- 1Department of Orthopedics, The Seventh Medical Center of General Hospital of People's Liberation Army (PLA), Beijing, China
- 2Institute of Integrative Medicine, Shaanxi University of Chinese Medicine, Xi'an, China
- 3Department of Stomatology, The Specialty Medical Center Rocket Force of People's Liberation Army (PLA), Beijing, China
- 4Department of Orthopedics, Baoji Central Hospital, Baoji, China
- 5Department of Cardiac Surgery, Xijing Hospital, Air Force Medical University, Xi'an, China
- 6Department of Orthopedics, Yongchuan Hospital of Chongqing Medical University, Chongqing, China
Lumbar disc herniation is among the common phenotypes of degenerative lumbar spine diseases, significantly affecting patients' quality of life. The practice pattern is diverse. Choosing conservative measures or surgical treatments is still controversial in some areas. For those who have failed conservative treatment, surgery with or without instrumentation is recommended, causing significant expenditures and frustrating complications, that should not be ignored. In the article, we performed a literature review and summarized the evidence by subheadings to unravel the cons of surgical intervention for lumbar disc herniation. There are tetrad critical issues about surgical treatment of lumbar disc herniation, i.e., favorable natural history, insufficient evidence in a recommendation of fusion surgery for patients, metallosis, and implant removal. Firstly, accumulating evidence reveals immune privilege and auto-immunity hallmarks of human lumbar discs within the closed niche. Progenitor cells within human discs further expand the capacity with the endogenous repair. Clinical watchful follow-up studies with repeated diagnostic imaging reveal spontaneous resolution for lumbar disc herniation, even calcified tissues. Secondly, emerging evidence indicates long-term complications of lumbar fusion, such as adjacent segment disease, pseudarthrosis, implant failure, and sagittal spinal imbalance, which get increasing attention. Thirdly, systemic and local reactions (metallosis) for metal instrumentation have been noted with long-term health concerns and toxicity. Fourthly, the indications and timing for spinal implant removal have not reached a consensus. Other challenging issues include postoperative lumbar stiffness. The review provided evidence from a negative perspective for surgeons and patients who attempt to choose surgical treatment. Collectively, the emerging underlying evidence questions the benefits of traditional surgery for patients with lumbar disc herniation. Therefore, the long-term effects of surgery should be closely observed. Surgical decisions should be made prudently for each patient.
Introduction
As one of the most burdensome health issues globally, low back pain (LBP) causes vast expenditures in treatment and sick leave from work (1). According to the Global Burden of Disease Study 2013, LBP is one of the most common musculoskeletal diseases amongst 301 acute and chronic diseases and injuries based on data from 188 countries during 1990–2013 (2). Degenerative diseases of the intervertebral discs, such as lumbar disc herniation (LDH, MeSH: intervertebral disc displacement), represent part of the most common causes of LBP (3). The prevalence of LDH is 2% in the general population (4) and 1.42% in adolescents (5), according to SweSpine. Except for the presence of cauda equina syndrome, plegia, and sensory-motoric deficits, controversy still exists regarding the indications for surgical intervention.
Whether to choose conservative measures or surgical treatments is still controversial for LDH in some areas (6). The traditional surgical procedures of LDH are various according to the disease, including pure decompression, decompression with non-instrumented fusion, decompression with instrumented fusion, minimally invasive decompression with fusion, decompression associated with a dynamic stabilization system, etc. Patients with isolated herniated lumbar discs causing radiculopathy are recommended to undergo the primary disc excision operation, such as open discectomy, endoscopic discectomy, or laminectomy in the guideline. Lumbar spinal fusion is not recommended as a routine treatment for these patients. However, lumbar spinal fusion is recommended for patients with herniated discs who have severe degenerative changes or obvious intersegmental instability caused by the herniated discs. Besides, reoperative discectomy and fusion is a potential treatment option in patients with recurrent disc herniations associated with significant deformity, instability, or chronic axial low back pain (7). Reoperative discectomy and fusion are believed superior in minimizing mechanical instability and recurrence compared to reoperative discectomy for the recurrent cases (8).
A retrospective study consisting of 18,590 patients with LDH who underwent surgical treatment showed that open discectomy was the most common procedure (68.9%) in the primary operation, followed by endoscopic discectomy (16.1%), laminectomy (7.9%), fusion (3.9%), and nucleolysis (3.2%) (9). Although pure decompression is the most recommended surgical procedure for the purely herniated with neurological symptoms, the reoperation rates were considerably high, with 18.6, 13.8, and 12.4% after laminectomy, open discectomy, and endoscopic discectomy, respectively (9). As a matter of fact, removing part of the lumbar disc might induce a secondary complex situation that can bring spinal instability (10). And it turns out an initial lumbar discectomy for the patients with LDH is statistically associated with an increased likelihood of lumbar fusion in the future (11).
The selection of treatment strategy for LDH should be based on the severity of the disease and the patient's overall condition (12). Whether to choose non-surgical or surgical treatment and which surgical procedure is selected depends on the severity of symptoms and the clinical-pathological correlate. However, the decision-making of treatment strategy is partially preference-sensitive, depending on the surgeon's preference, which is influenced by the doctors' experience and patients' expectations.
Although surgical treatment has been demonstrated as an effective treatment strategy with the advantages, such as rapid symptoms relief, increased stability, facilitated bone healing, and restored alignment, several disadvantages or complications in the long term have also been noted (13). These complications, i.e., adjacent segment degeneration, metallosis, and additional ionizing radiation exposure, have been widely reported in the last two decades. There is accumulating evidence that questions the benefits of traditional surgery for patients with LDH (14, 15). Given that the pros of surgical treatment have been well-documented in the literature, we will emphasize the cons from four aspects in the current review.
Natural History Issue of LDH
Immune Privilege of NP
Closed Niche
Physiologically, the human lumbar disc comprises three subparts, i.e., the sandwiched central nucleus pulposus (NP), peripheral annulus fibrosus (AF), and adjacent cartilage endplates. The local environment of NP cells is similar to a closed niche (16) (Figure 1). Furthermore, the disc belongs to one of the largest avascular structures in the human body. The blood vessels and innervations terminate in the outer layer of AF of the healthy disc (17). The closest distance from the center of NP to the blood supply is as far as 7–8 mm (18). The nutrition of NP mainly derives from the osmosis of cartilage endplate and AF (18, 19). Therefore, human NP in the disc remains untouched from the immune system, being the physiologic basis of human discs as immune-privileged organs.
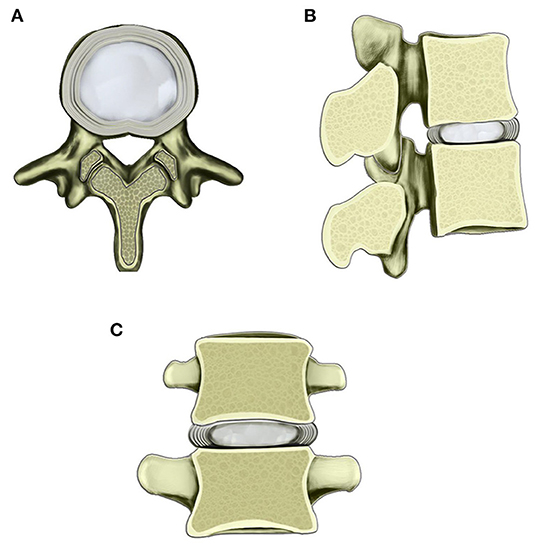
Figure 1. Schematic diagram of the normal lumbar disc within the scope of the vertebral body. (A) Transverse view of normal lumbar disc. (B) Sagittal view of lumbar disc and adjacent vertebral bodies. (C) Coronary view of lumbar disc and adjacent vertebral bodies. The border of the central nucleus pulposus and surrounding AF is clear.
FasL-Fas Network as Underlying Mechanisms of NP Immune-Privilege
FasL (Fas ligand, CD178) localizes in human NP cells strategically as a death factor, which can bind with Fas (CD95, death receptor) of invasive immune cells and endothelial cells (20, 21). The binding of FasL to Fas induces apoptosis (22) of the invasive immune cells, maintaining the immune-privilege characteristic of the intact human NP (23). It has been reported that the cells' morphological alterations and chromosomal DNA degradation in apoptosis occur within a few hours in vitro (24).
Breakdown of the Immune Privilege and Disc Degeneration
Kaneyama et al. (25) found a significant decrease of FasL expression in the degenerated discs compared with the non-degenerate discs, implying the potential protective role of FasL against degeneration. Fas and FasL's expression on stabbed-disc cells is significantly higher than those in normal disc cells (26). When the physiological barrier is damaged, an autoimmune reaction is evoked. Immune cells expressing with FasL bind with NP cells expressing with Fas, which induces the NP cells apoptosis. At the same time, up-regulated FasL in NP co-expressing with Fas induces apoptosis of disc cells via the paracrine pathway. Deregulated FasL and Fas contributing to the abnormal apoptosis of NP cells may be possible pathogenesis of intervertebral disc degeneration (IDD).
Emerging evidence indicates that various physiologic and pathologic processes are regulated by the coding (mRNAs)-non-coding RNA (ncRNA) network. Types of ncRNAs, such as microRNA (miRNA) and long non-coding RNA (lncRNA), are involved in various physiologic and pathologic processes in IDD, which were reported previously. We found that several miRNAs are differentially expressed in degenerative NP, including the down-regulated miR-155. Further investigation revealed that miR-155 plays a regulatory role in FasL-Fas apoptotic signaling pathway. Deregulated miR-155 increases the expression level of Fas-associated death domain-containing protein (FADD) and caspase-3, promoting Fas-mediated apoptosis in IDD (27). Following that, a lncRNA-mRNA microarray analysis of human NP was conducted in 2014 (28). Up-regulated expression of enhancer-like lncRNA RP11-296A18.3 was observed, inducing the overexpression of Fas-associated protein factor-1 which induces the Fas-mediated apoptosis of NP cells at last. Subsequently, Cui et al. indicated that another lncRNA, MAGI2-AS3, is down-regulated in IDD, which is inversely related to the FasL level in NP cells (29). Decrease expression of lncRNA MAGI2-AS3 may promote FasL expression and trigger the FasL-Fas apoptotic signaling pathway, resulting in the apoptosis of NP cells. We addressed the Fas-FasL interacting network between NP, immune cells, and certain modulation factors (21), organizing global researchers for a hot topic issue on IDD (30).
Endogenous Repair Basis
During the regeneration process of various organs, endogenous repair exists, including liver, gut, skin, muscle, kidney, and bone (31). Each organ has a specific capacity for endogenous repair. Accumulating evidence indicates that endogenous repair exists in the human disc, with progenitor cells as crucial contributors (32, 33). In 2007, Risbud et al. (34) first identified human NP and AF cells expressing specific stem cell types of surface markers from degenerative discs. Moreover, these cells can differentiate into chondrogenic, osteogenic, and adipogenic lineages. After that, multiple lines from in vivo and in vitro studies indicated the existence of progenitor cells in human intervertebral discs. Intervertebral disc cells expressing Tie 2 represent a subtype progenitor cell group with discogenic differentiation potential and enhanced regeneration (35).
Besides these basic lines of evidence, various clinical factors contribute to the disruption of the barrier, including trauma/microtrauma during daily life, aging/pathologic alterations (such as scoliosis) with cartilage endplate (CEP) degeneration, iatrogenic, congenital factors, and/or vertebral endplate morphology (36).
Emerging Etiology Evidence of LDH
Clinical Evidence of Spontaneous Resorption of Herniated Intervertebral Discs
Cribb et al. reported a dramatic regression of massive herniation in 14/15 patients after an average 24 months follow-up (range: 5–56 months) (37). Compared with bulges and focal protrusions, broad-based herniation and sequestrations improve more (38). Not only massive soft herniation but large calcified disc herniation could be absorbed as well (39). Other spinal herniation, such as cervical/thoracic disc herniation with/without calcification, has also been reported with spontaneous resolution (40, 41).
Repeated MRIs revealed the shrinkage of herniated discs gradually, with 76% or more absorbed in 1 year (Figure 2). Moreover, Panagopoulos et al. summarized 12 studies in a systematic review (42). Amongst 901 middle-aged LDH patients, 15% to 93% were partially or entirely relieved by 1 year with repeated MRI observations. Zhong et al. conducted a meta-analysis with 11 cohort studies and revealed that the overall incidence of spontaneous regression in LDH patients was 66.66%, with a regional difference (43).
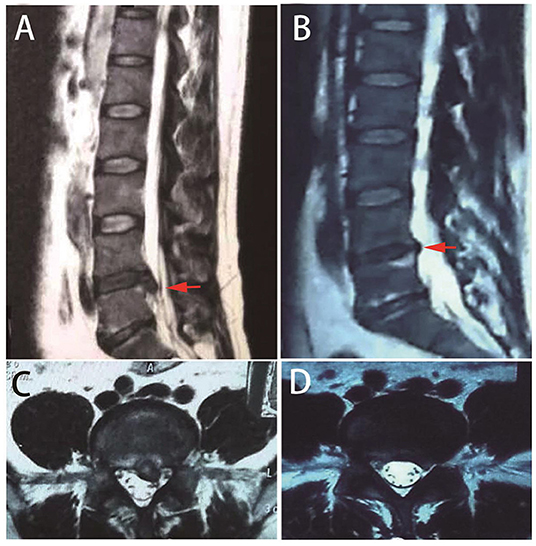
Figure 2. Repeated MRIs of a typical case with spontaneous resolution. A 39-year-old male patient presented with low back pain and sciatica. MRI indicates lumbar disc herniation at L5/S1 [(A,C) red arrows]. One year later, repeated MRI indicates herniation resolution [(B,D) red arrows].
Clinical symptoms, such as sciatica and motor and sensory deficits, can gradually improve in non-surgical treatment LDH cases (44, 45). However, changes in the size of herniated intervertebral discs on MRI are not significantly correlated with the development of clinical symptoms. For instance, sciatica is influenced by multiple factors. Not only the relief of the mechanical compression but also the decreased severity of the inflammatory or chemical irritation contribute to the alleviation of the clinical symptoms (46).
Autoimmune Response and Inflammation Cascade Underlying the Spontaneous Resorption of Herniated Intervertebral Discs
Human intervertebral discs, particularly NP, belong to immune-privileged sites. The initial immune-privileged scenarios change dramatically when NP protrudes out from the closed niche (Figure 3). The herniated tissue is recognized as a foreign antigen by the autoimmune system, attracting immune cells and auto-antibodies, triggering an autoimmune response, and inflammation cascade (47).
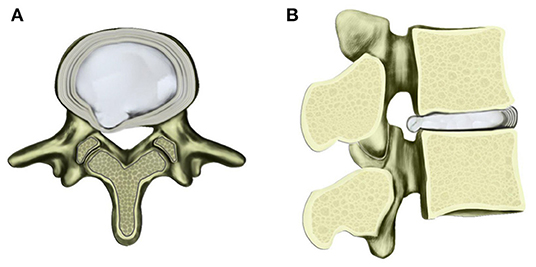
Figure 3. Schematic diagram of contained herniated lumbar disc with transverse (A) and sagittal views (B). Under multiple factors, the nucleus pulposus protrudes toward posterior and lateral direction with AF fibers ruptured to a certain extent.
In 1965, Bobechko and Hirsh revealed that an autoimmune response is induced when NP of rabbits is exposed to the systemic circulation, giving rise to the auto-antibodies production in lymph nodes (48). Subsequently, a high level of IgG and IgM was found in herniated human intervertebral discs (49, 50). Satoh et al. indicated that the antigen-antibody complexes exist particularly in the pericellular space of NP cells rather than the NP cell membrane. This implied that the newly produced substances are surrounding the NP cell, such as polysaccharides, are playing roles of auto-antigen in the immune response (51). Later, evidence of several studies suggested that not only humoral immune response but cellular immune response also exists in the autoimmune response to the herniated substance. Geiss et al. reported that activated T and B cells infiltration is observed in autologous porcine NP exposed to the autoimmune system (52), including IL-4-producing Th2 cells, which participate in the humoral immune system response (53). By using the immunohistochemical analyses, Ikeda et al. (54) and Park et al. (55) found that a small number of T cells and many macrophages are infiltrating the herniated NP tissue. Murai et al. indicated that macrophages and NK cells are the early immune responder after the exposure, then are the T and B cells (56).
Neovascularization has been widely reported contributing to the resorption mechanism. With many newly formed vessels around the disc fragments, granulation tissue was observed on the herniated NP tissue (57). Several inflammatory factors or cytokines, such as tumor necrosis factor–α, midkine, vascular endothelial growth factor, and fibroblast growth factor 2, have been identified as the inducer of angiogenesis (45, 58–62). Macrophages migrate through the newly formed vessels and converge around the disc fragments (58, 63, 64). The infiltrating macrophages produce high levels of matrix metalloproteinase (MMP), including MMP-3 and MMP-7 (65). Cells from herniated discs undergo autoself-induced apoptosis progress via autocrine or paracrine Fas-FasL mechanisms (55). A high level of matrix enzyme degrades the aggrecan and collagen in the herniated material. Finally, the fragment of the tissue and apoptotic cells is absorbed by the macrophages and disc cells via phagocytosis (65–67).
The Hypothesis of the Spontaneous Regression in LDH Natural History
There are three hypotheses to explain the mechanism of spontaneous resorption in LDH. The first hypothesis is the dehydration and shrinkage of the herniated material (68). The second mechanism is supposed that the herniated disc, which is elastic and not separated from the main part in the intervertebral disc space, retracts back to the central place gradually (69). The third is the mechanism mentioned in the previous segment. The herniated disc is identified as a foreign antigen, inducing an autoimmune response and inflammatory cascade. Then, the matrix substance and apoptotic cells are degraded and absorbed by the macrophages via phagocytosis (52). It is supposed that all of the three mechanisms contribute to the spontaneous resorption process (70).
Non-Surgical Treatment—the Foremost Option for LDH Patients Without Serious Symptoms
LDH is treated with surgical or non-surgical measures. Non-surgical treatments of LDH include various methods, such as bed rest, lumbar supports, physical therapy, spinal manipulation, oral analgesics, muscle relaxants, epidural steroid injections, and behavioral therapy (71). Except for the presence of cauda equina syndrome and neurologic impairment, controversy still exists regarding the indications for surgical intervention. North American Spine Society's (NASS) clinical guideline for LDH with radiculopathy indicated that the evidence in a recommendation for urgent surgery is insufficient for LDH patients with motor deficits (72). Several prospective controlled studies suggested that patients undergoing non-surgical treatment should only switch to surgical treatment with exacerbated symptoms (73, 74). Either surgical or conservative measures are suggested effective both in the short and long term for patients with less severe symptoms (72). The recommendation of Danish national clinical guidelines of recent onset lumbar nerve root compression advised at least 12 weeks of a conservative treatment to LDH patients before being considered for operation unless ongoing severe symptoms such as severe pain and disability (75). However, the North American Spine Society's (NASS) clinical guideline for LDH with radiculopathy suggested that LDH patients whose symptoms are severe enough to warrant surgery seek surgical intervention in 6 months. They indicated that earlier surgery (within 6 months to 1 year) is related to faster recovery and better long-term outcomes (72).
Although surgery is effective for LDH patients with radiculopathy in the short term, the surgical complication, repeat operation, and symptomatic recurrent LDH are unavoidable frustrating issues for part of them. A meta-analysis including 34,639 surgical cases of LDH revealed that the overall incidence of complications is 2.7%, while 2.1% of the patients had repeat operations within 3 months (76). Consistently, another study conducted in the US revealed that the average reoperation rate for LDH patients is 1.9% at 90 days, 6.4% at 1 year, and 13.8% at 4 years. Decompressions without fusion account for the majority of re-operative procedures (73%), while fusion with or without decompression nearly makes up for the rest (25.7%) (77). Apart from the undesirable operational effect, symptomatic recurrent LDH is another cause of reoperation. A small part of patients (5–15%) experience unfavorable events, and 4% to 6% undergo surgery in 2 years (78–81).
Although the surgical intervention has the advantages, i.e., rapid relief of symptoms and faster recovery of neurological deficits in the short term (13), several randomized controlled trials (RCTs) showed that the difference between conservative and surgical treatment in LDH patients with radiculopathy is non-significant 1 year later after diagnosis (82). Considering that spontaneous resorption of herniated discs commonly exists in the natural history of LDH, symptoms in a proportion of the patients will resolve on their own. Parts of the patients with LDH, particularly those without serious symptoms, are likely to benefit from the conservative treatment. Part of the patients' clinical symptoms will be alleviated or even completely disappear in a short time (83). Therefore, we suggested that non-surgical treatment is the foremost recommended measure for LDH patients without serious symptoms, such as cauda equina syndrome and motor deficits, which may achieve the same clinical outcomes and avoid various discomfort caused by the operation.
A Long-Term Complication of Lumbar Fusion
In the US, the annual incidence of spinal fusion surgeries has increased over 600% from the 1990s to 2011. Nowadays, 450 000 spinal fusion cases are performed yearly (84). The national trend has been persistent during different observational periods (85). Whereas, spinal fusion with instrumentation increases healthcare expenditures, a surge of serious complications associated with the fusion has been observed as well. Increased local stress and compensatory motion on the non-operated adjacent levels after fusion procedure were both reported, giving rise to many problems, such as adjacent segment diseases (ASD) (86).
Adjacent Segment Disease
ASD was defined as presenting a new clinical symptomatic degenerative disease corresponding to an adjacent level following spinal fusion at an index segment (87). ASD was represented by a series of pathological changes at the adjacent segments, such as disc height loss, disc herniation, canal stenosis, osteophyte formation, spondylolisthesis, and scoliosis (88). The incidence of ASD has been reported to vary from 4 to 45.7% of patients undergoing mono-segmental and multi-level fusion (88–91). Strikingly, multiple-repeated ASD following posterior lumbar interbody fusion of a single segment has been reported (92). Four patients among 1,112 consecutive patients developed multiple-repeated ASD with multiple repeated surgeries, even fusion upper to T1.
The etiology, incidence rate, and treatment strategies for ASD remain undefined. Risk factors of ASD include obesity, natural degeneration with aging, increased stress in intra-disc, preoperative disc degeneration, intraoperative superior facet joint violation, fusion at more than four levels, adjacent cranial segment, the upper shift of lumbar motion center, and decreased sacral slope (87, 88, 93–95). The incidence rate of ASD varies in terms of studied patient samples, follow-up time frame, the number of fusion segments, fusion techniques (180 or 360 degrees), and patients' age (87, 94). Regarding treatment strategies, a systematic review and meta-analysis indicated little available evidence addressing the optimal treatment options for patients with ASD for stenosis with or without instability (96).
Adjacent segment degeneration in the radiograph is the initial stage of ASD, referring to the degeneration of adjacent levels in diagnostic imaging (such as MRI) without clinical symptoms. Several researchers investigated the prevalence of radiographic adjacent segment degeneration and reported that the incidence is ranged from 9 to 27% in the lumbar spine (97, 98). A considerable proportion of the patients underwent an additional operation in the next few years. A series of risk factors were revealed in the published paper for the adjacent segment degeneration, which is similar to ASD (99). A systematic review indicated that the difference among the fusion procedures results in the variation of incidence in adjacent segment degeneration (99). Both aspects have been suggested as the key factors to avoid adjacent segment degeneration, including the reservation of posterior elements in the fusion procedure and perioperative treatment of osteoporosis.
Other Complications Associated With Fusion Surgery
A systematic review of the literature of lumbar fusion for degenerative disorders, including 160 studies, revealed that the overall complication rate of lumbar fusion procedure is 14% (100). Apart from ASD, other long-term complications associated with fusion surgery, such as pseudarthrosis, implant failure, and sagittal spinal imbalance, were also widely reported in the literature (101, 102). The overall fusion rate for patients undergoing lumbar fusion procedures was reported as 88.5% (100). Smoking, metabolic disorders, surgical instrumentation and technique, and fusion location have been demonstrated as the risk factors for pseudarthrosis (103, 104). In addition to this, osteopenia and osteoporosis have been suggested as another risk factor for pseudarthrosis, and implant failure, such as screw loosening (105, 106). Post-operative back pain was reported in the patients undergoing lumbar fusion procedures. In-depth investigation showed that poor post-operative spinal sagittal alignment is related to prolonged back pain (107). Apart from that, the sagittal spinal imbalance was also associated with the body imbalance, which induces falls (108). The causes of sagittal imbalance are multifactorial, including pseudarthrosis at the lumbosacral junction, adjacent segment disease, and high pelvic incidence (109). The restoration or correction of sagittal alignment is important to the patients' surgical outcome and quality of life.
Metallosis Issue
Metal Debris and Elevated ion Level in Arthroplasty
Due to electrochemical corrosion and/or mechanical wear, surgical metallic implants have gained increasing attention in recent years. As early as 1973, Coleman et al. (110) presented the first line of evidence on a raised level of cobalt and chromium in the blood and urine of patients with metallic total hip replacements. Submicrometer metal particles within macrophages in the liver and/or the spleen were observed in patients undergoing primary and revision total hip arthroplasty (111).
The elevated level of systemic metal particles accumulating in the end organs, such as the heart (112), liver (111), and spleen (111), resulting in systemic metal toxicity, such as cobalt toxicity (113–116), even causing death (117). Apart from that, intracellular phagocytosis of particulate debris by macrophages can trigger the release of proinflammatory cytokines in the surrounding tissue, inducing aseptic fibrosis, local necrosis, or loosening of a device secondary to metal corrosion (118). Such type of metal debris staining complication is termed metallosis (119). Metallosis is a potentially fatal complication originally found in patients after arthroplasty, which is generally associated with metal or non-metallic implant wear (120). By analyzing whole blood metal and ion levels in 185 patients undergoing bilateral Birmingham Hip Resurfacing, Matharu et al. (121) proposed that the optimal threshold was 5.5 μg/L for distinguishing patients with and without adverse reactions metal debris.
Systemic and Local Reactions Related to Spinal Metallic Implants
Joint prostheses and spinal instrumentation have different biomechanical effects on the human body. Regardless of corrosion mechanisms, mechanical wear is the predominating reason for the metallosis after arthroplasty, whereas fretting wear is the primary cause for metallosis after spinal instrumentation (119). It is generally speculated that the inevitable micromotion at the metal-metal junctions may lead to fretting corrosion and production of the particulate metallic debris after spinal instrumentation. Spinal metallic implants are currently made of titanium alloy, containing 90% titanium, 6% aluminum, and 4% vanadium. Other metal components exist in spinal implants containing niobium. It is widely reported that Ti6Al4V is highly susceptible to fretting corrosion due to a mixed microstructure when the titania passivation layer is disrupted (122). In contrast to these findings, a long-term test showed that the titanium and cobalt chrome constructs are more resistant to fretting corrosion than stainless steel (123).
In 1999, Wang et al. (124) reported that wear debris is generated in the tissue surrounding titanium spinal implants from nine patients undergoing prior lumbar decompression and fusion procedure and reoperation. Metal levels were higher in patients with pseudarthrosis than patients with a solid spinal fusion (30.36 μg/g of dry tissue vs. 0.586 μg/g of dry tissue). In 2003, Kaisai et al. (125) studied metal concentrations in the serum and hair of 46 patients with titanium alloy spinal implants, using inductively coupled plasma emission spectroscopy. Accordingly, they noted that one-third of involved patients exhibited higher serum or hair metal concentrations following surgery. Titanium or aluminum may have distant organ accumulation from the spinal implants. In 2008, Richardson et al. (126) reported higher serum titanium levels in 30 patients with titanium alloy spinal instrumentation prospectively in comparison with controls (2.6 vs. 0.71 μg/L), using high resolution inductively coupled plasma-mass spectrometry [HR-ICP-MS, detection limit for titanium as 0.25 μg/L (ppm)]. Instrumented spinal fusion can result in abnormally elevated serum titanium, aluminum, and niobium levels in pediatric patients undergoing instrumented spinal arthrodesis to correct scoliosis and kyphosis (127–129). A systematic review concerning the concentration of metal ions following multi-level spinal fusion, which includes 18 studies and encompasses 653 patients, showed that metal ions are elevated after instrumented spinal fusion, notably Cr levels from stainless steel implants, and Ti from titanium implants (130). Moreover, serum metal ion levels correlate positively with fusion segments and numbers of spinal implants.
The Harmful Effect of Metallosis After Spinal Implantation
Accumulating evidence has unraveled local and systemic reactions to metal spinal implants. Metal particulate debris deposited in the soft tissue surrounding spinal implants was shown to activate a macrophage response that triggers the release of proinflammatory cytokines, leading to mild chronic inflammation, and stimulating the formation of the metal debris granuloma (131). The chronic inflammation irritated by the metal debris was suggested to be associated with the late operative site pain, which is eliminated until the implant is removed (132). Several researchers reported that the intraspinal extradural granuloma resulting from the foreign body reaction to the metallic wear debris contributes to the compression of the neurological elements and neurological symptoms mimicking the lumbar spinal stenosis (133–135). Moreover, metal debris has been shown to induce the mature osteoclast precursor and apoptosis of osteoblast, increase the peri-prosthetic bone resorption, and inhibit osteogenesis. These effects result in implant debris-related osteolysis, aseptic fibrosis, local necrosis, or implant loosening (136–140).
Metal debris has also been displayed to stimulate the immune system to induce a series of type IV delayed-type IV hypersensitivity responses (141, 142). These immunogenic reactions are presented as anorexia, fatigue (143), severe dermatitis (144), urticarial (145), and vasculitis (145). In addition, much evidence indicates that degraded metal particles from spinal metallic implants can enter the systemic blood circulation and deposit in the heart, liver, and spleen. The average level of serum titanium is similar to that of patients undergoing arthroplasty. Although a few findings have been reported, the long-term impact of elevated serum metal concentrations on patients with a spinal implant is not entirely clear. Furthermore, there has been no established threshold above which metal concentrations will be toxic after the spinal instrumented surgery. Removing the spinal implantation at the right time may be a method to avoid metallosis.
Spinal Implant Removal
The latest updated guidelines (NG59) drafted by the National Institute for Health and Care Excellence in the UK (https://www.nice.org.uk/guidance/ng59) states that fusion for non-specific low back pain should be strictly used only for RCTs (146). The guidelines reflect those lessons obtained from clinical practice and reports. There have been no established indications for spinal implant removal until now. Therefore, whether to proceed depends on the surgeon's preference.
Even though the application of spinal instrumentation increased the probability of successful spinal fusion, stress shielding induced osteoporosis on account of the rigid fixation and increased the risk of recurrent fracture after implant removal (106, 147, 148). Acquired spondylolysis has been a well-recognized stress fracture after posterior lumbar fusion since 1963 (149). Nevertheless, adverse events have been reported following pedicle screw constructs removal, including pedicle stress fracture due to iatrogenic weakness of the pedicles following removal (150, 151), vertebral compression fracture within a solid lumbar fusion mass (152), or recurrent vertebral fracture following pedicle screw removal for index burst fracture (153). Therefore, the surgeon should attach attention to the implant removal time and method, avoiding implant removal failure.
Other Challenges
Besides those mentioned above existing challenging issues, there are other questions to be solved, including decreased quality of life due to lumbar rigidity radiation exposure from perioperative and follow-up diagnostic imaging. In addition to common clinical outcome measures for lumbar spine surgery, indicators have been noted reflecting lumbar rigidity due to the decrease in kinematic units following lumbar fusion. Sciubba et al. (154) evaluated the impact of stiffness on activities of daily living following instrumented total lumbar fusion. The most affected activities of daily living included dressing or bathing the lower half of the body and performing personal hygiene functions after toileting.
By adding instrumentation, patients have to experience additional ionizing radiation exposure for the orientation of pedicle screws during surgery with fluoroscopy (155–157) observations on repeated radiographs for clinical outcome follow-up. Compared to pure decompression, adding instrumentation will result in more cumulative radiation exposure for surgeons, medical staff in operating theaters, and patients. Importantly, the awareness of such potential harms is low amongst medical professionals (158) and patients (159, 160).
Residual and recurring back pain after surgery is common in LDH surgeries. The proportion of patients reporting short-term (6–24 months) and long-term (>24 months) recurrent back pain ranged from 3–34% to 5–36%, respectively in a systematic literature review (79). Some people who have persistent pain postoperative are still unclear (161). Severe endplate changes, such as endplate avulsion, damaged the lumbar stability and maybe resulted in a higher recurrence rate and residual back pain (162, 163). LBP has been suggested to be associated with postural and structural asymmetries. CEP degeneration accompanied by loss of cellularity results in the asymmetric loading of the lumbar spine in LDH. Fusion surgery provides the stabilization and maybe correct asymmetry of the lumbar spine in part (164). However, the current operation aims not to solve the imbalance of load, nor can it completely solve the problem. Asymmetry of lumbar loading may still be one of the causes of residual back pain postoperative. The predictors of residual LBP after decompression included more severe LBP at baseline, degenerative scoliosis, and Cobb angle size (165).
As the second most mobile part of the human axis, the lumbar spine and related LDH have been a hot topic for the medical community (164). Nevertheless, LBP, most commonly caused by a herniated disc, is a constant concern (166). For decades, lumbar discectomy has been done by neurosurgeons as the general surgical practice to solve the disease (167, 168). The discovery of X-Ray brought about a shift of paradigm in the practice of neurosurgery (169). The introduction of microsurgical techniques led to an essential evolution in lumbar disc surgery (170). For those who have failed conservative treatment, surgery is the only option that must be considered. However, while the operation solves the symptoms, it also brings problems that can not be ignored. This paper summarizes the cons of surgical treatment from different perspectives. These summaries are useful supplements to the present literature, providing a unique vision for surgeons and patients who attempt to choose surgical treatment.
Conclusions
Due to various triggering factors, lumbar surgeries with or without implementation increase rapidly with great health expenditures. In the review, we analyzed the tetrad critical issues about surgical intervention for LDH, i.e., favorable natural history, long-term complication of lumbar fusion, metallosis, and implant removal (Figure 4). Based on the limited evidence available so far, lumbar surgery solves the symptoms for the patients with LDH and brings a new series of unexpected problems. Therefore, the long-term effects of surgery should be closely observed. Surgical decisions should be made prudently for each patient.
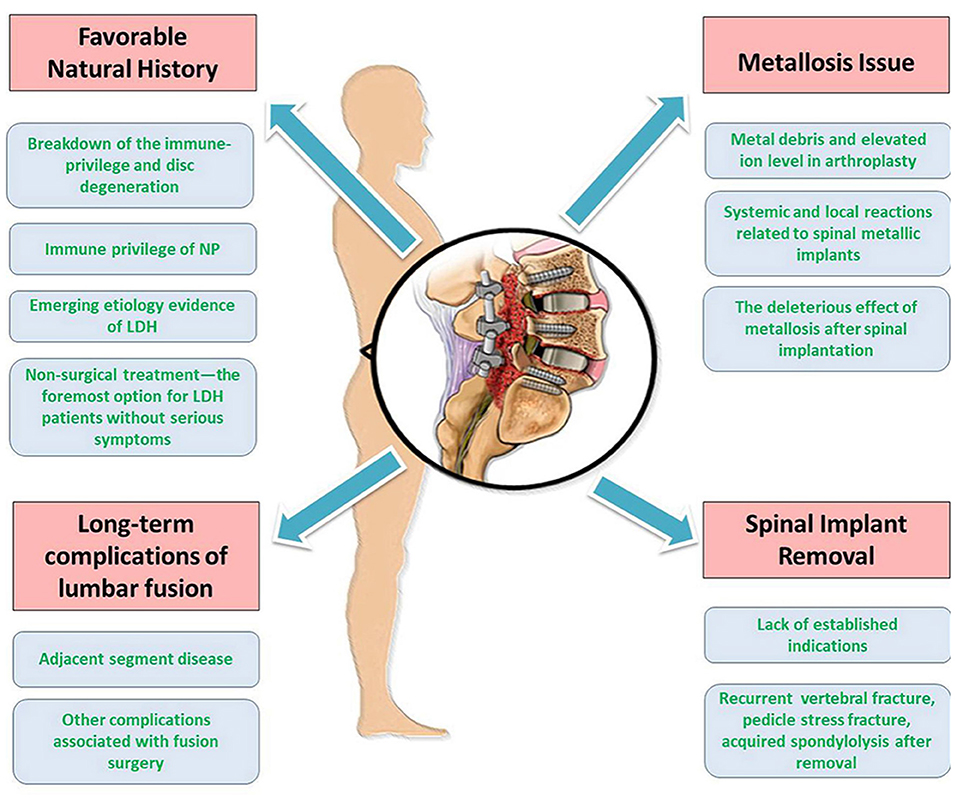
Figure 4. Summarizations of the different aspects of the review. There are tetrad critical issues pertaining to surgical treatment of LDH, i.e., favorable natural history, long-term complications of lumbar fusion, metallosis issue, and spinal implant removal.
Author Contributions
HW conceived the study. ZW, HS, and TL investigated and retrieved the published papers, as well as wrote the original draft. FS, JZ, ZL, and KM reviewed and edited the final version of the manuscript. All authors have read and agreed to the final version of the manuscript.
Funding
This work was supported by the National Natural Science Foundation of China (Grant no. 81572182).
Conflict of Interest
The authors declare that the research was conducted in the absence of any commercial or financial relationships that could be construed as a potential conflict of interest.
The reviewer F-LW declared a shared affiliation with the author ZL to the handling editor at the time of the review.
Publisher's Note
All claims expressed in this article are solely those of the authors and do not necessarily represent those of their affiliated organizations, or those of the publisher, the editors and the reviewers. Any product that may be evaluated in this article, or claim that may be made by its manufacturer, is not guaranteed or endorsed by the publisher.
Abbreviations
NP, nucleus pulposus; AF, annulus fibrosus; ASD, adjacent segment disease; CEP, cartilage endplate; FADD, Fas-associated death domain containing protein; FasL, Fas ligand; IDD, intervertebral disc degeneration; LBP, low back pain; LDH, lumbar disc herniation; lncRNA, long noncoding RNA; miRNA, microRNA; MMP, metalloproteinase; MRI, magnetic resonance imaging; NASS, North American Spine Society; ncRNA, noncoding RNA; RCTs, randomized controlled trials.
References
1. Steffens D, Maher CG, Pereira LS, Stevens ML, Oliveira VC, Chapple M, et al. Prevention of low back pain: a systematic review and meta-analysis. JAMA Intern Med. (2016) 176:199–208. doi: 10.1001/jamainternmed.2015.7431
2. Vos T, Barber RM, Bell B, Bertozzi-Villa A, Biryukov S, Bolliger I, et al. Global, regional, and national incidence, prevalence, and years lived with disability for 301 acute and chronic diseases and injuries in 188 countries, 1990–2013: a systematic analysis for the global burden of disease study 2013. Lancet. (2015). 386:1545–602. doi: 10.1016/S0140-6736(16)31678-6
3. Apfel CC, Cakmakkaya OS, Martin W, Richmond C, Macario A, George E, et al. Restoration of disk height through non-surgical spinal decompression is associated with decreased discogenic low back pain: a retrospective cohort study. BMC Musculoskelet Disord. (2010) 11:155. doi: 10.1186/1471-2474-11-155
4. Teraguchi M, Yoshimura N, Hashizume H, Muraki S, Yamada H, Minamide A, et al. Prevalence and distribution of intervertebral disc degeneration over the entire spine in a population-based cohort: the Wakayama spine study. Osteoarthritis Cartilage. (2014) 22:104–10. doi: 10.1016/j.joca.2013.10.019
5. Lagerback T, Elkan P, Moller H, Grauers A, Diarbakerli E, Gerdhem P. An observational study on the outcome after surgery for lumbar disc herniation in adolescents compared with adults based on the Swedish Spine Register. Spine J. (2015) 15:1241–7. doi: 10.1016/j.spinee.2015.02.024
6. Latka D, Miekisiak G, Jarmuzek P, Lachowski M, Kaczmarczyk J. Treatment of lumbar disc herniation with radiculopathy. Clinical practice guidelines endorsed by the polish society of spinal surgery. Neurol Neurochir Pol. (2016) 50:101–8. doi: 10.1016/j.pjnns.2015.12.001
7. Wang JC, Dailey AT, Mummaneni PV, Ghogawala Z, Resnick DK, Watters WC, et al. Guideline update for the performance of fusion procedures for degenerative disease of the lumbar spine. Part 8: lumbar fusion for disc herniation and radiculopathy. J Neurosurg Spine. (2014) 21:48–53. doi: 10.3171/2014.4.SPINE14271
8. Arif S, Brady Z, Enchev Y, Peev N. Is fusion the most suitable treatment option for recurrent lumbar disc herniation? A systematic review. Neurol Res. (2020) 42:1034–42. doi: 10.1080/01616412.2020.1787661
9. Kim CH, Chung CK, Park CS, Choi B, Kim MJ, Park BJ. Reoperation rate after surgery for lumbar herniated intervertebral disc disease: nationwide cohort study. Spine. (2013) 38:581–90. doi: 10.1097/BRS.0b013e318274f9a7
10. Tacconi L. Lumbar discectomy: has it got any ill-effects? J Spine Surg. (2018) 4:677–80. doi: 10.21037/jss.2018.07.05
11. Castillo H, Chintapalli RTV, Boyajian HH, Cruz SA, Morgan VK, Shi LL, et al. Lumbar discectomy is associated with higher rates of lumbar fusion. Spine J. (2019) 19:487–92. doi: 10.1016/j.spinee.2018.05.016
12. Lorio Morgan KC, Araghi Ali, Inzana Jason, Yue James J. International society for the advancement of spine surgery policy 2019-surgical treatment of lumbar disc herniation with radiculopathy. Int J Spine Surg. (2020) 14:17. doi: 10.14444/7001
13. Jacobs WC, van Tulder M, Arts M, Rubinstein SM, van Middelkoop M, Ostelo R, et al. Surgery versus conservative management of sciatica due to a lumbar herniated disc: a systematic review. Eur Spine J. (2011) 20:513–22. doi: 10.1007/s00586-010-1603-7
14. Zaina F, Tomkins-Lane C, Carragee E, Negrini S. Surgical versus non-surgical treatment for lumbar spinal stenosis. Spine. (2016) 41:CD010264. doi: 10.1002/14651858.CD010264.pub2
15. Delitto A, Piva SR, Moore CG, Welch WC. Surgery versus nonsurgical treatment of lumbar spinal stenosis. Ann Intern Med. (2015) 163:397–8. doi: 10.7326/L15-5129-4
16. Choi H, Johnson ZI, Risbud MV. Understanding nucleus pulposus cell phenotype: a prerequisite for stem cell based therapies to treat intervertebral disc degeneration. Curr Stem Cell Res Ther. (2015) 10:307–16. doi: 10.2174/1574888X10666150113112149
17. Roberts S, Evans H, Trivedi J, Menage J. Histology and pathology of the human intervertebral disc. J Bone Joint Surg Am. (2006) 88 (Suppl. 2):10–4. doi: 10.2106/JBJS.F.00019
18. Katz MM, Hargens AR, Garfin SR. Intervertebral disc nutrition. Diffusion versus convection. Clin Orthop Relat Res. (1986) 210:243–5. doi: 10.1097/00003086-198609000-00035
19. Ferguson SJ, Ito K, Nolte LP. Fluid flow and convective transport of solutes within the intervertebral disc. J Biomech. (2004) 37:213–21. doi: 10.1016/S0021-9290(03)00250-1
20. Liu ZH, Sun Z, Wang HQ, Ge J, Jiang TS, Chen YF, et al. FasL expression on human nucleus pulposus cells contributes to the immune privilege of intervertebral disc by interacting with immunocytes. Int J Med Sci. (2013) 10:1053–60. doi: 10.7150/ijms.6223
21. Ma CJ, Liu X, Che L, Liu ZH, Samartzis D, Wang HQ. Stem cell therapies for intervertebral disc degeneration: immune privilege reinforcement by Fas/FasL regulating machinery. Curr Stem Cell Res Ther. (2015) 10:285–95. doi: 10.2174/1574888X10666150416114027
22. Cohen PL, Eisenberg RA. The lpr and gld genes in systemic autoimmunity: life and death in the Fas lane. Immunol Today. (1992) 13:427–8. doi: 10.1016/0167-5699(92)90066-G
23. Takada T, Nishida K, Doita M, Kurosaka M. Fas ligand exists on intervertebral disc cells: a potential molecular mechanism for immune privilege of the disc. Spine. (2002) 27:1526–30. doi: 10.1097/00007632-200207150-00009
24. Suda T, Takahashi T, Golstein P, Nagata S. Molecular cloning and expression of the Fas ligand, a novel member of the tumor necrosis factor family. Cell. (1993) 75:1169–78. doi: 10.1016/0092-8674(93)90326-L
25. Kaneyama S, Nishida K, Takada T, Suzuki T, Shimomura T, Maeno K, et al. Fas ligand expression on human nucleus pulposus cells decreases with disc degeneration processes. J Orthop Sci. (2008) 13:130–5. doi: 10.1007/s00776-007-1204-4
26. Wang J, Tang T, Yang H, Yao X, Chen L, Liu W, et al. The expression of Fas ligand on normal and stabbed-disc cells in a rabbit model of intervertebral disc degeneration: a possible pathogenesis. J Neurosurg Spine. (2007) 6:425–30. doi: 10.3171/spi.2007.6.5.425
27. Wang HQ, Yu XD, Liu ZH, Cheng X, Samartzis D, Jia LT, et al. Deregulated miR-155 promotes Fas-mediated apoptosis in human intervertebral disc degeneration by targeting FADD and caspase-3. J Pathol. (2011) 225:232–42. doi: 10.1002/path.2931
28. Wan ZY, Song F, Sun Z, Chen YF, Zhang WL, Samartzis D, et al. Aberrantly expressed long noncoding RNAs in human intervertebral disc degeneration: a microarray related study. Arthritis Res Ther. (2014) 16:465. doi: 10.1186/s13075-014-0465-5
29. Cui S, Liu Z, Tang B, Wang Z, Li B. LncRNA MAGI2-AS3 is down-regulated in intervertebral disc degeneration and participates in the regulation of FasL expression in nucleus pulposus cells. BMC Musculoskelet Disord. (2020) 21:149. doi: 10.1186/s12891-020-3086-y
30. Wang HQ. Bring stem cell therapies to cure intervertebral disc degeneration to the forefront. Curr Stem Cell Res Ther. (2015) 10:284. doi: 10.2174/1574888X1004150513162605
31. Forbes SJ, Rosenthal N. Preparing the ground for tissue regeneration: from mechanism to therapy. Nat Med. (2014) 20:857–69. doi: 10.1038/nm.3653
32. Li Z, Peroglio M, Alini M, Grad S. Potential and limitations of intervertebral disc endogenous repair. Curr Stem Cell Res Ther. (2015) 10:329–38. doi: 10.2174/1574888X10666150305105114
33. Sakai D, Nakamura Y, Nakai T, Mishima T, Kato S, Grad S, et al. Exhaustion of nucleus pulposus progenitor cells with ageing and degeneration of the intervertebral disc. Nat Commun. (2012) 3:1264. doi: 10.1038/ncomms2226
34. Risbud MV, Guttapalli A, Tsai TT, Lee JY, Danielson KG, Vaccaro AR, et al. Evidence for skeletal progenitor cells in the degenerate human intervertebral disc. Spine. (2007) 32:2537–44. doi: 10.1097/BRS.0b013e318158dea6
35. Wangler S, Peroglio M, Menzel U, Benneker LM, Haglund L, Sakai D, et al. Mesenchymal stem cell homing into intervertebral discs enhances the tie2-positive progenitor cell population, prevents cell death, and induces a proliferative response. Spine. (2019) 44:1613–22. doi: 10.1097/BRS.0000000000003150
36. Duran S, Cavusoglu M, Hatipoglu HG, Sozmen Ciliz D, Sakman B. Association between measures of vertebral endplate morphology and lumbar intervertebral disc degeneration. Can Assoc Radiol J. (2017) 68:210–6. doi: 10.1016/j.carj.2016.11.002
37. Cribb GL, Jaffray DC, Cassar-Pullicino VN. Observations on the natural history of massive lumbar disc herniation. J Bone Joint Surg Br. (2007) 89:782–4. doi: 10.1302/0301-620X.89B6.18712
38. Jensen TS, Albert HB, Soerensen JS, Manniche C, Leboeuf-Yde C. Natural course of disc morphology in patients with sciatica: an MRI study using a standardized qualitative classification system. Spine. (2006) 31:1605–12; discussion 1613. doi: 10.1097/01.brs.0000221992.77779.37
39. Guinto FC Jr., Hashim H, Stumer M. CT demonstration of disk regression after conservative therapy. AJNR Am J Neuroradiol. (1984) 5:632–3.
40. Ahmad FU, Schallert E, Bregy A, Post JD, Vanni S. Disappearing large calcified thoracic disc herniation in a patient with thalassaemia. BMJ Case Rep. (2016) 2016:bcr-2015-213166. doi: 10.1136/bcr-2015-213166
41. Kobayashi N, Asamoto S, Doi H, Ikeda Y, Matusmoto K. Spontaneous regression of herniated cervical disc. Spine J. (2003) 3:171–3. doi: 10.1016/S1529-9430(02)00556-9
42. Panagopoulos J, Hush J, Steffens D, Hancock MJ. Do MRI findings change over a period of up to 1 year in patients with low back pain and/or sciatica?: a systematic review. Spine. (2017) 42:504–12. doi: 10.1097/BRS.0000000000001790
43. Zhong M, Liu JT, Jiang H, Mo W, Yu PF, Li XC, et al. Incidence of spontaneous resorption of lumbar disc herniation: a meta-analysis. Pain Phys. (2017) 20:E45–52. doi: 10.36076/ppj.2017.1.E45
44. Deyo RA, Mirza SK. Clinical practice. Herniated lumbar intervertebral disk. N Engl J Med. (2016) 374:1763–72. doi: 10.1056/NEJMcp1512658
45. Zhou G, Dai L, Jiang X, Ma Z, Ping J, Li J, et al. Effects of human midkine on spontaneous resorption of herniated intervertebral discs. Int Orthop. (2010) 34:103–8. doi: 10.1007/s00264-009-0740-2
46. Seo JY, Roh YH, Kim YH, Ha KY. Three-dimensional analysis of volumetric changes in herniated discs of the lumbar spine: does spontaneous resorption of herniated discs always occur? Eur Spine J. (2016) 25:1393–402. doi: 10.1007/s00586-014-3587-1
47. Sun Z, Wang HQ, Liu ZH, Chang L, Chen YF, Zhang YZ, et al. Down-regulated CK8 expression in human intervertebral disc degeneration. Int J Med Sci. (2013) 10:948–56. doi: 10.7150/ijms.5642
48. Bobechko WP, Hirsch C. Auto-Immune response to nucleus pulposus in the rabbit. J Bone Joint Surg Br. (1965) 47:574–80. doi: 10.1302/0301-620X.47B3.574
49. Bisla RS, Marchisello PJ, Lockshin MD, Hart DM, Marcus RE, Granda J. Auto-immunological basis of disk degeneration. Clin Orthop Relat Res. (1976) 121:205–11. doi: 10.1097/00003086-197611000-00033
50. Guner A, Oktay G, Kerman M, Guner G. Immunoglobulins and alpha-1-proteinase inhibitor in human intervertebral disc material. Biochem Soc Trans. (1995) 23:212S. doi: 10.1042/bst023212s
51. Satoh K, Konno S, Nishiyama K, Olmarker K, Kikuchi S. Presence and distribution of antigen-antibody complexes in the herniated nucleus pulposus. Spine. (1999) 24:1980–4. doi: 10.1097/00007632-199910010-00003
52. Geiss A, Larsson K, Rydevik B, Takahashi I, Olmarker K. Autoimmune properties of nucleus pulposus: an experimental study in pigs. Spine. (2007) 32:168–73. doi: 10.1097/01.brs.0000251651.61844.2d
53. Geiss A, Larsson K, Junevik K, Rydevik B, Olmarker K. Autologous nucleus pulposus primes T cells to develop into interleukin-4-producing effector cells: an experimental study on the autoimmune properties of nucleus pulposus. J Orthop Res. (2009) 27:97–103. doi: 10.1002/jor.20691
54. Ikeda T, Nakamura T, Kikuchi T, Umeda S, Senda H, Takagi K. Pathomechanism of spontaneous regression of the herniated lumbar disc: histologic and immunohistochemical study. J Spinal Disord. (1996) 9:136–40. doi: 10.1097/00002517-199604000-00009
55. Park JB, Chang H, Kim KW. Expression of Fas ligand and apoptosis of disc cells in herniated lumbar disc tissue. Spine. (2001) 26:618–21. doi: 10.1097/00007632-200103150-00011
56. Murai K, Sakai D, Nakamura Y, Nakai T, Igarashi T, Seo N, et al. Primary immune system responders to nucleus pulposus cells: evidence for immune response in disc herniation. Eur Cell Mater. (2010) 19:13–21. doi: 10.22203/eCM.v019a02
57. Haro H, Kato T, Komori H, Osada M, Shinomiya K. Vascular endothelial growth factor (VEGF)-induced angiogenesis in herniated disc resorption. J Orthop Res. (2002) 20:409–15. doi: 10.1016/S0736-0266(01)00150-4
58. Kokubo Y, Uchida K, Kobayashi S, Yayama T, Sato R, Nakajima H, et al. Herniated and spondylotic intervertebral discs of the human cervical spine: histological and immunohistological findings in 500 en bloc surgical samples. Laboratory investigation. J Neurosurg Spine. (2008) 9:285–95. doi: 10.3171/SPI/2008/9/9/285
59. Eap C, Bennis S, Blauwblomme T, Compaore P, Chamsedine A, Mireau E, et al. [Spontaneous resorption of thoracic calcified disc herniation: report of two cases and review of the literature]. Neurochirurgie. (2012) 58:353–7. doi: 10.1016/j.neuchi.2012.05.005
60. Haro H, Domoto T, Maekawa S, Horiuchi T, Komori H, Hamada Y. Resorption of thoracic disc herniation. Report of 2 cases. J Neurosurg Spine. (2008) 8:300–4. doi: 10.3171/SPI/2008/8/3/300
61. Kato T, Haro H, Komori H, Shinomiya K. Sequential dynamics of inflammatory cytokine, angiogenesis inducing factor and matrix degrading enzymes during spontaneous resorption of the herniated disc. J Orthop Res. (2004) 22:895–900. doi: 10.1016/j.orthres.2003.11.008
62. Xu N, Wei F, Liu X, Jiang L, Liu Z. Calcific discitis with giant thoracic disc herniations in adults. Eur Spine J. (2016) 25 (Suppl. 1):204–8. doi: 10.1007/s00586-016-4402-y
63. Tolonen J, Gronblad M, Virri J, Seitsalo S, Rytomaa T, Karaharju E. Transforming growth factor beta receptor induction in herniated intervertebral disc tissue: an immunohistochemical study. Eur Spine J. (2001) 10:172–6. doi: 10.1007/s005860000213
64. Zigouris A, Batistatou A, Alexiou GA, Pachatouridis D, Mihos E, Drosos D, et al. Correlation of matrix metalloproteinases-1 and−3 with patient age and grade of lumbar disc herniation. J Neurosurg Spine. (2011) 14:268–72. doi: 10.3171/2010.9.SPINE09935
65. Haro H, Murakami S, Komori H, Okawa A, Shinomiya K. Chemonucleolysis with human stromelysin-1. Spine. (1997) 22:1098–104. doi: 10.1097/00007632-199705150-00009
66. Jones P, Gardner L, Menage J, Williams GT, Roberts S. Intervertebral disc cells as competent phagocytes in vitro: implications for cell death in disc degeneration. Arthritis Res Ther. (2008) 10:R86. doi: 10.1186/ar2466
67. Yang X, Zhang Q, Hao X, Guo X, Wang L. Spontaneous regression of herniated lumbar discs: report of one illustrative case and review of the literature. Clin Neurol Neurosurg. (2016) 143:86–9. doi: 10.1016/j.clineuro.2016.02.020
68. Kim SG, Yang JC, Kim TW, Park KH. Spontaneous regression of extruded lumbar disc herniation: three cases report. Korean J Spine. (2013) 10:78–81. doi: 10.14245/kjs.2013.10.2.78
69. Teplick JG, Haskin ME. Spontaneous regression of herniated nucleus pulposus. AJR Am J Roentgenol. (1985) 145:371–5. doi: 10.2214/ajr.145.2.371
70. Turk O, Yaldiz C. Spontaneous regression of cervical discs: retrospective analysis of 14 cases. Medicine. (2019) 98:e14521. doi: 10.1097/MD.0000000000014521
71. Awad JN, Moskovich R. Lumbar disc herniations: surgical versus non-surgical treatment. Clin Orthop Relat Res. (2006) 443:183–97. doi: 10.1097/01.blo.0000198724.54891.3a
72. Kreiner DS, Hwang SW, Easa JE, Resnick DK, Baisden JL, Bess S, et al. An evidence-based clinical guideline for the diagnosis and treatment of lumbar disc herniation with radiculopathy. Spine J. (2014) 14:180–91. doi: 10.1016/j.spinee.2013.08.003
73. Nygaard OP, Kloster R, Solberg T. Duration of leg pain as a predictor of outcome after surgery for lumbar disc herniation: a prospective cohort study with 1-year follow up. J Neurosurg. (2000) 92 (2 Suppl):131–4. doi: 10.3171/spi.2000.92.2.0131
74. Fisher C, Noonan V, Bishop P, Boyd M, Fairholm D, Wing P, et al. Outcome evaluation of the operative management of lumbar disc herniation causing sciatica. J Neurosurg. (2004) 100 (4 Suppl):317–24. doi: 10.3171/spi.2004.100.4.0317
75. Kjær P, Junker K, Kongsted A, Fournier G, Hartvigsen J, Kirkeskov L. National Klinisk Retningslinje for Ikke-Kirurgisk Behandling af Lumbal Nerverodspåvirkning (Lumbal Radikulopati). København: afSundhedsstyrelsen (2016). Available online at: http://www.sst.dk.Dansk
76. Fjeld OR, Grovle L, Helgeland J, Smastuen MC, Solberg TK, Zwart JA, et al. Complications, reoperations, readmissions, and length of hospital stay in 34 639 surgical cases of lumbar disc herniation. Bone Joint J. (2019) 101-B:470–7. doi: 10.1302/0301-620X.101B4.BJJ-2018-1184.R1
77. Martin BI, Mirza SK, Flum DR, Wickizer TM, Heagerty PJ, Lenkoski AF, et al. Repeat surgery after lumbar decompression for herniated disc: the quality implications of hospital and surgeon variation. Spine J. (2012) 12:89–97. doi: 10.1016/j.spinee.2011.11.010
78. Huang W, Han Z, Liu J, Yu L, Yu X. Risk factors for recurrent lumbar disc herniation: a systematic review and meta-analysis. Medicine. (2016) 95:e2378. doi: 10.1097/MD.0000000000002378
79. Parker SL, Mendenhall SK, Godil SS, Sivasubramanian P, Cahill K, Ziewacz J, et al. Incidence of low back pain after lumbar discectomy for herniated disc and its effect on patient-reported outcomes. Clin Orthop Relat Res. (2015) 473:1988–99. doi: 10.1007/s11999-015-4193-1
80. Tanavalee C, Limthongkul W, Yingsakmongkol W, Luksanapruksa P, Singhatanadgige W. A comparison between repeat discectomy versus fusion for the treatment of recurrent lumbar disc herniation: systematic review and meta-analysis. J Clin Neurosci. (2019) 66:202–8. doi: 10.1016/j.jocn.2019.05.004
81. Virk SS, Diwan A, Phillips FM, Sandhu H, Khan SN. What is the rate of revision discectomies after primary discectomy on a national scale? Clin Orthop Relat Res. (2017) 475:2752–62. doi: 10.1007/s11999-017-5467-6
82. McMorland G, Suter E, Casha S, du Plessis SJ, Hurlbert RJ. Manipulation or microdiskectomy for sciatica? A prospective randomized clinical study. J Manipulative Physiol Ther. (2010) 33:576–84. doi: 10.1016/j.jmpt.2010.08.013
83. Gautschi OP, Stienen MN, Schaller K. [Spontaneous regression of lumbar and cervical disc herniations - a well established phenomenon]. Praxis. (2013) 102:675–80. doi: 10.1024/1661-8157/a001298
84. Deyo RA. Fusion surgery for lumbar degenerative disc disease: still more questions than answers. Spine J. (2015) 15:272–4. doi: 10.1016/j.spinee.2014.11.004
85. Rajaee SS, Bae HW, Kanim LE, Delamarter RB. Spinal fusion in the United States: analysis of trends from 1998 to 2008. Spine. (2012) 37:67–76. doi: 10.1097/BRS.0b013e31820cccfb
86. Lee CK, Langrana NA. Lumbosacral spinal fusion. A biomechanical study. Spine. (1984) 9:574–81. doi: 10.1097/00007632-198409000-00007
87. Scemama C, Magrino B, Gillet P, Guigui P. Risk of adjacent-segment disease requiring surgery after short lumbar fusion: results of the French spine surgery society series. J Neurosurg Spine. (2016) 25:46–51. doi: 10.3171/2015.11.SPINE15700
88. Bagheri SR, Alimohammadi E, Zamani Froushani A, Abdi A. Adjacent segment disease after posterior lumbar instrumentation surgery for degenerative disease: Incidence and risk factors. J Orthop Surg. (2019) 27:2309499019842378. doi: 10.1177/2309499019842378
89. de la Garza-Ramos R, Kerezoudis P, Sciubba DM, Bydon A, Witham TF, Bydon M. The effect of preoperative diagnosis on the incidence of adjacent segment disease after lumbar fusion. J Neurosurg Sci. (2018) 62:4–9. doi: 10.23736/S0390-5616.16.03492-5
90. Bydon M, Macki M, Kerezoudis P, Sciubba DM, Wolinsky JP, Witham TF, et al. The incidence of adjacent segment disease after lumbar discectomy: a study of 751 patients. J Clin Neurosci. (2017) 35:42–6. doi: 10.1016/j.jocn.2016.09.027
91. Sato S, Yagi M, Machida M, Yasuda A, Konomi T, Miyake A, et al. Reoperation rate and risk factors of elective spinal surgery for degenerative spondylolisthesis: minimum 5-year follow-up. Spine J. (2015) 15:1536–44. doi: 10.1016/j.spinee.2015.02.009
92. Nagamoto Y, Okuda S, Matsumoto T, Sugiura T, Takahashi Y, Iwasaki M. Multiple-Repeated adjacent segment disease after posterior lumbar interbody fusion. World Neurosurg. (2019) 121:e808–16. doi: 10.1016/j.wneu.2018.09.227
93. Ramirez-Villaescusa J, Lopez-Torres Hidalgo J, Martin-Benlloch A, Ruiz-Picazo D, Gomar-Sancho F. Risk factors related to adjacent segment degeneration: retrospective observational cohort study and survivorship analysis of adjacent unfused segments. Br J Neurosurg. (2019) 33:17–24. doi: 10.1080/02688697.2018.1523365
94. Tobert DG, Antoci V, Patel SP, Saadat E, Bono CM. Adjacent segment disease in the cervical and lumbar spine. Clin Spine Surg. (2017) 30:94–101. doi: 10.1097/BSD.0000000000000442
95. Wang H, Ma L, Yang D, Wang T, Liu S, Yang S, et al. Incidence and risk factors of adjacent segment disease following posterior decompression and instrumented fusion for degenerative lumbar disorders. Medicine. (2017) 96:e6032. doi: 10.1097/MD.0000000000006032
96. Drysch A, Ajiboye RM, Sharma A, Li J, Reza T, Harley D, et al. Effectiveness of reoperations for adjacent segment disease following lumbar spinal fusion. Orthopedics. (2018) 41:e161–7. doi: 10.3928/01477447-20170621-02
97. Xia XP, Chen HL, Cheng HB. Prevalence of adjacent segment degeneration after spine surgery: a systematic review and meta-analysis. Spine. (2013) 38:597–608. doi: 10.1097/BRS.0b013e318273a2ea
98. Bae JS, Lee SH, Kim JS, Jung B, Choi G. Adjacent segment degeneration after lumbar interbody fusion with percutaneous pedicle screw fixation for adult low-grade isthmic spondylolisthesis: minimum 3 years of follow-up. Neurosurgery. (2010) 67:1600–7; discussion 1607–8. doi: 10.1227/NEU.0b013e3181f91697
99. Hashimoto K, Aizawa T, Kanno H, Itoi E. Adjacent segment degeneration after fusion spinal surgery-a systematic review. Int Orthop. (2019) 43:987–93. doi: 10.1007/s00264-018-4241-z
100. Makanji H, Schoenfeld AJ, Bhalla A, Bono CM. Critical analysis of trends in lumbar fusion for degenerative disorders revisited: influence of technique on fusion rate and clinical outcomes. Eur Spine J. (2018) 27:1868–76. doi: 10.1007/s00586-018-5544-x
101. Zencica P, Chaloupka R, Hladikova J, Krbec M. [Adjacent segment degeneration after lumbosacral fusion in spondylolisthesis: a retrospective radiological and clinical analysis]. Acta Chir Orthop Traumatol Cech. (2010) 77:124–30.
102. Zhong ZM, Deviren V, Tay B, Burch S, Berven SH. Adjacent segment disease after instrumented fusion for adult lumbar spondylolisthesis: incidence and risk factors. Clin Neurol Neurosurg. (2017) 156:29–34. doi: 10.1016/j.clineuro.2017.02.020
103. Bydon M, De la Garza-Ramos R, Abt NB, Gokaslan ZL, Wolinsky JP, Sciubba DM, et al. Impact of smoking on complication and pseudarthrosis rates after single- and 2-level posterolateral fusion of the lumbar spine. Spine. (2014) 39:1765–70. doi: 10.1097/BRS.0000000000000527
104. Raizman NM, O'Brien JR, Poehling-Monaghan KL, Yu WD. Pseudarthrosis of the spine. J Am Acad Orthop Surg. (2009) 17:494–503. doi: 10.5435/00124635-200908000-00003
105. Khalid SI, Nunna RS, Maasarani S, Belmont E, Deme P, Chilakapati S, et al. Association of osteopenia and osteoporosis with higher rates of pseudarthrosis and revision surgery in adult patients undergoing single-level lumbar fusion. Neurosurg Focus. (2020) 49:E6. doi: 10.3171/2020.5.FOCUS20289
106. Liao JC, Chiu PY, Chen WJ, Chen LH, Niu CC. Surgical outcomes after instrumented lumbar surgery in patients of eighty years of age and older. BMC Musculoskelet Disord. (2016) 17:402. doi: 10.1186/s12891-016-1239-9
107. Lazennec JY, Ramare S, Arafati N, Laudet CG, Gorin M, Roger B, et al. Sagittal alignment in lumbosacral fusion: relations between radiological parameters and pain. Eur Spine J. (2000) 9:47–55. doi: 10.1007/s005860050008
108. Lee BH, Park JO, Kim HS, Suk KS, Lee SY, Lee HM, et al. Spinal sagittal balance status affects postoperative actual falls and quality of life after decompression and fusion in-situ surgery in patients with lumbar spinal stenosis. Clin Neurol Neurosurg. (2016) 148:52–9. doi: 10.1016/j.clineuro.2016.06.016
109. Cho KJ, Suk SI, Park SR, Kim JH, Kang SB, Kim HS, et al. Risk factors of sagittal decompensation after long posterior instrumentation and fusion for degenerative lumbar scoliosis. Spine. (2010) 35:1595–601. doi: 10.1097/BRS.0b013e3181bdad89
110. Coleman RF, Herrington J, Scales JT. Concentration of wear products in hair, blood, and urine after total hip replacement. Br Med J. (1973) 1:527–9. doi: 10.1136/bmj.1.5852.527
111. Urban RM, Tomlinson MJ, Hall DJ, Jacobs JJ. Accumulation in liver and spleen of metal particles generated at nonbearing surfaces in hip arthroplasty. J Arthroplasty. (2004) 19 (8 Suppl. 3):94–101. doi: 10.1016/j.arth.2004.09.013
112. Wyles CC, Wright TC, Bois MC, Amin MS, Fayyaz A, Jenkins SM, et al. Myocardial cobalt levels are elevated in the setting of total hip arthroplasty. J Bone Joint Surg Am. (2017) 99:e118. doi: 10.2106/JBJS.17.00159
113. Bradberry SM, Wilkinson JM, Ferner RE. Systemic toxicity related to metal hip prostheses. Clin Toxicol. (2014) 52:837–47. doi: 10.3109/15563650.2014.944977
114. Cheung AC, Banerjee S, Cherian JJ, Wong F, Butany J, Gilbert C, et al. Systemic cobalt toxicity from total hip arthroplasties: review of a rare condition part 1 - history, mechanism, measurements, and pathophysiology. Bone Joint J. (2016) 98-B:6–13. doi: 10.1302/0301-620X.98B1.36374
115. Devlin JJ, Pomerleau AC, Brent J, Morgan BW, Deitchman S, Schwartz M. Clinical features, testing, and management of patients with suspected prosthetic hip-associated cobalt toxicity: a systematic review of cases. J Med Toxicol. (2013) 9:405–15. doi: 10.1007/s13181-013-0320-0
116. Zywiel MG, Cherian JJ, Banerjee S, Cheung AC, Wong F, Butany J, et al. Systemic cobalt toxicity from total hip arthroplasties: review of a rare condition part 2. Measurement, risk factors, and step-wise approach to treatment. Bone Joint J. (2016) 98-B:14–20. doi: 10.1302/0301-620X.98B1.36712
117. Fox KA, Phillips TM, Yanta JH, Abesamis MG. Fatal cobalt toxicity after total hip arthroplasty revision for fractured ceramic components. Clin Toxicol. (2016) 54:874–7. doi: 10.1080/15563650.2016.1214274
118. Milosev L, Antolic V, Minovic A, Cor A, Herman S, Pavlovcic V, et al. Extensive metallosis and necrosis in failed prostheses with cemented titanium-alloy stems and ceramic heads. J Bone Joint Surg Br. (2000) 82:352–7. doi: 10.1302/0301-620X.82B3.0820352
119. Ayers R, Miller M, Schowinsky J, Burger E, Patel V, Kleck C. Three cases of metallosis associated with spine instrumentation. J Mater Sci Mater Med. (2017) 29:3. doi: 10.1007/s10856-017-6011-7
120. Pesce V, Maccagnano G, Vicenti G, Notarnicola A, Lovreglio P, Soleo L, et al. First case report of vanadium metallosis after ceramic-on-ceramic total hip arthroplasty. J Biol Regul Homeost Agents. (2013) 27:1063–8.
121. Matharu GS, Berryman F, Brash L, Pynsent PB, Dunlop DJ, Treacy RB. Can blood metal ion levels be used to identify patients with bilateral birmingham hip resurfacings who are at risk of adverse reactions to metal debris? Bone Joint J. (2016) 98-B:1455–62. doi: 10.1302/0301-620X.98B11.38042
122. Ganesh BKC, Ramanaih N. Effect of heat treatment on dry sliding wear of Ti-6Al-4V and Ti-6Al-7Nb implant alloys. Int J Microstruc Mater Propert. (2013) 8:171–84. doi: 10.1504/IJMMP.2013.055382
123. Singh V, Shorez JP, Mali SA, Hallab NJ, Gilbert JL. Material dependent fretting corrosion in spinal fusion devices: evaluation of onset and long-term response. J Biomed Mater Res B Appl Biomater. (2018) 106:2858–68. doi: 10.1002/jbm.b.34067
124. Wang JC, Yu WD, Sandhu HS, Betts F, Bhuta S, Delamarter RB. Metal debris from titanium spinal implants. Spine. (1999) 24:899–903. doi: 10.1097/00007632-199905010-00011
125. Kasai Y, Iida R, Uchida A. Metal concentrations in the serum and hair of patients with titanium alloy spinal implants. Spine. (2003) 28:1320–6. doi: 10.1097/01.BRS.0000065482.41115.B4
126. Richardson TD, Pineda SJ, Strenge KB, Van Fleet TA, MacGregor M, Milbrandt JC, et al. Serum titanium levels after instrumented spinal arthrodesis. Spine. (2008) 33:792–6. doi: 10.1097/BRS.0b013e318169574d
127. Teoh KH, von Ruhland C, Evans SL, James SH, Jones A, Howes J, et al. Metallosis following implantation of magnetically controlled growing rods in the treatment of scoliosis: a case series. Bone Joint J. (2016) 98-B:1662–7. doi: 10.1302/0301-620X.98B12.38061
128. Yang JH, Ham CH, Hwang YG, Suh SW. Metallosis: a complication in the guided growing rod system used in treatment of scoliosis. Indian J Orthop. (2017) 51:714–8. doi: 10.4103/0019-5413.217692
129. Sherman B, Crowell T. Corrosion of harrington rod in idiopathic scoliosis: long-term effects. Eur Spine J. (2018) 27 (Suppl. 3):298–302. doi: 10.1007/s00586-017-5183-7
130. Siddiqi O, Urquhart JC, Rasoulinejad P. A systematic review of metal ion concentrations following instrumented spinal fusion. Spine Deform. (2021) 9:13–40. doi: 10.1007/s43390-020-00177-3
131. Gaine WJ, Andrew SM, Chadwick P, Cooke E, Williamson JB. Late operative site pain with isola posterior instrumentation requiring implant removal: infection or metal reaction? Spine. (2001) 26:583–7. doi: 10.1097/00007632-200103010-00027
132. Senaran H, Atilla P, Kaymaz F, Acaroglu E, Surat A. Ultrastructural analysis of metallic debris and tissue reaction around spinal implants in patients with late operative site pain. Spine. (2004) 29:1618–23; discussion 1623. doi: 10.1097/01.BRS.0000133646.40087.8B
133. Li YC, Yang SC, Hsu CT, Tu YK. Capsulated metallic debris tumor mass mimicking adjacent segment disease: a case report. Clin Spine Surg. (2016) 29:E532–5. doi: 10.1097/BSD.0b013e318292e685
134. Fernandez-Baillo N, Sanchez Marquez JM, Conde Gallego E, Martin Esteban A. Intraspinal metalloma causing lumbar stenosis after interbody fusion with cylindrical titanium cages. Acta Orthop Belg. (2012) 78:811–4.
135. Tezer M, Kuzgun U, Hamzaoglu A, Ozturk C, Kabukcuoglu F, Sirvanci M. Intraspinal metalloma resulting in late paraparesis. Arch Orthop Trauma Surg. (2005) 125:417–21. doi: 10.1007/s00402-005-0802-x
136. Sabokbar A, Kudo O, Athanasou NA. Two distinct cellular mechanisms of osteoclast formation and bone resorption in periprosthetic osteolysis. J Orthop Res. (2003) 21:73–80. doi: 10.1016/S0736-0266(02)00106-7
137. Vermes C, Chandrasekaran R, Jacobs JJ, Galante JO, Roebuck KA, Glant TT. The effects of particulate wear debris, cytokines, and growth factors on the functions of MG-63 osteoblasts. J Bone Joint Surg Am. (2001) 83:201–11. doi: 10.2106/00004623-200102000-00007
138. Hallab NJ, Cunningham BW, Jacobs JJ. Spinal implant debris-induced osteolysis. Spine. (2003) 28:S125–38. doi: 10.1097/00007632-200310151-00006
139. Botolin S, Merritt C, Erickson M. Aseptic loosening of pedicle screw as a result of metal wear debris in a pediatric patient. Spine. (2013) 38:E38–42. doi: 10.1097/BRS.0b013e3182793e51
140. Templeton PA, Zerhouni E. Computed tomography of the solitary pulmonary nodule and focal pulmonary disease. Curr Opin Radiol. (1989) 1:25–30.
141. Hallab NJ. A review of the biologic effects of spine implant debris: fact from fiction. SAS J. (2009) 3:143–60. doi: 10.1016/j.esas.2009.11.005
142. Kim J. A rare case of delayed hypersensitivity reaction to metal ions secondary to a remnant pedicle screw fragment after spinal arthrodesis. Acta Orthop Traumatol Turc. (2020) 54:461–4. doi: 10.5152/j.aott.2020.20148
143. Towers WS, Kurtom K. Rare systemic response to titanium spinal fusion implant: case report. Cureus. (2020) 12:e7109. doi: 10.7759/cureus.7109
144. Gordon PM, White MI, Scotland TR. Generalized sensitivity from an implanted orthopaedic antibiotic minichain containing nickel. Contact Dermatitis. (1994) 30:181–2. doi: 10.1111/j.1600-0536.1994.tb00709.x
145. King L Jr., Fransway A, Adkins RB. Chronic urticaria due to surgical clips. N Engl J Med. (1993) 329:1583–4. doi: 10.1056/NEJM199311183292121
146. Todd NV. The surgical treatment of non-specific low back pain. Bone Joint J. (2017) 99-B:1003–5. doi: 10.1302/0301-620X.99B8.BJJ-2017-0199.R1
147. Teles AR, Yavin D, Zafeiris CP, Thomas KC, Lewkonia P, Nicholls FH, et al. Fractures after removal of spinal instrumentation: revisiting the stress-shielding effect of instrumentation in spine fusion. World Neurosurg. (2018) 116:e1137–43. doi: 10.1016/j.wneu.2018.05.187
148. Mataki K, Fukushima M, Kaneoka K, Ikeda K, Kumagai H, Nagashima K, et al. Vertebral fracture after removing pedicle screws used for posterior lumbar interbody fusion: a case report. J Clin Neurosci. (2018) 57:182–4. doi: 10.1016/j.jocn.2018.04.019
149. Harris RI, Wiley JJ. Acquired spondylolysis as a sequel to spine fusion. J Bone Joint Surg Am. (1963) 45:1159–70. doi: 10.2106/00004623-196345060-00003
150. Ha KY, Kim YH. Bilateral pedicle stress fracture after instrumented posterolateral lumbar fusion: a case report. Spine. (2003) 28:E158–60. doi: 10.1097/01.BRS.0000058951.16468.24
151. Macdessi SJ, Leong AK, Bentivoglio JE. Pedicle fracture after instrumented posterolateral lumbar fusion: a case report. Spine. (2001) 26:580–2. doi: 10.1097/00007632-200103010-00026
152. Kim SK, Chung JY, Seo HY, Lee WG. Vertebral compression fracture within a solid fusion mass without trauma after removal of pedicle screws. Spine J. (2016) 16:e219–23. doi: 10.1016/j.spinee.2015.11.053
153. Cappuccio M, De Iure F, Amendola L, Martucci A. Vertebral body compression fracture after percutaneous pedicle screw removal in a young man. J Orthop Traumatol. (2015) 16:343–5. doi: 10.1007/s10195-014-0328-5
154. Sciubba DM, Scheer JK, Smith JS, Lafage V, Klineberg E, Gupta M, et al. Which daily functions are most affected by stiffness following total lumbar fusion: comparison of upper thoracic and thoracolumbar proximal endpoints. Spine. (2015) 40:1338–44. doi: 10.1097/BRS.0000000000000968
155. Harrison Farber S, Nayar G, Desai R, Reiser EW, Byrd SA, Chi D, et al. Radiation exposure to the surgeon during minimally invasive spine procedures is directly estimated by patient dose. Eur Spine J. (2018) 27:1911–7. doi: 10.1007/s00586-018-5653-6
156. Kaminski L, Cordemans V, Cartiaux O, Van Cauter M. Radiation exposure to the patients in thoracic and lumbar spine fusion using a new intraoperative cone-beam computed tomography imaging technique: a preliminary study. Eur Spine J. (2017) 26:2811–7. doi: 10.1007/s00586-017-4968-z
157. Pitteloud N, Gamulin A, Barea C, Damet J, Racloz G, Sans-Merce M. Radiation exposure using the O-arm((R)) surgical imaging system. Eur Spine J. (2017) 26:651–7. doi: 10.1007/s00586-016-4773-0
158. Hobbs JB, Goldstein N, Lind KE, Elder D, Dodd GD III, Borgstede JP. Physician knowledge of radiation exposure and risk in medical imaging. J Am Coll Radiol. (2018) 15 (1 Pt. A):34–43. doi: 10.1016/j.jacr.2017.08.034
159. Ukkola L, Oikarinen H, Henner A, Haapea M, Tervonen O. Patient information regarding medical radiation exposure is inadequate: patients' experience in a university hospital. Radiography. (2017) 23:e114–9. doi: 10.1016/j.radi.2017.04.001
160. Bohl DD, Hijji FY, Massel DH, Mayo BC, Long WW, Modi KD, et al. Patient knowledge regarding radiation exposure from spinal imaging. Spine J. (2017) 17:305–12. doi: 10.1016/j.spinee.2016.09.017
161. Kanat A, Yazar U, Kazdal H, Sonmez OF. Introducing a new risk factor for lumbar disc herniation in females: vertical angle of the sacral curvature. J Korean Neurosurg Soc. (2012) 52:447–51. doi: 10.3340/jkns.2012.52.5.447
162. Chen Y, Yang H, Wang Y, Zou J. Relationship of endplate changes and low back pain after discectomy. Clin Neurol Neurosurg. (2019) 184:105449. doi: 10.1016/j.clineuro.2019.105449
163. Feng ZY, Hu XJ, Zheng QQ, Battié MC, Chen Z, Wang Y. Cartilaginous endplate avulsion is associated with modic changes and endplate defects, and residual back and leg pain following lumbar discectomy. Osteoarthritis Cartilage. (2021) 29:707–17. doi: 10.1016/j.joca.2021.01.010
164. Kanat A, Yazar U, Ozdemir B, Kazdal H, Balik MS. Neglected knowledge: asymmetric features of lumbar disc disease. Asian J Neurosurg. (2017) 12:199–202.
165. Oba H, Takahashi J, Tsutsumimoto T, Ikegami S, Ohta H, Yui M, et al. Predictors of improvement in low back pain after lumbar decompression surgery: prospective study of 140 patients. J Orthop Sci. (2017) 22:641–6. doi: 10.1016/j.jos.2017.03.011
166. Akca N, Ozdemir B, Kanat A, Batcik OE, Yazar U, Zorba OU. Describing a new syndrome in L5-S1 disc herniation: sexual and sphincter dysfunction without pain and muscle weakness. J Craniovertebr Junction Spine. (2014) 5:146–50. doi: 10.4103/0974-8237.147076
168. Ozdemir B, Kanat A, Batcik OE, Erturk C, Celiker FB, Guvercin AR, et al. First report of perforation of ligamentum flavum by sequestrated lumbar intervertebral disc. J Craniovertebr Junction Spine. (2017) 8:70–3. doi: 10.4103/0974-8237.199867
169. Kanat A, Tsianaka E, Gasenzer ER, Drosos E. Some interesting points of competition of X-ray using during the greco-ottoman war in 1897 and development of neurosurgical radiology: a reminiscence. Turk Neurosurg. (2021) 8:70–3. doi: 10.5137/1019-5149.JTN.33484-20.3
Keywords: adjacent segment disease, instrumentation, lumbar disc herniation, lumbar fusion, metallosis
Citation: Wan ZY, Shan H, Liu TF, Song F, Zhang J, Liu ZH, Ma KL and Wang HQ (2022) Emerging Issues Questioning the Current Treatment Strategies for Lumbar Disc Herniation. Front. Surg. 9:814531. doi: 10.3389/fsurg.2022.814531
Received: 13 November 2021; Accepted: 04 March 2022;
Published: 28 March 2022.
Edited by:
Jaimo Ahn, University of Michigan, United StatesReviewed by:
Ali Fahir Ozer, Koç University, TurkeyFei-Long Wei, Fourth Military Medical University (Air Force Medical University), China
Ayhan Kanat, Recep Tayyip Erdoǧan University, Turkey
Copyright © 2022 Wan, Shan, Liu, Song, Zhang, Liu, Ma and Wang. This is an open-access article distributed under the terms of the Creative Commons Attribution License (CC BY). The use, distribution or reproduction in other forums is permitted, provided the original author(s) and the copyright owner(s) are credited and that the original publication in this journal is cited, in accordance with accepted academic practice. No use, distribution or reproduction is permitted which does not comply with these terms.
*Correspondence: Hai Q. Wang, ZHJ3YW5naHFAMTYzLmNvbQ==; aHF3YW5nQHNudGNtLmVkdS5jbg==
†These authors have contributed equally to this work