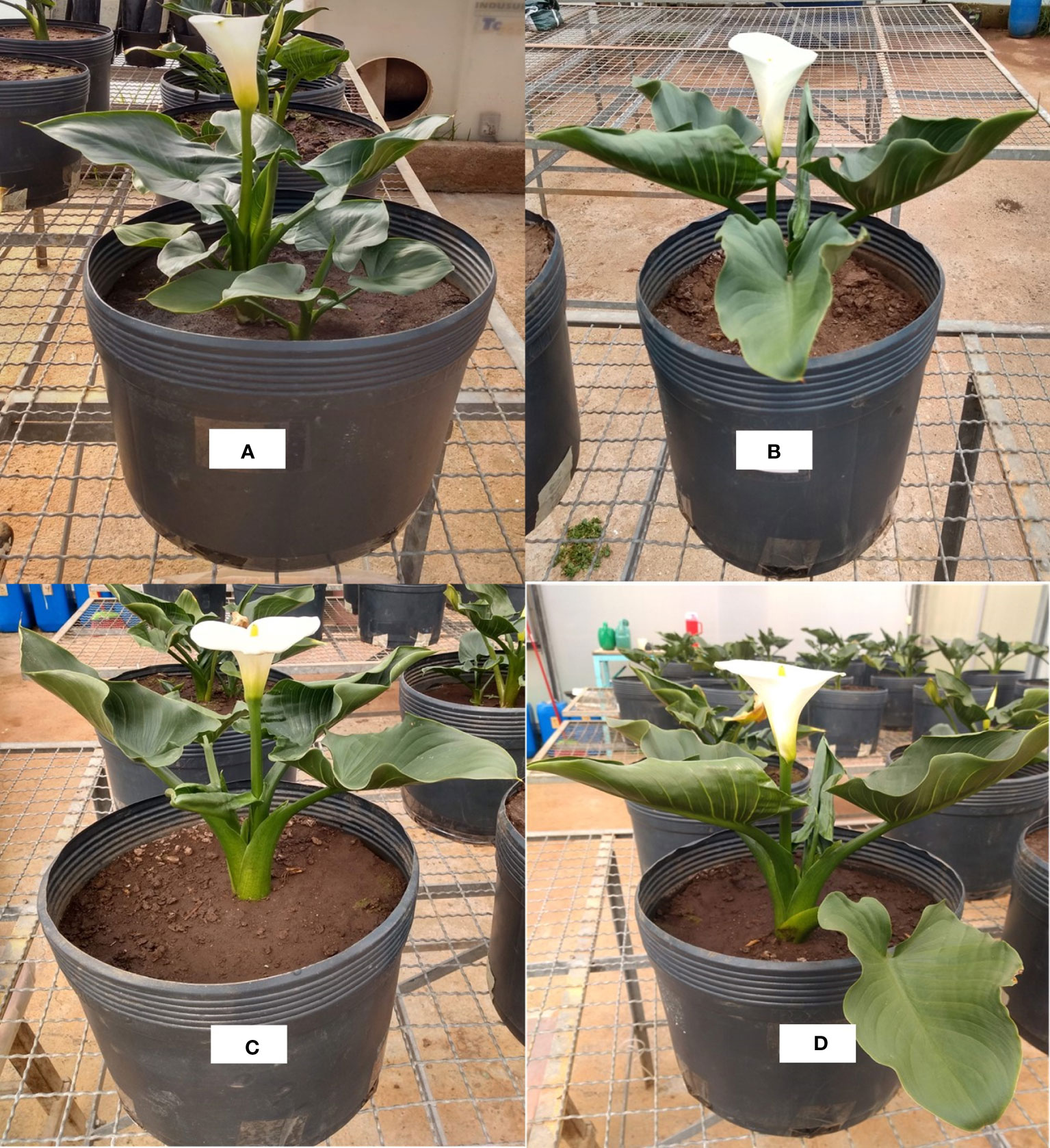
Figure 1 Aspect of calla lily grown in substrates with or without enrofloxacin addition. (A) Soil + manure; (B) Contaminated soil + manure; (C) Soil + contaminated manure; (D) Contaminated soil + contaminated manure.
Enrofloxacin concentration and distribution in plants
No Enro was detected in plant organs grown in non-contaminated substrate (S + M) (Figure 2). Regardless of the plant organ, greater Enro concentration was observed in plants grown in the presence of contaminated manure (S + CM and CS + CM) (Figure 2). Significant interaction between treatments and plant organs was observed for Enro concentration in plants (F=184.17; P<0.0001). When grown in contaminated substrate (CS + M, S + CM and CS + CM), the greatest concentration of Enro was detected in roots, followed by leaves while it did not significantly differ between flowers and rhizomes (Figure 2A).
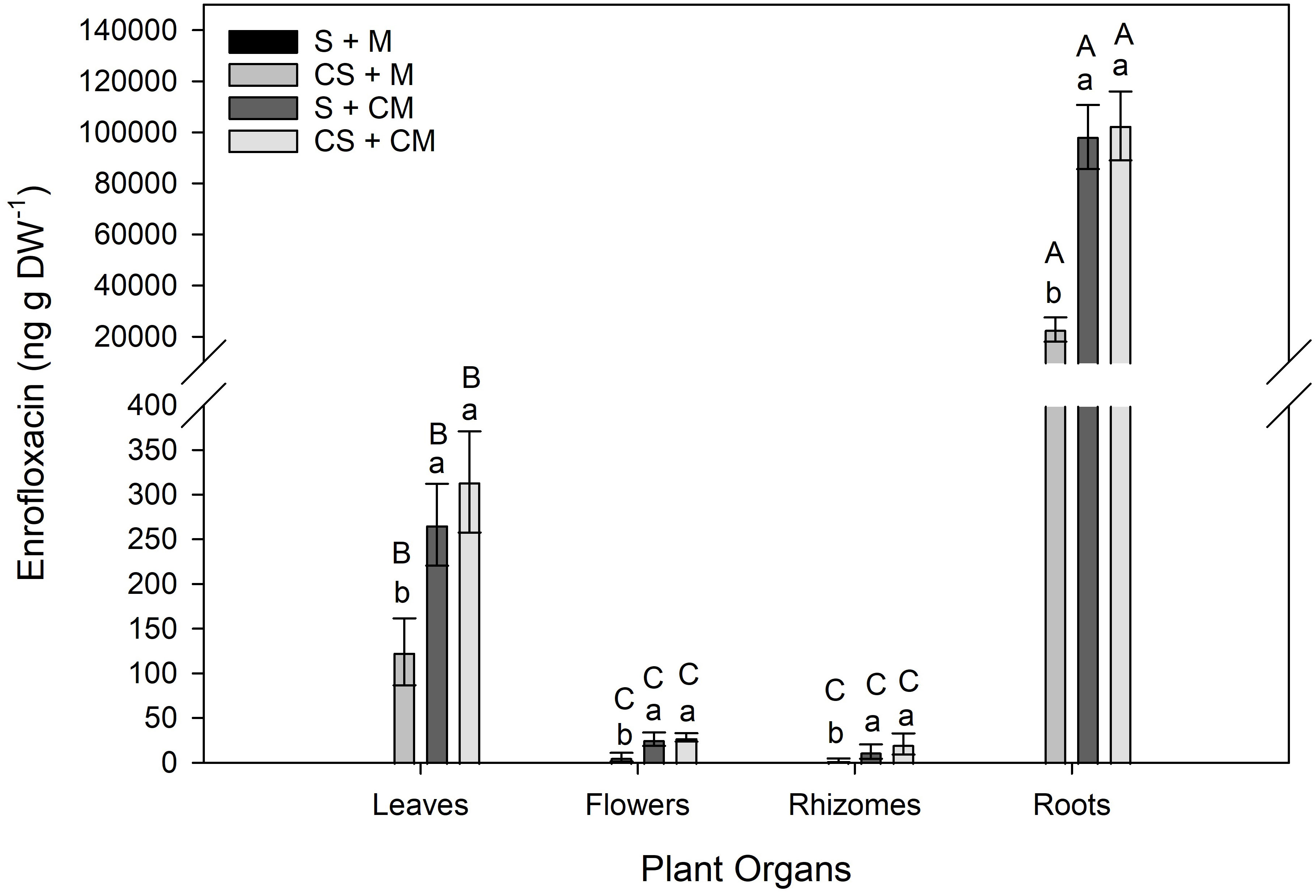
Figure 2 Enrofloxacin concentration in different organs in calla lily plants grown in substrates with or without enrofloxacin addition. Bars represent the means ± SD of five replicates. Lower case letters indicate significant differences between treatments for the same plant organ; uppercase letters indicate significant differences in the pharmaceutical concentration of plant organs of the same treatment. Soil (S) = 0 mg Enro kg-1; manure (M) = 0 mg Enro kg-1; Contaminated soil (CS) = 1.9 mg Enro kg-1; Contaminated manure (CM) = 50.4 mg Enro kg-1.
Phytoremediation capacity
Significantly lower (P<0.05) residual concentrations of Enro were observed in substrate in the presence of plants as compared to the pots without plants (Figure 3A). Natural Enro degradation (F = 255.20; P<0.0001) was the highest and the removal efficiency the lowest (F= 7.89; P<0.001) in substrate where only soil was contaminated with Enro (CS + M). Translocation (TF) and bioconcentration factor (BCF) values were lower than 1, regardless of the treatment (Table 2). For all the treatments, the BCF was higher in roots than in shoots (Table 2). The Enro-TF was greater in plants grown in CS + M, in relation to those grown in substrate receiving contaminated manure (S + CM and CS + CM) (Table 2).
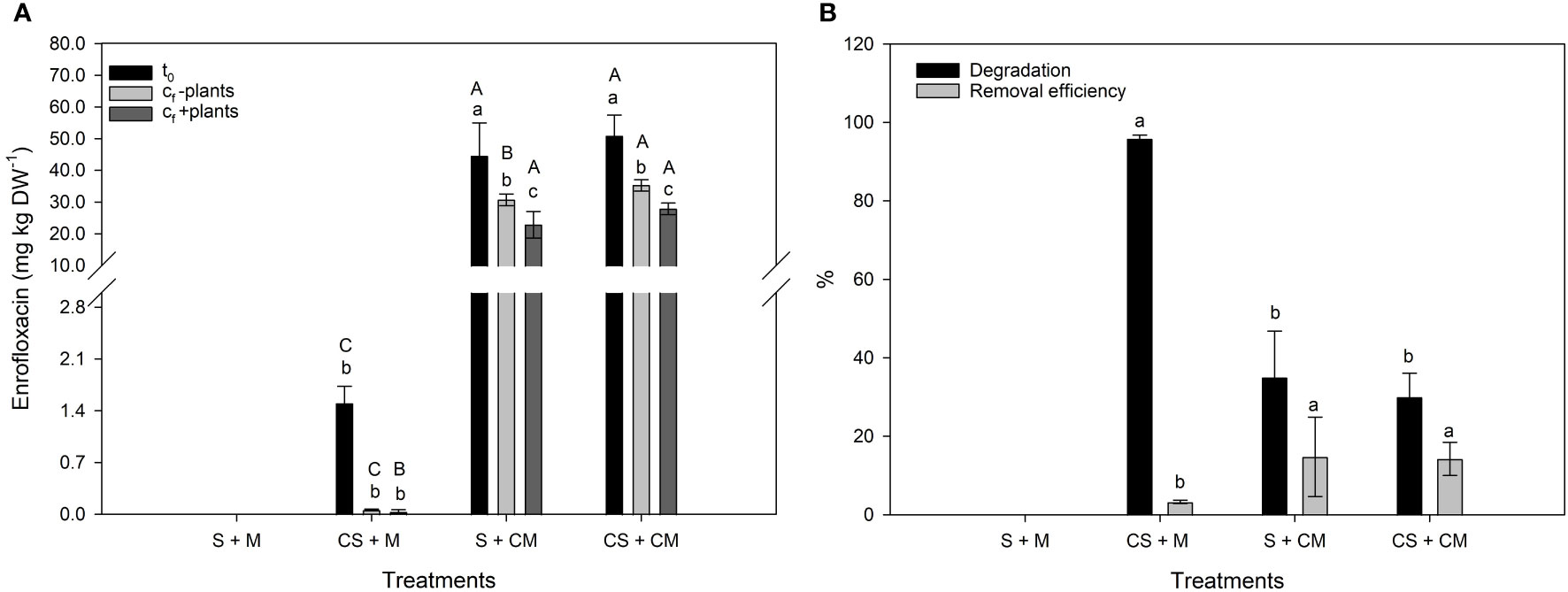
Figure 3 (A) Enrofloxacin concentrations in substrate at the initial (C0) and final time (Cf) in the presence (+plants) or absence (-plants) of calla lily plants. Lower case letters indicate significant differences in Enro concentrations between t0, Cf - plants and Cf + plants within the same treatment; uppercase letters indicate significant differences in the pharmaceutical concentration between treatments within t0, Cf - plants and Cf + plants. (B) Natural degradation in substrates and Enro-removal efficiency of calla lily plants. Different letters indicate significant differences between treatments. Bars represent the means ± SD of five replicates. Soil (S) = 0 mg Enro kg-1; manure (M) = 0 mg Enro kg-1; Contaminated soil (CS) = 1.9 mg Enro kg-1; Contaminated manure (CM) = 50.4 mg Enro kg-1.
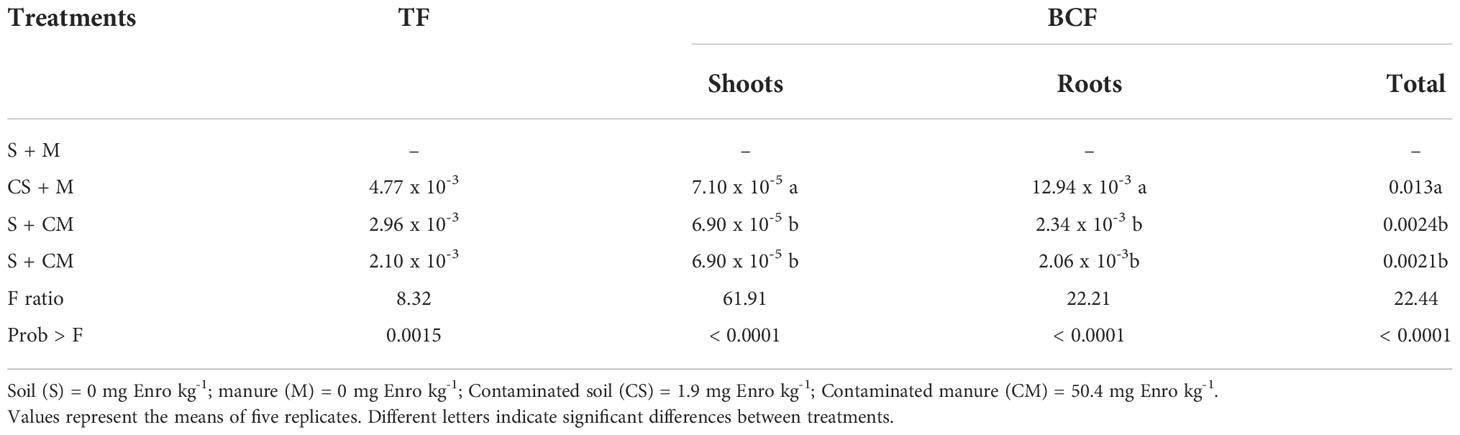
Table 2 Translocation factor (TF) and bioconcentration factor (BCF) in calla lily plants grown in substrates with or without enrofloxacin addition.
Discussion
The non-observed effect of the investigated concentrations of Enro in substrates indicate the plant-tolerance to the pharmaceutical. Enro does not appear to interfere with the photosynthetic metabolism of plants, as it was observed in the aquatic macrophytes Lemna minor (44) and Elodea canadensis (45). Similarly, here, the presence of the drug on plant substrates did not affect photosynthetic pigment levels (as evaluated by SPAD) and biomass production (Table 1).
Shoot biomass production is critical for predicting the commercial value of ornamental plants and is intrinsically related to photosynthetic rates (46) The calla lily tolerance to Enro was also seen by no-effects of Enro on H2O2 concentrations and antioxidant enzymes (SOD and CAT) in the plant leaves. Oxidative damage has been observed in plants exposed to Enro and were associated with Enro-phytotoxicity. For instance, increased ROS formation was followed by increased activity of antioxidant enzymes and decreased concentration of photosynthetic pigments in Scenedesmus obliquus (47) and Chlorella vulgaris (48).
Therefore, the absence of phytotoxic symptoms associated with the maintenance of plant biomass and flower production indicates that calla lily plants are tolerant to Enro and their commercial production may not be disrupted by the use of substrates (such as manure or soil) contaminated with the pharmaceutical.
Although the tolerance of calla lily plants to Enro brings good perspectives for the plant production aspect, the capacity of these plants to accumulate Enro could result in environmental problems as the plants may act as vehicles of the pharmaceutical, transporting it to uncontaminated areas. However, using ornamental plants in the bioaccumulation of Enro presents, to a certain extent, a lower risk to human health hazards because they avoid direct intoxication that could happen through crop plants (35, 49).
Accumulation of Enro in plant tissues have been observed in several agriculture important species, such as corn, soybeans, beans (19), lettuce, common barley (Hordeum vulgare L.), cucumber (Cucumis sativus L.) (50) and wheat (Triticum aestivum) (20). According to Minden et al. (51), Brassica napus and Triticum aestivum are highly likely to be exposed to antibiotics due to soil fertilization with slurry or manure and antibiotics affected the characteristics of these plants, even in small concentrations. The absorption and accumulation of Enro in plants is related to the transpiration rate, involving both energy-dependent active and energy-independent passive processes (52, 53). Once absorbed by roots, Enro can be moved through plant tissues and be transferred to the shoots using the transpiration current pathway (52). The distribution in plant tissues will depend on the physiological and morphoanatomical differences of each species, in addition to the ability to absorb and metabolize antibiotics (19, 54). In this context, the accumulation and distribution of Enro between plant organs must be investigated to attest the safety of the plant’s marketable products - in our case, flowers, and potted plants, mainly.
The greatest part of the observed Enro in plant organs was found in roots, resulting in their greatest BCF in relation to shoots. As indicated by its lower TF (Figure 2B), indeed, Enro was preferentially allocated in subterranean organs, mainly in roots (Figure 2A). Yan et al. (55) also observed greater concentrations of the cipro, another FQ, in the roots of Eichhornia crassipes in relation to their shoots. As a result of the lower translocation of the antimicrobial to shoots, only residual concentrations of Enro were found in flowers (up to 28.37 ng g DW-1). When considering the toxicological aspects, translocating less contaminants to the aerial parts is a desirable characteristic in a bioaccumulating species as the transfer of toxic elements to other links in the trophic chain is also minimized.
Furthermore, the residual concentration found in calla lily flowers is minimal compared to those that would be required to significantly contribute to environmental contamination and, therefore, the sale of calla lily flowers produced under Enro-contaminated conditions is safe. For instance, considering a bouquet comprising 15 flowers (~1.6 g DW flower-1), a maximum of only 680 ng of Enro will be transported by plants. However, special attention must be given when the plants are marketed in pots, because when considering the sum of all organs, leaves, flowers, roots and rhizome, the levels of Enro found were a matter of concern. Roots constitute up to 30% of the total biomass of calla lily plants during the initial period of production, although this percentage reduces overtime with plant maturity (56). Based on their maximum Enro contents, the leaves of calla lily carry up to 988 ng of Enro each; in addition, 3.5 g of roots and 3.7 g of rhizome (average for a plant), carry together, up to 35.9 µg of Enro. Therefore, considering a pot with four leaves and one flower, approximately 350 µg of Enro will be loaded (including the concentration of subterranean organs). This amount of Enro must be observed depending on the quantity and destination of plants sold in pots. Thus, pots that are sold alone do not pose an environmental risk, but if several plants are used, for example, to compose gardens, calla lilies can constitute a vehicle for Enro transmission to uncontaminated environments. The inappropriate use can raise environmental concerns (57). In this case, when they evaluated the use of ornamental plants in phytoremediation, Khan et al. (49) proposed establishing environmental quality standards to avoid secondary pollution using environmentally acceptable and sustainable methods. As a way of ensuring environmental safety, we suggest the commercialization of only calla lily flowers. In addition to attesting the safety of commercialization of calla lily flowers grown under substrate contaminated by Enro, our study also suggests the species to be considered for phytoremediation purposes. It can be observed that ornamental species are an excellent option to diversify crop production in small farms. In addition, these plants have high biomass production, tolerance to abiotic stresses and, particularly, do not enter the food chain (13, 58), which are desirable characteristics for phytoremediation programs.
Compared to other common species used in the phytoremediation system, the destination of plants is more flexible. In addition to the commercialization of cut flowers and foliage, small farms can use ornamental plants to attract tourism (59, 60). Besides that, rural tourism associated with constructed wetlands to reduce livestock contaminants provide new opportunities to familiar farms promoting greener activities for sustainable agriculture (61, 62). The use of ornamental plants in phytoremediation processes reduces the risk of economic losses for the horticultural market (49).
Several studies have reported the phytoremediator capacity of calla lily plants for metal reclaim (9, 11) and for wastewater treatment (12, 63). When considering total plant biomass, plants were able to accumulate up to 100 µg Enro in their tissues, from which up to 99% of Enro was found in plant roots. The BCF lower than 1 (typical in excluding species) associated with the much lower TF (Table 2) indicates the phytostabilization ability of calla lily. In phytostabilization, contaminants are absorbed by plants and are prevented from migrating to shoots (64, 65). Once accumulated in roots, the contaminants are no longer available for moving through environmental matrices, avoiding them entering water, and mainly into the food chain (66–68). Moreover, by allocating Enro in their roots, plants prevent deleterious effects to photosynthetic organs (23, 45, 69), preventing negative effects of Enro on plant yields. Considering an average weight of 10 g of dry mass per plant, and a population density of 10,000 plants ha-1 in producing sites (70), the growth of calla lily in contaminated substrates can remove up to 10,000 mg of Enro per hectare until the first flower production, which can be safety sold. Interestingly, TF was greater in plants grown in CS + M in relation to those grown in substrate receiving contaminated manure (S + CM and CS + CM). This can be related to the total Enro-concentration found inside plants which was greater in S + CM (98511.11 ng g-1 DW)and CS + CM (102914.5 ng g-1 DW) than CS + M plants (22920.53 ng g-1 DW). Often, when in lower concentration inside plants, xenobiotics preset great translocation factor due to the availability of more sites in which they can be placed in plant shoots.
Although the plants have shown the ability to uptake and accumulate Enro, their calculated removal efficiency was < 15% (Figure 3B). It is important to note that the substrate plays an important role in the absorption and translocation of antimicrobials by plants (41), and the physical-chemical properties of the molecule determine the half-life in the environment. Overall, FQs present a high tendency to sorption to solids with slowly degraded rates (half-lives > 60 d), which agrees with our findings, as there was a significant amount in the soil after 180 days of exposure. This occurs as a result of the mechanisms, such as surface complexation, H-binding, cation bridge, ion exchange and hydrophobic partition affect antibiotic sorption in the surface and subsurface (71, 72). Generally, Brazilian soils have pH-dependent charges and low cation exchange capacities (CECs), and thus lower sorption potential for cationic species, such as FQs (72). However, studies have shown that the sorption of FQs is high and rapid (less than 24 h) in tropical soil (72).
The solubility of the compound plays an important role in the capture and translocation of chemicals by plants, because the absorption of the drug is higher when the compound is present in the solution of the soil pores, because water acts as a carrier of chemicals to the roots through symplastic and apoplastic pathways (66). Therefore, adsorption models for antibiotics in the soil still need further study, as often traditional Freundlich or Langmuir models may not exhibit antibiotic adsorption behaviors under different edaphoclimatic conditions, and thus they may not be able to reflect the precise adsorption mechanisms and the effect of plants on adsorption and transport behaviors of these pharmaceutics in the soil (73).
Conclusion
The use of Enro-contaminated soil or manure for growing calla lily plants did not result in impacts on plant productivity. Although plants uptake and accumulate the pharmaceutical in their tissues, up to 99% of the total Enro absorbed was retained in the roots, with only residual concentrations observed in flowers. The commercialization of cut flowers is safe, it offers a reduced risk of biomagnification compared to cultivated plants, but we emphasize that the production of plants under Enro contaminated condition to be sold as pots merits more attention as a relatively high amount of Enro can be transported in the whole plant body. Enro tolerance in plants associated with its ability to accumulate Enro places calla as a potential phytoremediation species, which should be considered when associating economic and environmental gains. Finally, the production of calla lilly plants has dual benefits, remedial and recreational at the same time, therefore, its use should be promoted when designing the phytoremediation strategy of soils and manures contaminated with Enro. However, the use of post-remediation biomass must be demonstrably safe.
Data availability statement
The raw data supporting the conclusions of this article will be made available by the authors, without undue reservation.
Author contributions
CSR, DNMC, MVR, PJ and MPG conceptualization. CSR, LYK., JCMB, LTM, AG, and MPG performed the experiments and analyzed the data; CSR, DNMC, LTM and MPG wrote the original draft; MPG and PJ were responsible for funding acquisition and project administration; DC, MR, and MG supervision; and CR, LM. and MG writing – review & editing. All authors contributed to the article and approved the submitted version.
Funding
This research was financed in part by the Coordenação de Aperfeiçoamento de Pessoal de Nível Superior - Brazil (CAPES) - Finance Code 001, and the Conselho Nacional de Desenvolvimento Científico e Tecnológico (CNPq, Brazil) – Finance Code 406190/2018-6. MG received research productivity grants from CNPq.
Conflict of interest
The authors declare that they have no known competing financial interests or personal relationships that could have influenced the work reported in this paper.
Publisher’s note
All claims expressed in this article are solely those of the authors and do not necessarily represent those of their affiliated organizations, or those of the publisher, the editors and the reviewers. Any product that may be evaluated in this article, or claim that may be made by its manufacturer, is not guaranteed or endorsed by the publisher.
References
2. USDA NASS. Floriculture crops 2019 summary. (SW Washington, DC, USA: National Agricultural Statistic Service) (2020).
3. European Commision. Flowers and ornamental plants production. (Brussels, Belgium: EIP-AGRI network) (2020).
4. Erickson E, Adam S, Russo L, Wojcik V, Patch HM, Grozinger CM. More than meets the eye? the role of annual ornamental flowers in supporting pollinators. Environ Entomol (2020) 49(1):178–88. doi: 10.1093/ee/nvz133
5. Reis SN, dos Reis MV, do Nascimento AMP. Pandemic, social isolation and the importance of people-plant interaction. Ornam Hortic (2020) 26(3):399–412. doi: 10.1590/2447-536x.v26i3.2185
6. Junqueira AH, Peetz M da S. Sustainability in Brazilian floriculture: introductory notes to a systemic approach. Ornam Hortic (2018) 24(2):155–62. doi: 10.14295/oh.v24i2.1253
7. Lowenstein DM, Matteson KC, Minor ES. Evaluating the dependence of urban pollinators on ornamental, non-native, and ‘weedy’ floral resources. Urban Ecosyst (2019) 22(2):293–302. doi: 10.1007/s11252-018-0817-z
8. Rossi JP, Garcia J, Roques A, Rousselet J. Trees outside forests in agricultural landscapes: spatial distribution and impact on habitat connectivity for forest organisms. Landsc Ecol (2016) 31(2):243–54. doi: 10.1007/s10980-015-0239-8
9. Casierra-Posada F, Blanke MM, Guerrero-Guío JC. Iron tolerance in calla lilies (Zantedeschia aethiopica). Gesunde Pflanz. (2014) 66(2):63–8. doi: 10.1007/s10343-014-0316-y
10. Tejeda A, Zurita F. Capacity of two ornamental species (Iris sibirica and Zantedeschia aethiopica) to take up, translocate, and accumulate carbamazepine under hydroponic conditions. Water (Switzerland). (2020) 12(5):8–17. doi: 10.3390/w12051272
11. Del-Toro-Sánchez CL, Zurita F, Gutiérrez-Lomelí M, Solis-Sánchez B, Wence-Chávez L, Rodríguez-Sahagún A, et al. Modulation of antioxidant defense system after long term arsenic exposure in Zantedeschia aethiopica and anemopsis californica. Ecotoxicol Environ Saf (2013) 94:67–72. doi: 10.1016/j.ecoenv.2013.04.024
12. Chen Y, Bracy RP, Owings AD, Merhaut DJ. Nitrogen and phosphorous removal by ornamental and wetland plants in a greenhouse recirculation research system. HortScience (2009) 44(6):1704–11. doi: 10.21273/HORTSCI.44.6.1704
13. Casierra Posada F, Nieto P, Ulrichs C. Crecimiento, producción y calidad de flores en calas (Zantedeschia aethiopica (L.) k. spreng) expuestas a diferente calidad de luz. Rev UDCA Actual Divulg Científica (2012) 15(1):97–105. doi: 10.31910/rudca.v15.n1.2012.806
14. Almeida EFA, Paiva PDO, Frazão JEM, Santos FHS, Resende FA, Campos ML. Produção de copo-de-leite em resposta à adubação com NPK e esterco bovino. Rev Bras Hortic Ornam. (2012) 18(2):129–34. doi: 10.14295/rbho.v18i2.684
15. Mandal A, Patra A, Singh D, Swarup A, Ebhinmasto R. Effect of long-term application of manure and fertilizer on biological and biochemical activities in soil during crop development stages. Bioresour Technol (2007) 98(18):3585–92. doi: 10.1016/j.biortech.2006.11.027
16. Zhou SYiD, Zhu D, Giles M, Daniell T, Neilson R. Yang X ru. does reduced usage of antibiotics in livestock production mitigate the spread of antibiotic resistance in soil, earthworm guts, and the phyllosphere? Environ Int (2020) 136(September 2019):105359. doi: 10.1016/j.envint.2019.105359
17. Checcucci A, Trevisi P, Luise D, Modesto M, Blasioli S, Braschi I, et al. Exploring the animal waste resistome: The spread of antimicrobial resistance genes through the use of livestock manure. Front Microbiol (2020) 11(July):1–9. doi: 10.3389/fmicb.2020.01416
18. Lima T, Domingues S, Da Silva GJ. Manure as a potential hotspot for antibiotic resistance dissemination by horizontal gene transfer events. Vet Sci (2020) 7(3):110. doi: 10.3390/vetsci7030110
19. Marques RZ, Wistuba N, Brito JCM, Bernardoni V, Rocha DC, Gomes MP. Crop irrigation (soybean, bean, and corn) with enrofloxacin-contaminated water leads to yield reductions and antibiotic accumulation. Ecotoxicol Environ Saf. (2021) 216(January):112193. doi: 10.1016/j.ecoenv.2021.112193
20. Riaz L, Mahmood T, Coyne MS, Khalid A, Rashid A, Hayat MT, et al. Physiological and antioxidant response of wheat (Triticum aestivum) seedlings to fluoroquinolone antibiotics. Chemosphere (2017) 177:250–7. doi: 10.1016/j.chemosphere.2017.03.033
21. Nunes B, Veiga V, Frankenbach S, Serôdio J, Pinto G. Evaluation of physiological changes induced by the fluoroquinolone antibiotic ciprofloxacin in the freshwater macrophyte species lemna minor and lemna gibba. Environ Toxicol Pharmacol (2019) 72(January):103242. doi: 10.1016/j.etap.2019.103242
22. Aristilde L, Melis A, Sposito G. Inhibition of photosynthesis by a fluoroquinolone antibiotic. Environ Sci Technol (2010) 44(4):1444–50. doi: 10.1021/es902665n
23. Gomes MP, de Brito JCM, Bicalho EM, Silva JG, de Fátima Gomides M, Garcia QS, et al. Ciprofloxacin vs. temperature: Antibiotic toxicity in the free-floating liverwort ricciocarpus natans from a climate change perspective. Chemosphere (2018) 202:410–9. doi: 10.1016/j.chemosphere.2018.03.048
24. Gomes MP, Gonçalves CA, de Brito JCM, Souza AM, da Silva Cruz FV, Bicalho EM, et al. Ciprofloxacin induces oxidative stress in duckweed (Lemna minor l.): Implications for energy metabolism and antibiotic-uptake ability. J Hazard Mater (2017) 328:140–9. doi: 10.1016/j.jhazmat.2017.01.005
25. Panja S, Sarkar D, Li K, Datta R. Uptake and transformation of ciprofloxacin by vetiver grass (Chrysopogon zizanioides). Int Biodeterior Biodegrad. (2019) 142(April):200–10. doi: 10.1016/j.ibiod.2019.05.023
26. Camotti Bastos M, Rheinheimer dos Santos D, Aubertheau É, de Castro Lima JAM, Le Guet T, Caner L, et al. Antibiotics and microbial resistance in Brazilian soils under manure application. L Degrad Dev (2018) 29(8):2472–84. doi: 10.1002/ldr.2964
27. Gao L, Shi Y, Li W, Niu H, Liu J, Cai Y. Occurrence of antibiotics in eight sewage treatment plants in Beijing, China. Chemosphere (2012) 86(6):665–71. doi: 10.1016/j.chemosphere.2011.11.019
28. Leal RMP, Figueira RF, Tornisielo VL, Regitano JB. Occurrence and sorption of fluoroquinolones in poultry litters and soils from são paulo state, Brazil. Sci Total Environ (2012) 432:344–9. doi: 10.1016/j.scitotenv.2012.06.002
29. Zhao L, Dong YH, Wang H. Residues of veterinary antibiotics in manures from feedlot livestock in eight provinces of China. Sci Total Environ (2010) 408(5):1069–75. doi: 10.1016/j.scitotenv.2009.11.014
30. Parente ET, Azeredo A, Vollú RE, Zonta E, Torres M, Azevedo-silva CE, et al. Chemosphere fluoroquinolones in agricultural soils : Multi-temporal variation and risks in Rio de Janeiro upland region. Chemosphere (2019) 219:409–17. doi: 10.1016/j.chemosphere.2018.11.184
31. Tandon SA, Kumar R, Yadav SA. Pytoremediation of fluoroquinolone group of antibiotics from waste water. Nat Sci (2013) 05(12):21–7. doi: 10.4236/ns.2013.512A004
32. Regitano JB, Leal RMP. Comportamento e impacto ambiental de antibióticos usados na produção animal brasileira. Rev Bras Ciec do Solo. (2010) 34(3):601–16. doi: 10.1590/S0100-06832010000300002
33. Li X, Zhu W, Meng G, Guo R, Wang Y. Phytoremediation of alkaline soils co-contaminated with cadmium and tetracycline antibiotics using the ornamental hyperaccumulators Mirabilis jalapa l. and Tagetes patula l. Environ Sci pollut Res (2020) 27(12):14175–83. doi: 10.1007/s11356-020-07975-2
34. Liu J, Xin X, Zhou Q. Phytoremediation of contaminated soils using ornamental plants. Environ Rev (2018) 26(1):43–54. doi: 10.1139/er-2017-0022
35. Rocha CS, Rocha DC, Kochi LY, Carneiro DNM, dos Reis MV, Gomes MP. Phytoremediation by ornamental plants: a beautiful and ecological alternative. Environ Sci pollut Res (2022) 29(3):3336–54. doi: 10.1007/s11356-021-17307-7
36. Velikova V, Yordanov I, Edreva A. Oxidative stress and some antioxidant systems in acid rain-treated bean plants: Protective role of exogenous polyamines. Plant Sci (2000) 151(1):59–66. doi: 10.1016/S0168-9452(99)00197-1
37. Gomes MP, Le Manac’h SG, Moingt M, Smedbol E, Paquet S, Labrecque M, et al. Impact of phosphate on glyphosate uptake and toxicity in willow. J Hazardous Materials (2016) 304:269–79. doi: 10.1016/j.jhazmat.2015.10.043
38. Beyer WF, Fridovich I. Assaying for superoxide dismutase activity: Some large consequences of minor changes in conditions. Anal Biochem (1987) 161(2):559–66. doi: 10.1016/0003-2697(87)90489-1
40. Bradford MM. A rapid and sensitive method for the quantitation of microgram quantities of protein utilizing the principle of protein-dye binding. Anal Bio (1976) 72:248–54. doi: 10.1016/0003-2697(76)90527-3
41. Migliore L, Cozzolino S, Fiori M. Phytotoxicity to and uptake of enrofloxacin in crop plants. Chemosphere (2003) 52:1233–44. doi: 10.1016/S0045-6535(03)00272-8
42. Shi L, Zhou XF, Zhang YL, Gu GW. Simultaneous determination of 8 fluoroquinolone antibiotics in sewage treatment plants by solid-phase extraction and liquid chromatography with fluorescence detection. Water Sci Technol (2009) 59(4):805–13. doi: 10.2166/wst.2009.062
43. Mendes EJ, Malage L, Rocha DC, Kitamura RSA, Gomes SMA, Navarro-Silva MA, et al. Isolated and combined effects of glyphosate and its by-product aminomethylphosphonic acid on the physiology and water remediation capacity of salvinia molesta. J Hazard Mater (2021) 417(September):125694. doi: 10.1016/j.jhazmat.2021.125694
44. Gomes MP, Moreira Brito JC, Cristina Rocha D, Navarro-Silva MAM, Juneau P, Brito JCM, et al. Individual and combined effects of amoxicillin, enrofloxacin, and oxytetracycline on lemna minor physiology. Ecotoxicol Environ Saf (2020) 203(March):111025. doi: 10.1016/j.ecoenv.2020.111025
45. Gomes MP, Tavares DS, Richardi VS, Marques RZ, Wistuba N, Moreira de Brito JC, et al. Enrofloxacin and roundup® interactive effects on the aquatic macrophyte elodea canadensis physiology. Environ pollut (2019) 249:453–62. doi: 10.1016/j.envpol.2019.03.026
46. Ferrante A, Trivellini A, Scuderi D, Romano D, Vernieri P. Post-production physiology and handling of ornamental potted plants. Postharvest Biol Technol (2015) 100:99–108. doi: 10.1016/j.postharvbio.2014.09.005
47. Qin H, Chen L, Lu N, Zhao Y, Yuan X. Toxic effects of enrofloxacin on scenedesmus obliquus. Front Environ Sci Eng China. (2012) 6(1):107–16. doi: 10.1007/s11783-011-0327-1
48. Wang G, Zhang Q, Li J, Chen X, Lang Q, Kuang S. Combined effects of erythromycin and enrofloxacin on antioxidant enzymes and photosynthesis-related gene transcription in chlorella vulgaris. Aquat Toxicol (2019) 212:138–45. doi: 10.1016/j.aquatox.2019.05.004
49. Khan AHA, Kiyani A, Mirza CR, Butt TA, Barros R, Ali B, et al. Ornamental plants for the phytoremediation of heavy metals: Present knowledge and future perspectives. Environ Res (2021) 195:110780. doi: 10.1016/j.envres.2021.110780
50. Lillenberg M, Litvin SV, Nei L, Roasto M, Sepp K. Enrofloxacin and ciprofloxacin uptake by plants from soil. Agron Res (2010) 8(1):807–14.
51. Minden, V, Deloy A, Volkert A. M., Leonhardt S. D., Pufa G.. Antibiotics impact plant traits, even at small concentrations. AoB PLANTS (2017) 9:plx010. doi: doi.org/10.1093/aobpla/plx01
52. Dodgen LK, Ueda A, Wu X, Parker DR, Gan J. Effect of transpiration on plant accumulation and translocation of PPCP/EDCs. Environ pollut (2015) 198:144–53. doi: 10.1016/j.envpol.2015.01.002
53. Zhang C, Xue J, Cheng D, Feng Y, Liu Y, Aly HM, et al. Uptake, translocation and distribution of three veterinary antibiotics in Zea mays l. Environ pollut (2019) 250:47–57. doi: 10.1016/j.envpol.2019.03.110
54. Gomes MP, Rocha DC, Moreira de Brito JC, Tavares DS, Marques RZ, Soffiatti P, et al. Emerging contaminants in water used for maize irrigation: Economic and food safety losses associated with ciprofloxacin and glyphosate. Ecotoxicol Environ Saf (2020) 196(March):110549. doi: 10.1016/j.ecoenv.2020.110549
55. Yan Y, Pengmao Y, Xu X, Zhang L, Wang G, Jin Q, et al. Migration of antibiotic ciprofloxacin during phytoremediation of contaminated water and identification of transformation products. Aquat. Toxicol (2020) 219:1–7. doi: doi.org/10.1016/j.aquatox.2019.105374
56. Carneiro DNM. Acúmulo de nutrientes em copo-De-Leite em fase inicial de cultivo. Dissertação (Mestrado em Fitotecnia)-Universidade Federal de Lavras, Lavras (2009) 53.
57. Aftab N, Saleem K, Khan A, Butt T, Mirza C, Hussain J, et al. Cosmos sulphureus Cav. is more tolerant to lead than copper and chromium in hydroponics system. Int. J. Environ. Sci. Technol. (2021) 18:2325–34. doi: 10.1007/s13762-020-02981-w
58. Fonseca AS, Segeren MI. Nutrient uptake in two species of calla lily (Zantedeschia sp.) under fertigation. Acta Hortic (2013) 1000:377–84. doi: 10.17660/ActaHortic.2013.1000.52
59. Gao J, Barbieri C, Valdivia C. Agricultural landscape preferences: Implications for agritourism development. J Travel Res (2014) 53(3):366–79. doi: 10.1177/0047287513496471
60. Chase L, Stewart M, Schilling B, Smith B, Walk M. Agritourism: Toward a conceptual framework for industry analysis. J Agric Food Syst Community Dev (2018) 8(1):1–7. doi: 10.5304/jafscd.2018.081.016
61. Xu H, Wang C, Wu J, Liang Y, Jiao Y, Nazneen S. Human poverty alleviation through rural women’s tourism entrepreneurship. J China Tour Res (2018) 14(4):445–60. doi: 10.1080/19388160.2018.1507860
62. Raynolds LT. Gender equity, labor rights, and women’s empowerment: lessons from fairtrade certification in Ecuador flower plantations. Agric Hum Values. (2021) 38(3):657–75. doi: 10.1007/s10460-020-10171-0
63. Figueiredo JR, Almeida, Fabiana EA, Freitas GDF, Reis Ns, Carvalho LMdeC. Development of calla lily as result of fertilization with biofertilizer. Rev Bras Agropecuária Sustentável. (2014) 4(2):1–5. doi: 10.1590/2447-536x.v28i2.2476
64. McCutcheon SC, Susarla S, Medina VF. Phytoremediation: An ecological solution to organic chemical contamination. Ecol Eng (2002) 18(5):647–58. doi: 10.1016/S0925-8574(02)00026-5
65. Buscaroli A. An overview of indexes to evaluate terrestrial plants for phytoremediation purposes (Review). Ecol Indic (2017) 82(June):367–80. doi: 10.1016/j.ecolind.2017.07.003
66. Yu X, Chen J, Liu X, Sun Y, He H. The mechanism of uptake and translocation of antibiotics by pak choi (Brassica rapa subsp. chinensis). Sci Total Environ (2022) 810:151748. doi: 10.1016/j.scitotenv.2021.151748
67. Pullagurala VLR, Rawat S, Adisa IO, Hernandez-Viezcas JA, Peralta-Videa JR, Gardea-Torresdey JL. Plant uptake and translocation of contaminants of emerging concern in soil. Sci Total Environ (2018) 636:1585–96. doi: 10.1016/j.scitotenv.2018.04.375
68. Christou A, Papadavid G, Dalias P, Fotopoulos V, Michael C, Bayona JM, et al. Ranking of crop plants according to their potential to uptake and accumulate contaminants of emerging concern. Environ Res (2019) 170(November 2018):422–32. doi: 10.1016/j.envres.2018.12.048
69. Linke GE, Chen J, Xiaoxuan W, Zhang S, Qiao X, Xiyun And C, et al. Aquatic photochemistry of fluoroquinolone antibiotics: kinetics, pathways, and multivariate effects of main water constituents. Environ Sci Technol (2010) 44(7):2400–5. doi: 10.1021/es902852v
70. Silva GR. Comportamento do copo-de-leite-em função de níveis de sombreamento, espaçamentos de plantio e períodos de convivência com plantas daninhas. (2017) 4:59. Tese (Doutorado em Fitotecnia)-Universidade Federal de Viçosa, Viçosa.
71. Parente CET, Brito EMS, Azeredo A, Meire RO, Malm O. Fluoroquinolone antibiotics and their interactions in agricultural soils - a review. Orbital (2019) 11(1):42–52. doi: 10.17807/orbital.v11i1.1352
72. Leal RMP, Alleoni LRF, Tornisielo VL, Regitano JB. Sorption of fluoroquinolones and sulfonamides in 13 Brazilian soils. Chemosphere (2013) 92(8):979–85. doi: 10.1016/j.chemosphere.2013.03.018
Keywords: Zantedeschia aethiopica, ornamental plant, contaminants, phytoremediation, soil
Citation: Rocha CdS, Kochi LY, Brito JCM, Maranho LT, Carneiro DNM, Reis MVd, Gauthier A, Juneau P and Gomes MP (2022) Calla lily production in enrofloxacin-contaminated soil and manure: An attractive alternative coupling income generation with antimicrobial removal from the environment. Front. Soil Sci. 2:1060937. doi: 10.3389/fsoil.2022.1060937
Received: 03 October 2022; Accepted: 18 November 2022;
Published: 02 December 2022.
Edited by:
Jianxu Wang, Institute of Geochemistry, Chinese Academy of Sciences (CAS), ChinaReviewed by:
Huifang Ma, Qilu University of Technology, ChinaKang Mao, Institute of Geochemistry, Chinese Academy of Sciences (CAS), China
Copyright © 2022 Rocha, Kochi, Brito, Maranho, Carneiro, Reis, Gauthier, Juneau and Gomes. This is an open-access article distributed under the terms of the Creative Commons Attribution License (CC BY). The use, distribution or reproduction in other forums is permitted, provided the original author(s) and the copyright owner(s) are credited and that the original publication in this journal is cited, in accordance with accepted academic practice. No use, distribution or reproduction is permitted which does not comply with these terms.
*Correspondence: Marcelo Pedrosa Gomes, bWFyY2Vsby5nb21lc0B1ZnByLmJy