- 1A Flinders Centre of Research Excellence, College of Medicine and Public Health, Adelaide Institute for Sleep Health, Flinders University, Daw Park, SA, Australia
- 2Neuroscience Research Australia, Randwick, NSW, Australia
- 3Sleep Health Service, Respiratory and Sleep Services, Southern Adelaide Local Health Network, Adelaide, SA, Australia
- 4The NHMRC Centre of Research Excellence, NEUROSLEEP, Woolcock Institute of Medical Research, The University of Sydney, Sydney, NSW, Australia
Sleep-disordered breathing (SDB), encompassing both obstructive and central sleep apnea, is prevalent in at least 50% of stroke patients. Small studies have shown vast improvements in post-stroke functional recovery outcomes after the treatment of SDB by continuous positive airway pressure. However, compliance to this therapy is very poor in this complex patient group. There are alternative therapy options for SDB that may be more amenable for use in at least some post-stroke patients, including mandibular advancement, supine avoidance, and oxygen therapy. There are few studies, however, that demonstrate efficacy and compliance with these alternative therapies currently. Furthermore, novel SDB-phenotyping approaches may help to provide important clinical information to direct therapy selection in individual patients. Prior to realizing individualized therapy, we need a better understanding of the pathophysiology of SDB in post-stroke patients, including the role of inherent phenotypic traits, as well as the contribution of stroke size and location. This review summarizes the available literature on SDB pathophysiology and treatment in post-stroke patients, identifies gaps in the literature, and sets out areas for further research.
Introduction
Sleep-disordered breathing (SDB) encompasses a range of respiratory sleep disorders which affects ~20% of the middle-aged population (1, 2). The known risk factors for SDB include obesity, age, and male gender (3). SDB is associated with a range of negative health outcomes including excessive daytime sleepiness leading to neurobehavioral and cognitive dysfunction (4), hypertension (5), and diabetes (6).
Stroke is one of the leading causes of morbidity and mortality. Stroke can occur ischemically, where there is a blockage of a cerebral artery, or hemorrhagically, where the cerebral artery has ruptured. Hemorrhagic strokes only comprise 15–20% of strokes, but account for almost half of stroke deaths. There is a strong link between SDB and stroke risk (7); however, the effectiveness of continuous positive airway pressure (CPAP), the gold-standard treatment of SDB, in reducing stroke risk is not supported. For example, a recent large randomized control trial (RCT) did not support CPAP as a risk reduction strategy for recurrent stroke in patients with moderate to severe OSA, although in highly compliant CPAP users, there may be some protective effect (8). SDB is more severe during the acute stroke phase; however, despite improvement over time, 53% of stroke patients demonstrate at least moderate–severe OSA after 1 month according to a meta-analysis (9). Post-stroke acute SDB, however, has received less attention in the literature, but there is emerging evidence that post-stroke SDB is very common and is associated with adverse effects, including poorer stroke recovery outcomes (10, 11). Emerging evidence, however, suggests that CPAP treatment in post-stroke patients may lead to faster functional recovery and reduction in the length of hospitalization and frequency of re-hospitalization (12–14).
It is important to improve our understanding of the pathophysiology of post-stroke SDB in order to initiate appropriate rehabilitation therapy to facilitate faster recovery in stroke patients. This review will briefly summarize and highlight the growing literature on post-stroke prevalence and the clinical presentation of SDB. Furthermore, with the increased use of neuroimaging and a greater understanding of SDB pathophysiology, recent studies have begun examining whether stroke lesion location and size are associated with SDB presentation. Lastly, we will review the emerging novel SDB-phenotyping approaches that may be clinically useful to better characterize the nature of SDB in post-stroke patients and help guide targeted post-stroke therapy.
Prevalence and Pathophysiology of SDB Post Stroke
The most common form of SDB is obstructive sleep apnea (OSA), which is the reduction (hypopnea) or cessation (apnea) of airflow during sleep as a consequence of upper-airway collapse. Another form of SDB is central sleep apnea (CSA), which is also characterized by cessation of respiration but as a consequence of loss in central respiratory drive and effort, rather than physical upper-airway collapse (Figure 1).
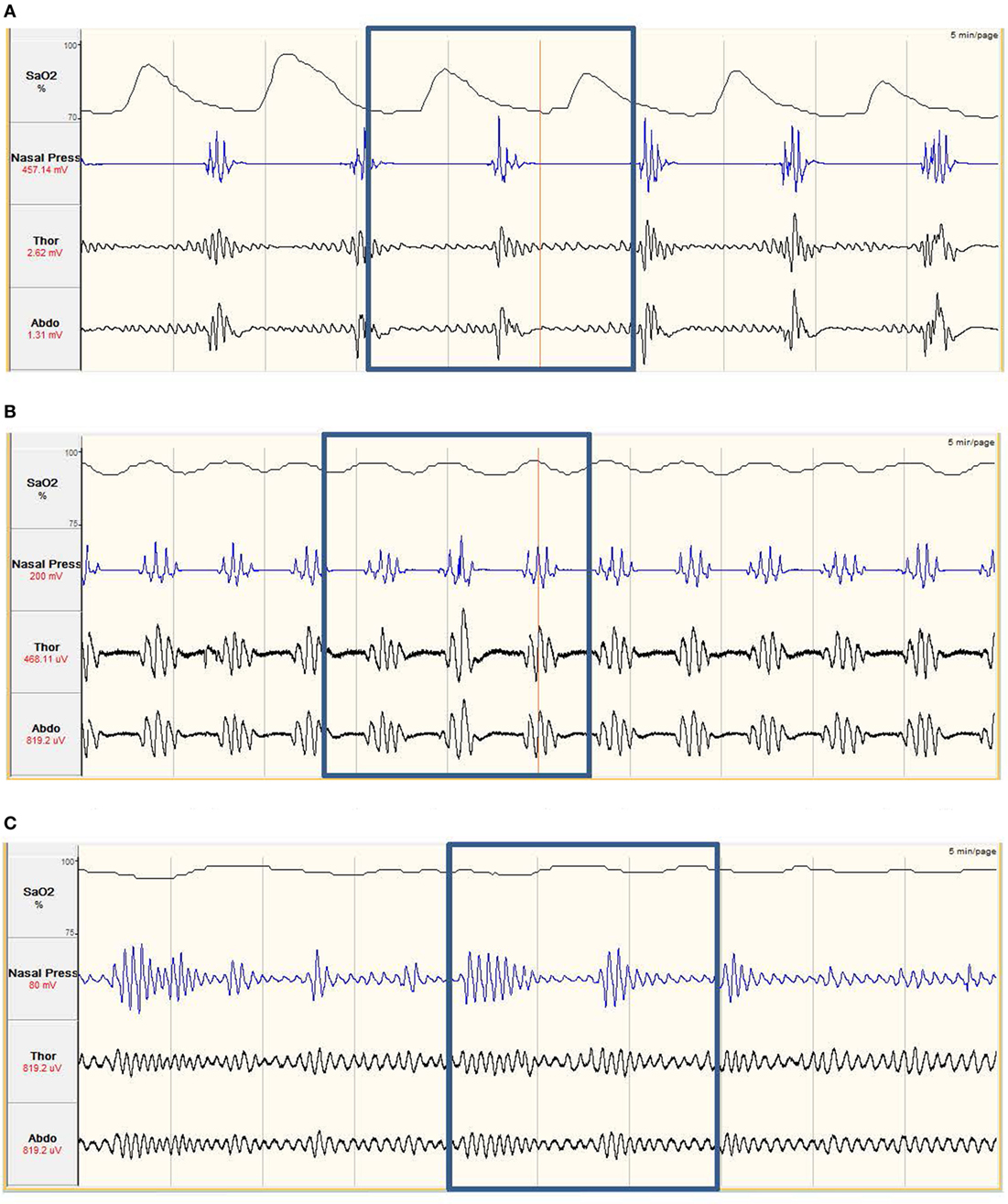
Figure 1. (A) Obstructive sleep apnea. The nasal pressure (second tracing from top) goes through periods of ‘flattening’, where airflow has ceased. The abdominal and thoracic respiratory bands (third and fourth tracing) show continued effort to breath, with effort increasing prior to the recovery of breathing. The continued respiratory effort with no airflow implies the airway has collapsed. The oxygen saturation (SaO2%, top tracing) shows periods of desaturation. The desaturation, and recovery, is delayed compared to the respiratory effort. Obstructive apneas occur multiple times in the example. (B) Central sleep apnea. The nasal pressure (second tracing from top) goes through periods of ‘flattening’, where airflow has ceased. This is combined with the abdominal and thoracic respiratory bands, which are also ‘flattening’, showing no effort to breathe. This combination of no airflow and no effort to breathe imply the neural drive to breathe is impaired. Central apneas occur multiple times in the example and also result in periods of SaO2% (top tracing) desaturation. (C) Hypopnea. The nasal pressure shows periods of increased and decreased breathing, coupled with increased and decreased respiratory effort. As airflow is still maintained but leads to decreases in SaO2% (top tracing) of at least 3%, this is classed as a hypopnea.
The gold-standard diagnosis for SDB is performed by overnight laboratory polysomnography (PSG), comprising electroencephalography (EEG) and respiratory measures (pulse oximetry, nasal pressure, and respiratory effort). PSG, however, can be cumbersome and time-consuming, making it impractical in some clinical settings such as post-stroke (15). As such, the vast majority of studies examining SDB and stroke use respiratory polygraphy, which is a limited channel sleep study measuring respiratory parameters without EEG. There is good agreement between the limited channel studies compared with gold-standard PSG (16, 17) suggesting that the prevalence estimates for SDB post stroke are reasonable.
Prevalence of SDB after Stroke Compared to Prevalence in Non-Stroke Patients
There is cross-sectional evidence suggesting that SDB is more common among stroke patients, compared with the general population, with a recent meta-analysis showing that SDB affects >50% of stroke patients (9). Furthermore, studies have demonstrated that stroke patients experience a higher prevalence of CSA (>5 respiratory events/hour) compared with non-stroke SDB patients (18–20).
An important limiting factor in this area is determining whether there was preexisting SDB prior to the stroke and how this may influence post-stroke acute SDB pathophysiology. Few studies have used questionnaires to quantify snoring prevalence prior to the stroke (21, 22), and there are screening questionnaires for SDB that may provide information on whether SDB was present prior to the stroke. Nevertheless, the use of these questionnaires would be subject to recall bias. Ultimately, unless there has been a prior objective clinical diagnosis of SDB, it is very difficult to determine the relationship between prior and post-stroke SDB.
Treatment of SDB Improves Clinical Recovery in Stroke Patients
Untreated SDB in stroke patients leads to numerous adverse outcomes during acute and chronic recovery from stroke including higher long-term mortality (23–25) and reduced functional outcomes (10, 25, 26).
Traditionally, as the majority of SDB events in the general population are obstructive in nature, this was considered to be caused by a collapsible airway (27, 28). CPAP acts as a pneumatic splint via the application of pressurized air to prevent airway collapse. There are different types of positive airway pressure (PAP) machines that can operate at a set pressure, or other machines that can automatically detect the pressure needed to maintain airway patency (known as an auto-CPAP, or APAP). CPAP usage of more than 4 h a night, for at least 70% of nights, is considered clinically compliant (29). Adaptive servo-ventilation (ASV) has in the past been used to treat CSA; however, a recent study has questioned the efficacy of this treatment (30). Furthermore, there has been no research examining the effects of ASV in post-stroke SDB patients.
Successful treatment of SDB by both CPAP (13) and APAP (12) in stroke patients has been shown to improve NIH Stroke Scale. In addition, treatment has been shown to reduce the risk of mortality by 32% (14). Yet, despite the well-documented benefits of PAP, compliance to therapy in general populations remains poor, with several studies showing an average nightly usage of less than 4 h a night, and in many cases, less than half the nights of the week. Furthermore, up to 50% of patients abandon CPAP treatment in the long term (8, 31). Many of the reasons for low compliance in the general population include mask discomfort, anxiety, and difficulty using CPAP therapy (32, 33). Compliance with CPAP appears to be even worse in stroke patients with SDB, with uptake in stroke populations regularly below 50% (23, 34). Furthermore, those that do use CPAP often use it for less than 4 h/night (34–36).
Despite the prevalence of CSA in stroke patients, no study has attempted to treat CSA directly in SDB patients. This is further exacerbated by an RCT highlighting that the previous treatment strategy of CSA, ASV, provided no protection against CSA-related death (30). While CSA is not caused by a collapsed airway, CPAP has been shown to have some efficacy in reducing CSA; however, this is due to addressing non-stroke causes in CSA, such as decreased left-ventricular ejection fraction (37, 38). Thus, it is yet unclear whether CPAP would benefit stroke-related CSA. Nevertheless, given the documented benefits of the treatment of SDB on the functional recovery of stroke patients, it is imperative to develop strategies and care pathways to motivate and encourage SDB treatment in stroke patients. These may include the use of non-CPAP-related treatments in conjunction with novel respiratory phenotyping techniques, which may be more suitable and acceptable for stroke populations during recovery in both hospital and home environments.
Other Potential Treatments for Post-Stroke SDB
The development of non-CPAP treatments for SDB has been growing. Diet and physical exercise to reduce obesity has shown promise to reduce the severity of SDB in non-stroke populations (39, 40); however, as in general populations, it is difficult to achieve and maintain in non-stroke patients (41, 42). The functional impairment caused by stroke, such as immobility, means weight loss is difficult to implement. Furthermore, during the post-stroke period, stroke patients with variable degrees of hemiparesis often lose muscle mass but increase fat mass (43), leading to obesity, which can worsen SDB.
Obstructive sleep apnea is often more severe in the supine position compared to that in lateral postures (44). Recent technological developments in supine avoidance devices for OSA have shown promising results in terms of efficacy in reducing OSA severity as well as treatment adherence (45, 46). A small RCT involving SDB stroke patients using a supine avoidance pillow showed a significant decrease in apnea/hypopnea index (AHI) while using the pillow; however, larger studies are needed to properly elucidate the effect of supine avoidance on SDB in stroke patients (47). Furthermore, this technique may not be suitable for all stroke patients, particularly those with hemiparesis, as they may not be able to shift their body weight to, or maintain, a lateral position.
Mandibular advancement splints (MAS) move the mandible forward, thereby opening the airway and are effective at reducing OSA in 30–50% of patients (48). This therapy may be beneficial in some stroke patients and may be better suited and tolerated in stroke populations compared to CPAP treatment; however, MAS has not been tested in stroke patients thus far.
Surgical interventions to reduce OSA severity have shown promise in non-stroke populations; however, studies are lacking in this area (49). Furthermore, given the potential complications from stroke, surgery may not be a feasible option.
Oxygen therapy has also been evaluated as a potential treatment option in OSA (50) and would seem to be an appropriate, minimally invasive therapy in post-stroke patients with SDB. The effectiveness of oxygen therapy may be limited, however, with evidence suggesting that it is effective at significantly reducing oxygen desaturation, but may prolong apneic events (50). More recent evidence suggests that only certain OSA patients with particular phenotypic traits respond positively to oxygen therapy (51), highlighting the need for future research on simple phenotyping and therapies in post-stroke patients.
Despite the availability of various treatment options for OSA, there has been very limited research examining the acceptance and efficacy of non-CPAP therapies on improving SDB and functional recovery in stroke patients.
Phenotyping SDB in Stroke Patients to Inform Novel Treatment Strategies
There are several nonanatomical contributors to SDB, being a low-respiratory arousal threshold, abnormal chemoreflex response to differing carbon dioxide (CO2) levels, and low activation of the main upper-airway dilator muscle, the genioglossus (52). This knowledge has allowed for the development of emerging “tailored” treatments for those that have these nonanatomical contributors to SDB. Low arousal threshold can be treated by the use of sedative (53), abnormal chemoreflex has been treated by supplementary oxygen (54), and low activation of the upper-airway dilator muscles can be improved by targeted oral exercises (55). Importantly, these SDB phenotypes can be identified from diagnostic PSG studies (56, 57); however, the characterization of these phenotypes has not been performed in post-stroke SDB patients.
Does Stroke Lesion Size and Location Impact SDB Presentation?
The three nonanatomical contributors to SDB are controlled through the brainstem. Thus, it is biologically plausible that stroke lesions that involve the brainstem may contribute to SDB. This may explain why stroke patients experience higher rates of SDB (58). If stroke lesion location does contribute to SDB, this knowledge, combined with SDB phenotype information, could potentially be used clinically to inform the optimal mode of therapy for a particular patient.
Neural Centers of Respiratory Control
Within the brainstem, the medulla oblongata plays the primary role in respiratory control (59). The medulla oblongata contains central chemoreceptors (with peripheral chemoreceptors located in the carotid bodies). The chemoreflex response controls respiration through a loop-gain feedback system depending on the partial pressure of CO2 detected (PaCO2). In people with “normal” chemosensitivity, an increase in PaCO2 will increase respiration, while a decrease in PaCO2 will reduce respiration. In those with “abnormal” chemosensitivity, increases in PaCO2 lead to above normal increase in ventilation, which can contribute to CSA (60). Lesions to the respiratory centers within the medulla have shown decreased chemosensitivity during wakefulness, sleep, and even exercise (61).1
The medulla also receives input from the respiratory center within the pons to innervate the pharyngeal muscles, which play an important role in maintaining the patency of the upper airway, as well as the regulation of diaphragm activity. The largest pharyngeal muscle, the genioglossus, is innervated by the hypoglossal nerve, which originates from the brainstem (62) and acts to pull the tongue forward. SDB patients experience a reduction in genioglossus activation during sleep, which importantly contributes to upper-airway collapsibility (63, 64). Several studies have shown that brainstem strokes affect pharyngeal muscle activity (65, 66) causing dysphagia and can contribute to higher rates and severity of SDB observed in stroke patients. This implies that stroke-induced damage to the respiratory brain regions innervating the genioglossus muscle activity may, at least in part, explain the increase in upper-airway collapsibility in stroke patients.
Lesion Location
Severity and Type of SDB
Early studies examining brainstem strokes showed lesions to this region led to the development of Cheyne-Stokes respiration (periodic breathing occuring whilst awake) (67, 68). Lee et al. was the first to show high levels of CSA in patients who had suffered a brainstem lesion (67). Several case–control studies suggest that there is a link between brainstem stroke and SDB severity and in particular increased CSA severity (69, 70). The limited research in this area, however, means larger prospective studies are needed to make strong conclusion regarding this relationship.
The advent of neuroimaging has allowed for a more detailed examination of the potential role that lesion location might have on SDB in stroke patients. These studies have produced conflicting findings on whether stroke in the brainstem contributes to SDB and whether it contributes to specific types of SDB (obstructive vs central). Infratentorial lesions, which encompass both the brainstem and cerebellum, have been shown to generally result in higher AHI (20, 71) when compared to cortical lesions. Likewise, another study (18) has shown that when compared to cortical strokes, brainstem strokes have been associated with three times greater odds of significant SDB, particularly CSA, along with greater nocturnal desaturations (26). No study has yet examined whether brainstem lesions are associated with changes in respiratory arousal threshold. Given that a low arousal threshold contributes to unstable respiratory control and reduces the likelihood of entering slow-wave sleep, while a high arousal threshold may exacerbate arterial hypoxemia, it is important to determine whether stroke in the brainstem changes arousal threshold.
By contrast, other studies have found that non-brainstem regions might be more related to SDB in stroke patients. For example, Siccoli et al. found that patients who experience total anterior circulatory strokes had the highest AHI compared to other brain regions assessed, with CSA making up 40% of the overall AHI (19). Furthermore, the authors showed that the second highest AHI resulted from strokes in the pons, which is part of the midbrain, where CSA made up only 12% of AHI events. Ahn et al. found that bilateral cerebral lesions were associated with significantly higher SDB severity, while stroke in other regions, including the brainstem, was not associated with SDB, and the authors did not report on the type of SDB (72). De Paolis et al. have hypothesized that cerebral infarctions can lead to increased cranial pressure leading to a reduction in cerebral blood volume, which would precipitate hypocapnia, leading to unstable ventilatory control and CSA (73). This hypothesis may partly explain why another study (74) has showed a spontaneous decrease in SDB, in particular CSA after 3 months, despite not showing SDB severity or the type to be associated with lesion location.
Several studies have also shown that there is no association between lesion location and SDB severity and SDB type (74–78); however, the reasons for this are unclear. It is important to note that brainstem-specific strokes contribute to less than 10% of the number of cortical strokes, limiting the power to demonstrate lesion location impact on SDB (18, 77).
In summary, it appears that the literature on lesion location and SDB is mixed and inconclusive. There is large variation in methodology and patient populations, lesion location definitions, and even the timing of the SDB measurement relative to stroke onset, which may contribute to conflicting results.
Brainstem and Pharyngeal Muscles
Despite the importance of the pharyngeal muscles in maintaining upper-airway patency, there has been relatively little research directly examining the potential role of damage to the pharyngeal nerves in post-stroke SDB patients, despite studies showing both brainstem lesions (79) and lesions to the pharyngeal cortex (80), leading to dysphagia. Turkington et al. showed that BMI and neck circumference, but not the presence of dysphagia, contributed to worse SDB severity (81). Brown et al. showed that stroke patients with SDB had a narrower retropalatal distance, which is the size of the opening of the airway, compared to non-SDB. Importantly, dysphagia was not different between SDB and non-SDB stroke patients (82). By contrast, Martinez-Garcia et al. showed that stroke patients with brainstem stroke were 1.73 times more likely to experience dysphagia and a significantly higher number of obstructive apnea events compared to stroke patients without dysphagia during the acute phase (83). Furthermore, stroke patients with dysphagia experienced larger reductions in AHI, particularly obstructive apneas, during recovery.
Lesion Size
Few studies have examined how the size of stroke, regardless of whether the stroke in hemorrhagic or ischemic, effects SDB prevalence and type. Ahn et al. demonstrated that bilateral hemisphere lesions resulted in a significantly higher SDB severity compared to strokes that occurred in a single area (72). By contrast, Brown et al. compared brainstem to cortical strokes and showed that the lesion size was not associated with the severity of SDB (18). Siccoli et al. found that the worst SDB severity occurred with total anterior circulatory stroke, implying that the lesion size was a significant contributor to SDB severity (19). Yet, lesions occurring in the pons, a specific area of the brainstem, resulted in the second largest AHI, questioning whether the size of stroke is a contributing factor.
Differences between Ischemic and Hemorrhagic Strokes on SDB Severity
Only a single study has examined SDB differences between ischemic and hemorrhagic strokes (78). SDB severity immediately post stroke was similar between ischemic and hemorrhagic strokes, but after 3 months, SDB severity remained unchanged in ischemic stroke, but was significantly reduced for those who experienced hemorrhagic stroke. The type of SDB was not recorded; thus, it is difficult to determine whether stroke type leads to differences in SDB type from this study. The authors suggest that the observed reduction in SDB in the hemorrhagic stroke group after 3 months may have been due to reductions in intracerebral pressure (73). More research is needed on the impact of stroke type on SDB as it appears that this information might be clinically meaningful when evaluating prognosis and therapy options in post-stroke SDB.
Discussion
This review has highlighted that post-stroke SDB is significantly more prevalent compared to non-stroke populations. This is likely driven by lesion damage to respiratory control neurocircuitry in the brainstem and/or the cortex, and due to the complexity of these regions, the causes and the resulting type of SDB differ significantly between individual stroke patients. The literature on lesion location and size is limited and mixed with some studies reporting a link between brainstem stroke and SDB, in particular CSA, while others show cortical strokes to be more important and yet others showing no association between lesion location and SDB. It is too early to make strong conclusions on this, and further research is necessary to determine the link between stroke location/size and SDB.
Although SDB may reduce and resolve in severity in many patients over time, in parallel with functional recovery after stroke, SDB can significantly hinder and delay post-stroke rehabilitation, resulting in increased hospitalization and slower recovery. Importantly, the small number of studies that have examined the therapeutic effect of CPAP on recovery outcomes in stroke patients has reported significantly faster functional recovery, reduced hospitalization time, and frequency of re-hospitalization. This provides good rationale for attempting to treat post-stroke SDB to improve clinical outcomes, but clearly using CPAP in this patient group presents significant challenges due to disabling and varying impact of stroke, leading to limited CPAP adherence and use in hospital environment. There are several alternate options to treat SDB, such as MAS and supine avoidance that may be suitable in some patients to at least reduce SDB severity. New SDB-phenotyping techniques are being developed, which will inform regarding the nature and cause of SDB in individual patients, and as a result, new therapies are being developed to target phenotypic causes of SDB to provide tailored treatment for individual patients. Although at present many of these phenotyping techniques are complex and experimental, making them out of scope for clinical use, there are efforts to utilize simple sleep recordings from oximetry and flow measurements suitable for in-hospital assessment. These new phenotyping and therapy developments together with stroke location and size data could provide important clinical information to help prove SDB therapy in post-stroke and improve clinical outcomes for patients. In conclusion, the relative lack of studies examining SDB in the aftermath of stroke, along with the discrepancies in findings, highlights the need for more research in this area. Specifically, research links lesion location to SDB phenotypes toward informing what drives SDB post stroke in individual patients and guiding the choice of SDB treatment and stroke rehabilitation.
Author Contributions
DS: manuscript concept, preparation, write-up, reviewing, and editing; RM: manuscript reviewing and editing; SM: manuscript reviewing and editing; AV: manuscript concept, preparation, write-up, reviewing, and editing.
Conflict of Interest Statement
The authors declare that the research was conducted in the absence of any commercial or financial relationships that could be construed as a potential conflict of interest.
Footnote
- ^Patients in this study had focal lesions to the rostrolateral medulla; however, this study did not specify how the lesions occurred.
References
1. Adams R, Appleton S, Taylor A, McEvoy D, Wittert G. Are the ICSD-3 criteria for sleep apnoea syndrome too inclusive? Lancet Respir Med (2016) 4(5):e19–20. doi:10.1016/S2213-2600(16)00109-0
2. Heinzer R, Vat S, Marques-Vidal P, Marti-Soler H, Andries D, Tobback N, et al. Prevalence of sleep-disordered breathing in the general population: the HypnoLaus study. Lancet Respir Med (2015) 3(4):310–8. doi:10.1016/S2213-2600(15)00043-0
3. Eckert DJ, Malhotra A. Pathophysiology of adult obstructive sleep apnea. Proc Am Thorac Soc (2008) 5(2):144–53. doi:10.1513/pats.200707-114MG
4. Beebe DW. Neurobehavioral effects of obstructive sleep apnea: an overview and heuristic model. Curr Opin Pulm Med (2005) 11(6):494–500. doi:10.1097/01.mcp.0000183059.52924.39
5. Appleton SL, Vakulin A, Martin SA, Lang CJ, Wittert GA, Taylor AW, et al. Hypertension is associated with undiagnosed OSA during rapid eye movement sleep. Chest (2016) 150(3):495–505. doi:10.1016/j.chest.2016.03.010
6. Appleton SL, Vakulin A, McEvoy RD, Wittert GA, Martin SA, Grant JF, et al. Nocturnal hypoxemia and severe obstructive sleep apnea are associated with incident type 2 diabetes in a population cohort of men. J Clin Sleep Med (2015) 11(6):609–14. doi:10.5664/jcsm.4768
7. Redline S, Yenokyan G, Gottlieb DJ, Shahar E, O’Connor GT, Resnick HE, et al. Obstructive sleep apnea–hypopnea and incident stroke: the sleep heart health study. Am J Respir Crit Care Med (2010) 182(2):269–77. doi:10.1164/rccm.200911-1746OC
8. McEvoy RD, Antic NA, Heeley E, Luo Y, Ou Q, Zhang X, et al. CPAP for prevention of cardiovascular events in obstructive sleep apnea. N Engl J Med (2016) 375(10):919–31. doi:10.1056/NEJMoa1606599
9. Johnson KG, Johnson DC. Frequency of sleep apnea in stroke and TIA patients: a meta-analysis. J Clin Sleep Med (2010) 6(2):131–7.
10. Kaneko Y, Hajek VE, Zivanovic V, Raboud J, Bradley TD. Relationship of sleep apnea to functional capacity and length of hospitalization following stroke. Sleep (2003) 26(3):293–7. doi:10.1093/sleep/26.3.293
11. Mansukhani MP, Bellolio MF, Kolla BP, Enduri S, Somers VK, Stead LG. Worse outcome after stroke in patients with obstructive sleep apnea: an observational cohort study. J Stroke Cerebrovasc Dis (2011) 20(5):401–5. doi:10.1016/j.jstrokecerebrovasdis.2010.02.011
12. Bravata DM, Concato J, Fried T, Ranjbar N, Sadarangani T, McClain V, et al. Continuous positive airway pressure: evaluation of a novel therapy for patients with acute ischemic stroke. Sleep (2011) 34(9):1271–7. doi:10.5665/SLEEP.1254
13. Minnerup J, Ritter MA, Wersching H, Kemmling A, Okegwo A, Schmidt A, et al. Continuous positive airway pressure ventilation for acute ischemic stroke: a randomized feasibility study. Stroke (2012) 43(4):1137–9. doi:10.1161/STROKEAHA.111.637611
14. Martínez-García MÁ, Soler-Cataluña JJ, Ejarque-Martínez L, Soriano Y, Román-Sánchez P, Illa FB, et al. Continuous positive airway pressure treatment reduces mortality in patients with ischemic stroke and obstructive sleep apnea: a 5-year follow-up study. Am J Respir Crit Care Med (2009) 180(1):36–41. doi:10.1164/rccm.200808-1341OC
15. Collop NA, Anderson WM, Boehlecke B, Claman D, Goldberg R, Gottlieb DJ, et al. Clinical guidelines for the use of unattended portable monitors in the diagnosis of obstructive sleep apnea in adult patients. J Clin Sleep Med (2007) 3(7):737–47.
16. Santos-Silva R, Sartori DE, Truksinas V, Truksinas E, Alonso FFFD, Tufik S, et al. Validation of a portable monitoring system for the diagnosis of obstructive sleep apnea syndrome. Sleep (2009) 32(5):629–36. doi:10.1093/sleep/32.5.629
17. Zou D, Grote L, Peker Y, Lindblad U, Hedner J. Validation a portable monitoring device for sleep apnea diagnosis in a population based cohort using synchronized home polysomnography. Sleep (2006) 29(3):367–74. doi:10.1093/sleep/29.3.367
18. Brown DL, McDermott M, Mowla A, De Lott L, Morgenstern LB, Kerber KA, et al. Brainstem infarction and sleep-disordered breathing in the BASIC sleep apnea study. Sleep Med (2014) 15(8):887–91. doi:10.1016/j.sleep.2014.04.003
19. Siccoli MM, Valko PO, Hermann DM, Bassetti CL. Central periodic breathing during sleep in 74 patients with acute ischemic stroke—neurogenic and cardiogenic factors. J Neurol (2008) 255(11):1687–92. doi:10.1007/s00415-008-0981-9
20. Bassetti C, Aldrich MS, Quint D. Sleep-disordered breathing in patients with acute supra- and infratentorial strokes—a prospective study of 39 patients. Stroke (1997) 28(9):1765–72. doi:10.1161/01.STR.28.9.1765
21. Palomaki H. Snoring and the risk of ischemic brain infarction. Stroke (1991) 22(8):1021–5. doi:10.1161/01.STR.22.8.1021
22. Spriggs D, French J, Murdy J, Curless R, Bates D, James O. Snoring increases the risk of stroke and adversely affects prognosis. QJM (1992) 83(4):555–62.
23. Bassetti CL, Milanova M, Gugger M. Sleep-disordered breathing and acute ischemic stroke: diagnosis, risk factors, treatment, evolution, and long-term clinical outcome. Stroke (2006) 37(4):967–72. doi:10.1161/01.STR.0000208215.49243.c3
24. Dyken ME, Somers VK, Yamada T, Ren ZY, Zimmerman MB. Investigating the relationship between stroke and obstructive sleep apnea. Stroke (1996) 27(3):401–7. doi:10.1161/01.STR.27.3.401
25. Turkington PM, Allgar V, Bamford J, Wanklyn P, Elliott MW. Effect of upper airway obstruction in acute stroke on functional outcome at 6 months. Thorax (2004) 59(5):367–71. doi:10.1136/thx.2003.005348
26. Good DC, Henkle JQ, Gelber D, Welsh J, Verhulst S. Sleep-disordered breathing and poor functional outcome after stroke. Stroke (1996) 27(2):252–9. doi:10.1161/01.STR.27.2.252
27. Lowe AA, Gionhaku N, Takeuchi K, Fleetham JA. Three-dimensional CT reconstructions of tongue and airway in adult subjects with obstructive sleep apnea. Am J Orthod Dentofacial Orthop (1986) 90(5):364–74. doi:10.1016/0889-5406(86)90002-8
28. Solow B, Skov S, Ovesen J, Norup PW, Wildschiødtz G. Airway dimensions and head posture in obstructive sleep apnoea. Eur J Orthod (1996) 18(6):571–9. doi:10.1093/ejo/18.6.571
29. Rotenberg BW, Murariu D, Pang KP. Trends in CPAP adherence over twenty years of data collection: a flattened curve. J Otolaryngol Head Neck Surg (2016) 45(1):43. doi:10.1186/s40463-016-0156-0
30. Cowie MR, Woehrle H, Wegscheider K, Angermann C, D’Ortho MP, Erdmann E, et al. Adaptive servo-ventilation for central sleep apnea in systolic heart failure. N Engl J Med (2015) 373(12):1095–105. doi:10.1056/NEJMoa1506459
31. Marin JM, Carrizo SJ, Vicente E, Agusti AGN. Long-term cardiovascular outcomes in men with obstructive sleep apnoea–hypopnoea with or without treatment with continuous positive airway pressure: an observational study. Lancet (2005) 365(9464):1046–53. doi:10.1016/S0140-6736(05)74229-X
32. McArdle N, Devereux G, Heidarnejad H, Engleman HM, Mackay TW, Douglas NJ. Long-term use of CPAP therapy for sleep apnea/hypopnea syndrome. Am J Respir Crit Care Med (1999) 159(4 I):1108–14. doi:10.1164/ajrccm.159.4.9807111
33. Zozula R, Rosen R. Compliance with continuous positive airway pressure therapy: assessing and improving treatment outcomes. Curr Opin Pulm Med (2001) 7(6):391–8. doi:10.1097/00063198-200111000-00005
34. Hsu CY, Vennelle M, Li HY, Engleman HM, Dennis MS, Douglas NJ. Sleep-disordered breathing after stroke: a randomised controlled trial of continuous positive airway pressure. J Neurol Neurosurg Psychiatry (2006) 77(10):1143–9. doi:10.1136/jnnp.2005.086686
35. Aaronson JA, Hofman WF, Van Bennekom CAM, Van Bezeij T, Van Den Aardweg JG, Groet E, et al. Effects of continuous positive airway pressure on cognitive and functional outcome of stroke patients with obstructive sleep apnea: a randomized controlled trial. J Clin Sleep Med (2016) 12(4):533–41. doi:10.5664/jcsm.5684
36. Hui DS, Choy DK, Wong LK, Ko FW, Li TS, Woo J, et al. Prevalence of sleep-disordered breathing and continuous positive airway pressure compliance: results in Chinese patients with first-ever ischemic stroke. Chest (2002) 122(3):852–60. doi:10.1378/chest.122.3.852
37. Arzt M, Floras JS, Logan AG, Kimoff RJ, Series F, Morrison D, et al. Suppression of central sleep apnea by continuous positive airway pressure and transplant-free survival in heart failure: a post hoc analysis of the Canadian continuous positive airway pressure for patients with central sleep apnea and heart failure trial (CANPAP). Circulation (2007) 115(25):3173–80. doi:10.1161/CIRCULATIONAHA.106.683482
38. Egea CJ, Aizpuru F, Pinto JA, Ayuela JM, Ballester E, Zamarrón C, et al. Cardiac function after CPAP therapy in patients with chronic heart failure and sleep apnea: a multicenter study. Sleep Med (2008) 9(6):660–6. doi:10.1016/j.sleep.2007.06.018
39. Foster GD, Borradaile KE, Sanders MH, Millman R, Zammit G, Newman AB, et al. A randomized study on the effect of weight loss on obstructive sleep apnea among obese patients with type 2 diabetes: the sleep AHEAD study. Arch Intern Med (2009) 169(17):1619–26. doi:10.1001/archinternmed.2009.266
40. Kuna ST, Reboussin DM, Borradaile KE, Sanders MH, Millman RP, Zammit G, et al. Long-term effect of weight loss on obstructive sleep apnea severity in obese patients with type 2 diabetes. Sleep (2013) 36(5):641–9. doi:10.5665/sleep.2618
41. Jeffery RW, Epstein LH, Wilson GT, Drewnowski A, Stunkard AJ, Wing RR, et al. Long-term maintenance of weight loss: current status. Health Psychol (2000) 19(1 Suppl):5–16. doi:10.1037/0278-6133.19.Suppl1.5
42. Ulen CG, Huizinga MM, Beech B, Elasy TA. Weight regain prevention. Clin Diabetes (2008) 26(3):100–13. doi:10.2337/diaclin.26.3.100
43. Jorgensen L, Jacobsen BK. Changes in muscle mass, fat mass, and bone mineral content in the legs after stroke: a 1 year prospective study. Bone (2001) 28(6):655–9. doi:10.1016/S8756-3282(01)00434-3
44. Walsh JH, Leigh MS, Paduch A, Maddison KJ, Armstrong JJ, Sampson DD, et al. Effect of body posture on pharyngeal shape and size in adults with and without obstructive sleep apnea. Sleep (2008) 31(11):1543–9. doi:10.1093/sleep/31.11.1543
45. Van Maanen JP, De Vries N. Long-term effectiveness and compliance of positional therapy with the sleep position trainer in the treatment of positional obstructive sleep apnea syndrome. Sleep (2014) 37(7):1209–15. doi:10.5665/sleep.3840
46. Eijsvogel MM, Ubbink R, Dekker J, Oppersma E, De Jongh FH, Van Der Palen J, et al. Sleep position trainer versus tennis ball technique in positional obstructive sleep apnea syndrome. J Clin Sleep Med (2015) 11(2):139–47. doi:10.5664/jcsm.4460
47. Svatikova A, Chervin RD, Wing JJ, Sanchez BN, Migda EM, Brown DL. Positional therapy in ischemic stroke patients with obstructive sleep apnea. Sleep Med (2011) 12(3):262–6. doi:10.1016/j.sleep.2010.12.008
48. Lim J, Lasserson TJ, Fleetham J, Wright J. Oral appliances for obstructive sleep apnoea. Cochrane Database Syst Rev (2006) 1:CD004435. doi:10.1002/14651858.CD004435.pub3
49. Kotecha BT, Hall AC. Role of surgery in adult obstructive sleep apnoea. Sleep Med Rev (2014) 18(5):405–13. doi:10.1016/j.smrv.2014.02.003
50. Mehta V, Vasu TS, Phillips B, Chung F. Obstructive sleep apnea and oxygen therapy: a systematic review of the literature and meta-analysis. J Clin Sleep Med (2013) 9(3):271–9. doi:10.5664/jcsm.2500
51. Landry SA, Joosten SA, Sands SA, White DP, Malhotra A, Wellman A, et al. Response to a combination of oxygen and a hypnotic as treatment for obstructive sleep apnoea is predicted by a patient’s therapeutic CPAP requirement. Respirology (2017) 22(6):1219–24. doi:10.1111/resp.13044
52. Eckert DJ, White DP, Jordan AS, Malhotra A, Wellman A. Defining phenotypic causes of obstructive sleep apnea: identification of novel therapeutic targets. Am J Respir Crit Care Med (2013) 188(8):996–1004. doi:10.1164/rccm.201303-0448OC
53. Carter SG, Berger MS, Carberry JC, Bilston LE, Butler JE, Tong BKY, et al. Zopiclone increases the arousal threshold without impairing genioglossus activity in obstructive sleep apnea. Sleep (2016) 39(4):757–66. doi:10.5665/sleep.5622
54. Wellman A, Malhotra A, Jordan AS, Stevenson KE, Gautam S, White DP. Effect of oxygen in obstructive sleep apnea: role of loop gain. Respir Physiol Neurobiol (2008) 162(2):144–51. doi:10.1016/j.resp.2008.05.019
55. Guimarães KC, Drager LF, Genta PR, Marcondes BF, Lorenzi-Filhoy G. Effects of oropharyngeal exercises on patients with moderate obstructive sleep apnea syndrome. Am J Respir Crit Care Med (2009) 179(10):962–6. doi:10.1164/rccm.200806-981OC
56. Landry SA, Joosten SA, Eckert DJ, Jordan AS, Sands SA, White DP, et al. Therapeutic CPAP level predicts upper airway collapsibility in patients with obstructive sleep apnea. Sleep (2017) 40(6). doi:10.1093/sleep/zsx056
57. Joosten SA, Landry SA, Sands SA, Terrill PI, Mann D, Andara C, et al. Dynamic loop gain increases upon adopting the supine body position during sleep in patients with obstructive sleep apnoea. Respirology (2017) 22(8):1662–9. doi:10.1111/resp.13108
58. Nogués MA, Roncoroni AJ, Benarroch E. Breathing control in neurological diseases. Clin Auton Res (2002) 12(6):440–9. doi:10.1007/s10286-002-0067-1
59. Pattinson KTS, Mitsis GD, Harvey AK, Jbabdi S, Dirckx S, Mayhew SD, et al. Determination of the human brainstem respiratory control network and its cortical connections in vivo using functional and structural imaging. Neuroimage (2009) 44(2):295–305. doi:10.1016/j.neuroimage.2008.09.007
60. Eckert DJ, Jordan AS, Merchia P, Malhotra A. Central sleep apnea: pathophysiology and treatment. Chest (2007) 131(2):595–607. doi:10.1378/chest.06.2287
61. Morrell MJ, Heywood P, Moosavi SH, Guz A, Stevens J. Unilateral focal lesions in the rostrolateral medulla influence chemosensitivity and breathing measured during wakefulness, sleep, and exercise. J Neurol Neurosurg Psychiatry (1999) 67(5):637–45. doi:10.1136/jnnp.67.5.637
62. Smith JC, Goldberg SJ, Shall MS. Phenotype and contractile properties of mammalian tongue muscles innervated by the hypoglossal nerve. Respir Physiol Neurobiol (2005) 147:253–62. doi:10.1016/j.resp.2005.02.016
63. Patil SP, Schneider H, Marx JJ, Gladmon E, Schwartz AR, Smith PL. Neuromechanical control of upper airway patency during sleep. J Appl Physiol (2007) 102(2):547–56. doi:10.1152/japplphysiol.00282.2006
64. Sands SA, Eckert DJ, Jordan AS, Edwards BA, Owens RL, Butler JP, et al. Enhanced upper-airway muscle responsiveness is a distinct feature of overweight/obese individuals without sleep apnea. Am J Respir Crit Care Med (2014) 190(8):930–7. doi:10.1164/rccm.201404-0783OC
65. Barer DH. The natural history and functional consequences of dysphagia after hemispheric stroke. J Neurol Neurosurg Psychiatry (1989) 52(2):236–41. doi:10.1136/jnnp.52.2.236
66. Gordon C, Hewer RL, Wade DT. Dysphagia in acute stroke. Br Med J (1987) 295(6595):411–4. doi:10.1136/bmj.295.6595.411
67. Lee MC, Klassen AC, Heaney LM, Resch JA. Respiratory rate and pattern disturbances in acute brain stem infarction. Stroke (1976) 7(4):382–5. doi:10.1161/01.STR.7.4.382
68. Nachtmann A, Siebler M, Rose G, Sitzer M, Steinmetz H. Cheyne-stokes respiration in ischemic stroke. Neurology (1995) 45(4):820–1. doi:10.1212/WNL.45.4.820
69. Askenasy JJ, Goldhammer I. Sleep apnea as a feature of bulbar stroke. Stroke (1988) 19:637–9. doi:10.1161/01.STR.19.5.637
70. Faludi B, Tóth M, Pusch G, Komoly S. Dynamic changes in sleep-related breathing abnormalities in bilateral paramedian mesencephalon and thalamus stroke: a follow-up case study. Sleep and Breathing (2016) 20:237–42. doi:10.1007/s11325-015-1212-0
71. Manconi M, Zavalko I, Cereda C, Pisarenco I, Ott S, Fulda S, et al. Longitudinal polysomnographic assessment from acute to subacute phase in infratentorial versus supratentorial stroke. Cerebrovasc Dis (2014) 37(2):85–93. doi:10.1159/000356323
72. Ahn SH, Kim JH, Kim DU, Choo IS, Lee HJ, Kim HW. Interaction between sleep-disordered breathing and acute ischemic stroke. J Clin Neurol (2013) 9(1):9–13. doi:10.3988/jcn.2013.9.1.9
73. De Paolis F, Colizzi E, Milioli G, Grassi A, Riccardi S, Parrino L, et al. Acute shift of a case of moderate obstructive sleep apnea syndrome towards one of severe central sleep apnea syndrome after an ischemic stroke. Sleep Med (2012) 13(6):763–6. doi:10.1016/j.sleep.2012.01.012
74. Parra O, Arboix A, Bechich S, García-Eroles L, Montserrat JM, López JA, et al. Time course of sleep-related breathing disorders in first-ever stroke or transient ischemic attack. Am J Respir Crit Care Med (2000) 161(2 I):375–80. doi:10.1164/ajrccm.161.2.9903139
75. Fisse AL, Kemmling A, Teuber A, Wersching H, Young P, Dittrich R, et al. The association of lesion location and sleep related breathing disorder in patients with acute ischemic stroke. PLoS One (2017) 12(1):e0171243. doi:10.1371/journal.pone.0171243
76. Nopmaneejumruslers C, Kaneko Y, Hajek V, Zivanovic V, Bradley TD. Cheyne-stokes respiration in stroke: relationship to hypocapnia and occult cardiac dysfunction. Am J Respir Crit Care Med (2005) 171(9):1048–52. doi:10.1164/rccm.200411-1591OC
77. Stahl SM, Yaggi HK, Taylor S, Qin L, Ivan CS, Austin C, et al. Infarct location and sleep apnea: evaluating the potential association in acute ischemic stroke. Sleep Med (2015) 16(10):1198–203. doi:10.1016/j.sleep.2015.07.003
78. Szucs A, Vitrai J, Janszky J, Migleczi G, Bodizs R, Halasz P, et al. Pathological sleep apnoea frequency remains permanent in ischaemic stroke and it is transient in haemorrhagic stroke. Eur Neurol (2002) 47(1):15–9. doi:10.1159/000047941
79. Martin JH, Diamond B, Aviv JE, Sacco RL, Keen MS, Zagar D, et al. Supraglottic and pharyngeal sensory abnormalities in stroke patients with dysphagia. Ann Otol Rhinol Laryngol (1996) 105(2):92–7. doi:10.1177/000348949610500202
80. Michou E, Mistry S, Jefferson S, Singh S, Rothwell J, Hamdy S. Targeting unlesioned pharyngeal motor cortex improves swallowing in healthy individuals and after dysphagic stroke. Gastroenterology (2012) 142(1):29–38. doi:10.1053/j.gastro.2011.09.040
81. Turkington PM, Bamford J, Wanklyn P, Elliott MW. Prevalence and predictors of upper airway obstruction in the first 24 hours after acute stroke. Stroke (2002) 33(8):2037–42. doi:10.1161/01.STR.0000023576.94311.27
82. Brown DL, Bapuraj JR, Mukherji SK, Chervin RD, Concannon M, Helman JI, et al. MRI of the pharynx in ischemic stroke patients with and without obstructive sleep apnea. Sleep Med (2010) 11(6):540–4. doi:10.1016/j.sleep.2010.01.008
Keywords: stroke, sleep apnea, hypopnea, treatment, phenotyping
Citation: Stevens D, Martins RT, Mukherjee S and Vakulin A (2018) Post-Stroke Sleep-Disordered Breathing—Pathophysiology and Therapy Options. Front. Surg. 5:9. doi: 10.3389/fsurg.2018.00009
Received: 02 December 2017; Accepted: 01 February 2018;
Published: 26 February 2018
Edited by:
Haralampos Gouveris, Universitätsmedizin der Johannes Gutenberg-Universität Mainz, GermanyReviewed by:
Nikolaus Ernst Wolter, Hospital for Sick Children, CanadaBoguslaw Mikaszewski, Gdańsk Medical University, Poland
Copyright: © 2018 Stevens, Martins, Mukherjee and Vakulin. This is an open-access article distributed under the terms of the Creative Commons Attribution License (CC BY). The use, distribution or reproduction in other forums is permitted, provided the original author(s) and the copyright owner are credited and that the original publication in this journal is cited, in accordance with accepted academic practice. No use, distribution or reproduction is permitted which does not comply with these terms.
*Correspondence: Andrew Vakulin, YW5kcmV3LnZha3VsaW5AZmxpbmRlcnMuZWR1LmF1