- 1Department of Neuroscience, Section Anatomy, University Medical Center Groningen, University of Groningen, Groningen, Netherlands
- 2Núcleo de Cognição e Sistemas Complexos, Centro de Matemática, Computação e Cognição, Universidade Federal do ABC, Santo André, SP, Brasil
Glucocorticoids are known to enhance the consolidation of memory of emotionally arousing experiences by acting upon a network of interconnected brain regions. Although animal studies typically do not consider the insular cortex (IC) to be part of this network, the present findings indicate that the IC is importantly involved in regulating glucocorticoid effects on memory consolidation of emotionally arousing inhibitory avoidance training. The specific glucocorticoid receptor (GR) agonist RU 28362 (3 or 10 ng in 0.5 μl) infused bilaterally into the IC of male Sprague–Dawley rats immediately after one-trial inhibitory avoidance training dose-dependently enhanced 48 h retention performance. Moreover, training on the inhibitory avoidance task increased neuronal activity of the IC, as assessed by an increased number of cells expressing immunoreactivity for phosphorylated extracellular signal-regulated kinase 1/2 (pERK1/2). However, systemic administration of a memory-enhancing dose of corticosterone (1 mg/kg) after inhibitory avoidance training rapidly reduced the number of pERK1/2-positive cells in the IC, suggesting that glucocorticoid administration reduces overall neuronal activity of the IC. To investigate which components of the inhibitory avoidance training experience were influenced by the intra-IC glucocorticoid administration, in the last experiment rats were trained on a modified inhibitory avoidance task in which context exposure and footshock training occur on two sequential days. RU 28362 administration into the IC enhanced later retention when infused immediately after either the context or footshock training. Thus, these findings indicate that the IC mediates glucocorticoid effects on the consolidation of memory of different components of inhibitory avoidance training and suggest that the IC might be an important element of the rodent brain network involved in emotional regulation of learning and memory.
Introduction
Glucocorticoid hormones—stress hormones released from the adrenal cortex—are known to strengthen the consolidation of memory of emotionally arousing experiences (de Kloet et al., 1999; Roozendaal, 2000; Joëls et al., 2006; Sandi and Pinelo-Nava, 2007; de Quervain et al., 2009; Roozendaal and McGaugh, 2011; Schwabe et al., 2012). Most animal studies investigating glucocorticoid-induced enhancement of memory consolidation examined their effects on a network of interacting brain regions involved in emotional regulation of memory, including the basolateral amygdala (BLA), prefrontal cortex and hippocampus (Micheau et al., 1984; Roozendaal and McGaugh, 1997a,b, 2011; Roozendaal et al., 2008, 2009b; Miranda et al., 2008b). Although human neuroimaging studies generally support an involvement of these brain regions, as well as their functional interaction, in emotionally influenced learning and memory (Buchel et al., 1998; Richardson et al., 2004; Marschner et al., 2008; Shin and Liberzon, 2010), they also consistently point to a key role for the insular cortex (IC) (Craig, 2009; Menon and Uddin, 2010; Shin and Liberzon, 2010; Hartley et al., 2011; Ille et al., 2011). Extensive evidence indicates that the IC, which receives autonomic, visceral, and somatosensory inputs (Saper, 1982; Augustine, 1996), might be part of a “salience network” involved in the detection of novel and salient information (Downar et al., 2002; Seeley et al., 2007; Menon and Uddin, 2010) that is collectively upregulated in response to an acute stressful event and after highly stressful experiences (van Marle et al., 2010; Hermans et al., 2011; van Wingen et al., 2011). Accordingly, increased anterior insula activity has been reported during the subjective awareness of both positive and negative emotions (Craig, 2009; Menon and Uddin, 2010) as well as during the encoding and recall of a broad spectrum of emotionally salient learning tasks (Buchel et al., 1998; Alvarez et al., 2008, 2011; Marschner et al., 2008; King et al., 2009; Rasch et al., 2009).
Contrasting the human literature, the IC never received a prominent position in animal research investigating stress hormone or emotional arousal effects on learning and memory. Although Bermudez-Rattoni and colleagues have reported several findings supporting the view that the IC might be involved herein (Bermudez-Rattoni et al., 1991, 1997, 2005; Bermudez-Rattoni and McGaugh, 1991; Nerad et al., 1996; Gutierrez et al., 1999; Miranda and Bermudez-Rattoni, 2007), most animal studies have been limited to investigating its involvement in the formation and maintenance of taste memory (Berman and Dudai, 2001; Bermudez-Rattoni et al., 2004; Shema et al., 2007; Nunez-Jaramillo et al., 2010; Stehberg et al., 2011). The present study investigated whether the IC is implicated in regulating glucocorticoid effects on the consolidation of memory of emotionally arousing inhibitory avoidance training. In the first experiment, we investigated whether the specific glucocorticoid receptor (GR) agonist RU 28362 administered into the IC immediately after one-trial inhibitory avoidance training enhances long-term retention of the training experience. Next, we examined whether a systemic injection of corticosterone, the major endogenous glucocorticoid in rodents, given immediately after inhibitory avoidance training recruits the IC and induces changes in cellular activity within this brain region. Cellular activity was assessed by determining phosphorylation levels of extracellular signal-regulated kinase 1/2 (pERK1/2), a signaling cascade implicated in neuronal activity and synaptic plasticity (Thomas and Huganir, 2004; Peng et al., 2010). To gain a better understanding of the precise role of the IC in regulating glucocorticoid effects on inhibitory avoidance memory, in the last experiment we employed a modified inhibitory avoidance procedure (Malin and McGaugh, 2006; Medina et al., 2007; Roozendaal et al., 2009b) that allows investigating which components of the inhibitory avoidance experience (i.e., memory of the context or the footshock) were influenced by the glucocorticoid infusion.
Materials and Methods
Subjects
Male adult Sprague–Dawley rats (280–320 g at time of surgery) from Charles River Breeding Laboratories (Kisslegg, Germany) were kept individually in a temperature-controlled (22°C) colony room and maintained on a standard 12 h light: 12 h dark cycle (07:00–19:00 h lights on) with ad libitum access to food and water. Training and testing were performed during the light phase of the cycle between 10:00 and 15:00 h. All procedures were in compliance with the European Community's Council Directive (86/609/EEC) and approved by the Institutional Animal Care and Use Committee of the University of Groningen, The Netherlands.
Surgery for Cannula Implantation
Animals, adapted to the vivarium for at least 1 week, were anesthetized with a subcutaneous injection of ketamine (37.5 mg/kg of body weight; Alfasan) and dexmedetomidine (0.25 mg/kg; Orion), and received the non-steroidal analgesic carprofen (4 mg/kg, s.c.; Pfizer). Oxygen (35%) mixed with ambient air was administered during surgery such that blood oxygenation levels would not drop below 90% (Fornari et al., 2012). The skull was positioned in a stereotaxic frame (Kopf Instruments), and two stainless-steel guide cannulae (15 mm; 23 gauge; Small Parts, Inc.) were implanted bilaterally with the cannula tips 2.0 mm above the anterior IC. The coordinates were based on the atlas of Paxinos and Watson (2007): anteroposterior: +1.0 mm from Bregma; mediolateral: ±5.5 mm from midline; dorsoventral: −4.8 mm from Bregma; incisor bar: −3.3 mm from interaural. The cannulae were affixed to the skull with two anchoring screws and dental cement. Stylets (15 mm long 00 insect dissection pins), inserted into each cannula to maintain patency, were removed only for the infusion of drugs. After surgery, the rats received a subcutaneous injection of 3 ml of saline to facilitate clearance of drugs and prevent dehydration, and were subsequently administered atipamezole hydrochloride (Antisedan, 0.25 mg/kg, s.c.; Orion) to reverse anesthesia. The rats were allowed to recover for a minimum of 7 days before initiation of training and were handled three times for 1 min each during this recovery period to accustom them to the infusion procedure.
Inhibitory Avoidance Apparatus and Procedures
For all experiments, rats were trained and tested in an inhibitory avoidance apparatus, consisting of a trough-shaped alley (91 cm long, 15 cm deep, 20 cm wide at the top, and 6.4 cm wide at the bottom) divided into two compartments, separated by a sliding door that opened by retracting into the floor (McGaugh et al., 1988). The starting compartment (30 cm) was made of opaque white plastic and was well lit; the shock compartment (60 cm) was made of dark, electrifiable metal plates and was not illuminated. Training and testing were conducted in a sound- and light-attenuated room.
For one-trial inhibitory avoidance training, the rats were placed in the starting compartment of the apparatus, facing away from the door, and were allowed to enter the dark (shock) compartment. After the rat stepped completely into the dark compartment, the sliding door was closed and a single inescapable footshock (0.35 mA; 1 s) was delivered. The rats were removed from the shock compartment 15 s later and, after drug treatment, returned to their home cages. For the modified, two-phase inhibitory avoidance procedure (Malin and McGaugh, 2006; Roozendaal et al., 2009b), on the first day (context training), the rat was placed into the starting compartment, facing away from the door, and allowed to freely explore the inhibitory avoidance apparatus for 3 min. On day 2 (shock training), each rat was placed directly into the dark compartment, facing away from the starting compartment, with the retractable door closed. The rat then received an inescapable footshock (0.75 mA; 1 s) and immediately afterward was removed from the training apparatus. For both one-trial and two-phase inhibitory avoidance, retention was tested 48 h after training by placing the rat into the starting compartment of the inhibitory avoidance apparatus and measuring the latency to re-enter the former shock compartment with all four paws (maximum latency of 600 s). Longer latencies were interpreted as indicating better retention. Shock was not administered on the retention test trial.
Local Drug Infusion into the Insular Cortex
The specific GR agonist RU 28362 (11β,17β-dihydroxy-6,21-dimethyl-17α-pregna-4,6-trien-20yn-3-one, 3 or 10 ng; a generous gift of Aventis, Frankfurt, Germany) was first dissolved in 100% ethanol and subsequently diluted in 0.9% saline to reach a final ethanol concentration of 0.5%. Receptor binding studies have shown that this compound has selective and high affinity for GRs (Teutsch et al., 1981). Bilateral infusions of RU 28362 or an equivalent volume of vehicle (0.5% ethanol in saline) into the IC were given immediately after one-trial inhibitory avoidance training or after either the context or footshock components of the modified, two-phase inhibitory avoidance task by using 30 gauge injection needles connected to a 10 μl Hamilton microsyringe with polyethylene (PE-20) tubing. The injection needle protruded 2.0 mm beyond the tip of the cannula and a 0.5 μl injection volume was infused over a period of 50 s by an automated syringe pump (Stoelting Co). The injection needles were retained within the cannulae for an additional 20 s after drug infusion to maximize diffusion and to prevent backflow of drug into the cannulae. The infusion volume was based on previous findings indicating that infusion of this volume into the IC (Miranda et al., 2008a; Roozendaal et al., 2010), but not the cortex dorsal to the IC (Bermudez-Rattoni et al., 2005), modulates memory consolidation. To control for time- and site-specificity, additional groups of rats received delayed infusions of RU 28362 or vehicle into the IC 3 h after the training trial or immediate post-training infusions into the somatosensory cortex, located approximately 1 mm dorsal to the IC. The use of post-training drug administration provides direct support for the view that the treatment affects memory consolidation processes and that retention performance is, thus, not confounded by possible effects on attentional, motivational, or sensory-perceptual mechanisms at the time of training or test (McGaugh, 1966).
Systemic Corticosterone Treatment
Corticosterone (1 mg/kg, Sigma-Aldrich) or vehicle, in a volume of 2 ml/kg body weight, was given subcutaneously immediately after the training trial. Corticosterone was dissolved in 5% ethanol in saline. This dose of corticosterone is known to enhance memory consolidation of different types of training (Hui et al., 2004; Okuda et al., 2004; Miranda et al., 2008a).
Cannula Placement Verification
Rats were deeply anesthetized with an overdose of sodium pentobarbital (≈100 mg/kg, i.p.) and perfused transcardially with a 0.9% saline solution followed by 4% formaldehyde. Following decapitation, the brains were removed and immersed in fresh 4% formaldehyde. At least 24 h before sectioning, the brains were submerged in a 25% sucrose (wt/vol) solution in water for cryoprotection. Coronal sections of 50 μm were cut on a cryostat, mounted on gelatin-coated slides, and stained with cresyl violet. The sections were examined under a light microscope and determination of the location of injection needle tips in the IC was made according to the atlas plates of Paxinos and Watson (2007), by an observer blind to drug treatment condition. Rats with injection needle placements outside the IC or with extensive tissue damage at the injection needle tips were excluded from analysis.
Immunohistochemistry
Thirty minutes after training and systemic corticosterone treatment, rats were perfused transcardially with ice-cold 0.01 M phosphate-buffered saline (PBS), pH 7.4, followed by ice-cold 4% paraformaldehyde in 0.1 M phosphate buffer (PB), pH 7.4. The brains were removed, post-fixed overnight at 4°C, and then transferred to a 25% sucrose solution in 0.1 M PB for 3–6 days at 4°C. Frozen coronal sections at the level of the anterior IC (0.2–2.5 mm anterior to Bregma) were cut at a thickness of 20 μm on a cryostat and collected in Tris-buffered saline (TBS) with 0.1% sodium azide and phosphatase inhibitors (20 mM sodium fluoride and 2 mM sodium orthovanadate).
Every eighth section was used for quantification. Free-floating sections were rinsed in 0.3% Triton X-100 in TBS with phosphatase inhibitors. To block non-specific binding, sections were incubated with TBS containing phosphatase inhibitors, 0.3% Triton X-100 and 5% normal donkey serum (nds, Jackson ImmunoResearch) for 1 h. Subsequently, sections were incubated with a cocktail of primary antibodies for pERK1/2 (rabbit anti-phospho-p44/42 MAP kinase, 1:1000; cell signaling #9101) and NeuN (mouse anti-NeuN, 1:100; Millipore Mab 377) or pERK1/2 and CaMK II (mouse anti-calcium/calmodulin-dependent protein kinase II, 1:400; Millipore #05–532) in TBS containing phosphatase inhibitors, 0.3% Triton X-100 and 1% nds for 48 h at 4°C. Sections were then rinsed several times with TBS and incubated with a cocktail of specific fluorochrome-conjugated antibodies (Alexa Fluor 488 donkey anti-rabbit 1:500 and Alexa Fluor 594 donkey anti-mouse 1:1000 or Alexa Fluor 633 goat anti-rabbit 1:500 and Alexa 488 donkey anti-mouse 1:500; Invitrogen) for 2 h in the dark. Sections were rinsed again in TBS, incubated with Hoechst dye (0.1 μg/ml, Invitrogen) for 30 min and mounted on gelatin-coated slides, using non-fading mounting medium for coverslipping and stored in the dark for further analysis.
Images of each section were acquired with TissueFaxs®, Zeiss AxioObserver Z1 Microscope System (Tissue-Gnostics GmbH, Vienna, Austria). The number of pERK1/2 immunopositive nuclei was quantified with ImageJ 1.43 m software (Girish and Vijayalakshmi, 2004; Papadopulos et al., 2007). Cell counts were determined at two levels within the anterior IC (2.5–1.7 mm and 1.6–0.7 mm anterior to Bregma), according to the standard atlas plates of Paxinos and Watson (2007), and expressed as number of pERK1/2-positive nuclei per mm2. Quantitative analysis of cell counts was performed blind to treatment condition. Double-labeling was examined with a Leica SP2 AOBS confocal microscope.
Statistics
Data are expressed as mean ± SEM. Inhibitory avoidance training and retention test latencies were analyzed with One-Way ANOVAs. Further analysis used Fisher's post-hoc tests to determine the source of the detected significances. To determine whether learning had occurred, paired t-tests were used to compare the training and retention latencies. Quantitative measures of pERK1/2 immunoreactivity in the IC were analyzed with Two-Way-ANOVAs, using Training (training vs. no training) and Corticosterone treatment (vehicle vs. corticosterone) as independent factors. The analyses were followed by Fisher's post-hoc tests, when appropriate. Individual comparisons with home-cage control groups were performed with Student t-tests for independent samples. For all comparisons, a probability level of < 0.05 was accepted as statistical significance. The number of rats per group is indicated in the figure legends.
Results
GR Agonist Administration into the Insular Cortex Enhances Memory Consolidation of Inhibitory Avoidance Training
This experiment examined whether the GR agonist RU 28362 infused into the IC enhances the consolidation of memory of inhibitory avoidance training. For that, bilateral infusions of RU 28362 (3 or 10 ng in 0.5 μl) or vehicle were administered into the IC immediately after one-trial inhibitory avoidance training and retention of the training was tested 48 h later. Control groups received delayed infusions of the GR agonist into the IC 3 h after the training trial to determine whether the GR agonist enhances retention by influencing time-dependent processes underlying memory consolidation.
Average step-through latencies for all groups during training, before footshock or drug treatment, were 9.9 ± 0.8 s (mean ± SEM). One-Way ANOVA for training latencies revealed no significant differences between groups [F(2, 31) = 0.17, p = 0.84, data not shown]. Forty-eight hour retention latencies of rats administered vehicle into the IC immediately after training were significantly longer than their latencies during the training trial (paired t-test: p < 0.05), indicating that the rats retained memory of the inhibitory avoidance experience. As is shown in Figure 1A, rats treated with the GR agonist immediately after training had significantly longer retention test latencies as compared with rats that received vehicle [F(2, 31) = 3.59, p < 0.05]. Fisher's post-hoc tests revealed that the lower dose of RU 28362 (3 ng) enhanced retention (p < 0.05, compared with vehicle), whereas retention latencies of animals given the higher dose (10 ng) approached, but failed to reach, significance (p = 0.07). As is shown in Figure 1B, the GR agonist administered into the IC 3 h after the training did not significantly alter retention latencies [F(2, 16) = 0.26, p = 0.78], indicating a time-limited involvement of the IC in mediating glucocorticoid effects on the consolidation of inhibitory avoidance memory.
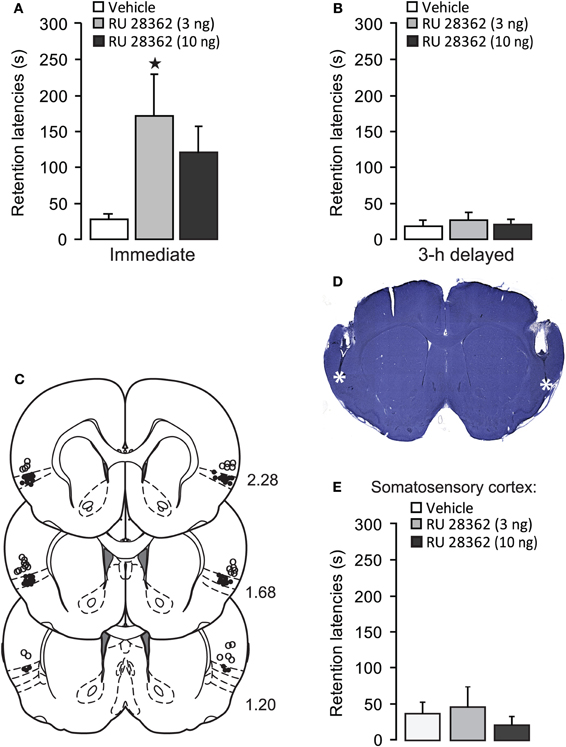
Figure 1. Glucocorticoid receptor agonist administration into the insular cortex enhances memory consolidation of inhibitory avoidance training. (A) Step-through latencies (mean ± SEM) in seconds on the 48 h inhibitory avoidance retention test of rats given bilateral infusions of the glucocorticoid receptor agonist RU 28362 (3 or 10 ng in 0.5 μl) into the IC immediately after training. *p < 0.05 as compared with the vehicle-treated group (n = 11–12 per group). (B) Step-through latencies (mean ± SEM) in seconds on the 48 h inhibitory avoidance retention test of rats given the glucocorticoid receptor agonist RU 28362 (3 or 10 ng in 0.5 μl) into the IC 3 h after training (n = 6–7 per group). (C) Location of injection needle tips within the IC of all rats included in the immediate infusions groups (black circles) and 15 rats with infusion needle tips in the somatosensory cortex as a control for site specificity (open circles). Adapted from Paxinos and Watson (2007). (D) Representative photomicrograph illustrating placement of cannulae and needle tips within the insular cortex. (E) Step-through latencies (mean ± SEM) in seconds on the 48 h inhibitory avoidance retention test of rats given bilateral infusions of the glucocorticoid receptor agonist RU 28362 (3 or 10 ng in 0.5 μl) into the somatosensory cortex, approximately 1 mm above the IC, immediately after training (n = 4–6 per group).
Figures 1C,D show cannula placement within the IC. All injection needle tips of rats included in the analysis were localized within the granular and dysgranular subdivisions of the IC. To control for site specificity, other groups of rats received immediate post-training infusions of vehicle or the GR agonist (3 or 10 ng in 0.5 μl) into the somatosensory cortex, approximately 1 mm above the IC (see, Figure 1C). One-Way ANOVA for 48 h retention latencies revealed no significant GR agonist effect [F(2, 12) = 0.29, p = 0.75, Figure 1E], indicating that the memory-modulatory effects of RU 28362 are localized within the IC.
Systemic Corticosterone Administration Immediately after One-Trial Inhibitory Avoidance Training Reduces pERK1/2-Immunoreactivity in the Insular Cortex
The findings described above indicate that direct pharmacological activation of GRs in the IC after inhibitory avoidance training enhances the consolidation of memory of this experience in a dose-, time- and site-specific manner. However, these findings do not indicate whether circulating glucocorticoids normally act upon the IC in regulating memory consolidation. Therefore, the next experiment examined whether a systemic injection of corticosterone after inhibitory avoidance training changes neuronal activity of the IC. Rats received a subcutaneous injection of corticosterone (1 mg/kg) or vehicle immediately after one-trial inhibitory avoidance training (or without training) and were sacrificed 30 min later. Brains were processed to investigate training- and drug-induced changes in the number of pERK1/2-positive cells at two locations within the anterior IC. ERK1/2, a member of the mitogen-activated protein (MAP) kinase family, is considered to be phosphorylated by elevated neuronal activity and synaptic plasticity (Thomas and Huganir, 2004; Peng et al., 2010). Home-cage control groups did not receive any training or drug treatment. Other rats received the same post-training injection of corticosterone or vehicle and their retention was tested 48 h later.
As is shown in Figure 2A, post-training injection of this dose of corticosterone significantly enhanced 48 h retention latencies (p < 0.05). Figure 2B shows the pattern of pERK1/2 expression in the IC. Immunoreactivity for pERK1/2 was found in somata as well as fibers (Figure 2C). Qualitative double-labeling indicated that the vast majority of pERK1/2-positive cells in the IC also showed immunoreactivity for the neuronal marker NeuN (Figure 2C) or CaMKII (Figure 2D), a marker for glutamatergic pyramidal cells (McDonald et al., 2002). These observations strongly suggest that pERK1/2 immunoreactivity within somata was mainly expressed in glutamate-rich pyramidal cells. Because most pERK1/2 immunoreactivity was concentrated in the superficial layers (II–III) of the agranular and dysgranular IC, cell counts were restricted to these regions, at two levels (2.5–1.7 mm and 1.6–0.7 mm anterior to Bregma), according to the standard atlas plates of Paxinos and Watson (2007). Figure 2E shows the number of pERK1/2-positive cells at these two levels of the IC of rats treated with corticosterone or vehicle immediately after inhibitory avoidance training or of rats that were not trained. Across all groups, the number of pERK1/2-positive cells at the more rostral level appeared to be higher than that at the more caudal level. At the rostral level, Two-Way ANOVA indicated a significant training effect [F(1, 14) = 11.63, p < 0.01] but no corticosterone effect [F(1, 14) = 1.95, p = 0.18] or interaction effect between these two parameters [F(1, 14) = 0.53, p = 0.48]. Fisher's post-hoc analyses indicated that rats that were trained on the inhibitory avoidance task and subsequently treated with vehicle had more pERK1/2-positive cells than non-trained vehicle-treated rats (p < 0.05). Corticosterone administration after inhibitory avoidance training did not significantly alter the number of pERK1/2-positive cells as compared to vehicle-treated rats. When compared to home-cage controls, student t-test analyses indicated that rats treated with either vehicle (p < 0.05) or corticosterone (p < 0.05) immediately after training had more pERK1/2-positive cells, whereas the number of pERK1/2-positive cells of non-trained rats administered vehicle or corticosterone did not differ from those of home-cage controls.
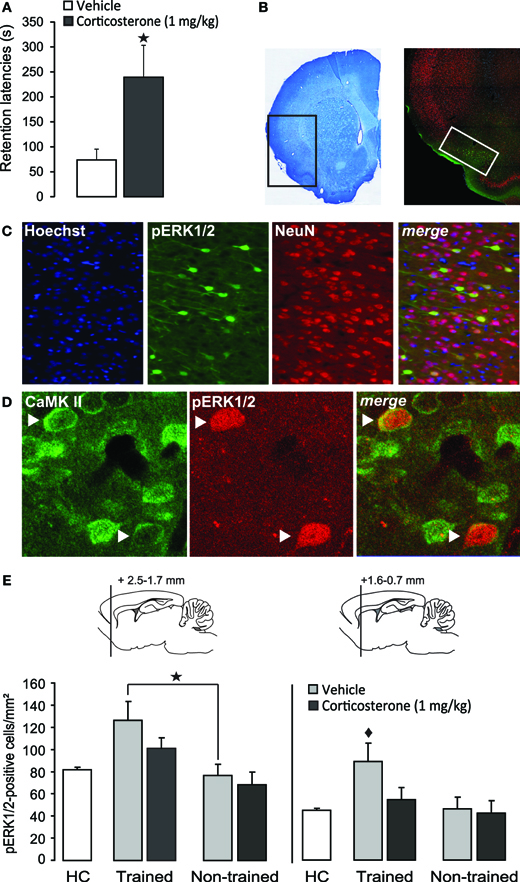
Figure 2. Systemic injection of a memory-enhancing dose of corticosterone after one-trial inhibitory avoidance training reduces pERK1/2-immunoreactivity in the insular cortex.(A) Step-through latencies (mean ± SEM) in seconds on a 48 h inhibitory avoidance retention test of rats given a subcutaneous injection of corticosterone (1 mg/kg) or vehicle immediately after training. *p < 0.05 (n = 8–9 per group). (B) Example of a Nissl-stained coronal section depicting the region that was acquired for the analyses of immunostaining and the distribution pattern of pERK1/2 expression in the insular cortex. The white square indicates the region analyzed for cell counts. (C) Triple localization of Hoechst (blue), pERK1/2 (green) and the neuronal marker NeuN (red) in the insular cortex. Merging the three channels demonstrates that all pERK1/2-positive cells exhibit immunoreactivity for NeuN. (D) Dual localization of pERK1/2 (red) and CamKII (green) immunoreactivity in the insular cortex. Merging of the red and green channels demonstrates that pERK1/2-positive cells also showed immunoreactivity for CamKII. Arrows point to cells with combined pERK1/2 and CamKII immunoreactivity. (E) Number of pERK1/2-positive cells (mean ± SEM) at two levels within the insular cortex as assessed 30 min after inhibitory avoidance training and systemic injection of corticosterone (n = 4) or vehicle (n = 4). Non-trained rats received an injection of corticosterone (n = 5) or vehicle (n = 5) but were not trained on the inhibitory avoidance task. Home-cage (HC) control rats (n = 6) did not receive any training or systemic injection. *p < 0.05 as compared with non-trained vehicle-treated rats. ♦p < 0.01 as compared with all other groups.
On the other hand, Two-Way ANOVA for the number of pERK1/2-positive cells at the more caudal level revealed significant effects of training [F(1, 14) = 21.66, p < 0.01], corticosterone treatment [F(1, 14) = 10.55, p < 0.01] and the interaction between them [F(1, 14) = 6.64, p < 0.05, Figure 2E]. Fisher's post-hoc analyses indicated that trained rats that were subsequently injected with vehicle had significantly more pERK1/2-positive cells than rats that were administered corticosterone after training (p < 0.01) or non-trained rats treated with either vehicle or corticosterone (p < 0.01). When compared to home-cage controls, the group that received vehicle immediately after training was the only one that showed increased cell counts (p < 0.0001). These findings indicate that training on the inhibitory avoidance task increases the number of pERK1/2-positive cells within this area of the IC, and that post-training administration of a memory-enhancing dose of corticosterone resulted in a significant decrease in the number of pERK1/2-positive cells (as compared to vehicle-treated trained rats), which did not differ significantly from home-cage controls or non-trained rats.
GR Agonist Infusions into the Insular Cortex Enhance Memory for both the Context and Footshock Components of Inhibitory Avoidance Training
The findings described above indicate that the IC is an important target structure for glucocorticoids in regulating memory consolidation of inhibitory avoidance training. However, with one-trial inhibitory avoidance it is not possible to investigate the relative involvement of a brain region in memory consolidation of the contextual information independently from that of the footshock. To address this issue, a modified two-phase inhibitory avoidance training procedure had been developed in which context training and footshock training occur on two sequential days (Malin and McGaugh, 2006; Medina et al., 2007; Roozendaal et al., 2009b). In the next experiment, we used this modified inhibitory avoidance training procedure to investigate whether GR agonist administration into the IC enhances memory consolidation of contextual information, the footshock experience, or of both components of training.
Figure 3 shows 48 h retention latencies of rats administered the GR agonist (3 or 10 ng in 0.5 μl) into the IC after either the context or footshock component of inhibitory avoidance training. One-Way ANOVAs for retention latencies revealed significant group effects of rats given intra-IC infusions of RU 28362 immediately after either context [F(2, 37) = 3.50, p < 0.05] or footshock training [F (2, 37) = 4.04, p < 0.05]. Retention latencies of rats given intra-IC infusions of the 3 and 10 ng doses of RU 28362 immediately after context training (both, p < 0.05), or infusions of the 3 ng dose of RU 28362 after footshock training (p < 0.01) were significantly longer than those of their respective vehicle controls. Importantly, as is shown in Table 1, both doses of RU 28362 administered into the IC after either context or shock training to animals that did not receive the other component of training did not enhance 48 h retention latencies [context: F(2, 15) = 0.70, p = 0.51; footshock: F(2, 15) = 0.88, p = 0.44], indicating that the expression of the enhanced memory depends on the learning about both context and footshock.
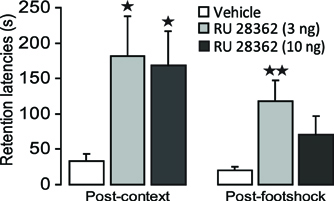
Figure 3. Glucocorticoid receptor agonist infusions into the insular cortex enhance memory consolidation of both the context and footshock components of inhibitory avoidance training. Step-through latencies (mean ± SEM) in seconds on the 48 h inhibitory avoidance retention test of rats given bilateral infusions of the glucocorticoid receptor agonist RU 28362 (3 or 10 ng in 0.5 μl) into the insular cortex immediately after either context or shock training on the two-phase modified inhibitory avoidance task (n = 12–14 per group). *p < 0.05, **p < 0.01, as compared with the corresponding vehicle-treated group.
Discussion
The aim of this study was to investigate whether the IC is involved in regulating glucocorticoid effects on memory consolidation of inhibitory avoidance training. Our findings indicate that the specific GR agonist RU 28362 infused bilaterally into the IC after inhibitory avoidance training induced dose- and time-dependent enhancement of the consolidation of memory of this training experience. Moreover, training on the inhibitory avoidance task increased neuronal activity of the IC, as assessed with immunoreactivity for pERK1/2. Unexpectedly, systemic injection of a memory-enhancing dose of corticosterone after inhibitory avoidance training rapidly reduced pERK1/2 expression in the IC. Lastly, we show that post-training activation of GRs in the IC enhanced the consolidation of memory of both the footshock and contextual components of inhibitory avoidance training. These findings provide evidence that the IC is an important target structure for glucocorticoids in regulating the consolidation of different components of inhibitory avoidance memory.
It is well established that glucocorticoid hormones, via GR activation, enhance memory consolidation of emotionally arousing training experiences (de Kloet et al., 1999; Sandi and Pinelo-Nava, 2007; Roozendaal et al., 2009a; Schwabe et al., 2012). However, most studies investigating glucocorticoid effects on the formation and stabilization of memory, and its molecular underpinnings, examined glucocorticoid actions on a network of interconnected brain regions such as the BLA and hippocampus (Revest et al., 2005; Sandi and Pinelo-Nava, 2007; Roozendaal et al., 2009b; Groeneweg et al., 2011). Surprisingly little is known concerning glucocorticoid effects on the IC or other cortical regions in regulating learning and memory. Our finding that RU 28362 infused into the IC immediately after inhibitory avoidance training enhanced later retention is in line with other recent evidence indicating that corticosterone or GR agonist administration into the IC also enhances the consolidation of memory of conditioned taste aversion (Miranda et al., 2008a) and object recognition training (Roozendaal et al., 2010). More generally, findings from human and, to a lesser extent, animal studies indicate that the IC is involved in memory formation of emotionally arousing experiences. Human neuroimaging studies reported activation of the IC during the encoding of aversive conditioning paradigms (Buchel et al., 1998; Alvarez et al., 2008, 2011; Marschner et al., 2008). In rats, lesions of the IC, made before training, disrupted memory of emotionally arousing water-maze spatial (Bermudez-Rattoni et al., 1991; Nerad et al., 1996) and inhibitory avoidance training (Bermudez-Rattoni and McGaugh, 1991). Although such neuroimaging studies in humans and permanent lesion studies in animals obviously cannot determine whether the memory effects were attributable to specific influences on memory consolidation, our findings using immediate post-training or delayed drug delivery provide compelling evidence for the view that the IC is involved in time-dependent processes underlying the consolidation of memory (Bermudez-Rattoni et al., 1991, 2005; Gutierrez et al., 1999; Miranda and McGaugh, 2004; Roozendaal et al., 2010).
To investigate whether inhibitory avoidance training normally recruits the IC, we examined the pattern of pERK1/2 expression in this cortical region 30 min after inhibitory avoidance training. ERK is a rapidly activated protein that has been implicated in neuronal activity as well as neuroplasticity and memory consolidation (Atkins et al., 1998; Schafe et al., 2000; Thomas and Huganir, 2004). Exposure to the inhibitory avoidance training procedure increased the number of pERK1/2-positive cells within the IC of rats treated with vehicle. Qualitative immuno-staining indicated that most pERK1/2-positive cells were glutamate-rich pyramidal cells. These findings are consistent with the evidence that ERK1/2 is activated in neurons in response to excitatory glutamatergic activity (Fiore et al., 1993; Xia et al., 1996), and suggest that the elevated pERK1/2 immunoreactivity reflects an increased neuronal activity within this region (Fujita et al., 2010). Such findings are in agreement with the above-mentioned human neuroimaging studies indicating enhanced IC activity during affective processing and the encoding of emotionally arousing information (Buchel et al., 1998; Alvarez et al., 2008, 2011; Marschner et al., 2008; King et al., 2009; Rasch et al., 2009; Hermans et al., 2011). The pattern of pERK1/2 expression in the present study was similar to that of Kobayashi et al. (2010) reporting that novel sucrose stimulation increased the number of pERK1/2-positive cells in the IC, when compared to sucrose-experienced rats. As in our study, they found that pyramidal neurons, but not GABAergic interneurons, in the upper layers of the IC were most susceptible to ERK1/2 phosphorylation. However, the difference between naïve and sucrose-experienced rats was only found in the gustatory region—dysgranular and granular subdivisions—of the IC (Kobayashi et al., 2010), whereas in the present study most pERK1/2 immunoreactivity was concentrated in the agranular and dysgranular regions, and not in the gustatory region. Other studies also indicated increased pERK1/2 levels in the IC in response to an unfamiliar taste stimulus (Berman et al., 1998, 2000), and that such activation is necessary for taste learning (Berman et al., 1998). These findings suggest that ERK1/2 activation in the IC may contribute to the detection of novelty and/or memory formation (Berman et al., 1998; Bermudez-Rattoni, 2004; Kobayashi et al., 2010).
A more puzzling observation is that a memory-enhancing dose of corticosterone administered immediately after inhibitory avoidance training resulted in a rapid decrease in the number of pERK1/2-positive cells within the IC. Corticosterone administration to non-trained rats did not induce such a reduction. The selective influence of corticosterone on trained, and not naïve, rats is consistent with prior evidence indicating that RU 28362 infused into the IC interacts with training-induced noradrenergic activation in enhancing memory consolidation (Roozendaal et al., 2010). However, the direction of the corticosterone effect on pERK1/2 immunoreactivity appears to contrast prior evidence indicating that glucocorticoid effects on memory consolidation involving other brain regions require an upregulation of pERK1/2-MAP kinase activity. Previously, we reported that a memory-enhancing dose of RU 28362 infused into either the BLA or medial prefrontal cortex after inhibitory avoidance training rapidly elevated pERK1/2 levels in the other brain site (medial prefrontal cortex or BLA, respectively) (Roozendaal et al., 2009b). Moreover, stress and corticosterone are known to interact with training-associated glutamatergic activity within the hippocampus to increase the expression and enzymatic activity of the MAP kinase pathway (Revest et al., 2005; Gutierrez-Mecinas et al., 2011). A blockade of pERK1/2 signaling with a MEK inhibitor infused into any of these brain regions prevented glucocorticoid effects on memory consolidation. Thus, although we only examined pERK1/2 immunoreactivity in somata, and not in fibers, these findings suggest that glucocorticoids, at least at a 30 min time interval, might exert an opposite influence on the pERK1/2-MAP-kinase pathway, and possibly neuronal activity, in the IC as compared to the BLA or hippocampus. Emerging evidence supports the view that glucocorticoids can activate or suppress synaptic plasticity and neuronal activity in a highly time-dependent and brain region-specific manner (Joëls et al., 2011).
Our finding that corticosterone-induced enhancement of inhibitory avoidance memory is associated with a reduced pERK1/2 expression, and possibly neuronal activity, of the IC is in agreement with that of a recent functional magnetic resonance imaging study in humans showing that the combined oral administration of cortisol and the noradrenergic stimulant yohimbine shortly before the encoding of emotionally arousing pictures led to a strong deactivation of the IC, along with the hippocampus and orbitofrontal cortex (van Stegeren et al., 2010). Moreover, the magnitude of this deactivation correlated with enhanced recall of the material assessed 1 week later. Highly comparable, another study reported that human participants who responded with a large increase in cortisol when confronted with a psychosocial stressor, as opposed to low-responders, also showed deactivation of a network of limbic regions that includes the right anterior insula (Pruessner et al., 2008). The crucial question is whether and how such overall deactivation of the IC might contribute to the enhanced consolidation of memory processing. Pruessner et al. (2008) interpreted the deactivation of limbic regions in their study as a stress-mediated regulatory influence on hypothalamic-pituitary-adrenal (HPA) axis activity. However, no findings are available indicating whether or not the IC per se is involved in HPA-axis regulation. Alternatively, based on the involvement of frontal areas, including the anterior IC, in emotional regulation, attention, and focusing (Dolcos et al., 2004, 2007), van Stegeren et al. (2010) suggested that a reduced BOLD signal in these areas could reflect either a loss of top-down inhibition, and therefore activation (disinhibition) of other brain regions, or an increased signal-to-noise ratio, resulting in a shift of attentional processing from a peripherally to a centrally focused view. In line with this hypothesis, others have proposed that the anterior IC is part of a “salience network” which detects salient stimuli (events) and triggers appropriate control signals to regulate behavior and homeostatic state (Downar et al., 2002; Seeley et al., 2007; Menon and Uddin, 2010). It is possible, therefore, that a reduced neuronal activity of the IC after exogenous glucocorticoid administration induces a “reallocation of resources,” thereby increasing the detection of the most relevant stimuli and enhancing the consolidation of memory of these experiences (Cahill and van Stegeren, 2003; van Stegeren et al., 2010).
Such a potential role of the IC, as part of the salience network, in novelty and salience detection fits well with our finding that local activation of GRs with RU 28362 enhanced the memory of exposure to both the contextual and aversive components of inhibitory avoidance training. Similarly, findings of human neuroimaging studies indicated activation of the IC in fear conditioning studies during the presentation of either specific conditioned stimuli or context (Buchel et al., 1998, 1999; Alvarez et al., 2008; Marschner et al., 2008). A broad involvement of the IC in regulating the consolidation of memory of emotionally salient experiences fits also well with other findings of animal studies indicating that post-training pharmacological manipulation of IC activity modulates memory consolidation of training on many different kinds of learning tasks, including spatial water-maze (Gutierrez et al., 1999), conditioned taste aversion (Miranda and McGaugh, 2004; Miranda et al., 2008a,b; Shema et al., 2009) and object recognition (Bermudez-Rattoni et al., 2005; Roozendaal et al., 2010). The extensive network of connections between the IC and other cortical regions, including the prefrontal, cingulate, perirhinal and entorhinal cortices (Mufson et al., 1981; Augustine, 1996; Shi and Cassell, 1998b; Hoistad and Barbas, 2008), might account for such a general modulatory influence on memory. There are also dense reciprocal connections between the IC and BLA (McDonald and Jackson, 1987; Shi and Cassell, 1998a,b). Extensive evidence indicates that the BLA influences memory consolidation of emotionally arousing training experiences by regulating neuroplasticity and information storage processes in other brain regions, including the hippocampus and dorsal striatum (McGaugh, 2002; Roozendaal and McGaugh, 2011). Therefore, it is possible that the IC and BLA share a functional commonality and cooperate in regulating memory consolidation. Indeed, some findings provide evidence for a necessary interaction between the IC and BLA in strengthening the consolidation of memory of emotionally arousing training. Post-training infusion of a cAMP analog into the IC is known to enhance memory consolidation of both conditioned taste aversion and inhibitory avoidance training. However, concurrent blockade of noradrenergic transmission in the BLA with the β-adrenoceptor antagonist propranolol prevents this memory enhancement (Miranda and McGaugh, 2004). Findings of human studies indicating an increased connectivity between the amygdala and IC during the encoding of emotionally arousing material (Rasch et al., 2009) support a functional interaction between the BLA and IC in regulating memory consolidation.
In summary, the present findings provide evidence for the view that the IC is importantly involved in regulating glucocorticoid effects on memory consolidation of emotionally arousing inhibitory avoidance training. These findings indicate that the IC deserves a prominent position in animal research investigating the neural basis of emotional regulation of learning and memory.
Conflict of Interest Statement
The authors declare that the research was conducted in the absence of any commercial or financial relationships that could be construed as a potential conflict of interest.
Acknowledgments
We thank James L. McGaugh, Bruce S. McEwen, and Guillén Fernández for critical reading of the manuscript and Klaas Sjollema for technical assistance with microscopical imaging, performed at the UMCG Imaging Center (UMIC), which is supported by the Netherlands Organization for Health Research and Development (ZonMW grant 40–00506–98–9021). Research was supported by IBRO Fellowship 2009 (RVF).
References
Alvarez, R. P., Biggs, A., Chen, G., Pine, D. S., and Grillon, C. (2008). Contextual fear conditioning in humans: cortical-hippocampal and amygdala contributions. J. Neurosci. 28, 6211–6219.
Alvarez, R. P., Chen, G., Bodurka, J., Kaplan, R., and Grillon, C. (2011). Phasic and sustained fear in humans elicits distinct patterns of brain activity. Neuroimage 55, 389–400.
Atkins, C. M., Selcher, J. C., Petraitis, J. J., Trzaskos, J. M., and Sweatt, J. D. (1998). The MAPK cascade is required for mammalian associative learning. Nat. Neurosci. 1, 602–609.
Augustine, J. R. (1996). Circuitry and functional aspects of the insular lobe in primates including humans. Brain Res. Brain Res. Rev. 22, 229–244.
Berman, D. E., and Dudai, Y. (2001). Memory extinction, learning anew, and learning the new: dissociations in the molecular machinery of learning in cortex. Science 291, 2417–2419.
Berman, D. E., Hazvi, S., Neduva, V., and Dudai, Y. (2000). The role of identified neurotransmitter systems in the response of insular cortex to unfamiliar taste: activation of ERK1-2 and formation of a memory trace. J. Neurosci. 20, 7017–7023.
Berman, D. E., Hazvi, S., Rosenblum, K., Seger, R., and Dudai, Y. (1998). Specific and differential activation of mitogen-activated protein kinase cascades by unfamiliar taste in the insular cortex of the behaving rat. J. Neurosci. 18, 10037–10044.
Bermudez-Rattoni, F. (2004). Molecular mechanisms of taste-recognition memory. Nat. Rev. Neurosci. 5, 209–217.
Bermudez-Rattoni, F., Introini-Collison, I., Coleman-Mesches, K., and McGaugh, J. L. (1997). Insular cortex and amygdala lesions induced after aversive training impair retention: effects of degree of training. Neurobiol. Learn. Mem. 67, 57–63.
Bermudez-Rattoni, F., Introini-Collison, I. B., and McGaugh, J. L. (1991). Reversible inactivation of the insular cortex by tetrodotoxin produces retrograde and anterograde amnesia for inhibitory avoidance and spatial learning. Proc. Natl. Acad. Sci. U.S.A. 88, 5379–5382.
Bermudez-Rattoni, F., and McGaugh, J. L. (1991). Insular cortex and amygdala lesions differentially affect acquisition on inhibitory avoidance and conditioned taste aversion. Brain Res. 549, 165–170.
Bermudez-Rattoni, F., Okuda, S., Roozendaal, B., and McGaugh, J. L. (2005). Insular cortex is involved in consolidation of object recognition memory. Learn. Mem. 12, 447–449.
Bermudez-Rattoni, F., Ramirez-Lugo, L., Gutierrez, R., and Miranda, M. I. (2004). Molecular signals into the insular cortex and amygdala during aversive gustatory memory formation. Cell. Mol. Neurobiol. 24, 25–36.
Buchel, C., Dolan, R. J., Armony, J. L., and Friston, K. J. (1999). Amygdala-hippocampal involvement in human aversive trace conditioning revealed through event-related functional magnetic resonance imaging. J. Neurosci. 19, 10869–10876.
Buchel, C., Morris, J., Dolan, R. J., and Friston, K. J. (1998). Brain systems mediating aversive conditioning: an event-related fMRI study. Neuron 20, 947–957.
Cahill, L., and van Stegeren, A. (2003). Sex-related impairment of memory for emotional events with beta-adrenergic blockade. Neurobiol. Learn. Mem. 79, 81–88.
Craig, A. D. (2009). How do you feel–now? The anterior insula and human awareness. Nat. Rev. Neurosci. 10, 59–70.
de Kloet, E. R., Oitzl, M. S., and Joëls, M. (1999). Stress and cognition: are corticosteroids good or bad guys? Trends Neurosci. 22, 422–426.
de Quervain, D. J., Aerni, A., Schelling, G., and Roozendaal, B. (2009). Glucocorticoids and the regulation of memory in health and disease. Front. Neuroendocrinol. 30, 358–370.
Dolcos, F., Labar, K. S., and Cabeza, R. (2004). Dissociable effects of arousal and valence on prefrontal activity indexing emotional evaluation and subsequent memory: an event-related fMRI study. Neuroimage 23, 64–74.
Dolcos, F., Miller, B., Kragel, P., Jha, A., and McCarthy, G. (2007). Regional brain differences in the effect of distraction during the delay interval of a working memory task. Brain Res. 1152, 171–181.
Downar, J., Crawley, A. P., Mikulis, D. J., and Davis, K. D. (2002). A cortical network sensitive to stimulus salience in a neutral behavioral context across multiple sensory modalities. J. Neurophysiol. 87, 615–620.
Fiore, R. S., Murphy, T. H., Sanghera, J. S., Pelech, S. L., and Baraban, J. M. (1993). Activation of p42 mitogen-activated protein kinase by glutamate receptor stimulation in rat primary cortical cultures. J. Neurochem. 61, 1626–1633.
Fornari, R. V., Wichmann, R., Atsak, P., Atucha, E., Barsegyan, A., Beldjoud, H., Messanvi, F., Thuring, C. M., and Roozendaal, B. (2012). Rodent stereotaxic surgery and animal welfare outcome improvements for behavioral neuroscience. J. Vis. Exp. 59:e3528. doi: 10.3791/3528.
Fujita, S., Adachi, K., Koshikawa, N., and Kobayashi, M. (2010). Spatiotemporal dynamics of excitation in rat insular cortex: intrinsic corticocortical circuit regulates caudal-rostro excitatory propagation from the insular to frontal cortex. Neuroscience 165, 278–292.
Girish, V., and Vijayalakshmi, A. (2004). Affordable image analysis using NIH Image/ImageJ. Indian J. Cancer 41, 47.
Groeneweg, F. L., Karst, H., De Kloet, E. R., and Joëls, M. (2011). Rapid non-genomic effects of corticosteroids and their role in the central stress response. J. Endocrinol. 209, 153–167.
Gutierrez-Mecinas, M., Trollope, A. F., Collins, A., Morfett, H., Hesketh, S. A., Kersante, F., and Reul, J. M. (2011). Long-lasting behavioral responses to stress involve a direct interaction of glucocorticoid receptors with ERK1/2-MSK1-Elk-1 signaling. Proc. Natl. Acad. Sci. U.S.A. 108, 13806–13811.
Gutierrez, H., Hernandez-Echeagaray, E., Ramirez-Amaya, V., and Bermudez-Rattoni, F. (1999). Blockade of N-methyl-D-aspartate receptors in the insular cortex disrupts taste aversion and spatial memory formation. Neuroscience 89, 751–758.
Hartley, C. A., Fischl, B., and Phelps, E. A. (2011). Brain structure correlates of individual differences in the acquisition and inhibition of conditioned fear. Cereb. Cortex 21, 1954–1962.
Hermans, E. J., van Marle, H. J., Ossewaarde, L., Henckens, M. J., Qin, S., van Kesteren, M. T., Schoots, V. C., Cousijn, H., Rijpkema, M., Oostenveld, R., and Fernandez, G. (2011). Stress-related noradrenergic activity prompts large-scale neural network reconfiguration. Science 334, 1151–1153.
Hoistad, M., and Barbas, H. (2008). Sequence of information processing for emotions through pathways linking temporal and insular cortices with the amygdala. Neuroimage 40, 1016–1033.
Hui, G. K., Figueroa, I. R., Poytress, B. S., Roozendaal, B., McGaugh, J. L., and Weinberger, N. M. (2004). Memory enhancement of classical fear conditioning by post-training injections of corticosterone in rats. Neurobiol. Learn. Mem. 81, 67–74.
Ille, R., Schafer, A., Scharmuller, W., Enzinger, C., Schoggl, H., Kapfhammer, H. P., and Schienle, A. (2011). Emotion recognition and experience in Huntington disease: a voxel-based morphometry study. J. Psychiatry Neurosci. 36, 383–390.
Joëls, M., Fernandez, G., and Roozendaal, B. (2011). Stress and emotional memory: a matter of timing. Trends Cogn. Sci. 15, 280–288.
Joëls, M., Pu, Z., Wiegert, O., Oitzl, M. S., and Krugers, H. J. (2006). Learning under stress: how does it work? Trends Cogn. Sci. 10, 152–158.
King, A. P., Abelson, J. L., Britton, J. C., Phan, K. L., Taylor, S. F., and Liberzon, I. (2009). Medial prefrontal cortex and right insula activity predict plasma ACTH response to trauma recall. Neuroimage 47, 872–880.
Kobayashi, M., Fujita, S., Takei, H., Song, L., Chen, S., Suzuki, I., Yoshida, A., Iwata, K., and Koshikawa, N. (2010). Functional mapping of gustatory neurons in the insular cortex revealed by pERK-immunohistochemistry and in vivo optical imaging. Synapse 64, 323–334.
Malin, E. L., and McGaugh, J. L. (2006). Differential involvement of the hippocampus, anterior cingulate cortex, and basolateral amygdala in memory for context and footshock. Proc. Natl. Acad. Sci. U.S.A. 103, 1959–1963.
Marschner, A., Kalisch, R., Vervliet, B., Vansteenwegen, D., and Buchel, C. (2008). Dissociable roles for the hippocampus and the amygdala in human cued versus context fear conditioning. J. Neurosci. 28, 9030–9036.
McDonald, A. J., and Jackson, T. R. (1987). Amygdaloid connections with posterior insular and temporal cortical areas in the rat. J. Comp. Neurol. 262, 59–77.
McDonald, A. J., Muller, J. F., and Mascagni, F. (2002). GABAergic innervation of alpha type II calcium/calmodulin-dependent protein kinase immunoreactive pyramidal neurons in the rat basolateral amygdala. J. Comp. Neurol. 446, 199–218.
McGaugh, J. L. (2002). Memory consolidation and the amygdala: a systems perspective. Trends Neurosci. 25, 456–461.
McGaugh, J. L., Introini-Collison, I. B., and Nagahara, A. H. (1988). Memory-enhancing effects of posttraining naloxone: involvement of beta-noradrenergic influences in the amygdaloid complex. Brain Res. 446, 37–49.
Medina, A. C., Charles, J. R., Espinoza-Gonzalez, V., Sanchez-Resendis, O., Prado-Alcala, R. A., Roozendaal, B., and Quirarte, G. L. (2007). Glucocorticoid administration into the dorsal striatum facilitates memory consolidation of inhibitory avoidance training but not of the context or footshock components. Learn. Mem. 14, 673–677.
Menon, V., and Uddin, L. Q. (2010). Saliency, switching, attention and control: a network model of insula function. Brain Struct. Funct. 214, 655–667.
Micheau, J., Destrade, C., and Soumireu-Mourat, B. (1984). Time-dependent effects of posttraining intrahippocampal injections of corticosterone on retention of appetitive learning tasks in mice. Eur. J. Pharmacol. 106, 39–46.
Miranda, M. I., and Bermudez-Rattoni, F. (2007). Cholinergic activity in the insular cortex is necessary for acquisition and consolidation of contextual memory. Neurobiol. Learn. Mem. 87, 343–351.
Miranda, M. I., and McGaugh, J. L. (2004). Enhancement of inhibitory avoidance and conditioned taste aversion memory with insular cortex infusions of 8-Br-cAMP: involvement of the basolateral amygdala. Learn. Mem. 11, 312–317.
Miranda, M. I., Quirarte, G. L., Rodriguez-Garcia, G., McGaugh, J. L., and Roozendaal, B. (2008a). Glucocorticoids enhance taste aversion memory via actions in the insular cortex and basolateral amygdala. Learn. Mem. 15, 468–476.
Miranda, M. I., Rodriguez-Garcia, G., Reyes-Lopez, J. V., Ferry, B., and Ferreira, G. (2008b). Differential effects of beta-adrenergic receptor blockade in basolateral amygdala or insular cortex on incidental and associative taste learning. Neurobiol. Learn. Mem. 90, 54–61.
Mufson, E. J., Mesulam, M. M., and Pandya, D. N. (1981). Insular interconnections with the amygdala in the rhesus monkey. Neuroscience 6, 1231–1248.
Nerad, L., Ramirez-Amaya, V., Ormsby, C. E., and Bermudez-Rattoni, F. (1996). Differential effects of anterior and posterior insular cortex lesions on the acquisition of conditioned taste aversion and spatial learning. Neurobiol. Learn. Mem. 66, 44–50.
Nunez-Jaramillo, L., Ramirez-Lugo, L., Herrera-Morales, W., and Miranda, M. I. (2010). Taste memory formation: latest advances and challenges. Behav. Brain Res. 207, 232–248.
Okuda, S., Roozendaal, B., and McGaugh, J. L. (2004). Glucocorticoid effects on object recognition memory require training-associated emotional arousal. Proc. Natl. Acad. Sci. U.S.A. 101, 853–858.
Papadopulos, F., Spinelli, M., Valente, S., Foroni, L., Orrico, C., Alviano, F., and Pasquinelli, G. (2007). Common tasks in microscopic and ultrastructural image analysis using ImageJ. Ultrastruct. Pathol. 31, 401–407.
Peng, S., Zhang, Y., Zhang, J., Wang, H., and Ren, B. (2010). ERK in learning and memory: a review of recent research. Int. J. Mol. Sci. 11, 222–232.
Pruessner, J. C., Dedovic, K., Khalili-Mahani, N., Engert, V., Pruessner, M., Buss, C., Renwick, R., Dagher, A., Meaney, M. J., and Lupien, S. (2008). Deactivation of the limbic system during acute psychosocial stress: evidence from positron emission tomography and functional magnetic resonance imaging studies. Biol. Psychiatry 63, 234–240.
Rasch, B., Spalek, K., Buholzer, S., Luechinger, R., Boesiger, P., Papassotiropoulos, A., and de Quervain, D. J. (2009). A genetic variation of the noradrenergic system is related to differential amygdala activation during encoding of emotional memories. Proc. Natl. Acad. Sci. U.S.A. 106, 19191–19196.
Revest, J. M., Di Blasi, F., Kitchener, P., Rouge-Pont, F., Desmedt, A., Turiault, M., Tronche, F., and Piazza, P. V. (2005). The MAPK pathway and Egr-1 mediate stress-related behavioral effects of glucocorticoids. Nat. Neurosci. 8, 664–672.
Richardson, M. P., Strange, B. A., and Dolan, R. J. (2004). Encoding of emotional memories depends on amygdala and hippocampus and their interactions. Nat. Neurosci. 7, 278–285.
Roozendaal, B. (2000). Glucocorticoids and the regulation of memory consolidation. Psychoneuroendocrinology 25, 213–238.
Roozendaal, B., Barsegyan, A., and Lee, S. (2008). Adrenal stress hormones, amygdala activation, and memory for emotionally arousing experiences. Prog. Brain Res. 167, 79–97.
Roozendaal, B., Hernandez, A., Cabrera, S. M., Hagewoud, R., Malvaez, M., Stefanko, D. P., Haettig, J., and Wood, M. A. (2010). Membrane-associated glucocorticoid activity is necessary for modulation of long-term memory via chromatin modification. J. Neurosci. 30, 5037–5046.
Roozendaal, B., and McGaugh, J. L. (1997a). Basolateral amygdala lesions block the memory-enhancing effect of glucocorticoid administration in the dorsal hippocampus of rats. Eur. J. Neurosci. 9, 76–83.
Roozendaal, B., and McGaugh, J. L. (1997b). Glucocorticoid receptor agonist and antagonist administration into the basolateral but not central amygdala modulates memory storage. Neurobiol. Learn. Mem. 67, 176–179.
Roozendaal, B., McEwen, B. S., and Chattarji, S. (2009a). Stress, memory and the amygdala. Nat. Rev. Neurosci. 10, 423–433.
Roozendaal, B., McReynolds, J. R., van der Zee, E. A., Lee, S., McGaugh, J. L., and McIntyre, C. K. (2009b). Glucocorticoid effects on memory consolidation depend on functional interactions between the medial prefrontal cortex and basolateral amygdala. J. Neurosci. 29, 14299–14308.
Sandi, C., and Pinelo-Nava, M. T. (2007). Stress and memory: behavioral effects and neurobiological mechanisms. Neural Plast. 2007, 78970.
Saper, C. B. (1982). Convergence of autonomic and limbic connections in the insular cortex of the rat. J. Comp. Neurol. 210, 163–173.
Schafe, G. E., Atkins, C. M., Swank, M. W., Bauer, E. P., Sweatt, J. D., and Ledoux, J. E. (2000). Activation of ERK/MAP kinase in the amygdala is required for memory consolidation of pavlovian fear conditioning. J. Neurosci. 20, 8177–8187.
Schwabe, L., Joëls, M., Roozendaal, B., Wolf, O. T., and Oitzl, M. S. (2012). Stress effects on memory: an update and integration. Neurosci. Biobehav. Rev. [epub ahead of print].
Seeley, W. W., Menon, V., Schatzberg, A. F., Keller, J., Glover, G. H., Kenna, H., Reiss, A. L., and Greicius, M. D. (2007). Dissociable intrinsic connectivity networks for salience processing and executive control. J. Neurosci. 27, 2349–2356.
Shema, R., Hazvi, S., Sacktor, T. C., and Dudai, Y. (2009). Boundary conditions for the maintenance of memory by PKMzeta in neocortex. Learn. Mem. 16, 122–128.
Shema, R., Sacktor, T. C., and Dudai, Y. (2007). Rapid erasure of long-term memory associations in the cortex by an inhibitor of PKM zeta. Science 317, 951–953.
Shi, C. J., and Cassell, M. D. (1998a). Cascade projections from somatosensory cortex to the rat basolateral amygdala via the parietal insular cortex. J. Comp. Neurol. 399, 469–491.
Shi, C. J., and Cassell, M. D. (1998b). Cortical, thalamic, and amygdaloid connections of the anterior and posterior insular cortices. J. Comp. Neurol. 399, 440–468.
Shin, L. M., and Liberzon, I. (2010). The neurocircuitry of fear, stress, and anxiety disorders. Neuropsychopharmacology 35, 169–191.
Stehberg, J., Moraga-Amaro, R., and Simon, F. (2011). The role of the insular cortex in taste function. Neurobiol. Learn. Mem. 96, 130–135.
Teutsch, G., Costerousse, G., Deraedt, R., Benzoni, J., Fortin, M., and Philibert, D. (1981). 17 alpha-alkynyl-11 beta, 17-dihydroxyandrostane derivatives: a new class of potent glucocorticoids. Steroids 38, 651–665.
Thomas, G. M., and Huganir, R. L. (2004). MAPK cascade signalling and synaptic plasticity. Nat. Rev. Neurosci. 5, 173–183.
van Marle, H. J., Hermans, E. J., Qin, S., and Fernandez, G. (2010). Enhanced resting-state connectivity of amygdala in the immediate aftermath of acute psychological stress. Neuroimage 53, 348–354.
van Stegeren, A. H., Roozendaal, B., Kindt, M., Wolf, O. T., and Joëls, M. (2010). Interacting noradrenergic and corticosteroid systems shift human brain activation patterns during encoding. Neurobiol. Learn. Mem. 93, 56–65.
van Wingen, G. A., Geuze, E., Vermetten, E., and Fernandez, G. (2011). Perceived threat predicts the neural sequelae of combat stress. Mol. Psychiatry 16, 664–671.
Keywords: stress, emotional memory, corticosterone, pERK, insula, glucocorticoid receptor
Citation: Fornari RV, Wichmann R, Atucha E, Desprez T, Eggens-Meijer E and Roozendaal B (2012) Involvement of the insular cortex in regulating glucocorticoid effects on memory consolidation of inhibitory avoidance training. Front. Behav. Neurosci. 6:10. doi: 10.3389/fnbeh.2012.00010
Received: 20 January 2012; Paper pending published: 14 February 2012;
Accepted: 28 February 2012; Published online: 15 March 2012.
Edited by:
Antonella Gasbarri, University of l'Aquila, ItalyReviewed by:
Federico Bermudez-Rattoni, Universidad Nacional Autónoma de México, MexicoIván Izquierdo, Pontifícia Universidade Católica do Rio Grande do Sul, Brazil
Copyright: © 2012 Fornari, Wichmann, Atucha, Desprez, Eggens-Meijer and Roozendaal. This is an open-access article distributed under the terms of the Creative Commons Attribution Non Commercial License, which permits non-commercial use, distribution, and reproduction in other forums, provided the original authors and source are credited.
*Correspondence: Benno Roozendaal, Department of Neuroscience, Section Anatomy, University Medical Center Groningen, University of Groningen, Antonius Deusinglaan 1, 9713 AV Groningen, Netherlands. e-mail:Yi5yb296ZW5kYWFsQHVtY2cubmw=
† These authors contributed equally to this work.