Nutritional properties of underutilized legumes and intercropped maize
- 1Food Security and Safety Focus Area, Faculty of Natural and Agricultural Sciences, North-West University, Mmabatho, South Africa
- 2Genetic Resources Centre, International Institute of Tropical Agriculture, Ibadan, Nigeria
- 3Department of Microbiology, Obafemi Awolowo University, Ile-Ife, Nigeria
Over the years, intercropping which is majorly carried out on legumes and cereal has been practiced to maximize the utilization of land resources and increase the productivity over a piece of land. Most studies on intercropping focus on the yield and yield parameters, leaving out other important aspects such as the nutritional value of the harvested crops. Underutilized legumes are crops which have very scanty information available on them which reduces their general acceptability and utilization. The effects of intercropping on the nutrient status of underutilized legumes and maize in an intercropping system are not well understood. Therefore, the objective of the research was to assess the effect of intercropping on the nutrient and antinutrient parameters of African Yam Bean (AYB), and Winged bean (WB) when monocropped and when intercropped with maize. Hence, this research sought to answer the following questions: (i) does intercropping with maize affect the nutrient and anti-nutrient properties of AYB and WB (ii) does intercropping with AYB and WB and the application of urea fertilizer affect the nutrient and anti-nutrient properties of maize. Five accessions each of AYB and WB were separately intercropped with two accessions of maize. The research was carried out on the research field of the International Institute of Tropical Agriculture, Ibadan, Nigeria. The field was laid out in a Randomized Complete Block Design in triplicates. The treatment consisted of sole and intercropped legumes and maize as well as maize planted with urea fertilizer. The result from the research revealed that intercropping had effects on the nutrient and anti-nutrient properties of AYB, WB and maize, even though each accession responded differently to the intercropping. For instance, in the legumes, intercropping with M2 led to a reduction in the moisture content of AYB accessions TSs4, TSs30, and TSs77, and an increase in the moisture content in winged bean accession Tpt51. Equally, a reduction in the protein content of African yam bean accessions TSs4 and 101 when intercropped with both maize accessions was observed (P ≤ 0.05). On the other hand, an increase was observed in the protein content of AYB accession TSs30 (intercropped with M2), as well as WB accession 15-4 (intercropped with M2) and Tpt51 (intercropped with M1). A reduced tannin content was observed in WB accession Tpt12 intercropped with both maize accessions, while an increased the tannin content was observed in AYB accessions TSs4, TSs101, and WB accessions 15-4, and Tpt32 when intercropped with both maize accessions (P ≤ 0.05). Finally, intercropping increased the fat content in both maize accessions, increased the tryptophan content of M1 when intercropped with Tpt32, and increased the carbohydrate content in M1 intercropped with Tpt51. In conclusion, the results from this experiment revealed that intercropping affected the nutritional and antinutritional properties of the maize and legumes. Of all the Accessions of the underutilized legumes used, AYB accession TSs30 responded better to intercropping by having a better nutritional value (an increased ash, protein, fat and carbohydrate contents as well as a reduced moisture content); it is therefore necessary to further explore this accession to ensure that the potentials embedded in itis maximally tapped into to enhance food security.
1 Introduction
Nutritional insecurity is a global challenge in recent times, in order to increase food availability, some farming practices such as intercropping are being utilized. A large proportion of the world population, especially from developing African countries, are deficient in micronutrient (1). The unavailability of these nutrients could be due to the presence of high anti-nutrients in food crops. Anti-nutrients are organic or synthetic substances that inhibit digestion as well as nutrient utilization, intake and absorption (2, 3). Anti-nutrients in food include lectins, enzyme inhibitors (a-amylase, trypsin, and chymotrypsin), polyphenols, phytic acids, tannin, and saponin (4). Intercropping (mixed cropping) is the presence of two different crops on the same land in a period that cut across the vegetative periods of both plants. Reports are available on the nutritional and anti-nutritional composition of African yam bean, Sphenostylis stenocarpa (AYB) (5, 6) and Winged Bean, Psophocarpus tetragonolobus (WB) grains (7, 8) when solely planted but insufficient information are available on these parameters when intercropped.
AYB (9) and WB (10) reported that underutilized legumes are legumes that are not well-known due to scanty research information available on them. WB has been reported to be highly nutritious and contains low anti-nutrients (8), while AYB has been reported to contain high nutrient but need more processing to reduce its anti-nutritional properties to make it ideal for consumption (5). The effects of nutritional and anti-nutritional components cannot be undermined in food products, as they highly correlate with food security and safety. Moisture content is an essential factor that affects the processing and storage of food products (11), a high moisture content makes crops susceptible to microbial proliferation and vice-versa. Iron is vital in maintaining vigorous hair, nails, skin and in the production of haemoglobin in the body (12). Ash content in food depicts the total mineral (K, Zn, Mg, Ca, Fe, Cu, Mg, and Na) composition in food products (13), fat is useful in determining the shelf-life and safety of food (14) and protein serves as a building block of life which is responsible for the repair of damaged tissues and cells (15). Tryptophan is an important amino acid gotten from plants and needed in the formation of protein in living cells which after consumption, is transformed into serotonin, kynurenine, nicotinamide and melatonin (16). Carbohydrate is essential as a source of energy to all living things, phosphorus in legumes is present in phytic acid form and it is well known to inhibit the availability of micronutrients (iron, calcium, manganese, zinc, and magnesium) in humans (2). Consumption of anti-nutrients could lead to bloating, rashes, nausea, headache, and nutrient deficiency (17). Intercropping has been reported to affect the nutritional component of the crops involved, for instance, Raza et al. (18) carried out a research where soybean and maize were intercropped and the researchers revealed that intercropping led to an improved nutrient status in the legume and a reduce nutritional content in maize. Equally, Soe Htet et al. (19) reported a higher protein content in the silage of intercropped maize and soybean. Majority of the research available on intercropping of legumes and cereals focused on the yield and yield parameters with very few works on the nutrient and anti-nutrient parameters. Therefore, the objective of the study is to assess the nutritional and anti-nutritional properties of intercropped and solely cropped grains of underutilized legumes and maize; this will give an insight on the effect of intercropping on these parameters, consequently giving room to tap into the potentials of intercropping in these crops to improve food security. Hence this research sought to answer the following questions: (i) does intercropping with maize affect the nutrient and anti-nutrient properties of African yam bean and winged bean? (ii) does intercropping with AYB and WB and urea fertilizer application affect the nutrient and anti-nutrient properties of maize?
Materials and methods
2.1 Study site and treatment
The nutritional and anti-nutritional composition of the maize, AYB and WB seeds were evaluated using the research field of the International Institute of Tropical Agriculture, Ibadan which is located at latitude 7° 22’ 36.24” N and longitude 3° 56’ 23.23” E. The treatment contained sole and intercropped AYB, WB and maize and also contained maize planted with urea fertilizer. Samples of all harvested seeds were evaluated at the Food and Nutrition Sciences Laboratory of the International Institute of Tropical Agriculture, Ibadan, Nigeria. The field was laid out in a Randomized Completely Block Design (RCBD) in three replicates and the planting density was ratio 1:1.The field was manually weeded at regular intervals and the maize were sprayed with Chlorantraniliprole insecticide (ampligo) at the rate of 100g per litre to prevent the invasion of armyworms. In the intercropped plots, AYB and WB seeds were sown at a distance of 25 cm from maize, while for the sole maize and legumes, the planting distance were 25 cm and 75 cm respectively. Maize plots where urea fertilizer was applied also had a planting distance of 25 cm and the urea was applied at the rate of 90kg/ha as a positive control treatment. Different fields were utilized for the planting of AYB and WB. The maize seeds were planted four weeks after the legumes. Five accessions of AYB (TSs30, TSs77, TSs68, TSs101, and TSs4), five accessions of WB (Tpt53, 15-4, Tpt12, Tpt51, Tpt32), and two accessions of maize (Tzm1310 and Tzm188) were used for the experiment. The phytate, ash, moisture, protein, fat, tannin, tryptophan and carbohydrate contents of the seeds were evaluated in the sole and intercropped treatments.
2.2 Determination of ash content
Two grams of the samples were weighed into the crucible and combusted in a muffle furnace at 550°C until it turned to ash at a constant weight. The crucible was transferred to a desiccator and weighed after that (20). The percentage ash content was calculated as shown in equation I.
Where: CA = Weight of the crucible with ash, C = Weight of empty crucible, SW = Sample weight
2.3 Determination of total carbohydrate
The carbohydrate value was estimated using the formula in equation II below (21).
Where: P = Percentage protein, A = Percentage ash, M = Percentage moisture, F = Percentage fat
2.4 Determination of crude fat
Three grams of each sample was weighed on a filter paper and placed in an extraction thimble. Fat was extracted using petroleum ether in an extracting can as a solvent, with the aid of Foss soxtec 2055 fat extractor (22).
The percentage crude fat content was calculated as shown in equation III below
Where: CO = Weight of the extracting container with the extracting oil (solvent), C = Weight of the empty extracting container
2.5 Determination of tryptophan
About four grams of the prepared sample was hydrolyzed while in an alkaline state, barium hydroxide solvent was added and kept on heat at 100°C. The hydrolysate that resulted was evaluated using reverse-phase liquid chromatography with UV detection at 28 nm (23).
2.6 Determination of moisture content
Three grams of each sample was weighed into the moisture canister and dried on the oven (Memmert, GmbH, Model-30-750) till constant weight at a temperature of 105°C and then weighed again after cooling in a desiccator (24).
The percentage moisture was calculated as shown in equation IV below
Where: DLBD = Weight of sample, dish and dish lid before drying, DLAD = Weight of sample, dish and dish lid after drying, DL = Weight of empty dish and dish lid
2.7 Determination of crude protein
The protein content in the seeds was evaluated using the Kjeldahl method, this was done by weighing around 0.2 grams of the ground seed sample and digesting them before titration was carried out. A control referred to as a blank titre was prepared and the percentage protein content, as well as the protein content of each sample, was displayed on the automated machine screen. Foss Tecator™ Digestor and Kjectec 2200 distillation machine were used for the digestion and titration respectively.
2.8 Phytate and iron content
The phytic acid precipitation and extraction was carried out using the procedure of Wheeler and Ferrel (25). Thereafter, the iron content was quantified as described by Makeover 1970. Ratio four to six (4:6) Fe/p atomic ratio was adopted to determine the phytic acid value of the sample.
2.9 Tannin content determination
The tannin was determined using the method of Adegunwa et al. (26). About 0.5g of each sample was weighed and transferred into 50 ml distilled water and adequately mixed; the solution was left to stay at a temperature of 28°C for 30 minutes and filtered through No. 42 Whatman filter paper to obtain an extract. Two (2) ml of the obtained extract was transferred into a volumetric flask (50 ml). Standard solutions were also made using 2 ml each of distilled water and tannin solution. A saturated solution of Na2CO3 (2.5 ml) and Folins reagent was added to the sample and the standard solutions, and thereafter, they were filled up to a volume of 50 ml and incubated for 90 minutes at 28°C. The absorbance of the solution was measured in a spectrophotometer.
2.10 Data analysis
All data obtained from the study were subjected to Analysis of variance using (ANOVA) using SAS software 9.4 version at significance level p< 0.05. The means were separated using Fishers LSD.
3 Results
3.1 Nutrient contents in intercropped and monocropped AYB and WB
The ash content in the legume seeds ranged between 3.49-3.77% in AYB and 4.09-4.36% in WB (monocrop situation) and 3.03-4.47% (AYB) and 3.76-4.33% (WB) under intercropping condition (P ≤ 0.05). The moisture content in the legume seeds ranged between 11.04-11.56% in AYB and 9.24-10.66% in WB (monocrop situation) and 8.89-11.49% (AYB) and 8.95-9.75% (WB) under intercropping condition (Tables 1, 2). The fat content in the legume seeds ranged between 1.42-5.17% in AYB to 13.31-15.42% in WB (monocrop situation) and 1.19-4.56% (AYB) and 8.44-17.00% (WB) under intercropping condition. The protein content in the legume seeds ranged between 19.78-23.97% in AYB and 33.53-36.37% in WB (monocrop situation) and 18.56-35.23% (AYB) and 33.32-37.91% (WB) under intercropping condition. The tryptophan content in the legume seeds ranged between 0.12-0.17% in AYB and 0.55-0.82% in WB (monocrop situation) and 0.02-0.17% (AYB) and 0.55-0.82% (WB) under intercropping condition (P ≤ 0.05). The carbohydrate content in the legume seeds ranged between 57.98-63.79% in AYB and 35.18-37.11% in WB (monocrop situation) and 46.86-64.81% (AYB) and 34.08-43.37% (WB) under intercropping condition. There was a significant difference between the sole and intercropped AYB and WB in terms of their phytate, ash, fat, moisture, protein, tannin, moisture content, tryptophan and carbohydrates contents (Table 1 and 2). The moisture content of AYB accessions TSs4, TSs30, and TSs77 were lower when intercropped with M2. WB accession Tpt51 had a higher moisture content when intercropped with both maize accessions. Intercropping with both accessions of maize reduced the protein contents of AYB accessions TSs4 and TSs101, intercropping with M2 increased the protein content of AYB accession TSs30 and WB accession 15-4, while intercropping M1 with WB accession Tpt51 gave a higher protein content in the legume. Equally, intercropping reduced the tryptophan content in AYB accession TSs68, while WB accession Tpt12 had an increased tryptophan when solely cropped compared to when intercropped. Intercropping with M2 increased the carbohydrate content in WB accessions Tpt51 and 15-4, while intercropping with M1 increased the carbohydrate content in WB accession Tpt12 and AYB accession TSs30 (Table 1 and 2).
3.2 Anti-nutrient content in intercropped and monocropped AYB and WB
The phytate content in the seeds ranged between 3.44-4.68% in AYB and 3.37-6.56% in WB (monocrop situation) and 3.74-5.92% (AYB) (Table 1) and 3.74-8.07% (WB) (Table 2) under intercropping condition (P ≤ 0.05). The tannin contents in the seeds ranged between 0.05-2.0% in AYB and 0.12- 0.43% in WB (monocrop situation) and 0.10-0.32% (AYB) and 0.10-0.52% (WB) under intercropping condition. Intercropping with both maize accessions increased the phytate content in AYB accession TSs4 and WB accession 15-4, Tpt12, and Tpt32, while intercropping with M2 increased the phytate content of AYB accession TSs30 and WB accession Tpt51. Intercropping with both maize accessions reduce the tannin content in WB accession Tpt12. On the other hand, intercropping with both accessions of maize increased the tannin contents of AYB accessions TSs4, TSs101, and WB accessions 15-4, and Tpt32, while intercropping with both maize accessions reduced the tannin content in WB accession Tpt53. Intercropping with M2 increased the tannin content in WB accession Tpt51.
3.3 Nutrient contents of maize planted with urea fertilizer and intercropped maize
The tryptophan content in the maize grains on the AYB plots ranged between 0.03-0.05% in the monocropped grains and 0.05-3.93% in the intercropped grains (Table 3). The carbohydrate content in the maize grains on the AYB treatment ranged between 74.79-77.75% in the monocropped grains and 73.45-75.86% in the intercropped grains. The ash content in the maize grains on the AYB treatment ranged between 1.68-1.85% in the monocropped grains and 1.28-3.10% in the intercropped grains. The moisture content in the maize grains on the AYB treatment ranged between 8.05-8.98% in the monocropped grains and 7.16-8.18% in the intercropped grains. The fat content in the maize grains on the AYB treatment ranged between 2.77-3.75% in the monocropped grains and 4.02-5.96% in the intercropped grains. The protein content in the maize grains on the AYB treatment ranged between 9.75-10.64% in the monocropped grains and 10.30-12.11% in the intercropped grains (P ≤ 0.05).
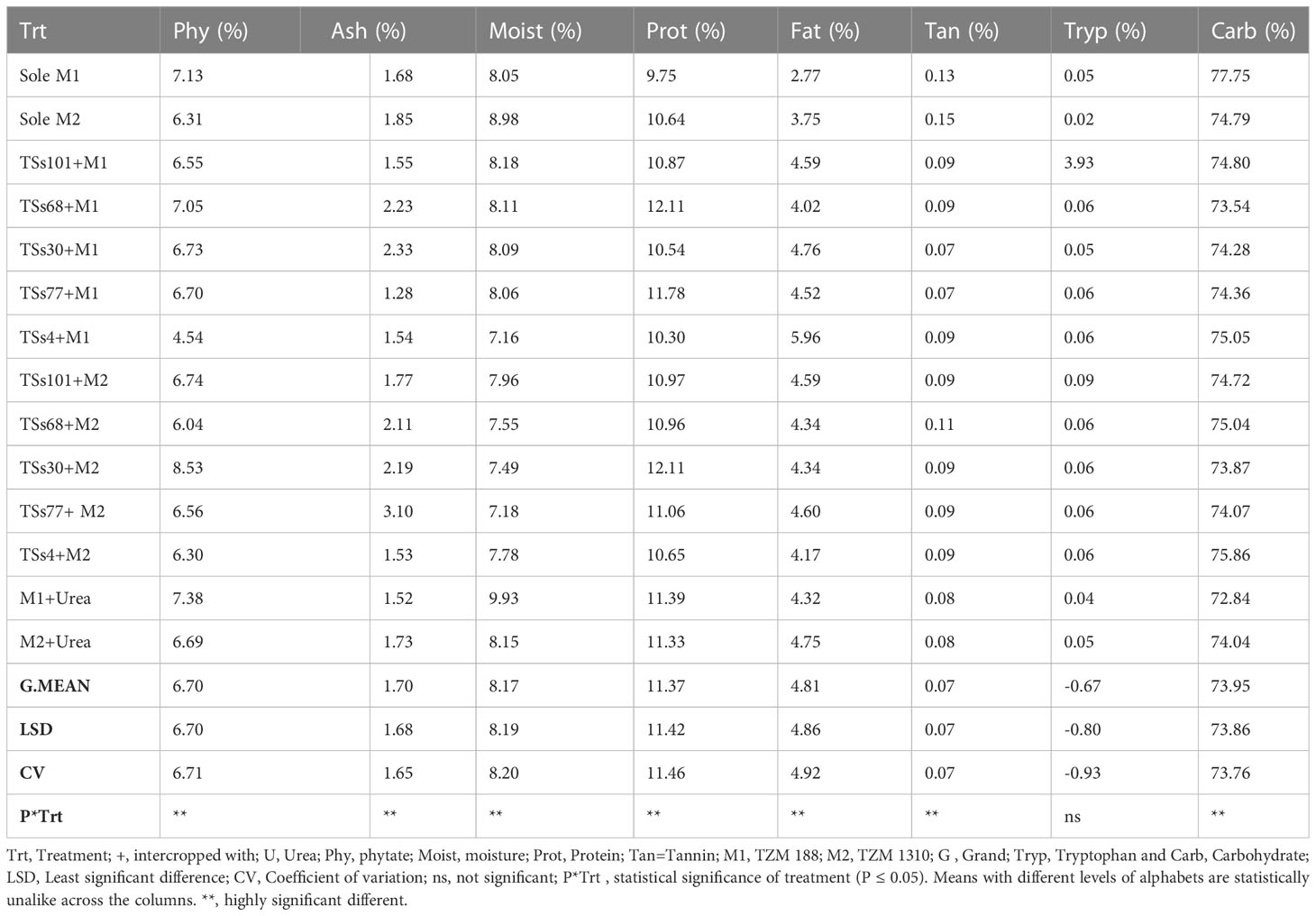
Table 3 Effect of intercropping AYB and urea fertilizer on the nutritional and anti-nutritional contents of maize.
On the AYB plots, the moisture content in both maize accessions were significantly higher when urea fertilizer was applied, which is directly opposite the result that was observed on the winged bean plots. The grains obtained when M1 was intercropped with AYB accessions TSs101 and TSs68 might not have a long shelf life compared to their sole counterparts because they had higher moisture content which could enhance microbial proliferation. The moisture content of the maize reduced when M2 was intercropped with AYB accessions TSs101, TSs4, TSs68 and TSs30. These are preferable to the formerly mentioned grains because they are less prone to microbial proliferation and spoilage; this consequently would increase their shelf life. Moisture content increased when M2 was intercropped with Tpt53. The protein content in both maize accessions when urea fertilizer was applied increased; this is due to the increase in the availability of nitrogen in the soil.
The tryptophan content in the maize seeds on the WB treatment ranged between 0.06-0.07% in the monocropped grains and 0.05-0.08% in the intercropped grains (Table 4). The carbohydrate content in the maize seeds on the WB treatment ranged between 72.46-73.96% in the monocropped grains and 66.35-75.31% in the intercropped grains (P ≤ 0.05). The ash content in the maize seeds on the WB treatment ranged between 1.37-1.50% in the monocropped grains and 1.30-1.58% in the intercropped grains.
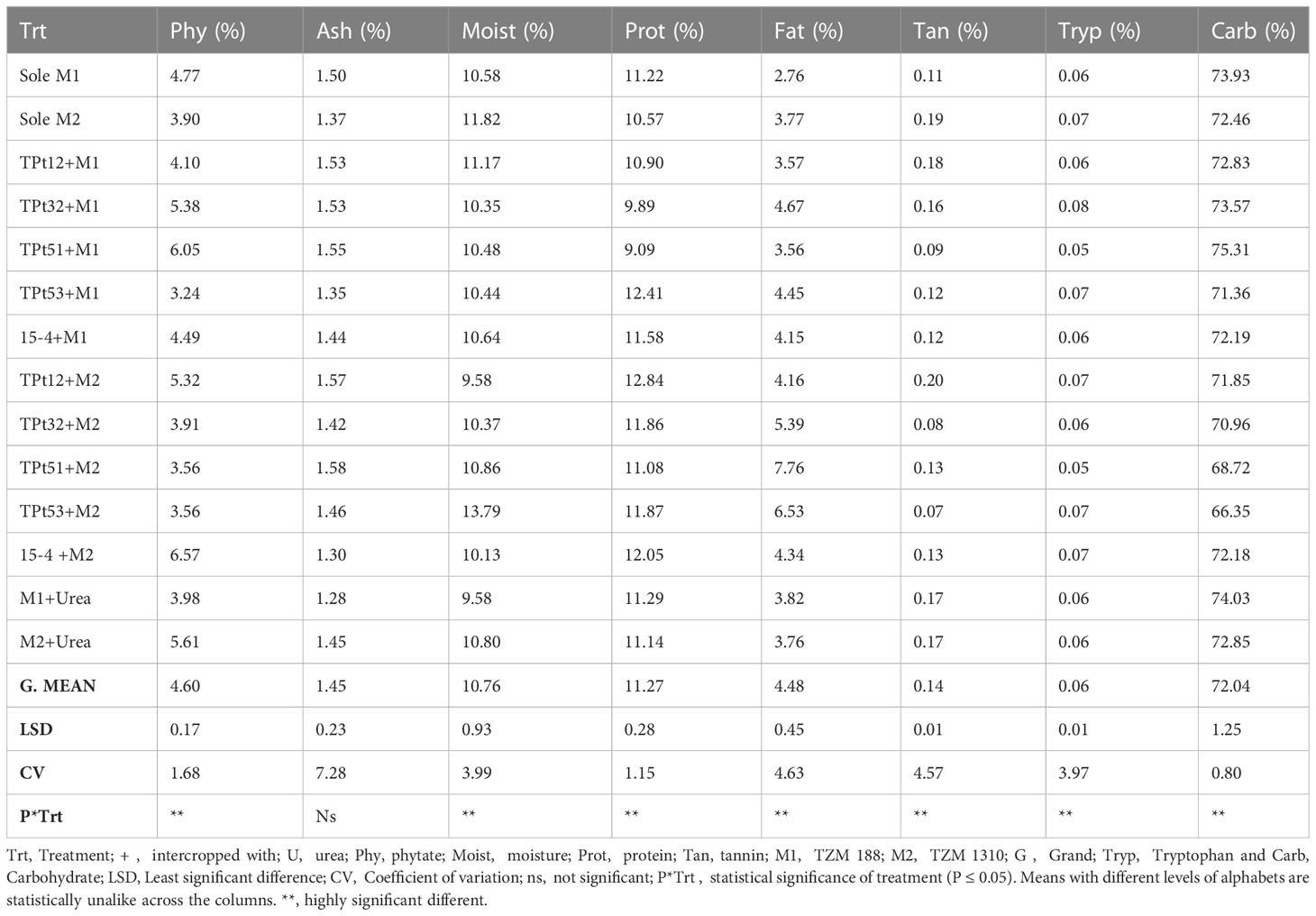
Table 4 Effect of intercropping WB and urea fertilizer on the nutritional and anti-nutritional contents of maize.
The moisture content in the maize seeds on the WB treatment ranged between 10.58-11.82% in the monocropped grains and 9.58-13.79% in the intercropped grains. The fat content in the maize seeds on the WB treatment ranged between 2.76-3.77% in the monocropped grains and 3.56-7.76% in the intercropped grains. The protein content in the maize seeds on the WB treatment ranged between 10.58- 11.52% in the monocropped grains and 9.09-12.84% in the intercropped grains (P ≤ 0.05).
On the WB plots, the protein content in M1 reduced when intercropped with Tpt12, Tpt32, and Tpt51, the fat content in M1 increased when urea fertilizer was applied but was not different between M2 in the sole and urea treatments. No reduction in the fat content of maize was observed when intercropped with all AYB and WB accessions; hence, intercropping had no detrimental effect on the fat content of maize (Table 3 and 4). The fat content in both maize accessions increased when intercropped with all AYB accessions. On the AYB plots, the tryptophan content in M1 and M2 were not affected by urea application and intercropping, except when M1 was intercropped with TSs101, where an increase in the tryptophan content was observed. On the WB experiment, M1 had an increased tryptophan when intercropped with Tpt32 and a reduced tryptophan content when intercropped with Tpt51 and 15-4, while M2 had a reduced tryptophan content when intercropped with Tpt51. On the WB plots, there was an increase in the carbohydrate content in M1 when intercropped with Tpt51 and there was a reduction in the carbohydrate content of M1 when intercropped with Tpt53 and 15-4. Equally, a reduction in the carbohydrate content was observed in M2 when it was intercropped with Tpt51, Tpt53, and Tpt32 (Table 4).
3.4 Anti-nutrients content of maize with urea fertilizer and intercropped maize
The phytate content in the maize grains on the AYB plots ranged between 6.31-7.13% in the monocropped grains and 4.54-8.53% in the intercropped grains (P ≤ 0.05). The tannin content in the maize grains on the AYB plots ranged between 0.13-0.15% in the monocropped grains and 0.07-0.11% in the intercropped grains. The tannin content in the maize seeds on the WB plot ranged between 0.11-1.19% in the monocropped grains and 0.07-0.20% in the intercropped grains. The phytate content in the maize seeds on the WB plots ranged between 3.90-4.77% in the monocropped grains and 3.24-6.57% in the intercropped grains. On the winged bean plots, the phytate content in sole M1 was higher than what was observed when urea fertilizer was applied, while the exact opposite was observed in M2. Furthermore, intercropping all AYB accessions with M1 and M2 reduced the tannin content in both accessions of maize. In WB, the tannin content in M2 reduced when intercropped with all WB accessions except in Tpt12. A reduction in tannin content was also observed when M1 was intercropped with Tpt51. However, when M1 was intercropped with WB accessions Tpt12 and Tpt32, the tannin content in the maize grains increased.
4 Discussions
Intercropping with M1 increased the phytate content in AYB accessions TSs101 and TSs77 as well as WB accession Tpt53, this implies that intercropping led to increased phytate content in some accessions and may result in the unavailability of some micronutrient, through chelation (27). The increase could be as a result of the beneath ground plant-plant interaction between the legumes and the maize (28). The range of the phytate content observed in the sole and intercropped treatments falls within the range (3.37-8.07%) which is similar to the values reported by Adegboyega et al. (8). Our result is different from the reports of Ajibola and Olapade (6), who reported a much lower value of phytate content in African yam bean seeds. Intercropping with both maize accessions reduced the ash content of AYB accession TSs4, intercropping with M1 reduced the ash content of AYB accession TSs101 and WB accession 15-4, while intercropping with M2 reduced the ash content in WB accessions Tpt51 and Tpt53; this could be because these accessions are less competitive in taking up minerals in an intercropping situation. This report is contrary to the reports of Iqbal et al. (2), who reported an increase in ash content during intercrop. The reduction in the ash content observed also could be because of the high phytate contents in these accessions, thereby leading to a reduction in the availability of the minerals since minerals are directly related to ash content (29).
TSs30 had a higher ash content when intercropped with M2, this result aligns closely with the result of Ajpas (30), who revealed that the ash content was higher in legumes when the planting ratio was 50:50, compared to the sole cropping. The ash content observed in all treatments of AYB and WB was less than five, which is lower than what was reported in the finding of Oner and Aykutlu (31). A reduction in the ash content could lead to a reduction in the nutrient value of the seeds. The moisture contents in AYB accessions TSs68 and WB accessions 15-4, Tpt12, Tpt32 and Tpt53 were not different when intercropped with both maize accessions, this aligns with the study of Ajpas (30). High moisture content could reduce the shelf life of seeds after harvest. Consequently, seeds after harvest must be properly handled (30), processed or stored to reduce food spoilage. Moisture content observed from all the WB seeds were within the stipulated standard of 10% which is recommended for a long shelf life of seeds (32), all seeds had a moisture content less than 10 except for the sole WB accession Tpt51 whose value was slightly higher (10.66%). The moisture content observed in AYB seeds were higher than those found in the WB and was above the recommended value stipulated by Olalekan and Bosede (32) except for accession TSs30 when intercropped with M2, which was lower (8.89%). The moisture contents which were found from the AYB seeds in our study were lower than that observed by Ajibola and Olapade (6), who reported a moisture content of 12.30 – 14.95%. Our results from both AYB and WB are different from the result of Adegboyega et al. (8) and (9), who observed lower moisture contents in WB seeds. Our finding further indicates that WB accession Tpt51 planted solely might not be able to withstand a longer shelf life due to its high moisture content. In contrast, AYB accession TSs30 intercropped with M1 might be have a longer shelf-life compared to other seeds. Intercropping with M1 reduced the protein content of WB accession Tpt12. This is lower than that observed in soybean (42.92 - 45.38%) (31). High protein in food depicts high nitrogen in the soil, which is incorporated into the plant tissue (33).
The protein content in WB was higher (above 30) than that observed in AYB; only TSs30 had a protein content higher than 30. This result is similar to the research of Adegboyega et al. (8), who observed protein contents with lowest value of 28.43 and highest value of 31.13%. The lower protein content found in AYB could be a result of the environment, weather, morphology and genetic makeup of the plant or other conditions that affect nitrogen availability and nutrient uptake. It could also be as a result of reduced nitrogen fixed; this aligns with the findings of Zorb et al. (34) who stated that when nitrogen availability is limited, it leads to a reduced amino acid synthesis and consequently a reduced protein content (34). WB had a remarkable higher fat content compared to AYB. The fat content in the monocropped seeds ranged between 1.43-5.18% in AYB, and 13.31-15.42% in WB and 1.19- 4.56% and 8.44-17.00% in intercropped AYB and WB, respectively, Ajibola and Olapade (6) recorded a range of 1.86-2.03% which is low compared to the range observed in our study. Intercropping reduced the fat content in WB accession Tpt51. Intercropping with M1 reduced the fat content in AYB accession TSs4 and intercropping with M2 reduced the fat content in AYB accession TSs77, our result is different from the work of Adegboyega et al. (8), whose result ranged from 13.87-19.01%; this is in the exception of Tpt51 and 15-4 when intercropped with M2. The fat content in AYB accession TSs101 and WB accessions Tpt12 and Tpt53 were higher when intercropped with both maize accessions; this result is similar to the findings of Biszczak et al. (35), who also observed an increased fat content in a legume-based intercrop. Intercropping with M2 increased the fat content of TSs4 and TSs30, intercropping with M1 increased the fat content in WB accession 15-4, this result is similar to the findings of Liu et al. (36), who also observed an increased fat content in intercropped soybean. These researchers claimed that their result was so as a result of the spatial photosynthetic activity radiance (PAR) which increased with reduced treatment. The increased fat content observed in these accessions could be because there was a little or no shading effects on them. The tannin contents in the monocropped seeds ranged between 0.05-0.15% in AYB, and 0.12-0.43% in WB and 0.10-0.33% and 0.10-0.52% in intercropped AYB and WB respectively, these values are higher than the values (0.0023-0.0026%) reported by Ajibola and Olapade (6).
All tannin contents recorded in this study was lower than that observed by Adegboyega et al. (8), who reported a tannin content value of 1.36-3.43% in winged bean. Intercropping with both maize accession increased the tryptophan content in WB accession Tpt51, while intercropping with M1 increased the tryptophan in AYB accession TSs77 and WB accessions Tpt 32, and Tpt53 (Table 1 and 2). Comai et al. (37) recorded a tryptophan of between 2.24 and 58.2% which is above the values obtained in this study. The difference in the carbohydrate content when AYB and WB accessions were monocropped and intercropped were not significant (Table 1 and 2), Ajibola and Olapade (6) reported values ranging between 59.29-59.79% which falls within the value observed in some accessions in this study. Intercropping with both maize accessions increased the carbohydrate content in AYB accessions TSs101 and TSs4, this is similar to the result obtained by Arshad et al. (38), who also recorded an increase in carbohydrate content when sorghum and legumes were intercropped. Generally, the carbohydrate value recorded in this study were higher than that recorded by Amoo et al. (7). Adegboyega et al. (8) reported the presence of carbohydrates in the range of 34.53 and 39.76% in AYB and WB. Intercropping with M2 did not favour AYB accessions TSs30, TSs77, and WB accessions Tpt32, and Tpt53 as it reduced the carbohydrate content, this result is similar to that of Myo et al. (39) who also observed a reduced carbohydrate in intercropped soybean owing to an increased shading effect.
For the maize, intercropping with TSs30 increased the phytate content (40), this implies that the maize produced in this treatment will contain less mineral content and have a lesser nutritional value. A significantly higher phytate content was observed in M1 when it was intercropped with Tpt32, Tpt51 and in M2 when it was intercropped with Tpt12 and 15-4, this could pose a challenge to the nutritional utilization of maize (41). A lower phytate content was observed in M1 when it was intercropped with Tpt12, Tpt53 and 15-4 and in M2 when it was intercropped with Tpt53 and Tpt51, this could be due to the farming practiced adopted (27). Though urea fertilizer application has been reported to improve the yield of maize Hu et al. (42), a reduction in the ash content observed in both maize accessions when urea fertilizer was applied could prove that urea fertilizer might not be efficient to enhance food security when viewed from the nutritional aspect. The ash content increased when M1 was intercropped with TSs30 and TSs68, and when M2 was intercropped with TSs77, this result is different from the report of Walters et al. (43), who reported that intercropping did not bring about a substantial difference in the mineral contents of intercropped species. The ash content of the maize reduced when M1 was intercropped with TSs101, TSs4 andTSs77, and when M2 was intercropped with TSs4, this aligns closely with the report of Nwadike and Saliu (44), who also observed a reduced ash content during intercropping. Ash depicts the mineral content and shows that the maize intercropped with these accessions were able to take up more nutrient from the soil compared with those in sole cropping, these legume accessions were capable of reducing the mineral components of maize, probably due to competition for nutrient in the soil. On the WB plot, intercropping and urea fertilizer application did not affect the ash content of both maize accessions. The effect of intercropping on the ash content of maize grains was accession dependent.
The moisture content of maize increased significantly when M1 was intercropped with TSs101 (8.13%) and TSs68 (8.11%), these values were lower than what was reported by Weinberg et al. (45) who observed a moisture content of 14 - 22% in harvested maize grains and further explained that maize grains within this range are capable of having a long shelf life due to their protection against microbial damage. Moisture content reduced when M2 was intercropped with Tpt12, Tpt51 and 15-4, this result is contrary to the result of Moussa et al. (46), who reported an increase in the moisture content of vegetables when urea fertilizer was applied. The protein content in M1 increased when intercropped with all AYB accessions and increased in M2 when it was intercropped with TSs30 and TSs77, this result aligns closely with the reports of Iqbal et al. (2), who also reported an increase in protein content during sorghum-legume intercropping. The protein content increased in M1 when intercropped with WB accessions Tpt53 and 15-4 and in M2 when intercropped with all WB accessions, this is similar to the reports of Gallo et al. (47), who also observed an increase in protein content when maize was intercropped with pigeon pea.
On the WB plot, fat content increased in M1 when intercropped with all WB accessions and increased in M2 only when intercropped with Tpt32, Tpt51, and 15-4, this implies that the maize was able to absorb more fat when intercropped with these WB accessions; the increase could be linked to underground plant-plant interactions that occurred during intercropping (48). When urea fertilizer was applied, the tannin content was reduced in M1 and increased in M2, this variability could be associated with the plant genetic composition. This result is different from the result of Hashim et al. (49) who observed no significant differences in sole maize and maize planted with chemical and bio-fertilizers. The tannin contents found in sole maize in the research carried out by Hashim et al. (49) was between 0.07 and 0.11; these values are close to the values observed in this study.
Some of the values obtained in this research (Table 3 and 4) are similar to those found in the study by Sarika et al. (50), who reported a tryptophan content in maize ranging between 0.027 – 0.117%. On the AYB experiment, intercropping and urea reduced the carbohydrate content in M1; this could be because the legumes and M1 competed for the soil carbon and also because the urea made nitrogen to be more available and made no changes to the urea content of the soil. The application of urea could lead to a reduction in total soluble sugars (carbohydrate) in grains (51–54). This is because sugars are needed in all energy-producing reactions (54). Intercropping with TSs4 increased the carbohydrate content in M2. Htet et al. (55) gave a report similar to this, where they also observed an increase in carbohydrate content of sole maize compared to their intercropped counterparts.
5 Conclusions
Intercropping has been reported to be a way of ensuring food security over the years as it helps to increase the overall productivity over a piece of land. From the results of this study, intercropping had both positive and negative effects on the nutrient and antinutrient parameters of the maize and legumes and these effects are accession dependent. For instance, positive effects such as an increase in the fat, ash, protein, carbohydrate, tryptophan, and a reduction in the phytate, moisture, tannin contents in some AYB, maize and WB accessions were observed. On the other hand, some negative effects were also linked to intercropping which include an increased phytate and moisture contents as well as a reduced fat, protein and carbohydrate content. Of all the legumes, AYB accession TSs30 responded better to intercropping by having a better nutritional value. This accession had an increased ash, protein, fat and carbohydrate contents as well as a reduced moisture content; hence, it should be further explored to ensure that the potentials embedded in it is maximally tapped into, in order enhance food security.
Data availability statement
The original contributions presented in the study are included in the article/supplementary material. Further inquiries can be directed to the corresponding author.
Author contributions
MSA carried out the fieldwork, processed the experimental data, interpreted the results, managed the literature searches, and wrote the first draft of the manuscript. MSA, OAO, MA, OO and OOB conceptualized the research, designed the study, reviewed and proofread the manuscript. All authors contributed to the article and approved the submitted version.
Funding
This research was funded by Global Crop Diversity Trust through Genetic Resources Center, International Institute of Tropical Agriculture, Ibadan, Nigeria.
Acknowledgments
The authors will like to appreciate the Genetic Resources Centre of the International Institute of Tropical Agriculture, who funded the research and also awarded a Graduate Research Fellowship award to MSA. In addition, the authors sincerely appreciate the North-West University, South Africa, for the Institutional bursary awarded to the first author. OOB also appreciate the National Research Foundation of South Africa for funding researches in her lab with grant number (UID123634 and UID132595).
Conflict of interest
The authors declare that the research was conducted in the absence of any commercial or financial relationships that could be construed as a potential conflict of interest.
Publisher’s note
All claims expressed in this article are solely those of the authors and do not necessarily represent those of their affiliated organizations, or those of the publisher, the editors and the reviewers. Any product that may be evaluated in this article, or claim that may be made by its manufacturer, is not guaranteed or endorsed by the publisher.
References
1. Gupta RK, Gangoliya SS, Singh NK. Reduction of phytic acid and enhancement of bioavailable micronutrients in food grains. J Food Sci Technol (2015) 52:676–84. doi: 10.1007/s13197-013-0978-y
2. Iqbal MA, Hamid A, Ahmad T, Siddiqui MH, Hussain I, Ali S, et al. Forage sorghum-legumes intercropping: effect on growth, yields, nutritional quality and economic returns. J Bragantia (2018) 78:82–95. doi: 10.1590/1678-4499.2017363
3. Popova A, Mihaylova D. Antinutrients in plant-based foods: a review. Open Biotechnol J (2019) 13(1):68–73. doi: 10.2174/1874070701913010068
4. Petroski W, Minich DM. Is there such a thing as “anti-nutrients”? a narrative review of perceived problematic plant compounds. Nutrients (2020) 12(10):2929. doi: 10.3390/nu12102929
5. Ndidi US, Ndidi CU, Olagunju A, Muhammad A, Billy FG, Okpe O. Proximate, antinutrients and mineral composition of raw and processed (Boiled and Roasted) Sphenostylis stenocarpa seeds from Southern Kaduna, Northwest Nigeria.. International Scholarly Research Notices 2014(1): 1–9. doi: 10.1155/2014/280837
6. Ajibola GO, Olapade AA. Physical, proximate and anti-nutritional composition of African yam bean (Sphenostylis stenocarpa) seeds varieties. J Food Res (2016) 5(2):67. doi: 10.5539/jfr.v5n2p67
7. Amoo I, Adebayo O, Oyeleye A. Chemical evaluation of winged beans (Psophocarpus tetragonolobus), pitanga cherries (Eugenia uniflora) and orchid fruit (Orchid fruit myristica). Afr J Food Agriculture Nutr Dev (2006) 6(2):1–12. doi: 10.4314/ajfand.v6i2.71734
8. Adegboyega TT, Abberton MT, AbdelGadir AAH, Dianda M, Maziya-Dixon B, Oyatomi OA, et al. Nutrient and antinutrient composition of winged bean (Psophocarpus tetragonolobus (L.) DC.) seeds and tubers. J Food Qual (2019) 2019:1–8. doi: 10.1155/2019/3075208
9. Soetan K. Antinutritional factors of five selected underutilized legumes. Food Sci Qual Manage (2018) 76:92–100.
10. Yang S, Grall A, Chapman MA. Origin and diversification of winged bean (Psophocarpus tetragonolobus (L.) DC.), a multipurpose underutilized legume. Am J Bot (2018) 105(5):888–97. doi: 10.1002/ajb2.1093
11. Sharma S, Barkauskaite S, Jaiswal AK, Jaiswal S. Essential oils as additives in active food packaging. Food Chem (2021) 343:128403. doi: 10.1016/j.foodchem.2020.128403
12. Hirobe T. Iron and skin health: iron stimulates skin function. Handb diet Nutr skin (2012) 1:196–214. doi: 10.3920/978-90-8686-729-5_12
13. Bilge G, Sezer B, Eseller KE, Berberoglu H, Koksel H, Boyaci IH. Ash analysis of flour sample by using laser-induced breakdown spectroscopy. Spectrochimica Acta Part B: Atomic Spectrosc (2016) 124:74–8. doi: 10.1016/j.sab.2016.08.023
14. Pike OA, O’Keefe S. Fat characterization. Food Anal (2017) 2017:407–29. doi: 10.1007/978-3-319-45776-5_23
15. Damodaran S. Food proteins: an overview. Food Proteins their Appl (2017) 1:1–24. doi: 10.1201/9780203755617-1
16. Friedman M. Analysis, nutrition, and health benefits of tryptophan. Int J Tryptophan Res (2018) 11:1178646918802282. doi: 10.1177/1178646918802282
17. Essack H, Odhav B, Mellem JJ. Screening of traditional South African leafy vegetables for specific anti-nutritional factors before and after processing.. Food Science and Technology (2017) 37:462–71.
18. Raza MA, Bin Khalid MH, Zhang X, Feng LY, Khan I, Hassan MJ, et al. Effect of planting patterns on yield, nutrient accumulation and distribution in maize and soybean under relay intercropping systems. Sci Rep (2019) 9(1):4947. doi: 10.1038/s41598-019-41364-1
19. Soe Htet MN, Hai J-B, Bo PT, Gong X-W, Liu C-J, Dang K, et al. Evaluation of nutritive values through comparison of forage yield and silage quality of mono-cropped and intercropped maize-soybean harvested at two maturity stages. Agriculture (2021) 11(5):452. doi: 10.3390/agriculture11050452
20. Liu K. Effects of sample size, dry ashing temperature and duration on determination of ash content in algae and other biomass. Algal Res (2019) 40:101486. doi: 10.1016/j.algal.2019.101486
21. Hailu Kassegn H. Determination of proximate composition and bioactive compounds of the Abyssinian purple wheat. Cogent Food Agric (2018) 4(1):1421415. doi: 10.1080/23311932.2017.1421415
22. Elife K, Akbaş P, Ceyhan G, Erdem TK, Alkan H. Determination the fatty acid composition of the rumex patientia l. leaves and in vitro antimicrobial activity of their different extracts. J.S.D.Ü.F.B.E.D (2020) 24(2):362–7. doi: 10.19113/sdufenbed.643154
23. Brooks EL, Mutengwa VS, Abdalla A, Yeoman MS, Patel BA. Determination of tryptophan metabolism from biological tissues and fluids using high performance liquid chromatography with simultaneous dual electrochemical detection. Analyst (2019) 144(20):6011–8. doi: 10.1039/C9AN01501A
24. Baiyeri S, Uguru M, Ogbonna P, Samuel-Baiyeri C, Okechukwu R, Kumaga F, et al. Evaluation of the nutritional composition of the seeds of some selected African yam bean (Sphenostylis stenocarpa hochst ex. a. rich (Harms)) accessions. Agro-Science (2018) 17(2):37–44. doi: 10.4314/as.v17i2.5
25. Wheeler E, Ferrel R. A method for phytic acid determination in wheat and wheat fractions. Cereal Chem (1971) 48(3):312–20.
26. Adegunwa M, Alamu E, Omitogun L. Effect of processing on the nutritional contents of yam and cocoyam tubers. J Appl Biosci (2011) 46:3086–92.
27. Silva EO, Bracarense APF. Phytic acid: from antinutritional to multiple protection factor of organic systems. J Food Sci (2016) 81(6):R1357–62. doi: 10.1111/1750-3841.13320
28. Nissar J, Ahad T, Naik H, Hussain S. A review phytic acid: as antinutrient or nutraceutical. Pharmacogn. Phytochem (2017) 6(6):1554–60.
29. Iqbal J, Lehri LA, Shah SM, Nadeem M. Combustion analysis of coal using coal water slurry technique. Eng Technol Res (2017) 2:49.
30. Ajpas A. Effects of spatial arrangements of groundnut-maize intercrop on growth, yield and proximate composition of groundnut. AJPAS J (2017) 5:1–7.
31. Oner F, Aykutlu H. The effect of maize-soybean intercropping systems on a set of technological and physiological properties. J Appl Ecol Environ Res (2019) 17(2):2149–65. doi: 10.15666/aeer/1702_21492165
32. Olalekan AJ, Bosede BF. Comparative study on chemical composition and functional properties of three Nigerian legumes (jack beans, pigeon pea and cowpea). J Emerging Trends Eng Appl Sci (2010) 1(1):89–95.
33. Firestone D. Official methods and recommended practices of the AOCS. Illinois, United States: AOCS (2009).
34. Zorb C, Ludewig U, Hawkesford MJ. Perspective on wheat yield and quality with reduced nitrogen supply. Trends Plant Sci (2018) 23(11):1029–37. doi: 10.1016/j.tplants.2018.08.012
35. Biszczak W, Różyło K, Kraska P. Yielding parameters, nutritional value of soybean seed and weed infestation in relay-strip intercropping system with buckwheat. Acta Agriculturae Scandinavica Section B—Soil Plant Sci (2020) 70(8):640–7. doi: 10.1080/09064710.2020.1831586
36. Liu J, Yang C-q, Zhang Q, Lou Y, Wu H-j, Deng J-c, et al. Partial improvements in the flavor quality of soybean seeds using intercropping systems with appropriate shading. Food Chem (2016) 207:107–14. doi: 10.1016/j.foodchem.2016.03.059
37. Comai S, Bertazzo A, Bailoni L, Zancato M, Costa CV, Allegri G. Protein and non-protein (free and protein-bound) tryptophan in legume seeds. Food Chem (2007) 103(2):657–61. doi: 10.1016/j.foodchem.2006.07.045
38. Arshad M, Nawaz R, Ahmad S, Shah GA, Faiz F, Ahmad N, et al. Growth, yield and nutritional performance of sweet sorghum and legumes in sole and intercropping influenced by type of legume, nitrogen level and air quality. Polish J Environ Stud (2020) 29(1):533–43. doi: 10.15244/pjoes/104461
39. Myo EM, Ge B, Ma J, Cui H, Liu B, Shi L, et al. Indole-3-acetic acid production by streptomyces fradiae NKZ-259 and its formulation to enhance plant growth. BMC Microbiol (2019) 19(1):1–14. doi: 10.1186/s12866-019-1528-1
40. Xia H, Wang L, Jiao N, Mei P, Wang Z, Lan Y, et al. Luxury absorption of phosphorus exists in maize when intercropping with legumes or oilseed rape–covering different locations and years. Agronomy (2019) 9(6):314. doi: 10.3390/agronomy9060314
41. Das A, Raychaudhuri U, Chakraborty R. Cereal based functional food of Indian subcontinent: a review. J Food Sci Technol (2012) 49(6):665–72. doi: 10.1007/s13197-011-0474-1
42. Hu H, Ning T, Li Z, Han H, Zhang Z, Qin S, et al. Coupling effects of urea types and subsoiling on nitrogen–water use and yield of different varieties of maize in northern China. Field Crops Res (2013) 142:85–94. doi: 10.1016/j.fcr.2012.12.001
43. Walters H, Carpenter-Boggs L, Desta K, Yan L, Matanguihan J, Murphy K. Effect of irrigation, intercrop, and cultivar on agronomic and nutritional characteristics of quinoa. Agroecol. Sustain Food Syst (2016) 40(8):783–803. doi: 10.1080/21683565.2016.1177805
44. Nwadike N, Saliu T. Effect of bambara nut and cowpea intercropped with maize at different times on nutritive quality of maize for ruminant feeding. Mal. J Anim. Sci (2017) 20:79–87.
45. Weinberg Z, Yan Y, Chen Y, Finkelman S, Ashbell G, Navarro S. The effect of moisture level on high-moisture maize (Zea mays l.) under hermetic storage conditions–in vitro studies. J Stored Products Res (2008) 44(2):136–44. doi: 10.1016/j.jspr.2007.08.006
46. Moussa MID, Alashi AM, Sossa-Vihotogbe CNA, Akponikpe PBI, Baco MN, Djènontin AJ, et al. Proximate composition, mineral profi le and trypsin-inhibitory activity of West African leafy vegetables: infl uence of urea micro-dosing and harvest time. Polish J Food Nutr Sci (2020) 70(2):179–88. doi: 10.31883/pjfns/119674
47. Gallo A, Fontanetti A, Guimarães N, Morinigo KP, de Souza MD, da Silva RF. Agronomic characteristics, nutritional status and yield of corn intercropped with dwarf pigeon pea in different spatial arrangements of plants. J.R.d.C.A (2018) 41(2):356–66. doi: 10.19084/RCA17297
48. Zuo Y, Zhang F, Li X, Cao Y. Studies on the improvement in iron nutrition of peanut by intercropping with maize on a calcareous soil. Plant Soil (2000) 220(1):13–25. doi: 10.1023/A:1004724219988
49. Hashim AF, Hatim AA, Somaya SM. Effects of chemical and bio-fertilizers on yield, yield components and grain quality of maize (Zea mays l.). Afr J Agric Res (2016) 11(45):4654–60. doi: 10.5897/AJAR2016.11619
50. Sarika K, Hossain F, Muthusamy V, Baveja A, Zunjare R, Goswami R, et al. Exploration of novel opaque16 mutation as a source for high-lysine and-tryptophan in maize endosperm. Indian J Genet (2017) 77(1):59–64. doi: 10.5958/0975-6906.2017.00008.6
51. Xia G, Cheng L. Foliar urea application in the fall affects both nitrogen and carbon storage in youngConcord’grapevines grown under a wide range of nitrogen supply. HortScience (2004) 39(4):827E–828. doi: 10.21273/HORTSCI.39.4.827E
52. Hirano T, Saito Y, Ushimaru H, Michiyama H. The effect of the amount of nitrogen fertilizer on starch metabolism in leaf sheath of japonica and indica rice varieties during the heading period. Plant production Sci (2005) 8(2):122–30. doi: 10.1626/pps.8.122
53. Pan J, Cui K, Wei D, Huang J, Xiang J, Nie L. Relationships of non-structural carbohydrates accumulation and translocation with yield formation in rice recombinant inbred lines under two nitrogen levels. Physiol. Plantarum (2011) 141(4):321–31. doi: 10.1111/j.1399-3054.2010.01441.x
54. Elhanafi L, Houhou M, Rais C, Mansouri I, Elghadraoui L, Greche H. Impact of excessive nitrogen fertilization on the biochemical quality, phenolic compounds, and antioxidant power of sesamum indicum l seeds. J Food Qual (2019) 2019:1–6. doi: 10.1155/2019/9428092
Keywords: carbohydrate, moisture content, nutrients, phytate, tannin
Citation: Ayilara MS, Abberton M, Oyatomi OA, Odeyemi O and Babalola OO (2023) Nutritional properties of underutilized legumes and intercropped maize. Front. Soil Sci. 3:1047847. doi: 10.3389/fsoil.2023.1047847
Received: 18 September 2022; Accepted: 27 April 2023;
Published: 10 May 2023.
Edited by:
Benedicta Essel Ayamba, Council for Scientific and Industrial Research (CSIR), GhanaReviewed by:
Jaime H. Mejías, Instituto de Investigaciones Agropecuarias, ChileDebasis Mitra, National Rice Research Institute (ICAR), India
Copyright © 2023 Ayilara, Abberton, Oyatomi, Odeyemi and Babalola. This is an open-access article distributed under the terms of the Creative Commons Attribution License (CC BY). The use, distribution or reproduction in other forums is permitted, provided the original author(s) and the copyright owner(s) are credited and that the original publication in this journal is cited, in accordance with accepted academic practice. No use, distribution or reproduction is permitted which does not comply with these terms.
*Correspondence: Michael Abberton, m.abberton@cgiar.org