- Department of Infection Biology, University Hospital Erlangen and Friedrich-Alexander University Erlangen-Nuremberg (FAU), Erlangen, Germany
Eosinophils represent a granulocyte cell type that is strongly associated with type 2 inflammatory conditions. During steady state conditions few eosinophils are found in lung tissue, though they may contribute to homeostasis. In allergic airway inflammation, eosinophils are strongly increased and associated to disease severity. The underlying type 2 immune response tightly regulates eosinophil development, recruitment, survival, and heterogeneity. Inflammatory eosinophils in the lung are unfavourable, as they can cause tissue damage, amplify type 2 immunity and induce bronchial obstruction by expelling granular proteins and cytokines. In this review we provide an overview about mechanisms regulating development of eosinophils in the bone marrow and their extravasation into the lung including recent findings on induction and diversity of eosinophilia in allergic airway inflammation.
Introduction
Eosinophils were firstly described in 1879 by Paul Ehrlich, who named them “eosinophils” because they appeared as granulated cells with an affinity for eosin staining (1). The granules of eosinophils contain various potent defensive proteins like major basic protein (MBP, PRG2), eosinophil cationic protein (ECP, RNASE3), eosinophil peroxidase (EPO, EPX), and eosinophil-derived neurotoxin (EDN, RNASE2), which can be toxic to helminths, viruses and bacteria, but can also damage host cells (2). Eosinophils are highly conserved cells across many vertebrate species (3). Human and mouse eosinophils both have been well characterized and they share various similarities but also harbour differences. One example are surface markers, which are frequently used to define eosinophils in flow cytometric or immunohistologic analyses. They share the expression of CCR3 and CD11b (ITGAM, human and mouse), show expression of the functional paralogues SIGLEC-8 (human) and SIGLEC-F (mouse) and express the orthologue proteins EMR1 (ADGRE1, human) and F4/80 (Adgre1, mouse). They differ in the expression of the IgE-receptor FcεRI, which is only present on human eosinophils (4, 5). Murine and human eosinophils are comparable in size and occur in a similar frequency in the blood, however, human eosinophils display greater granularity (6).
In healthy individuals, eosinophils make up less than 5% of leukocytes in peripheral blood and occur in the lung only in low numbers. They are short lived as they survive only about 8–12 h in blood circulation and up to 12 days in some tissues (7). Despite the low frequency of eosinophils in the homeostatic lung, eosinophils were shown to play a role in lung homeostasis. As such, they were described to inhibit the maturation of dendritic cells (DCs) and the type 2 helper T-cell (Th2) response in allergy (8). In eosinophil-associated diseases eosinophil counts in the blood increase, also termed as eosinophilia. Eosinophilia can occur especially during parasitic infections, in allergic diseases and certain autoimmune diseases (9). The incidence of allergic diseases and asthma is increasing worldwide and constitutes a major health concern (10). Depending on the eosinophil counts in blood, allergic asthma is classified either as eosinophilic or non-eosinophilic. The latter is rather characterized by the absence of blood ILC2s and a mixed granulocytic or paucigranulocytic phenotype including neutrophils (11). In eosinophilic asthma, eosinophil counts in the blood of asthma patients correlated with severity of asthma and exacerbation rates (12). Moreover, eosinophilic asthma can be subdivided into atopic (allergic) and non-atopic (non-allergic) asthma. Atopic asthma is classified by the contribution of type 2T-helper cells (Th2) cells, whereas in non-atopic asthma primarily type 2 innate lymphoid cells (ILC2) are responsible for eosinophilia (13). The determination of such asthma endotypes is crucial for selection of the appropriate treatment options. Severe asthma is commonly treated with glucocorticoids, corticosteroids, long-acting beta-adrenergic receptor agonists and leukotriene receptor antagonists. However, as eosinophils contribute to asthma severity and exacerbations, reduction of eosinophil numbers is another treatment option in eosinophilic asthma. One target of treatment is IL-5, which drives eosinophil differentiation and survival. Therefore, IL-5 neutralizing antibodies like Reslizumab and Mepolizumab and the IL-5 receptor antagonist Benralizumab are used to treat eosinophilic asthma and can reduce exacerbations by approximately 50%. As IL-4 and IL-13 cytokines are considered to be crucial for eosinophil recruitment, inhibition of these cytokines or the IL-4 receptor alpha chain is another treatment option to reduce eosinophils, although with rather inconsistent effects on exacerbation rates (14).
As eosinophils are a hallmark of eosinophilic asthma and contribute to severity of asthma, it is of great interest to understand how eosinophils develop in the bone marrow and how recruitment and migration of eosinophils to the lung is regulated.
Eosinophil development in the bone marrow
It is long known, that eosinophils develop in the bone marrow from a CD34+ progenitor population. Further development from this progenitor into eosinophils is dependent on various transcription factors like c/EBPα, c/EBPε, PU.1, IRF8, Gata-1 and Gata-2 (15). Especially Gata-1 is important for eosinophil development, as impaired expression of Gata-1 in the ΔdblGATA mouse strain selectively depletes all eosinophils (16) and constitutive expression of Gata-1 in human progenitors enforces eosinophil fate (17). Drissen et al. (18) propose a new model of haematopoiesis in which a megakaryocyte and an eosinophil-mast cell progenitor (EoMP) population arise separately from the macrophage/neutrophil lineage in a Gata-1-dependent manner. This EoMP gives rise to eosinophils, mast cells, and basophils (18). The lineage commitment between basophils and eosinophils depends on the sequence of transcription factor activation. c/EBPα expression prior to Gata-2 seems to commit progenitors to become eosinophils, the reverse sequence of expression rather induces basophils (19). c/EBPα is essential in eosinophil development as it binds to promoters of eosinophil-determining genes and inhibits friend of Gata (Fog) expression, which at some point inhibits expression of eosinophil-determining genes (20). Recently, Jorssen et al. confirmed the aforementioned Gata-1 dependent lineage fate by using combined scRNA sequencing and transcriptomic analysis (21). Their approach also identified four distinct stages of eosinophil development. One important step in eosinophil development is the upregulation of the chemokine receptor CCR3, which is critical for chemotaxis of eosinophils towards inflamed tissues (22).
IL-5 is a prominent cytokine in eosinophil development and IL-5 overexpressing mice show a massive eosinophilia (23). In line, the IL-5 receptor alpha chain (also named CD125) is expressed by early eosinophil progenitors (21, 23). However, IL-5 is not essential for basal eosinophil development as IL-5 blocking antibodies reduce eosinophil counts in eosinophilic diseases drastically, but not completely (24). Further, IL-5 knock-out (k.o.) mice still harbour a low number of eosinophils in homoeostasis (25). The mechanism of how eosinophils develop in the absence of IL-5 still needs to be investigated. The IL-5, IL-3 and GM-CSF receptors share the common beta chain (βc), which is known to activate various signalling pathways, including the MAPK pathway by a βc-Shc-Grb2 cascade (26). Our group showed that Grb2 plays an important role for eosinophil development (27). Moreover, it was reported that Grb2 binds to the phosphatase PTPN11 (SHP-2) in IL-5 stimulated eosinophils (28). SHP-2, which also can be induced by IL-3, IL-5, and GM-CSF, was described as essential factor for eosinophil bone marrow development and as an inducer of c/EBPα expression (29–31). These studies provide hints on how cytokines might regulate eosinophil development. However, a direct link between receptor signalling and eosinophil-lineage inducing transcription factors is missing.
Eosinophil development was further shown to depend on IL-33. IL-33 is a member of the IL-1 cytokine family and signalling is mediated via the IL-33 receptor ST2 (IL1RL1) (32). IL-33 is mainly produced by epithelial cells and released in case of epithelial damage, which is also occurring in allergic airway inflammation and mainly stimulates ILC2s and Th2 cells. In mice that lack IL-33 or the Il-33 receptor ST2, eosinophils could not develop properly, and eosinophil numbers in blood and periphery were strongly reduced (33). Mature eosinophils were shown to be ST2 positive, but whether eosinophil precursors in the bone marrow express ST2 on the surface is controversial in literature. Whereas Tsuzuki et al. found ST2 expression on eosinophil progenitors (34), other data could not confirm this finding (33). Interestingly, Drissen et al. found Il1rl1 (the gene encoding ST2) expression in Gata1-EGFP positive progenitors, however, they could not confirm ST2 expression on the surface of the respective cells (18). This raises the question whether the effect of IL-33 on eosinophil development is direct or indirect via other cells responsive to IL-33. Our group has performed analysis on mixed bone marrow chimeras, reconstituted with a 50:50 mix of Il1rl1−/− and WT bone marrow. In this setup, Il1rl1−/− and WT eosinophils share the same environment so that alterations in the ratio of Il1rl1−/− and WT eosinophils indicate cell-intrinsic effects of Il1rl1 deficiency. As Il1rl1−/− eosinophils accumulated in the lung with similar numbers as WT eosinophils after allergen challenge, the effect of IL-33 on eosinophil development appears to be non-intrinsic and rather indirect most likely by activation of ILC2s (35).
Despite substantial knowledge about transcription factor regulation in eosinophils development, it is not fully elucidated how extracellular factors induce the shift toward eosinophil commitment.
Chemotactic migration of eosinophils towards the inflamed lung
After eosinophils have matured in the bone marrow, they will enter the blood circulation and stay there for 8–12 h. In case of airway inflammation, chemokines are released, which attract eosinophils to the lung (Figure 1). The major chemokines for eosinophil attraction are eotaxin-1 (CCL11), -2 (CCL24), and -3 (CCL26), which primarily interact with the C-C-chemokine receptor 3 (CCR3). Hence, CCR3-deficient mice show reduced eosinophil infiltration into allergen-challenged lungs, in line with a prominent role of CCR3 for eosinophil attraction to the lung tissue (36). A comparison of ovalbumin (OVA)-induced asthma in eotaxin-deficient mice showed that CCL24 seems to have a greater impact on eosinophil recruitment into the bronchoalveolar lavage (BAL) than CCL11 (37). Besides CCL11 and CCL24, CCL26 was described as a third eotaxin, which can be induced by IL-4 in an endothelial cell line (38). CCL26 could also be detected in asthmatic patients and was even suggested to be more effective in eosinophil recruitment as compared to CCL11 and CCL24 (39, 40). A further parameter for regulation of immune cell migration is protein modification by sialylation, which affects various chemokine-chemokine receptor interactions. Recently it was shown, that sialylation by the sialyltransferase ST3Gal-IV is necessary for binding of CCL11 to CCR3. Therefore, ST3Gal-IV knockout mice show decreased BAL eosinophils upon lung allergen challenge (41). Whether sialylation is also necessary for binding of CCL24 and CCL26 to CCR3 is currently unknown.
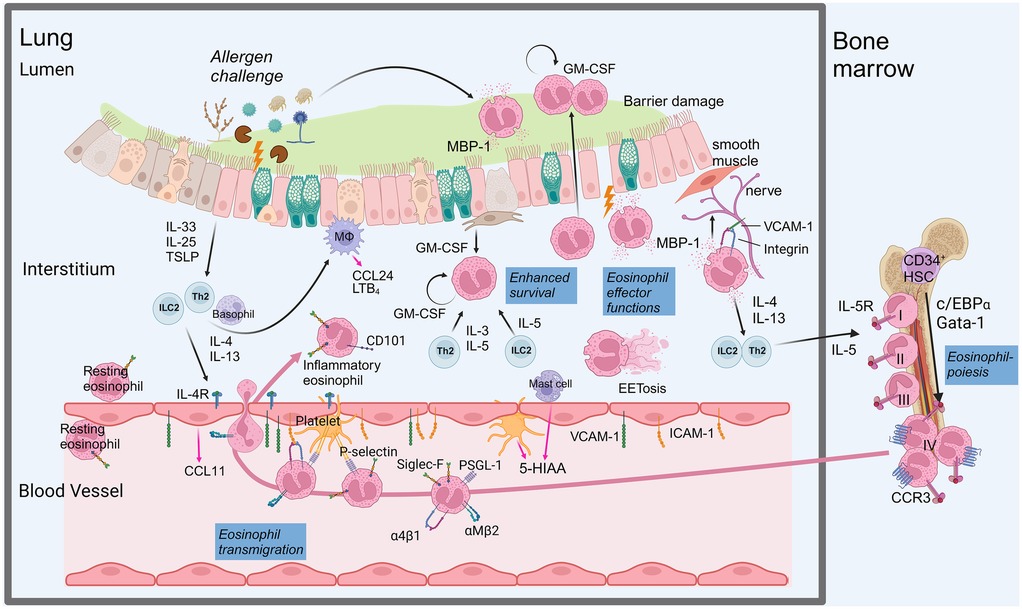
Figure 1. Regulation of allergen-induced lung eosinophilia. Allergens that reach the lung can damage the epithelium, leading to the secretion of alarmins, which then elicit a type 2 immune response. Type 2 cytokines induce eosinophil-chemotactic factors (pink arrows) and prime the endothelium to allow eosinophil transmigration. Thus, inflammatory eosinophils are recruited to the lung, undergo adhesion, rolling, and transmigration through the endothelial layer. In the lung tissue eosinophil survival is enhanced by paracrine and autocrine GM-CSF as well as T cell-derived IL-3 or IL-5 from Th2 cells or ILC2s. Inflammatory eosinophils execute various functions, like damaging the epithelium, activating nerves and inducing further activation of type 2 immune cells. Inflammatory factors, released by lung cells feedback to the bone marrow to increase eosinophil development, mainly driven by IL-5. Eosinophil development in the bone marrow can be divided into four stages and depends on combined action of transcription factors. Created in BioRender. Pollock, J. (2025) https://BioRender.com/m48o078.
Moreover, besides eotaxins, CCR3 can bind CCL5, CCL7, CCL13, and CCL15 (42, 43). Of these, CCL7 was suggested to be relevant for eosinophil recruitment to the lung in type 2 immunity (44, 45). Additionally, CCL5 and CCL13 were suggested to induce eosinophil migration (46, 47). Whether these effects are mediated via CCR3 on eosinophils or other chemokine-receptors still has to be determined.
Because knock-out of CCR3 does not fully blunt eosinophil lung recruitment in mouse studies, it seems that CCR3-independent mechanisms for eosinophil recruitment exist. Indeed, several studies suggested that eosinophils could express other chemokine receptors like CCR1, CCR4, and CXCR4 (48, 49). However, the latter seemed to have no function in eosinophil migration to inflammatory sites (50). CCR1 is important for eosinophils to be responsive to the ligand CCL3 (Macrophage inflammatory protein 1a) (49). Moreover, by using CCL6-deficient mice, it was suggested that in asthmatic mice eosinophil-derived CCL6 can bind to CCR1 and thus induces chemotaxis of eosinophils (51). Another study suggests, that dendritic cell derived CCL17 and CCL22 drives early eosinophil recruitment via eosinophil-expressed CCR4 (52).
Beside chemokines, other factors have been investigated in the course of eosinophil chemotaxis. Prostaglandin D2 induced shape changes, which seem to mobilize eosinophils from the bone marrow and prime them for chemotaxis (53, 54). Interestingly eosinophils migrated towards nematodes in a leukotriene B4 (LTB4)-dependent manner, which is rather known as a chemo-attractive factor for neutrophils (55, 56). Of note, LTB4 was also shown to be increased in asthmatic individuals (57). Therefore, there might be a link between LTB4 and eosinophil chemotaxis in asthma. Moreover, it was shown that mast cells and platelets can be a source for the serotonin metabolite 5-hydroxyindoleacetic acid (5-HIAA), which induces eosinophil-recruitment via the G-protein coupled receptor (GPCR) GPR35 into Cryptococcus neoformans infected lungs (58).
While different factors are long known in eosinophil chemotaxis to the inflamed lung, a comprehensive understanding of their combined action in vivo is necessary to envision future treatment options that are precise and safe.
Transmigration of eosinophils across endothelial cells
After eosinophils got attracted to the lung, they cross the lung endothelial cell layer to enter the lung tissue. Therefore, eosinophils undergo rolling, adhesion and subsequently transmigration through the endothelial layer.
Interaction of P-selectin glycoprotein ligand-1 (PSGL-1) on eosinophils with P-selectin seems to be important for the transmigration process. P-selectin is expressed on endothelial cells, but also on platelets. Interestingly, platelets were reported to be recruited to the endothelium in murine allergic asthma models and platelets are also found in the lung of asthmatic patients (59). Platelet-deficient mice showed reduced eosinophilia in an allergic model and it is proposed that the missing PSGL-1-P-selectin interaction is responsible for this phenotype (60). Other studies suggested that the P-selectin mediated interaction of platelets with eosinophils, upregulates and activates integrin β1 on eosinophils, which might be relevant for transmigration (61, 62). Moreover, as mentioned before platelets were described as source for 5-HIAA, which promoted eosinophil attraction to lung tissue (58). This indicates that platelets in proximity to endothelial cells play a major role for eosinophil transmigration.
Furthermore, attachment to endothelial cells is important in eosinophil transmigration. Whether eosinophils attach also to P-selectin on endothelial cells in vivo is not firmly proven, as administration of anti-P-selectin antibodies blocked P-selectin on both endothelial cells and platelets. However, endothelial cells express additional selective adhesion proteins, like VCAM-1 and ICAM-1, which are important for eosinophil attachment via integrin heterodimer interaction. Eosinophils express the integrin heterodimers α4β1 (VLA-1) and α4β7, which can interact with VCAM-1 as well as the integrin dimer αMβ2 which is able to interact with ICAM-1 (63). Blocking of different integrins expressed on eosinophils, suggested a prominent role for integrin α4 for eosinophil adhesion (64). Integrin α4β7 also interacts with MAdCAM-1 (65), but there is no evidence whether this is necessary for eosinophil transmigration to lung tissue. In vitro data suggests that eosinophils role more efficiently on VCAM-1- as compared to MAdCAM-1-expressing endothelial cells (66). Noteworthy, airway eosinophils from allergen-challenged patients displayed increased integrin αMβ2 expression and enhanced attachment to VCAM-1 and ICAM-1 (67).
VCAM-1 expression on endothelial cells is mainly induced by TNF-α and IL-1β. However, the type 2 immune cytokines, IL-4 and IL-13 were also shown to affect VCAM-1 expression on endothelial cells. One study suggested that IL-4 induced STAT6 can bind to the Vcam-1 promotor (68). In the skin, basophil-derived IL-4 could upregulate VCAM-1 on endothelial cells, which was necessary for eosinophil transmigration (69). Similar results were observed in house dust mite (HDM)-challenged mice, were basophil-deficient animals did not show increased levels of VCAM-1 (70). These studies indicate that VCAM-1 expression is enhanced by type 2 cytokines and facilitates eosinophil recruitment to the lung.
After attachment to adhesion molecules, eosinophils must actively migrate through the endothelial cell layer. A recent study showed that IL-13 downregulates miR-1 in endothelial cells, thereby activating various eosinophil trafficking genes (71). One of them is thrombopoietin (Mgl), which seems to alter P-selectin on platelets and might support eosinophil transmigration as mentioned before (72). The serotonin precursor 5-HTP was found to inhibit eosinophil transmigration via binding to the respective receptor in endothelial cells in vitro. Nucleotide polymorphisms in the gene encoding this receptor were found to be associated with asthma (73, 74). However, the mechanism how this receptor-activation inhibits eosinophil transmigration was not investigated so far.
Besides some basic understanding of how eosinophils attach to endothelial cells, their trans-endothelial migration is hardly studied. In contrast, the transmigration process of neutrophils is better understood and the integrin heterodimer αMβ2 appears to play a crucial role in this process (75). Eosinophils also express this dimer and a similar function in eosinophils is further suggested by β2-deficient mice that show reduced eosinophil migration to lung tissue (76). Additionally, it was suggested that this integrin mediates migration of eosinophils via binding to periostin, an extracellular matrix protein expressed by epithelial and endothelial cells, and secreted by IL-4- and IL-13-stimulated fibroblasts (77–79).
Overall, these studies indicate that rolling, adhesion and actual transmigration of eosinophils in the lung is tightly regulated and enhanced by type 2 immune responses.
Effects of type 2 immune cells on pulmonary eosinophilia
The type 2 immune cytokines IL-4 and IL-13 are essential for eosinophil recruitment to the allergic lung, as depletion of both cytokines abolishes eosinophilia (80). IL-4 and IL-13 are closely related and share various functions, however, also differences are observed. Whereas IL-4 can bind to the IL-4 receptor type I (IL-4RI), both cytokines can activate the IL-4RII which consists of an IL-4Rα and IL-13Rα1 subunit. Binding of IL-4/IL-13 to these receptors leads to activation of STAT6, which can induce a great variety of STAT6-dependent genes (81). In humans, STAT6 is associated with asthma and other allergic diseases, as patients with a gain of function mutation in Stat6 showed a variety of allergic diseases, including asthma (82). In contrast, loss-of-function variants of STAT6 rather protect individuals from allergic asthma (83).
Th2 cells are main producers of IL-4 and IL-13 and were thought to be the crucial driver of eosinophilia. However, in Rag1-deficient mice which lack T and B cells or Th2-deficient mice, eosinophilia is only abrogated in some asthma models. More precisely, in Rag1-deficient mice eosinophilia is absent in models of HDM-induced asthma, allergic bronchopulmonary aspergillosis or OVA-induced asthma (84–87). In a more mechanistic approach, using mice that only lack IL-4/IL-13 in T cells, our group showed that cytokines of Th2 cells are responsible for induction of eosinophilia in OVA-induced asthma as well as in an ABPA mouse model (80, 88). Therefore, HDM-induced asthma, allergic bronchopulmonary aspergillosis or OVA-induced asthma represent Th2-dependent asthma subtypes [reviewed in (89)]. Besides Th2 cells, ILC2s are a major source of IL-13, but also produce IL-4 to a minor extent. Interestingly, mice which lack ILC2s showed reduced eosinophilia in an HDM model, despite the fact that Th2 cells are induced normally (90). Therefore, ILC2s might also contribute to eosinophilia in OVA-induced asthma or ABPA, which was not investigated. In contrast to the Th2-dependent asthma models, the models using papain extract or Alternaria-induced asthma still elicit eosinophilia in Rag1-deficient mice (91, 92). However, when using ILC2-deficient mice, eosinophilia is abrogated in both asthma models, indicating a major role for ILC2s in eosinophil recruitment (90). In line, in human asthma ILC2s and a strong Th2 signature are associated with eosinophilia (93, 94).
Lung epithelial cells and fibroblast express the IL-4RI and can be induced by IL-4/IL-13 signalling. Indeed, chimeric mouse experiments demonstrated that STAT6 in non-hematopoietic cells is necessary for eotaxin induction and eosinophil recruitment (95). Recently, it was demonstrated that mainly IL-13 induces CCL11 and CCL24 in fibroblasts and monocytes, which was required for eosinophil attraction in HDM-induced murine asthma (96). In addition, murine and human endothelial cells respond to IL-4 and IL-13, which can induce CCL26 (38, 71). Beside secretion of eotoxins, epithelial cells also release IL-33 which has been shown to induce NF-κB signalling in eosinophils, thus activate them (97).
Besides the response of structural lung cells to IL-4/IL-13, macrophages are also responsive to type 2 cytokines. Especially alveolar macrophages (AMs) reside in lung tissue during homeostasis. Chlodronate-based depletion of AMs, led to increased eosinophils in lung tissue and indicates a dampening role of AMs against eosinophilia in steady state (98). However, during allergic lung inflammation macrophages get polarised into M2 macrophages, dependent on STAT6 signalling. Inhibition of this conversion reduced eosinophilia in a mouse model of allergic lung inflammation (99). Despite, stimulation of macrophages with IL-4 also directly induces CCL24 expression (100), no direct effect of macrophages on eosinophil recruitment during allergic lung inflammation could be demonstrated so far.
Basophils are another relevant source of IL-4/IL-13. Whether they contribute to eosinophilia in allergic airway inflammation is controversial in the literature. Our group has established a basophil-deficient mouse (Mcpt8Cre) line, in which OVA still induced eosinophilia in the lung (101). Other groups depleted basophils via treatment with either, anti-FcεR1α or anti-CD200R3 antibodies and observed reduced eosinophilia in murine OVA or HDM asthma models (102, 103). Recently, basophils were shown to regulate the entry of Th2 cells into the lung during HDM-induced asthma, thus eosinophilia was reduced in Mcpt8Cre mice (70). Another study, which used the papain model showed that basophils could induce cytokine production in ILC2s and thereby promoted lung eosinophilia (104). Whether basophil-derived cytokines have direct impact on eotaxin production has not been reported, but it seems that basophils can indirectly induce eosinophilia via Th2 cells and ILC2s.
It is known that various type 2 immune cells have impact on eosinophilia, however, the complex mechanism of the cascade how type 2 cytokines regulate eosinophils could enhance our understanding of asthma pathology.
Eosinophil heterogeneity and survival in inflamed lung tissue
The function of eosinophils in the lung differs between steady state and inflammatory conditions and the phenotype of murine resident (Siglec-Flo/int, CD101lo) and inflammatory eosinophils (Siglec-Fhi, CD101hi) is well defined. The resident eosinophils are established in the lung early in live, around postnatal days 3–14 (105). They show an immune regulatory and homeostatic phenotype and seem to inhibit Th2 cells (8, 106). Therefore, they are considered to play a crucial role in lung homeostasis [reviewed in (107, 108)]. Inflammatory eosinophils display higher integrin expression, which indicates an infiltrating phenotype (106, 109, 110). Notably, these eosinophils are increased in OVA- or HDM-induced asthma models and asthmatic patients correspondingly display an elevated CD101+ eosinophil level (111, 112). Furthermore, CD101 positive and negative eosinophils differ in their airway localisation after Nippostrongylus brasiliensis infection and in an HDM asthma model. CD101− eosinophils were located rather in the vasculature, whereas CD101+ ones were primarily found in the extravascular lung space (111, 113). In vitro, IL-4 stimulation of mouse eosinophils strongly upregulates the expression of Cd101 (114), suggesting a role in eosinophil activation. Siglec-F upregulation on inflammatory eosinophils might also directly modulate their behaviour as signalling via Siglec F has been associated with apoptosis, but also with context-dependent enhancement of cytokine and chemokine secretion in vitro (35, 115, 116). Both characteristics are also described for Siglec-8, the human paralog of Siglec-F, but in vivo confirmation beyond apoptosis induction is missing (35, 117). Interestingly, it was shown that the eosinophils, which migrated through an endothelial cell layer upregulated CD69 and CD35 expression (118). This indicates that infiltrating eosinophils in allergic lung inflammation not only have a distinct localisation in the lung but also display a different phenotype compared to resting eosinophils.
In steady state, there are almost no eosinophils located in the BAL fluid. However, in allergic lung inflammation, inflammatory eosinophils, but not resting eosinophils, accumulate in the BAL fluid (106). Flow cytometric analysis of eosinophils, indicated that murine BAL fluid eosinophils and inflammatory lung tissue eosinophils slightly differ in their integrin expression, whereas they show similar CD101 levels (119). Noteworthy, a similar effect on activated integrin status was also observed for human BAL eosinophils (120). Recently, it was shown that IL-33 activated ILC2s secrete lipid droplets which could recruit neutrophils, but not eosinophils, to the BAL fluid in a CXCR2-dependent manner (121). Whether eosinophils are recruited actively to the BAL fluid via a similar mechanism has not been investigated. However, loss of epithelial barrier integrity, which is a key feature of asthma, could also lead to a rather passive accumulation of eosinophils in the BAL fluid. Asthma-inducing allergens often comprise proteases, which are able to digest junction proteins important for epithelial integrity (122). Similarly, IL-13 was shown to downregulate tight junction proteins, thus also damage barrier function (123, 124). If these effects on barrier integrity are important for eosinophil accumulation in the BAL fluid has not been extensively studied.
In contrast to resting eosinophils, some inflammatory eosinophils also showed expression of Trem-1, which correlated with an enhanced apoptosis-related gene signature (119). Understanding eosinophil survival during allergic lung inflammation is important, as reduced apoptosis of eosinophils correlates with asthma severity in humans (125). Interestingly, enhanced adhesion to fibroblasts correlated with the survival of eosinophils (126). Thus, localization in lung tissue might be beneficial for their survival. However, other mechanisms have been identified which enhance eosinophil survival, especially, the factors GM-CSF, IL-3 and IL-5 (127). Our group provided evidence that these factors inhibit eosinophil apoptosis by NF-κB mediated upregulation of Bcl-xL (128). Epithelial cells and fibroblast can be a source of GM-CSF, which reduces the eosinophil apoptosis rate ex vivo (129, 130). Interestingly, IL-33 can induce GM-CSF release from eosinophils, which prevented apoptosis in an autocrine manner (116). Furthermore, a recent study investigated the effect of Th2-derived extracellular vesicles and found a beneficial role of these EVs for eosinophil survival in an IL-3 dependent manner (131).
Further research in the field of tension between effector function, survival and resolution is mandatory to unravel the relevance of observed eosinophil heterogeneity.
Contribution of eosinophils to allergic lung inflammation
Whereas lung resident eosinophils seem to supress type 2 driven immunity via dendritic cells (8), inflammatory eosinophils have a more pro-inflammatory phenotype. In patients, high eosinophilia correlates with asthma severity and depletion of eosinophils has a beneficial effect in some settings, thus it is of interest to understand the function of eosinophils in allergic lung inflammation.
Gleich and colleagues discussed in their review the biological consequences of eosinophil deficiency (132). They pointed out the fact that patients lacking eosinophils, still can develop severe asthma. However, it has been shown that the number of eosinophils correlates with asthma severity and anti-IL-5 or anti-IL-5 receptor treatment showed beneficial effects in asthma patients (12, 133, 134). Thus, it seems that eosinophils have an unfavourable role during allergic lung inflammation. Eosinophils are able to produce various granular proteins and cytokines. Especially the granule protein major basic protein 1 (MBP-1) was shown to induce damage in bronchial epithelial cells (135). Recently, two publications provided mechanistic insights on how MBP-1 affects lung epithelial cells. On the one hand, MBP-1 is suggested to induce ferroptosis in the epithelium via activation of the mTORC1 signalling pathway (136). On the other hand, MBP-1 was shown to induce formation of small pores in lipid bilayers, indicating that MBP-1 might damage the membrane of human bronchial epithelial cells (137).
Besides affecting epithelial cells, eosinophil granular proteins can also modulate nerves, which is critical for bronchoconstriction. Recently, a close proximity between eosinophils and nerve bundles was observed to be increased in lungs from patients with fatal asthma (138). It was shown that activated eosinophils can bind to VCAM-1 and ICAM-1 expressed on nerves, which additionally induced their degranulation (139). Thereby, eosinophils release MBP-1, which is an antagonist of the muscarinic M2 receptor. Inhibition of this receptor leads to increased acetylcholine release by parasympathic nerves, which induces smooth muscle contraction [reviewed in (140)]. Moreover, in mice and asthmatic humans, eosinophils correlated with increased sensory nerve density, which elevated airway hyperresponsiveness (141). These studies indicate a role for eosinophil-nerve interaction leading to bronchial obstruction in allergic asthma.
Another interesting process that was described in asthma is the release of DNA-containing eosinophil extracellular traps (EET). The ability of eosinophils to form EETs was higher in patients with severe asthma compared to non-severe asthma. The EETs derived from asthmatic patient's eosinophils, induced disconnection of epithelial cells and pro-inflammatory cytokine release by epithelial cells in vitro (142). Similar results could be observed in mouse experiments, where intranasal treatment of mice with EETs induced cytokine upregulation in epithelial cells. Additionally, ILC2 activation and eosinophil recruitment were induced in these mice (143). These studies indicate that EETs might contribute to the pathological role of eosinophils in asthma.
Eosinophils also produce the cytokines IL-4 and IL-13 (144–146). Experiments with eosinophil-deficient mice demonstrated that eosinophils are required for T cell recruitment into OVA-challenged lungs and high Th2 cytokine level (147). We observed similar effects in a mouse ABPA model, where eosinophils were required for a strong Th2 response, type-2 cytokine production and IgE induction (88). Besides the effect of eosinophils on Th2 cells, a recent report also describes an effect on ILC2s. Eosinophil-deficient (iPHIL) mice and mice lacking IL-4/IL-13 producing eosinophils displayed a reduced ILC2 response in HDM- and OVA-induced asthma models. Moreover, effects of eosinophils on ILC2 proliferation, recruitment and activation in an in vitro setting were proposed (148). These data suggest a positive feedback-loop, in which eosinophils induce Th2 cells and ILC2s, which are in turn important for eosinophil recruitment.
In contrast to the effects observed in acute inflammation, eosinophils are also discussed to contribute to resolution of inflammation. Indeed, eosinophil-deficient PHIL mice showed reduced resolution of airway inflammation, because of reduction in IL-10 producing eosinophils (149). Resolution of inflammation requires efficient removal of apoptotic cells, which would otherwise trigger further inflammation and is a mainly macrophage-dependent process. In lymph nodes, eosinophils were shown to induce CXCL13 expressing macrophages which contributed to the resolution of inflammation (150). Moreover, eosinophils themselves have been reported to take up apoptotic cells which then induced an anti-inflammatory gene expression signature in eosinophils, related to wound healing responses (151).
According to these recent studies on eosinophil functions, it becomes more evident that eosinophils indeed play a major role during allergic airway inflammation.
Conclusion
Impaired lung function can drastically impair the quality of life and increased numbers of eosinophils in the lung correlate with severity and exacerbations in asthma. Despite the availability of modern drugs that efficiently reduce eosinophilia, our understanding of eosinophil development, tissue recruitment, effector functions and survival remains incomplete and requires further investigations.
Eosinophilia in allergic lung inflammation is tightly regulated. De novo production of eosinophils in the bone marrow is increased via IL-5 release from Th2 cells and ILC2s. As published data indicates that eosinophilia is not completely abolished in IL-5 ko mice, further investigation on how eosinophil development is promoted by type 2 immunity is needed. From the bone marrow, eosinophils are recruited into the lung mainly in a chemotactic manner. The newly recruited eosinophils appear to have an inflammatory phenotype. Knowledge about the eosinophil transmigration process into the lung is limited and requires further investigation. In addition, it remains unclear how positioning within the tissue environment influences the effector functions of inflammatory and resting eosinophils. To further study the complex biology of different eosinophil subtypes, single-cell sequencing was used to describe distinct eosinophil subtypes in the intestine (152). This approach has not been used to study lung eosinophils so far, but could give insights into various lung eosinophil subtypes. Another study failed to gain single cell RNA-sequencing data from bone marrow eosinophils, most probably because eosinophils express large quantities of RNAses (21). Therefore, proteomic approaches might be better suited to study differences between eosinophil subtypes.
Despite the fact that many studies examined eosinophilia in allergic lung inflammation, the greater context still lacks functional understanding. Moreover, distinct eosinophil subtypes may execute specific functions in the context of type 2 immune responses. A better understanding of eosinophil subset features and functional importance is crucial to develop new therapeutic approaches to interfere with eosinophil associated pathology.
Author contributions
L-MG: Conceptualization, Validation, Writing – original draft, Writing – review & editing, Visualization. DR: Conceptualization, Validation, Visualization, Writing – original draft, Writing – review & editing. DV: Conceptualization, Validation, Writing – original draft, Writing – review & editing, Funding acquisition, Project administration, Supervision.
Funding
The author(s) declare that financial support was received for the research and/or publication of this article. This work was supported by the Deutsche Forschungsgemeinschaft with grants GRK2740 “Immunomicrotope” (447268119) and FOR2599_P4 to DV, and RA 3732/1-1 to DR.
Acknowledgments
We thank Jonathan Pollock for help with creating the figure and all members of the Voehringer lab for their insightful comments.
Conflict of interest
The authors declare that the research was conducted in the absence of any commercial or financial relationships that could be construed as a potential conflict of interest.
Generative AI statement
The author(s) declare that no Generative AI was used in the creation of this manuscript.
Publisher's note
All claims expressed in this article are solely those of the authors and do not necessarily represent those of their affiliated organizations, or those of the publisher, the editors and the reviewers. Any product that may be evaluated in this article, or claim that may be made by its manufacturer, is not guaranteed or endorsed by the publisher.
References
1. Kay AB. The early history of the eosinophil. Clin Exp Allergy. (2015) 45(3):575–82. doi: 10.1111/cea.12480
2. Acharya KR, Ackerman SJ. Eosinophil granule proteins: form and function. J Biol Chem. (2014) 289(25):17406–15. doi: 10.1074/jbc.R113.546218
3. Stacy NI, Ackerman SJ. A tribute to eosinophils from a comparative and evolutionary perspective. J Allergy Clin Immunol. (2021) 147(3):1115–6. doi: 10.1016/j.jaci.2020.12.002
4. de Andres B, Rakasz E, Hagen M, McCormik ML, Mueller AL, Elliot D, et al. Lack of fc-epsilon receptors on murine eosinophils: implications for the functional significance of elevated IgE and eosinophils in parasitic infections. Blood. (1997) 89(10):3826–36. doi: 10.1182/blood.V89.10.3826
5. Gounni AS, Lamkhioued B, Ochiai K, Tanaka Y, Delaporte E, Capron A, et al. High-affinity IgE receptor on eosinophils is involved in defence against parasites. Nature. (1994) 367(6459):183–6. doi: 10.1038/367183a0
6. Lee JJ, Jacobsen EA, Ochkur SI, McGarry MP, Condjella RM, Doyle AD, et al. Human versus mouse eosinophils: “that which we call an eosinophil, by any other name would stain as red”. J Allergy Clin Immunol. (2012) 130(3):572–84. doi: 10.1016/j.jaci.2012.07.025
7. Aoki A, Hirahara K, Kiuchi M, Nakayama T. Eosinophils: cells known for over 140 years with broad and new functions. Allergol Int. (2021) 70(1):3–8. doi: 10.1016/j.alit.2020.09.002
8. Jacobsen EA, Zellner KR, Colbert D, Lee NA, Lee JJ. Eosinophils regulate dendritic cells and Th2 pulmonary immune responses following allergen provocation. J Immunol. (2011) 187(11):6059–68. doi: 10.4049/jimmunol.1102299
9. Leru PM. Eosinophilic disorders: evaluation of current classification and diagnostic criteria, proposal of a practical diagnostic algorithm. Clin Transl Allergy. (2019) 9:36. doi: 10.1186/s13601-019-0277-4
10. Pawankar R. Allergic diseases and asthma: a global public health concern and a call to action. World Allergy Organ J. (2014) 7(1):12. doi: 10.1186/1939-4551-7-12
11. Carr TF, Zeki AA, Kraft M. Eosinophilic and noneosinophilic asthma. Am J Respir Crit Care Med. (2018) 197(1):22–37. doi: 10.1164/rccm.201611-2232PP
12. Hussain M, Liu G. Eosinophilic asthma: pathophysiology and therapeutic horizons. Cells. (2024) 13(5):384. doi: 10.3390/cells13050384
13. Lambrecht BN, Hammad H. The immunology of asthma. Nat Immunol. (2015) 16(1):45–56. doi: 10.1038/ni.3049
14. Cusack RP, Whetstone CE, Xie Y, Ranjbar M, Gauvreau GM. Regulation of eosinophilia in asthma-new therapeutic approaches for asthma treatment. Cells. (2021) 10(4):817. doi: 10.3390/cells10040817
15. Mack EA, Pear WS. Transcription factor and cytokine regulation of eosinophil lineage commitment. Curr Opin Hematol. (2020) 27(1):27–33. doi: 10.1097/MOH.0000000000000552
16. Yu C, Cantor AB, Yang H, Browne C, Wells RA, Fujiwara Y, et al. Targeted deletion of a high-affinity GATA-binding site in the GATA-1 promoter leads to selective loss of the eosinophil lineage in vivo. J Exp Med. (2002) 195(11):1387–95. doi: 10.1084/jem.20020656
17. Hirasawa R, Shimizu R, Takahashi S, Osawa M, Takayanagi S, Kato Y, et al. Essential and instructive roles of GATA factors in eosinophil development. J Exp Med. (2002) 195(11):1379–86. doi: 10.1084/jem.20020170
18. Drissen R, Buza-Vidas N, Woll P, Thongjuea S, Gambardella A, Giustacchini A, et al. Distinct myeloid progenitor-differentiation pathways identified through single-cell RNA sequencing. Nat Immunol. (2016) 17(6):666–76. doi: 10.1038/ni.3412
19. Iwasaki H, Mizuno S, Arinobu Y, Ozawa H, Mori Y, Shigematsu H, et al. The order of expression of transcription factors directs hierarchical specification of hematopoietic lineages. Genes Dev. (2006) 20(21):3010–21. doi: 10.1101/gad.1493506
20. Querfurth E, Schuster M, Kulessa H, Crispino JD, Doderlein G, Orkin SH, et al. Antagonism between C/EBPbeta and FOG in eosinophil lineage commitment of multipotent hematopoietic progenitors. Genes Dev. (2000) 14(19):2515–25. doi: 10.1101/gad.177200
21. Jorssen J, Van Hulst G, Mollers K, Pujol J, Petrellis G, Baptista AP, et al. Single-cell proteomics and transcriptomics capture eosinophil development and identify the role of IL-5 in their lineage transit amplification. Immunity. (2024) 57(7):1549–66.e8. doi: 10.1016/j.immuni.2024.04.027
22. Ma W, Bryce PJ, Humbles AA, Laouini D, Yalcindag A, Alenius H, et al. CCR3 is essential for skin eosinophilia and airway hyperresponsiveness in a murine model of allergic skin inflammation. J Clin Invest. (2002) 109(5):621–8. doi: 10.1172/JCI14097
23. Lee NA, McGarry MP, Larson KA, Horton MA, Kristensen AB, Lee JJ. Expression of IL-5 in thymocytes/T cells leads to the development of a massive eosinophilia, extramedullary eosinophilopoiesis, and unique histopathologies. J Immunol. (1997) 158(3):1332–44. doi: 10.4049/jimmunol.158.3.1332
24. Buttner C, Lun A, Splettstoesser T, Kunkel G, Renz H. Monoclonal anti-interleukin-5 treatment suppresses eosinophil but not T-cell functions. Eur Respir J. (2003) 21(5):799–803. doi: 10.1183/09031936.03.00027302
25. Kopf M, Brombacher F, Hodgkin PD, Ramsay AJ, Milbourne EA, Dai WJ, et al. IL-5-deficient mice have a developmental defect in CD5+ B-1 cells and lack eosinophilia but have normal antibody and cytotoxic T cell responses. Immunity. (1996) 4(1):15–24. doi: 10.1016/s1074-7613(00)80294-0
26. Martinez-Moczygemba M, Huston DP. Biology of common beta receptor-signaling cytokines: IL-3, IL-5, and GM-CSF. J Allergy Clin Immunol. (2003) 112(4):653–65; quiz 666. doi: 10.1016/j.jaci.2003.08.015
27. Willebrand R, Dietschmann A, Nitschke L, Krappmann S, Voehringer D. Murine eosinophil development and allergic lung eosinophilia are largely dependent on the signaling adaptor GRB2. Eur J Immunol. (2018) 48(11):1786–95. doi: 10.1002/eji.201847555
28. Pazdrak K, Adachi T, Alam R. Src homology 2 protein tyrosine phosphatase (SHPTP2)/src homology 2 phosphatase 2 (SHP2) tyrosine phosphatase is a positive regulator of the interleukin 5 receptor signal transduction pathways leading to the prolongation of eosinophil survival. J Exp Med. (1997) 186(4):561–8. doi: 10.1084/jem.186.4.561
29. Qu CK. Role of the SHP-2 tyrosine phosphatase in cytokine-induced signaling and cellular response. Biochim Biophys Acta. (2002) 1592(3):297–301. doi: 10.1016/s0167-4889(02)00322-1
30. Zhang L, Friedman AD. SHP2 tyrosine phosphatase stimulates CEBPA gene expression to mediate cytokine-dependent granulopoiesis. Blood. (2011) 118(8):2266–74. doi: 10.1182/blood-2011-01-331157
31. Xia LX, Hua W, Jin Y, Tian BP, Qiu ZW, Zhang C, et al. Eosinophil differentiation in the bone marrow is promoted by protein tyrosine phosphatase SHP2. Cell Death Dis. (2016) 7(4):e2175. doi: 10.1038/cddis.2016.74
32. Schmitz J, Owyang A, Oldham E, Song Y, Murphy E, McClanahan TK, et al. IL-33, an interleukin-1-like cytokine that signals via the IL-1 receptor-related protein ST2 and induces T helper type 2-associated cytokines. Immunity. (2005) 23(5):479–90. doi: 10.1016/j.immuni.2005.09.015
33. Johnston LK, Hsu CL, Krier-Burris RA, Chhiba KD, Chien KB, McKenzie A, et al. IL-33 precedes IL-5 in regulating eosinophil commitment and is required for eosinophil homeostasis. J Immunol. (2016) 197(9):3445–53. doi: 10.4049/jimmunol.1600611
34. Tsuzuki H, Arinobu Y, Miyawaki K, Takaki A, Ota SI, Ota Y, et al. Functional interleukin-33 receptors are expressed in early progenitor stages of allergy-related granulocytes. Immunology. (2017) 150(1):64–73. doi: 10.1111/imm.12667
35. Westermann S, Dietschmann A, Doehler D, Castiglione K, Bochner BS, Voehringer D, et al. Siglec-F promotes IL-33-induced cytokine release from bone marrow-derived eosinophils independently of the ITIM and ITIM-like motif phosphorylation. J Immunol. (2022) 208(3):732–44. doi: 10.4049/jimmunol.2100184
36. Humbles AA, Lu B, Friend DS, Okinaga S, Lora J, Al-Garawi A, et al. The murine CCR3 receptor regulates both the role of eosinophils and mast cells in allergen-induced airway inflammation and hyperresponsiveness. Proc Natl Acad Sci U S A. (2002) 99(3):1479–84. doi: 10.1073/pnas.261462598
37. Pope SM, Zimmermann N, Stringer KF, Karow ML, Rothenberg ME. The eotaxin chemokines and CCR3 are fundamental regulators of allergen-induced pulmonary eosinophilia. J Immunol. (2005) 175(8):5341–50. doi: 10.4049/jimmunol.175.8.5341
38. Shinkai A, Yoshisue H, Koike M, Shoji E, Nakagawa S, Saito A, et al. A novel human CC chemokine, eotaxin-3, which is expressed in IL-4-stimulated vascular endothelial cells, exhibits potent activity toward eosinophils. J Immunol. (1999) 163(3):1602–10. doi: 10.4049/jimmunol.163.3.1602
39. Larose MC, Chakir J, Archambault AS, Joubert P, Provost V, Laviolette M, et al. Correlation between CCL26 production by human bronchial epithelial cells and airway eosinophils: involvement in patients with severe eosinophilic asthma. J Allergy Clin Immunol. (2015) 136(4):904–13. doi: 10.1016/j.jaci.2015.02.039
40. Provost V, Larose MC, Langlois A, Rola-Pleszczynski M, Flamand N, Laviolette M. CCL26/eotaxin-3 is more effective to induce the migration of eosinophils of asthmatics than CCL11/eotaxin-1 and CCL24/eotaxin-2. J Leukoc Biol. (2013) 94(2):213–22. doi: 10.1189/jlb.0212074
41. Immler R, Nussbaumer K, Doerner A, El Bounkari O, Huber S, Abisch J, et al. CCR3-dependent Eosinophil recruitment is regulated by sialyltransferase ST3Gal-IV. Proc Natl Acad Sci U S A. (2024) 121(19):e2319057121. doi: 10.1073/pnas.2319057121
42. Fathollahpour A, Abyaneh FA, Darabi B, Ebrahimi M, Kooti W, Kalmarzi RN. Aspirin-exacerbated respiratory disease polymorphisms; a review study. Gene. (2023) 870:147326. doi: 10.1016/j.gene.2023.147326
43. Shimizu Y, Dobashi K. CC-chemokine CCL15 expression and possible implications for the pathogenesis of IgE-related severe asthma. Mediators Inflamm. (2012) 2012:475253. doi: 10.1155/2012/475253
44. Shang XZ, Chiu BC, Stolberg V, Lukacs NW, Kunkel SL, Murphy HS, et al. Eosinophil recruitment in type-2 hypersensitivity pulmonary granulomas: source and contribution of monocyte chemotactic protein-3 (CCL7). Am J Pathol. (2002) 161(1):257–66. doi: 10.1016/S0002-9440(10)64177-6
45. Zhang YL, Han DH, Kim DY, Lee CH, Rhee CS. Role of interleukin-17A on the chemotactic responses to CCL7 in a murine allergic rhinitis model. PLoS One. (2017) 12(1):e0169353. doi: 10.1371/journal.pone.0169353
46. Elsner J, Petering H, Hochstetter R, Kimmig D, Wells TN, Kapp A, et al. The CC chemokine antagonist met-RANTES inhibits eosinophil effector functions through the chemokine receptors CCR1 and CCR3. Eur J Immunol. (1997) 27(11):2892–8. doi: 10.1002/eji.1830271122
47. Garcia-Zepeda EA, Combadiere C, Rothenberg ME, Sarafi MN, Lavigne F, Hamid Q, et al. Human monocyte chemoattractant protein (MCP)-4 is a novel CC chemokine with activities on monocytes, eosinophils, and basophils induced in allergic and nonallergic inflammation that signals through the CC chemokine receptors (CCR)-2 and -3. J Immunol. (1996) 157(12):5613–26. doi: 10.4049/jimmunol.157.12.5613
48. Liu LY, Jarjour NN, Busse WW, Kelly EA. Chemokine receptor expression on human eosinophils from peripheral blood and bronchoalveolar lavage fluid after segmental antigen challenge. J Allergy Clin Immunol. (2003) 112(3):556–62. doi: 10.1016/s0091-6749(03)01798-6
49. Sabroe I, Hartnell A, Jopling LA, Bel S, Ponath PD, Pease JE, et al. Differential regulation of eosinophil chemokine signaling via CCR3 and non-CCR3 pathways. J Immunol. (1999) 162(5):2946–55. doi: 10.4049/jimmunol.162.5.2946
50. Nagase H, Miyamasu M, Yamaguchi M, Fujisawa T, Ohta K, Yamamoto K, et al. Expression of CXCR4 in eosinophils: functional analyses and cytokine-mediated regulation. J Immunol. (2000) 164(11):5935–43. doi: 10.4049/jimmunol.164.11.5935
51. Du X, Li F, Zhang C, Li N, Huang H, Shao Z, et al. Eosinophil-derived chemokine (hCCL15/23, mCCL6) interacts with CCR1 to promote eosinophilic airway inflammation. Signal Transduct Target Ther. (2021) 6(1):91. doi: 10.1038/s41392-021-00482-x
52. Yi S, Zhai J, Niu R, Zhu G, Wang M, Liu J, et al. Eosinophil recruitment is dynamically regulated by interplay among lung dendritic cell subsets after allergen challenge. Nat Commun. (2018) 9(1):3879. doi: 10.1038/s41467-018-06316-9
53. Carstensen S, Gress C, Erpenbeck VJ, Kazani SD, Hohlfeld JM, Sandham DA, et al. Prostaglandin D(2) metabolites activate asthmatic patient-derived type 2 innate lymphoid cells and eosinophils via the DP(2) receptor. Respir Res. (2021) 22(1):262. doi: 10.1186/s12931-021-01852-3
54. Heinemann A, Schuligoi R, Sabroe I, Hartnell A, Peskar BA. Delta 12-prostaglandin J2, a plasma metabolite of prostaglandin D2, causes eosinophil mobilization from the bone marrow and primes eosinophils for chemotaxis. J Immunol. (2003) 170(9):4752–8. doi: 10.4049/jimmunol.170.9.4752
55. Patnode ML, Bando JK, Krummel MF, Locksley RM, Rosen SD. Leukotriene B4 amplifies eosinophil accumulation in response to nematodes. J Exp Med. (2014) 211(7):1281–8. doi: 10.1084/jem.20132336
56. Van Bruggen S, Jarrot PA, Thomas E, Sheehy CE, Silva CMS, Hsu AY, et al. NLRP3 is essential for neutrophil polarization and chemotaxis in response to leukotriene B4 gradient. Proc Natl Acad Sci U S A. (2023) 120(35):e2303814120. doi: 10.1073/pnas.2303814120
57. Higham A, Cadden P, Southworth T, Rossall M, Kolsum U, Lea S, et al. Leukotriene B4 levels in sputum from asthma patients. ERJ Open Res. (2016) 2(4):00088-2015. doi: 10.1183/23120541.00088-2015
58. De Giovanni M, Dang EV, Chen KY, An J, Madhani HD, Cyster JG. Platelets and mast cells promote pathogenic eosinophil recruitment during invasive fungal infection via the 5-HIAA-GPR35 ligand-receptor system. Immunity. (2023) 56(7):1548–60.e5. doi: 10.1016/j.immuni.2023.05.006
59. Shah SA, Kanabar V, Riffo-Vasquez Y, Mohamed Z, Cleary SJ, Corrigan C, et al. Platelets independently recruit into asthmatic lungs and models of allergic inflammation via CCR3. Am J Respir Cell Mol Biol. (2021) 64(5):557–68. doi: 10.1165/rcmb.2020-0425OC
60. Pitchford SC, Momi S, Giannini S, Casali L, Spina D, Page CP, et al. Platelet P-selectin is required for pulmonary eosinophil and lymphocyte recruitment in a murine model of allergic inflammation. Blood. (2005) 105(5):2074–81. doi: 10.1182/blood-2004-06-2282
61. Johansson MW, Mosher DF. Activation of beta1 integrins on blood eosinophils by P-selectin. Am J Respir Cell Mol Biol. (2011) 45(4):889–97. doi: 10.1165/rcmb.2010-0402OC
62. Johansson MW, Han ST, Gunderson KA, Busse WW, Jarjour NN, Mosher DF. Platelet activation, P-selectin, and eosinophil beta1-integrin activation in asthma. Am J Respir Crit Care Med. (2012) 185(5):498–507. doi: 10.1164/rccm.201109-1712OC
63. Barthel SR, Johansson MW, McNamee DM, Mosher DF. Roles of integrin activation in eosinophil function and the eosinophilic inflammation of asthma. J Leukoc Biol. (2008) 83(1):1–12. doi: 10.1189/jlb.0607344
64. Sharma P, Sharma A, Srivastava M. In vivo neutralization of alpha4 and beta7 integrins inhibits eosinophil trafficking and prevents lung injury during tropical pulmonary eosinophilia in mice. Eur J Immunol. (2017) 47(9):1501–12. doi: 10.1002/eji.201747086
65. Walsh GM, Symon FA, Lazarovils AL, Wardlaw AJ. Integrin alpha 4 beta 7 mediates human eosinophil interaction with MAdCAM-1, VCAM-1 and fibronectin. Immunology. (1996) 89(1):112–9. doi: 10.1046/j.1365-2567.1996.d01-713.x
66. Sriramarao P, DiScipio RG, Cobb RR, Cybulsky M, Stachnick G, Castaneda D, et al. VCAM-1 is more effective than MAdCAM-1 in supporting eosinophil rolling under conditions of shear flow. Blood. (2000) 95(2):592–601. doi: 10.1182/blood.V95.2.592
67. Barthel SR, Jarjour NN, Mosher DF, Johansson MW. Dissection of the hyperadhesive phenotype of airway eosinophils in asthma. Am J Respir Cell Mol Biol. (2006) 35(3):378–86. doi: 10.1165/rcmb.2006-0027OC
68. Tozawa H, Kanki Y, Suehiro J, Tsutsumi S, Kohro T, Wada Y, et al. Genome-wide approaches reveal functional interleukin-4-inducible STAT6 binding to the vascular cell adhesion molecule 1 promoter. Mol Cell Biol. (2011) 31(11):2196–209. doi: 10.1128/MCB.01430-10
69. Cheng LE, Sullivan BM, Retana LE, Allen CD, Liang HE, Locksley RM. IgE-activated basophils regulate eosinophil tissue entry by modulating endothelial function. J Exp Med. (2015) 212(4):513–24. doi: 10.1084/jem.20141671
70. Schuijs MJ, Brenis Gomez CM, Bick F, Van Moorleghem J, Vanheerswynghels M, van Loo G, et al. Interleukin-33-activated basophils promote asthma by regulating Th2 cell entry into lung tissue. J Exp Med. (2024) 221(12):e20240103. doi: 10.1084/jem.20240103
71. Korde A, Ahangari F, Haslip M, Zhang X, Liu Q, Cohn L, et al. An endothelial microRNA-1-regulated network controls eosinophil trafficking in asthma and chronic rhinosinusitis. J Allergy Clin Immunol. (2020) 145(2):550–62. doi: 10.1016/j.jaci.2019.10.031
72. Tibbles HE, Navara CS, Hupke MA, Vassilev AO, Uckun FM. Thrombopoietin induces p-selectin expression on platelets and subsequent platelet/leukocyte interactions. Biochem Biophys Res Commun. (2002) 292(4):987–91. doi: 10.1006/bbrc.2002.6759
73. Walker MT, Bloodworth JC, Kountz TS, McCarty SL, Green JE, Ferrie RP, et al. 5-HTP inhibits eosinophilia via intracellular endothelial 5-HTRs; SNPs in 5-HTRs associate with asthmatic lung function. Front Allergy. (2024) 5:1385168. doi: 10.3389/falgy.2024.1385168
74. Abdala-Valencia H, Berdnikovs S, McCary CA, Urick D, Mahadevia R, Marchese ME, et al. Inhibition of allergic inflammation by supplementation with 5-hydroxytryptophan. Am J Physiol Lung Cell Mol Physiol. (2012) 303(8):L642–60. doi: 10.1152/ajplung.00406.2011
75. Lin WC, Fessler MB. Regulatory mechanisms of neutrophil migration from the circulation to the airspace. Cell Mol Life Sci. (2021) 78(9):4095–124. doi: 10.1007/s00018-021-03768-z
76. Banerjee ER, Jiang Y, Henderson WR Jr, Scott LM, Papayannopoulou T. Alpha4 and beta2 integrins have nonredundant roles for asthma development, but for optimal allergen sensitization only alpha4 is critical. Exp Hematol. (2007) 35(4):605–17. doi: 10.1016/j.exphem.2007.01.052
77. Johansson MW, Annis DS, Mosher DF. Alpha(M)beta(2) integrin-mediated adhesion and motility of IL-5-stimulated eosinophils on periostin. Am J Respir Cell Mol Biol. (2013) 48(4):503–10. doi: 10.1165/rcmb.2012-0150OC
78. Zhong ZY, Tang Y. Upregulation of periostin prevents high glucose-induced mitochondrial apoptosis in human umbilical vein endothelial cells via activation of Nrf2/HO-1 signaling. Cell Physiol Biochem. (2016) 39(1):71–80. doi: 10.1159/000445606
79. Takayama G, Arima K, Kanaji T, Toda S, Tanaka H, Shoji S, et al. Periostin: a novel component of subepithelial fibrosis of bronchial asthma downstream of IL-4 and IL-13 signals. J Allergy Clin Immunol. (2006) 118(1):98–104. doi: 10.1016/j.jaci.2006.02.046
80. Oeser K, Maxeiner J, Symowski C, Stassen M, Voehringer D. T cells are the critical source of IL-4/IL-13 in a mouse model of allergic asthma. Allergy. (2015) 70(11):1440–9. doi: 10.1111/all.12705
81. Goenka S, Kaplan MH. Transcriptional regulation by STAT6. Immunol Res. (2011) 50(1):87–96. doi: 10.1007/s12026-011-8205-2
82. Sharma M, Leung D, Momenilandi M, Jones LCW, Pacillo L, James AE, et al. Human germline heterozygous gain-of-function STAT6 variants cause severe allergic disease. J Exp Med. (2023) 220(5):e20221755. doi: 10.1084/jem.20221755
83. Kristjansdottir K, Norddahl GL, Ivarsdottir EV, Halldorsson GH, Einarsson G, Bjarnadottir K, et al. A partial loss-of-function variant in STAT6 protects against type 2 asthma. J Allergy Clin Immunol. (2024) 155:228–35. doi: 10.1016/j.jaci.2024.10.002
84. Gonzalo JA, Lloyd CM, Kremer L, Finger E, Martinez AC, Siegelman MH, et al. Eosinophil recruitment to the lung in a murine model of allergic inflammation. The role of T cells, chemokines, and adhesion receptors. J Clin Invest. (1996) 98(10):2332–45. doi: 10.1172/JCI119045
85. Halim TY, Krauss RH, Sun AC, Takei F. Lung natural helper cells are a critical source of Th2 cell-type cytokines in protease allergen-induced airway inflammation. Immunity. (2012) 36(3):451–63. doi: 10.1016/j.immuni.2011.12.020
86. Li BW, de Bruijn MJ, Tindemans I, Lukkes M, KleinJan A, Hoogsteden HC, et al. T cells are necessary for ILC2 activation in house dust mite-induced allergic airway inflammation in mice. Eur J Immunol. (2016) 46(6):1392–403. doi: 10.1002/eji.201546119
87. Porter PC, Roberts L, Fields A, Knight M, Qian Y, Delclos GL, et al. Necessary and sufficient role for T helper cells to prevent fungal dissemination in allergic lung disease. Infect Immun. (2011) 79(11):4459–71. doi: 10.1128/IAI.05209-11
88. Dietschmann A, Schruefer S, Krappmann S, Voehringer D. Th2 cells promote eosinophil-independent pathology in a murine model of allergic bronchopulmonary aspergillosis. Eur J Immunol. (2020) 50(7):1044–56. doi: 10.1002/eji.201948411
89. Helfrich S, Mindt BC, Fritz JH, Duerr CU. Group 2 innate lymphoid cells in respiratory allergic inflammation. Front Immunol. (2019) 10:930. doi: 10.3389/fimmu.2019.00930
90. Jarick KJ, Topczewska PM, Jakob MO, Yano H, Arifuzzaman M, Gao X, et al. Non-redundant functions of group 2 innate lymphoid cells. Nature. (2022) 611(7937):794–800. doi: 10.1038/s41586-022-05395-5
91. Gurram RK, Wei D, Yu Q, Butcher MJ, Chen X, Cui K, et al. Crosstalk between ILC2s and Th2 cells varies among mouse models. Cell Rep. (2023) 42(2):112073. doi: 10.1016/j.celrep.2023.112073
92. Verma M, Michalec L, Sripada A, McKay J, Sirohi K, Verma D, et al. The molecular and epigenetic mechanisms of innate lymphoid cell (ILC) memory and its relevance for asthma. J Exp Med. (2021) 218(7):e20201354. doi: 10.1084/jem.20201354
93. Liu T, Wu J, Zhao J, Wang J, Zhang Y, Liu L, et al. Type 2 innate lymphoid cells: a novel biomarker of eosinophilic airway inflammation in patients with mild to moderate asthma. Respir Med. (2015) 109(11):1391–6. doi: 10.1016/j.rmed.2015.09.016
94. Peters MC, Mekonnen ZK, Yuan S, Bhakta NR, Woodruff PG, Fahy JV. Measures of gene expression in sputum cells can identify TH2-high and TH2-low subtypes of asthma. J Allergy Clin Immunol. (2014) 133(2):388–94. doi: 10.1016/j.jaci.2013.07.036
95. Chapoval SP, Dasgupta P, Smith EP, DeTolla LJ, Lipsky MM, Kelly-Welch AE, et al. STAT6 expression in multiple cell types mediates the cooperative development of allergic airway disease. J Immunol. (2011) 186(4):2571–83. doi: 10.4049/jimmunol.1002567
96. Gazzinelli-Guimaraes PH, Golec DP, Karmele EP, Sciurba J, Bara-Garcia P, Hill T, et al. Eosinophil trafficking in allergen-mediated pulmonary inflammation relies on IL-13-driven CCL-11 and CCL-24 production by tissue fibroblasts and myeloid cells. J Allergy Clin Immunol Glob. (2023) 2(4):100131. doi: 10.1016/j.jacig.2023.100131
97. Bouffi C, Rochman M, Zust CB, Stucke EM, Kartashov A, Fulkerson PC, et al. IL-33 markedly activates murine eosinophils by an NF-kappaB-dependent mechanism differentially dependent upon an IL-4-driven autoinflammatory loop. J Immunol. (2013) 191(8):4317–25. doi: 10.4049/jimmunol.1301465
98. Zaslona Z, Przybranowski S, Wilke C, van Rooijen N, Teitz-Tennenbaum S, Osterholzer JJ, et al. Resident alveolar macrophages suppress, whereas recruited monocytes promote, allergic lung inflammation in murine models of asthma. J Immunol. (2014) 193(8):4245–53. doi: 10.4049/jimmunol.1400580
99. Draijer C, Robbe P, Boorsma CE, Hylkema MN, Melgert BN. Dual role of YM1+ M2 macrophages in allergic lung inflammation. Sci Rep. (2018) 8(1):5105. doi: 10.1038/s41598-018-23269-7
100. Watanabe K, Jose PJ, Rankin SM. Eotaxin-2 generation is differentially regulated by lipopolysaccharide and IL-4 in monocytes and macrophages. J Immunol. (2002) 168(4):1911–8. doi: 10.4049/jimmunol.168.4.1911
101. Ohnmacht C, Schwartz C, Panzer M, Schiedewitz I, Naumann R, Voehringer D. Basophils orchestrate chronic allergic dermatitis and protective immunity against helminths. Immunity. (2010) 33(3):364–74. doi: 10.1016/j.immuni.2010.08.011
102. Hammad H, Plantinga M, Deswarte K, Pouliot P, Willart MA, Kool M, et al. Inflammatory dendritic cells–not basophils–are necessary and sufficient for induction of Th2 immunity to inhaled house dust mite allergen. J Exp Med. (2010) 207(10):2097–111. doi: 10.1084/jem.20101563
103. Zhong W, Su W, Zhang Y, Liu Q, Wu J, Di C, et al. Basophils as a primary inducer of the T helper type 2 immunity in ovalbumin-induced allergic airway inflammation. Immunology. (2014) 142(2):202–15. doi: 10.1111/imm.12240
104. Motomura Y, Morita H, Moro K, Nakae S, Artis D, Endo TA, et al. Basophil-derived interleukin-4 controls the function of natural helper cells, a member of ILC2s, in lung inflammation. Immunity. (2014) 40(5):758–71. doi: 10.1016/j.immuni.2014.04.013
105. Loffredo LF, Coden ME, Jeong BM, Walker MT, Anekalla KR, Doan TC, et al. Eosinophil accumulation in postnatal lung is specific to the primary septation phase of development. Sci Rep. (2020) 10(1):4425. doi: 10.1038/s41598-020-61420-5
106. Mesnil C, Raulier S, Paulissen G, Xiao X, Birrell MA, Pirottin D, et al. Lung-resident eosinophils represent a distinct regulatory eosinophil subset. J Clin Invest. (2016) 126(9):3279–95. doi: 10.1172/JCI85664
107. Gurtner A, Crepaz D, Arnold IC. Emerging functions of tissue-resident eosinophils. J Exp Med. (2023) 220(7):e20221435. doi: 10.1084/jem.20221435
108. Arnold IC, Munitz A. Spatial adaptation of eosinophils and their emerging roles in homeostasis, infection and disease. Nat Rev Immunol. (2024) 24(12):858–77. doi: 10.1038/s41577-024-01048-y
109. Abdala Valencia H, Loffredo LF, Misharin AV, Berdnikovs S. Phenotypic plasticity and targeting of siglec-F(high) CD11c(low) eosinophils to the airway in a murine model of asthma. Allergy. (2016) 71(2):267–71. doi: 10.1111/all.12776
110. Johansson MW, Kelly EA, Busse WW, Jarjour NN, Mosher DF. Up-regulation and activation of eosinophil integrins in blood and airway after segmental lung antigen challenge. J Immunol. (2008) 180(11):7622–35. doi: 10.4049/jimmunol.180.11.7622
111. Noble SL, Vacca F, Hilligan KL, Mules TC, Le Gros G, Inns S. Helminth infection induces a distinct subset of CD101(hi) lung tissue-infiltrating eosinophils that are differentially regulated by type 2 cytokines. Immunol Cell Biol. (2024) 102(8):734–46. doi: 10.1111/imcb.12796
112. Zhu C, Weng QY, Zhou LR, Cao C, Li F, Wu YF, et al. Homeostatic and early-recruited CD101(-) eosinophils suppress endotoxin-induced acute lung injury. Eur Respir J. (2020) 56(5):1902354. doi: 10.1183/13993003.02354-2019
113. Bruggemann TR, Peh HY, Tavares LP, Nijmeh J, Shay AE, Rezende RM, et al. Eosinophil phenotypes are functionally regulated by resolvin D2 during allergic lung inflammation. Am J Respir Cell Mol Biol. (2023) 69(6):666–77. doi: 10.1165/rcmb.2023-0121OC
114. Dolitzky A, Shapira G, Grisaru-Tal S, Hazut I, Avlas S, Gordon Y, et al. Transcriptional profiling of mouse eosinophils identifies distinct gene signatures following cellular activation. Front Immunol. (2021) 12:802839. doi: 10.3389/fimmu.2021.802839
115. Zhang M, Angata T, Cho JY, Miller M, Broide DH, Varki A. Defining the in vivo function of siglec-F, a CD33-related siglec expressed on mouse eosinophils. Blood. (2007) 109(10):4280–7. doi: 10.1182/blood-2006-08-039255
116. Willebrand R, Voehringer D. IL-33-induced cytokine secretion and survival of mouse eosinophils is promoted by autocrine GM-CSF. PLoS One. (2016) 11(9):e0163751. doi: 10.1371/journal.pone.0163751
117. Nutku E, Aizawa H, Hudson SA, Bochner BS. Ligation of siglec-8: a selective mechanism for induction of human eosinophil apoptosis. Blood. (2003) 101(12):5014–20. doi: 10.1182/blood-2002-10-3058
118. Dallaire MJ, Ferland C, Page N, Lavigne S, Davoine F, Laviolette M. Endothelial cells modulate eosinophil surface markers and mediator release. Eur Respir J. (2003) 21(6):918–24. doi: 10.1183/09031936.03.00102002
119. Bowen JL, Keck K, Baruah S, Nguyen KH, Thurman AL, Pezzulo AA, et al. Eosinophil expression of triggering receptor expressed on myeloid cells 1 (TREM-1) restricts type 2 lung inflammation. J Leukoc Biol. (2024) 116(2):409–23. doi: 10.1093/jleuko/qiae061
120. Johansson MW, Gunderson KA, Kelly EA, Denlinger LC, Jarjour NN, Mosher DF. Anti-IL-5 attenuates activation and surface density of beta(2) -integrins on circulating eosinophils after segmental antigen challenge. Clin Exp Allergy. (2013) 43(3):292–303. doi: 10.1111/j.1365-2222.2012.04065.x
121. Rao Z, Liu S, Li Z, Wang Q, Gao F, Peng H, et al. Alarmin-loaded extracellular lipid droplets induce airway neutrophil infiltration during type 2 inflammation. Immunity. (2024) 57(11):2514–29.e7. doi: 10.1016/j.immuni.2024.09.001
122. Matsumura Y. Role of allergen source-derived proteases in sensitization via airway epithelial cells. J Allergy (Cairo). (2012) 2012:903659. doi: 10.1155/2012/903659
123. Sweerus K, Lachowicz-Scroggins M, Gordon E, LaFemina M, Huang X, Parikh M, et al. Claudin-18 deficiency is associated with airway epithelial barrier dysfunction and asthma. J Allergy Clin Immunol. (2017) 139(1):72–81.e1. doi: 10.1016/j.jaci.2016.02.035
124. Sugita K, Steer CA, Martinez-Gonzalez I, Altunbulakli C, Morita H, Castro-Giner F, et al. Type 2 innate lymphoid cells disrupt bronchial epithelial barrier integrity by targeting tight junctions through IL-13 in asthmatic patients. J Allergy Clin Immunol. (2018) 141(1):300–10.e11. doi: 10.1016/j.jaci.2017.02.038
125. Vignola AM, Chanez P, Chiappara G, Siena L, Merendino A, Reina C, et al. Evaluation of apoptosis of eosinophils, macrophages, and T lymphocytes in mucosal biopsy specimens of patients with asthma and chronic bronchitis. J Allergy Clin Immunol. (1999) 103(4):563–73. doi: 10.1016/s0091-6749(99)70225-3
126. Januskevicius A, Janulaityte I, Kalinauskaite-Zukauske V, Gosens R, Malakauskas K. The enhanced adhesion of eosinophils is associated with their prolonged viability and pro-proliferative effect in asthma. J Clin Med. (2019) 8(9):1274. doi: 10.3390/jcm8091274
127. Esnault S, Kelly EA. Essential mechanisms of differential activation of eosinophils by IL-3 compared to GM-CSF and IL-5. Crit Rev Immunol. (2016) 36(5):429–44. doi: 10.1615/CritRevImmunol.2017020172
128. Schwartz C, Willebrand R, Huber S, Rupec RA, Wu D, Locksley R, et al. Eosinophil-specific deletion of IkappaBalpha in mice reveals a critical role of NF-kappaB-induced bcl-xL for inhibition of apoptosis. Blood. (2015) 125(25):3896–904. doi: 10.1182/blood-2014-10-607788
129. Nobs SP, Kayhan M, Kopf M. GM-CSF intrinsically controls eosinophil accumulation in the setting of allergic airway inflammation. J Allergy Clin Immunol. (2019) 143(4):1513–24.e2. doi: 10.1016/j.jaci.2018.08.044
130. Vancheri C, Gauldie J, Bienenstock J, Cox G, Scicchitano R, Stanisz A, et al. Human lung fibroblast-derived granulocyte-macrophage colony stimulating factor (GM-CSF) mediates eosinophil survival in vitro. Am J Respir Cell Mol Biol. (1989) 1(4):289–95. doi: 10.1165/ajrcmb/1.4.289
131. Bunn KE, Giese-Byrne BG, Pua HH. Th2 cell extracellular vesicles promote eosinophil survival through the cytokine cargo IL-3 and prolong airway eosinophilia. bioRxiv. (2024). doi: 10.1101/2024.07.23.600647
132. Gleich GJ, Klion AD, Lee JJ, Weller PF. The consequences of not having eosinophils. Allergy. (2013) 68(7):829–35. doi: 10.1111/all.12169
133. Moore WC, Stach-Klysh A, Corbridge T, Packnett E, McMorrow D, Richards M, et al. Real-world mepolizumab treatment in patients with severe asthma decreased exacerbations, oral corticosteroid use, and healthcare resource utilization and costs over 4 years: a retrospective analysis. J Asthma. (2025) 22:1–13. doi: 10.1080/02770903.2025.2450640
134. Stolz D, Schuoler C, Charbonnier F, Bridevaux PO, Jandus P, Leuppi JD, et al. Early and sustained response to benralizumab in severe, eosinophilic asthma: a real-world observational study. J Asthma Allergy. (2024) 17:1301–12. doi: 10.2147/JAA.S495867
135. Kato M, Ishioka T, Kita H, Kozawa K, Hayashi Y, Kimura H. Eosinophil granular proteins damage bronchial epithelial cells infected with respiratory syncytial virus. Int Arch Allergy Immunol. (2012) 158(Suppl 1):11–8. doi: 10.1159/000337752
136. Pan M, Zhang L, Chang S, Jiang X, Shen J, Feng X, et al. Poly-l-arginine promotes ferroptosis in asthmatic airway epithelial cells by modulating PBX1/GABARAPL1 axis. Int J Biol Macromol. (2025) 286:138478. doi: 10.1016/j.ijbiomac.2024.138478
137. Gigon L, Muller P, Haenni B, Iacovache I, Barbo M, Gosheva G, et al. Membrane damage by MBP-1 is mediated by pore formation and amplified by mtDNA. Cell Rep. (2024) 43(4):114084. doi: 10.1016/j.celrep.2024.114084
138. Dragunas G, Koster CS, de Souza Xavier Costa N, Melgert BN, Munhoz CD, Gosens R, et al. Neuroplasticity and neuroimmune interactions in fatal asthma. Allergy. (2024) 80:462–73. doi: 10.1111/all.16373
139. Sawatzky DA, Kingham PJ, Court E, Kumaravel B, Fryer AD, Jacoby DB, et al. Eosinophil adhesion to cholinergic nerves via ICAM-1 and VCAM-1 and associated eosinophil degranulation. Am J Physiol Lung Cell Mol Physiol. (2002) 282(6):L1279–88. doi: 10.1152/ajplung.00279.2001
140. Drake MG, Lebold KM, Roth-Carter QR, Pincus AB, Blum ED, Proskocil BJ, et al. Eosinophil and airway nerve interactions in asthma. J Leukoc Biol. (2018) 104(1):61–7. doi: 10.1002/JLB.3MR1117-426R
141. Drake MG, Scott GD, Blum ED, Lebold KM, Nie Z, Lee JJ, et al. Eosinophils increase airway sensory nerve density in mice and in human asthma. Sci Transl Med. (2018) 10(457):eaar8477. doi: 10.1126/scitranslmed.aar8477
142. Choi Y, Le Pham D, Lee DH, Lee SH, Kim SH, Park HS. Biological function of eosinophil extracellular traps in patients with severe eosinophilic asthma. Exp Mol Med. (2018) 50(8):1–8. doi: 10.1038/s12276-018-0136-8
143. Choi Y, Kim YM, Lee HR, Mun J, Sim S, Lee DH, et al. Eosinophil extracellular traps activate type 2 innate lymphoid cells through stimulating airway epithelium in severe asthma. Allergy. (2020) 75(1):95–103. doi: 10.1111/all.13997
144. Uchida AM, Ro G, Qiang L, Peterson KA, Round J, Dougan M, et al. Human differentiated eosinophils release IL-13 in response to IL-33 stimulation. Front Immunol. (2022) 13:946643. doi: 10.3389/fimmu.2022.946643
145. Piehler D, Stenzel W, Grahnert A, Held J, Richter L, Kohler G, et al. Eosinophils contribute to IL-4 production and shape the T-helper cytokine profile and inflammatory response in pulmonary cryptococcosis. Am J Pathol. (2011) 179(2):733–44. doi: 10.1016/j.ajpath.2011.04.025
146. Bjerke T, Gaustadnes M, Nielsen S, Nielsen LP, Schiotz PO, Rudiger N, et al. Human blood eosinophils produce and secrete interleukin 4. Respir Med. (1996) 90(5):271–7. doi: 10.1016/s0954-6111(96)90098-0
147. Jacobsen EA, Ochkur SI, Pero RS, Taranova AG, Protheroe CA, Colbert DC, et al. Allergic pulmonary inflammation in mice is dependent on eosinophil-induced recruitment of effector T cells. J Exp Med. (2008) 205(3):699–710. doi: 10.1084/jem.20071840
148. LeSuer WE, Kienzl M, Ochkur SI, Schicho R, Doyle AD, Wright BL, et al. Eosinophils promote effector functions of lung group 2 innate lymphoid cells in allergic airway inflammation in mice. J Allergy Clin Immunol. (2023) 152(2):469–85.e10. doi: 10.1016/j.jaci.2023.03.023
149. Takeda K, Shiraishi Y, Ashino S, Han J, Jia Y, Wang M, et al. Eosinophils contribute to the resolution of lung-allergic responses following repeated allergen challenge. J Allergy Clin Immunol. (2015) 135(2):451–60. doi: 10.1016/j.jaci.2014.08.014
150. Tani Y, Isobe Y, Imoto Y, Segi-Nishida E, Sugimoto Y, Arai H, et al. Eosinophils control the resolution of inflammation and draining lymph node hypertrophy through the proresolving mediators and CXCL13 pathway in mice. FASEB J. (2014) 28(9):4036–43. doi: 10.1096/fj.14-251132
151. Dolitzky A, Hazut I, Avlas S, Grisaru-Tal S, Itan M, Zaffran I, et al. Differential regulation of type 1 and type 2 mouse eosinophil activation by apoptotic cells. Front Immunol. (2022) 13:1041660. doi: 10.3389/fimmu.2022.1041660
Keywords: eosinophils, allergic airway inflammation, type 2 immunity, asthma, IL-5
Citation: Graf L-M, Radtke D and Voehringer D (2025) Regulation of eosinophil recruitment and heterogeneity during allergic airway inflammation. Front. Allergy 6:1585142. doi: 10.3389/falgy.2025.1585142
Received: 28 February 2025; Accepted: 31 March 2025;
Published: 10 April 2025.
Edited by:
Nives Zimmermann, University of Cincinnati, United StatesReviewed by:
Mats W. Johansson, University of Wisconsin-Madison, United StatesIan Paul Lewkowich, Cincinnati Children's Hospital Medical Center, United States
Copyright: © 2025 Graf, Radtke and Voehringer. This is an open-access article distributed under the terms of the Creative Commons Attribution License (CC BY). The use, distribution or reproduction in other forums is permitted, provided the original author(s) and the copyright owner(s) are credited and that the original publication in this journal is cited, in accordance with accepted academic practice. No use, distribution or reproduction is permitted which does not comply with these terms.
*Correspondence: David Voehringer, ZGF2aWQudm9laHJpbmdlckB1ay1lcmxhbmdlbi5kZQ==