- 1Ecology, Ecological Restoration and Landscape, Faculty of Agronomic Sciences, Université de Lubumbashi, Lubumbashi, Africa
- 2BIOSE—Biosystem Engineering Department, Water-Soil-Plant Unit, Gembloux Agro-Bio Tech, University of Liege, Gembloux, Belgium
- 3Plant Ecology and Biogeochemistry, Université Libre de Bruxelles, Brussels, Belgium
Among mine wastes, tailings are known to have the largest environmental impact, as they have high concentrations of trace elements and are susceptible to wind dispersal and water erosion. A tree plantation trial was installed at Kipushi tailing (DR Congo) in order to mitigate the contaminant dispersal in the surrounding areas. Fifteen years later, the present study was conducted for the purpose of investigating the macronutrient and metal content in amended holes and assessing the performance of tree species through root behavior in the tailings dams. Results show elevated available P, K, Ca, and Mg concentration in the surface and amended layers, which is higher than the unpolluted soil of the miombo woodland. Trace metals were manifold higher compared to the pedo-geochemical background of the region, with Cu and Co concentration tending to increase in the organic matter-rich layers, while Zn, Cd, Pb, and As remained higher in tailings. Compared to the tailing layer, roots grew well in the amended layers, but few roots ranging from very fine to big from all the surviving species were able to grow beyond the amended layers, indicating the possibility of tree survival on the tailings dams over many years. Acacia polyacantha and Psidium guajava are species that showed a higher quantity of roots in the unamended tailing layers. Leaves should be avoided for human or animal consumption, but as the concentration of Cu, Zn, and Co in guava was lower, there is no indication of hazards in case of their consumption. Therefore, the use of well-adapted tree species on the mix-up of the organic amendments with the uncontaminated topsoil seemed to be a good technique for the reclamation of larger polluted areas.
Introduction
Free of human interference, the soil trace element content is largely dependent on that of the soil parent material and acting weathering processes (1). An increasingly industrialized society has, however, led to the widespread introduction of trace metals and metalloids into our environment, causing acute and diffuse contamination of soil (1, 2). Among mine wastes, tailings are known to have the largest environmental impact, as they have the highest concentrations of trace elements and are the most susceptible to the wind dispersal and water erosion (3, 4), which are the main mechanisms for the expansion of metals from tailings. The effects of these pollutants can reach local and, in some cases, regional scales, affecting urban and agricultural areas (5). Consequently, these pollutants can pose a risk to human health if no appropriate method of contaminant disposal or rehabilitation has taken place (6–8). That is why the last few decades have seen the rise of phytoremediation technologies, which notably include contaminant extraction or stabilization and plant-based, generally termed “phytoextraction and phytostabilization” (9, 10). Phytostabilization is employed to treat trace-element polluted soils involving the establishment of a vegetation cap on tailings dams that prevents pollution spread by erosion, water percolation, leaching, and wind dispersal of the toxic dust in a cost-effective manner (11–13). However, the selection of appropriate plant species is crucial to achieving successful phytostabilization (14). The selected plants should develop an extensive root system and large biomass in the presence of high concentrations of trace elements while keeping the translocation of trace elements from roots to shoots as low as possible and thereby limiting the propagation of metals into the food chain (12, 15–17). The stabilization of pollutants can be an important remediation option for large areas with high and multi-elemental contamination (1). Many abandoned and post-operative tailings dams are barren with bare soil or have minimal to slow natural vegetative colonization (2, 16). Common physicochemical limitations to plant growth on tailings dams include extreme pH, high salt content, lack of required nutrients, metal toxicities, high bulk density, slow water infiltration, poor water retention, and low air permeability (18, 19). To overcome these limitations, amendments may be added (20). In situ stabilization of metals can be combined with phytostabilization to overcome the physicochemical limitations and establish a green cover. If such revegetation is performed in combination with immobilizing agents, it can be considered as aided phytostabilization (13, 21). Moreover, the use of organic amendments such as organic wastes presents double advantages: readily available in high quantity and provides nutrients up to adequate levels for most plant species (21). The use of a tree vegetation cap on trace element polluted lands offers the advantages of perenniality and an important deep root system (15), but more concerns are focused on the tree root behaviors in tailings amended with organic matters; the results of Guittonny-Larchevêque and Pednault (22) showed that the mixing of compost with tailings improved their macroporosity and tree survival, although root growth was restricted to the amended tailings, resulting in lower growth and survival rates. Then, this paper aimed (1) to investigate the concentration of macro- and micronutrients in the tailing layers and in plants 15 years after amendment (2) to assess tree species performance through root development in tailings (3) to assess the relationship between the tree root development and the physicochemical characteristics of tailing layers.
Materials and Methods
Study Area
The study was conducted at Kipushi, a mining town located 30 km west of the city of Lubumbashi, in Upper Katanga province, DR Congo (11° 46’ 27.1’’ N and 27° 16’ 21.6’’ E with an altitude of 1,300 m). The climate is the same as in Lubumbashi characterized by 6-month respective dry (May–October) and rainy (November–April) seasons, where 1,300 mm of annual precipitation is recorded. Temperatures range from 16 to 33°C, with the lowest values in the first half of the dry season (June–July). Three large tailings dams are found in this mining town, which have been filled by liquid discharges from the processing of copper-zinc sulfide ores since 1960 (23). Due to its proximity to the residential areas, its size, and the hazard to public health, one of these three tailings (Katapula tailing) was selected for the trial of tree planting in 2004. This tailings dam covers around 146 hectares, but the tree plantation covered an area of 1.5 hectares.
Soil Amendment and Tree Planting
The organic amendment was collected from the public dumping mixed with the topsoil under some ruderal species. After sorting plastics and other solid wastes, the organic and soil amendment were put in the planting holes (planters’ explanation). The circular planting holes had, on average, a 2-m diameter and a 1-m depth in which all the tailing soil was removed and replaced by the amendments, and the following seedlings composed of different species have been planted since 2005: Acacia auriculiformis, Acacia polyacantha, Albizia lebbeck, Cupressus lusitanica, Leucaena leucocephala, Pinus sylvestris, Psidium guajava, Senna siamea, Senna spectabilis, and Syzygium guineense; most of these woody species are commonly used for land afforestation in the Upper Katanga.
Sampling Design
Fourteen years later, profiles were dug at the base of 10 trees of different species depicting the amended and unamended layer by two profiles per species (20 profiles in total). These profiles had four distinct layers; in these layers, the soil structure, texture, and consistency were described, and the root abundance (number of roots dm−2), size (expressed in mm), orientation, distribution, and penetration were evaluated according to the soil description in the manual of Delecour and Kindermans (24) (Table 1).
Root Development Description
The tree root development was described by their abundance, size, distribution, orientation, and penetration within soil layers. Root abundance was the estimation of the number of roots per dm2 of soil according to the five clusters: (1) no root (0 root dm−2), (2) very few (<15 roots dm−2), (3) few (15–75 roots dm−2), (4) numerous (75–200 roots dm−2), and (5) very numerous (>200 roots dm−2). The root size was the determination of the diameter of roots through 7 clusters: (1) very fine (<1 mm), (2) fine (1–2 mm), (3) medium (2–5 mm), (4) big (>5 mm), (5) very fine to fine (all roots < 2 mm), (6) very fine to medium (all roots < 5 mm), and (7) very fine to big (the root of all diameters). Root distribution is characterized by the density of roots within soil layers: (1) regular, (2) fairly regular, and (3) irregular. Root orientation shows the preferential orientation of roots within the layer: (1) horizontal and (2) quelconque (when a root took both horizontal and vertical orientation mixed up). Root penetration shows the behavior of fine and very fine roots in relation to structural aggregates: (1) inped (the root penetrates in the aggregates) and (2) exped (the root passes between the aggregates) (24).
Soil Sampling and Analysis
A soil sample of 150 g was collected at each layer of the profile, it being 4 composite samples per two profiles on 1 species and 40 samples for 10 species. After collection, the soil was air-dried for 72 h, ground and sieved through a 2-mm mesh, and then dried in the proofer at 40°C for 24 h to reduce the remaining moisture. The total organic carbon, pH KCl, extractible macronutrients (P, K, Ca, and Mg) with EDTA, and pseudo-total concentration of trace elements (Zn, Cu, Co, Cd, Pb, Ni, As, and Cr) with aqua regia were analyzed at the Provincial Centre for Agriculture and Rurality (CPAR) of La Hulpe, Belgium.
Data Analysis
One-way analysis of variance (ANOVA) was applied to compare the means of different tailing layers; after Shapiro–Wilk test, data were transformed using logarithms to assure normal distribution. Where significant p-values (p < 0.05) were obtained, differences between modality means were evaluated using Tukey’s HSD post hoc test (p < 0.05). Root development description was displayed using stacked bar charts where the horizontal axis would show profile layers and the vertical axis would show groups of root characteristics.
Root development (root growth) in relation to physicochemical characteristics of layers in the tailings was also addressed through a two-dimensional principal coordinate analysis (PCoA). With this ordination technique, the relationship between soil characteristics, root development, and layer of soil (or tailings) was displayed. All analyses were conducted using R software (version 4.0.3).
Results
Soil Physicochemical Properties of Layers at the Amended Holes
All the measured parameters in Table 2 (pH, TOC, and macronutrients) showed a very high significant difference between layers after one-way ANOVA (p < 0.001). The unamended layer (layer 3 NA) had a significant level of pH with approximately one-half higher (7.5 ± 0.1) than other layers that had a similar level of pH, the superficial layer (layer 1) exhibited the highest total organic carbon (4.8 g 100 g−1 of soil), but the amended layer (layer 3 A) tended to be higher than the unamended layer (layer 3 NA), it being 2.5 and 1.6 g 100 g−1 of soil, respectively; however, this difference was not statistically significant. Phosphorus and potassium were higher in organic matter-rich layers (layer 1 and layer 3 A), with 14.8 and 15 mg 100 g−1 for P, respectively, and 14.4 and 13.8 mg 100 g−1 for K, respectively; the unamended layer showed the lowest P and K content in this soil (3.4 and 4.8 mg 100 g−1, respectively). As for pH, Ca and Mg content were higher in the unamended layer, with Ca being 1,523 mg 100 g−1 and Mg being 352.8 mg 100 g−1, whereas the lowest values of Ca and Mg were observed in layer 1 (404.1 and 203.7 mg 100 g−1, respectively).

Table 2 Extractible macronutrients of soil layers at the amended holes in the tailings dam (with acetate EDTA, n = 10, unit: mg 100 g−1 of soil).
All the observed parameters in Table 3 (trace metals) showed a very high significant difference between layers after one-way ANOVA (p < 0.001), except Cr (p = 0.36).
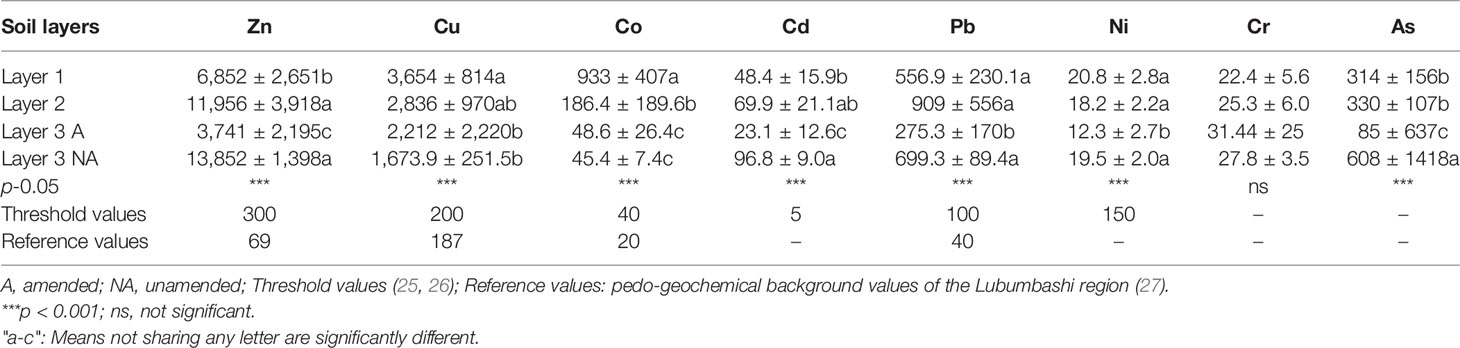
Table 3 Pseudo-total metal concentrations of soil layers at the amended holes in the tailings dam (mg kg−1, n = 10).
The unamended layer (layer 3 NA) and intermediate layer (layer 2) had the highest Zn and Cd content (p < 0.001), with Zn being 13,852 and 11,956 mg kg−1, respectively, and Cd being 96.8 and 69.9 mg kg−1 respectively; the amended layer (layer 3 A) had the lowest concentration in these two elements (Zn: 3741 mg kg−1 and Cd: 23.1 mg kg−1). The superficial layer (layer 1) exhibited a higher concentration of Cu (3654 mg kg−1) and Co (933 mg kg−1) in comparison to other layers, but this was similar to layer 2 concerning Cu, while the amended and unamended layer had a similar concentration of both Cu and Co. The amended layer had lower Pb and Ni concentrations compared to other layers and As remained high in the unamended layer and the lowest value was observed on the amended layer. All trace metal concentrations from different layers of this studied tailings dam plantation were manifold higher than the international threshold values and the pedo-geochemical background throughout the region.
The soil compactness differed from one layer to another (Figure 1); the superficial (L1) layer was totally dominated by soft soil, layer 2 (L2) was fairly compact, the amended layer (LA) was dominated by compact soil, while the unamended layer (LNA) was dominated by compact to very compact soil (Figure 1).
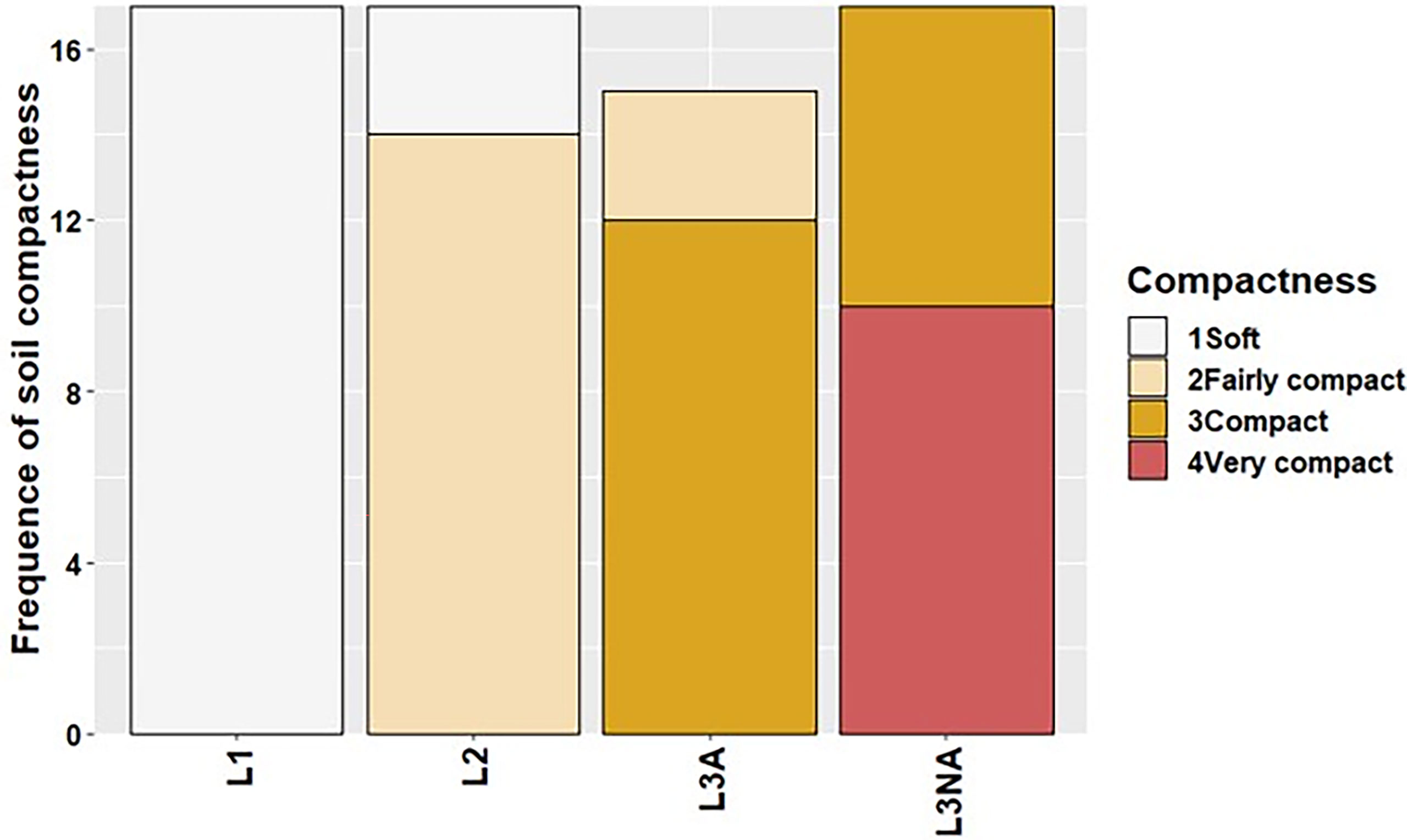
Figure 1 Soil compactness of layers in the profiles (L1: layer 1, L2: layer 2, LA: layer 3 amended, LNA: layer 3 unamended).
Tree Root Development in the Layers of Amended Holes
The development of tree roots in layers of the tailing soil was described through 4 different characteristics, the abundance, the size, the orientation, and distribution (Figure 2).
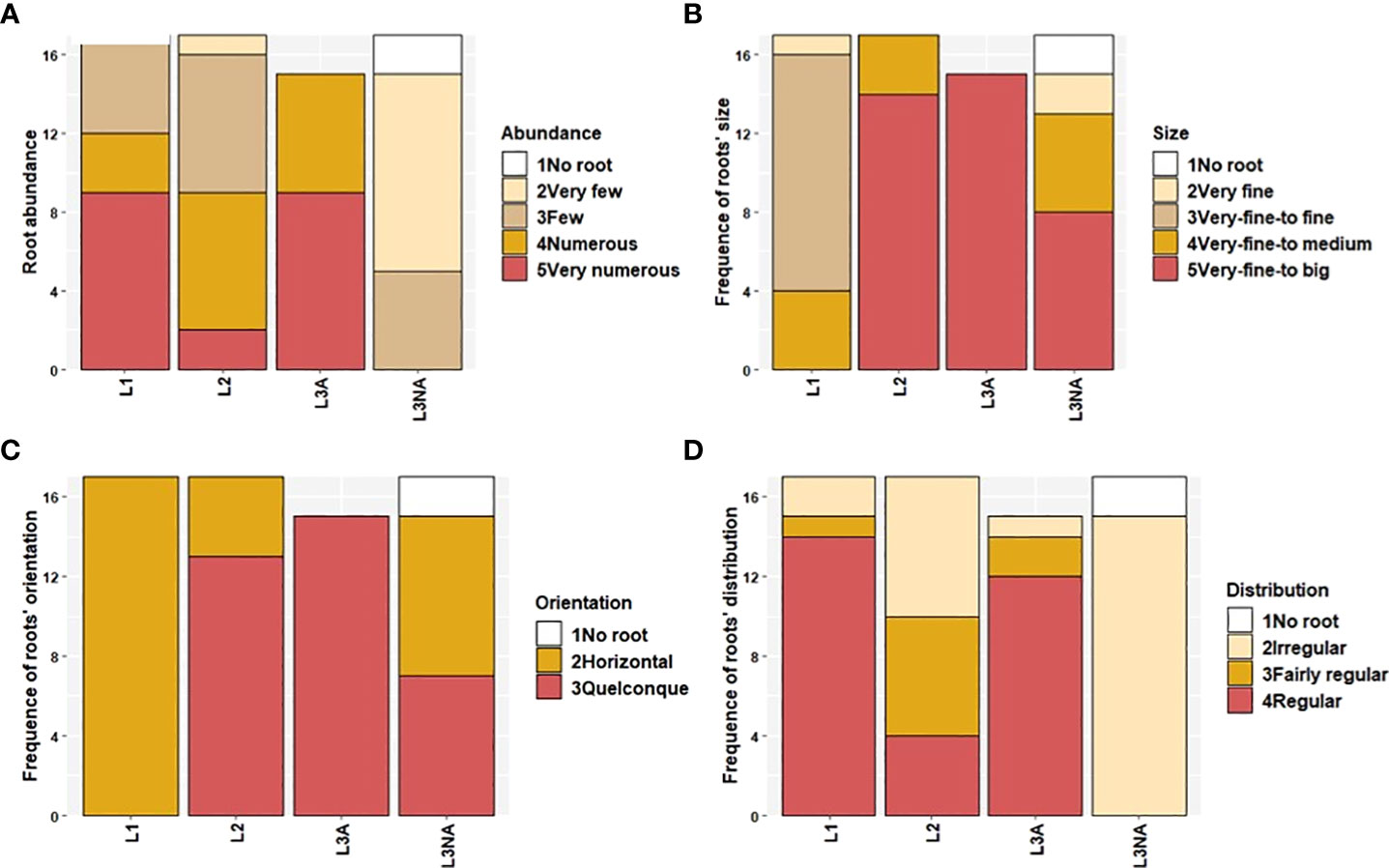
Figure 2 Tree root characteristics in different layers of soil [L1: layer 1, L2: layer 2, L3A: layer 3 amended, L3NA: layer 3 unamended; (A) root abundance, (B) root size, (C) root orientation, (D) root distribution].
Root abundance (Figure 2A): layers 1 and 3 amended had a large proportion of very numerous roots than other layers. Layer 2 was dominated by an equal proportion of numerous roots and few roots, while layer 3 unamended was dominated by a large share of very few roots followed by few roots with the presence of no root layers.
Root size (Figure 2B): layers 2 and 3 amended had a larger share of very fine to big roots followed by layer 3 unamended, and layer 1 (L1) was dominated by a larger share of very fine to fine roots.
Root orientation (Figure 2C): layer 2 (L2) and layer 3 amended (LA) were dominated by a larger share of quelconque (a root that took both horizontal and vertical orientation), L1 was totally dominated by horizontal roots, and the LNA had horizontal and mixed horizontal and vertical orientation, with a little share of no root.
Root distribution (Figure 2D): The L1 and LA layers were largely dominated by a share of regular root distribution, L2 had an equal share of fairly regular and irregular root distribution followed by a little share of regular distribution, while LNA was largely dominated by irregular root distribution followed by no root layers.
Root Development According to Tree Species
Root abundance in amended layers ranged from 75 to 200 roots dm−2 and >200 roots dm−2 with P. sylvestris, A. lebbeck, L. leucocephala, S. siamea, S. spectabilis, and S. guineense showing a root abundance >200 roots dm−2, whereas in the unamended layers, the root abundance ranged from <15 roots dm−2 and 15 to 75 roots dm−2 and only A. polyacantha and P. guajava had a high root abundance that ranged from 15 to 75 roots dm−2 while the rest of the tree species were under 15 roots dm−2. Root size of all tree species ranged from very fine to big in amended layers but only A. lebbeck, L. leucocephala, S. siamea, and A. polyacantha had root sizes ranging from very fine to big in the unamended layers, while S. spectabilis and P. guajava had only very fine roots in these unamended layers. The distribution of roots in the amended layers was regular to fairly regular in all tree species with the exception of P. guajava, which had irregular root distribution in these layers, but root distribution was irregular in all tree species in the unamended layers (Table 4).
Trace Metal Concentration in Tree Tissues
In addition to tree root behavior in different tailing layers, Figure 3 displays the metal accumulation status of tree species in this tailings dam. Most of the tree species had higher Co, Cu, and Zn concentrations in roots than in leaves. Trees had Cu concentrations in leaves and roots within the normal range, except for S. spectabilis, which had higher Cu concentrations in roots accompanied by a slightly higher concentration of Zn in leaves, whereas, A. auriculiformis, C. lusitanica, and P. guajava had a higher concentration of Zn in their roots.
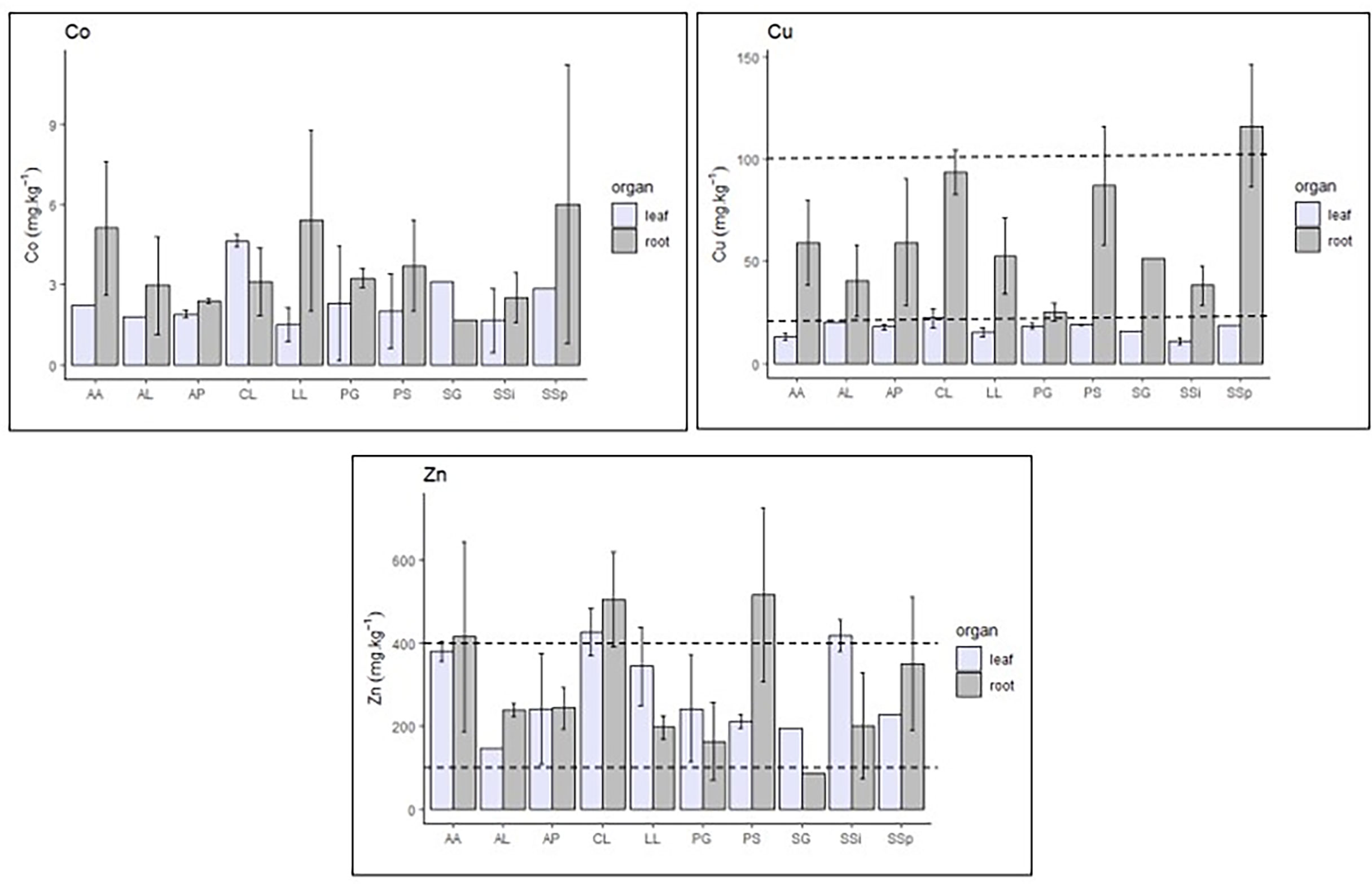
Figure 3 Accumulation of Co, Cu, and Zn (mg kg−1) in different tissues of trees on the tailings. AA: A. auriculiformis, AL: A. lebbeck, AP: A. polyacantha, CL: C. lusitanica, LL: L. leucocephala, PG: P. guajava, PS: P. sylvestris, SG: S. guineense, SSi: S. siamea, SSp: S. spectabilis. Dotted lines are normal ranges of trace elements in plants, Cu: 20–100 mg kg−1 and Zn: 100–400 mg kg−1 (12).
Root Development According to Physicochemical Characteristics of the Soil
PCoA was applied to investigate the relationships between root parameters and physicochemical characteristics of the soil (tailings). The first two dimensions of PCoA (PCoA1 and PCoA2) explained 59.4% of the total variation, with component 1 accounting for 40.4% and component 2 accounting for 19% of the variance (Figure 4). This analysis showed that root abundance was positively correlated to K and P within the amended and middle layer, and the size, orientation, and penetration of roots were influenced by the compactness of the substrates (see details in Figures 1, 2); root distribution covaried with Ca, Mg, Zn, and pH (this correlation was high in the unamended layer). Cu and Co appeared to have a strong affinity with TOC within the surface layer (Figure 4).
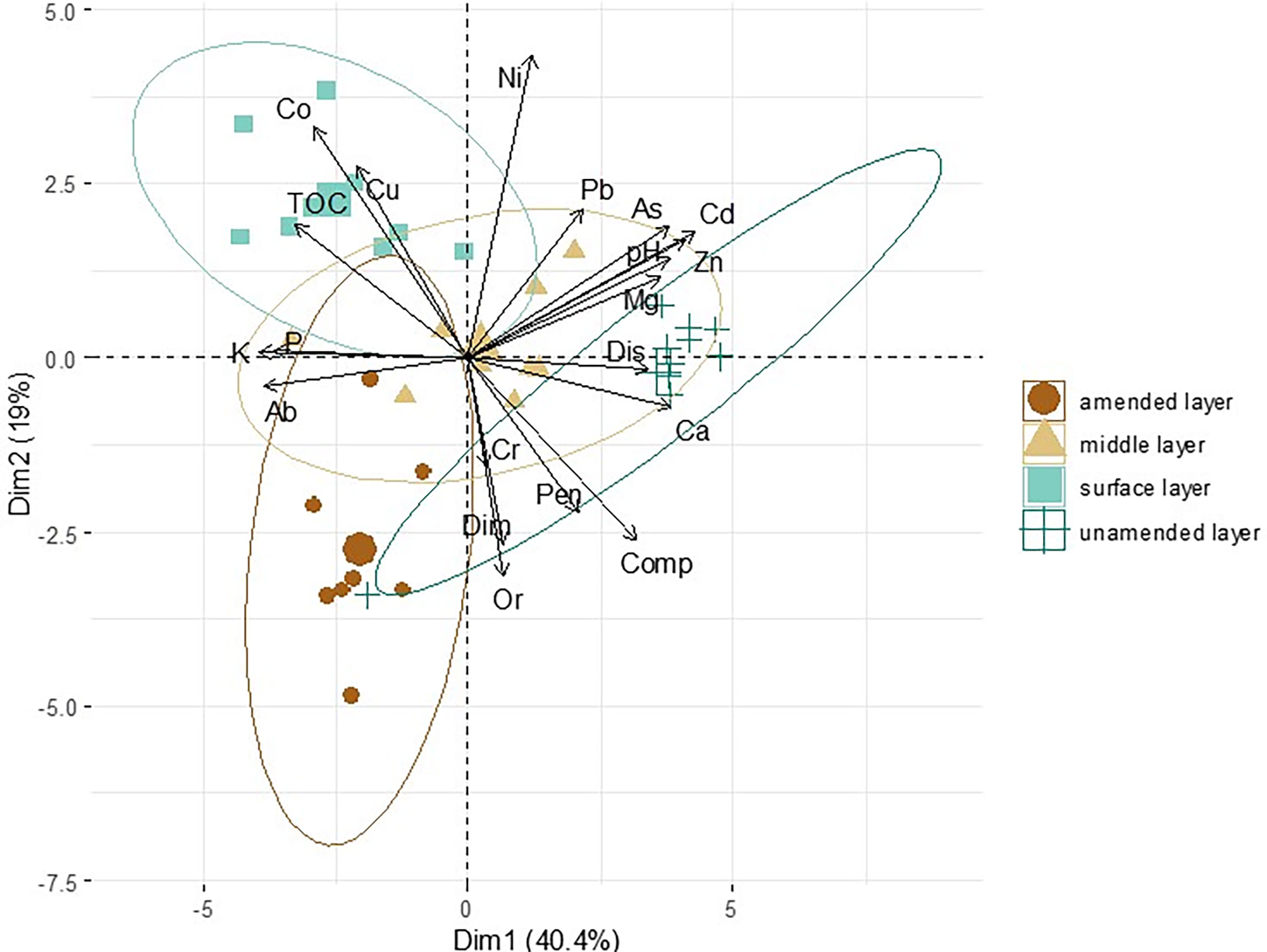
Figure 4 Principal coordinate analysis (PCoA) of root parameters according to the physicochemical characteristics of tailing soil (Ab: abundance of roots, Dim: dimension of roots, Dis: distribution of roots, Or: orientation of roots, Pen: penetration of roots, and Comp: compactness of soil).
Discussion
Soil Condition 15 Years After Amendment and Tree Planting
The nutrient concentration in the amended layers of tailings was still adequate for tree survival since it was much higher or similar compared to uncontaminated soils from the nearest miombo woodland (28) (tailings: P: 15 mg 100 g−1, K: 13.8 mg 100 g−1, Mg: 133.1 mg 100 g−1, Ca: 671.2 mg 100 g−1; miombo woodland soil: P: 0.3 mg 100 g−1, K: 15.5 mg 100 g−1, Mg: 4.3 mg 100 g−1, Ca: 4.8 mg 100 g−1). The high Ca, Mg, and alkaline pH (7.5) in this soil is due to the lime effect added by the mining companies during the metallurgical process (16, 23) and influenced this high pH and Ca and Mg concentration in the amended and surface layer, thus contributing to the soil fertility. The high TOC, P, and K concentrations on the surface layer (layers 1 and 2) prove that the decomposition and mineralization of the litter are ongoing in spite of the high metal concentration in the soil, and can provide more nutrients to the in-depth layers. The unamended layers had very compact to compact (Figure 1) soil since they are constituted by the original material of the tailings that commonly have high bulk density, compaction due to the fine soil texture, and low infiltration rate (18, 29, 30). Festin (19) showed that soil compaction coupled with poor macro-nutrient availability and the toxic levels of heavy metals are major barriers to revegetation of mine waste sites. All layers had pseudo-total trace metal concentrations manifold higher than international threshold values (25, 26), with Zn, Cd, and As being higher in the unamended layer (tailing layer) than in other layers. The maximum values of Zn in tailing layers were 200 times higher than the pedo-geochemical background of the Lubumbashi region, 47 times for Co, 20 times for Cu, and 17 times for Pb (27). The high values and particular distribution pattern of zinc in this layer (tailing layer) are due to the zinc-rich ore minerals that were exploited by the Gécamines’ plant at Kipushi (23). The Cu and Co tended to be higher in the top layers (litter-rich layer) and lower in the tailing layer, while Pb and Ni were lowest in the amended layer (Table 3). The organic matter content, dust falling and caught by tree leaves, speciation, and the affinity between chemical elements may be the cause of variation of metal concentration in these layers (31).
Root Behavior According to Soil Layers, Tree Species, and Soil Characteristics
Guittonny-Larchevêque and Pednault (22) in a reclamation trial of tailings showed that roots of trees grown on compost mixed with tailings were restricted to the amended tailings, resulting in lower tree growth and survival rates, while those grown on uncontaminated topsoil amendment had roots growing in the tailings under the soil layers. Amendments in this study were a mixture of organic wastes from dumping with topsoil, and the result showed that tree roots could well develop in the amended layers (very abundant, from very fine to big and regularly distributed), given that nutrient contents were higher and substrates were less compact in this layer compared to the unamended tailing layer that had few to very few roots and irregularly distributed. Nevertheless, the presence of roots in the unamended tailing layer (from very fine to medium or big) indicates that root development in tailings was possible despite the high compactness of the substrates and the elevated total concentration of Zn, Cd, and Pb. Of the ten species, A. polyacantha and P. guajava (Table 4) showed a higher number of roots (15 to 75 roots dm−2 of soil) in the unamended tailing layers compared to 8 other tree species (less than 15 roots dm−2 of soil), and among the two, A. polyacantha appeared to have root sizes from very fine to big, while P. guajava had only very fine roots in these layers. These two species (A. polyacantha and P. guajava) were confirmed by Kambing'a and Syampungani (32), Bazihizina et al. (33) Perlatti et al. (34), and Festin et al. (16) as highly tolerant species to metal-rich soils. A. polyacantha is a native species mostly found in the lowlands of miombo woodland, and this was the initial state of this area before pollution. New (35) also showed that Acacia species are considered to be invasive as they have the potential to survive and adapt to varying environments. They have a wider ecological amplitude, and as leguminous plants, they are capable of fixing atmospheric nitrogen into the soil and therefore tend to improve the nutrient status of the soils with time. P. guajava has been proven to have a high tolerance to metal stress (36). Bazihizina et al. (33) tested the tolerance of the species to Ni in the hydroponic experiments and attested that P. guajava could have good growth on treatments with higher concentrations of Ni with lower translocation to shoots, suggesting a potential role for the phytostabilization of polluted soils.
Species that are less sensitive to stress factors (in this case, metal toxicity and soil compactness) need to be promoted to implement phytostabilization of tailings dam with fewer amendments. The more the constraints in the tailings dam, i.e., poor soil nutrients, high concentration of heavy metals, and compactness were reduced by the application amendments, the more improved was the root behavior (22, 37). The abundance of roots was positively influenced by the fertility of the soil, whereas the size, orientation, and penetration of roots were not related to fertility or to the toxicity of metals, but to the compactness of the substrate.
The pseudo-total concentration of Cu and Co appeared to be very high in surface and amended layers than in the tailing layer (unamended layer) with high affinity to TOC (Figure 4), but this elevated concentration of Cu and Co did not impair root development in the amended layer, likely due to the organic matter effect reducing trace metal mobility or toxicity (8, 20, 38), and the high affinity of Cu and Co with the TOC may be due to the ability of the organic matter to sorb some metals as copper confirmed by Pourret etal. (31).
Heavy Metal Concentration Status of Ten Tree Species on the Tailings Dam
In spite of elevated metal concentration in soil, ranging from 3,741 to 13,852 for Zn, from 1,674 to 3,654 for Cu, and from 45 to 933 for Co and manifold higher compared to the pedo-geochemical background, the trace metal content in tissues (leaf and root) of ten different tree species was within or slightly above the range limit for Zn and Cu. It is also worth noting that the higher accumulation of metals in roots than in the above part of trees is a good characteristic of species for phytostabilization (12, 16, 39). Nevertheless, leaves of S. simea and C. lusitanica need to be avoided as their Zn content could go beyond the normal range. The Co threshold range in non-edible tissues of plants for the soil of the Copperbelt region is yet to be clearly discussed. However, considering the threshold of 0.02–1 mg kg−1 for Co (40), leaves should be avoided for human or animal consumption.
However, P. guajava is known for producing edible fruits, but are guavas from the tailing plantation contaminated? The Co, Cu, and Zn concentrations in fruits of P. guajava as shown in Table 5 were all within the normal range, but consumption of leaves is to be avoided because of the Co concentration that was beyond the normal range.

Table 5 Trace metal concentration in fruits and leaves of P. guajava from the tailing tree plantation.
Conclusion
Fifteen years after amendment with organic waste mixed with topsoil and tree planting on the tailings dam, the nutrient concentration in the amended holes was still affordable for tree survival, although Cu and Co concentration tended to increase in organic matter-rich layers. Tree roots behaved well in the amended layer (very abundant, all sizes, and regularly distributed) in comparison to the tailing layer; however, a few roots of the surviving species ranging from very fine to big went horizontally beyond the amended layer, indicating the possibility of tree survival on the tailings dams over many years. Of the 10 species, A. polyacantha and P. guajava showed an abundant root development in the unamended tailing layers compared to the other 8 tree species. Species that are less sensitive to stress factors (in this case, metal toxicity and soil compactness) need to be promoted to implement phytostabilization of tailings dams with fewer amendments, and the topsoil from the uncontaminated areas should be added to the organic amendments for the rehabilitation of larger areas.
This case study shows the feasibility of a long-term phytostabilization using trees in the metal-polluted lands due to the fact that amendments could overthrow limits on metal toxicity and soil compactness, and tree roots, in turn, attempted to grow beyond the area dominated by the amendments. Moreover, the metal concentrations in leaves were within or slightly higher than the normal range, with the caution that they are not for human or animal consumption, but given the lower content of Cu, Zn, and Co in guava, there is no indication of hazards in case of their consumption.
Data Availability Statement
The original contributions presented in the study are included in the article/supplementary material. Further inquiries can be directed to the corresponding authors.
Author Contributions
JK, MS, and GC designed the research; JK, SL, and MN performed the sampling and analyzed the data; JK wrote the manuscript; MS and GC corrected the manuscript. All authors contributed to the article and approved the submitted version.
Conflict of Interest
The authors declare that the research was conducted in the absence of any commercial or financial relationships that could be construed as a potential conflict of interest.
Publisher’s Note
All claims expressed in this article are solely those of the authors and do not necessarily represent those of their affiliated organizations, or those of the publisher, the editors and the reviewers. Any product that may be evaluated in this article, or claim that may be made by its manufacturer, is not guaranteed or endorsed by the publisher.
References
1. Kidd P, Barcelo J, Bernal MP, Navari-Izzo F, Poschenrieder C, Shilev S, et al. Trace Element Behaviour at the Root–Soil Interface: Implications in Phytoremediation. Environ Exp Bot (2009) 67(2009):243–59. doi: 10.1016/j.envexpbot.2009.06.013
2. Kaniki AT, Tumba K. Management of Mineral Processing Tailings and Metallurgical Slags of the Congolese Copperbelt: Environmental Stakes and Perspectives. J Cleaner Production (2019) 210:1406–13. doi: 10.1016/j.jclepro.2018.11.131
3. Dudka S, Adriano DC. Environmental Impacts of Metal Ore Mining and Processing: A Review. J Environ Qual (1997) 26(3):590–602. doi: 10.2134/jeq1997.00472425002600030003x
4. Wong JWC, Ip CM, Wong MH. Acid-Forming Capacity of Pb-Zn Mine Tailings and Its Implications for Mine Rehabilitation. Environ Geochem Health (1998) 20:149–55. doi: 10.1023/A:1006589124204
5. Kien CN, Noi NV, Son LT, Ngoc HM, Tanaka S, Nishina T, et al. Heavy Metal Contamination of Agricultural Soils Around a Chromite Mine in Vietnam. Soil Sci Plant Nutr (2010) 56(2):344–56. doi: 10.1111/j.1747-0765.2010.00451.x
6. Conesa HM, Schulin R, Nowack B. A Laboratory Study on Revegetation and Metal Uptake in Native Plant Species From Neutral Mine Tailings. Water Air Soil pollut (2007) 183(1-4):201–12. doi: 10.1007/s11270-007-9369-1
7. Banza CLN, Nawrot TS, Haufroi V, Decrée S, De Putter T, Smolders E, Nemery B. High Human Exposure to Cobalt and Other Metals in Katanga. A Mining Area of the Democratic Republic of Congo. Environ Res (2009) 109(6):745–52. doi: 10.1016/j.envres.2009.04.012
8. Shutcha MN, Mubemba MM, Faucon MP, Luhembwe MN, Visser M, Colinet G, et al. Phytostabilisation of Copper-Contaminated Soil in Katanga: An Experiment With Three Native Grasses and Two Amendments. Int J Phytoremediation (2010) 12(6):616–32. doi: 10.1080/15226510903390411
9. Pilon-Smits E. Phytoremediation. Annu Rev Plant Biol Vol (2005) 56:15–39. doi: 10.1146/annurev.arplant.56.032604.144214
10. Kidd P, Mench M, Alvarez-Lopez V, Bert V, Dimitriou I, Friesl-Hanl W, et al. Agronomic Practices for Improving Gentle Remediation of Trace Element-Contaminated Soils. Int J Phytoremediation (2015) 17(11):1005–37. doi: 10.1080/15226514.2014.1003788
11. Cunningham SD, Berti WR, Huang JW. Phytoremediation of Contaminated Soils. Trends Biotechnol (1995) 13:393–7. doi: 10.1016/S0167-7799(00)88987-8
12. Mendez MO, Maier RM. Phytostabilization of Mine Tailings in Arid and Semiarid Environments—an Emerging Remediation Technology. Environ Health Perspect (2008) 116(3):278–83. doi: 10.1289/ehp.10608
13. Alvarenga P, Gonçalves AP, Fernandes RM, De Varennes A, Vallini G, Duarte E, et al. Organic Residues as Immobilizing Agents in Aided Phytostabilization:(I) Effects on Soil Chemical Characteristics. Chemosphere (2009) 74(10):1292–300. doi: 10.1016/j.chemosphere.2008.11.063
14. Boisson S, Le Stradic S, Collignon J, Séleck M, Malaisse F, Shutcha MN, et al. Potential of Copper-Tolerant Grasses to Implement Phytostabilisation Strategies on Polluted Soils in South DR Congo. Environ Sci pollut Res (2016) 23(14):13693–705. doi: 10.1007/s11356-015-5442-2
15. Pulford ID, Watson C. Phytoremediation of Heavy Metal-Contaminated Land by Trees-a Review. Environ Int (2003) 29(4):529–40. doi: 10.1016/S0160-4120(02)00152-6
16. Festin ES, Salk C, Tigabu M, Syampungani S, Odén PC. Biological Traits of Tropical Trees Suitable for Restoration of Copper-Polluted Lands. Ecol Eng (2019) 138:118–25. doi: 10.1016/j.ecoleng.2019.07.010
17. Gómez-Sagasti MT, Alkorta I, Becerril JM, Epelde L, Anza M, Garbisu C. Microbial Monitoring of the Recovery of Soil Quality During Heavy Metal Phytoremediation. Water Air Soil Pollut (2012) 223(6):3249–62. doi: 10.1007/s11270-012-1106-8
18. Wong MH. Ecological Restoration of Mine Degraded Soils, With Emphasis on Metal Contaminated Soils. Chemosphere (2003) 50:(2003) 775–780. doi: 10.1016/S0045-6535(02)00232-1
19. Festin ES. Post-Mining Restoration in Zambia. Screening Native Tree Species for Phytoremediation Potential. Ph.D. Thesis. In: Faculty of Forest Science, Southern Swedish Research Centre, Alnarp (2020). p. 77.
20. Lee SH, Ji W, Lee WS, Koo N, Koh IH, Kim MS, et al. Influence of Amendments and Aided Phytostabilization on Metal Availability and Mobility in Pb/Zn Mine Tailings. J Environ Manage (2014) 139:15–21. doi: 10.1016/j.jenvman.2014.02.019
21. Labidi S, Firmin S, Verdin A, Bidar G, Laruelle F, Douay F, et al. Nature of Fly Ash Amendments Differently Influences Oxidative Stress Alleviation in Four Forest Tree Species and Metal Trace Element Phytostabilization in Aged Contaminated Soil: A Long-Term Field Experiment. Ecotoxicol Environ Saf (2017) 138:190–8. doi: 10.1016/j.ecoenv.2016.12.027
22. Guittonny-Larchevêque M, Pednault C. Substrate Comparison for Short-Term Success of a Multispecies Tree Plantation in Thickened Tailings of a Boreal Gold Mine. New Forests (2016) 47(5):763–81. doi: 10.1007/s11056-016-9543-7
23. Kitobo WS. Dépollution Et Valorisation Des Rejets Miniers Sulfurés Du Katanga. Cas Des Tailings De L’ancien Concentrateur De Kipushi (Doctoral Dissertation. Thèse De Doctorat. Faculté Des Sciences Appliquées. Université De Liège. (2009). p. 254.
24. Delecour F, Kindermans M. Manuel De Description Des Sols. Faculté Des Sci Agronomiques l'Etat Service la Sci du Sol (1977), 62.
25. Kataba-Pendias A, Pendias H. Trace Elements in Soils and Plants. Boca Raton, FL: CRC Press (2001).
26. Baize D. Teneurs Totales En Éléments Traces Métalliques Dans Les Sols (France): Références Et Stratégies D'interprétation. Programme ASPITET Editions Quae (1997) 410.
27. Shutcha MN, Mukobo RP, Muyumba KD, Mpundu MM, Faucon MP, Lubalega KT, et al. Fond Pédogéochimique Et Cartographie Des Pollutions Des Sols À Lubumbashi. In: Bogaert J, Gilles C, Gregory M, editors. Anthropisation Des Paysages Katangais. Gembloux, Belgique: Presses Universitaires de Liége-Agronomiqe Gembloux (2018). p. 215–28.
28. Mushagalusha FC. Mécanismes De Structuration Des Communautés Végétales Sur Les Hautes Termitières Dans La Plaine De Lubumbashi (RD Congo). PhD Thesis. DR Congo: University of Lubumbashi (2018). p. 181.
29. Titshall LW, Hughes JC, Bester HC. Characterisation of Alkaline Tailings From a Lead/Zinc Mine in South Africa and Evaluation of Their Revegetation Potential Using Five Indigenous Grass Species. South Afr J Plant Soil (2013) 30(2):97–105. doi: 10.1080/02571862.2013.807361
30. Ginocchio R, León-Lobos P, Arellano EC, Anic V, Ovalle JF, Baker AJM. Soil Physicochemical Factors as Environmental Filters for Spontaneous Plant Colonization of Abandoned Tailing Dumps. Environ Sci pollut Res (2017) 24(15):13484–96. doi: 10.1007/s11356-017-8894-8
31. Pourret O, Lange B, Bonhoure J, Colinet G, Decrée S, Mahy G, et al. Assessment of Soil Metal Distribution and Environmental Impact of Mining in Katanga (Democratic Republic of Congo). Appl Geochem (2016) 64:43–55. doi: 10.1016/j.apgeochem.2015.07.012
32. Kambing'a MK, Syampungani S. Performance of Tree Species Growing on Tailings Dam Soils in Zambia: A Basis for Selection of Species for Re-Vegetating Tailings Dams. J Environ Sci Eng B (2012) 1(7B):827.
33. Bazihizina N, Redwan M, Taiti C, Giordano C, Monetti E, Masi E, et al. Root Based Responses Account for Psidium Guajava Survival at High Nickel Concentration. J Plant Physiol (2015) 174:137–46. doi: 10.1016/j.jplph.2014.10.011
34. Perlatti F, Ferreira TO, da Costa Roberto FA, Romero RE, Sartor LR, Otero XL. Trace Metal/Metalloid Concentrations in Waste Rock, Soils and Spontaneous Plants in the Surroundings of an Abandoned Mine in Semi-Arid NE-Brazil. Environ Earth Sci (2015) 74(6):5427–41. doi: 10.1007/s12665-015-4556-7
35. New TR. A Biology of Acacias. In: A Biology of Acacias. Oxford: Oxford University Press in Association With La Trobe University Press (1984).
36. Perry CT, Divan AM Jr., Rodriguez MTR, Atz VL. Psidium Guajava as a Bioaccumulator of Nickel Around an Oil Refinery, Southern Brazil. Ecotoxicol Environ Saf (2010) 73(4):647–54. doi: 10.1016/j.ecoenv.2009.10.001
37. Brennan A, Jiménez EM, Puschenreiter M, Alburquerque JA, Switzer C. Effects of Biochar Amendment on Root Traits and Contaminant Availability of Maize Plants in a Copper and Arsenic Impacted Soil. Plant Soil (2014) 379(1):351–60. doi: 10.1007/s11104-014-2074-0
38. Mollon LC, Norton GJ, Trakal L, Moreno-Jimenez E, Elouali FZ, Hough RL, et al. Mobility and Toxicity of Heavy Metal (Loid) s Arising From Contaminated Wood Ash Application to a Pasture Grassland Soil. Environ pollut (2016) 218:419–27. doi: 10.1016/j.envpol.2016.07.021
39. Rana V, Maiti SK. Differential Distribution of Metals in Tree Tissues Growing on Reclaimed Coal Mine Overburden Dumps, Jharia Coal Field (India). Environ Sci pollut Res (2018) 25:9745–58. doi: 10.1007/s11356-018-1254-5
40. Elderfield H. Chemical Elements in the Environment. Factsheets for the Geochemist and Environmental Scientist. Berlin, Heidelberg, New York, London, Paris, Tokyo, Hong Kong: Springer-Verlag (2000). p. 398, ISBN: 110.00,£ 64.50, SFr 152.00, Ös 1227.00 (hard covers). ISBN 3. REIMANN, C. & CARITAT P. de. 1998.
Keywords: trace metal, tailings, amendment, tree species, reclamation
Citation: Mwanasomwe JK, Langunu S, Nsenga NS, Shutcha MN and Colinet G (2022) Effect of Organic Amendment on the Physicochemical Characteristics of Tailings Dam Soil and Root Development of Tree Species, Fifteen Years After Planting. Front. Soil Sci. 2:934999. doi: 10.3389/fsoil.2022.934999
Received: 03 May 2022; Accepted: 15 June 2022;
Published: 14 July 2022.
Edited by:
Spyridon Koutroubas, Democritus University of Thrace, GreeceReviewed by:
Jianxu Wang, Institute of Geochemistry (CAS), ChinaTamer A. Elbana, National Research Centre, Egypt
Copyright © 2022 Mwanasomwe, Langunu, Nsenga, Shutcha and Colinet. This is an open-access article distributed under the terms of the Creative Commons Attribution License (CC BY). The use, distribution or reproduction in other forums is permitted, provided the original author(s) and the copyright owner(s) are credited and that the original publication in this journal is cited, in accordance with accepted academic practice. No use, distribution or reproduction is permitted which does not comply with these terms.
*Correspondence: Jacques Kilela Mwanasomwe, amtpbGVsYUB1bGllZ2UuYmU=; Gilles Colinet, R2lsbGVzLkNvbGluZXRAdWxpZWdlLmJl