- 1Department of Orthopedic Surgery, Taichung Veterans General Hospital, Taichung, Taiwan
- 2Department of Physical Therapy, HungKuang University, Taichung, Taiwan
- 3Department of Nursing, Jenteh Junior College of Medicine, Nursing and Management, Miaoli, Taiwan
- 4Department of Computer Science and Information Engineering, College of Computing and Informatics, Providence University, Taichung, Taiwan
- 5Department of Biomedical Engineering, College of Intelligent Technology, HungKuang University, Taichung, Taiwan
- 6College of Medicine, National Chung Hsing University, Taichung, Taiwan
- 7Department of Food Science and Technology, Hungkuang University, Taichung, Taiwan
- 8Department of Rehabilitation Science, Jenteh Junior College of Medicine, Nursing and Management, Miaoli, Taiwan
Oblique lumbar interbody fusion (OLIF) is a popular technique for the treatment of degenerative lumbar spinal disease. There are no clear guidelines on whether direct posterior decompression (PD) is necessary after OLIF. The purpose of this study was to analyze the effect of the indirect decompression obtained from OLIF in patients with lumbar foraminal stenosis. We retrospectively reviewed 33 patients who underwent OLIF surgery for degenerative lumbar spinal disease between 1 January 2018, and 30 June 2019. The inclusion criteria included patients who were diagnosed with lumbar foraminal stenosis by preoperative MRI. The exclusion criteria included the presence of central canal stenosis, spinal infection, vertebral fractures, and spinal malignancies. The clinical results, evaluated using the visual analogue scale of back pain (VAS-Back), VAS of leg pain (VAS-Leg), and Oswestry disability index (ODI), were recorded. The radiologic parameters were also measured. The VAS-Back, VAS-Leg, and ODI showed significant improvement in both the PD and non-posterior decompression (Non-PD) groups postoperatively (all, p < 0.05). Patients in the Non-PD group showed better results than those in the PD group in the VAS-Back at 12- and 24 months postoperatively (0.00 vs. 3.00 postoperatively at 12 months, p = 0.030; 0.00 vs. 4.00 postoperatively at 24 months, p = 0.009). In addition, the ODI at 24 months postoperatively showed better improvement in the Non-PD group (8.89 vs. 24.44, p = 0.038). The disc height in both the PD and the Non-PD groups increased significantly postoperatively (all, p < 0.05), but the restoration of foraminal height was significantly different only in the Non-PD group. There was no statistically significant difference in cage position, cage subsidence, fusion grade, or screw loosening between the PD and the Non-PD groups. Indirect decompression via OLIF for lumbar foraminal stenosis showed favorable outcomes. The use of interbody cages and posterior instrumentation was sufficient for relieving symptoms in patients with lumbar foraminal stenosis. Additional direct posterior decompression may deteriorate results in the follow-up period.
Introduction
Spinal fusion is a popular surgical treatment for degenerative lumbar spinal disease such as spinal stenosis, spondylolisthesis, or disc herniation (1). There are various lumbar spinal fusion techniques, including anterior lumbar interbody fusion, posterior lumbar interbody fusion (PLIF), and transforaminal lumbar interbody fusion (TLIF). The retroperitoneal approach, which was first introduced by Mayer in 1997, is a minimally invasive technique for decreasing surgery-related comorbidities (2). Several modifications of this technique were developed in subsequent years. Silvestre et al. used a similar approach, which is referred to as oblique lumbar interbody fusion (OLIF), and presented the first results about complications and morbidities (3). OLIF has the advantages of less blood loss, shorter hospital stays, and faster recovery when compared with conventional posterior approaches (4). Previous studies have confirmed its achievement of indirect neural decompression through the restoration of disc height and extension of the thecal sac (5). Shimizu et al. demonstrated that OLIF had good short-term clinical outcomes, comparable to those obtained with TLIF and PLIF, for severe degenerative lumbar stenosis (6). Some authors have stated that indirect decompression could achieve adequate neural decompression through direct lateral interbody fusion (DLIF), lateral lumbar interbody fusion (LLIF), or extreme lateral interbody fusion (XLIF) (7–9). The fundamental concept of these lateral interbody fusion techniques is the “indirect decompression” effect through the restoration of intervertebral disc height and foraminal height (FH). Shimizu et al. confirmed that lateral interbody fusion without posterior decompression (PD) achieved expansion of the thecal sac and restoration of disc height in severe canal stenosis (7). However, most of these studies have focused on DLIF, LLIF, and XLIF and it remains unclear whether indirect decompression alone is sufficient to relieve low back pain or radicular pain in patients receiving OLIF. Furthermore, 0%–60% of patients who received these indirect decompression procedures underwent additional posterior laminectomy (10, 11). The posterior decompression procedures have the advantage of the direct decompression of the nerve root. However, they may cause iatrogenic injuries to the paravertebral muscles and disruption of the posterior tension mechanism (12). There are no clear guidelines on whether direct posterior decompression is necessary after OLIF.
Therefore, the aim of this study was to analyze the effect of the indirect decompression obtained from OLIF in patients with lumbar foraminal stenosis (FS), in terms of clinical and radiologic outcomes. We also investigated whether additional direct posterior decompression affected the outcomes in these patients.
Methods
We retrospectively reviewed 33 patients who underwent OLIF surgery for degenerative lumbar spinal disease between 1 January 2018 and 30 June 2019. The inclusion criteria included patients who were diagnosed with lumbar FS by preoperative MRI. The radiologic criteria of lumbar spinal stenosis were summarized in a systematic review article (13). FS is diagnosed by nerve root compression in the foraminal zone with obliteration of the perineural intraforaminal fat (14). The exclusion criteria included the presence of central canal stenosis, spinal infection, vertebral fractures, and spinal malignancies. These patients were divided into posterior decompression (PD) or non-posterior decompression (Non-PD) groups according to whether direct posterior decompression was performed. The minimum follow-up period was at least 24 months. All the surgeries were performed by experienced spine surgeons at our institute.
We performed the OLIF procedure as described by Woods et al. (15). The Clydesdale cage (Medtronic, TN, USA) was used, and a morselized bone allograft or synthetic bone graft substitute (Actifuse, Baxter, IL, USA) was packed into the cage to enable fusion. After the performance of the OLIF procedures, posterior instrumentation with pedicle screws was used in all cases. Importantly, the surgeons informed patients of the pros and cons of additional posterior decompression procedure before the operation. Proper suggestions were provided by the surgeons, and the patients made the final decision on whether the posterior decompression procedure was performed. If direct posterior decompression was planned, the surgeons decided the exact procedure, including laminectomy, laminotomy, or discectomy according to their experience and preference. All the posterior decompression procedures were performed before the insertion of pedicle screws. Adequate decompression of the dural sac and nerve roots at lateral recess and neuroforamen was checked meticulously, and hemostasis was performed (Figure 1).
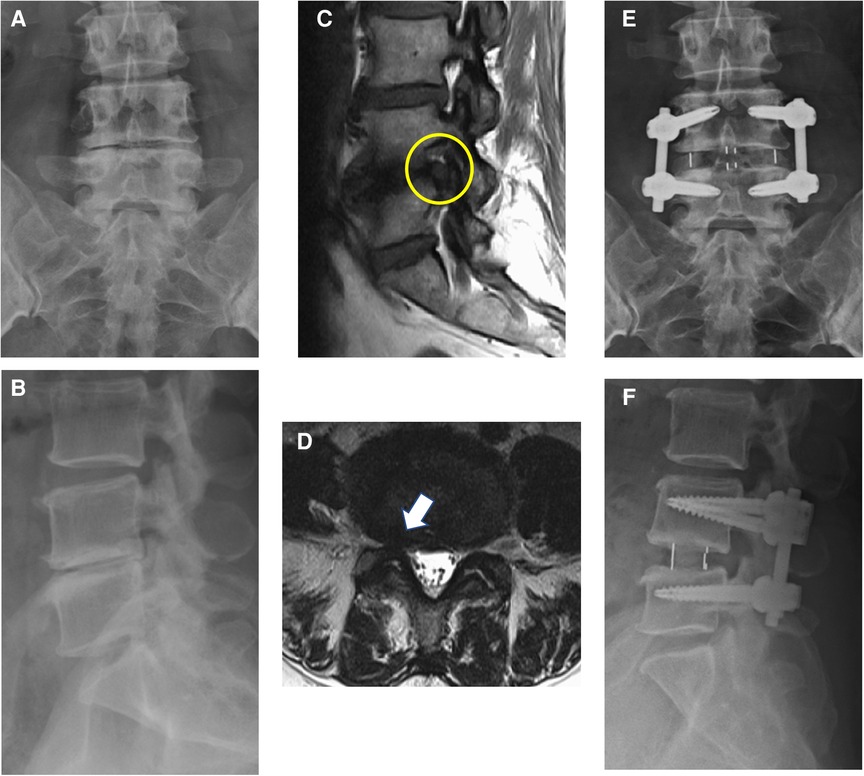
Figure 1. Anterior–posterior view (A) and lateral view (B) of a 67-year-old woman with degenerative disc disease. Sagittal T1-weighted MRI (C) and axial T2-weighted MRI (D) showed foraminal stenosis of L4/L5 (yellow circle and white arrow). Postoperative anterior–posterior view (E) and lateral view (F) of this patient. OLIF, L4/L5, with posterior instrumentation was done.
The clinical outcomes were evaluated using the visual analogue scale of back pain (VAS-Back), VAS of leg pain (VAS-Leg), and Oswestry disability index (ODI), which were recorded preoperatively and at the postoperative 1-, 3-, 6-, 12-,and 24-month follow-ups. The minimum clinically important difference (MCID) for the patient-reported outcome measures in this study was defined as a 30% reduction from baseline of pain and disabilities (16). The radiologic parameters, including the index level of the anterior disc height (ADH), posterior disc height (PDH), average disc height (DH), FH, lumbar lordosis (LL), and segmental lordosis (SL), were measured preoperatively, postoperatively, and at the last follow-up in the outpatient clinic (Figure 2). Additionally, we analyzed the cage position and cage-related parameters at the last follow-up time. The normalized mean cage center position was defined as the value of the distance between the cage center to the posterior vertebral border divided by the width of inferior end plate on the lateral view of the x-ray (17). The grading of cage subsidence was determined according to Marchi et al. (18): Grade 0, 0%–24%; Grade I, 25%–49%; Grade II, 50%–74%; and Grade III, 75%–100% collapse of the vertebral end plate. The fusion grade was classified according to Ailon et al. (19): Grade I, definite union; Grade II, probable union; Grade III, probable non-union; Grade IV, definite non-union. Finally, the perioperative parameters and postoperative complications were recorded by chart review.
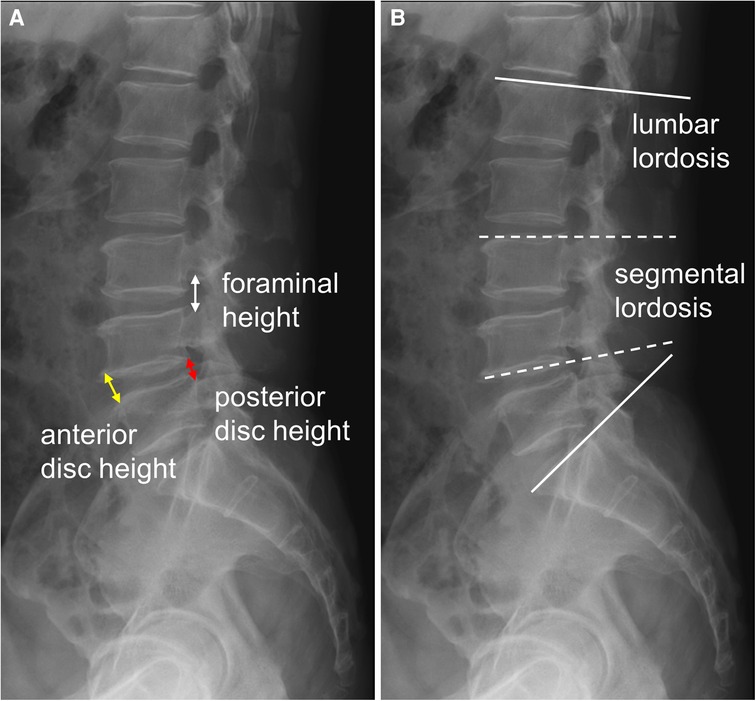
Figure 2. The radiologic parameters (A) anterior disc height (ADH, yellow arrow): the distance of the anterior disc space; posterior disc height (PDH, red arrow): the distance of the posterior disc space; average disc height (DH): (ADH + PDH)/2; foraminal height (FH, white arrow): the distance between the pedicles of upper and lower levels; (B) lumbar lordosis (LL, solid line): the angle of L1 to S1 upper endplate; segmental lordosis (SL, dotted line): the angle of the upper endplate of the upper vertebra and the lower endplate of the lower vertebra of the index level.
Statistical analyses
Statistical analyses were performed using the Statistical Package for the Social Sciences (IBM SPSS version 22.0; International Business Machines Corp., New York, USA). The Friedman test was used for comparison of the postoperative values of each clinical and radiologic outcome with the preoperative values. The Bonferroni test was used for the post hoc analysis. The chi-square test was used to compare the qualitative variables between the groups, and the Mann–Whitney U test to compare the quantitative variables between the groups. A p-value < 0.05 was statistically significant.
Results
A total of 33 patients were included in this study, with 16 patients in the Non-PD group and 17 patients in the PD group. There were no significant differences between the two groups in terms of age, sex, BMI, operative levels, and follow-up time. The patient demographics are given in Table 1.
The VAS-Back, VAS-Leg, and ODI showed significant improvement in both the PD and Non-PD groups postoperatively (all, p < 0.05) (Table 2). All the pain scales achieved MCID (a reduction of 2.4 points for the VAS of the back and leg) postoperatively at the 1-month follow-up. The disability scores achieved MCID (a reduction of 16.67 points for ODI) at 3 months postoperatively in the Non-PD group and at 6 months postoperatively in the PD group.
Patients in the Non-PD group showed better results than those in the PD group in the VAS-Back at 12 months and 24 months postoperatively (0.00 vs. 3.00 postoperatively at 12 months, p = 0.030; 0.00 vs. 4.00 postoperatively at 24 months, p = 0.009) (Figure 3). In addition, the ODI at 24 months postoperatively showed better improvement in the Non-PD group (8.89 vs. 24.44, p = 0.038).
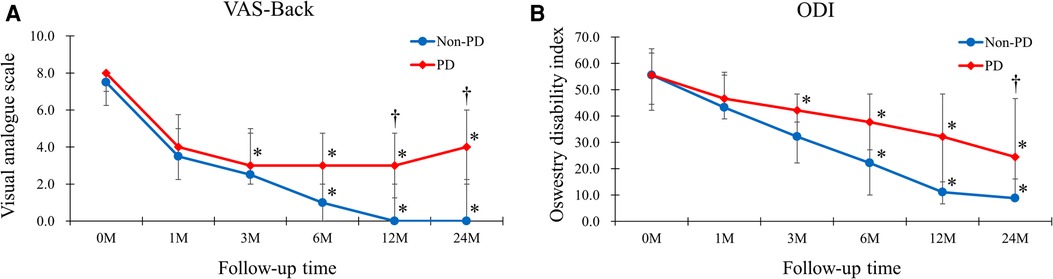
Figure 3. The VAS-Back and ODI of the Non-PD and PD groups. (A) Patients in the Non-PD group had better results than those of PD group in the VAS-Back at 12-months and 24-months postoperatively (0.00 vs. 3.00 postoperatively at 12-months, p = 0.030; 0.00 vs. 4.00 postoperatively at 24-months, p = 0.009). (B) The ODI at 24-months postoperatively showed better improvement in the Non-PD group (8.89 vs. 24.44, p = 0.038).
The ADH, PDH, and DH in both the PD and Non-PD groups increased significantly postoperatively (all, p < 0.05) (Table 3). The results were obtained at the last follow-up. The restoration of FH was significantly different only in the Non-PD group. However, the LL and SL had no significant increase after OLIF in both groups.
A comparison of the PD and Non-PD groups showed that the latter had a better improvement ratio in terms of ADH, PDH, and DH than the former. There was no significant difference in FH, LL, and SL between the two groups.
There was no statistically significant difference in cage position, cage subsidence, fusion grade, or screw loosening between the PD and the Non-PD groups (Table 4). High-grade cage subsidence (Grades II and III) occurred in 18.2% patients of the Non-PD group and 13% patients of the PD group. Importantly, all the patients achieved adequate spinal fusion on image presentation at the last follow-up time.
The estimated blood loss was similar in both groups (Table 5). Regarding postoperative minor complications, only one patient in each group experienced postoperative ileus. Numbness of the thigh occurred in three patients (18.8%) in the Non-PD group and two patients (11.8%) in the PD group. Besides, in the PD group, one patient had dural tear and another one had superficial wound infection. No major complication or reoperation was recorded in either group.
Discussion
Lumbar interbody fusion techniques such as TLIF and PLIF have become well-developed methods for treating degenerative lumbar spinal disease (1). These posterior approaches could decompress the neural elements directly and provide initial stability through the use of interbody cages and pedicle screws. Nevertheless, the posterior structures would be damaged simultaneously (12). OLIF is a lateral-approach technique using the corridor between the psoas muscle and the aorta. It avoids violations of the psoas and lumbosacral plexus injuries and has a high fusion rate (15). It has been shown to significantly improve clinical outcomes, and its fusion rate was 97.9% at 6 months (15). Our data showed a comparatively high fusion rate, with all patients having achieved successful fusion at the 2-year follow-up period. Another study showed that stand-alone minimally invasive lateral interbody fusion could relieve neurologic symptoms and improve the quality of life in selected patient populations (20). Furthermore, the rate of high-grade cage subsidence was 9% and not related directly to the clinical outcomes (20). According to our results, 15% of patients exhibited high-grade cage subsidence. The phenomenon of subsidence was multifactorial, including bone mineral density, disc height, and cage position (21). There was no difference in the ratio of cage subsidence between the Non-PD and the PD groups. Additional posterior decompression procedure may not affect the probability of cage subsidence. Also, the cage was inserted a little anterior to the center of the lower end plate in both groups without intergroup difference in cage position. Yao et al. considered that anterior placement of the TLIF cage may reduce the risk of cage subsidence (21). A systematic review reported that the cage position had no influence on the indirect decompression effect in XLIF (22). More evidence is needed to confirm the relationship between cage position and indirect decompression effect.
Recently, some studies demonstrated that the “indirect decompression” effect via OLIF showed good short-term clinical and radiologic outcomes (5, 23). Kim et al. found that OLIF increased the DH and sagittal angle significantly at the 1-year follow-up. However, the FH did not change (24). Shimizu et al. demonstrated similar clinical outcomes between OLIF and conventional TLIF/PLIF in the treatment of severe spinal stenosis, while OLIF was shown to have better radiographic outcomes (6). For adjacent segment disease after posterior lumbar fusion, OLIF has better short-term clinical outcomes and DH restoration than PLIF (4).
In our study, the neurologic symptoms caused by foraminal stenosis were much improved after OLIF. This means the radicular pain caused by nerve root compression at neuroforamen was efficiently relieved by means of the “indirect decompression” effect obtained from OLIF. In addition, the Non-PD patients showed better clinical results than those in the PD group in the VAS score for back pain at 12- and 24 months postoperatively and ODI at 24 months postoperatively. Theoretically, direct posterior decompression such as laminectomy or laminotomy could decompress the neural elements directly. The osteophytes and redundant ligamentum flavum can be removed meticulously, and the nerve root can be released. However, some authors have found that a posterior decompression procedure may cause iatrogenic injuries to the paraspinal musculature and disruptions of the posterior bony structure (12, 25). These additional procedures may contribute to paraspinal muscle atrophy and compromise the result of the index procedure (26). Besides, the integrity of the posterior complex between the fused segments and the adjacent segments could be damaged in laminectomy or laminotomy after lumbar spinal fusion (27). The development of adjacent instability would deteriorate the outcomes of spinal fusion and may lead to adjacent segment disease in the future. This is the reason why the patients in the PD group still had back pain in the 24-month follow-up period. In our opinion, the efficacy of indirect decompression is sufficient for lumbar FS. Additional direct posterior decompression is not necessary in these cases.
On the other hand, OLIF restored the ADH, PDH, and DH effectively by the implantation of a larger interbody cage via the lateral approach. This result is compatible with previous studies. Sato et al. confirmed that OLIF can significantly improve the DH and spinal canal area (28). The clinical symptoms were relieved by reducing the bulging disc and stretching the redundant ligamentum flavum. Furthermore, the improvement ratio of these parameters is greater in the Non-PD group than in the PD group. The reason may be that the collapse of intervertebral discs was more severe in the Non-PD group preoperatively. More potential restoration of DH is expected. Surprisingly, the FH was increased only in the Non-PD group postoperatively. Chang et al. stated that OLIF showed favorable outcomes in the restoration of FH and that the improvement ratio of the FH was correlated with radicular pain and disability (29). This may explain why patients in the Non-PD group had better clinical outcomes than those in the PD group.
There is no significant improvement in LL and SL after OLIF in this study. Previous literature stated that LLIF had great capacity for coronal deformity correction, but the ability to achieve sagittal plane correction is limited (30). Recently, some studies showed marked sagittal deformity correction in OLIF (31, 32). More studies are needed to discuss the change in sagittal parameters in OLIF.
There are some debates on the indirect decompression effect of the lateral interbody fusion technique. Wang et al. described evidence that bony lateral recess stenosis is an independent risk factor for the failure of the indirect decompression in XLIF (33). Oliveira et al. concluded that congenital stenosis or locked facets may limit the efficacy of indirect decompression in XLIF (10). XLIF is relatively contraindicated for severe central spinal stenosis due to a risk of the need for secondary operation. In addition, a previous study suggested open laminectomy in the presence of fused facet joints or large herniated discs (34). However, some studies had the opposite opinion about these points. Malham et al. and Park et al. reported that facet degeneration does not impair the amount of direct decompression in XLIF (25, 35). Another study announced that locked facets are not a relative contraindication for XLIF (36). A recent systematic review found that only severe central canal stenosis in preoperative images is likely to cause failure of indirect decompression in XLIF (22). In contrast to the experience in XLIF, most articles about OLIF excluded these factors (6, 7, 29). According to our reports, the indirect decompression effect via OLIF is sufficient for lumbar FS. If the patients' symptoms were mainly caused by neural compression at neuroforamen, OLIF without direct posterior decompression is a reasonable treatment. Additional direct decompression may be not beneficial in these cases, irrespective of whether facet degeneration is present. However, if the patients are diagnosed with severe central canal stenosis, obvious osteophyte compromising lateral recess, or large disc herniation, direct posterior decompression is considered.
OLIF is a relatively safe procedure with few postoperative minor complications. Postoperative ileus occurred in two patients due to a manipulation of the retroperitoneum. The symptoms improved during hospitalization. About 15% of patients experienced numbness of the anterior thigh after operation with intact motor function. The sensory deficit may be caused by a retraction of the genitofemoral nerve (24), and it was relieved spontaneously within three months of follow-up at the outpatient clinic. Dural tear happened in one patient when laminectomy was performed. The tear site was repaired by tissue glue and CSF leakage was checked meticulously. The patient had no associated complication afterward.
This study had some limitations. First, this was a retrospective study. The plan for additional direct posterior decompression depended on the patients' decision and the surgeons' preference. This may have led to a patient selection bias. Second, this was a mid-term follow-up study, with a median follow-up time of 31.69 months. Third, this was a single-center study, and thus, its generalizability may be inadequate. Fourth, the number of patients was limited, and a larger sample size is necessary in further studies.
The use of OLIF for lumbar FS showed favorable clinical and radiologic outcomes during the 2-year follow-up period. Moreover, the use of interbody cages and posterior instrumentation without direct decompression was sufficient for the relief of symptoms in patients with lumbar FS. Additionally, direct posterior decompression may not be necessary in these patients.
Data availability statement
The raw data supporting the conclusions of this article will be made available by the authors, without undue reservation.
Ethics statement
The studies involving human participants were reviewed and approved by The Ethics Committee of Taichung Veterans General Hospital (protocol code: CE21055A). Written informed consent for participation was not required for this study in accordance with the national legislation and the institutional requirements.
Author contributions
S-CT, C-CP, and Y-HL contributed to the conception and design of the study. Y-CW and C-MS organized the database. S-CT wrote the first draft of the manuscript. K-HC, C-HL, C-CP, and S-CT wrote sections of the manuscript. All authors contributed to the article and approved the submitted version.
Funding
This research received no external funding.
Acknowledgments
The authors thank the Biostatistics Task Force of Taichung Veterans General Hospital and Mr. Chen Jun-Peng for statistical analysis.
Conflict of interest
The authors declare that the research was conducted in the absence of any commercial or financial relationships that could be construed as a potential conflict of interest.
Publisher's note
All claims expressed in this article are solely those of the authors and do not necessarily represent those of their affiliated organizations, or those of the publisher, the editors and the reviewers. Any product that may be evaluated in this article, or claim that may be made by its manufacturer, is not guaranteed or endorsed by the publisher.
References
1. de Kunder SL, Rijkers K, Caelers I, de Bie RA, Koehler PJ, van Santbrink H. Lumbar interbody fusion: a historical overview and a future perspective. Spine. (2018) 43(16):1161–8. doi: 10.1097/BRS.0000000000002534
2. Mayer HM. A new microsurgical technique for minimally invasive anterior lumbar interbody fusion. Spine. (1997) 22(6):691–9; discussion 700. doi: 10.1097/00007632-199703150-00023
3. Silvestre C, Mac-Thiong JM, Hilmi R, Roussouly P. Complications and morbidities of mini-open anterior retroperitoneal lumbar interbody fusion: oblique lumbar interbody fusion in 179 patients. Asian Spine J. (2012) 6(2):89–97. doi: 10.4184/asj.2012.6.2.89
4. Zhu G, Hao Y, Yu L, Cai Y, Yang X. Comparing stand-alone oblique lumbar interbody fusion with posterior lumbar interbody fusion for revision of rostral adjacent segment disease: a strobe-compliant study. Medicine (Baltimore). (2018) 97(40):e12680. doi: 10.1097/MD.0000000000012680
5. Fujibayashi S, Hynes RA, Otsuki B, Kimura H, Takemoto M, Matsuda S. Effect of indirect neural decompression through oblique lateral interbody fusion for degenerative lumbar disease. Spine. (2015) 40(3):E175–82. doi: 10.1097/BRS.0000000000000703
6. Shimizu T, Fujibayashi S, Otsuki B, Murata K, Matsuda S. Indirect decompression via oblique lateral interbody fusion for severe degenerative lumbar spinal stenosis: a comparative study with direct decompression transforaminal/posterior lumbar interbody fusion. Spine J. (2021) 21(6):963–71. doi: 10.1016/j.spinee.2021.01.025
7. Shimizu T, Fujibayashi S, Otsuki B, Murata K, Matsuda S. Indirect decompression with lateral interbody fusion for severe degenerative lumbar spinal stenosis: minimum 1-year MRI follow-up. J Neurosurg Spine. (2020) 33(1):27–34. doi: 10.3171/2020.1.SPINE191412
8. Scherman DB, Rao PJ, Phan K, Mungovan SF, Faulder K, Dandie G. Outcomes of direct lateral interbody fusion (DLIF) in an Australian cohort. J Spine Surg. (2019) 5(1):1–12. doi: 10.21037/jss.2019.01.08
9. Formica M, Quarto E, Zanirato A, Mosconi L, Vallerga D, Zotta I, et al. Lateral lumbar interbody fusion: what is the evidence of indirect neural decompression? A systematic review of the literature. Hss J. (2020) 16(2):143–54. doi: 10.1007/s11420-019-09734-7
10. Oliveira L, Marchi L, Coutinho E, Pimenta L. A radiographic assessment of the ability of the extreme lateral interbody fusion procedure to indirectly decompress the neural elements. Spine. (2010) 35(26 Suppl):S331–7. doi: 10.1097/BRS.0b013e3182022db0
11. Wang MY, Vasudevan R, Mindea SA. Minimally invasive lateral interbody fusion for the treatment of rostral adjacent-segment lumbar degenerative stenosis without supplemental pedicle screw fixation. J Neurosurg Spine. (2014) 21(6):861–6. doi: 10.3171/2014.8.Spine13841
12. Mobbs RJ, Phan K, Malham G, Seex K, Rao PJ. Lumbar interbody fusion: techniques, indications and comparison of interbody fusion options including PLIF, TLIF, MI-TLIF, OLIF/ATP, LLIF and ALIF. J Spine Surg. (2015) 1(1):2–18. doi: 10.3978/j.issn.2414-469X.2015.10.05
13. Andreisek G, Imhof M, Wertli M, Winklhofer S, Pfirrmann CW, Hodler J, et al. A systematic review of semiquantitative and qualitative radiologic criteria for the diagnosis of lumbar spinal stenosis. AJR Am J Roentgenol. (2013) 201(5):W735–46. doi: 10.2214/AJR.12.10163
14. Lee S, Lee JW, Yeom JS, Kim KJ, Kim HJ, Chung SK, et al. A practical MRI grading system for lumbar foraminal stenosis. AJR Am J Roentgenol. (2010) 194(4):1095–8. doi: 10.2214/AJR.09.2772
15. Woods KR, Billys JB, Hynes RA. Technical description of oblique lateral interbody fusion at L1–L5 (OLIF25) and at L5–S1 (OLIF51) and evaluation of complication and fusion rates. Spine J. (2017) 17(4):545–53. doi: 10.1016/j.spinee.2016.10.026
16. Asher AM, Oleisky ER, Pennings JS, Khan I, Sivaganesan A, Devin CJ, et al. Measuring clinically relevant improvement after lumbar spine surgery: is it time for something new? Spine J. (2020) 20(6):847–56. doi: 10.1016/j.spinee.2020.01.010
17. Siu TL, Najafi E, Lin K. A radiographic analysis of cage positioning in lateral transpsoas lumbar interbody fusion. J Orthop. (2017) 14(1):142–6. doi: 10.1016/j.jor.2016.10.028
18. Marchi L, Abdala N, Oliveira L, Amaral R, Coutinho E, Pimenta L. Radiographic and clinical evaluation of cage subsidence after stand-alone lateral interbody fusion. J Neurosurg Spine. (2013) 19(1):110–8. doi: 10.3171/2013.4.Spine12319
19. Ailon T, Hamilton DK, Klineberg E, Daniels AH, Lafage V, Bess S, et al. Radiographic fusion grade does not impact health-related quality of life in the absence of instrumentation failure for patients undergoing posterior instrumented fusion for adult spinal deformity. World Neurosurg. (2018) 117:e1–7. doi: 10.1016/j.wneu.2018.04.127
20. Ahmadian A, Bach K, Bolinger B, Malham GM, Okonkwo DO, Kanter AS, et al. Stand-alone minimally invasive lateral lumbar interbody fusion: multicenter clinical outcomes. J Clin Neurosci. (2015) 22(4):740–6. doi: 10.1016/j.jocn.2014.08.036
21. Yao YC, Chou PH, Lin HH, Wang ST, Liu CL, Chang MC. Risk factors of cage subsidence in patients received minimally invasive transforaminal lumbar interbody fusion. Spine. (2020) 45(19):E1279–e85. doi: 10.1097/brs.0000000000003557
22. Lang G, Perrech M, Navarro-Ramirez R, Hussain I, Pennicooke B, Maryam F, et al. Potential and limitations of neural decompression in extreme lateral interbody fusion: a systematic review. World Neurosurg. (2017) 101:99–113. doi: 10.1016/j.wneu.2017.01.080
23. Beng TB, Kotani Y, Sia U, Gonchar I. Effect of indirect neural decompression with oblique lateral interbody fusion was influenced by preoperative lumbar lordosis in adult spinal deformity surgery. Asian Spine J. (2019) 13(5):809–14. doi: 10.31616/asj.2018.0283
24. Kim D, Choi B, Kim HY, Lee S. Clinical and radiographic outcomes of oblique lumbar interbody fusion at 1 year: a preliminary report of a single institution experience. J Min Inv Spine Surg Techn. (2020) 5(1):1–7. doi: 10.21182/jmisst.2020.00087
25. Park D, Mummaneni PV, Mehra R, Kwon Y, Kim S, Ruan HB, et al. Predictors of the need for laminectomy after indirect decompression via initial anterior or lateral lumbar interbody fusion. J Neurosurg Spine. (2020) 32(6):781–7. doi: 10.3171/2019.11.SPINE19314
26. Pourtaheri S, Issa K, Lord E, Ajiboye R, Drysch A, Hwang K, et al. Paraspinal muscle atrophy after lumbar spine surgery. Orthopedics. (2016) 39(2):e209–14. doi: 10.3928/01477447-20160129-07
27. . Lai PL, Chen LH, Niu CC, Fu TS, Chen WJ. Relation between laminectomy and development of adjacent segment instability after lumbar fusion with pedicle fixation. Spine. (2004) 29(22):2527–32; discussion 32. doi: 10.1097/01.brs.0000144408.02918.20
28. Sato J, Ohtori S, Orita S, Yamauchi K, Eguchi Y, Ochiai N, et al. Radiographic evaluation of indirect decompression of mini-open anterior retroperitoneal lumbar interbody fusion: oblique lateral interbody fusion for degenerated lumbar spondylolisthesis. Eur Spine J. (2017) 26(3):671–8. doi: 10.1007/s00586-015-4170-0
29. Chang SY, Nam Y, Lee J, Chang BS, Lee CK, Kim H. Clinical significance of radiologic improvement following single-level oblique lateral interbody fusion with percutaneous pedicle screw fixation. Orthopedics. (2020) 43(4):e283–e90. doi: 10.3928/01477447-20200521-02
30. Taba HA, Williams SK. Lateral lumbar interbody fusion. Neurosurg Clin N Am. (2020) 31(1):33–42. doi: 10.1016/j.nec.2019.08.004
31. Park SW, Ko MJ, Kim YB, Le Huec JC. Correction of marked sagittal deformity with circumferential minimally invasive surgery using oblique lateral interbody fusion in adult spinal deformity. J Orthop Surg Res. (2020) 15(1):13. doi: 10.1186/s13018-020-1545-7
32. Cho MS, Seo EM. Efficacy and radiographic analysis of oblique lumbar interbody fusion in treating lumbar degenerative spondylolisthesis with sagittal imbalance. Neurosurg Rev. (2021) 44(4):2181–9. doi: 10.1007/s10143-020-01390-4
33. Wang TY, Nayar G, Brown CR, Pimenta L, Karikari IO, Isaacs RE. Bony lateral recess stenosis and other radiographic predictors of failed indirect decompression via extreme lateral interbody fusion: multi-institutional analysis of 101 consecutive spinal levels. World Neurosurg. (2017) 106:819–26. doi: 10.1016/j.wneu.2017.07.045
34. Castellvi AE, Nienke TW, Marulanda GA, Murtagh RD, Santoni BG. Indirect decompression of lumbar stenosis with transpsoas interbody cages and percutaneous posterior instrumentation. Clin Orthop Relat Res. (2014) 472(6):1784–91. doi: 10.1007/s11999-014-3464-6
35. Malham GM, Parker RM, Goss B, Blecher CM, Ballok ZE. Indirect foraminal decompression is independent of metabolically active facet arthropathy in extreme lateral interbody fusion. Spine. (2014) 39(22):E1303–10. doi: 10.1097/brs.0000000000000551
Keywords: oblique lumbar interbody fusion, lumbar foraminal stenosis, indirect decompression, direct decompression, laminotomy, laminectomy
Citation: Tseng S, Lin Y, Wu Y, Shih C, Chen K, Lee C and Pan C (2022) Indirect decompression via oblique lumbar interbody fusion is sufficient for treatment of lumbar foraminal stenosis. Front. Surg. 9:911514. doi: 10.3389/fsurg.2022.911514
Received: 2 April 2022; Accepted: 8 July 2022;
Published: 18 August 2022.
Edited by:
Luca Ricciardi, Sapienza University of Rome, ItalyReviewed by:
Suyash Singh, All India Institute of Medical Sciences, IndiaJavier Quillo-Olvera, The Brain and Spine Care, Minimally Invasive Spine Surgery Group, Mexico
© 2022 Sheng-Chieh, Yu-Hsien, Yun-Che, Cheng-Min, Kun-Hui, Cheng-Hung and Chien-Chou. This is an open-access article distributed under the terms of the Creative Commons Attribution License (CC BY). The use, distribution or reproduction in other forums is permitted, provided the original author(s) and the copyright owner(s) are credited and that the original publication in this journal is cited, in accordance with accepted academic practice. No use, distribution or reproduction is permitted which does not comply with these terms.
*Correspondence: Chien-Chou Pan YWRvbmlzdmdoQGdtYWlsLmNvbQ==
Specialty Section: This article was submitted to Orthopedic Surgery, a section of the journal Frontiers in Surgery
Abbreviations: ADH, anterior disc height; DH, average disc height; DLIF, direct lateral interbody fusion; FH, foraminal height; FS, foraminal stenosis; LL, lumbar lordosis; LLIF, lateral lumbar interbody fusion; Non-PD, non-posterior decompression; ODI, Oswestry disability index; OLIF, oblique lumbar interbody fusion; PD, Posterior decompression; PDH, posterior disc height; PLIF, posterior lumbar interbody fusion; SL, segmental lordosis; TLIF, transforaminal lumbar interbody fusion; VAS-Back, visual analogue scale of back pain; VAS-Leg, visual analogue scale of leg pain; XLIF, extreme lateral interbody fusion