- 1 Departamento de Fisiología (Fisiología Animal II), Facultad de Ciencias Biológicas, Universidad Complutense de Madrid, Madrid, Spain
- 2 Instituto de Investigación Sanitaria del Hospital Clínico San Carlos, Madrid, Spain
- 3 Instituto de Neurociencias de Alicante, Universidad Miguel Hernández – CSIC, San Juan de Alicante, Spain
- 4 Laboratorio de Medicina Regenerativa, Hospital Carlos Haya de Malaga, Fundacion IMABIS, Malaga, Spain
- 5 Neurocentre Magendie, INSERM, Université Bordeaux 2, Bordeaux, France
- 6 Department of Pharmacology, Institute of Biological Sciences, Universidade Federal de Minas Gerais, Belo Horizonte, MG, Brazil
- 7 Department of Pharmacology, School of Medicine of Ribeirão Preto, University of São Paulo, Ribeirão Preto, SP, Brazil
Public concern on mental health has noticeably increased given the high prevalence of neuropsychiatric disorders. Cognition and emotionality are the most affected functions in neuropsychiatric disorders, i.e., anxiety disorders, depression, and schizophrenia. In this review, most relevant literature on the role of the endocannabinoid (eCB) system in neuropsychiatric disorders will be presented. Evidence from clinical and animal studies is provided for the participation of CB1 and CB2 receptors (CB1R and CB2R) in the above mentioned neuropsychiatric disorders. CBRs are crucial in some of the emotional and cognitive impairments reported, although more research is required to understand the specific role of the eCB system in neuropsychiatric disorders. Cannabidiol (CBD), the main non-psychotropic component of the Cannabis sativa plant, has shown therapeutic potential in several neuropsychiatric disorders. Although further studies are needed, recent studies indicate that CBD therapeutic effects may partially depend on facilitation of eCB-mediated neurotransmission. Last but not least, this review includes recent findings on the role of the eCB system in eating disorders. A deregulation of the eCB system has been proposed to be in the bases of several neuropsychiatric disorders, including eating disorders. Cannabis consumption has been related to the appearance of psychotic symptoms and schizophrenia. In contrast, the pharmacological manipulation of this eCB system has been proposed as a potential strategy for the treatment of anxiety disorders, depression, and anorexia nervosa. In conclusion, the eCB system plays a critical role in psychiatry; however, detrimental consequences of manipulating this endogenous system cannot be underestimated over the potential and promising perspectives of its therapeutic manipulation.
Psychiatric disorders severely compromise the well-being of those affected causing serious psychological distress in the general population. These disorders have a relatively high prevalence [Kessler et al., 2005; Substance Abuse and Mental Health Services Administration, SAMHSA (2006)], can have an early onset (i.e., schizophrenia in young adulthood) or a relapsing-remitting course (as in mood and anxiety disorders), and frequently have disabling symptoms. Cognition and emotion regulation are the most affected functions in neuropsychiatric disorders. In fact, such functions have been reported to be critically impaired in patients suffering from anxiety disorders, schizophrenia, and major depression (Hyman, 2008; Dere et al., 2010).
Anxiety is an adaptive component of the acute stress response under circumstances that threaten the integrity of the individual, and thus can be regarded as a “normal” emotion. However, if anxiety is disproportional in intensity or chronicity, or is not associated with any actual risk, it constitutes a maladaptive response or even a neuropsychiatric disorder. Indeed, anxiety disorders are marked by excessive fear (and avoidance), often in response to specific objects or situations and in the absence of true danger. Anxiety disorders, such as panic disorder, social and specific phobia, generalized anxiety disorder and post-traumatic stress disorder (PTSD) are highly prevalent and strongly disabling class of neuropsychiatric disorders (Bekker and van Mens-Verhulst, 2007; Shin and Liberzon, 2010). Depression is characterized by abnormal representation and regulation of affect, mood and emotion. Anhedonia, that is, decreased levels of emotional activation after presentation of rewarding stimuli is generally considered as a core symptom of depressive patients (Davidson et al., 2002; Levens and Gotlib, 2009). Cognitive impairments are frequently observed in patients with anxiety disorders and depression. Although mild anxiety seems to be associated with better cognitive performance, severe anxiety symptoms are negatively associated with cognitive functioning (Bierman et al., 2005; Gualtieri and Morgan, 2008). More particularly, PTSD, that develops after prolonged inescapable stress experience of exceptional severity (Rubin et al., 2008), has been associated with a number of cognitive impairments, including basic deficits in attention, concentration, and memory (Isaac et al., 2006). In contrast, depressive symptoms are always negatively associated with cognitive performance. Actually, low episodic memory performance has been proposed as a premorbid marker of depression (Airaksinen et al., 2007). Finally, schizophrenia is characterized by profound disruption in cognition and emotion, affecting the most fundamental human attributes. A wide diversity of symptoms is described in schizophrenic patients including hallucinations and delusions, together with remarkable cognitive deficits that critically influence the course of the disorder (Barch, 2005).
Cannabis is one of the illicit drugs more frequently abused in the western societies. A great variety of chemical compounds are present in the plan of Cannabis sativa, mainly delta-9-tetrahydrocannabinol (THC), responsible of the addictive and psychoactive properties of cannabis, and cannabidiol (CBD). Cannabis, as a drug of abuse, induces changes in the central nervous system (CNS) that may lead to dependence. Indeed, the development of a dependence syndrome is among the most probable adverse effects of cannabis consumption (Budney et al., 2004; Fattore et al., 2008). Cannabis consumption induces euphoria, and is frequently accompanied by decreases in anxiety, although acute aversive emotional reactions to cannabis have also been reported (consult Crippa et al., 2009 for review). Notable cognitive impairments have been observed following marijuana intake in humans, and a contribution neurochemical processes occurring in both prefrontal cortex and hippocampus have been proposed (Egerton et al., 2006; Cohen et al., 2008). Therefore, public concern is growing in relation to the adverse effects of regular use on adolescent psychosocial development and mental health (Jager and Ramsey, 2008; Hall and Degenhardt, 2009). There is increasing evidence indicating a close relationship between cannabis consumption and an increased risk for depression, anxiety disorders, psychotic symptoms, or even schizophrenia (Degenhardt et al., 2003; Manzanares et al., 2004; Sundram, 2006; Di Forti et al., 2007; Leweke and Koethe, 2008)).
Cannabis derivatives, also known as phytocannabinoids, influence the CNS through activation of the endocannabinoid (eCB) system, mainly composed by the endogenous ligands (endocannabinoids, eCBs) and their specific membrane receptors, together with the enzymatic machinery in charge of eCB synthesis and inactivation (Andre and Gonthier, 2010; Maccarrone et al., 2010). Endocannabinoids have been shown to modulate neurotransmission, mainly acting as retrograde transmitters (Marsicano and Lutz, 2006), and have been involved in a plethora of physiological functions. Data from human and animal studies have consistently demonstrated that the eCB system is pivotal for emotional homeostasis and cognitive function (Viveros et al., 2007; Moreira and Lutz, 2008; Solowij and Battisti, 2008; Marco and Viveros, 2009). In turn, deregulation of the eCB system has been associated with psychopathological conditions that compromise emotional and cognitive function, such as anxiety-related disorders, depression, and schizophrenia. Herein, we will review the latest breakthroughs on the role played by the eCB system in neuropsychiatric disorders, focusing in emotional, and cognitive impairments that critically affect individuals’ well-being.
A Brief Update on the Endocannabinoid (eCB) System
The eCB system system modulates the neurotransmission at inhibitory and excitatory synapses in brain regions relevant to the regulation of pain, emotion, motivation, and cognition (Viveros et al., 2005; Wotjak, 2005; Moreira and Lutz, 2008; Guindon and Hohmann, 2009; Finn, 2010; Moreira and Wotjak, 2010). In the last decades, investigation of the eCB system has considerably increased and our understanding of this system has achieved remarkable aims (see Andre and Gonthier, 2010; Maccarrone et al., 2010 for an updated review). Endocannabinoids, the endogenous ligands, are polyunsaturated fatty acid derivatives that bind to cannabinoid receptors. Two types of cannabinoid receptors have been characterized to date, CB1 (Herkenham et al., 1991) and CB2 receptors (Munro et al., 1993) both metabotropic receptors coupled to Gαi/o proteins. CB1Rs are expressed ubiquitously throughout the brain; they are found at highest concentrations in the hippocampus, neocortex, basal ganglia, and cerebellum; while a moderate presence is observed in the basolateral amygdala, hypothalamus, and midbrain (Herkenham et al., 1991; Mailleux and Vanderhaeghen, 1992; Glass et al., 1997). Apart from neurons, CB1Rs have also been described in non-neuronal cells such as astrocytes (Bouaboula et al., 1995; Sanchez et al., 1998), microglia (Waksman et al., 1999; Walter et al., 2003), and oligodendrocytes (Molina-Holgado et al., 2002; for review consult Mackie, 2005). In contrast, discrepant opinions exist regarding the pattern of expression of CB2Rs. Initially, CB2R was identified at high levels in peripheral immune tissues, as rat spleen and immune cells in humans (Munro et al., 1993; Galiegue et al., 1995), in a lower extent, in the muscle, liver, intestine, and testis (Liu et al., 2009), as well as in the adipose tissue (Roche et al., 2006). Additionally, CB2R was also found in the brain under pathological conditions, i.e., in tumors (Joosten et al., 2002), glioma (Guzman et al., 2001), neuropathic pain (Ibrahim et al., 2003), senile plaques in Alzheimer’s disease (Ehrhart et al., 2005), arteriosclerotic plaques (Steffens et al., 2005), while no CB2R expression was found in the brain under normal physiological conditions (Chakrabarti et al., 1995; Derocq et al., 1995; Schatz et al., 1997; Griffin et al., 1999; Carlisle et al., 2002). However, more recently CB2Rs have been identified in cerebellum and brainstem (Van Sickle et al., 2005). Indeed, further studies have now described the presence of CB2Rs, both gene and protein expression, in different brain regions under normal physiological conditions, including cerebral cortex, striatum, hippocampus, amygdala, periaqueductal gray (PAG), and several hypothalamic nuclei (Gong et al., 2006; Onaivi, 2006; García-Gutiérrez et al., 2010). Although CB1Rs and CB2Rs are well-known and characterized, numerous pharmacological studies have suggested the existence of additional cannabinoid receptors. In this regard, eCB ligands have been reported to bind to the transient receptor potential vanilloid type 1 (TRPV1) ion channel (Starowicz et al., 2007), and two G protein-coupled receptors, GPR55 and GPR119, have been proposed as novel potential cannabinoid receptors (Baker et al., 2006). Moreover, increasing evidence now suggests that eCBs are also natural activators of the peroxisome proliferator-activated receptor (PPAR) family of nuclear receptors (O’Sullivan and Kendall, 2010).
Endocannabinoids, due to their lipophilic nature, are synthesized and released “on demand” by the cleavage of membrane phospholipid precursors in response to diverse physiological and pathological stimuli. The two most widely studied eCBs are N-arachidonoyl-ethanolamide (AEA), also called anandamide, and 2-arachidonoylglycerol (2-AG). The biological actions of these polyunsaturated lipids are controlled by key agents responsible for their synthesis, transport, and degradation. eCBs can passively diffuse through lipid membranes, but a high affinity transporter, not yet identified, seems to accelerate this process. A fatty acid amide hydrolase (FAAH) is the main AEA hydrolase, whereas 2-AG inactivation is mainly afforded by the enzyme monoacylglycerol lipase (MGL), and by novel 2-AG-hydrolyzing lipases recently identified (consult Ahn et al., 2008; Andre and Gonthier, 2010; Maccarrone et al., 2010; Pamplona and Takahashi, 2011; Ueda et al., 2011; for more detailed information and/or an updated review).
Involvement of CB1Rs in Neuropsychiatric Disorders
CB1Rs in Anxiety Disorders
There is substantial evidence from both human and animal studies for a role of the eCB system in the control of emotional states. CB1Rs, as mentioned above (A Brief Update on the Endocannabinoid (eCB) System), is widely distributed in brain areas associated with emotional regulation and stress responsiveness such as prefrontal cortex, hippocampus, amygdala, and hypothalamus (Mackie, 2005), thus a role for eCB signaling in anxiety-related disorders might be suggested. Genetic and pharmacological blockade of CB1Rs further support a role for the eCB system in emotional homeostasis, and thus in anxiety-related disorders. Mutant mice lacking CB1Rs (CB1R knock-out mice, CB1KO) display increased anxiety levels compared to control animals (wild-type) in a variety of behavioral paradigms, i.e., the light–dark box, the elevated plus-maze test, and the social interaction test, as well as increased aggressiveness as measured in the resident–intruder test (Haller et al., 2002; Martin et al., 2002b; Urigüen et al., 2004; but see also Marsicano et al., 2002; Haller et al., 2004). Notwithstanding, baseline trait levels of emotionality critically affect animals’ performance in theses tests, and it is in turn notably influenced by both genetic strain and environmental testing conditions (Clement et al., 2002; Yilmazer-Hanke, 2008). In this regard, mutant CB1R mice exclusively exhibited an anxious phenotype under aversive conditions, i.e., high illumination and first exposure in the elevated plus-maze and the social interaction test (Haller et al., 2002, 2004; Marsicano et al., 2002; Martin et al., 2002a; Urigüen et al., 2004). Similarly, systemic administration of rimonabant (SR141716A), a cannabinoid antagonist, induces an anxiogenic profile in rats, i.e., elevated plus-maze and defensive withdrawal test (Navarro et al., 1997; Arevalo et al., 2001) in particular if the animals are tested under highly aversive conditions, i.e., brightly lit environments (Haller et al., 2004), although contradictory results have been obtained in mice, i.e., elevated plus-maze (Akinshola et al., 1999; Haller et al., 2002; Rodgers et al., 2003). It is worth noting that clinical data resemble rat literature, and in humans rimonabant has been associated with increased anxiety and depressed mood (Doggrell, 2008; Rosenstock et al., 2008; Scheen, 2008; Van Gaal et al., 2008). Indeed, these adverse psychiatric effects of rimonabant led to the withdrawal of this anti-obesity drug from the European market1 (Doc. Ref. EMEA/CHMP/537777/2008). In addition, CB1KO seem not to respond to the anxiolytic actions of benzodiazepines (Urigüen et al., 2004) and further studies demonstrated that CB1R is critically involved in the control of GABAergic neurotransmission, and so in the anxiolytic actions of benzodiazepines (García-Gutiérrez and Manzanares, 2010; Urigüen et al., 2011). Given that benzodiazepines are one of the most prescribed anxiolytic drugs, the participation of CB1R in their pharmacological action additionally supports the eCB system as a fundamental piece in anxiety disorders (see also Viveros et al., 2005; Wotjak, 2005; Marco and Viveros, 2009; Finn, 2010; Moreira and Wotjak, 2010 for review).
The role of CB1R in learning and memory is well documented (for review, Wotjak, 2005; Lutz, 2007). CB1R has been specifically involved in the facilitation of behavioral adaptation after the acquisition of aversive memories. Marsicano et al. (2002) demonstrated that the eCB system has a central function in extinction of aversive memories. Genetic disruption of CB1Rs strongly impaired short-term and long-term extinction in auditory fear-conditioning tests, in the absence of changes in memory acquisition and consolidation processes (Marsicano et al., 2002). Interestingly, eCBs seem to be specifically involved in extinction of aversive memories since extinction of appetitive memories were not affected in CB1KO mice (Holter et al., 2005). Similarly, the pharmacological blockade of CB1Rs led to a significant impairment in extinction, when rimonabant was administered prior to extinction training in the fear-potentiated startle test (Chhatwal et al., 2005). Recent evidence suggests that eCBs may primarily affect habituation-like processing, thought to be more related to acute fear relief (Kamprath et al., 2006). In this context, it has been postulated that only if a certain threshold of averseness is exceeded by a stimulus and/or test situation is the eCB system activated to exert fear alleviating effects (Kamprath et al., 2009). Consequently, Moreira and Wotjak (2010) hypothesized that the eCB system may have a prevailing protective role to prevent exaggerated fear responses. If this hypothesis is confirmed, then the eCB system may underlie the aberrant memory processing and impaired adaptation to changed environmental conditions that has been described in several human neuropsychiatric disorders, such as PTSD (Isaac et al., 2006), and new therapeutic opportunities could be offered for the management of PTSD. In fact, a clinical trial (phase IV) on the efficacy of THC treatment for the management of PTSD is going on. Adult subjects of both genders are being currently recruited and first results will be soon available2.
In humans, cannabis is mainly consumed due to its euphoriant properties, which are usually accompanied by decreases in anxiety. However, dysphoric reactions, feelings of anxiety, panic, paranoia, and psychosis are also frequently reported (see Crippa et al., 2009 for review). Similarly, in rodents a bidirectional profile regarding anxiety-like responses has been reported with low doses of cannabinoid compounds exerting anxiolytic-like effects while the opposite is observed following the administration of high doses (consult for review, Viveros et al., 2005; Moreira and Lutz, 2008). Despite basal emotional state as well as contextual testing conditions are critical in this respect, putative neural mechanisms underlying this biphasic profile have been thoroughly investigated. Anxiolytic- and anxiogenic-like effects of cannabinoid agonists appear to be mediated through the same neurotransmitter systems although by activating different receptors. The endogenous opioid system is involved in the regulation of cannabinoid-induced anxiety-like responses; pharmacological studies indicate that anxiolytic-like responses are mediated by μ- and δ-opioid receptors (Berrendero and Maldonado, 2002) while κ-opioid receptors might be involved in the anxiogenic-like responses (Marin et al., 2003).The serotonergic system, particularly 5-HT1A receptors (5-HT1ARs), participate in the anxiety-related effects of cannabinoid compounds although controversial results have been reported (Marco et al., 2004; Braida et al., 2007). GABAergic and glutamatergic neurotransmissions have also been involved in the anxiety-like responses to cannabinoid compounds. CB1Rs have been localized on both glutamatergic (Domenici et al., 2006; Kawamura et al., 2006; Monory et al., 2006) and GABAergic (Katona et al., 1999, 2001) neurons but such receptors may differ in their sensitivity to cannabinoid compounds. Actually, differences in the cannabinoid sensitivity of glutamatergic and GABAergic neurotransmission between mice and rats was suggested to underlie the differences in cannabinoid-induced anxiety-related responses previously described (Haller et al., 2007). More recently, the contribution of GABAergic and glutamatergic neurotransmission in the biphasic emotional effects of cannabinoids has been analyzed by using KO mice specifically lacking CB1R in GABAergic neurons (GABA-CB1KO) or glutamatergic forebrain neurons (Glu-CB1-KO). The presence of CB1Rs on the glutamatergic terminals may be considered as a requirement for the anxiolytic-like responses elicited following the administration of low doses of a cannabinoid agonist. In contrast, CB1Rs on GABAergic terminals seem to be involved in the anxiogenic-like effects associated to high doses of cannabinoid compounds (Lutz et al., 2010). Taken together, cannabinoid agonists, depending upon their chemical structure and dosage, may act on a diversity of cannabinoid and non-cannabinoid receptors [see above, A Brief Update on the Endocannabinoid (eCB) System] present in distinct neuroanatomical regions and differing in their binding properties. Despite the great efforts devoted to understand the biphasic profile of cannabinoid-induced effects, not exclusive of emotional-related responses, a consensus on the underlying mechanisms has not yet been reached.
Our knowledge on the role of the eCB system in emotion and anxiety disorders has notably increased in the last decade. Data presently available provide evidence for an intrinsic eCB tone that may control emotional homeostasis, mainly acting through CB1R activation. Equilibrium in eCB signaling is pivotal not only to maintain adequate baseline anxiety levels, but also to promote recovery and/or adaptation to stressful and aversive situations. Disequilibrium or malfunctioning of the eCB system might contribute to the etiology of anxiety-related disorders (Sundram, 2006; Marco and Viveros, 2009; Finn, 2010; Parolaro et al., 2010), whereas the pharmacological enhancement of eCB activation may provide a promising therapeutic tool for the management of such disorders (Pacher et al., 2006; Piomelli et al., 2006). Given the successful results accomplished in animal studies, great expectations exist for the future clinical exploitation of this system.
CB1Rs in Depression
Several hypotheses for the neurobiological basis of depression have been formulated (Nestler et al., 2002), and, in the last years, a deregulation of the eCB system has been proposed (for review consult Vinod and Hungund, 2006; Parolaro et al., 2010; Gorzalka and Hill, 2011). Evidence for a relationship between the eCB system and human depression has arisen. Clinical populations diagnosed with depression are found to have reduced levels of circulating eCBs (Hill et al., 2009) and an up-regulation of CB1R was observed in the prefrontal cortex of subjects with major depression who died by suicide (Hungund et al., 2004). Furthermore, a genetic risk factor for depression in Parkinson’s disease was found to be associated with polymorphisms of human gene for CB1R (CNR1), mapped to chromosome 6q14–15 (Barrero et al., 2005). More recent studies have confirmed that polymorphisms in the CNR1 gene are a risk factor for depression, and have suggested that the CNR1 gene influences vulnerability to psychosocial adversity to later develop depressive symptoms (Juhasz et al., 2009).
Evidence from animal models further support the participation of CB1R in depression. Genetic deletion of CB1R has been reported to induce a behavioral state analogous to depression in experimental animals. CB1KO mice became anhedonic before than wild-type mice when exposed to chronic mild stress (CMS), so lack of CB1R may render animals more vulnerable to the anhedonic effect of chronic stress (Martin et al., 2002a). CB1KO mice have been reported to exhibit a decreased sensitivity to rewarding stimuli (Sanchis-Segura et al., 2004) and a depressive-like phenotype in both the forced–swim test (FST; Steiner et al., 2008) and the test suspension test (TST; Aso et al., 2008). A deficit in extinction of aversive memories has also been reported (Marsicano et al., 2002). Impairments in working memory, measured as spontaneous alternation, have been described in CB1KO mice (Ledent et al., 1999), although performance in other cognitive tasks, i.e., object recognition and active avoidance, was not found to be affected (Reibaud et al., 1999; Maccarrone et al., 2002; Martin et al., 2002a). In addition, anomalies in the hypothalamus–pituitary–adrenal (HPA) axis have been described in CB1KO mice. In particular, an hyperactivity of the HPA axis as suggested by the higher corticosterone levels registered in CB1KO mice after exposure to stress compared to control wild-type animals (Urigüen et al., 2004). Taken together, genetic depletion of the CB1R resulted in a “depressive-like” phenotype at the preclinical level; CB1KO mice displayed an anhedonic state, emotional changes, cognitive deficits, an increased HPA axis activity as well as impairments in stress adaptation (reviewed by Vinod and Hungund, 2006; Parolaro et al., 2010; Gorzalka and Hill, 2011). Brain derived neurotrophic factor (BDNF) is considered a biochemical marker of depression. Actually, depression has been associated to a reduced expression of BDNF in the hippocampus (Yu and Chen, 2010). Accordingly, decreased BDNF levels have been observed in the hippocampus of CB1KO mice (Aso et al., 2008; Steiner et al., 2008). In a more recent study, gene expression of CB1KO versus control wild-type mice has been analyzed by using microarrays technology (Aso et al., 2011). The study revealed an altered gene expression pattern in CB1KO mice (at basal conditions) that may contribute to the depressive-like phenotype and to the increased reactivity to stress previously described in these mutant animals (Aso et al., 2011). A comparative study following repeated exposure to stress was also performed, and most differences in stress reactivity were observed in the raphe nucleus, a brain region closely related to depression (Aso et al., 2011).
Apart from evidences from the genetic and pharmacological blockade of CB1R, changes in receptor expression have also been described in diverse animal models of depression (Table 1). A consistent increase of CB1R expression in the prefrontal cortex has been reported in different animal models of depression, i.e., CMS (Bortolato et al., 2007), chronic unpredictable stress (Hill et al., 2008), and bilateral olfactory bulbectomy (Rodriguez-Gaztelumendi et al., 2009). Noticeably, a similar effect was found for cortical CB1R expression in a population of depressed suicides (Hungund et al., 2004). In contrast, decreases in CB1R expression have been reported in hippocampus (Hill et al., 2005, 2008; Reich et al., 2009), hypothalamus, ventral striatum (nucleus accumbens; Hill et al., 2008), and midbrain (Bortolato et al., 2007). However, discrepancies regarding changes in CB1R expression in animal models of depression have been found, and may probably be due to differences in the animal model employed and/or in the technique used. Sex differences have been reported for several aspects of pathologies, however, male and female animals are hardly considered in preclinical studies. In the CMS model of depression a down-regulation of hippocampal CB1R has been observed among adult male animals (Hill et al., 2005, 2008; Reich et al., 2009), whereas a CB1R up-regulation was found exclusively in the dorsal hippocampus of females (Reich et al., 2009). In accordance with these findings, sex differences in the eCB system might be hypothesized, at least in relation to stress-responding circuitries. However, more research is still needed to better understand the behavioral implications of the regional and sexual specific changes in brain CB1R expression.
As for anxiety disorders, a dysfunction of the eCB system has been proposed to be in the bases of depression (Vinod and Hungund, 2006; Parolaro et al., 2010; Gorzalka and Hill, 2011). Enhancing the levels of eCBs by inhibiting their deactivation has become a promising antidepressant strategy (Pacher et al., 2006; Bambico and Gobbi, 2008). In contrast, inactivation of CB1Rs can have detrimental consequences provoking depressive-like symptoms. In fact, rimonabant adverse effects included not only increased anxiety, but also depression and suicidal ideations (Doggrell, 2008; Rosenstock et al., 2008; Scheen, 2008; Van Gaal et al., 2008). In this line, an association between depression and prolonged cannabis consumption and its withdrawal have also been reported (Degenhardt et al., 2003). Despite appealing, existing literature suggests caution in the pharmacological exploitation of the eCB system. Indeed, further investigation is necessary to understand the clinical limits of such manipulation that may differ among sexes, age, and individuals.
CB1Rs in Schizophrenia
The association between cannabis use and psychosis has long been recognized (see for review, D’Souza et al., 2009), and recent advances in the neurobiology of cannabinoids have renewed interest in the association between cannabis and schizophrenia (see Muller-Vahl and Emrich, 2008; Fernandez-Espejo et al., 2009; Parolaro et al., 2010 for review). There are several lines of evidence that support an association between an altered eCB system and the pathogenesis of schizophrenia. In clinical studies, up-regulation of CB1R has been described in cortical brain regions such as the dorsolateral prefrontal cortex (Dean et al., 2001) and in cingulate cortex (Zavitsanou et al., 2004; Newell et al., 2006) of schizophrenic patients. However, investigation in post mortem schizophrenic brains have yielded contrasting results, and a both no changes or even decreases in CB1R expression have also been described. No changes in the superior temporal gyrus (Deng et al., 2007), and the anterior cingulate cortex (Koethe et al., 2007) have been found as well as decreases in CB1R expression in the prefrontal cortex (Eggan et al., 2008). Unfortunately, most of the studies did not consider the pharmacological treatment given to patients, a confounding factor that may have altered the results achieved. Indeed, antipsychotics have been reported to decrease prefrontal cortex CB1R expression in schizophrenic patients in the absence of changes in drug-free schizophrenics (Uriguen et al., 2009).
In addition, genetic studies have indicated that variants within the CNR1 gene are directly associated with schizophrenia. Individuals with a 9-repeat allele of an AAT-repeat polymorphism of the CNR1 gene showed a 2.3-fold higher susceptibility to the hebephrenic form of schizophrenia in a Japanese population (Ujike and Morita, 2004), that was further confirmed in a population of the Central Valley of Costa Rica (Chavarria-Siles et al., 2008). However, such an association was no longer present if more general types of schizophrenia were considered. In addition, the presence of a polymorphism (G allele) of CNR1 has been associated with a better therapeutic effect of antipsychotics (Hamdani et al., 2008). Despite numerous data that support this association, negative data have also been found (Tsai et al., 2000; Seifert et al., 2007); therefore, the debate about the existence of a real relationship between CNR1 mutations and schizophrenia is still open and deserves further investigations.
Contribution of CB1Rs to schizophrenia has also been investigated in animal models that mimic some of the symptoms of the disease (Table 2). The schizophrenic-like effects induced by the administration of phencyclidine (PCP), a N-methyl-D-aspartate (NMDA) antagonist, in wild-type mice (e.g., increased locomotion, stereotyped behaviors, and decreased social interactions) were not observed in CB1KO mice. In contrast, PCP administration in CB1KO mice decreased locomotion, notably enhanced ataxia and stereotypy but induced no changes in social interaction. Since genetic CB1R blockade dramatically alters the behavioral consequences of PCP, this receptor may play a critical role in schizophrenia, although a differential participation in the negative (e.g., social disruption) and positive symptoms (e.g., stereotypy) of schizophrenia was hypothesized (Haller et al., 2005). Repeated PCP injections have been extensively used to induce enduring cognitive deficits with particular relevance to schizophrenia (consult Amitai et al., 2007; Grayson et al., 2007 as examples), and the participation of the eCB system in this PCP model of cognitive dysfunction have been analyzed (Vigano et al., 2009). Chronic-intermittent PCP administration induced an enhancement in CB1R density in the amygdala and in the ventral tegmental area when compared to the control group. Similarly, CB1R functionality was also altered in several brain areas implicated in schizophrenia; in particular, it was reduced in the prefrontal cortex, hippocampus, substantia nigra, and cerebellum, and increased in the globus pallidus. Alterations in endocannabinoid levels mainly in the prefrontal cortex, i.e., an increase in the levels of 2-AG in PCP-treated rats, were also found. These findings allowed authors to suggest that a maladaptation of the endocannabinoid system might contribute to the glutamatergic-related cognitive symptoms encountered in schizophrenia disorders (Vigano et al., 2009). Furthermore, chronic THC administration worsened cognitive performance in this animal model (Vigano et al., 2009), providing evidence for the hypothesis that cannabis consumption may be a risk factor for development or worsening of the schizophrenia disorder.
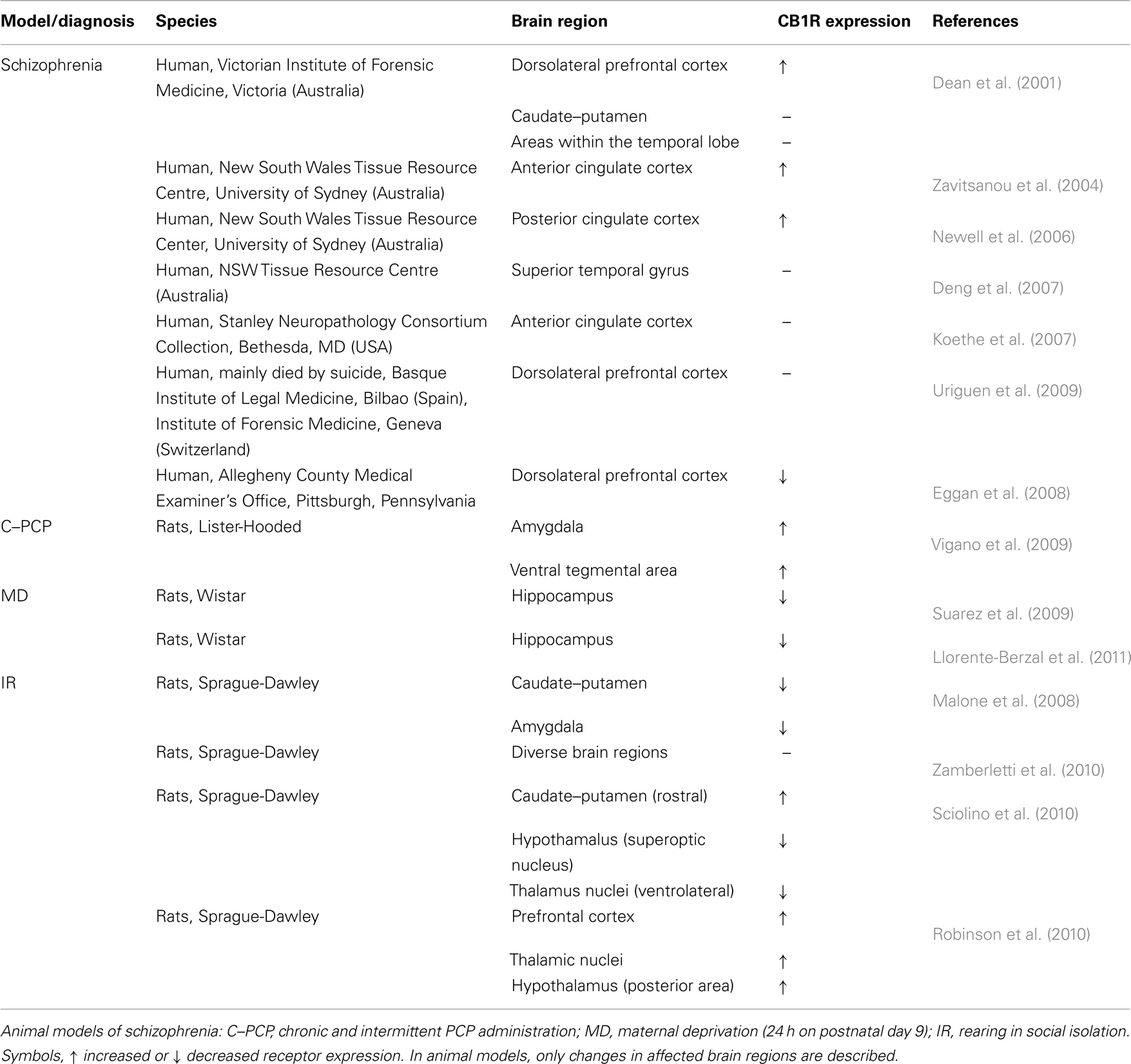
Table 2. Changes in CB1R expression in schizophrenia and animal models of neuropsychiatric disorders.
Increasing evidence gives support to the fact that schizophrenia is a subtle disorder of brain development and plasticity (Lewis and Levitt, 2002; Tyrka et al., 2008), thus reinforcing the neurodevelopment hypothesis of schizophrenia. Brain developmental abnormalities, often related to early traumatic experiences, have been extensively associated to schizophrenia (Lewis and Levitt, 2002; Tyrka et al., 2008). Therefore, changes in CB1R expression have been analyzed in schizophrenia animal models with a base in neurodevelopment, i.e., early maternal deprivation (Ellenbroek and Riva, 2003; Marco et al., 2009). At adulthood, maternally deprived animals (24 h at postnatal day 9) show behavioral abnormalities that resemble psychotic-like symptoms (Ellenbroek and Riva, 2003) including notable cognitive impairments (Llorente et al., 2011; Llorente-Berzal et al., 2011). Notably, a long-lasting decrease in CB1R expression has been found in maternally deprived animals within the hippocampus. Such a reduction in CB1R expression was observed in the short-term, at postnatal day 13, in both male and female rat pups (Suarez et al., 2009), as well as in the long-term, at adulthood (Llorente-Berzal et al., 2011), thus suggesting a endurable impact of early maternal deprivation in the eCB system that may contribute to some of the behavioral anomalies observed in these animals. Rearing rats in isolation has also been used as a model for the investigation of schizophrenia (consult Fone and Porkess, 2008 for review). Following isolation rearing, rats display social, and cognitive impairments. In particular, hyperlocomotion and increased aggressiveness have been reported, together with deficits in memory recognition and a reduce PPI response (Sciolino et al., 2010; Zamberletti et al., 2010). In relation to changes in the eCB system, raising rats in isolation led to a significant decrease in CB1R expression in caudate–putamen and amygdala (Malone et al., 2008). However, discrepant data have been achieved in more recent studies using this animal model of schizophrenia. Increases in CB1R expression has been described in the caudate–putamen of isolated-reared rats (Sciolino et al., 2010) as well as in prefrontal cortex, certain thalamic nuclei and the posterior area of the hypothalamus (Robinson et al., 2010). In contrast, decreases in receptor expression have been reported in the supraoptic nucleus of the hypothalamus and in the ventrolateral thalamic nucleus (Sciolino et al., 2010). In another study, in the absence of changes in CB1R expression, isolated-reared rats presented a consistent decrease in CB1R functionality in most of the regions analyzed, i.e., prefrontal cortex, nucleus accumbens, caudate–putamen, hippocampus, and ventral tegmental area (Zamberletti et al., 2010). Discrepancies may be due to the different techniques employed to evaluate CB1R density, to differences in the rat strains used, as well as in the duration of the isolation rearing protocol. Even though, taken together, these results indicate that the eCB system is altered in this animal model of schizophrenia, i.e., rearing in social isolation. In spite of the current discrepancies regarding CB1R changes in animal models of schizophrenia, present findings point to the eCB system as a pivotal neuromodulatory pathway that may have a critical relevance in the psychotic-related behaviors observed in these animals, i.e., altered emotionality and social and cognitive deficits. However, further research is needed to better understand the region-specific CB1R changes here described, and to establish a direct correlation between such changes and the behavioral anomalies reported.
There is now evidence demonstrating an association between increased rates of cannabis use and new cases of schizophrenia (see for review, Di Forti et al., 2007; Cohen et al., 2008; Leweke and Koethe, 2008). Epidemiological studies suggest a high incidence of schizophrenia within marijuana smokers (Moore et al., 2007) and long-term users of cannabis exhibit similar cognitive deficits to those seen in schizophrenia (Solowij and Michie, 2007). Cannabis has been considered a risk factor for development or worsening of the schizophrenia disorder, and evidence indicating that young people at genetic high risk for schizophrenia are particularly vulnerable to mental health problems associated with cannabis use is now available (Hollis et al., 2008). Moreover, cannabis use has been associated with a decrease in age of onset of schizophrenia, frequently related with a poorer outcome (Sugranyes et al., 2009). Literature from animal models further support adolescence as a highly vulnerable age for the consequences of cannabis exposure (Schneider, 2008). Adolescent chronic cannabinoid treatment leads to long-lasting behavioral deficits. Decreased emotionality (Biscaia et al., 2003; Wegener and Koch, 2009), although no changes in locomotor activity nor in object recognition memory have been reported (Schneider et al., 2005). Lasting disruption of pre-pulse inhibition (Schneider et al., 2005; Wegener and Koch, 2009) as well as persistent deficits in social recognition and impaired social interaction have been described following adolescent cannabinoid administration (Leweke and Schneider, 2011). Such behavioral anomalies were restored by antipsychotic treatment, further confirming the suitability of chronic pubertal cannabinoid administration as an animal model for diverse aspect of schizophrenia (Wegener and Koch, 2009; Leweke and Schneider, 2011). Despite more research is needed, reducing and/or limiting cannabis consumption in our society, especially among vulnerable populations (adolescents and people at risk for psychopathologies) might be convenient in order to reduce dependence and mental health risks in society.
A Role for CB2Rs in Neuropsychiatric Disorders
Despite CB2R was initially claimed as a peripheral cannabinoid receptor, its presence in CNS is still controversial [see A Brief Update on the Endocannabinoid (eCB) System]. As previously mentioned, CB2R has been detected in a diversity of brain regions including cerebral cortex, hippocampus, amygdala, hypothalamus, and cerebellum (Van Sickle et al., 2005; Gong et al., 2006; Onaivi, 2006; García-Gutiérrez et al., 2010), thus suggesting a role for CB2Rs in emotional and cognitive function.
CB2Rs in Mood Disorders: Anxiety and Depression
Recent results from mice with genetically modified CB2R suggest that CB2R-signaling is clearly involved in the regulation of emotional behavior (Table 3). Mice lacking CB2R (CB2R knock-out mice, CB2KO) presented increased vulnerability to stressful stimuli in the light–dark box, the elevated plus-maze, and the TST (Ortega-Alvaro et al., 2011). In contrast, transgenic mice over-expressing CB2R in the CNS (CB2xP; Racz et al., 2008a,b) presented a clear endophenotype resistant to stressful stimuli in the light–dark box and elevated plus-maze tests (Ortega-Alvaro et al., 2011). These results are not consistent with those reported by Onaivi et al. (2008b) showing that intracerebroventricular administration of an antisense oligonucleotide directed against CB2R mRNA resulted in anxiolytic-like effects in mice. Discrepancies between these two studies may be due to the fact that (1) the effects of intracerebroventricular administration of CB2 antisense oligonucleotide could act on different brain regions that may differ from those where CB2R are over-expressed in CB2xP mice, and (2) the different genetic background used in both studies (DBA/2, C57BL/6, BALB/c, and Swiss ICR). Similarly, CB2xP mice exhibited an endophenotype resistant to acute depressogenic-like stimuli (novelty-suppressed feeding test, NSFT, and TST) and CMS. Indeed, 6 weeks after CMS, CB2xP mice presented reduced passive coping behavior in the TST and did not experience anhedonia (García-Gutiérrez et al., 2010). The marked behavioral alterations occurring in CB2xP mice were associated with changes in BDNF, a well-known biochemical marker of depression (see also section “CB1RS in depression”). BDNF plays an important role in adult neurogenesis by modulating survival and plasticity of adult neurons and glia cells (Huang and Reichardt, 2001). As previously mentioned, depression has been associated to a decrease in hippocampal BDNF expression (Yu and Chen, 2010), probably related to the reported reduction in hippocampal neurogenesis previously described among patients with mood disorders (Sheline, 2000). In accordance, a similar decrease in hippocampal BDNF has been reported in animals exposed to CMS, probably indicating diminished hippocampal neurogenesis (Manji et al., 2001; Nestler et al., 2002). Interestingly, CMS failed to produce any modification in BDNF protein and gene expressions in the hippocampus of CB2xP mice (García-Gutiérrez et al., 2010). All these data support the role of CB2R in the normalization of reduced BDNF expression of mice exposed to CMS and suggests the involvement of CB2R in the regulation of mood disorders. In line with these findings, an association between cannabinoid CB2R polymorphism Q63R has been detected in Japanese depressed subjects (Onaivi et al., 2008a).
Taken together, these results allow us to hypothesize that the overexpression of CB2R decreases the vulnerability to depressogenic stimuli. The idea to induce CB2R overexpression by pharmacological manipulation was carried out by treating chronically wild-type mice with the cannabinoid CB2R antagonist, AM630. Indeed, 4 weeks of administration with AM630 increased CB2R gene expression (thus mimicking the phenotype of CB2xP mice), reversed the CMS-induced reduction of immobility evaluated in the TST, the diminished sucrose solution intake, and the diminished CB2R and BDNF gene and protein expression in the hippocampus (García-Gutiérrez et al., 2010). However, previous studies reported a lack of effects after the administration of AM630 on the intake of sucrose solution in CMS (Onaivi et al., 2008a). These discrepancies may be due to: (1) individual and species differences between the strains used and (2) different dosage or pattern of administration of AM630. Onaivi and colleagues used doses of 1 or 3 mg/kg once a day. In contrast, García-Gutiérrez et al. (2010) studied the effects of AM630 (1 mg/kg) administered twice a day.
In addition, the behavioral picture of CB2xP mice was paralleled with alterations in the HPA axis. Changes in the HPA axis have been associated with anxiety-related disorders in rodents and humans (see also previous section). The reduced secretion of HPA axis hormones was also detected in patients with stress-related disorders. Indeed, hypocortisolism was observed in patients with PTSD, fatigue syndrome, fibromyalgia, and other somatoform disorders (Heim et al., 2000). Restraint stress slightly increased pro-opiomelanocortin (POMC) gene expression (22%) in the arcuate nucleus of hypothalamus in CB2xP mice whereas failed to produce any modification in corticotropin releasing factor (CRF) gene expression of the paraventricular nucleus (García-Gutiérrez and Manzanares, 2011). These results suggest that CB2R may be contributing to the maintenance of the steady state control of the HPA axis. The fact that the overexpression of CB2R blocked the effects of stress on CRF gene expression points to the idea that the mechanism controlling the HPA axis in CB2xP mice may be acting at the level of synthesis or release of CRF. The overexpression of CB2R was also accompanied by changes in the GABAergic system, more particularly by alterations of GABA-A receptor subunits. Benzodiazepines are anxiolytic drugs often used in the treatment of certain anxiety or mood related disorders. Benzodiazepines, acting through their binding on the interface of α and γ subunits of the GABA-A receptor complex are known to act as anxiolytics promoting the inhibitory actions of the GABA neurotransmitter in the CNS (Da Settimo et al., 2007). Recent studies suggested that GABA-A receptors containing α2 and γ2, enriched in corticolimbic structures mediate the anxiolytic effect of benzodiazepines (Low et al., 2000). The administration of alprazolam, a well-known anxiolytic benzodiazepine, failed to produce any effect in CB2xP mice at either of the doses used (45 and 70 μg/kg; García-Gutiérrez and Manzanares, 2011). This behavioral scenario was associated to changes in the expression of α2 and γ2 subunits of GABA-A receptors in specific brain areas. Increased GABA-A α2 and γ2 subunits receptor gene expression was found in the amygdala and hippocampus of CB2xP mice (García-Gutiérrez and Manzanares, 2011). The increased gene expression of both GABAergic subunits may be related, at least in part, with the lack of the anxiolytic effect of benzodiazepines in CB2xP mice. Moreover, these results support the potential implication of CB2R in the regulation of GABAergic system. In this respect, recent studies revealed a suppression of GABAergic inhibitory signaling in the entorhinal cortex–hippocampal slices following the administration of the cannabinoid CB2R agonist, JWH133 (50 nM). Interestingly, these effects could be blocked by prior administration of AM630 (50 nM) supporting the involvement of CB2R in the effects of JWH133 on GABAergic signaling (Morgan et al., 2009). These results further strengthen the involvement of CB2R in the regulation of GABAergic release from neuronal terminals.
Pharmacological manipulation of CB2R may alter the response to anxiogenic or depressogenic-like stimuli (see Table 3 for details). In rodents, the pharmacological manipulation of CB2R by the administration of agonists or antagonists resulted in controversial reported effects on emotional behavior. Acute administration of GW405833 (100 mg/kg), a CB2R agonist, induced anxiolytic effects in the marble burying test (Valenzano et al., 2005). Indeed, an anxiolytic effect in the elevated plus-maze test was reported after the acute administration of the CB2R agonist, JWH015 (Onaivi, 2006). In contrast, the same group reported anxiogenic effects of JWH015 in the light–dark box (Onaivi et al., 2006). The present discrepancies may be due to: (1) the drugs used, (2) the route of drug administration, (3) the doses used, and (4) the strain of mice studied. Indeed, the fact that the doses of the CB2R agonist used resulted in motor alterations may have masked the interpretation of these behavioral effects. A recent publication evaluated the effects of acute and chronic administration of JWH133, a CB2R agonist, and AM630, a CB2R antagonist, on emotional behavior at doses that did not modify motor activity. Acute administration of JWH133 failed to produce any modification in the response to acute stimuli in the light–dark test. In contrast, the acute blockade of CB2R by AM630 resulted in anxiogenic effects. The fact that the anxiogenic effects of AM630 were blocked by the previous administration of JWH133 supported the involvement of CB2R in the acute effects of AM630 on emotional behavior (García-Gutiérrez et al., 2011). However, chronic blockade of CB2R by the antagonist AM630 resulted in anxiolytic effect associated with increased CB2R gene and reduced CB2R protein expression in cortex and amygdala. In contrast, chronic activation of CB2Rs by JWH133 resulted in an opposite behavioral and molecular alterations. Mice chronically treated with JWH133 presented an anxiogenic effect associated with reduced CB2R gene and increased CB2R protein expression in cortex and amygdala. Indeed, the administration of JWH133 and AM630 are associated with alterations in GABAergic system. Chronic blockade of CB2R by AM630 increased GABA-Aα2 and GABA-Aγ2 gene expressions in the cortex and amygdala. In contrast, the protein expression of these genes was reduced by chronic treatment with AM630 in the brain regions mentioned. Interestingly, activation of CB2R by JWH133 reduced the GABA-Aα2 and GABA-Aγ2 gene expression and increased its protein expression in the cortex and amygdala. The opposite behavioral and molecular changes observed between chronic CB2R blockade (AM630) or activation (JWH133) gives support to the key role of these targets (CB2R, GABA-Aα2, and GABA-Aγ2) in the behavioral effects of AM630 or JWH133. These results provide new insights into the different molecular events related to GABA-A receptor gene and protein expression produced by chronic manipulation of CB2R with CB2R agonism or antagonism.
In summary, the data presented here provide evidence for the interesting putative role of CB2R in anxiety and depressive-like disorders and as a possible target for the development of a novel class of anxiolytic or antidepressant drug. In this respect, the administration of AM630 clearly decreased the anxious state of DBA/2 mice (García-Gutiérrez et al., 2011). Further pharmacological studies are necessary to explore the potential therapeutic uses of cannabinoid CB2R in humans and the precise mechanisms underlying these effects.
CB2Rs in Schizophrenia
Different evidences support the involvement of CB2R in schizophrenia disorders. Clinical remission of schizophrenia has been reported to be accompanied by significant decreases in AEA and CB2R mRNA levels in peripheral blood mononuclear cells (Giuffrida et al., 2004). In addition, a recent publication revealed a close relation between diminished CB2R function (polymorphism Q63R) and increased susceptibility to schizophrenia in the presence of other risk factors (Ishiguro et al., 2010b).
The use of genetically modified mice led to further investigate the potential role of CB2R in schizophrenic-related disorders. Similarly to the alterations observed in schizophrenic patients (Braff et al., 2001; Iyer et al., 2008; Peralta et al., 2010), the lack of CB2Rs resulted in alterations of motor activity, anxiety and depressive disorders, and cognitive deficits including impaired sensoriomotor gating (Ortega-Alvaro et al., 2011). CB2KO mice exhibited decreased spontaneous motor activity and increased sensitivity to the motor stimulant effects of acute cocaine administration in the open field test. Indeed, as mentioned before, CB2KO mice presented increased vulnerability to anxiogenic (light–dark box and elevated plus-maze) and depressogenic-like stimuli (TST). Furthermore, CB2KO mice showed disrupted short- and long-term memory consolidation in the step down inhibitory avoidance paradigm. Indeed, CB2KO mice presented a significantly reduced pre-pulse inhibition of the acoustic startle response (PPI), alteration observed in rodent models of schizophrenia and schizophrenic patients (Braff et al., 2001). Interestingly, the PPI deficit observed in CB2KO mice was markedly enhanced after chronic oral treatment with risperidone, an antipsychotic drug (see Table 3 for details).
It is known that schizophrenia is associated with brain abnormalities induced during the development of the CNS (Rapoport et al., 2005; Ross et al., 2006). A number of findings suggest a pro-neurogenic role of CB2R in the control of fundamental neural cell processes (Harkany et al., 2007; Galve-Roperh et al., 2008; Katona and Freund, 2008). Therefore, it can be hypothesized that the lack of CB2R might impair neural development, thus inducing relevant alterations in several brain areas. In this respect, CB2KO mice presented increased dopamine D2 receptor (D2R) and adrenergic α2C receptor (α2CR) gene expression in prefrontal cortex and locus coeruleus, and decreased serotoninergic 5-HT2C (5-HT2CR) and 5-HT2A receptors (5-HT2AR) gene expression in the dorsal raphe and the prefrontal cortex, respectively. Interestingly, risperidone treatment to CB2KO mice induced a reduction in the gene expression of D2R, 5-HT2CR, and 5-HT2AR in the prefrontal cortex, and α2CR in the locus coeruleus; but increased 5-HT2CR and 5-HT2AR gene expression in the dorsal raphe. Despite additional targets may be involved in the behavioral alterations observed in CB2KO mice, the fact that the risperidone tended to “normalize” the molecular alterations observed in these mice supports the involvement of dopaminergic, serotoninergic, and adrenergic alterations in the PPI deficit of CB2KO mice (Ortega-Alvaro et al., 2011). These results suggest that CB2R deletion was related to the observed schizophrenia-like behaviors. Pharmacological manipulation of CB2R may be further explored as a potential therapeutic target for the treatment of schizophrenia-related disorders.
A Distinctive Role of Cannabidiol (CBD) in Neuropsychiatric Disorders
Cannabidiol (CBD) is the main non-psychotropic phytocannabinoid found in the C. sativa plant, constituting up to 40% of its extract. Recent comprehensive reviews indicate that CBD is one of the most promising candidates for therapeutic use in a wide range of disorders, including neuropsychiatric (Mechoulam et al., 2007; Zuardi, 2008; Izzo et al., 2009). As discussed bellow, part of its effects seems to depend on facilitation of eCB-mediated neurotransmission.
CBD in Anxiety and Depression
Initial studies in laboratory animals produced contradictory results. Whereas Zuardi and Karniol (1983) showed that low doses (10 mg/kg) of CBD attenuated conditioned emotional responses in rats, Silveira Filho and Tufik (1981) failed to find any effect of a much higher dose (100 mg/kg) in a conflict paradigm. These apparently opposite results were subsequently explained by Guimarães et al. (1990), who verified that CBD causes an inverted U-shaped dose-related anxiolytic response curve in the elevated plus-maze, with the anxiolytic doses ranging from 2.5 to 20 mg/kg. Subsequent studies employing diverse animal models, including the elevated plus-maze, Vogel conflict test, contextual fear conditioning, marble burying test, and attenuation of stress responses, confirmed that systemically injected CBD decreases anxiety-like behaviors in rodents (Onaivi et al., 1990; Guimarães et al., 1994; Moreira et al., 2006; Resstel et al., 2006; Casarotto et al., 2010).
More recently the brain sites responsible of these effects have been investigated by direct brain administration of CBD. The drug caused anxiolytic-like effects after microinjection into the dorsolateral periaqueductal gray (dlPAG), bed nucleus of the stria terminalis (BNST), and prelimbic medial prefrontal cortex (Campos and Guimarães, 2008; Moreira et al., 2009; Alves et al., 2010; Lemos et al., 2010; Soares et al., 2010; Gomes et al., 2011). It also facilitated extinction in a contextual aversive conditioning model after intracerebral ventricular administration (Bitencourt et al., 2008). Interestingly, in the infralimbic prefrontal cortex CBD induced an anxiogenic effect, facilitating conditioned emotional responses (Lemos et al., 2010). Moreover, no consistent effect was found in the amygdala (Lisboa and Guimarães, unpublished results). Taken together, these results indicate that the anxiolytic effects of CBD depend on drug action in specific brain areas related to defensive responses.
Clinical studies have confirmed that CBD possess anxiolytic properties. In addition to prevent the anxiogenic effects of high doses of THC (Zuardi et al., 1982), CBD was able to decrease anxiety in healthy subjects submitted to a simulated public speaking paradigm (Zuardi et al., 1993a). Using a similar paradigm, Bergamaschi et al. (2011) have recently shown that the drug reduces public speaking anxiety in treatment-naïve social phobic patients. In agreement with these findings, neuroimaging studies show that CBD can change brain activity in regions related to emotional responses. It impairs connectivity between the prefrontal and subcortical regions (Fusar-Poli et al., 2010), attenuates blood oxygenation level-dependent responses to fearful faces in the amygdala and cingulate cortex (Fusar-Poli et al., 2009) and decreases activation in the left amygdala–hippocampal complex and left posterior cingulate gyrus (Crippa et al., 2004). In relation to depressive-related disorders, CBD has been reported to prevent the physiological and delayed anxiogenic consequences of restraint stress in animal models (Resstel et al., 2009), an effect that has been related to depressive responses (Guimarães et al., 1993). More recently, CBD was shown, similarly to the prototype antidepressant imipramine, to decrease immobility time in the forced swimming test (Zanelati et al., 2010). Although more studies are clearly needed, these initial results suggest that CBD does possess antidepressive properties.
CBD and Psychosis/schizophrenia
Several studies performed in laboratory animals during the 1970s indicated that CBD could interact with the main cannabinoid present in the C. sativa plant, delta-9-tetrahydrocannabinol (THC, for review see Zuardi et al., 2006a; Zuardi, 2008). In a seminal study published in 1982, Zuardi et al. (1982) observed that CBD could block the psychotomimetic and anxiogenic effects of THC in healthy volunteers. This observation led to the hypothesis that CBD could have antipsychotic and/or anxiolytic properties. The former proposal was supported by preclinical results indicating that the drug is able to reduce the stereotypies and hyperlocomotion caused by apomorphine and amphetamine, respectively, without causing catalepsy or increasing prolactin levels (Zuardi et al., 1991, 1993b; Moreira and Guimarães, 2005). This “atypical” antipsychotic profile was further confirmed in a c-Fos study where CBD, similarly to clozapine, increased c-Fos expression in limbic areas and prefrontal cortex, but not in the dorsal striatum (Guimarães et al., 2004).
In addition to dopamine-based models predictive of antipsychotic activity, CBD effects have also been tested in glutamate-based models. CBD blocked the hyperlocomotion induced by ketamine (Moreira and Guimarães, 2005) as well as the disruption of pre-pulse inhibition induced by MK-801 (Long et al., 2006). CBD was also able to restore the deficit in social interactions induced by this drug (Gururajan et al., 2011).
The possible antipsychotic profile of CBD indicated by these preclinical results is further supported by clinical studies (for review see Zuardi, 2008). In agreement with the initial report by Zuardi et al. (1982), showing that CBD is able to antagonize the psychotomimetic effects of THC in healthy volunteers, the presence of this compound in Cannabis strains seems to be protective against the occurrence of psychotic reactions (Morgan and Curran, 2008) as well as Cannabis-associated decrease in hippocampal volume (Demirakca et al., 2011). CBD is also able to attenuate psychosis symptoms induced by ketamine or L-DOPA in healthy volunteers and Parkinson’s patients, respectively (Zuardi et al., 2009). In accordance with these results, preliminary studies in schizophrenic patients showed positive therapeutic effects of CBD (Zuardi et al., 1995, 2006b).
CBD Mechanisms and the ECB System
Results of experiments aimed at elucidating CBD mechanisms are usually complicated by the common bell-shaped dose–response curves produced by this drug (Campos and Guimarães, 2008; Izzo et al., 2009). Even so, it is now clear that multiple pharmacological actions are involved in the wide range biological effects induced by CBD (Izzo et al., 2009). These actions include complex interactions with the eCB system. CBD was initially described as possessing little affinity for cannabinoid receptors (Petitet et al., 1998; Thomas et al., 1998). A more recent study, nonetheless, has suggested that CBD can antagonize CB1Rs and CB2Rs at relatively low concentrations (Thomas et al., 2007). This antagonism, however, seems to be non-competitive in nature, with evidence suggesting that CBD could act as a CB1R or CB2R inverse agonist (Pertwee, 2008). Contrasting with these findings, CBD could also facilitate eCB-mediated neurotransmission by decreasing AEA hydrolysis or reuptake (Bisogno et al., 2001).
The findings regarding eCBs involvement in CBD effects, however, have been mainly obtained in studies performed in vitro and little is known about the involvement of these mechanisms in the central effects of the drug. To investigate this issue, we initially tested if facilitation of eCB-mediated neurotransmission could explain the anxiolytic effects of CBD in the dlPAG. This hypothesis was based on our previous study showing that direct injection of AEA into this region induces anxiolytic-like effects that were prevented by prior administration of AM251, a CB1R antagonist (Moreira et al., 2007). Surprisingly, the same dose of AM251 that had antagonized AEA failed to prevent CBD anxiolytic effects in the dlPAG (Campos and Guimarães, 2008). Considering that CBD could also act, at μM concentration range, as an agonist of 5-HT1ARs in vitro (Russo et al., 2005) and in vivo (Mishima et al., 2005), we decided to test if CBD effects would be prevented by local pre-treatment with WAY100635, a selective 5-HT1AR antagonist. Supporting this hypothesis, this drug completely blocked the anxiolytic effects of CBD (Campos and Guimarães, 2008). Following this initial result, we have now confirmed that WAY100635 is able to prevent CBD anxiolytic effects after intracerebral injections into the BNST (Gomes et al., 2011) or dlPAG (Soares et al., 2010) as well as following CBD systemic administration (Resstel et al., 2009). 5-HT1A mechanisms are also responsible for the antidepressive-like effects of CBD in the forced swimming test (Zanelati et al., 2010). Despite these findings, which clearly related 5-HT1A-mediated neurotransmission with CBD anxiolytic and antidepressive effects, more recent results showed that the eCB system is also involved in at least some of the central effects of CBD. For example, the facilitatory effect of intracerebroventricular (i.c.v.) administration of CBD in contextual fear-conditioning extinction was prevented by Rimonabant (Bitencourt et al., 2008). Also, the plastic effects of repeated CBD administration seem to involve eCB mechanisms. For example, chronic CBD treatment was shown to increase adult neurogenesis in the dentate gyrus, an effect that was absent in CB1KO mice (Wolf et al., 2010). In line with these results, the in vitro proliferative effects of CBD on embryonic hippocampal cells were prevented by CB1 or CB2 receptor antagonists (Campos et al., 2010).
We have also recently found that a CB1R antagonist, but not a 5-HT1AR, is able to prevent the effects of CBD in the marble burying test (Casarotto et al., 2010). Although initially proposed as an animal model aimed at detecting possible anxiolytic drug effects, it is now thought to evaluate a natural, repetitive behavior that can become compulsive. This test, therefore, has been proposed to model aspects of obsessive–compulsive disorder (OCD; Thomas et al., 2009). CBD effects in this model, depending on CB1R rather than on 5-HT1AR, gives support to the interpretation that the marble burying test (and OCD) engages brain mechanisms somehow different from those of related to classical animal models of anxiety (Witkin, 2008).
Both CBD and AEA can activate TRPV1Rs (Bisogno et al., 2001). These receptors are expressed in several brain areas related to anxiety such as the amygdala, hippocampus, prefrontal cortex, and PAG (Cristino et al., 2006). Activation of TRPV1 receptors can facilitate glutamate release (Marsch et al., 2007; Xing and Li, 2007), the main excitatory neurotransmitter in the CNS. Since antagonism of glutamate and TRPV1Rs in the dlPAG induce anxiolytic-like effects (Aguiar and Guimarães, 2009; Terzian et al., 2009), we hypothesized that at higher doses CBD could also be activating local TRPV1Rs (directly and maybe by inhibiting AEA metabolism/uptake), facilitating glutamate neurotransmission and increasing anxiety. Corroborating this proposal, we found that a low dose of capsazepine, a TRPV1R antagonist, was able to turn the higher but ineffective dose of CBD into an anxiolytic one (Campos and Guimarães, 2009). Interaction with TRPV1Rs has also been suggested to explain the antipsychotic-like effects of CBD on MK-801 induced disruption of PPI (Long et al., 2006). Other mechanisms have also been proposed to account for the effects of CBD, for example blockade of adenosine uptake (Carrier et al., 2006) and antagonism of the putative cannabinoid receptor GPR55 (Mechoulam et al., 2007). Although the former has been related to CBD anxiolytic properties in a preliminary study (Carrier et al., 2007), the involvement of these mechanisms in the central effects of this drug remains to be further investigated. In summary, preclinical and clinical studies indicate that CBD has therapeutic potential in several neuropsychiatric disorders that depend on multiple mechanisms, including interaction with the eCB system. In addition, considering its safety profile (Mechoulam et al., 2007; Zuardi, 2008; Izzo et al., 2009), CBD could be a useful pharmacological tool to modulate this system.
The eCB System and Eating Disorders: Toward a New Therapeutically Valid Approach?
Pathophysiology of Eating Disorders
Food intake or eating is the process by which edible substances are consumed in order to balance the energy expenditure in living creatures. This process relies in physiologic mechanisms regulating appetite and the natural drive to eat. In some conditions human feeding behavior is altered leading to diseases, collectively known as eating disorders. These are a group of disorders characterized by physiological and psychological disturbances in appetite or food intake. They can be divided into three main pathologies, i.e., binge eating, bulimia nervosa (BN), and anorexia nervosa (AN). Binge-eating disorder is associated with three or more of the following: eating until feeling uncomfortably full; eating large amounts of food when not physically hungry; eating much more rapidly than normal; eating alone due to embarrassment; feeling of disgust, depression, or guilt after overeating. Criteria includes occurrence on average, at least 2 days a week for 6 months. Binge eating is not associated with compensatory behavior (i.e., purging, excessive exercise, etc.) and does not co-occur exclusively with BN or AN (From DSM-IV, 1994). BN is characterized by a cycle of binge-eating followed by purging to avert weight gain. Purging methods often include self-induced vomiting, use of laxatives or diuretics, excessive exercise, and fasting. AN is characterized by the loss of appetite and is associated with other features including an excessive fear of becoming overweight, body image disturbances, significant weight loss, refusal to maintain minimal normal weight, excessive exercise, and amenorrhea (Walker, 1994). In this review we will not include obesity because actually it is not formally considered an eating disorder. However, we would like to underline the increasing evidence dealing with specific changes in the CNS of obese people, including those occurring in brain areas involved in the rewarding aspects of food (reviewed in (Volkow and Wise, 2005). Likewise, and maybe reflecting direct central consequences of obesity, it is noteworthy the high incidence of anxiety and depression (also present in classical eating disorders) in obese people, affecting around 50% of this population. Also deserving greater consideration are the striking similarities in the pathophysiologic sequel occurring with obesity and addiction, also suggesting a re-evaluation of how these diseases are classified (Volkow and Wise, 2005).
Eating disorders can be chronic and disabling conditions characterized by aberrant patterns of feeding behavior and weight regulation, including abnormal attitudes and perceptions toward body weight and shape (Kaye, 2008). Indeed, AN has the highest mortality rate among neuropsychiatric diseases (Lowe et al., 2001). The etiologies of these diseases are at present poorly understood, but both AN and BN occur most frequently in adolescent females. This increased incidence and prevalence may very well be a direct reflection of cultural pressures for thinness (Strober et al., 1995). However, the discrete occurrence and heritability suggest there are some biological vulnerabilities involved in these diseases (Kaye, 2008). In fact, twin studies on AN and BN suggest there is a 50–80% genetic contribution to these diseases (Bulik et al., 1998; Klump et al., 2001). However, there is little knowledge about the connection between psychological symptoms and the neuropathophysiology associated with these diseases and on how such genetic vulnerabilities impact on brain pathways and what systems are primarily involved. Because of the neuropsychiatric nature of these diseases, the monoamine systems (i.e., serotonin, dopamine, and norepinephrine pathways) have been explored in greater detail. Among these, the serotoninergic system may be the more adversely affected and its deregulation is present in AN patients. However, the response to selective serotonin reuptake inhibitors is variable among patients suffering different subtypes of the illness, and the efficacy of such medication has been also questioned due to the common occurrence of relapse (Kaye et al., 2001; Walsh et al., 2006). Current research on eating disorders also points to a deregulation of neuronal circuits involved in food intake, including those related to emotional and reward pathways linked to feeding behavior (Stoving et al., 2009). In particular, a deranged leptin signaling system has been found in AN and BN (Monteleone et al., 2004; Holtkamp et al., 2006) and it has been hypothesized that the reward systems could be compromised leading to food intake-related dysphoria that would promote a vicious cycle of decreasing eating in order to avoid the dysphoric consequences of food consumption (Kaye, 2008). In this context, the reward system could have an important role since it integrates “liking” (pleasure/palatability) and “wanting” (appetite/incentive motivation) perceptions associated with food and, thus, AN and BN could be considered as dependency syndromes.
The Role of the ECB System in Energy Homeostasis
The eCB system is strategically located in all the key points involved in food intake and energy expenditure, both at the central and the peripheral level. Thus, it is perhaps one of the few that can coordinate all the players involved in energy balance (reviewed in Pagotto et al., 2006; Matias and Di Marzo, 2007). Together with its action on peripheral tissues, the eCB system influences feeding behavior at the CNS by acting on circuits located in the hypothalamus, the reward system and the brain stem, with the overall net effect being anabolic (reviewed in Di Marzo et al., 2009).
Briefly, the hypothalamus is a key brain structure involved in energy balance homeostasis. Despite the low expression of CB1R in the hypothalamus, a number of studies demonstrate that eCBs through CB1Rs exerts a profound influence on the hypothalamic regulation of food intake (reviewed in Bermudez-Silva et al., 2011). CB1Rs are also important in the hypothalamic leptin-mediated anorectic effects (Di Marzo et al., 2001). Leptin inhibits eCB production in the hypothalamus and, conversely, hypothalamic eCBs are increased in genetically obese rodents lacking leptin or its receptor (Di Marzo et al., 2001). The reward system is a group of brain structures which regulate and control behavior by inducing pleasurable effects. The major rewarding pathway in the brain is the mesolimbic pathway that goes from the ventral tegmental area via the medial forebrain bundle to nucleus accumbens, which is the primary release site for the main brain’s pleasure chemical, i.e., the neurotransmitter dopamine. CB1Rs are expressed in presynaptic glutamatergic and GABAergic nerve terminals in the ventral tegmental area, and eCBs are synthesized by ventral tegmental area dopamine neurons, having a role in the fine-tuned regulation of these cells (Maldonado et al., 2006). While it is still unclear exactly what cell populations express CB1Rs in the nucleus accumbens, it seems that eCBs within this area are able of increasing food intake in a CB1-dependent manner (Kirkham et al., 2002). Additional studies have also reported that eCBs acting in the nucleus accumbens modulate the palatability of food (Mahler et al., 2007). The brainstem is also a relevant player in food intake regulation: satiety signals from the stomach and duodenum reach the brainstem through sensory and vagal fibers. Among these, cholecystokinin (CCK) and peptide YY have been related with the eCB system (reviewed in Di Marzo et al., 2009; Bermudez-Silva et al., 2010). CB1Rs are expressed in the brainstem and in vagal afferent neurons modulating these signals (Burdyga et al., 2004; DiPatrizio and Simansky, 2008). Furthermore, eCB tone changes in the brainstem during the different phases of eating (reviewed in Di Marzo et al., 2009).
The ECB System in Eating Disorders
The widespread role of the eCB system in regulating energy balance has spawned investigations into putative defects in eCB signaling that may underlie eating disorders. Increased blood levels of AEA have been found in both AN and binge-eating disorder patients, but not in BN patients (Monteleone et al., 2005). Indeed, AEA levels were significantly and inversely correlated with plasma leptin concentrations in both healthy controls and anorexic women. Interestingly, there is evidence to suggest that hypoleptinemia in AN patients may be an important factor underlying the excessive physical activity (Holtkamp et al., 2006), one of the hallmarks in AN. Thus, these results suggest that alterations in the eCB system associated with deregulated leptin signaling could be involved in the pathophysiology of AN. It is well-known that the eCB system and leptin interact functionally at the molecular level (reviewed in Bermudez-Silva et al., 2011), and thus it is easy to draw a theoretical frame in support of the important role played by both systems in AN and the therapeutic potential of leptin and cannabinoids in this disease (Stoving et al., 2009). Furthermore, elevated levels of CB1R but not CB2R mRNA have been found in the blood of females with AN and BN, further supporting the hypothesis of deregulated eCB signaling in eating disorders (Frieling et al., 2009). Paradoxically, these authors found an association between lower CB1R expression and more severe forms of the disorders.
AEA belongs to the lipid family of acylethanolamides. Another member of this group of lipids, named oleoylethanolamide, has also an important role on energy balance by promoting satiety and lipolysis through the activation of the PPARα (Fu et al., 2003). This molecule has an anorexigenic action by inducing oxytocin expression in the paraventricular nucleus of the hypothalamus and, interestingly, preliminary clinical results have shown altered levels of oleoylethanolamide in the cerebrospinal fluid and plasma of subjects recovered from eating disorders (Gaetani et al., 2008). These preliminary observations could extend the findings of altered levels of eCBs in eating disorders to a more general involvement of acylethanolamides.
Given the important contribution of genetics to AN and BN (in fact, the heritability estimates are similar to disorders typically viewed as biological like schizophrenia and bipolar disorder) human genetic association studies have been performed in order to identify genes involved in these pathologies, including genes belonging to the eCB system. Among these, CNR1 and CNR2 (the genes encoding cannabinoid CB1Rs and CB2Rs, respectively), as well as the genes encoding the main enzyme responsible in the degradation of AEA (FAAH), NAAA (N-Acylethanolamine-hydrolyzing acid amidase, which functions similar to FAAH but has a different optimal pH), and MAGL have been studied. The first family based study involved 52 families (parents with one or two affected siblings) that were genotyped for the (AAT) trinucleotide repeat of CNR1 gene. The distribution of alleles transmitted to the patients was not found to be significantly different from the non-transmitted parental alleles. However, upon dividing the samples to restricting and binging/purging subtypes of AN, the data analysis revealed a preferential transmission of different alleles in each of the subtypes, suggesting restricting AN and binging/purging AN may be associated with different alleles of the CNR1 gene (Siegfried et al., 2004). However, a subsequent study involving up to 91 German AN trios (patient with AN and both biological parents) was unable to confirm these results, nor did it show an association for any of 15 single nucleotide polymorphisms representative of regions with restricted haplotype diversity in FAAH, NAAA, and MAGL genes (Muller et al., 2008). Another study in 115 overweight/obese subjects with binge-eating disorder, 74 non-binge-eating disorder patients with obesity and 110 normal weight healthy controls investigated one of these FAAH polymorphisms, previously implicated in obesity in binge-eating disorder, and reporting a lack of association (Monteleone et al., 2008) and in a more recent article these authors studied the association of this FAAH polymorphism and the CNR1 polymorphism in both AN and BN, in 134 patients with AN, 180 patients with BN and 148 normal weight healthy controls (Monteleone et al., 2009). The authors found a significant increase in the frequency of both polymorphisms in AN and BN patients, a result in sharp contrast with the previous findings by Muller et al. (2008) that showed a lack of association of these polymorphisms with AN. Additionally, Monteleone et al. (2009) found a synergistic effect of the two polymorphisms in AN but not in BN. Finally, a recent article has detected an association of a CNR2 polymorphism with both AN and BN (Ishiguro et al., 2010a) in a study comprising in 204 subjects with eating disorders and 1876 healthy volunteers in Japanese population. Taken together, the human genetic association studies show evidence of association between eCB system genes and eating disorders, but further studies are necessary to definitively confirm these findings.
Therapeutic Use of Cannabinoid Drugs in Eating Disorders
Cannabis preparations have been used for both medicinal and recreational purposes for centuries. Its ancient medicinal use has been primarily related to ameliorate pain and increase appetite in disease states. However, because of their psychostimulant properties and the lack of an adequate body of knowledge, their use in western medicine has been excluded until recently. During the last 20 years this picture has dramatically changed. There has been an exponential increase in the knowledge of the molecular mechanisms underlying cannabinoid effects, and morphological, physiological and pathophysiological studies have shown that the molecular system supporting these effects (i.e., the eCB system), is ubiquitous and has a highly relevant role in maintaining whole body homeostasis and, especially, energy homeostasis (Matias and Di Marzo, 2007). This fact has led to an increased interest in the medical use of cannabinoid-related drugs. Thus, in 1985 the Food and Drug Administration approved Marinol® (dronabinol), a synthetically derived THC preparation, to relieve nausea, and vomiting associated with chemotherapy in cancer patients who have failed to respond adequately to other antiemetics, and in 1992 this compound was also approved for inducing appetite in AIDS patients suffering from cachexia (Nelson et al., 1994; Beal et al., 1995). Similarly, Nabilone® (a synthetic cannabinoid that mimics THC) was also approved in 1985 for ameliorating the nausea of cancer chemotherapy. A more controversial step forward was the use of a cannabinoid CB1R antagonist/inverse agonist (rimonabant) for management of complicated obesity. Although the Food and Drug Administration never approved this drug, the European Medicine Agency did and Acomplia® (the commercial name of rimonabant) was in the market for approximately 2 years. Despite the weight loss and improved cardiometabolic profile observed in obese patients, the drug had to be removed from the market due to its undesirable central side effects (see previous sections, but also Bermudez-Silva et al., 2010 for review). More recently, Sativex® (the combination of THC and CBD) has been marketed in Canada and European countries like the United Kingdom and Spain for the treatment of spasticity due to multiple sclerosis, and it is currently in phase III clinical development for the treatment of cancer pain.
Taken into account the good therapeutic management of cannabinoids in cachexia and malnutrition associated with cancer and AIDS, it looks feasible that this kind of pharmacotherapy could be also useful in the treatment of eating disorders. Unfortunately, there are only two small trials assessing cannabinoid treatment in AN (reviewed in Stoving et al., 2009). The former involved 11 AN patients in a 4-week crossover trial and THC treatment resulted in increased sleep disturbances and interpersonal sensitivity, whereas there was no significant effect on weight gain (Gross et al., 1983). Unfortunately, this study raised several concerns given it was an in-patient study and the occasional tube feeding was used. In addition, THC was compared to diazepam instead of placebo, which could be a confounding factor given diazepam has also been reported to increase food intake per se (Naruse et al., 1991). The latter involved nine AN out-patients treated with THC. The results showed a significant improvement of depression and perfectionism scores without improving weight gain (Berry, 2006).
Currently, there is an ongoing phase III clinical trial involving 22 subjects to reveal if severe chronic AN patients treated with Marinol® have significant improvement on weight, with secondary objectives of the study being evaluation of eating disorder inventory scale, motor and inner restlessness and endocrine parameters3 (EudraCT Number: 2007-005631-29). With this very limited number of performed trials (the last one being still not finished) it seems clear that no conclusions can be drawn out regarding the therapeutic validity of a cannabinoid-based approach in eating disorders. However, the satisfactory clinical use of cannabinoid agonists in other pathologies demands and encourages the development of further clinical trials on eating disorders patients. Interestingly, a very recent preclinical study in rodent have shown that the main active constituent of cannabis, THC, is able of reducing the weight loss associated with the development of AN via a mechanism involving reduced energy expenditure (Verty et al., 2011), thus providing encouraging preclinical data on the validity of a eCB-based therapy in AN.
Concluding Remarks
Evidence for a critical role of the eCB system in neuropsychiatric disorders has been provided, and special attention has been paid to its contribution to the emotional and cognitive deficits compromised in these disorders. Not only CB1Rs, but also CB2Rs and CBD, through facilitation of eCB-mediated neurotransmission, have been involved in the emotional and cognitive deficits reported in anxiety disorders, depression, and schizophrenia. Indeed, a deregulation of the eCB system seems to be in the bases of several neuropsychiatric disorders, including eating disorders. The pharmacological enhancement of eCB signaling has yield promising results in rodents, particularly as an anxiolytic and antidepressant therapy. Eating disorders may also benefit of this therapeutic approach, and a clinical trial with synthetic THC is ongoing for the management of severe AN. However, in spite of these potential benefits, further research is needed to prevent undesirable side effects. In fact, the prolonged and continuous activation of the eCB system, e.g., by chronic cannabis consumption, has been associated with an increased risk for schizophrenia. Alternatively, CBD may arise as an optimal candidate to modulate the eCB system. CBD has consistently demonstrated an anti-anxiety and antidepressant profile, and its potential as an antipsychotic drug is gaining relevance in preclinical and clinical studies. In conclusion, the eCB system is seriously involved in neuropsychiatric disorders. In spite of the promising results achieved in animal studies, detrimental consequences of manipulating this endogenous system cannot be underestimated over the potential and promising perspectives of its therapeutic manipulation.
Conflict of Interest Statement
The authors declare that the research was conducted in the absence of any commercial or financial relationships that could be construed as a potential conflict of interest.
Acknowledgments
Instituto de Salud Carlos III, Redes temáticas de Investigación Cooperativa en salud (ISCIII y FEDER): RD06/0001/1013 and RD06/0001/1004; Ministerio de Ciencia e Innovación: BFU2009-10109, GRUPOS UCM-BSCH (951579); Plan Nacional sobre Drogas SAS/1250/2009. Ministerio de Sanidad (10/02308). Francisco-Javier Bermudez-Silva is recipient of a research contract from the National System of Health (Instituto de Salud Carlos III; CP07/00283) and of a BAE from Instituto de Salud Carlos III (BA09/90066).
Footnotes
References
Aguiar, D. C., and Guimarães, F. S. (2009). Blockade of NMDA receptors and nitric oxide synthesis in the dorsolateral periaqueductal gray attenuates behavioral and cellular responses of rats exposed to a live predator. J. Neurosci. Res. 87, 2418–2429.
Ahn, K., Mckinney, M. K., and Cravatt, B. F. (2008). Enzymatic pathways that regulate endocannabinoid signaling in the nervous system. Chem. Rev. 108, 1687–1707.
Airaksinen, E., Wahlin, A., Forsell, Y., and Larsson, M. (2007). Low episodic memory performance as a premorbid marker of depression: evidence from a 3-year follow-up. Acta Psychiatr. Scand. 115, 458–465.
Akinshola, B. E., Chakrabarti, A., and Onaivi, E. S. (1999). In-vitro and in-vivo action of cannabinoids. Neurochem. Res. 24, 1233–1240.
Alves, F. H., Crestani, C. C., Gomes, F. V., Guimaraes, F. S., Correa, F. M., and Resstel, L. B. (2010). Cannabidiol injected into the bed nucleus of the stria terminalis modulates baroreflex activity through 5-HT1A receptors. Pharmacol. Res. 62, 228–236.
Amitai, N., Semenova, S., and Markou, A. (2007). Cognitive-disruptive effects of the psychotomimetic phencyclidine and attenuation by atypical antipsychotic medications in rats. Psychopharmacology (Berl.) 193, 521–537.
Andre, A., and Gonthier, M. P. (2010). The endocannabinoid system: its roles in energy balance and potential as a target for obesity treatment. Int. J. Biochem. Cell Biol. 42, 1788–1801.
Arevalo, C., De Miguel, R., and Hernandez-Tristan, R. (2001). Cannabinoid effects on anxiety-related behaviours and hypothalamic neurotransmitters. Pharmacol. Biochem. Behav. 70, 123–131.
Aso, E., Ozaita, A., Serra, M. A., and Maldonado, R. (2011). Genes differentially expressed in CB1 knockout mice: involvement in the depressive-like phenotype. Eur. Neuropsychopharmacol. 21, 11–22.
Aso, E., Ozaita, A., Valdizan, E. M., Ledent, C., Pazos, A., Maldonado, R., and Valverde, O. (2008). BDNF impairment in the hippocampus is related to enhanced despair behavior in CB1 knockout mice. J. Neurochem. 105, 565–572.
Baker, D., Pryce, G., Davies, W. L., and Hiley, C. R. (2006). In silico patent searching reveals a new cannabinoid receptor. Trends Pharmacol. Sci. 27, 1–4.
Bambico, F. R., and Gobbi, G. (2008). The cannabinoid CB1 receptor and the endocannabinoid anandamide: possible antidepressant targets. Expert Opin. Ther. Targets 12, 1347–1366.
Barch, D. M. (2005). The cognitive neuroscience of schizophrenia. Annu. Rev. Clin. Psychol. 1, 321–353.
Barrero, F. J., Ampuero, I., Morales, B., Vives, F., De Dios Luna Del Castillo, J., Hoenicka, J., and Garcia Yebenes, J. (2005). Depression in Parkinson’s disease is related to a genetic polymorphism of the cannabinoid receptor gene (CNR1). Pharmacogenomics J. 5, 135–141.
Beal, J. E., Olson, R., Laubenstein, L., Morales, J. O., Bellman, P., Yangco, B., Lefkowitz, L., Plasse, T. F., and Shepard, K. V. (1995). Dronabinol as a treatment for anorexia associated with weight loss in patients with AIDS. J. Pain Symptom Manage. 10, 89–97.
Bekker, M. H., and van Mens-Verhulst, J. (2007). Anxiety disorders: sex differences in prevalence, degree, and background, but gender-neutral treatment. Gend. Med. 4(Suppl. B), S178–S193.
Bergamaschi, M. M., Queiroz, R. H., Chagas, M. H., De Oliveira, D. C., De Martinis, B. S., Kapczinski, F., Quevedo, J., Roesler, R., Schroder, N., Nardi, A. E., Martin-Santos, R., Hallak, J. E., Zuardi, A. W., and Crippa, J. A. (2011). Cannabidiol reduces the anxiety induced by simulated public speaking in treatment-naive social phobia patients. Neuropsychopharmacology 36, 1219–1226.
Bermudez-Silva, F. J., Cardinal, P., and Cota, D. (2011). The role of the endocannabinoid system in the neuroendocrine regulation of energy balance. J. Psychopharmacol. PMID: 21824982. [Epub ahead of print].
Bermudez-Silva, F. J., Viveros, M. P., Mcpartland, J. M., and Rodriguez De Fonseca, F. (2010). The endocannabinoid system, eating behavior and energy homeostasis: the end or a new beginning? Pharmacol. Biochem. Behav. 95, 375–382.
Berrendero, F., and Maldonado, R. (2002). Involvement of the opioid system in the anxiolytic-like effects induced by Delta(9)-tetrahydrocannabinol. Psychopharmacology (Berl.) 163, 111–117.
Berry, E. M. (2006). “Pilot study of THC (2.5mgx2) in 9 ambulatory AN patients,” in The sixth Nordic Congress on Eating Disorders, Aarhus, Denmark.
Bierman, E. J., Comijs, H. C., Jonker, C., and Beekman, A. T. (2005). Effects of anxiety versus depression on cognition in later life. Am. J. Geriatr. Psychiatry 13, 686–693.
Biscaia, M., Marin, S., Fernandez, B., Marco, E. M., Rubio, M., Guaza, C., Ambrosio, E., and Viveros, M. P. (2003). Chronic treatment with CP 55,940 during the peri-adolescent period differentially affects the behavioural responses of male and female rats in adulthood. Psychopharmacology (Berl.) 170, 301–308.
Bisogno, T., Hanus, L., De Petrocellis, L., Tchilibon, S., Ponde, D. E., Brandi, I., Moriello, A. S., Davis, J. B., Mechoulam, R., and Di Marzo, V. (2001). Molecular targets for cannabidiol and its synthetic analogues: effect on vanilloid VR1 receptors and on the cellular uptake and enzymatic hydrolysis of anandamide. Br. J. Pharmacol. 134, 845–852.
Bitencourt, R. M., Pamplona, F. A., and Takahashi, R. N. (2008). Facilitation of contextual fear memory extinction and anti-anxiogenic effects of AM404 and cannabidiol in conditioned rats. Eur. Neuropsychopharmacol. 18, 849–859.
Bortolato, M., Mangieri, R. A., Fu, J., Kim, J. H., Arguello, O., Duranti, A., Tontini, A., Mor, M., Tarzia, G., and Piomelli, D. (2007). Antidepressant-like activity of the fatty acid amide hydrolase inhibitor URB597 in a rat model of chronic mild stress. Biol. Psychiatry 62, 1103–1110.
Bouaboula, M., Bourrie, B., Rinaldi-Carmona, M., Shire, D., Le Fur, G., and Casellas, P. (1995). Stimulation of cannabinoid receptor CB1 induces krox-24 expression in human astrocytoma cells. J. Biol. Chem. 270, 13973–13980.
Braff, D. L., Geyer, M. A., and Swerdlow, N. R. (2001). Human studies of prepulse inhibition of startle: normal subjects, patient groups, and pharmacological studies. Psychopharmacology (Berl.) 156, 234–258.
Braida, D., Limonta, V., Malabarba, L., Zani, A., and Sala, M. (2007). 5-HT1A receptors are involved in the anxiolytic effect of Delta9-tetrahydrocannabinol and AM 404, the anandamide transport inhibitor, in Sprague-Dawley rats. Eur. J. Pharmacol. 555, 156–163.
Budney, A. J., Hughes, J. R., Moore, B. A., and Vandrey, R. (2004). Review of the validity and significance of cannabis withdrawal syndrome. Am. J. Psychiatry 161, 1967–1977.
Bulik, C. M., Sullivan, P. F., and Kendler, K. S. (1998). Heritability of binge-eating and broadly defined bulimia nervosa. Biol. Psychiatry 44, 1210–1218.
Burdyga, G., Lal, S., Varro, A., Dimaline, R., Thompson, D. G., and Dockray, G. J. (2004). Expression of cannabinoid CB1 receptors by vagal afferent neurons is inhibited by cholecystokinin. J. Neurosci. 24, 2708–2715.
Campos, A. C., Aguado, T., Palazuellos, J., Guzman, M., Guimarães, F. S., and Roperh, I. G. (2010). Cannabidiol increases cell-proliferation by activating ERK1/2 MAP Kinase signalling. Can. Neurosci. [Abstract].
Campos, A. C., and Guimarães, F. S. (2008). Involvement of 5HT1A receptors in the anxiolytic-like effects of cannabidiol injected into the dorsolateral periaqueductal gray of rats. Psychopharmacology (Berl.) 199, 223–230.
Campos, A. C., and Guimarães, F. S. (2009). Evidence for a potential role for TRPV1 receptors in the dorsolateral periaqueductal gray in the attenuation of the anxiolytic effects of cannabinoids. Prog. Neuropsychopharmacol. Biol. Psychiatry 33, 1517–1521.
Carlisle, S. J., Marciano-Cabral, F., Staab, A., Ludwick, C., and Cabral, G. A. (2002). Differential expression of the CB2 cannabinoid receptor by rodent macrophages and macrophage-like cells in relation to cell activation. Int. Immunopharmacol. 2, 69–82.
Carrier, E. J., Auchampach, J. A., and Hillard, C. J. (2006). Inhibition of an equilibrative nucleoside transporter by cannabidiol: a mechanism of cannabinoid immunosuppression. Proc. Natl. Acad. Sci. U.S.A. 103, 7895–7900.
Carrier, E. J., Patel, S., Roelke, C. T., Shrestha, L., Meier, S., Gizewski, E., Fredholm, F. B., Auchampach, J. A., and Hillard, C. J. (2007). “The anxiolytic response to cannabidiol is modulated by the adenosine A1 receptor but not by the cannabinoid CB1 or CB2 receptors,” in International Cannabinoid Research Society (ICRS) Meeting, Saint-Sauveur, Québec, CA, 147.
Casarotto, P. C., Gomes, F. V., Resstel, L. B., and Guimaraes, F. S. (2010). Cannabidiol inhibitory effect on marble-burying behaviour: involvement of CB1 receptors. Behav. Pharmacol. 21, 353–358.
Chakrabarti, A., Onaivi, E. S., and Chaudhuri, G. (1995). Cloning and sequencing of a cDNA encoding the mouse brain-type cannabinoid receptor protein. DNA Seq. 5, 385–388.
Chavarria-Siles, I., Contreras-Rojas, J., Hare, E., Walss-Bass, C., Quezada, P., Dassori, A., Contreras, S., Medina, R., Ramirez, M., Salazar, R., Raventos, H., and Escamilla, M. A. (2008). Cannabinoid receptor 1 gene (CNR1) and susceptibility to a quantitative phenotype for hebephrenic schizophrenia. Am. J. Med. Genet. B Neuropsychiatr. Genet. 147, 279–284.
Chhatwal, J. P., Davis, M., Maguschak, K. A., and Ressler, K. J. (2005). Enhancing cannabinoid neurotransmission augments the extinction of conditioned fear. Neuropsychopharmacology 30, 516–524.
Clement, Y., Calatayud, F., and Belzung, C. (2002). Genetic basis of anxiety-like behaviour: a critical review. Brain Res. Bull. 57, 57–71.
Cohen, M., Solowij, N., and Carr, V. (2008). Cannabis, cannabinoids and schizophrenia: integration of the evidence. Aust. N. Z. J. Psychiatry 42, 357–368.
Crippa, J. A., Zuardi, A. W., Garrido, G. E., Wichert-Ana, L., Guarnieri, R., Ferrari, L., Azevedo-Marques, P. M., Hallak, J. E., Mcguire, P. K., and Filho Busatto, G. (2004). Effects of cannabidiol (CBD) on regional cerebral blood flow. Neuropsychopharmacology 29, 417–426.
Crippa, J. A., Zuardi, A. W., Martin-Santos, R., Bhattacharyya, S., Atakan, Z., Mcguire, P., and Fusar-Poli, P. (2009). Cannabis and anxiety: a critical review of the evidence. Hum. Psychopharmacol. 24, 515–523.
Cristino, L., De Petrocellis, L., Pryce, G., Baker, D., Guglielmotti, V., and Di Marzo, V. (2006). Immunohistochemical localization of cannabinoid type 1 and vanilloid transient receptor potential vanilloid type 1 receptors in the mouse brain. Neuroscience 139, 1405–1415.
Da Settimo, F., Taliani, S., Trincavelli, M. L., Montali, M., and Martini, C. (2007). GABA A/Bz receptor subtypes as targets for selective drugs. Curr. Med. Chem. 14, 2680–2701.
Davidson, R. J., Lewis, D. A., Alloy, L. B., Amaral, D. G., Bush, G., Cohen, J. D., Drevets, W. C., Farah, M. J., Kagan, J., Mcclelland, J. L., Nolen-Hoeksema, S., and Peterson, B. S. (2002). Neural and behavioral substrates of mood and mood regulation. Biol. Psychiatry 52, 478–502.
Dean, B., Sundram, S., Bradbury, R., Scarr, E., and Copolov, D. (2001). Studies on [3H]CP-55940 binding in the human central nervous system: regional specific changes in density of cannabinoid-1 receptors associated with schizophrenia and cannabis use. Neuroscience 103, 9–15.
Degenhardt, L., Hall, W., and Lynskey, M. (2003). Exploring the association between cannabis use and depression. Addiction 98, 1493–1504.
Demirakca, T., Sartorius, A., Ende, G., Meyer, N., Welzel, H., Skopp, G., Mann, K., and Hermann, D. (2011). Diminished gray matter in the hippocampus of cannabis users: possible protective effects of cannabidiol. Drug Alcohol Depend. 114, 242–245.
Deng, C., Han, M., and Huang, X. F. (2007). No changes in densities of cannabinoid receptors in the superior temporal gyrus in schizophrenia. Neurosci. Bull. 23, 341–347.
Dere, E., Pause, B. M., and Pietrowsky, R. (2010). Emotion and episodic memory in neuropsychiatric disorders. Behav. Brain Res. 215, 162–171.
Derocq, J. M., Segui, M., Marchand, J., Lefur, G., and Casellas, P. (1995). Cannabinoids enhance human B-cell growth at low nanomolar concentrations. FEBS Lett. 369, 177–182.
Di Forti, M., Morrison, P. D., Butt, A., and Murray, R. M. (2007). Cannabis use and psychiatric and cogitive disorders: the chicken or the egg? Curr. Opin. Psychiatry 20, 228–234.
Di Marzo, V., Goparaju, S. K., Wang, L., Liu, J., Batkai, S., Jarai, Z., Fezza, F., Miura, G. I., Palmiter, R. D., Sugiura, T., and Kunos, G. (2001). Leptin-regulated endocannabinoids are involved in maintaining food intake. Nature 410, 822–825.
Di Marzo, V., Ligresti, A., and Cristino, L. (2009). The endocannabinoid system as a link between homoeostatic and hedonic pathways involved in energy balance regulation. Int. J. Obes. (Lond.) 33(Suppl. 2), S18–S24.
DiPatrizio, N. V., and Simansky, K. J. (2008). Activating parabrachial cannabinoid CB1 receptors selectively stimulates feeding of palatable foods in rats. J. Neurosci. 28, 9702–9709.
Doggrell, S. A. (2008). Is rimonabant efficacious and safe in the treatment of obesity? Expert Opin. Pharmacother. 9, 2727–2731.
Domenici, M. R., Azad, S. C., Marsicano, G., Schierloh, A., Wotjak, C. T., Dodt, H. U., Zieglgansberger, W., Lutz, B., and Rammes, G. (2006). Cannabinoid receptor type 1 located on presynaptic terminals of principal neurons in the forebrain controls glutamatergic synaptic transmission. J. Neurosci. 26, 5794–5799.
D’Souza, D. C., Sewell, R. A., and Ranganathan, M. (2009). Cannabis and psychosis/schizophrenia: human studies. Eur. Arch. Psychiatry Clin. Neurosci. 259, 413–431.
Egerton, A., Allison, C., Brett, R. R., and Pratt, J. A. (2006). Cannabinoids and prefrontal cortical function: insights from preclinical studies. Neurosci. Biobehav. Rev. 30, 680–695.
Eggan, S. M., Hashimoto, T., and Lewis, D. A. (2008). Reduced cortical cannabinoid 1 receptor messenger RNA and protein expression in schizophrenia. Arch. Gen. Psychiatry 65, 772–784.
Ehrhart, J., Obregon, D., Mori, T., Hou, H., Sun, N., Bai, Y., Klein, T., Fernandez, F., Tan, J., and Shytle, R. D. (2005). Stimulation of cannabinoid receptor 2 (CB2) suppresses microglial activation. J. Neuroinflammation 2, 29.
Ellenbroek, B. A., and Riva, M. A. (2003). Early maternal deprivation as an animal model for schizophrenia. Clin. Neurosci. Res. 3, 297–302.
Fattore, L., Fadda, P., Spano, M. S., Pistis, M., and Fratta, W. (2008). Neurobiological mechanisms of cannabinoid addiction. Mol. Cell. Endocrinol. 286, S97–S107.
Fernandez-Espejo, E., Viveros, M. P., Nunez, L., Ellenbroek, B. A., and Rodriguez De Fonseca, F. (2009). Role of cannabis and endocannabinoids in the genesis of schizophrenia. Psychopharmacology (Berl.) 206, 531–549.
Finn, D. P. (2010). Endocannabinoid-mediated modulation of stress responses: physiological and pathophysiological significance. Immunobiology 215, 629–646.
Fone, K. C., and Porkess, M. V. (2008). Behavioural and neurochemical effects of post-weaning social isolation in rodents-relevance to developmental neuropsychiatric disorders. Neurosci. Biobehav. Rev. 32, 1087–1102.
Frieling, H., Albrecht, H., Jedtberg, S., Gozner, A., Lenz, B., Wilhelm, J., Hillemacher, T., De Zwaan, M., Kornhuber, J., and Bleich, S. (2009). Elevated cannabinoid 1 receptor mRNA is linked to eating disorder related behavior and attitudes in females with eating disorders. Psychoneuroendocrinology 34, 620–624.
Fu, J., Gaetani, S., Oveisi, F., Lo Verme, J., Serrano, A., Rodriguez De Fonseca, F., Rosengarth, A., Luecke, H., Di Giacomo, B., Tarzia, G., and Piomelli, D. (2003). Oleylethanolamide regulates feeding and body weight through activation of the nuclear receptor PPAR-alpha. Nature 425, 90–93.
Fusar-Poli, P., Allen, P., Bhattacharyya, S., Crippa, J. A., Mechelli, A., Borgwardt, S., Martin-Santos, R., Seal, M. L., O’carrol, C., Atakan, Z., Zuardi, A. W., and Mcguire, P. (2010). Modulation of effective connectivity during emotional processing by Delta 9-tetrahydrocannabinol and cannabidiol. Int. J. Neuropsychopharmacol. 13, 421–432.
Fusar-Poli, P., Crippa, J. A., Bhattacharyya, S., Borgwardt, S. J., Allen, P., Martin-Santos, R., Seal, M., Surguladze, S. A., O’carrol, C., Atakan, Z., Zuardi, A. W., and Mcguire, P. K. (2009). Distinct effects of {delta}9-tetrahydrocannabinol and cannabidiol on neural activation during emotional processing. Arch. Gen. Psychiatry 66, 95–105.
Gaetani, S., Kaye, W. H., Cuomo, V., and Piomelli, D. (2008). Role of endocannabinoids and their analogues in obesity and eating disorders. Eat. Weight Disord. 13, e42–e48.
Galiegue, S., Mary, S., Marchand, J., Dussossoy, D., Carriere, D., Carayon, P., Bouaboula, M., Shire, D., Le Fur, G., and Casellas, P. (1995). Expression of central and peripheral cannabinoid receptors in human immune tissues and leukocyte subpopulations. Eur. J. Biochem. 232, 54–61.
Galve-Roperh, I., Aguado, T., Palazuelos, J., and Guzman, M. (2008). Mechanisms of control of neuron survival by the endocannabinoid system. Curr. Pharm. Des. 14, 2279–2288.
García-Gutiérrez, M. S., and Manzanares, J. (2010). The cannabinoid CB1 receptor is involved in the anxiolytic, sedative and amnesic actions of benzodiazepines. J. Psychopharmacol. (Oxford) 24, 757–765.
García-Gutiérrez, M. S., and Manzanares, J. (2011). Overexpression of CB2 cannabinoid receptors decreased vulnerability to anxiety and impaired anxiolytic action of alprazolam in mice. J. Psychopharmacol. (Oxford) 25, 111–120.
García-Gutiérrez, M. S., Garcia-Bueno, B., Zoppi, S., Leza, J. C., and Manzanares, J. (2011). Chronic blockade of cannabinoid CB(2) receptors induces anxiolytic-like actions associated to alterations in GABA(A) receptors. Br. J. Pharmacol. doi: 10.1111/j.1476-5381.2011.01625.x. [Epub ahead of print].
García-Gutiérrez, M. S., Perez-Ortiz, J. M., Gutierrez-Adan, A., and Manzanares, J. (2010). Depression-resistant endophenotype in mice overexpressing cannabinoid CB(2) receptors. Br. J. Pharmacol. 160, 1773–1784.
Giuffrida, A., Leweke, F. M., Gerth, C. W., Schreiber, D., Koethe, D., Faulhaber, J., Klosterkotter, J., and Piomelli, D. (2004). Cerebrospinal anandamide levels are elevated in acute schizophrenia and are inversely correlated with psychotic symptoms. Neuropsychopharmacology 29, 2108–2114.
Glass, M., Dragunow, M., and Faull, R. L. (1997). Cannabinoid receptors in the human brain: a detailed anatomical and quantitative autoradiographic study in the fetal, neonatal and adult human brain. Neuroscience 77, 299–318.
Gomes, F. V., Resstel, L. B., and Guimaraes, F. S. (2011). The anxiolytic-like effects of cannabidiol injected into the bed nucleus of the stria terminalis are mediated by 5-HT1A receptors. Psychopharmacology (Berl.) 213, 465–473.
Gong, J. P., Onaivi, E. S., Ishiguro, H., Liu, Q. R., Tagliaferro, P. A., Brusco, A., and Uhl, G. R. (2006). Cannabinoid CB2 receptors: immunohistochemical localization in rat brain. Brain Res. 1071, 10–23.
Gorzalka, B. B., and Hill, M. N. (2011). Putative role of endocannabinoid signaling in the etiology of depression and actions of antidepressants. Prog. Neuropsychopharmacol. Biol. Psychiatry 35, 1575–1585.
Grayson, B., Idris, N. F., and Neill, J. C. (2007). Atypical antipsychotics attenuate a sub-chronic PCP-induced cognitive deficit in the novel object recognition task in the rat. Behav. Brain Res. 184, 31–38.
Griffin, G., Wray, E. J., Tao, Q., Mcallister, S. D., Rorrer, W. K., Aung, M. M., Martin, B. R., and Abood, M. E. (1999). Evaluation of the cannabinoid CB2 receptor-selective antagonist, SR144528: further evidence for cannabinoid CB2 receptor absence in the rat central nervous system. Eur. J. Pharmacol. 377, 117–125.
Gross, H., Ebert, M. H., Faden, V. B., Goldberg, S. C., Kaye, W. H., Caine, E. D., Hawks, R., and Zinberg, N. (1983). A double-blind trial of delta 9-tetrahydrocannabinol in primary anorexia nervosa. J. Clin. Psychopharmacol. 3, 165–171.
Gualtieri, C. T., and Morgan, D. W. (2008). The frequency of cognitive impairment in patients with anxiety, depression, and bipolar disorder: an unaccounted source of variance in clinical trials. J. Clin. Psychiatry 69, 1122–1130.
Guimarães, F. S., Chiaretti, T. M., Graeff, F. G., and Zuardi, A. W. (1990). Antianxiety effect of cannabidiol in the elevated plus-maze. Psychopharmacology (Berl.) 100, 558–559.
Guimarães, F. S., De Aguiar, J. C., Mechoulam, R., and Breuer, A. (1994). Anxiolytic effect of cannabidiol derivatives in the elevated plus-maze. Gen. Pharmacol. 25, 161–164.
Guimarães, F. S., Del Bel, E. A., Padovan, C. M., Netto, S. M., and De Almeida, R. T. (1993). Hippocampal 5-HT receptors and consolidation of stressful memories. Behav. Brain Res. 58, 133–139.
Guimarães, V. M., Zuardi, A. W., Del Bel, E. A., and Guimaraes, F. S. (2004). Cannabidiol increases Fos expression in the nucleus accumbens but not in the dorsal striatum. Life Sci. 75, 633–638.
Guindon, J., and Hohmann, A. G. (2009). The endocannabinoid system and pain. CNS Neurol. Disord. Drug Targets 8, 403–421.
Gururajan, A., Taylor, D. A., and Malone, D. T. (2011). Effect of cannabidiol in a MK-801-rodent model of aspects of schizophrenia. Behav. Brain Res. 222, 299–308.
Guzman, M., Sanchez, C., and Galve-Roperh, I. (2001). Control of the cell survival/death decision by cannabinoids. J. Mol. Med. 78, 613–625.
Hall, W., and Degenhardt, L. (2009). Adverse health effects of non-medical cannabis use. Lancet 374, 1383–1391.
Haller, J., Bakos, N., Szirmay, M., Ledent, C., and Freund, T. F. (2002). The effects of genetic and pharmacological blockade of the CB1 cannabinoid receptor on anxiety. Eur. J. Neurosci. 16, 1395–1398.
Haller, J., Matyas, F., Soproni, K., Varga, B., Barsy, B., Nemeth, B., Mikics, E., Freund, T. F., and Hajos, N. (2007). Correlated species differences in the effects of cannabinoid ligands on anxiety and on GABAergic and glutamatergic synaptic transmission. Eur. J. Neurosci. 25, 2445–2456.
Haller, J., Szirmai, M., Varga, B., Ledent, C., and Freund, T. F. (2005). Cannabinoid CB1 receptor dependent effects of the NMDA antagonist phencyclidine in the social withdrawal model of schizophrenia. Behav. Pharmacol. 16, 415–422.
Haller, J., Varga, B., Ledent, C., Barna, I., and Freund, T. F. (2004). Context-dependent effects of CB1 cannabinoid gene disruption on anxiety-like and social behaviour in mice. Eur. J. Neurosci. 19, 1906–1912.
Hamdani, N., Tabeze, J. P., Ramoz, N., Ades, J., Hamon, M., Sarfati, Y., Boni, C., and Gorwood, P. (2008). The CNR1 gene as a pharmacogenetic factor for antipsychotics rather than a susceptibility gene for schizophrenia. Eur. Neuropsychopharmacol. 18, 34–40.
Harkany, T., Guzman, M., Galve-Roperh, I., Berghuis, P., Devi, L. A., and Mackie, K. (2007). The emerging functions of endocannabinoid signaling during CNS development. Trends Pharmacol. Sci. 28, 83–92.
Heim, C., Ehlert, U., and Hellhammer, D. H. (2000). The potential role of hypocortisolism in the pathophysiology of stress-related bodily disorders. Psychoneuroendocrinology 25, 1–35.
Herkenham, M., Lynn, A. B., Johnson, M. R., Melvin, L. S., De Costa, B. R., and Rice, K. C. (1991). Characterization and localization of cannabinoid receptors in rat brain: a quantitative in vitro autoradiographic study. J. Neurosci. 11, 563–583.
Hill, M. N., Carrier, E. J., Mclaughlin, R. J., Morrish, A. C., Meier, S. E., Hillard, C. J., and Gorzalka, B. B. (2008). Regional alterations in the endocannabinoid system in an animal model of depression: effects of concurrent antidepressant treatment. J. Neurochem. 106, 2322–2336.
Hill, M. N., Miller, G. E., Carrier, E. J., Gorzalka, B. B., and Hillard, C. J. (2009). Circulating endocannabinoids and N-acyl ethanolamines are differentially regulated in major depression and following exposure to social stress. Psychoneuroendocrinology 34, 1257–1262.
Hill, M. N., Patel, S., Carrier, E. J., Rademacher, D. J., Ormerod, B. K., Hillard, C. J., and Gorzalka, B. B. (2005). Downregulation of endocannabinoid signaling in the hippocampus following chronic unpredictable stress. Neuropsychopharmacology 30, 508–515.
Hollis, C., Groom, M. J., Das, D., Calton, T., Bates, A. T., Andrews, H. K., Jackson, G. M., and Liddle, P. F. (2008). Different psychological effects of cannabis use in adolescents at genetic high risk for schizophrenia and with attention deficit/hyperactivity disorder (ADHD). Schizophr. Res. 105, 216–223.
Holter, S. M., Kallnik, M., Wurst, W., Marsicano, G., Lutz, B., and Wotjak, C. T. (2005). Cannabinoid CB1 receptor is dispensable for memory extinction in an appetitively-motivated learning task. Eur. J. Pharmacol. 510, 69–74.
Holtkamp, K., Herpertz-Dahlmann, B., Hebebrand, K., Mika, C., Kratzsch, J., and Hebebrand, J. (2006). Physical activity and restlessness correlate with leptin levels in patients with adolescent anorexia nervosa. Biol. Psychiatry 60, 311–313.
Huang, E. J., and Reichardt, L. F. (2001). Neurotrophins: roles in neuronal development and function. Annu. Rev. Neurosci. 24, 677–736.
Hungund, B. L., Vinod, K. Y., Kassir, S. A., Basavarajappa, B. S., Yalamanchili, R., Cooper, T. B., Mann, J. J., and Arango, V. (2004). Upregulation of CB1 receptors and agonist-stimulated [35S]GTPgammaS binding in the prefrontal cortex of depressed suicide victims. Mol. Psychiatry 9, 184–190.
Ibrahim, M. M., Deng, H., Zvonok, A., Cockayne, D. A., Kwan, J., Mata, H. P., Vanderah, T. W., Lai, J., Porreca, F., Makriyannis, A., and Malan, T. P. Jr. (2003). Activation of CB2 cannabinoid receptors by AM1241 inhibits experimental neuropathic pain: pain inhibition by receptors not present in the CNS. Proc. Natl. Acad. Sci. U.S.A. 100, 10529–10533.
Isaac, C. L., Cushway, D., and Jones, G. V. (2006). Is posttraumatic stress disorder associated with specific deficits in episodic memory? Clin. Psychol. Rev. 26, 939–955.
Ishiguro, H., Carpio, O., Horiuchi, Y., Shu, A., Higuchi, S., Schanz, N., Benno, R., Arinami, T., and Onaivi, E. S. (2010a). A nonsynonymous polymorphism in cannabinoid CB2 receptor gene is associated with eating disorders in humans and food intake is modified in mice by its ligands. Synapse 64, 92–96.
Ishiguro, H., Horiuchi, Y., Ishikawa, M., Koga, M., Imai, K., Suzuki, Y., Morikawa, M., Inada, T., Watanabe, Y., Takahashi, M., Someya, T., Ujike, H., Iwata, N., Ozaki, N., Onaivi, E. S., Kunugi, H., Sasaki, T., Itokawa, M., Arai, M., Niizato, K., Iritani, S., Naka, I., Ohashi, J., Kakita, A., Takahashi, H., Nawa, H., and Arinami, T. (2010b). Brain cannabinoid CB2 receptor in schizophrenia. Biol. Psychiatry 67, 974–982.
Iyer, S. N., Boekestyn, L., Cassidy, C. M., King, S., Joober, R., and Malla, A. K. (2008). Signs and symptoms in the pre-psychotic phase: description and implications for diagnostic trajectories. Psychol. Med. 38, 1147–1156.
Izzo, A. A., Borrelli, F., Capasso, R., Di Marzo, V., and Mechoulam, R. (2009). Non-psychotropic plant cannabinoids: new therapeutic opportunities from an ancient herb. Trends Pharmacol. Sci. 30, 515–527.
Jager, G., and Ramsey, N. F. (2008). Long-term consequences of adolescent cannabis exposure on the development of cognition, brain structure and function: an overview of animal and human research. Curr. Drug Abuse Rev. 1, 114–123.
Joosten, M., Valk, P. J., Jorda, M. A., Vankan-Berkhoudt, Y., Verbakel, S., Van Den Broek, M., Beijen, A., Lowenberg, B., and Delwel, R. (2002). Leukemic predisposition of pSca-1/Cb2 transgenic mice. Exp. Hematol. 30, 142–149.
Juhasz, G., Chase, D., Pegg, E., Downey, D., Toth, Z. G., Stones, K., Platt, H., Mekli, K., Payton, A., Elliott, R., Anderson, I. M., and Deakin, J. F. (2009). CNR1 gene is associated with high neuroticism and low agreeableness and interacts with recent negative life events to predict current depressive symptoms. Neuropsychopharmacology 34, 2019–2027.
Kamprath, K., Marsicano, G., Tang, J., Monory, K., Bisogno, T., Di Marzo, V., Lutz, B., and Wotjak, C. T. (2006). Cannabinoid CB1 receptor mediates fear extinction via habituation-like processes. J. Neurosci. 26, 6677–6686.
Kamprath, K., Plendl, W., Marsicano, G., Deussing, J. M., Wurst, W., Lutz, B., and Wotjak, C. T. (2009). Endocannabinoids mediate acute fear adaptation via glutamatergic neurons independently of corticotropin-releasing hormone signaling. Genes Brain Behav. 8, 203–211.
Katona, I., and Freund, T. F. (2008). Endocannabinoid signaling as a synaptic circuit breaker in neurological disease. Nat. Med. 14, 923–930.
Katona, I., Rancz, E. A., Acsady, L., Ledent, C., Mackie, K., Hajos, N., and Freund, T. F. (2001). Distribution of CB1 cannabinoid receptors in the amygdala and their role in the control of GABAergic transmission. J. Neurosci. 21, 9506–9518.
Katona, I., Sperlagh, B., Sik, A., Kafalvi, A., Vizi, E. S., Mackie, K., and Freund, T. F. (1999). Presynaptically located CB1 cannabinoid receptors regulate GABA release from axon terminals of specific hippocampal interneurons. J. Neurosci. 19, 4544–4558.
Kawamura, Y., Fukaya, M., Maejima, T., Yoshida, T., Miura, E., Watanabe, M., Ohno-Shosaku, T., and Kano, M. (2006). The CB1 cannabinoid receptor is the major cannabinoid receptor at excitatory presynaptic sites in the hippocampus and cerebellum. J. Neurosci. 26, 2991–3001.
Kaye, W. H., Nagata, T., Weltzin, T. E., Hsu, L. K., Sokol, M. S., Mcconaha, C., Plotnicov, K. H., Weise, J., and Deep, D. (2001). Double-blind placebo-controlled administration of fluoxetine in restricting- and restricting-purging-type anorexia nervosa. Biol. Psychiatry 49, 644–652.
Kessler, R. C., Demler, O., Frank, R. G., Olfson, M., Pincus, H. A., Walters, E. E., Wang, P., Wells, K. B., and Zaslavsky, A. M. (2005). Prevalence and treatment of mental disorders, 1990 to 2003. N. Engl. J. Med. 352, 2515–2523.
Kirkham, T. C., Williams, C. M., Fezza, F., and Di Marzo, V. (2002). Endocannabinoid levels in rat limbic forebrain and hypothalamus in relation to fasting, feeding and satiation: stimulation of eating by 2-arachidonoyl glycerol. Br. J. Pharmacol. 136, 550–557.
Klump, K. L., Miller, K. B., Keel, P. K., Mcgue, M., and Iacono, W. G. (2001). Genetic and environmental influences on anorexia nervosa syndromes in a population-based twin sample. Psychol. Med. 31, 737–740.
Koethe, D., Llenos, I. C., Dulay, J. R., Hoyer, C., Torrey, E. F., Leweke, F. M., and Weis, S. (2007). Expression of CB1 cannabinoid receptor in the anterior cingulate cortex in schizophrenia, bipolar disorder, and major depression. J. Neural Transm. 114, 1055–1063.
Ledent, C., Valverde, O., Cossu, G., Petitet, F., Aubert, J. F., Beslot, F., Bohme, G. A., Imperato, A., Pedrazzini, T., Roques, B. P., Vassart, G., Fratta, W., and Parmentier, M. (1999). Unresponsiveness to cannabinoids and reduced addictive effects of opiates in CB1 receptor knockout mice. Science 283, 401–404.
Lemos, J. I., Resstel, L. B., and Guimaraes, F. S. (2010). Involvement of the prelimbic prefrontal cortex on cannabidiol-induced attenuation of contextual conditioned fear in rats. Behav. Brain Res. 207, 105–111.
Levens, S. M., and Gotlib, I. H. (2009). Impaired selection of relevant positive information in depression. Depress. Anxiety 26, 403–410.
Leweke, F. M., and Koethe, D. (2008). Cannabis and psychiatric disorders: it is not only addiction. Addict. Biol. 13, 264–275.
Leweke, F. M., and Schneider, M. (2011). Chronic pubertal cannabinoid treatment as a behavioural model for aspects of schizophrenia: effects of the atypical antipsychotic quetiapine. Int. J. Neuropsychopharmacol. 14, 43–51.
Lewis, D. A., and Levitt, P. (2002). Schizophrenia as a disorder of neurodevelopment. Annu. Rev. Neurosci. 25, 409–432.
Liu, Q. R., Pan, C. H., Hishimoto, A., Li, C. Y., Xi, Z. X., Llorente-Berzal, A., Viveros, M. P., Ishiguro, H., Arinami, T., Onaivi, E. S., and Uhl, G. R. (2009). Species differences in cannabinoid receptor 2 (CNR2 gene): identification of novel human and rodent CB2 isoforms, differential tissue expression and regulation by cannabinoid receptor ligands. Genes Brain Behav. 8, 519–530.
Llorente, R., Miguel-Blanco, C., Aisa, B., Lachize, S., Borcel, E., Meijer, O. C., Ramirez, M. J., De Kloet, E. R., and Viveros, M. P. (2011). Long term sex-dependent psychoneuroendocrine effects of maternal deprivation and juvenile unpredictable stress in rats. J. Neuroendocrinol. 23, 329–344.
Llorente-Berzal, A., Mela, V., Borcel, E., Valero, M., Lopez-Gallardo, M., Viveros, M. P., and Marco, E. M. (2011). Neurobehavioral and metabolic long-term consequences of neonatal maternal deprivation stress and adolescent olanzapine treatment in male and female rats. Neuropharmacology. PMID: 21819999. [Epub ahead of print].
Long, L. E., Malone, D. T., and Taylor, D. A. (2006). Cannabidiol reverses MK-801-induced disruption of prepulse inhibition in mice. Neuropsychopharmacology 31, 795–803.
Low, K., Crestani, F., Keist, R., Benke, D., Brunig, I., Benson, J. A., Fritschy, J. M., Rulicke, T., Bluethmann, H., Mohler, H., and Rudolph, U. (2000). Molecular and neuronal substrate for the selective attenuation of anxiety. Science 290, 131–134.
Lowe, B., Zipfel, S., Buchholz, C., Dupont, Y., Reas, D. L., and Herzog, W. (2001). Long-term outcome of anorexia nervosa in a prospective 21-year follow-up study. Psychol. Med. 31, 881–890.
Lutz, B., Aparisi, A., and Viveros, M. P. (2010). “The dual role of the endocannabinoid system as a regulator of anxiety responses,” in FENS Forum 2010, Amsterdam, The Netherlands. FENS Abstr., 088.035.
Maccarrone, M., Gasperi, V., Catani, M. V., Diep, T. A., Dainese, E., Hansen, H. S., and Avigliano, L. (2010). The endocannabinoid system and its relevance for nutrition. Annu. Rev. Nutr. 30, 423–440.
Maccarrone, M., Valverde, O., Barbaccia, M. L., Castane, A., Maldonado, R., Ledent, C., Parmentier, M., and Finazzi-Agro, A. (2002). Age-related changes of anandamide metabolism in CB1 cannabinoid receptor knockout mice: correlation with behaviour. Eur. J. Neurosci. 15, 1178–1186.
Mackie, K. (2005). “Distribution of cannabinoid receptors in the central and peripheral nervous system,” in Cannabinoids. Handbook of Experimental Pharmacology, ed. R. G. Pertwee (Berlin: Springer), 299–323.
Mahler, S. V., Smith, K. S., and Berridge, K. C. (2007). Endocannabinoid hedonic hotspot for sensory pleasure: anandamide in nucleus accumbens shell enhances “liking” of a sweet reward. Neuropsychopharmacology 32, 2267–2278.
Mailleux, P., and Vanderhaeghen, J. J. (1992). Distribution of neuronal cannabinoid receptor in the adult rat brain: a comparative receptor binding radioautography and in situ hybridization histochemistry. Neuroscience 48, 655–668.
Maldonado, R., Valverde, O., and Berrendero, F. (2006). Involvement of the endocannabinoid system in drug addiction. Trends Neurosci. 29, 225–232.
Malone, D. T., Kearn, C. S., Chongue, L., Mackie, K., and Taylor, D. A. (2008). Effect of social isolation on CB1 and D2 receptor and fatty acid amide hydrolase expression in rats. Neuroscience 152, 265–272.
Manji, H. K., Drevets, W. C., and Charney, D. S. (2001). The cellular neurobiology of depression. Nat. Med. 7, 541–547.
Manzanares, J., Uriguen, L., Rubio, G., and Palomo, T. (2004). Role of endocannabinoid system in mental diseases. Neurotox. Res. 6, 213–224.
Marco, E. M., Adriani, W., Llorente, R., Laviola, G., and Viveros, M. P. (2009). Detrimental psychophysiological effects of early maternal deprivation in adolescent and adult rodents: altered responses to cannabinoid exposure. Neurosci. Biobehav. Rev. 33, 498–507.
Marco, E. M., Perez-Alvarez, L., Borcel, E., Rubio, M., Guaza, C., Ambrosio, E., File, S. E., and Viveros, M. P. (2004). Involvement of 5-HT1A receptors in behavioural effects of the cannabinoid receptor agonist CP 55,940 in male rats. Behav. Pharmacol. 15, 21–27.
Marco, E. M., and Viveros, M. P. (2009). The critical role of the endocannabinoid system in emotional homeostasis: avoiding excess and deficiencies. Mini Rev. Med. Chem. 9, 1407–1415.
Marin, S., Marco, E., Biscaia, M., Fernandez, B., Rubio, M., Guaza, C., Schmidhammer, H., and Viveros, M. P. (2003). Involvement of the kappa-opioid receptor in the anxiogenic-like effect of CP 55,940 in male rats. Pharmacol. Biochem. Behav. 74, 649–656.
Marsch, R., Foeller, E., Rammes, G., Bunck, M., Kossl, M., Holsboer, F., Zieglgansberger, W., Landgraf, R., Lutz, B., and Wotjak, C. T. (2007). Reduced anxiety, conditioned fear, and hippocampal long-term potentiation in transient receptor potential vanilloid type 1 receptor-deficient mice. J. Neurosci. 27, 832–839.
Marsicano, G., and Lutz, B. (2006). Neuromodulatory functions of the endocannabinoid system. J. Endocrinol. Invest. 29, 27–46.
Marsicano, G., Wotjak, C. T., Azad, S. C., Bisogno, T., Rammes, G., Cascio, M. G., Hermann, H., Tang, J., Hofmann, C., Zieglgansberger, W., Di Marzo, V., and Lutz, B. (2002). The endogenous cannabinoid system controls extinction of aversive memories. Nature 418, 530–534.
Martin, M., Ledent, C., Parmentier, M., Maldonado, R., and Valverde, O. (2002a). Involvement of CB1 cannabinoid receptors in emotional behaviour. Psychopharmacology (Berl.) 159, 379–387.
Martin, M., Ledent, C., Parmentier, M., Maldonado, R., and Valverde, O. (2002b). Involvement of CB1 cannabinoid receptors in emotional behaviour. Psychopharmacology (Berl.) 159, 379–387.
Matias, I., and Di Marzo, V. (2007). Endocannabinoids and the control of energy balance. Trends Endocrinol. Metab. 18, 27–37.
Mechoulam, R., Peters, M., Murillo-Rodriguez, E., and Hanus, L. O. (2007). Cannabidiol – recent advances. Chem. Biodivers. 4, 1678–1692.
Mishima, K., Hayakawa, K., Abe, K., Ikeda, T., Egashira, N., Iwasaki, K., and Fujiwara, M. (2005). Cannabidiol prevents cerebral infarction via a serotonergic 5-hydroxytryptamine1A receptor-dependent mechanism. Stroke 36, 1077–1082.
Molina-Holgado, E., Vela, J. M., Arevalo-Martin, A., Almazan, G., Molina-Holgado, F., Borrell, J., and Guaza, C. (2002). Cannabinoids promote oligodendrocyte progenitor survival: involvement of cannabinoid receptors and phosphatidylinositol-3 kinase/Akt signaling. J. Neurosci. 22, 9742–9753.
Monory, K., Massa, F., Egertova, M., Eder, M., Blaudzun, H., Westenbroek, R., Kelsch, W., Jacob, W., Marsch, R., Ekker, M., Long, J., Rubenstein, J. L., Goebbels, S., Nave, K. A., During, M., Klugmann, M., Wolfel, B., Dodt, H. U., Zieglgansberger, W., Wotjak, C. T., Mackie, K., Elphick, M. R., Marsicano, G., and Lutz, B. (2006). The endocannabinoid system controls key epileptogenic circuits in the hippocampus. Neuron 51, 455–466.
Monteleone, P., Bifulco, M., Di Filippo, C., Gazzerro, P., Canestrelli, B., Monteleone, F., Proto, M. C., Di Genio, M., Grimaldi, C., and Maj, M. (2009). Association of CNR1 and FAAH endocannabinoid gene polymorphisms with anorexia nervosa and bulimia nervosa: evidence for synergistic effects. Genes Brain Behav. 8, 728–732.
Monteleone, P., Dilieto, A., Castaldo, E., and Maj, M. (2004). Leptin functioning in eating disorders. CNS Spectr. 9, 523–529.
Monteleone, P., Matias, I., Martiadis, V., De Petrocellis, L., Maj, M., and Di Marzo, V. (2005). Blood levels of the endocannabinoid anandamide are increased in anorexia nervosa and in binge-eating disorder, but not in bulimia nervosa. Neuropsychopharmacology 30, 1216–1221.
Monteleone, P., Tortorella, A., Martiadis, V., Di Filippo, C., Canestrelli, B., and Maj, M. (2008). The cDNA 385C to A missense polymorphism of the endocannabinoid degrading enzyme fatty acid amide hydrolase (FAAH) is associated with overweight/obesity but not with binge eating disorder in overweight/obese women. Psychoneuroendocrinology 33, 546–550.
Moore, T. H., Zammit, S., Lingford-Hughes, A., Barnes, T. R., Jones, P. B., Burke, M., and Lewis, G. (2007). Cannabis use and risk of psychotic or affective mental health outcomes: a systematic review. Lancet 370, 319–328.
Moreira, F. A., Aguiar, D. C., Campos, A. C., Lisboa, S. F., Terzian, A. L., Resstel, L. B., and Guimaraes, F. S. (2009). Antiaversive effects of cannabinoids: is the periaqueductal gray involved? Neural Plast. 2009, 625469.
Moreira, F. A., Aguiar, D. C., and Guimaraes, F. S. (2006). Anxiolytic-like effect of cannabidiol in the rat Vogel conflict test. Prog. Neuropsychopharmacol. Biol. Psychiatry 30, 1466–1471.
Moreira, F. A., Aguiar, D. C., and Guimaraes, F. S. (2007). Anxiolytic-like effect of cannabinoids injected into the rat dorsolateral periaqueductal gray. Neuropharmacology 52, 958–965.
Moreira, F. A., and Guimarães, F. S. (2005). Cannabidiol inhibits the hyperlocomotion induced by psychotomimetic drugs in mice. Eur. J. Pharmacol. 512, 199–205.
Moreira, F. A., and Lutz, B. (2008). The endocannabinoid system: emotion, learning and addiction. Addict. Biol. 13, 196–212.
Moreira, F. A., and Wotjak, C. T. (2010). Cannabinoids and anxiety. Curr. Top. Behav. Neurosci. 2, 429–450.
Morgan, C. J., and Curran, H. V. (2008). Effects of cannabidiol on schizophrenia-like symptoms in people who use cannabis. Br. J. Psychiatry 192, 306–307.
Morgan, N. H., Stanford, I. M., and Woodhall, G. L. (2009). Functional CB2 type cannabinoid receptors at CNS synapses. Neuropharmacology 57, 356–368.
Muller, T. D., Reichwald, K., Bronner, G., Kirschner, J., Nguyen, T. T., Scherag, A., Herzog, W., Herpertz-Dahlmann, B., Lichtner, P., Meitinger, T., Platzer, M., Schafer, H., Hebebrand, J., and Hinney, A. (2008). Lack of association of genetic variants in genes of the endocannabinoid system with anorexia nervosa. Child Adolesc. Psychiatry Ment. Health 2, 33.
Muller-Vahl, K. R., and Emrich, H. M. (2008). Cannabis and schizophrenia: towards a cannabinoid hypothesis of schizophrenia. Expert Rev. Neurother. 8, 1037–1048.
Munro, S., Thomas, K. L., and Abu-Shaar, M. (1993). Molecular characterization of a peripheral receptor for cannabinoids. Nature 365, 61–65.
Naruse, T., Amano, H., and Koizumi, Y. (1991). Possible involvement of dopamine D-1 and D-2 receptors in diazepam-induced hyperphagia in rats. Fundam. Clin. Pharmacol. 5, 677–693.
Navarro, M., Hernandez, E., Munoz, R. M., Del Arco, I., Villanua, M. A., Carrera, M. R., and Rodriguez De Fonseca, F. (1997). Acute administration of the CB1 cannabinoid receptor antagonist SR 141716A induces anxiety-like responses in the rat. Neuroreport 8, 491–496.
Nelson, K., Walsh, D., Deeter, P., and Sheehan, F. (1994). A phase II study of delta-9-tetrahydrocannabinol for appetite stimulation in cancer-associated anorexia. J. Palliat. Care 10, 14–18.
Nestler, E. J., Barrot, M., Dileone, R. J., Eisch, A. J., Gold, S. J., and Monteggia, L. M. (2002). Neurobiology of depression. Neuron 34, 13–25.
Newell, K. A., Deng, C., and Huang, X. F. (2006). Increased cannabinoid receptor density in the posterior cingulate cortex in schizophrenia. Exp. Brain Res. 172, 556–560.
Onaivi, E. S. (2006). Neuropsychobiological evidence for the functional presence and expression of cannabinoid CB2 receptors in the brain. Neuropsychobiology 54, 231–246.
Onaivi, E. S., Green, M. R., and Martin, B. R. (1990). Pharmacological characterization of cannabinoids in the elevated plus maze. J. Pharmacol. Exp. Ther. 253, 1002–1009.
Onaivi, E. S., Ishiguro, H., Gong, J. P., Patel, S., Meozzi, P. A., Myers, L., Perchuk, A., Mora, Z., Tagliaferro, P. A., Gardner, E., Brusco, A., Akinshola, B. E., Hope, B., Lujilde, J., Inada, T., Iwasaki, S., Macharia, D., Teasenfitz, L., Arinami, T., and Uhl, G. R. (2008a). Brain neuronal CB2 cannabinoid receptors in drug abuse and depression: from mice to human subjects. PLoS ONE 3, e1640. doi: 10.1371/journal.pone.0001640
Onaivi, E. S., Ishiguro, H., Gong, J. P., Patel, S., Meozzi, P. A., Myers, L., Perchuk, A., Mora, Z., Tagliaferro, P. A., Gardner, E., Brusco, A., Akinshola, B. E., Liu, Q. R., Chirwa, S. S., Hope, B., Lujilde, J., Inada, T., Iwasaki, S., Macharia, D., Teasenfitz, L., Arinami, T., and Uhl, G. R. (2008b). Functional expression of brain neuronal CB2 cannabinoid receptors are involved in the effects of drugs of abuse and in depression. Ann. N. Y. Acad. Sci. 1139, 434–449.
Onaivi, E. S., Ishiguro, H., Gong, J. P., Patel, S., Perchuk, A., Meozzi, P. A., Myers, L., Mora, Z., Tagliaferro, P., Gardner, E., Brusco, A., Akinshola, B. E., Liu, Q. R., Hope, B., Iwasaki, S., Arinami, T., Teasenfitz, L., and Uhl, G. R. (2006). Discovery of the presence and functional expression of cannabinoid CB2 receptors in brain. Ann. N. Y. Acad. Sci. 1074, 514–536.
Ortega-Alvaro, A., Aracil-Fernandez, A., García-Gutiérrez, M. S., Navarrete, F., and Manzanares, J. (2011). Deletion of CB2 cannabinoid receptor induces schizophrenia-related behaviors in mice. Neuropsychopharmacology 36, 1489–1504.
O’Sullivan, S. E., and Kendall, D. A. (2010). Cannabinoid activation of peroxisome proliferator-activated receptors: potential for modulation of inflammatory disease. Immunobiology 215, 611–616.
Pacher, P., Batkai, S., and Kunos, G. (2006). The endocannabinoid system as an emerging target of pharmacotherapy. Pharmacol. Rev. 58, 389–462.
Pagotto, U., Marsicano, G., Cota, D., Lutz, B., and Pasquali, R. (2006). The emerging role of the endocannabinoid system in endocrine regulation and energy balance. Endocr. Rev. 27, 73–100.
Pamplona, F. A., and Takahashi, R. N. (2011). Psychopharmacology of the endocannabinoids: far beyond anandamide. J. Psychopharmacol. PMID: 21652605. [Epub ahead of print].
Parolaro, D., Realini, N., Vigano, D., Guidali, C., and Rubino, T. (2010). The endocannabinoid system and psychiatric disorders. Exp. Neurol. 224, 3–14.
Peralta, V., Campos, M. S., De Jalon, E. G., and Cuesta, M. J. (2010). Motor behavior abnormalities in drug-naive patients with schizophrenia spectrum disorders. Mov. Disord. 25, 1068–1076.
Pertwee, R. G. (2008). The diverse CB1 and CB2 receptor pharmacology of three plant cannabinoids: delta9-tetrahydrocannabinol, cannabidiol and delta9-tetrahydrocannabivarin. Br. J. Pharmacol. 153, 199–215.
Petitet, F., Jeantaud, B., Reibaud, M., Imperato, A., and Dubroeucq, M. C. (1998). Complex pharmacology of natural cannabinoids: evidence for partial agonist activity of delta9-tetrahydrocannabinol and antagonist activity of cannabidiol on rat brain cannabinoid receptors. Life Sci. 63, PL1–PL6.
Piomelli, D., Tarzia, G., Duranti, A., Tontini, A., Mor, M., Compton, T. R., Dasse, O., Monaghan, E. P., Parrott, J. A., and Putman, D. (2006). Pharmacological profile of the selective FAAH inhibitor KDS-4103 (URB597). CNS Drug Rev. 12, 21–38.
Racz, I., Nadal, X., Alferink, J., Banos, J. E., Rehnelt, J., Martin, M., Pintado, B., Gutierrez-Adan, A., Sanguino, E., Bellora, N., Manzanares, J., Zimmer, A., and Maldonado, R. (2008a). Interferon-gamma is a critical modulator of CB(2) cannabinoid receptor signaling during neuropathic pain. J. Neurosci. 28, 12136–12145.
Racz, I., Nadal, X., Alferink, J., Banos, J. E., Rehnelt, J., Martin, M., Pintado, B., Gutierrez-Adan, A., Sanguino, E., Manzanares, J., Zimmer, A., and Maldonado, R. (2008b). Crucial role of CB(2) cannabinoid receptor in the regulation of central immune responses during neuropathic pain. J. Neurosci. 28, 12125–12135.
Rapoport, J. L., Addington, A. M., Frangou, S., and Psych, M. R. (2005). The neurodevelopmental model of schizophrenia: update 2005. Mol. Psychiatry 10, 434–449.
Reibaud, M., Obinu, M. C., Ledent, C., Parmentier, M., Bohme, G. A., and Imperato, A. (1999). Enhancement of memory in cannabinoid CB1 receptor knock-out mice. Eur. J. Pharmacol. 379, R1–R2.
Reich, C. G., Taylor, M. E., and Mccarthy, M. M. (2009). Differential effects of chronic unpredictable stress on hippocampal CB1 receptors in male and female rats. Behav. Brain Res. 203, 264–269.
Resstel, L. B., Joca, S. R., Moreira, F. A., Correa, F. M., and Guimaraes, F. S. (2006). Effects of cannabidiol and diazepam on behavioral and cardiovascular responses induced by contextual conditioned fear in rats. Behav. Brain Res. 172, 294–298.
Resstel, L. B., Tavares, R. F., Lisboa, S. F., Joca, S. R., Correa, F. M., and Guimaraes, F. S. (2009). 5-HT1A receptors are involved in the cannabidiol-induced attenuation of behavioural and cardiovascular responses to acute restraint stress in rats. Br. J. Pharmacol. 156, 181–188.
Robinson, S. A., Loiacono, R. E., Christopoulos, A., Sexton, P. M., and Malone, D. T. (2010). The effect of social isolation on rat brain expression of genes associated with endocannabinoid signaling. Brain Res. 1343, 153–167.
Roche, R., Hoareau, L., Bes-Houtmann, S., Gonthier, M. P., Laborde, C., Baron, J. F., Haffaf, Y., Cesari, M., and Festy, F. (2006). Presence of the cannabinoid receptors, CB1 and CB2, in human omental and subcutaneous adipocytes. Histochem. Cell Biol. 126, 177–187.
Rodgers, R. J., Haller, J., Halasz, J., and Mikics, E. (2003). “One-trial sensitization” to the anxiolytic-like effects of cannabinoid receptor antagonist SR141716A in the mouse elevated plus-maze. Eur. J. Neurosci. 17, 1279–1286.
Rodriguez-Gaztelumendi, A., Rojo, M. L., Pazos, A., and Diaz, A. (2009). Altered CB receptor-signaling in prefrontal cortex from an animal model of depression is reversed by chronic fluoxetine. J. Neurochem. 108, 1423–1433.
Rosenstock, J., Hollander, P., Chevalier, S., and Iranmanesh, A. (2008). SERENADE: the Study Evaluating Rimonabant Efficacy in Drug-naive Diabetic Patients: effects of monotherapy with rimonabant, the first selective CB1 receptor antagonist, on glycemic control, body weight, and lipid profile in drug-naive type 2 diabetes. Diabetes Care 31, 2169–2176.
Ross, C. A., Margolis, R. L., Reading, S. A., Pletnikov, M., and Coyle, J. T. (2006). Neurobiology of schizophrenia. Neuron 52, 139–153.
Rubin, D. C., Berntsen, D., and Bohni, M. K. (2008). A memory-based model of posttraumatic stress disorder: evaluating basic assumptions underlying the PTSD diagnosis. Psychol. Rev. 115, 985–1011.
Russo, E. B., Burnett, A., Hall, B., and Parker, K. K. (2005). Agonistic properties of cannabidiol at 5-HT1a receptors. Neurochem. Res. 30, 1037–1043.
Sanchez, C., Galve-Roperh, I., Canova, C., Brachet, P., and Guzman, M. (1998). Delta9-tetrahydrocannabinol induces apoptosis in C6 glioma cells. FEBS Lett. 436, 6–10.
Sanchis-Segura, C., Cline, B. H., Marsicano, G., Lutz, B., and Spanagel, R. (2004). Reduced sensitivity to reward in CB1 knockout mice. Psychopharmacology (Berl.) 176, 223–232.
Schatz, A. R., Lee, M., Condie, R. B., Pulaski, J. T., and Kaminski, N. E. (1997). Cannabinoid receptors CB1 and CB2: a characterization of expression and adenylate cyclase modulation within the immune system. Toxicol. Appl. Pharmacol. 142, 278–287.
Scheen, A. J. (2008). CB1 receptor blockade and its impact on cardiometabolic risk factors: overview of the RIO programme with rimonabant. J. Neuroendocrinol. 20(Suppl. 1), 139–146.
Schneider, M. (2008). Puberty as a highly vulnerable developmental period for the consequences of cannabis exposure. Addict. Biol. 13, 253–263.
Schneider, M., Drews, E., and Koch, M. (2005). Behavioral effects in adult rats of chronic prepubertal treatment with the cannabinoid receptor agonist WIN 55,212-2. Behav. Pharmacol. 16, 447–454.
Sciolino, N. R., Bortolato, M., Eisenstein, S. A., Fu, J., Oveisi, F., Hohmann, A. G., and Piomelli, D. (2010). Social isolation and chronic handling alter endocannabinoid signaling and behavioral reactivity to context in adult rats. Neuroscience 168, 371–386.
Seifert, J., Ossege, S., Emrich, H. M., Schneider, U., and Stuhrmann, M. (2007). No association of CNR1 gene variations with susceptibility to schizophrenia. Neurosci. Lett. 426, 29–33.
Sheline, Y. I. (2000). 3D MRI studies of neuroanatomic changes in unipolar major depression: the role of stress and medical comorbidity. Biol. Psychiatry 48, 791–800.
Shin, L. M., and Liberzon, I. (2010). The neurocircuitry of fear, stress, and anxiety disorders. Neuropsychopharmacology 35, 169–191.
Siegfried, Z., Kanyas, K., Latzer, Y., Karni, O., Bloch, M., Lerer, B., and Berry, E. M. (2004). Association study of cannabinoid receptor gene (CNR1) alleles and anorexia nervosa: differences between restricting and binging/purging subtypes. Am. J. Med. Genet. B Neuropsychiatr. Genet. 125B, 126–130.
Silveira Filho, N. G., and Tufik, S. (1981). Comparative effects between cannabidiol and diazepam on neophobia, food intake and conflict behavior. Res. Commun. Psychol. Psychiatr. Behav. 6, 251–266.
Soares, P., Campos, A. C., Bortoli, V. C., Zangrossi, H. Jr., Guimaraes, F. S., and Zuardi, A. W. (2010). Intra-dorsal periaqueductal gray administration of cannabidiol blocks panic-like response by activating 5-HT1A receptors. Behav. Brain Res. 213, 225–229.
Solowij, N., and Battisti, R. (2008). The chronic effects of cannabis on memory in humans: a review. Curr. Drug Abuse Rev. 1, 81–98.
Solowij, N., and Michie, P. T. (2007). Cannabis and cognitive dysfunction: parallels with endophenotypes of schizophrenia? J. Psychiatry Neurosci. 32, 30–52.
Starowicz, K., Nigam, S., and Di Marzo, V. (2007). Biochemistry and pharmacology of endovanilloids. Pharmacol. Ther. 114, 13–33.
Steffens, S., Veillard, N. R., Arnaud, C., Pelli, G., Burger, F., Staub, C., Karsak, M., Zimmer, A., Frossard, J. L., and Mach, F. (2005). Low dose oral cannabinoid therapy reduces progression of atherosclerosis in mice. Nature 434, 782–786.
Steiner, M. A., Wanisch, K., Monory, K., Marsicano, G., Borroni, E., Bachli, H., Holsboer, F., Lutz, B., and Wotjak, C. T. (2008). Impaired cannabinoid receptor type 1 signaling interferes with stress-coping behavior in mice. Pharmacogenomics J. 8, 196–208.
Stoving, R. K., Andries, A., Brixen, K., Flyvbjerg, A., Horder, K., and Frystyk, J. (2009). Leptin, ghrelin, and endocannabinoids: potential therapeutic targets in anorexia nervosa. J. Psychiatr. Res. 43, 671–679.
Strober, M., Schmidt-Lackner, S., Freeman, R., Bower, S., Lampert, C., and Deantonio, M. (1995). Recovery and relapse in adolescents with bipolar affective illness: a five-year naturalistic, prospective follow-up. J. Am. Acad. Child Adolesc. Psychiatry 34, 724–731.
Substance Abuse Mental Health Services Administration SAMHSA. (2006). Results from the 2005 National Survey on Drug Use and Health: National Finding. Office of Applied Studies, NSDUH Series H-30, DHHS (Publication No. SMA 06-4194), Rockville, MD.
Suarez, J., Llorente, R., Romero-Zerbo, S. Y., Mateos, B., Bermudez-Silva, F. J., De Fonseca, F. R., and Viveros, M. P. (2009). Early maternal deprivation induces gender-dependent changes on the expression of hippocampal CB(1) and CB(2) cannabinoid receptors of neonatal rats. Hippocampus 19, 623–632.
Sugranyes, G., Flamarique, I., Parellada, E., Baeza, I., Goti, J., Fernandez-Egea, E., and Bernardo, M. (2009). Cannabis use and age of diagnosis of schizophrenia. Eur. Psychiatry 24, 282–286.
Sundram, S. (2006). Cannabis and neurodevelopment: implications for psychiatric disorders. Hum Psychopharmacol 21, 245–254.
Terzian, A. L., Aguiar, D. C., Guimaraes, F. S., and Moreira, F. A. (2009). Modulation of anxiety-like behaviour by Transient Receptor Potential Vanilloid Type 1 (TRPV1) channels located in the dorsolateral periaqueductal gray. Eur. Neuropsychopharmacol. 19, 188–195.
Thomas, A., Baillie, G. L., Phillips, A. M., Razdan, R. K., Ross, R. A., and Pertwee, R. G. (2007). Cannabidiol displays unexpectedly high potency as an antagonist of CB1 and CB2 receptor agonists in vitro. Br. J. Pharmacol. 150, 613–623.
Thomas, A., Burant, A., Bui, N., Graham, D., Yuva-Paylor, L. A., and Paylor, R. (2009). Marble burying reflects a repetitive and perseverative behavior more than novelty-induced anxiety. Psychopharmacology (Berl.) 204, 361–373.
Thomas, B. F., Gilliam, A. F., Burch, D. F., Roche, M. J., and Seltzman, H. H. (1998). Comparative receptor binding analyses of cannabinoid agonists and antagonists. J. Pharmacol. Exp. Ther. 285, 285–292.
Tsai, S. J., Wang, Y. C., and Hong, C. J. (2000). Association study of a cannabinoid receptor gene (CNR1) polymorphism and schizophrenia. Psychiatr. Genet. 10, 149–151.
Tyrka, A. R., Wier, L., Price, L. H., Ross, N., Anderson, G. M., Wilkinson, C. W., and Carpenter, L. L. (2008). Childhood parental loss and adult hypothalamic-pituitary-adrenal function. Biol. Psychiatry 63, 1147–1154.
Ueda, N., Tsuboi, K., Uyama, T., and Ohnishi, T. (2011). Biosynthesis and degradation of the endocannabinoid 2-arachidonoylglycerol. Biofactors 37, 1–7.
Ujike, H., and Morita, Y. (2004). New perspectives in the studies on endocannabinoid and cannabis: cannabinoid receptors and schizophrenia. J. Pharmacol. Sci. 96, 376–381.
Uriguen, L., Garcia-Fuster, M. J., Callado, L. F., Morentin, B., La Harpe, R., Casado, V., Lluis, C., Franco, R., Garcia-Sevilla, J. A., and Meana, J. J. (2009). Immunodensity and mRNA expression of A2A adenosine, D2 dopamine, and CB1 cannabinoid receptors in postmortem frontal cortex of subjects with schizophrenia: effect of antipsychotic treatment. Psychopharmacology (Berl.) 206, 313–324.
Urigüen, L., Garc’ia-Gutírrez, M. S., and Manzanares, J. (2011). Decreased GABAA and GABAB receptor functional activity in cannabinoid CB1 receptor knockout mice. J. Psychopharmacol. (Oxford) 25, 105–110.
Urigüen, L., Perez-Rial, S., Ledent, C., Palomo, T., and Manzanares, J. (2004). Impaired action of anxiolytic drugs in mice deficient in cannabinoid CB1 receptors. Neuropharmacology 46, 966–973.
Valenzano, K. J., Tafesse, L., Lee, G., Harrison, J. E., Boulet, J. M., Gottshall, S. L., Mark, L., Pearson, M. S., Miller, W., Shan, S., Rabadi, L., Rotshteyn, Y., Chaffer, S. M., Turchin, P. I., Elsemore, D. A., Toth, M., Koetzner, L., and Whiteside, G. T. (2005). Pharmacological and pharmacokinetic characterization of the cannabinoid receptor 2 agonist, GW405833, utilizing rodent models of acute and chronic pain, anxiety, ataxia and catalepsy. Neuropharmacology 48, 658–672.
Van Gaal, L., Pi-Sunyer, X., Despres, J. P., Mccarthy, C., and Scheen, A. (2008). Efficacy and safety of rimonabant for improvement of multiple cardiometabolic risk factors in overweight/obese patients: pooled 1-year data from the rimonabant in obesity (RIO) program. Diabetes Care 31(Suppl. 2), S229–S240.
Van Sickle, M. D., Duncan, M., Kingsley, P. J., Mouihate, A., Urbani, P., Mackie, K., Stella, N., Makriyannis, A., Piomelli, D., Davison, J. S., Marnett, L. J., Di Marzo, V., Pittman, Q. J., Patel, K. D., and Sharkey, K. A. (2005). Identification and functional characterization of brainstem cannabinoid CB2 receptors. Science 310, 329–332.
Verty, A. N., Evetts, M. J., Crouch, G. J., Mcgregor, I. S., Stefanidis, A., and Oldfield, B. J. (2011). The cannabinoid receptor agonist THC attenuates weight loss in a rodent model of activity-based anorexia. Neuropsychopharmacology 36, 1349–1358.
Vigano, D., Guidali, C., Petrosino, S., Realini, N., Rubino, T., Di Marzo, V., and Parolaro, D. (2009). Involvement of the endocannabinoid system in phencyclidine-induced cognitive deficits modelling schizophrenia. Int. J. Neuropsychopharmacol. 12, 599–614.
Vinod, K. Y., and Hungund, B. L. (2006). Role of the endocannabinoid system in depression and suicide. Trends Pharmacol. Sci. 27, 539–545.
Viveros, M. P., Marco, E. M., and File, S. E. (2005). Endocannabinoid system and stress and anxiety responses. Pharmacol. Biochem. Behav. 81, 331–342.
Viveros, M. P., Marco, E. M., Llorente, R., and Lopez-Gallardo, M. (2007). Endocannabinoid system and synaptic plasticity: implications for emotional responses. Neural Plast. 2007, 52908.
Volkow, N. D., and Wise, R. A. (2005). How can drug addiction help us understand obesity? Nat. Neurosci. 8, 555–560.
Waksman, Y., Olson, J. M., Carlisle, S. J., and Cabral, G. A. (1999). The central cannabinoid receptor (CB1) mediates inhibition of nitric oxide production by rat microglial cells. J. Pharmacol. Exp. Ther. 288, 1357–1366.
Walker, A. (1994). Thesaurus of Psychological Index Terms. Washington, DC: American psychological Association.
Walsh, B. T., Kaplan, A. S., Attia, E., Olmsted, M., Parides, M., Carter, J. C., Pike, K. M., Devlin, M. J., Woodside, B., Roberto, C. A., and Rockert, W. (2006). Fluoxetine after weight restoration in anorexia nervosa: a randomized controlled trial. JAMA 295, 2605–2612.
Walter, L., Franklin, A., Witting, A., Wade, C., Xie, Y., Kunos, G., Mackie, K., and Stella, N. (2003). Nonpsychotropic cannabinoid receptors regulate microglial cell migration. J. Neurosci. 23, 1398–1405.
Wegener, N., and Koch, M. (2009). Behavioural disturbances and altered Fos protein expression in adult rats after chronic pubertal cannabinoid treatment. Brain Res. 1253, 81–91.
Witkin, J. M. (2008). Animal models of obsessive-compulsive disorder. Curr. Protoc. Neurosci. 45, 9.30.1–9.30.9.
Wolf, S. A., Bick-Sander, A., Fabel, K., Leal-Galicia, P., Tauber, S., Ramirez-Rodriguez, G., Muller, A., Melnik, A., Waltinger, T. P., Ullrich, O., and Kempermann, G. (2010). Cannabinoid receptor CB1 mediates baseline and activity-induced survival of new neurons in adult hippocampal neurogenesis. Cell Commun. Signal. 8, 12.
Wotjak, C. T. (2005). Role of endogenous cannabinoids in cognition and emotionality. Mini Rev. Med. Chem. 5, 659–670.
Xing, J., and Li, J. (2007). TRPV1 receptor mediates glutamatergic synaptic input to dorsolateral periaqueductal gray (dl-PAG) neurons. J. Neurophysiol. 97, 503–511.
Yilmazer-Hanke, D. M. (2008). Morphological correlates of emotional and cognitive behaviour: insights from studies on inbred and outbred rodent strains and their crosses. Behav. Pharmacol. 19, 403–434.
Yu, H., and Chen, Z. Y. (2010). The role of BDNF in depression on the basis of its location in the neural circuitry. Acta Pharmacol. Sin. 32, 3–11.
Zamberletti, E., Vigano, D., Guidali, C., Rubino, T., and Parolaro, D. (2010). Long-lasting recovery of psychotic-like symptoms in isolation-reared rats after chronic but not acute treatment with the cannabinoid antagonist AM251. Int. J. Neuropsychopharmacol. 1–14.
Zanelati, T. V., Biojone, C., Moreira, F. A., Guimaraes, F. S., and Joca, S. R. (2010). Antidepressant-like effects of cannabidiol in mice: possible involvement of 5-HT1A receptors. Br. J. Pharmacol. 159, 122–128.
Zavitsanou, K., Garrick, T., and Huang, X. F. (2004). Selective antagonist [3H]SR141716A binding to cannabinoid CB1 receptors is increased in the anterior cingulate cortex in schizophrenia. Prog. Neuropsychopharmacol. Biol. Psychiatry 28, 355–360.
Zuardi, A. W. (2008). Cannabidiol: from an inactive cannabinoid to a drug with wide spectrum of action. Rev. Bras. Psiquiatr. 30, 271–280.
Zuardi, A. W., Cosme, R. A., Graeff, F. G., and Guimarães, F. S. (1993a). Effects of ipsapirone and cannabidiol on human experimental anxiety. J. Psychopharmacol. 7, 82–88.
Zuardi, A. W., Guimaraes, F. S., and Moreira, A. C. (1993b). Effect of cannabidiol on plasma prolactin, growth hormone and cortisol in human volunteers. Braz. J. Med. Biol. Res. 26, 213–217.
Zuardi, A. W., Crippa, J. A., Hallak, J. E., Moreira, F. A., and Guimaraes, F. S. (2006a). Cannabidiol, a Cannabis sativa constituent, as an antipsychotic drug. Braz. J. Med. Biol. Res. 39, 421–429.
Zuardi, A. W., Hallak, J. E., Dursun, S. M., Morais, S. L., Sanches, R. F., Musty, R. E., and Crippa, J. A. (2006b). Cannabidiol monotherapy for treatment-resistant schizophrenia. J. Psychopharmacol. (Oxford) 20, 683–686.
Zuardi, A. W., Crippa, J. A., Hallak, J. E., Pinto, J. P., Chagas, M. H., Rodrigues, G. G., Dursun, S. M., and Tumas, V. (2009). Cannabidiol for the treatment of psychosis in Parkinson’s disease. J. Psychopharmacol. (Oxford) 23, 979–983.
Zuardi, A. W., and Karniol, I. G. (1983). Changes in the conditioned emotional response of rats induced by Δ9-THC, CBD and mixture of the two cannabinoids. Braz. Arch. Biol. Technol. 26, 391–397.
Zuardi, A. W., Morais, S. L., Guimaraes, F. S., and Mechoulam, R. (1995). Antipsychotic effect of cannabidiol. J. Clin. Psychiatry 56, 485–486.
Zuardi, A. W., Rodrigues, J. A., and Cunha, J. M. (1991). Effects of cannabidiol in animal models predictive of antipsychotic activity. Psychopharmacology (Berl.) 104, 260–264.
Keywords: cannabinoid receptor, cannabidiol, cognition, emotion, anxiety, depression, schizophrenia, eating disorders
Citation: Marco EM, García-Gutiérrez MS, Bermúdez-Silva F-J, Moreira FA, Guimarães F, Manzanares J and Viveros M-P (2011) Endocannabinoid system and psychiatry: in search of a neurobiological basis for detrimental and potential therapeutic effects. Front. Behav. Neurosci. 5:63. doi: 10.3389/fnbeh.2011.00063
Received: 11 June 2011;
Paper pending published: 06 July 2011;
Accepted: 09 September 2011;
Published online: 05 October 2011.
Edited by:
Viviana Trezza, Roma Tre University, ItalyReviewed by:
Carsten Wotjak, Max-Planck-Institute of Psychiatry, GermanyDaniela Parolaro, University of Insubria, Italy
Copyright: © 2011 Marco, García-Gutiérrez, Bermúdez-Silva, Moreira, Guimarães, Manzanares and Viveros. This is an open-access article subject to a non-exclusive license between the authors and Frontiers Media SA, which permits use, distribution and reproduction in other forums, provided the original authors and source are credited and other Frontiers conditions are complied with.
*Correspondence: Eva M. Marco and María-Paz Viveros, Departamento de Fisiología (Fisiología Animal II), Facultad de Ciencias Biológicas, Universidad Complutense de Madrid, Ciudad Universitaria, C/Jose Antonio Novais No 2, 28040 Madrid, Spain. e-mail:cGF6dml2ZXJAYmlvLnVjbS5lcw==;ZW1tYXJjb0BiaW8udWNtLmVz