Impacts of summer-seeded legume cover crops and termination-tillage method on nitrogen availability to corn in rotation
- Harrow Research and Development Centre, Agriculture and Agri-Food Canada, Harrow, ON, Canada
Summer-seeding legume cover crops can reduce erosion, mitigate nitrate leaching losses during the non-growing season, and provide bio-accumulated nitrogen (N) to the following crop. Very little information is available, however, on the most effective methods for terminating summer-seeded cover crops, or on the amount of N they can provide to subsequent crops. We therefore evaluated the impacts of selected legume cover crops and cover crop termination-tillage methods on corn (Zea mays L.) N credit and grain yield in a soybean (Glycine max Merr.) - winter wheat (Triticum aestivum L.)/cover crop - corn rotation over 3 consecutive years on a clay loam soil in southwestern Ontario. The cover crops were summer-seeded after wheat harvest, and included hairy vetch (Vicia villosa L. Roth), red clover (Trifolium pratense L.), white clover plus crimson clover mix (incarnatum and Trifolium alba L.), and a no cover crop control (CK). The cover crops were nested within termination-tillage method, including moldboard plow-down in fall, and herbicide spray-down in spring under strip-tillage or no-tillage then corn planting. Nitrogen fertilizer to corn was applied via side-dress at 200 kg N ha-1 to the CKs, and at 100 kg N ha-1 to the cover crops. Averaged over 3 years, above-ground biomass N level followed the pattern: hairy vetch > white clover plus crimson clover mix > red clover for fall plow-down termination. There were no significant differences in cover crop biomass N levels for spring termination. Corn response to cover crop was significantly affected by termination-tillage method and cover crop species, with poor corn stands after no-tillage, red clover and white plus crimson clover. Fall plow-down termination provided a range in N credit of 80-85 kg N ha-1, which was significantly greater than the N credit under herbicide spray-down in spring. Under spring strip-tillage and no-tillage, hairy vetch produced significantly greater corn grain yields than red clover and white plus crimson clover mix.
1 Introduction
Efficient management of cover crops can increase soil organic matter, reduce soil erosion, conserve root-zone soil moisture, and enhance nitrogen (N) fertility (1–3). Legume winter-annual cover crops can also be a substantial source of N for the following crop in a crop rotation, especially in humid continental climates (4, 5). For example, Blevins et al. (6) found in Kentucky that hairy vetch (HV, Vicia villosa Roth) can supply about 76 kg N ha-1 to corn and 125 kg N ha-1 to grain sorghum, while Ott and Hargrove (7) found that both HV and crimson clover (CC, Trifolium incarnatum L.) increased average corn yields throughout Georgia. In addition, Pott et al. (8) showed that the fertilizer N replacement value of HV could reach 151 kg N ha−1 for corn in low fertility soils in Brazil, and Ranells and Wagger (9) showed that CC can provide up to 2/3 of the N requirement for corn in North Carolina.
However, the effectiveness of cover crops for increasing crop productivity and grain yields varies substantially with weather, length of growing season, soil type, crop species, and cover crop characteristics such as biomass production, C:N ratio and residue decomposition rate (10–12). For example, HV can release up to 80% of its accumulated biomass N within 5 weeks after termination, while CC releases about 60% in the same time period (9, 13, 14). Relative to other legume species, CC has slower N release, a narrower range of soil types where optimum performance is achieved, and a tendency to deplete soil moisture (15, 16). In a laboratory incubation study, Yang et al. (17) found that potentially mineralizable N (as % of initial N) was similar (71-75%) among shoots of CC, HV and red clover (RC, Trifolium pratense L.), but was substantially lower for CC roots (36.2%) than for HV and RC roots (53.0%). Some studies showed that cover crop monocultures produce greater biomass than mixtures (18–20), but others have shown that mixtures produced more biomass than their single species counterparts (21). Antosh et al. (22) found that cover crop mixtures containing hairy vetch did not accumulate as much N as hairy vetch grown alone. Hence, a legume monoculture, such as hairy vetch, may be a better strategy if the primary goal is to increase soil N.
The effectiveness of legume cover crops for producing biomass, sequestering carbon and fixing atmospheric N is impacted by the time and method of cover crop termination. Termination times range from early to late fall of the same year that the cover crop is planted, or from early spring to late spring of the following year (23, 24). Termination methods range from aggressive tillage (e.g. moldboard or chisel plowing) which incorporates most or all of the cover crop biomass into the soil, to roller-crimping or herbicide spray-down which leaves a layer of cover crop mulch on the soil surface with minimal incorporation (25–28). Generally speaking, late-terminated cover crops accumulate more biomass and biomass N than early-terminated cover crops. Clark et al. (23) found that HV terminated in early to mid-April accumulated an average of 96 kg N ha–1, while late May termination accumulated up to 149 kg N ha–1. Parr et al. (11) found that HV terminated in mid-April accumulated 122 to 168 kg N ha–1, while late May termination produced 155 to 217 kg N ha–1. Coombs et al. (24) determined in an Ontario study that RC produced significantly greater plant available N in both soil and above-ground biomass when it was spring-terminated as opposed to fall-terminated. Cover crop termination via tillage versus crimping or spraying affects cover crop decomposition, with N release occurring faster and more completely from cover crops incorporated into the soil than from cover crops left lying on the soil surface (29). Cover crop surface mulches, on the other hand, can be very effective at suppressing weeds and conserving root zone soil and water (30–32), but may also reduce corn germination and early growth by delaying soil dry-down and warming (33, 34). For a sandy loam soil in southwestern Ontario, Yang et al. (35) obtained an early May above-ground biomass N accumulation of 240 kg N ha–1 from HV, 199 kg N ha–1 from RC, and 119 kg N ha–1 from CC. In that study, when the biomass was incorporated into the soil by moldboard plowing in spring, HV and RC provided a fertilizer N replacement value (N credit) of 150 kg N ha–1, and CC provided a fertilizer N replacement value of 139 kg N ha–1. In a meta-analysis of 86 field trials under humid temperate climatic conditions, Bourgeois et al. (36) showed that cover crops increased average corn grain yields by 13% relative to no cover crop.
The “classic” soybean (Glycine max (L.) Merr) - winter wheat (Triticum aestivum L.) – corn (Zea mays L.) rotation is very popular in southwestern Ontario (37). A ttempts to include cover crops in this rotation have thus far focused largely on surface broadcast of RC seed onto frozen ground (frost-seeding). Frost seeding has met with limited success, however, as there are often insufficient freeze-thaw cycles to incorporate the seed, thereby resulting in inconsistent RC stands (38, 39). An alternative approach may be to summer-seed RC or other cover crops into post-harvest wheat stubble when the soil is warm and dry.
We are not aware of any Ontario studies evaluating the performance of summer-seeded legume cover crops after winter wheat harvest.
The objectives of this study were consequently to determine for fine-textured soils in southwestern Ontario: i) the quantity of N accumulated in the above-ground biomass of selected legume cover crops when summer-seeded into post-harvest winter wheat stubble of a soybean-wheat-corn rotation; and ii) the impacts of cover crop species and termination method on corn N credit and corn grain yield.
2 Materials and methods
2.1 Field site and experimental design
The field trial was established in 2017 at the Honourable Eugene F. Whelan Experimental Farm, Agriculture and Agri-Food Canada, Woodslee, southwestern Ontario, Canada (42°13’N, 82°44’W). Southern Ontario has a humid continental climate with four distinct seasons, and during the study years (2017-2020), mean annual air temperature and precipitation at the site were 9.8°C and 874 mm, respectively (Table S1).
The soil was of the Brookston series (Orthic Humic Gleysol, Canadian 40) with a clay loam texture in the top 15 cm (sand = 331 g kg-1, silt = 374 g kg-1, clay = 295 g kg-1 via the pipette method of 41). Basic soil chemical characteristics included: pH of 6.3 (soil:water = 1:2); total carbon and total nitrogen contents of 18.8 g kg-1 and 1.83 g kg-1, respectively, via dry combustion (LECO CN-2000); available phosphorus of 15.0 mg kg-1 via Olsen method (42); and available potassium of 142 mg kg-1 via ICP-ammonium acetate extraction (43).
The cropping practice at the site for at least 4 years prior to the experiment was a corn – soybean - winter wheat/cover crop rotation. During that period, each phase of the rotation was fertilized according to standard soil test recommendations (37). For this study, the site was reorganized into three adjacent fields, allowing each phase of the corn-soybean-winter wheat rotation to be present each year. During the winter wheat phase, selected legume cover crops were planted into disked winter wheat stubble shortly after winter wheat harvest in mid to late summer of 2017, 2018 and 2019 (Table 1).
The field trial was a split-plot design with 4 replications having termination-tillage as the main plot and cover crop species as the sub-plot. The termination-tillage main treatments included: i) fall termination of the cover crop by moldboard plowing after first hard (killing) frost (mid to late November), then seed-bed preparation with secondary tillage and corn planting in the following spring (CT); ii) spring herbicide spray-termination of the cover crop, then no-till corn planting (NT); and iii) spring herbicide spray-termination of the cover crop followed by spring strip tillage, and then corn planting (ST). The cover crop split-plot treatments were randomly nested within the termination-tillage treatments, and included: i) hairy vetch (HV, seeding rate = 25 kg ha-1); ii) red clover (RC, seeding rate = 12 kg ha-1); iii) white clover plus crimson clover (WCCC) applied as a 50:50 mix (seeding rates = 12.5 kg ha-1 for each); and iv) no cover crop treatment (CK). Hence, there were a total of 12 termination-tillage-cover crop combinations per block, i.e. (CT, NT, ST) × (WCCC, HV, RC, CK). The individual plots were 9.2 m wide by 30 m long for the cover crops (12 corn rows at 0.76 m spacing), and 6.1 m wide by 30 m long for the controls (8 corn rows at 0.76 m spacing). The clovers were broadcast on the surface and planter chains lightly raked the soil so the seed was just under the surface. The HV was drill-planted to 10-15 mm depth with 180 mm row spacing.
All of the cover crops are considered winter-hardy in southwestern Ontario, and considerable re-growth usually occurs in the following spring if the cover crop was not intentionally terminated in the fall. It should also be noted that herbicide termination of white clover was often only partially effective, and the WCCC treatment was consequently changed in 2019 to crimson clover only (seeding rate = 25 kg ha-1), which may have impacted the final (3rd) year of the study. Seeding rates used in this study followed Ontario Ministry of Agriculture, Food, and Rural Affairs (OMAFRA) recommendations (http://www.omafra.gov.on.ca/english/crops/facts/cover_crops01/covercrops.htm).
2.2 Agronomic operations and sample collection
Detailed agronomic operations and sample collection are listed in Table 1. Accumulated above-ground biomass and biomass N in cover crops were determined from biomass samples collected by cutting the cover crop flush with the soil surface inside two demarcation frames (200 mm × 750 mm), then placing the cut material in paper bags, oven-drying (60 °C) for ~ 1 week, and then recording dry weights. Above-ground biomass and biomass N were determined in the fall from CT just before cover crop termination by moldboard plow-down to 180 mm depth (Nov. 29 2017, Nov. 19 2018, Nov. 21 2019), and in the following spring from NT and ST just before cover crop termination by herbicide spray-down (May 1 2018, May 14 2019, May 21 2020). Plow-down termination occurred in November after the first killing frost. Herbicide termination occurred in May using a mix of glyphosate (1.4 kg ha-1 a.i.), 2,4-D (0.56 kg ha-1 a.i.) and dicamba dimethylamine (0.6 kg ha-1 a.i.). Spring tillage occurred a few days before corn planting, and included strip-tilling the ST plots using a Pluribus-V type strip tiller (Dawn Equipment, IL, USA), plus disking, harrowing and packing the CT plots.
Corn (cultivar PO506AM) was seeded (May 31 2018, June 24 2019, June 5 2020) in 0.76 m rows at approximately 76800 seeds ha-1 using a Kinze no-till planter. Corn stands were determined 14 days after corn planting by counting emerged seedlings in two random 2-m row lengths per plot. Phosphorous and potassium fertilizers were applied at planting according to soil test recommendations (37). Nitrogen fertilizer (28-0-0 urea ammonium nitrate, UAN) was applied via side-dress (corn 6-leaf growth stage) at 200 kg N ha-1 to the no cover crop control plots, and at 100 kg N ha-1 to the cover crop plots. The N rate applied to the controls is within range of the rates typically recommended for corn production in southern Ontario (37). The cover crops received half the control N rate (i.e. 100 kg N ha-1) because legume cover crops are known to accumulate about 200 kg N ha-1 in biomass and roots (unpublished data), and we assumed 50% of biomass N would be available to the subsequent corn crop. Corn was harvested on Nov. 28 2018, Dec. 13 2019 and Nov. 19 2020, and grain yields determined at 15.5 wt. % seed moisture content.
Composited soil core samples (0 to 300-mm depth 17.5 mm diameter, n = 10) were collected from the corn treatments (about one month after corn planting and about two weeks after side-dress N application) to determine root zone and concentrations. The soil was occasionally too hard to sample, causing some of the collection dates to be missed or core depth to be limited to the top 150 mm. As a result, only data from top 150 mm are reported here. Corn chlorophyll status was measured at the R1 corn growth stage using a SPAD-502 leaf chlorophyll reader (Minolta Corporation, New Jersey, USA), and used as an estimate of N uptake status at the R1 corn stage.
2.3 Mineral soil nitrogen ( And ), biomass, grain N and C analyses
Soil inorganic nitrogen (-N and -N) was determined via the KCl extraction method of Tel and Heseltine (44) using the Lachat QuikChem 8500 Analyzer (HACH, Dusseldorf, Germany). Oven-dried biomass samples and corn grain were ground to 2 mm and total C and N contents were determined using an automatic CN analyzer (CN-2000, Leco Corp., Saint Joseph, MI, USA).
2.4 Calculation of fertilizer N replacement value by cover crops
Apparent fertilizer N replacement value, FNRVA (kg N ha-1), of legume cover crop was calculated for each of the 9 cover crop-termination-tillage combinations, i.e. (CT, NT, ST) x (WCCC, HV, RC), using:
where CYtrt (kg ha-1) is corn grain yield for the selected cover crop-termination-tillage treatment combination, and CYck (kg ha-1) is corn grain yield in the appropriate control, i.e. CT-CK, NT-CK or ST-CK. The numbers 200 and 100 are the amounts of fertilizer N (kg N ha-1) applied to the CKs and cover crops, respectively. Hence, Eq. (1) calculates apparent corn N credit relative to the grain yield and N application rate of the controls; and as a result, FNRVA = 100 kg N ha-1 is obtained when CYtrt = CYck, and FNRVA = 0 kg N/ha is obtained when CYtrt = 0.5×CYck. This approach was taken because it provides a value that is both readily calculated and more relevant to grain corn producers than the usual approach, i.e. difference in total above-ground biomass N between treatment and control (45).
2.5 Statistical analyses
Analysis of variance (ANOVA) was carried out using the Statistical Analysis System (SAS, Cary, N.C.). Cover crop accumulated biomass N levels were analyzed for November and May. Biomass N, soil N, chlorophyll reading, grain yield and FNRVA were analyzed using a split-plot experimental design, with tillage-termination method treated as the main factor, cover crop type as the split factor, and year as a repeated measure. Fisher’s protected least significant difference (LSD) test (α = 0.05) was used to identify significant treatment main effects.
3 Results
3.1 Air temperature and precipitation during the study period
Mean monthly air temperature and precipitation over the four-year study (2017-2020) are shown in Table S1. Year 2017 and 2020 had slightly higher average annual air temperature (10.4-10.5°C) than 2018 and 2019 (9.6-9.8°C), and 2018 and 2019 had similar air temperatures relative to the long-term average (9.6°C). The soil was periodically subjected to below-freezing temperatures during winter, with 2018 and 2019 having a colder January (-4.6 to -4.7°C) than 2020 (-1.4°C in February). These temperature differences significantly impacted cover crop re-growth and biomass accumulation in the spring. Annual precipitation for the study period was 845 mm for 2017, 922 mm for 2018, 816 mm for 2019, and 855 mm for 2020, which were all relatively close (-8% to +4%) to the long-term average (890 mm). Spring 2020 (April and May) was relatively dry (147 mm two-month total precipitation), whereas spring 2018 was somewhat wet (199 mm). Spring 2019, on the other hand, was wet enough (205 mm) to cause a 3-week delay in corn planting. In contrast to spring, summer 2020 (June to September) was substantially wetter (387 mm two-month total precipitation) relative to summer 2018 (300 mm) and summer 2019 (290 mm). These rainfall differences likely affected annual corn yields and N uptake.
3.2 Nitrogen and carbon contents in cover crop biomass
All three cover crops established well after summer-seeding into wheat stubble, and they all achieved 100% soil coverage by fall (Figure S1). Although the selected cover crops are considered winter hardy in southwestern Ontario, partial winter kill was observed in 2018 and 2019, particularly for crimson clover and hairy vetch; and this in turn affected cover crop re-growth and biomass accumulation in the following spring. Accordingly, accumulated cover crop above-ground biomass and biomass N were reported both for the first dormancy frost in late November just before plow-down (data from CT treatment only), and also for early May just before herbicide spray-down (data from ST and NT treatments pooled).
There was substantial annual variation in above-ground biomass N and C concentrations by the cover crop treatments (Figure 1). Nitrogen concentration was higher in HV (31.2 -31.7 g N kg-1) than in RC and WCCC (19.9 – 27.4 g N kg-1) in both fall and spring samples, and greater N concentration was found in WCCC (24.7 g N kg-1) than in RC (19.9 g N kg-1) in fall samples but not in spring samples (Figure 1A). All cover crops had significantly greater above-ground biomass C concentrations in spring (423 - 432 g kg-1) than in fall (335 – 388 g kg-1), and C concentration was higher in HV than in RC and WCCC in fall samples (Figure 1B). The November and May C:N ratios were 15.1 to 16.8 for RC and WCCC; however, the C:N ratio for HV was lower in November (12.4) and May (13.6) (Figure 1C).
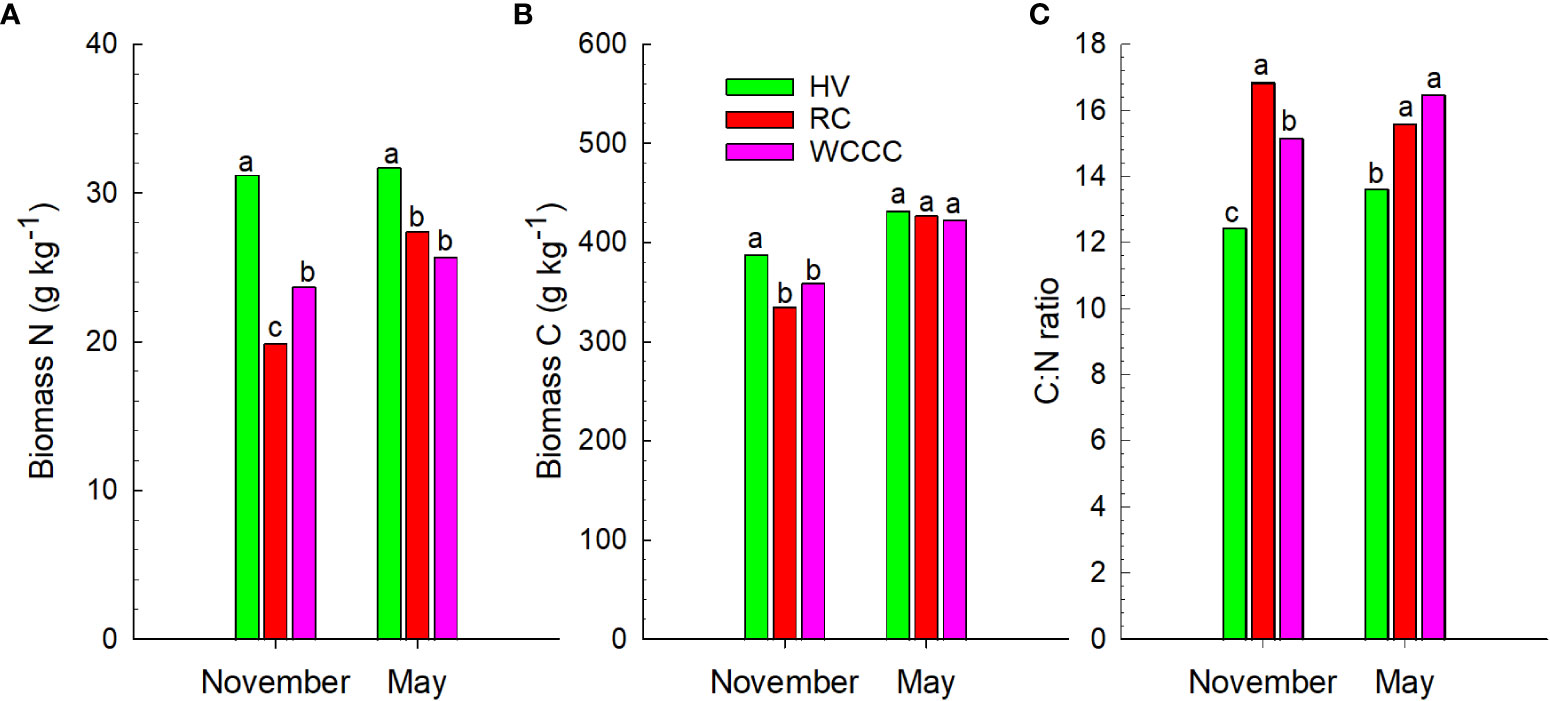
Figure 1 Three-year average (A) N content in biomass, (B) C content in biomass, and (C) C:N ratio for cover crop above-ground biomass at fall freeze-up (November) and late spring (May). HV, hairy vetch; RC, red clover; WCCC, white-crimson clover mix. Bars for the same parameter and sampling time are not significantly different (P = 0.05) if labelled with the same letter.
Annual average above-ground biomass and biomass N varied among year, season and cover crop (Table 2). In 2017-2018 and 2019-2020, cover crop biomass was significantly lower for RC (4.0 Mg ha-1) than HV (5.8 and 6.2 Mg ha-1) and WCCC (6.2 and 7.2 Mg ha-1) in November at the start of over-winter dormancy. November 2017 cover crop biomass N was RC (93 kg N ha-1)< WCCC (163 kg N ha-1)< HV (213 kg N ha-1), and November 2018 cover crop biomass N was RC (87 kg N ha-1)< WCCC (136 kg N ha-1)< HV (184 kg N ha-1). In 2019, November cover crop biomass N followed a different pattern with RC (85 kg N ha-1)< WCCC (175 kg N ha-1) = HV (182 kg N ha-1).
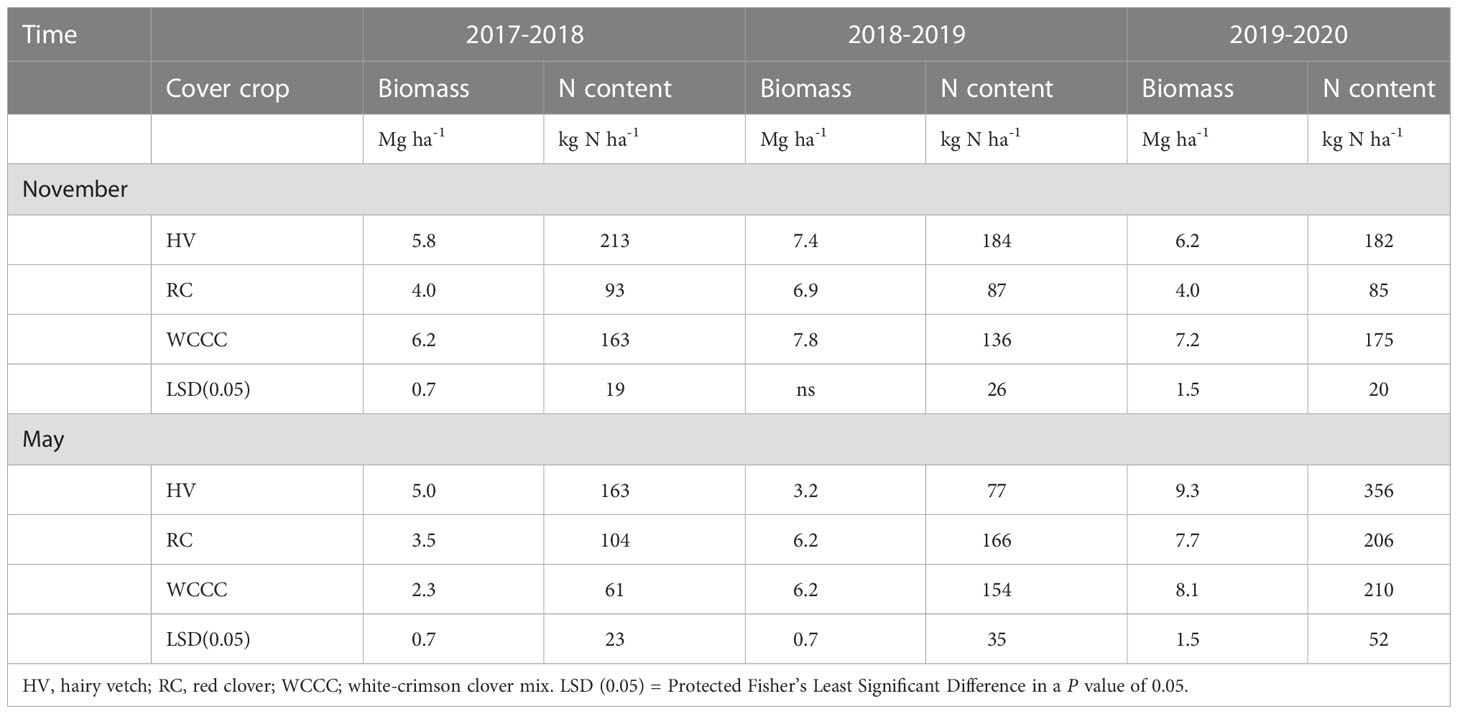
Table 2 Above-ground crop biomass and biomass nitrogen (N) in cover crop (HV, RC, WCCC) in November 2017, 2018 and 2019 shortly before cover crop termination by moldboard plow-down (CT treatment), and in May 2018, 2019 and 2020 shortly before cover crop termination by herbicide spray-down (NT and ST treatments).
Spring re-growth had a large impact on accumulated cover crop biomass and biomass N in the following May before herbicide spray-down. In May 2018, accumulated biomass and biomass N was HV > RC > WCCC; in May 2020, biomass N was HV > RC = WCCC, while accumulated biomass was HV > RC only; and in May 2019 HV had lower accumulated biomass and biomass N than both RC and WCCC. Over three years, HV had the greatest and RC had the lowest accumulated biomass and biomass N at freeze-up in late November; however, these differences always disappeared by early May of the following year. It was also found that WCCC and HV exhibited appreciable over-winter die-off in 2018 (HV and WCCC) and 2019 (HV), which was likely caused by cold air temperatures during December 2017 to January 2018, and during January to February 2019 (Table S1).
3.3 Impacts of cover crop species and termination-tillage method on corn stand
Corn planting date varied with spring weather, and a substantial planting delay occurred in 2019 due to wet soil conditions (Table 1). Corn emergence in CT was fast and even for all cover crops. In NT and ST, corn emerged later, and it emerged slower for RC and WCCC than for HV, especially under NT where above-ground cover crop biomass remained on the soil surface after termination by spring herbicide spray-down (Figure S2). Corn stands at 14 days after planting varied among year, season and cover crop (Figure 2). Averaged over cover crop and year, CT produced more uniform and greater corn stands (73,960 plants ha-1) than ST (69,030 plants ha-1) and NT (56,870 plants ha-1). In NT, 3-yr average corn stands followed the pattern: CK (72,450 plants ha-1) > HV (60,700 plants ha-1) > WCCC (52,220 plants ha-1) > RC (42,100 plants ha-1); and in ST, 3-yr average corn stands followed the trend: CK (73,270 plants ha-1) > WCCC (68,900 plants ha-1) = RC (68,350 plants ha-1) = HV (65,620 plants ha-1). Corn stands were not significantly different, however, among the CT treatments (72,730 - 75,190 plants ha-1). Compared to CT, overall average corn stand was 23% lower in NT and 6% lower in ST. In 2020, RC-NT had the lowest average corn stands (18860 plants ha-1), which was 24% of the average corn stands in the corresponding no cover crop control (CK-NT). This indicates that cover crop species and termination-tillage method significantly impact both corn emergence and seedling establishment; i.e. no difference between cover crops and control for CT, but significant reduction in corn emergence and plant stands between cover crops and control for NT and ST.
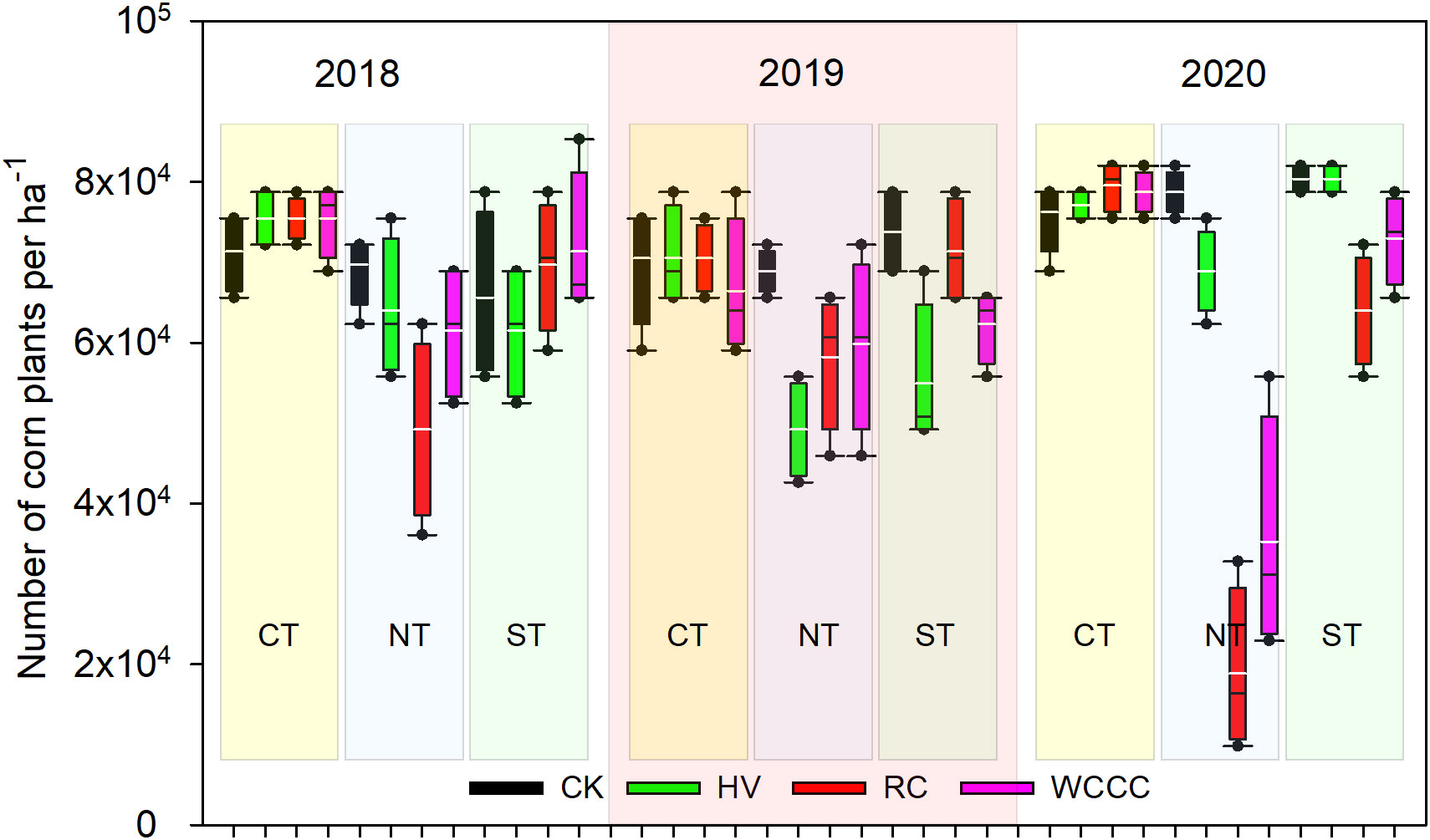
Figure 2 Corn stands (plant ha−1, two weeks after planting) by year from 2018 to 2020 grouped by cover crop species and cover crop termination-tillage method. CT, fall moldboard plow-down termination followed by spring planting; NT, spring herbicide spray-down termination followed by no-till corn planting; ST, spring herbicide spray-down termination followed by strip tilling and corn planting; CK, no cover crop control; HV, hairy vetch; RC, red clover; WCCC, white and crimson clover mix. Boxes indicate the 25th and 75th percentile; solid dots above and below the box indicate the 90th and 10th percentile, respectively; white horizontal lines indicate mean; black horizontal lines indicate median.
3.4 Effects of cover crop species and termination-tillage method on available soil N and corn N status
There were no cover crop or tillage-termination method interaction effects for plant available soil N, so only main effects are reported (Table 3). The no cover crop controls (CK-CT, CK-NT, CK-ST) contained significantly more soil -N and total soil mineral N (+ ) than the cover crop treatments in all three years; and in 2020, HV contained significantly more soil -N than RC. Significantly more soil -N was also found under NT and ST (60.0 and 64.7 mg N ha-1, respectively) relative to CT (44.8 mg N ha-1) in 2020 (data not reported), which likely reflects greater biomass N in 2020 than in the previous two years (Table 2). Although there was more mineral N under the controls due to the higher N application rate (200 kg N ha-1) than under the cover crops (100 kg N ha-1), N release and availability occurs throughout the growing season in the cover crop treatments, as evidenced by the plant chlorophyll SPAD readings described below.
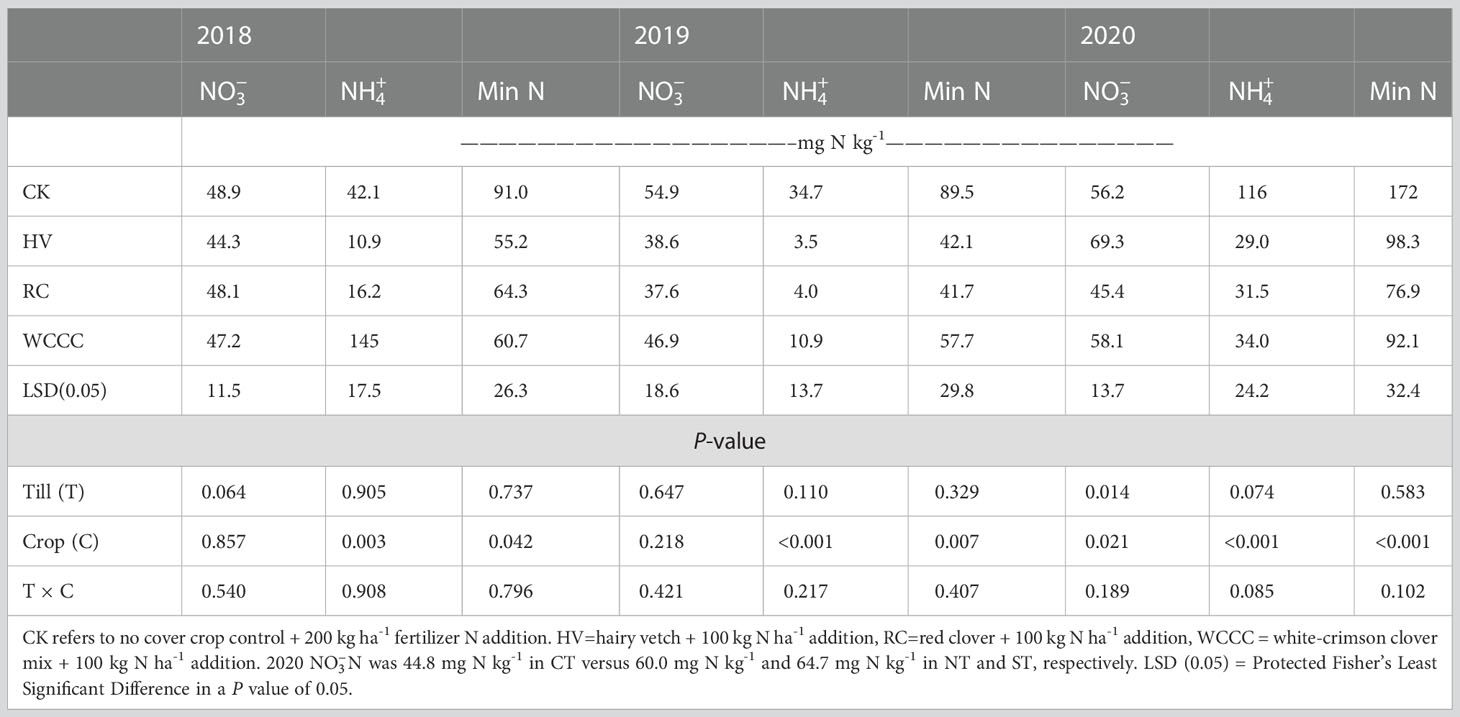
Table 3 Effects of cover crop species and termination-tillage method on soil mineral nitrogen concentrations in the 0-150 mm depth range at one month after corn planting each year.
There were also no tillage-termination method or cover crop interaction effects for corn plant chlorophyll levels (R1 growth stage) (Table 4), while the main effects of tillage-termination method were significant in 2018 and 2020, and cover crop significantly affected chlorophyll levels in 2019 and 2020. The SPAD readings were greater for CT (55.5) than ST (53.6) in 2018, and also greater for CK (51.8) than WCCC (47.9) in 2019. In 2020, SPAD readings were greater for CT (57.4) than ST (55.3), and they were also greater for CK (58.8) than for the cover crops (55.1-55.8). The SPAD readings were greater in 2018 and 2020 than in 2019.
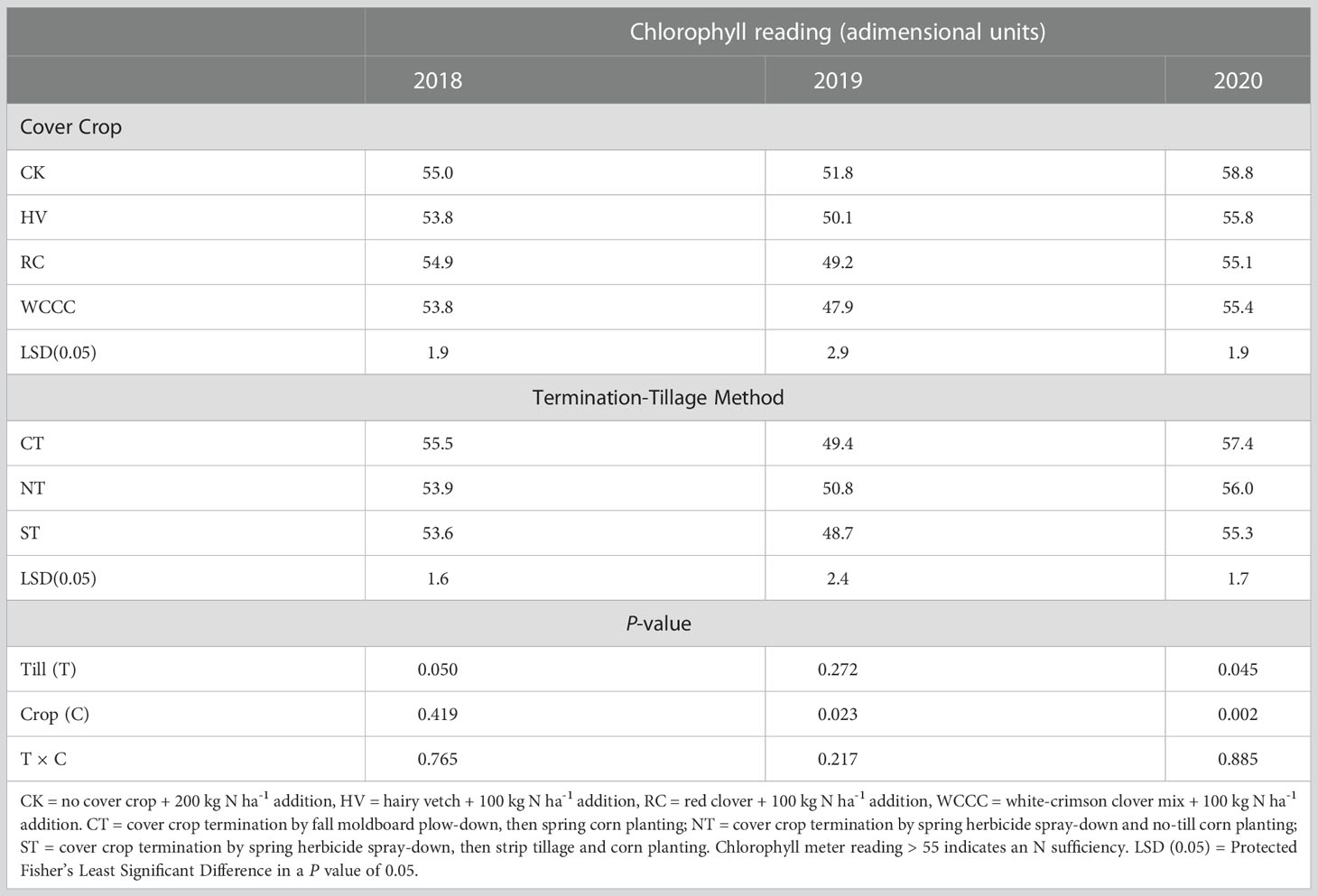
Table 4 SPAD-502 chlorophyll meter readings for corn at the R1 growth stage with respect to cover crop species and termination-tillage method in 2018, 2019 and 2020.
3.5 Effects of cover crop species, termination-tillage method, and nitrogen addition on corn grain yields
Cover crop species, termination-tillage method and their interactions significantly impacted corn grain yields (Table 5). Corn grain yields from the no cover crop controls were not different among tillage-termination method in all three years, whereas grain yields in the cover crop treatments were often affected by an interaction of cover crop and tillage-termination method. Corn yields were CT > NT = ST for hairy vetch in 2018 and 2019, CT > ST > NT for red clover in 2018 and CT > NT for red clover in 2019, and CT > ST > NT for white-crimson clover mix in 2018 and 2019. In 2020, corn yields were ST > CT for hairy vetch, CT = ST > NT for red clover, and CT > NT for white-crimson clover mix. HV had similar corn yields as other cover crops under CT and ST but HV had greater yields than RC and WCCC under NT in 2018. I n 2019, corn yields were CK > RC for fall plow-down, CK > HV = RC > WCCC for spring no-tillage, and CK > RC > WCCC for spring strip tillage. And in 2020, corn grain yields were CK > cover crops for fall plow-down, CK = HV > WCCC > RC for spring no-tillage, and CK = HV > RC = WCCC for spring strip tillage.
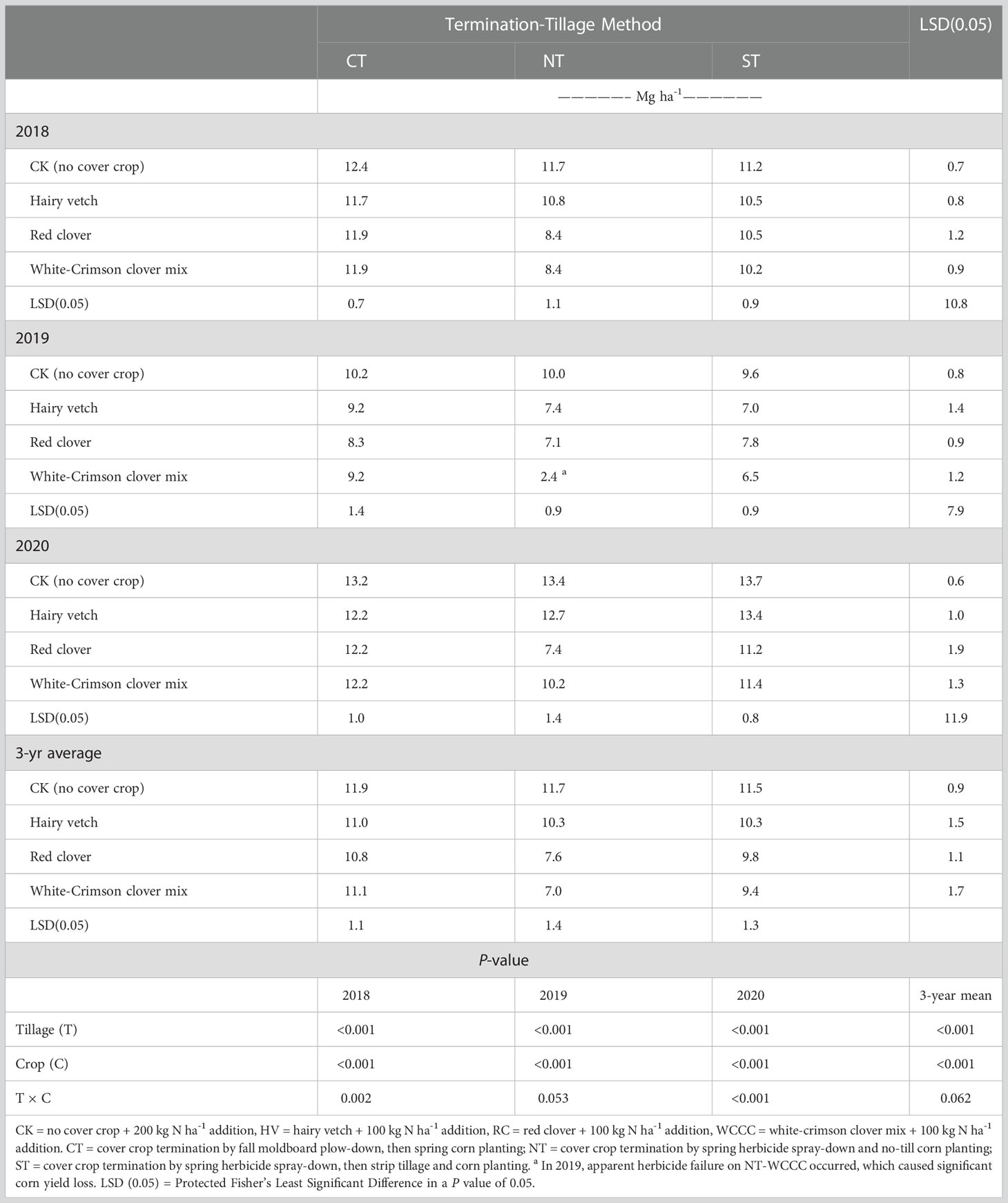
Table 5 Corn grain yields (15.5% seed moisture content) for cover crop species and termination-tillage method (2018-2020).
Year-to-year variation in corn grain yields reflected changes in weather and cover crop biomass (Tables S1, 2). While 2018 and 2020 received even and timely precipitation, corn planting was delayed substantially in 2019 (June 24, 2019), relative to the other two years (May 31 2018 and June 11 2020), as 2019 had the wettest spring in several decades for southwestern Ontario. Averaged over three years and termination-tillage method, corn grain yield was greatest for CK (11.7 Mg ha−1), then corn after HV (10.5 Mg ha−1), then corn after RC (9.4 Mg ha-1), and then corn after WCCC (9.2 Mg ha-1) (Table 5). Averaged over three years and cover crop species, corn grain yields were 11.2 Mg ha−1 for CT > 10.3 Mg ha−1 for ST > 9.2 Mg ha-1 for NT (Table 5). Hence, cover crop termination by fall moldboard plowing generated the largest corn grain yield, and spring ST was better than NT. The 2020 corn grain yield in HV-ST (13.4 Mg ha-1) was effectively equivalent to CK-ST (13.7 Mg ha-1). The poorest corn yield occurred under WCCC-NT in 2019 (2.4 Mg ha-1, Table 5), which were attributed to inadequate spring herbicide termination of white clover in that year.
3.6 Apparent fertilizer N replacement value, FNRVA
There was a significant interaction between crop and tillage-termination method for FNRVA in each year, and FNRVA varied widely from 35 to 92 kg N ha-1 in 2018, from 28 to 81 kg N ha-1 in 2019, and from 12 to 103 kg N ha-1 in 2020 (Table 6). In 2018, FNRVA was similar among cover crops for CT and ST, but FNRVA was greater for HV than RC and WCCC under NT. In 2019, FNRVA was similar among cover crop species for CT and NT, however, FNRVA was greater for RC than WCCC under ST. In 2020, FNRVA was similar among cover crop species for CT, but FNRVA was greater for HV than RC and WCCC under NT and ST. Year 2019 produced the smallest mean FNRVA (53 kg N ha-1) compared to 2018 (69 kg N ha-1) and 2020 (73 kg N ha-1). The small N credit in 2019 was likely caused by low grain yields in that year due to late planting, poor corn stands and inadequate herbicide termination of white clover (Table 5; Figure 2). High FNRVA occurred for HV in 2020 under all three termination-tillage treatments (85 – 103 kg N ha-1); however, the FNRVA values were lower for RC and WCCC under NT and ST, ranging from 12 to 73 kg N ha-1. The three-year average FNRVA was greatest for CT (83 kg N ha-1), followed by ST (63 kg N ha-1), and then NT (48 kg N ha-1); and it was greatest for HV (75 kg N ha-1), followed by WCCC (62 kg N ha-1), and then RC (58 kg N ha-1).
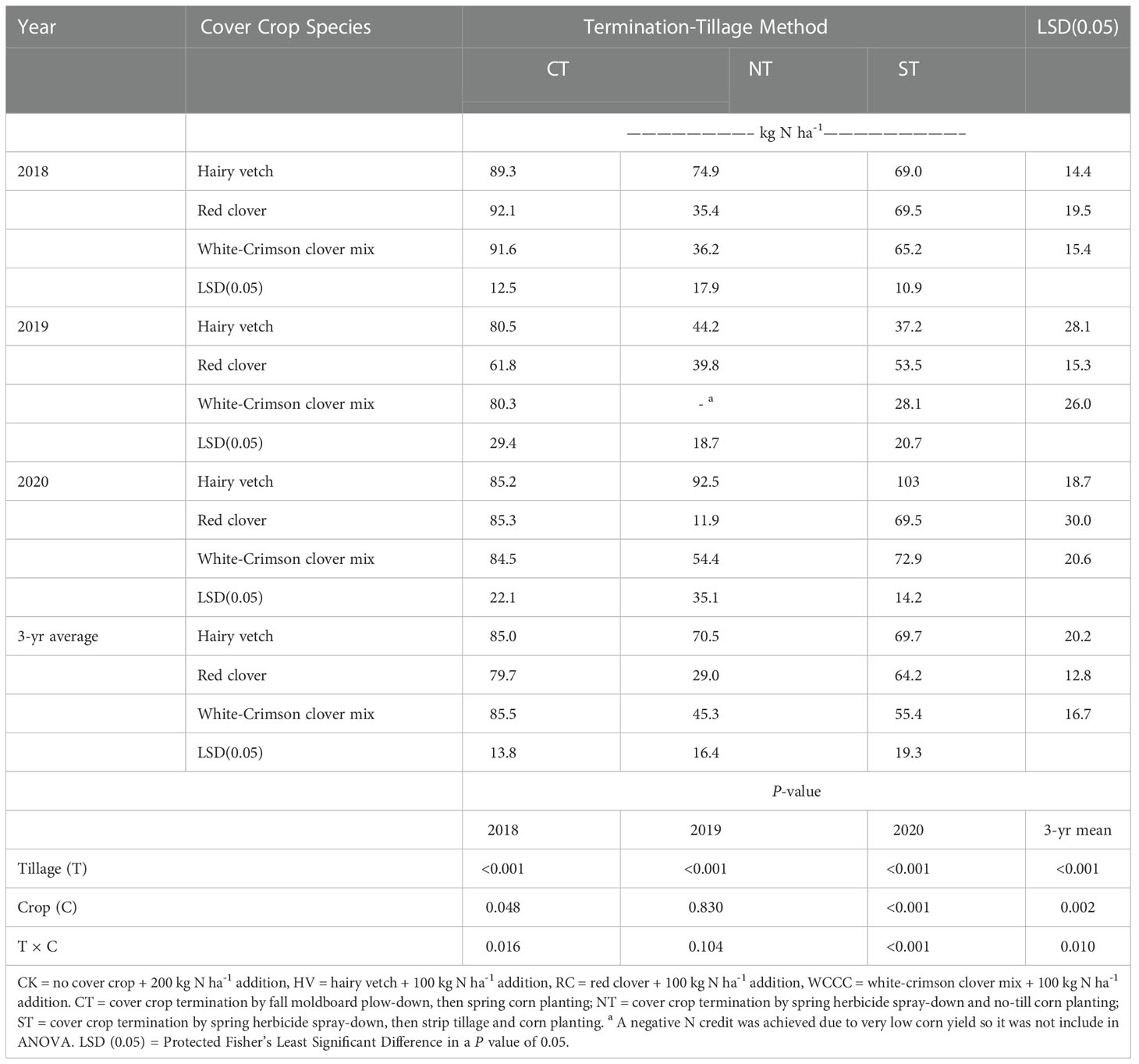
Table 6 Apparent fertilizer nitrogen replacement value, FNRVA (Eq. 1), to corn for different cover crop species and termination-tillage methods.
4 Discussion
Total global N fixation is on the order of 1.75 x 1011 kg N yr-1, with about half (i.e. ≈ 8 x 1010 kg N yr-1 or 20-200 kg N ha-1 yr-1) fixed biologically by leguminous plants, and the rest fixed by industrial activity including fertilizer production (46). Although farmers still predominantly use synthetically-produced N fertilizer, biologically fixed N from legume crops is being increasingly recognized as a viable N source for crop production. The ability of legume plants to fix N from the atmosphere varies with plant species, growth stage and dry matter content of plant biomass (47).
In this study, legume C:N ratios ranged from 12.3 to 16.8, which are slightly higher than those for clovers in a study conducted ~90 km northeast of our study site (9.4 to 14.2, 24), but comparable with the C:N ratios obtained for clovers (13.8 to 15.6) and HV (13.2 to 13.9) in a study conducted on a sandy loam soil about 30 km south of our study site (35). The variability in C:N ratios by crop species could be the result of differences in both development and relative plant component composition (i.e. leaves vs. stems vs. roots) at the time of sampling. Legumes with a lower C:N ratio will decompose faster and release N more quickly than legumes with higher C:N ratios (17). Therefore, terminated HV will usually release biomass N somewhat faster than terminated RC and WCCC. The amount of accumulated legume biomass can also be affected by sampling time, particularly during April and May when plant growth rate increases dramatically and often differs substantially among plant species (48). In this study, greater accumulated biomass and biomass N in 2020 is likely due to the fact that biomass sample collection in that year occurred 3 weeks later than in 2018, and one week later than in 2019 (Table 1). For example, two weeks additional growth between late April and early May increased HV biomass by 61% in North Carolina (13) and by 35% in Maryland (23).
Red clover is a long-established cover crop in southwestern Ontario, but summer-seeding into winter wheat stubble is extremely rare compared to traditional frost-seeding (e.g. 49, 50). Coombs et al. (24) found less RC biomass accumulation for late May to mid-July planting relative to mid-March to mid-April planting, which they attributed to length of available growing period. Yang et al. (35) found less biomass and biomass N by freeze-up for summer-seeded RC than HV or crimson clover. The spring and fall biomass for RC in this study (5.8 and 5.0 Mg ha-1, respectively) were in the range found for an organic crop production trial on a sandy loam about 30 km south (4.1 and 6.8 Mg ha-1, respectively; 35), but were greater than the amounts found for another sandy loam about 90 km northeast (0.7 and 3.1 Mg ha-1, respectively; 24), and also greater than “representative” values (0.7 and 4.2 Mg ha-1) reported for temperate climates (10, 51, 52). Coombs et al. (24) noted that when RC was allowed to over-winter, biomass increased relative to fall biomass for Ontario (49, 51, 53), Ohio and Michigan (45, 54). Over-winter increases in RC biomass occurred in this study, with an average fall biomass of 5.0 Mg ha-1 increasing to 5.9 Mg ha-1 by the following spring.
Hairy vetch and crimson clover are popular N sources for grain corn in the US (e.g. 11, 55). Hairy vetch is also used in Ontario because of its cold tolerance (35, 56, 57) whereas crimson clover is rarely used. The nitrogen content in HV above-ground biomass ranges from 75 kg N ha-1 to 288 kg N ha-1 at various sites in Alabama, Georgia, Kentucky, and North Carolina (11, 56, 58). Van Eerd (57) obtained relatively low biomass N (41 – 139 kg N ha-1) by freeze-up for HV planted in early August in southern Ontario, as well as little re-growth in the following spring. This differs from our results, and may reflect our milder climate and/or soil differences. This study showed that HV and crimson clover performed well for accumulating above-ground biomass and biomass N in both the fall and the following spring.
Corn leaf chlorophyll level has been used for determining plant N status when a reference N level is available and all factors other than N are constant (59). SPAD-502 chlorophyll readings from the well-fertilized controls (200 kg N ha-1) were used as the reference N level (60–63), and N deficiency in the cover crop treatments was deemed to occur when their SPAD readings fell below 95% of the reference. This occurred for WCCC in 2019 and for RC and WCCC in 2020. If, however, the 93% critical SPAD level of Piekielek et al. (61) is used, then none of the treatments were N deficient. The SPAD readings closely followed measured cover crop biomass N (Table 2), but showed no clear impacts on corn grain yields (Table 5). The SPAD readings at corn R1 growth stage were inconsistent with soil mineral N measured one month after corn planting (Table 3), indicating sustained release of N from legume decomposition (58, 64–66). The poor match between soil N or SPAD readings and corn yields indicates that soil N may not be a limiting factor for corn yield in this study. It appears instead that the primary factor affecting corn grain yields was corn stand number, which showed a highly significant positive correlation with corn grain yields (Figure S3).
Although results were not consistent among cover crops and years, this study never-the-less supports the general consensus that summer-seeding legume cover crops after winter wheat harvest can contribute substantial N to a succeeding corn crop in southwestern Ontario (35, 49, 57) and in the northern United States (58, 67, 68). The FNRVA in this study were comparable to the 65 to 130 kg N ha-1 from legume cover crops found in a Maryland study (69). The highest FNRVA (100 kg N ha-1) in our study is also equivalent to that obtained in an Ontario study where oat inter-seeded with red clover provided N to a following corn crop (70). In Ohio, Ngalla and Eckert (71) compared frost-seeding red clover into winter wheat with July seeding of red clover after wheat harvest. They found that frost-seeded red clover contributed 56 to 67 kg N ha-1 to the following corn, but summer-seeded red clover provided effectively no N benefit because of insufficient biomass accumulation.
Partial replacement of synthetic fertilizer N with legume cover crop N often caused a substantial grain yield penalty for no-tillage, especially for RC-NT and WCCC-NT. This occurred because surface mulches, such as cover crop residues, often delay dry-down and warming of Brookston clay loam soil in southern Ontario, and this in turn reduces corn germination and early growth. Hence, RC and WCCC cover crops are not recommended for fine-textured soils under NT in southern Ontario. On the other hand, NT after HV often produced acceptable plant stands, and the higher N benefit from HV contributed to acceptable corn yields. Higher N contribution from HV was also found in Brazil, and was deemed a viable strategy for boosting corn yield under NT (72). Vaughan et al. (73) found that combining HV with NT increased the yield potential of both monoculture and rotation corn in North Carolina.
In summary, the legume cover crops, hairy vetch, red clover, crimson clover and white-crimson clover mix, could all enhance the traditional soybean – winter wheat – corn rotation in southwestern Ontario when the cover crops are summer-seeded after wheat harvest. Hairy vetch was superior to the other cover crops, however, as it generated larger amounts of biomass and accumulated greater amounts of biomass nitrogen. Further, hairy vetch is easily terminated in the fall or spring, it allows good corn emergence, and it can provide the equivalent of 85 kg N ha-1 of fertilizer to the corn crop. Averaged over three years and three termination-tillage systems (i.e. fall moldboard plow-down with conventional tillage, spring herbicide spray-down with strip-tillage, spring herbicide spray-down with no-tillage), corn yields following hairy vetch were 13% greater than corn yields following red clover and 15% greater than corn yields following a white-crimson clover mix. If fall termination of the cover crop is desired, summer-seeding hairy vetch or white and crimson clovers are recommended for fine-textured soils in southwestern Ontario because they accumulate more biomass relative to red clover. If strong spring re-growth is desired, hairy vetch is more desirable than red, white and crimson clovers because clovers tend to be more difficult to terminate with herbicides in the spring, and they can reduce grain yields by delaying corn emergence and reducing final corn stands.
Data availability statement
The original contributions presented in the study are included in the article/Supplementary Material. Further inquiries can be directed to the corresponding author.
Author contributions
XY and WR conceptualized the work; CD managed soil and crop N analysis; M-AR collected and analysed the soil and plant samples. XY planned the experiment and wrote the first draft. All authors contributed to manuscript editing and approved the final submission.
Funding
This was a collaborative project between the Grain Farmers of Ontario (c2017ag24) and the Industry-lead Research and Development Program of Agriculture and Agri-Food Canada (AAFC, Organic Science Cluster3). Both the Grain Farmers of Ontario and AAFC provided funds for the study.
Acknowledgments
Technical assistance by the HRDC Farm Crew, Dr. T. Oloya, and several summer and co-op students is gratefully acknowledged.
Conflict of interest
The authors declare that the research was conducted in the absence of any commercial or financial relationships that could be construed as a potential conflict of interest.
Publisher’s note
All claims expressed in this article are solely those of the authors and do not necessarily represent those of their affiliated organizations, or those of the publisher, the editors and the reviewers. Any product that may be evaluated in this article, or claim that may be made by its manufacturer, is not guaranteed or endorsed by the publisher.
Supplementary material
The Supplementary Material for this article can be found online at: https://www.frontiersin.org/articles/10.3389/fsoil.2022.1082940/full#supplementary-material
References
1. Baldock JO, Higgs RL, Paulson WH, Jackobs JA, Shrader WD. Legume and mineral n effects on crop yields in several crop sequences in the upper Mississippi valley. Agron J (1981) 73:885–90. doi: 10.2134/agronj1981.00021962007300050031x
2. Torbert HA, Reeves DW, Mulvaney RL. Winter legume cover crop benefits to corn: Rotation vs. fixed-nitrogen effects. Agron J (1996) 88:527–35. doi: 10.2134/agronj1996.00021962008800040005x
3. Sharma P, Singh A, Kahlon C, Brar A, Grover K, Dia M, et al. The role of cover crops towards sustainable soil health and agriculture–a review paper. Am J @ Plant Sci (2018) 9:1935–51. doi: 10.4236/ajps.2018.99140
4. Mitchell WH, Teel MR. Winter-annual cover crops for no-tillage com production. Agron J (1977) 69:569–73. doi: 10.2134/agronj1977.00021962006900040011x
5. Ebelhar SA, Frye WW, Blevins RL. Nitrogen from legume cover crops for no-tillage corn. Agron J (1984) 76:51–5. doi: 10.2134/agronj1984.00021962007600010014x
6. Blevins RL, Herbek JH, Frye WW. Legume cover crops as a nitrogen source for no-till corn and grain sorghum. J Prod Agric (1992) 4:62–7. doi: 10.2134/agronj1990.00021962008200040023x
7. Ott SL, Hargrove WL. Profits and risks of using crimson clover and hairy vetch cover crops in no-till corn production. Am J Altern. Agric (1989) 4:65–70. doi: 10.1017/S0889189300002745
8. Pott LP, Amado TJC, Schwalbert RA, Gebert FH, Reimche GB, Pes LZ, et al. Effect of hairy vetch cover crop on maize nitrogen supply and productivity at varying yield environments in southern Brazil. Sci Total Environ (2021) 759:144313. doi: 10.1016/j.scitotenv.2020.144313
9. Ranells NN, Wagger MG. Nitrogen release from grass and legume cover crop monocultures and bicultures. Agron J (1996) 88:777–82. doi: 10.2134/agronj1996.00021962008800050015x
10. Snapp SS, Swinton SM, Labarta R, Mutch D, Black JR, Leep R, et al. Evaluating cover crops for benefits, costs and performance within cropping system niches. Agron J (2005) 97:322–32. doi: 10.2134/agronj2005.0322a
11. Parr M, Grossman JM, Reberg-Horton SC, Brinton C, Crozier C. Nitrogen delivery from legume cover crops in no-till organic corn production. Agron J (2011) 103:1578–90. doi: 10.2134/agronj2011.0007
12. Kaspar TC, Jaynes DB, Parkin TB, Moorman TB, Singer JW. Effectiveness of oat and rye cover crops in reducing nitrate losses in drainage water. agric. Water Manage (2012) 110:25–33. doi: 10.1016/j.agwat.2012.03.010
13. Wagger MG. Time of desiccation effects on plant composition and subsequent nitrogen release from several winter annual cover crops. Agron J (1989) 81:236–241. doi: 10.2134/agronj1989.00021962008100020020x
14. Radicetti E, Mincinelli R, Moscetti R, Campiglia E. Management of winter cover crop residue under different tillage conditions affects nitrogen utilization efficiency and yield of eggplant (Solanum melanogena l.) in Mediterranean environment. Soil Till. Res (2016) 155:329–38. doi: 10.1016/j.still.2015.09.004
15. Ewing RP, Wagger MG, Denton HP. Tillage and cover crop management effects on soil water and corn yield. Soil Sci Soc Am J (1991) 55:1081–5. doi: 10.2136/sssaj1991.03615995005500040031x
16. Reberg-Horton SC, Grossman JM, Kornecki TS, Meijer AD, Price AJ, Place GT, et al. Utilizing cover crop mulches to reduce tillage in organic systems in the southeastern USA. renew. Agric Food Syst (2012) 27:41–8. doi: 10.1017/S1742170511000469
17. Yang XM, Drury CF, Reynolds WD, Phillips LA. Nitrogen release from shoots and roots of crimson clover, hairy vetch, and red clover. Can J Soil Sci (2020) 100:179–88. doi: 10.1139/cjss-2019-0164
18. Appelgate SR, Lenssen AW, Wiedenhoeft MH, Kaspar TC. Cover crop options and mixes for upper Midwest corn-soybean systems. Agron J (2017) 109:968–84. doi: 10.2134/agronj2016.08.0453
19. Baraibar B, Hunter MC, Schipanski ME, Hamilton A, Mortensen DA. Weed suppression in cover crop monocultures and mixtures. Weed Sci (2018) 66:121–33. doi: 10.1017/wsc.2017.59
20. Finney DM, White CM, Kaye JP. Biomass production and carbon/nitrogen ratio influence ecosystem services from cover crop mixtures. Agron J (2016) 108:39–52. doi: 10.2134/agronj15.0182
21. Sainju UM, Whitehead WF, Singh BP. Biculture legume-cereal cover crops for enhanced biomass yield and carbon and nitrogen. Agron J (2005) 97:1403–12. doi: 10.2134/agronj2004.0274
22. Antosh E, Idowu J, Schutte B, Lehnhoff E. Winter cover crops effects on soil properties and sweet corn yield in semi-arid irrigated systems. Agron J (2020) 112:92–106. doi: 10.1002/agj2.20055
23. Clark AJ, Decker AM, Meisinger JJ, Mulford FR, McIntosh MS. Hairy vetch kill date effects on soil water and corn production. Agron J (1995) 87:579–85. doi: 10.2134/agronj1995.00021962008700030031x
24. Coombs C, Lauzon JD, Deen B, Van Eerd LL. Legume cover crop management on nitrogen dynamics and yield in grain corn systems. Field Crops Res (2017) 201:75–85. doi: 10.1016/j.fcr.2016.11.001
25. Sainju UM, Singh BP. Tillage, cover crop, and kill-planting date effects on corn yield and soil nitrogen. Agron J (2001) 93:878–86. doi: 10.2134/agronj2001.934878x
26. Quemada M, Cabrera ML, McCracken DV. Nitrogen released from surface-applied cover crop residues: evaluating CERES-n. Agron J (1997) 89:723–9. doi: 10.2134/agronj1997.00021962008900050003x
27. Wayman S, Cogger C, Benedict C, Burke I, Collins D, Bary A. The influence of cover crop variety, termination timing and termination method on mulch, weed cover and soil nitrate in reduced-tillage organic systems. Renewable Agric Food Syst (2015) 30:450–60. doi: 10.1017/S1742170514000246
28. Alonso-Ayuso M, Gabriel JL, Hontoria C, Ibáñez MÁ., Quemada M. The cover crop termination choice to designing sustainable cropping systems. Euro. J Agron (2020) 114:126000. doi: 10.1016/j.eja.2020.126000
29. Smith SJ, Sharpley AN. Soil nitrogen mineralization in the presence of surface and incorporated crop residues. Agron J (1990) 82:112–6. doi: 10.2134/agronj1990.00021962008200010025x
30. Creamer N, Dabney S. Killing cover crops mechanically: Review of recent literature and assessment of new research results. Am J Altern. Agric (2002) 17:32–40. doi: 10.1079/AJAA20014
31. Davis AS. Cover-crop roller–crimper contributes to weed management in no-till soybean. Weed Sci (2010) 58:300–9. doi: 10.1614/WS-D-09-00040.1
32. Navarro-Miró D, Blanco-Moreno JM, Ciacci C, Chamorro L, Testani E, Kristensen HL, et al. Agroecological service crops managed with roller crimper reduce weed density and weed species richness in organic vegetable systems across Europe. Agron Sustain Dev (2019) 39:55. doi: 10.1007/s13593-019-0597-8
33. Drury CF, Tan CS, Welacky TW, Oloya TO, Hamill AS, Weaver SE. Red clover and tillage influence on soil temperature, water content, and corn emergence. Agron J (1999) 91:101–8. doi: 10.2134/agronj1999.00021962009100010016x
34. Yang XM, Reynolds WD, Drury CF, Reeb MD. Cover crop effects on soil temperature in a clay loam soil in southwestern Ontario. Can J Soil Sci (2021) 101:761–70. doi: 10.1139/cjss-2021-0070
35. Yang XM, Drury CF, Reynolds WD, Reeb MD. Legume cover crops provide nitrogen to corn during a three-year transition to organic cropping. Agron J (2019) 111:3253–64. doi: 10.2134/agronj2018.10.0652
36. Bourgeois B, Charles A, Van Eerd LL, Tremblay N, Lynch DH, Bourgeois G, et al. Interactive effects between cover crop management and the environment modulate benefits to cash crop yields: a meta-analysis. Can J Plant Sci (2022) 102:656–78. doi: 10.1139/CJPS-2021-0177
37. Ontario Ministry of Agriculture, Food, and Rural Affairs (OMAFRA). Agronomy guide for field crops Vol. 812. Brown C, editor. Toronto, Canada: Queen’s Printer for Ontario (2016).
38. Blaser BC, Gibson LR, Singer JW, Jannink JL. Optimizing seeding rates for winter cereal grains and frost-seeded red clover intercrops. Agron J (2006) 98:1041–9. doi: 10.2134/agronj2005.0340
39. Loucks CES. Investigating red clover (Trifolium pratense l.) stand survival and uniformity when under-seeded to winter wheat (Triticum aestivum l. em. thell) under low soil moisture. Guelph, ON, Canada: University of Guleph (2017). Available at: https://atrium.lib.uoguelph.ca/xmlui/handle/10214/10161.
40. Soil Classification Working Group. The Canadian system of soil classification. publ. 1646. agriculture and agri-food Canada. Ottawa, ON, Canada: NRC Research Press (1998).
41. Kroetsch D, Wang C. Particle size distribution. In: Carter MR, Gregorich EG, editors. Soil sampling and methods of analysis, 2nd Ed. Boca Raton, FL: CRC Press (2008) 713–25.
42. Schoenau JJ, O’Halloran IP, CRC Press. Sodium bicarbonate-extractable phosphorus. In: Carter MR, Gregorich EG, editors. Soil sampling and methods of analysis. 2nd ed. Canadian society of soil science. Boca Raton, FL: CRC Press and Taylor & Francis Group, (2008) 89–94.
43. Hendershot WH, Lalande H, Duquette M. Ion exchange and exchangeable cations. In: Carter MR, Gregorich EG, editors. Soil sampling and methods of analysis. 2nd ed. Canadian society of soil science. Boca Raton, FL: CRC Press, (2008). p. 203–4.
44. Tel DA, Heseltine C. The analyses of KCL soil extracts for nitrate, nitrite and ammonium using a TRAACS 800 analyzer. commun. Soil Sci Plant Analy. (1990) 21:1681–8. doi: 10.1080/00103629009368331
45. Gentry LE, Snapp SS, Price RF, Gentry LF. Apparent red clover nitrogen credit to corn: evaluating cover crop introduction. Agron J (2013) 105:1658–64. doi: 10.2134/agronj2013.0089
46. Hillel D. Soil biodiversity. In: Hillel D, editor. Soil in the environment. San Diego, CA: Academic Press (2008) 163–74.
47. Liu YY, Wu LH, Baddeley JA, Watson CA. Models of biological nitrogen fixation of legumes. a review. Agron Sustain Dev (2011) 31:155–72. doi: 10.1051/agro/2010008
48. Teasdale JR, Devine TE, Mosjidis JA, Bellinder RR, Beste CE. Growth and development of hairy vetch cultivars in the northeastern united states as influenced by planting and harvesting date. Agron J (2004) 96:1266–71. doi: 10.2134/agronj2004.1266
49. Vyn TJ, Faber JG, Janovicek KJ, Beauchamp EG. Cover crop effects on nitrogen availability to corn following wheat. Agron J (2000) 92:915–24. doi: 10.2134/agronj2000.925915x
50. Loucks CES, Deen W, Gaudin ACM, Earl HJ, Bowley RS, Martin RC. Genotypic differences in red clover (Trifolium pratense l.) response under severe water deficit. Plant Soil (2018) 425:401–14. doi: 10.1007/s11104-018-3594-9
51. Gaudin A, Westra S, Loucks C, Janovicek K, Martin R, Deen W. Improving resilience of northern field crop systems using inter-seeded red clover - a review. Agron (2013) 3:148–80. doi: 10.3390/agronomy3010148
52. Gaudin A, Janovicek K, Martin RC, Deen W. Approaches to optimizing nitrogen fertilization in a winter wheat–red clover (Trifolium pratense l.) relay cropping system. Field Crops Res (2014) 155:192–201. doi: 10.1016/j.fcr.2013.09.005
53. Thilakarathna MS, Serran. S, Lauzon. J, Janovicek. K, Deen. B. Management of manure nitrogen using cover crops. Agron J (2015) 107:1595–607. doi: 10.2134/agronj14.0634
54. Henry DC, Mullen RW, Dygert CE, Diedrick KA, Sundermeier A. Nitrogen contribution from red clover for corn following wheat in western Ohio. Agron J (2010) 102:210–5. doi: 10.2134/agronj2009.0187
55. Spargo JT, Cavigelli MA, Mirsky SB, Meisinger JJ, Ackroyd VJ. Organic supplemental nitrogen sources for field corn production after a hairy vetch cover crop. Agron J (2016) 108:1992–2002. doi: 10.2134/agronj2015.0485
56. Dabney SM, Delgado JA, Meisinger JJ, Schomberg HH, Liebig MA, Kaspar T, et al. Chapter 9: Using cover crops and cropping systems for nitrogen management. In: Delgado JA, Follett RF, editors. Advances in nitrogen management for water quality. Ankeny, IA: Soil and Water Conservation Society, (2010). 230–81.
57. Van Eerd LL. Nitrogen dynamics and yields of fresh bean and sweet corn with different cover crops and planting dates. Nutr Cycl. Agroecosyst. (2018) 111:33–46. doi: 10.1007/s10705-018-9914-x
58. Liebman AM, Grossman J, Brown M, Wells MS, Reberg-Horton SS, Shi W. Legume cover crops and tillage impact nitrogen dynamics in organic corn production. Agron J (2018) 110:1046–57. doi: 10.2134/agronj2017.08.0474
59. Hawkins JA, Sawyer JE, Barker DW, Lundvall JP. Using relative chlorophyll meter values to determine nitrogen application rates for corn. Agron J (2007) 99:1034–40. doi: 10.2134/agronj2006.0309
60. Schepers JS. New diagnostic tools for tissue testing. Commun Soil Sci Plant Anal (1994) 25:817–26. doi: 10.1080/00103629409369082
61. Piekielek WP, Fox RH, Toth JD, Macneal KE. Use of a chlorophyll meter at the early dent stage of corn to evaluate nitrogen sufficiency. Agron J (1995) 87:403–8. doi: 10.2134/agronj1995.00021962008700030003x
62. Blackmer TM, Schepers JS. Techniques for monitoring crop nitrogen status in corn. commun. Soil Sci Plant Anal (1994) 25:1791–800. doi: 10.1080/00103629409369153
63. Varvel GE, Schepers JS, Francis DD. Ability for in-season correction of nitrogen deficiency in corn using chlorophyll meters. Soil Sci Soc Am J (1997) 61:1233–9. doi: 10.2136/sssaj1997.03615995006100040032x
64. Wilson DO, Hargrove WL. Release of nitrogen from crimson clover residue under two tillage systems. Soil Sci Soc Am J (1986) 50:1251–4. doi: 10.2136/sssaj1986.03615995005000050033x
65. Wagger MG, Cabrera ML, Ranells NN. Nitrogen and carbon cycling in relation to cover crop residue quality. J Soil Water Conserv (1998) 53:214–8. Available at: https://www.jswconline.org/content/jswc/53/3/214.full-text.pdf.
66. Cherr CM, Scholberg JMS, McSorley R. Green manure as nitrogen source for sweet corn in a warm-temperate environment. Agron J (2006) 98:1173–80. doi: 10.2134/agronj2005.0036
67. Griffin T, Liebman M, Jemison J. Cover crops for sweet corn production in a short-season environment. Agron J (2000) 92:144–51. doi: 10.2134/agronj2000.921144x
68. Tonitto C, David MB, Drink LE. Replacing bare fallows with covercrops in fertilizer-intensive cropping systems: A meta-analysis of crop yield and n dynamics. Agric Ecosys. Environ (2006) 112:58–72. doi: 10.1016/j.agee.2005.07.003
69. Holderbaum JF, Decker AM, Messinger JJ, Mulford FR, Vough LR. Fall-seeded legume cover crops for no-tillage corn in the humid East. Agron J (1990) 82:117–24. doi: 10.2134/agronj1990.00021962008200010026x
70. Bruulsema TW, Christie BR. Nitrogen contribution to succeeding corn from alfalfa and red clover. Agron J (1987) 79:96–100. doi: 10.2134/agronj1987.00021962007900010020x
71. Ngalla CF, Eckert DJ. Wheat-red clover interseeding as a nitrogen source for corn. 47– 48. In: Power JF, editor. The role of legumes in conservation tillage systems. proc. soil conserv. Soc. am., Athens. Ankeny, IA: Soil Water Conserv. Soc. Am. (1987) 27–9.
72. Pes LZ, Amado TJC, Gebert FH, Schwalbert RA, Pott LP. Hairy vetch role to mitigate crop yield gap in different yield environments at field level. Sci. Agricola (2022) 79:e20200327. doi: 10.1590/1678-992x-2020-0327
Keywords: cover crop, legumes, corn N credit, rotation, termination-tillage
Citation: Yang X, Reynolds WD, Drury CF and Reeb M-A (2023) Impacts of summer-seeded legume cover crops and termination-tillage method on nitrogen availability to corn in rotation. Front. Soil Sci. 2:1082940. doi: 10.3389/fsoil.2022.1082940
Received: 28 October 2022; Accepted: 19 December 2022;
Published: 10 January 2023.
Edited by:
Walter Daniel Carciochi, National University of Mar del Plata, ArgentinaReviewed by:
María Alonso-Ayuso, Aula Dei Experimental Station (CSIC), SpainLeonardo Novelli, Instituto Nacional de Tecnología Agropecuaria, Argentina
Copyright © 2023 Yang, Reynolds, Drury and Reeb. This is an open-access article distributed under the terms of the Creative Commons Attribution License (CC BY). The use, distribution or reproduction in other forums is permitted, provided the original author(s) and the copyright owner(s) are credited and that the original publication in this journal is cited, in accordance with accepted academic practice. No use, distribution or reproduction is permitted which does not comply with these terms.
*Correspondence: Xueming Yang, xueming.yang@agr.gc.ca