- 1Department of the Interventional Medical Center, the Affiliated Hospital of Qingdao University, Qingdao, China
- 2Qingdao Maternal and Child Health and Family Planning Service Center, Qingdao, China
- 3Medical Research Center, The Affiliated Hospital of Qingdao University, Qingdao, China
- 4Qingdao Cancer Institute, Qingdao University, Qingdao, China
Early detection of liver diseases holds paramount importance in optimizing treatment outcomes and prognosis, thereby significantly enhancing the likelihood of recovery while mitigating the risk of progression to liver cancer. Liver diseases encompass a spectrum of conditions, each potentially manifesting distinct enzymatic profiles. Monitoring these enzymes in situ facilitates timely intervention and therapeutic management. In recent years, the field of biosensor technology has witnessed remarkable advancement, owing to strides in biomedicine and computational sciences. Biosensors have garnered widespread utility across medical and biological domains, spanning the detection of disease biomarkers, drug release tracking, ion imaging, and fluorescence imaging within living organisms. These applications have markedly enhanced imaging resolution and have the potential to refine disease diagnosis accuracy for clinicians. A pivotal aspect in the successful application of this technology lies in the construction of fluorescence probes adept at swiftly and selectively identifying target enzymes by amalgamating liver disease enzymes with fluorescence probe technology. However, research in this niche area remains relatively scarce. Building upon this foundational understanding, the present review delineates the utilization of biosensors in the early diagnosis of liver disease. Serving as a theoretical framework, this review envisages the development of high-performance biosensors tailored for the early detection of liver cancer. Furthermore, it offers insights into the potential of biosensor technology to progress and broaden its practical applications, thus contributing to the advancement of diagnostic methodologies in liver disease management.
1 Introduction
The liver serves as the central hub for metabolic activities in the body, orchestrating the conversion of diverse substances, storage of glucose, and secretion of proteins (Asanuma et al., 2015; Asrani et al., 2019; Boursier et al., 2023). As a primary detoxification powerhouse, it possesses the remarkable ability to transform toxic substances into non-toxic or highly soluble compounds, facilitating their safe elimination from the body and safeguarding them against potential harm (Gonçalves et al., 2019; Fabrellas et al., 2020; Fallowfield and Ramachandran, 2021). These pivotal functions render the liver susceptible to pathological changes (Mitchell et al., 2011; Sohrabpour et al., 2012; Schuppan et al., 2018). Liver diseases can be instigated by a variety of factors, including viral infections, medications, organic toxins, ischemia, and autoimmune diseases (Zhang et al., 2020; Sung et al., 2021). Hepatocellular carcinoma (HCC), a prevalent and highly lethal liver disease, currently stands as the sixth most common cancer worldwide and ranks second among malignant tumors associated with cancer-related deaths (Zoubek et al., 2017; Author Anonymous, 2019). Detecting liver cancer in its early stages is of paramount importance. Early diagnosis not only optimizes treatment outcomes but also significantly enhances the survival rate for patients (Wang et al., 2023a). Therefore, the timely identification of liver cancer emerges as a critical factor in elevating the cure rate of this disease (Wang et al., 2023b).
Biomarkers, which are overexpressed in abnormal tissues and diseased cells, hold significant clinical relevance. Extensive clinical trials and practices have underscored the utility of monitoring biomarkers in tissues, serum, and excreta, including reactive oxygen species (ROS), enzymes, miRNAs, and antigens, for disease screening and prognostic evaluation (Li et al., 2015; Liu et al., 2019; Li et al., 2021; Li et al., 2022). Enzymes, in particular, have emerged as highly promising biomarkers, garnering substantial attention owing to their disease specificity and pivotal physiological and clinical roles (Long et al., 2012; Li et al., 2019; Guo et al., 2020; Tang et al., 2020). Notably, the detection of enzyme markers has assumed paramount importance in the strategy for early disease detection (Liu et al., 2018; Yang et al., 2020). For instance, monoamine oxidase B (MAO-B) has been found to be overexpressed in patients with early liver fibrosis, rendering it a promising early marker for liver fibrosis. Similarly, α-L-fucosylase (AFU) exhibits elevated activity in the early stages of liver cancer, preceding lesion formation, thus positioning it as an ideal biomarker for early liver cancer detection (Liu et al., 2018). Additionally, alkaline phosphatase (ALP) enjoys widespread recognition as a biomarker for liver cancer. Therefore, the development of optimal methods for real-time and in situ monitoring of enzymes holds the potential to significantly enhance the early and accurate diagnosis of liver diseases (Cheng et al., 2019; Han et al., 2021). To adeptly surveil these biochemical signals within biological entities, the scientific community has engendered an extensive array of organic fluorescent materials (OFMs) tailored for biosensor applications (Wu et al., 2011; Ma et al., 2015; Wang et al., 2016; Zhou et al., 2018; Lim et al., 2021). In juxtaposition with traditional imaging modalities, OFMs proffer a myriad of benefits, encompassing heightened biocompatibility, augmented resolution, and the capacity for real-time monitoring (Alifu et al., 2018; Wang et al., 2020; Geng et al., 2020; Zhang et al., 2022). Specifically, biosensors employing OFMs facilitate extended signal monitoring, thereby enabling meticulous exploration into the dynamics of vital physiological processes (Liu et al., 2017; He et al., 2018). This attribute is particularly advantageous for the longitudinal study of chronic pathologies such as neoplasms and diabetes mellitus (Lu et al., 2014; Qu et al., 2019). The merits of employing OFM-based biosensors also include the diminution of patient discomfort during therapeutic interventions and a notable enhancement in diagnostic precision when contrasted with conventional imaging techniques (Zhang et al., 2016; Zhang et al., 2019).
Building on this foundational substrate, the present scholarly review rigorously delineates the integration of biosensor technologies in the expedient identification of hepatic pathologies. While the existing corpus of literature has perfunctorily engaged with the topic of biosensors, a limited body of work has thoroughly investigated their application in the precocious detection of liver maladies, especially within the ambit of clinical diagnostics. This critique aspires to ameliorate this scholarly void by providing a comprehensive discourse on the myriad sensor architectures and design philosophies implemented in the creation of biosensors specifically designed for this intent. In concert with delineating a diverse array of sensor architectures and design methodologies, this examination delves into varied design strategies for biosensors, reflecting on their imminent clinical applicabilities. Conclusively, this review posits itself as an intellectual edifice for the development of high-precision biosensors aimed at the early identification of liver cancer. It transcends a mere exposition of current trends in biosensor technology to chart out prospective pathways for their enhancement and application in the realm of hepatic disease diagnosis. Additionally, Table 1 provides a snapshot of recent studies on biosensors for reference and further exploration.
2 Liver injury enzyme
Enzymes recognized as highly promising biomarkers, have garnered considerable attention owing to their disease-specific specificity and vital physiological and clinical roles (Nguyen et al., 1991; Okamura et al., 2000; Llovet et al., 2008; Wang et al., 2020; Bhaskar et al., 2023). Notably, the detection of enzyme biomarkers has emerged as a pivotal strategy for the early diagnosis of liver diseases (Lee, 2007; Xiong et al., 2019; Sun et al., 2021; Enbanathan and Iyer, 2022). Liver injury, exemplified by drug-induced liver injury (DILI), represents a significant pathological concern that has emerged as a prominent public health issue (Board and Anders, 2007; Board and Menon, 2013; McDonald and Tipton, 2022). DILI predominantly arises from accidental or intentional medication overdoses, consequently ranking among the leading causes of acute hepatic impairment. Recent advances in biomedical research have underscored the excessive generation of highly reactive oxygen/nitrogen species (ROS/RNS), notably ONOO−, within mitochondria following medication overdose, thereby precipitating severe hepatotoxicity (DiMasi, 2001; Rudin, 2005; Bertolini et al., 2006; Uetrecht, 2010; Amacher et al., 2013). Furthermore, the enzymatic activity of leucine aminopeptidase (LAP) is markedly elevated during hepatic dysfunction compared to physiological states (Aithal et al., 2011; Wang and Zhou, 2013; Atallah et al., 2021). Notably, both ONOO− and LAP have been substantiated as early diagnostic biomarkers for drug-induced liver diseases by numerous investigators (Pacher et al., 2007; Howell et al., 2018).
Based on the above, Xiaobing Zhang’s team first time to showcase the drug-induced hepatotoxicity pathways by use of a small-molecule fluorescent probe (Cheng et al., 2017), and further innovatively designed a novel near-infrared fluorescent dye with adjustable optical signals, employing it as the fluorescent precursor to synthesize two highly precise near-infrared fluorescent probes, namely, NIR-LAP and NIR-ONOO−, for detecting liver toxicity markers leucine aminopeptidase (LAP) and peroxynitrite (ONOO−), respectively (see Figure 1A) (Cheng et al., 2019). These probes exhibit no inherent fluorescence; however, upon interaction with LAP or ONOO−, they produce the product NIR-NH2, consequently restoring fluorescence. This unique feature enables the sensitive and accurate detection of LAP and ONOO−. The efficacy of these probes was demonstrated through the detection of LAP and ONOO− within cell mitochondria (see Figure 1B). Furthermore, the probes were utilized to visualize LAP and ONOO− fluctuations in mice with drug-induced liver injury (see Figure 1C). Leveraging the high sensitivity and specificity of the probes, an accurate evaluation of drug-induced liver toxicity became feasible. Lastly, the probes were successfully employed to assess the therapeutic effects of six liver-protective drugs on acetaminophen-induced liver toxicity (see Figure 1D). The high-fidelity NIR dye platform with an optically tunable group might provide an efficient method for the development of future probes applied in the pathological environment. This optically tunable, high-fidelity near-infrared dye not only offers a convenient and effective platform but also holds great promise for the design and development of pathological environmental probes. Nevertheless, while the NIR-LAP and NIR-ONOO− demonstrate considerable promise in monitoring the hepatic health status during pharmacotherapy, the evaluative metrics employed to gauge liver health are notably deficient in cogency. This deficiency represents a significant quandary within the domain of biosensor research, necessitating the incorporation of more rigorous, professional medical evaluative methodologies pertaining to hepatic function, such as the Histological Activity Index. This gap underscores an imperative need for the advancement of diagnostic criteria that can more effectively interface with the nuanced capabilities of biosensor technology in the context of liver health assessment.
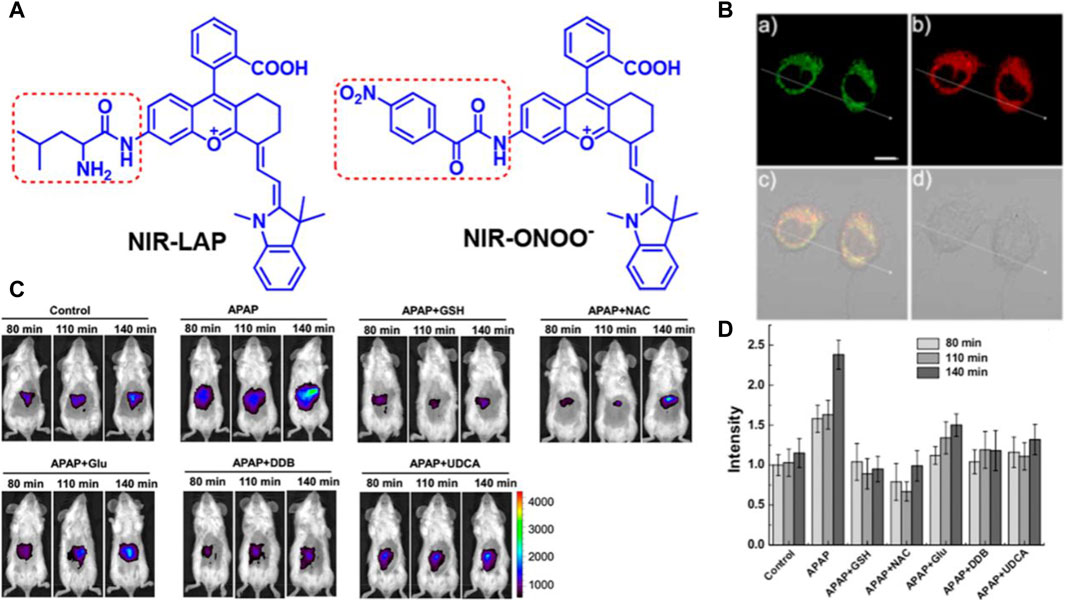
Figure 1. (A) The chemical structure of compounds NIR-LAP and NIR-ONOO−. Adapted with permission from (Cheng et al., 2019) (B) The co-localization of NIR-LAP in HepG2 cells (Pearson’s correlation coefficient (r) = 0.82). a) Green channel: Mito-Tracker Green (1 μM) stain (λex = 488 nm, λem = 500–550 nm); b) red channel: NIR-LAP (5 μM) stain (λex = 640 nm, λem = 663–738 nm); c) yellow: merged signal. d) reference. Scale bar: 10 μm. Adapted with permission from (Cheng et al., 2019) (C) representative images of BALB/c mice receiving saline (control), APAP (300 mg kg−1, intraperitoneally) alone, or with GSH (200 mg kg−1, intravenously), NAC (150 mg kg−1, intravenously), Glu (200 mg kg−1, intravenously), DDB (200 mg kg−1, intraperitoneally), UDCA (20 mg kg−1, intraperitoneally), followed by NIR-LAP (60 μL, 100 μM, intravenously) (λex = 640 nm, λem = 695–770 nm). Adapted with permission from (Cheng et al., 2019) (D) Relative fluorescence intensity in (C). Data are expressed as mean ± SD of three experiments. Adapted with permission from (Cheng et al., 2019).
Alcoholic liver diseases (ALDs) stand as the predominant cause of advanced liver conditions, representing a significant contributor to liver-related mortality on a global scale (Nath and Szabo, 2012). Alcohol-induced liver injury marks the early stages of ALDs, encompassing hepatic lesions, fatty liver, steatohepatitis, progressive fibrosis, cirrhosis, and, in severe cases, liver failure (Louvet and Mathurin, 2015). Detecting alcohol-induced liver injury holds paramount importance for devising optimal rehabilitation programs and effective treatment strategies. Addressing this, the research group led by Wu Shuizhu has introduced a water-soluble enzyme-activated fluorescent probe, named NIR-NO, designed for photoacoustic/fluorescence dual-mode imaging detection of alcohol-induced liver injury (Chen et al., 2020). In the chemical structure of the probe, the benzothiazole-anthracene chromophore serves as the signal reporter, while the N-oxide moiety, endowed with fluorescence-inhibiting ability, acts as a responsive group for cytochrome P450 (CYP) reductase (see Figure 2A). Upon interaction with CYP450 reductase, the substrate undergoes deoxygenation and reduction, resulting in the release of fluorophores and the generation of robust near-infrared fluorescence. Simultaneously, the probe’s absorption undergoes a redshift to the near-infrared region, yielding intense photoacoustic signals. Leveraging the dual signals of fluorescence and photoacoustic, the probe effectively detected mice with alcoholic liver injury (see Figure 2B). Moreover, the fluorescence intensity exhibited a noticeable increase with escalating ethanol (ETOH) concentrations, offering a means to indicate the stage of ALDs and aid in clinical diagnosis. This probe not only emerges as a promising tool for studying the physiological and pathological processes associated with ALDs but also holds potential significance when combined with sensors to estimate enzyme activity changes. Currently, a multitude of fluorescence imaging modalities are available for the in situ examination of enzymatic markers associated with hepatic carcinoma. However, persistent challenges call for continued refinement, especially in the innovation of supplementary near-infrared (NIR-II) and photoacoustic dual-mode fluorescent probes. The evolution of these technological modalities harbors the potential to markedly amplify both sensitivity and the signal-to-noise ratio, thus paving the way for more precise and effective diagnostic capabilities in the realm of liver cancer detection.
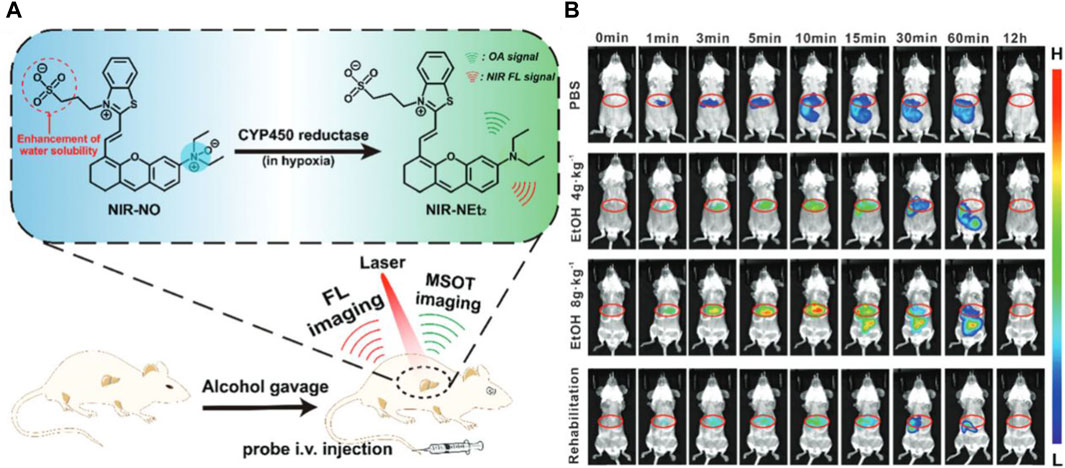
Figure 2. (A) Schematic display of the probe’s response to the over-expressed CYP450 reductase in the hepatic region and fluorescent and MSOT imaging of alcohol-induced liver injury in a mouse model. Adapted with permission from (Chen et al., 2020) (B) Time course for fluorescence imaging in vivo. The mice were pretreated with PBS, 4 g kg−1 EtOH, 8 g kg−1 EtOH, or 8 g kg−1 EtOH +200 mg kg−1 methadone once a day for three consecutive days, followed by tail intravenous injection of 2.7 mg kg−1 NIR-NO in PBS. Adapted with permission from (Chen et al., 2020).
3 Liver fibrosis enzyme
Liver fibrosis denotes the aberrant proliferation of connective tissue within the liver triggered by diverse pathogenic factors. Any form of liver injury initiates a cascade culminating in liver fibrosis during the organ’s reparative phase. Prolonged exposure to injurious stimuli can perpetuate the fibrotic process, ultimately leading to cirrhosis. Monoamine oxidase (MAO, EC 1.4.3.4) is a flavin-dependent enzyme that mediates the oxidation of amine substrates to corresponding imines (Westlund et al., 1985). Widely distributed in tissues, MAO exhibits the highest expression levels in the liver (Denney et al., 1982). MAO exists as two isoenzymes, namely, monoamine oxidase A (MAO-A) and monoamine oxidase B (MAO-B), with the latter implicated in collagen fiber formation and closely associated with liver fibrosis (Kim et al., 2018). Numerous investigations have identified elevated MAO-B levels in the serum of patients with early liver fibrosis, rendering it an ideal biomarker for early fibrosis diagnosis (Walker and Edmondson, 1994; Park et al., 2019). Hence, elucidating MAO-B activity levels in cells and in vivo holds promise for facilitating early liver fibrosis diagnosis and unveiling its pivotal role in fibrotic pathogenesis.
Rui Wang and colleagues have introduced two innovative near-infrared (NIR) fluorescent probes, MitoCy-NH2 and MitoHCy-NH2, designed for synergistic imaging of MAO-B and analysis of its role in oxidative stress within cellular and mouse aging models (Wang et al., 2018). These probes comprise three components: cyanine as the fluorescent moiety, propionamide as the recognition element, and triphenylphosphine cation as the mitochondrial targeting unit (Figure 3A). MitoCy-NH2 offers near-infrared fluorescence ratio signals (Figure 3B) and has been effectively utilized for the selective detection of MAO-B in H2O2-induced cellular and mouse aging models (Figure 3C). In a parallel endeavor, Yu Xiaoqi’s research team developed a 3-aminopropyl salicylaldehyde derivative probe, DEAN-MA, for MAO-B detection (Qin et al., 2019). Upon oxidation and enzymatic cleavage, the 3-aminopropyl groups are exposed to hydroxyl groups, triggering cyclization to generate coumarin with robust fluorescence (Figure 3D). Utilizing this probe, the authors successfully observed significantly higher MAO-B levels in human astrocytes (U87) compared to human liver cells (HL-7702) (Figure 3E). These observations emphatically highlight the considerable worth and prospective utility of probes within the sphere of Monoamine Oxidase B (MAO-B) research. Nevertheless, there is a cogent necessity for further enhancements to augment the stability of MAO-B probes. Such advancements are imperative to guarantee their persistent activity over extended durations within intricate biological matrices, thereby mitigating the likelihood of erroneous positive or negative results.
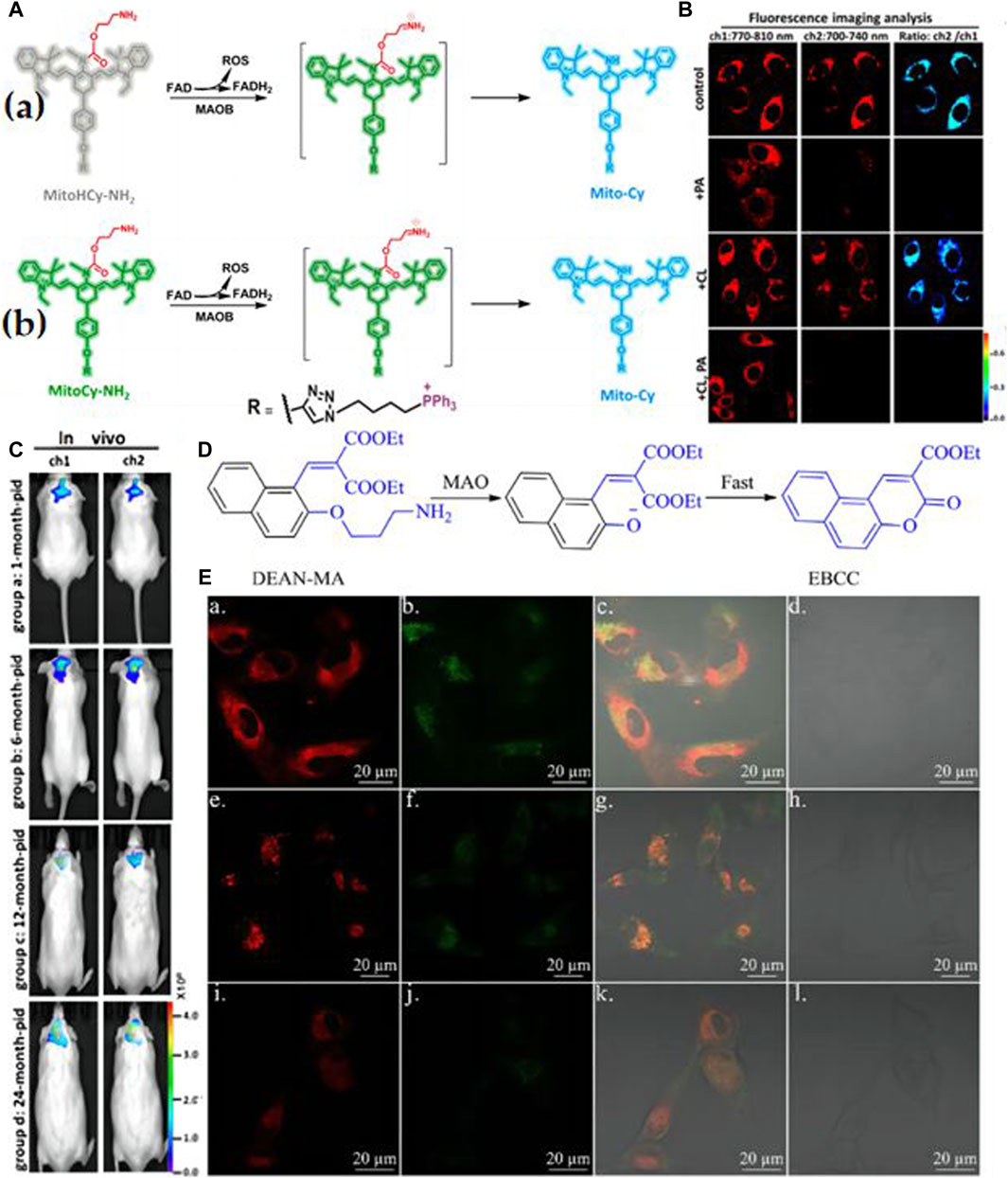
Figure 3. (A) a) Synergistic detection mechanism of MitoHCy-NH2 toward MAO-B and reactive oxygen species (ROS); b) the detection mechanism of MitoCy-NH2 toward MAO-B. Adapted with permission from (Wang et al., 2018). (B) Ratiometric fluorescence images for the selectivity of MitoCy-NH2 in SMMC7721 cells. Two fluorescence collection windows were used: channel 1, 750–800 nm (λex = 730 nm); channel 2,700–740 nm (λex = 650 nm). All the cells were washed three times with fresh DMEM before imaging. (a) Group a: incubated with 5 μM MitoCy-NH 2 for 100 min as control. Group b: incubated with 10 μM PA for 120 min, and then treated as described in group a. Group c: incubated with 10 μM CL for 120 min, and then treated as described in group a. Group d: incubated with 10 μM PA and 10 μM CL for 120 min, and then treated as described in group a. Adapted with permission from (Wang et al., 2018). (C) Imaging of MAO-B levels in mice brains with different ages. Fluorescence collection windows were the following: channel 1, 760–840 nm, λex = 730 nm; channel 2, 700–800 nm, λex = 650 nm. All the BALB/c mice were incubated with MitoCy-NH2 (100 μM, 50 μL in 1:99 DMSO/saline, v/v) for 30 min with intracranial injection. (a) In vivo imaging of mice in groups a−d; the ages of the mice were 1, 6, 12, and 24 months old, respectively. Adapted with permission from (Wang et al., 2018). (D) The “covalent assembly” strategy is applied in this work. Adapted with permission from (Qin et al., 2019). (E) The fluorescence image of U87 cells incubated with DEAN-MA and mito-tracker (a, b, c, d), lyso-tracker (e, f, g, h), and ER-tracker (i, j, k, l). a, e, i for the red channel of tracker; b, f, j for the blue channel of DEAN-MA; c, g, k for overlay; d, h, l for bright. H. Qin et al./Chinese Chemical Letters 30 (2019) 71–74 73. Adapted with permission from (Qin et al., 2019).
4 Liver cancer enzyme
Liver cancer, a formidable and often lethal malignancy, presents a challenging landscape with a low survival rate for affected individuals. Early detection and intervention stand out as pivotal strategies to enhance patient survival rates. Hepatocellular carcinoma, characterized by considerable heterogeneity, transcends being a mere mass of proliferating cancer cells; it is a complex tissue comprising various cell types (Rodriguez-Rios et al., 2022). Consequently, accurately identifying nascent nodes of liver cancer through physical means proves challenging. In contrast, a more practical approach involves precise identification of its specific molecular characteristics for early diagnosis, particularly focusing on its distinctive biomarkers (Wu et al., 2022). The ideal biomarker for early liver cancer should exhibit high sensitivity and specificity, enabling noninvasive detection with reproducible results. Presently, several new biomarkers, such as AFP, lectin-reactive alpha-fetoprotein, des-gamma-carboxyprothrombin, and Golgi protein 73, have been identified as effective indicators of liver cancer. Additionally, proteins with heightened expression in the liver emerge as potential candidate biomarkers, including carboxylesterase 1, hemoglobin alpha, alcohol dehydrogenase 1b, alcohol dehydrogenase 4, and glucose regulatory protein 78. These advancements in biomarker identification contribute to the ongoing pursuit of more accurate and reliable methods for early liver cancer diagnosis.
Carboxylesterase (CE), a prominent endoplasmic reticulum protein highly expressed in the liver, possesses the ability to be secreted outside the cell, disrupting calcium homeostasis within the endoplasmic reticulum lumen (Liu et al., 2018). This disruption can lead to endoplasmic reticulum dysfunction, inducing energy and endogenous metabolic impairments, oxidative stress, and ultimately resulting in liver cell injury and carcinogenesis (Li et al., 2019). Consequently, CE emerges as a potential diagnostic and analytical indicator for liver cancer. The design of CE fluorescent probes predominantly adopts the “inhibitor-derived” strategy, a classic approach in enzyme probe design (Fan et al., 2021; Wu et al., 2021). In this strategy, the active part or entire structure of a classic enzyme inhibitor is integrated and coupled with a fluorescent chromophore to regulate fluorescence release. The rational design of a practical probe with excellent specificity for specific enzymes and improved optical properties remains a significant challenge.
Guo et al. devised a water-soluble semi-cyanine fluorescent probe named 1 aimed at detecting cholinesterase (CE) activity through the incorporation of acetate bond recognition sites (Figure 4A) (Guo et al., 2022). In vitro fluorescence assays revealed that upon the addition of CE, the emission of the probe’s main peak at 510 nm was partially quenched and red-shifted to 580 nm. Notably, the probe exhibited high sensitivity to CE activity (with a detection limit of 0.98 × 10−6 U/mL) and demonstrated rapid response kinetics (<10 min) (Figure 4B). Moreover, owing to the presence of morpholine groups, the probe exhibited the capability to target lysosomes and monitor CE activity within these organelles. Successful application of the probe was demonstrated in the detection and imaging of CE activity in zebrafish (Figure 4C). In a separate study in 2021, the Han research group introduced a series of CE fluorescent probes utilizing carbamate as a foundational moiety named NIC−1, NIC-2, NIC-3, and NIC-4 (Figure 4D), subsequently employing them for super-resolution microscopy imaging of CE activity in live cells (Jia et al., 2021). Results underscored the efficacy of the NIC-4 as a CE inhibitor, whereby methyl substitution of nitrogen in the carbamate moiety effectively impeded the proton transfer process, thereby hindering nucleophilic attacks necessary for CE hydrolysis (Figure 4E). Intriguingly, cellular imaging elucidated the probe’s capability to dynamically track the flux of CE in live cells in real-time (Figure 4F).
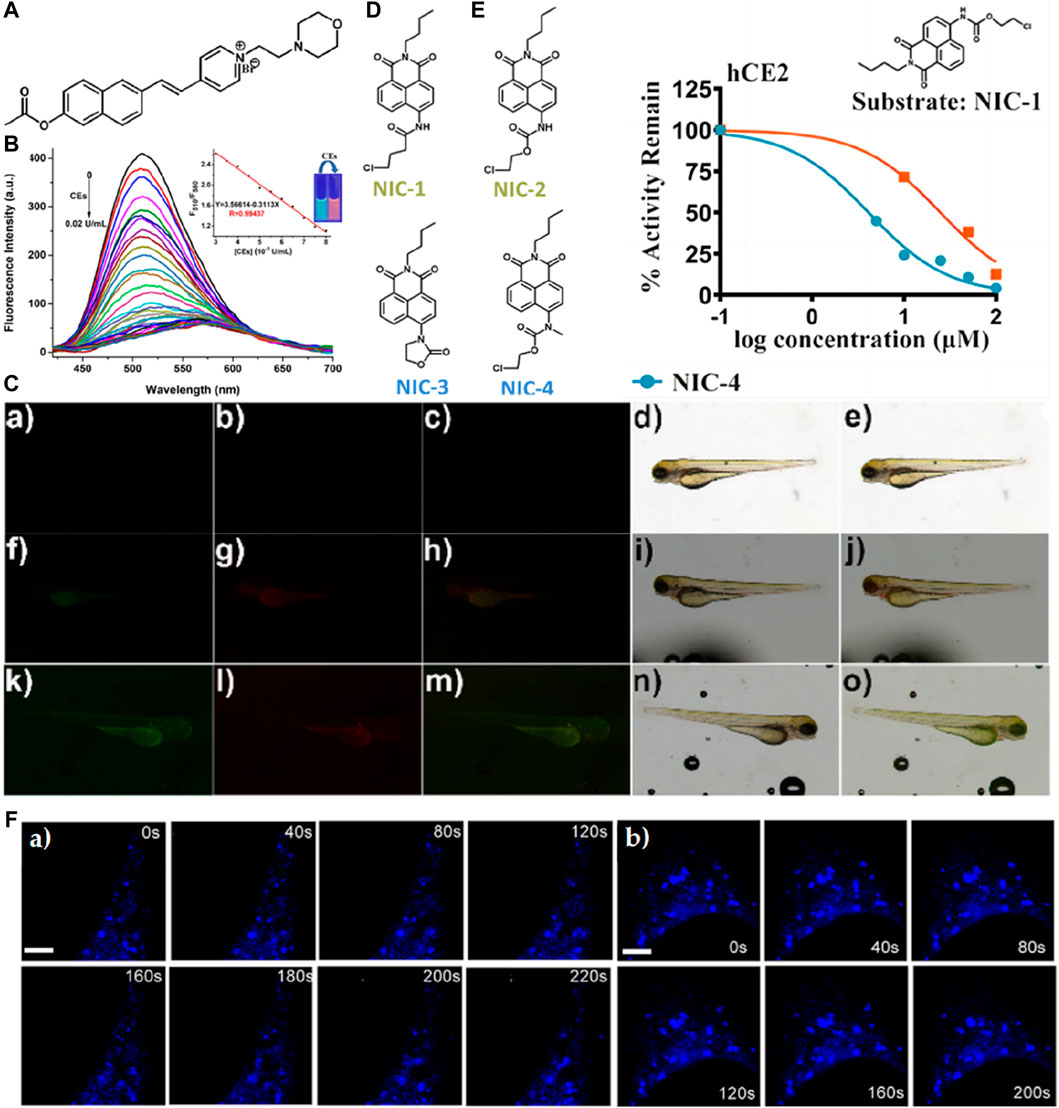
Figure 4. (A) The chemical structure of probe 1. (B) Fluorescence spectra of 10 μM probe 1 upon the addition of CEs (0–0.02 U/mL.) in PBS buffer solution (10 mM, pH 7.4). The insert shows the fluorescence intensity from 510 nm to 580 nm as a function of CE concentration with a linear relationship. The excitation wavelength was 360 nm. Excitation and emission slit widths are 5 nm each. Adapted with permission from (Guo et al., 2022). (C) Fluorescence images of the sensor in living zebrafish: (a, f, k) green channel; (b, g, l) red channel; (c, h, m) the merged images of (a, f, k) and (b, g, l); (d, i, n) bright field; (e, j, o) the merged images of (c, h, m) and (d, i, n). (a–e) in the absence of the probe; (f–j) incubated with probe 1 (10 μM) for 30 min; (k–o) pretreated with BNPP (100 μM) for 30 min before incubating with probe 1 for another 30 min; Adapted with permission from (Guo et al., 2022). (D) Structurally diversified carbamates designed and developed serve as inhibitors or substrates of hCEs in this study. Adapted with permission from (Jia et al., 2021). (E) Inhibition properties of NIC series. NIC−1 hydrolysis in hCE2. Inhibition types for NIC-3/4 were defined by the Lineweaver−Burk analysis. Adapted with permission from (Jia et al., 2021). (F) Time-lapse SIM images of different areas of hCE1 movement, representatively. For the time-lapse images, the time interval between each SIM image was set to 20 s with a course of 4 min. Scalebar: 5 μm in Figure c) and 4 μm in d). Adapted with permission from (Jia et al., 2021).
In 2022, Zhu’s team achieved a notable milestone by developing a near-infrared fluorescent probe named VPCPP (see Figure 5A) (Qi et al., 2022). This probe enables simultaneous monitoring of local microviscosity, micro-polarization, and CE in living cells through both blue and red channels. VPCPP demonstrated its ability to distinguish between cancer cells and normal cells, including BEAS-2B (human bronchial epithelium cell line), L02 (human normal liver cell line), HeLa (human cervical cancer cell line), MCF-7 (human breast adenocarcinoma cell line), and HepG2 (human liver hepatocellular carcinoma cell line) (see Figure 5B). The authors induced CE overexpression by employing aristolochic acid, a carcinogen capable of upregulating CE expression, and successfully imaged liver tumor-bearing mice using both two-channel and single-channel imaging (see Figures 5C, D). This breakthrough underscores the potential of VPCPP as a powerful tool for studying liver cancer and paves the way for future advancements in diagnostic and therapeutic strategies. Nonetheless, an issue that necessitates rectification persists within the domain of biosensor design; notably, the chemical architecture of many biosensors frequently encompasses a plethora of unstable structures, including ether bonds. Such configurations markedly compromise the stability of the sensors, particularly in scenarios necessitating long-term tracking. Furthermore, the incorporation of a fully conjugated structure within biosensors emerges as a beneficial strategy for bolstering their stability. This approach not only addresses the inherent fragility associated with certain chemical linkages but also paves the way for enhanced performance and reliability of biosensors in prolonged investigative applications.
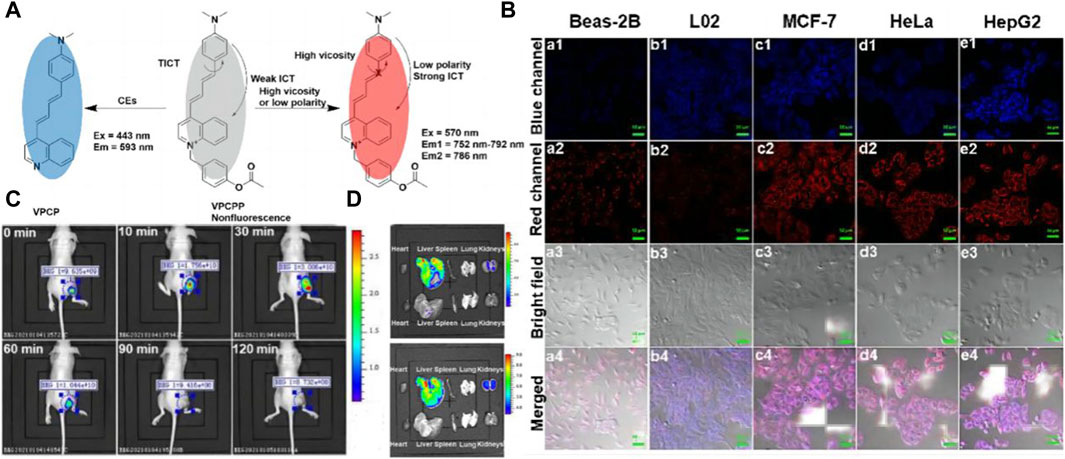
Figure 5. (A) OR Logic Gate-Based Fluorescent Probe VPCPP for Monitoring Local Microviscosity, Micropolarity, and CEs. Adapted with permission from (Qi et al., 2022) (B) Confocal fluorescence images for detecting local microviscosity, micropolarity, and CEs in various cell lines incubated with VPCPP (5 μM) for 1 h (a1−a4): Beas-2B cells. (b1−b4): L02 cells. (c1−c4): MCF-7 cells. (d1−d4): HeLa cells. (e1−e4): HepG2 cells. Adapted with permission from (Qi et al., 2022) (C) In vivo imaging of the 4T1 cells tumor-bearing mice after intratumoral injection of VPCPP (10 μM, 50 μL). Adapted with permission from (Qi et al., 2022) (D) Ex vivo imaging of main organs isolated from mice. Upline: the mouse intravenous injected with VPCPP (200 μM, 100 μL). Down the line: the mouse was intravenously injected with saline (0.9%, 100 μL). λex = 430 nm; λem = 575–650 nm and λex = 570 nm; λem = 695–770 nm. Adapted with permission from (Qi et al., 2022).
Ma’s research group devised and synthesized a novel red “turn-on” lysosome-targeted fluorescent probe, termed Lyso-CEs, by combining hemocyanins as a quenching group with an acetyl group as a recognition group (see Figure 6A) (Zhou et al., 2019). Lyso-CEs undergo facile hydrolysis, liberating the fluorophore hydroxy hemicyanine and emitting an off-on spectroscopic signal upon encountering CE. This probe exhibits high selectivity and sensitivity in the fluorescence detection and imaging of CE in live cells (see Figure 6B), serum, and tissues (see Figure 6C). In a parallel endeavor, Jiang et al. synthesized a two-photon ratiometric fluorescent probe named CEMT (see Figure 6D), leveraging coumarin as the fluorescent parent nucleus to target mitochondria. CEMT was designed for detecting and imaging CE activity and pH in mitochondria (Jiang et al., 2019). The combined influence of CE and pH induces noticeable changes in the fluorescence intensity of the probe (see Figure 6E), holding promise for monitoring drug delivery processes. In vitro cell imaging substantiates the exceptional targeting ability of CEMT, which tightly homes in on mitochondria. To monitor drug metabolism in the liver, drug administration was performed in mice via heart perfusion with vinyl acetate or benorilate, as depicted in Figure 6F. The results highlight the potential application of CEMT in CE-mediated acidification during anticancer drug administration. This comprehensive approach not only underscores the probe’s significance in biosensors but also emphasizes the critical role it plays in studying drug metabolism. To this juncture, notwithstanding the significant advancements realized in the fabrication of CE biosensors, there persists an imperative for the augmentation of their anti-interference faculties. This exigency emanates from the inherent vulnerability of CE sensors to potential reciprocal interference instigated by other esterases, most prominently AChE and BChE. These enzymes, sharing analogous recognition sites with CE, pose a substantial challenge to the specificity and accuracy of CE sensor readings, thereby underscoring the critical need for technological refinements to mitigate such interference (Zhu et al., 2015; Zhou and Ma, 2016; Zheng et al., 2017; Gao et al., 2020; Chen et al., 2021; Zhang et al., 2023).
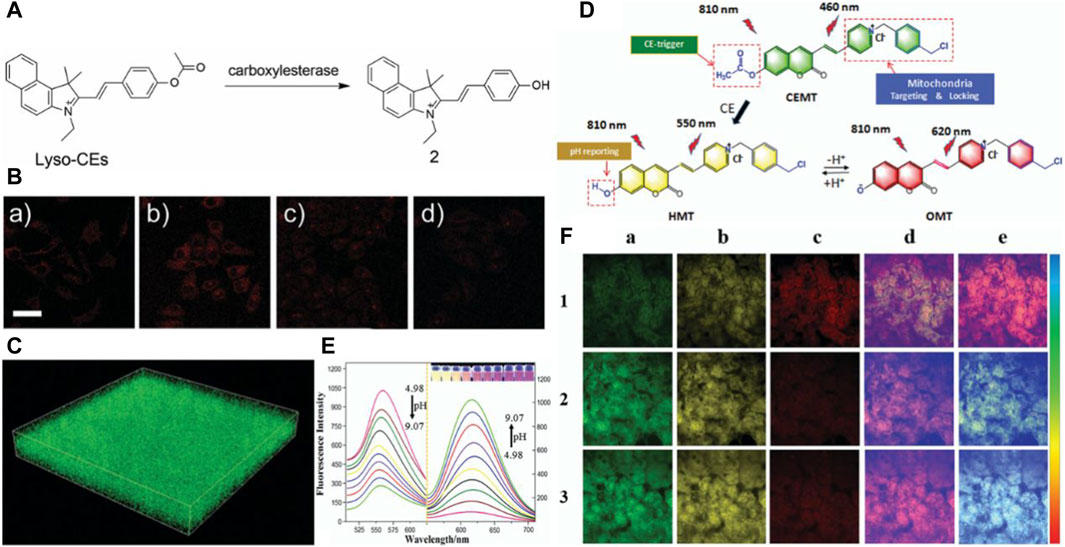
Figure 6. (A) The chemical structure of the probe Lyso-CEs and its reaction with CEs. Adapted with permission from (Zhou et al., 2019) (B) Confocal fluorescence images of cells. (a) A549 cells were incubated with 10 mM Lyso-CEs; (b) HepG2 cells were incubated with 10 mM Lyso-CEs; (c) HepG2 cells were pretreated with 50 mM AEBSF for 30 min, and then incubated with 10 mM Lyso-CEs; (d) HepG2 cells were pretreated with 100 mM AEBSF for 30 min, and then incubated with 10 mM Lyso-CEs. The bottom row is the corresponding differential interference contrast (DIC) images. Scale bar, 40 μm. Adapted with permission from (Zhou et al., 2019) (C) 3D fluorescence images in mice liver slices incubated with Lyso-CEs (10 mM) for 30 min at 37°C from confocal Z-scan imaging sections at a depth of 0–60 μm. lex = 514 nm, lem = 520–650 nm, scale bar: 100 μm. Adapted with permission from (Zhou et al., 2019) (D) Design and sensing mechanism of a ratiometric two-photon mitochondria-locked probe CEMT toward carboxylesterase (CE) activity and pH. Adapted with permission from (Jiang et al., 2019) (E) Fluorescence spectra change in the presence of 40 mM B–R buffer solution at various pH (4.98, 5.31, 5.74, 6.01, 6.50, 7.08, 7.42, 8.31, 8.59, 9.07) (lex = 410 nm and 530 nm). Adapted with permission from (Jiang et al., 2019) (F) Two-photon fluorescence images for the liver of mice with cardiac perfusion: (1) CEMT (20 mM) for 10 min (2) Pretreated with vinyl acetate (1 mM) before with CEMT (20 mM). (3) Pretreated with benorilate (1 mM) before with CEMT (20 mM) for 10 min. Images were captured using 810 nm for two-photon excitation. Emission window: (a) green channel (400,500 nm). (b) Yellow channel (500–570 nm). (c) Red channel (570–710 nm). (d) Ratio imaging: Iyellow/Igreen. (e) Ratio imaging: Ired/Iyellow. Scale bar: 20 mm. Adapted with permission from (Jiang et al., 2019).
5 Conclusion
Liver disease stands as a prevalent and highly detrimental condition with a propensity for progressing into liver cancer. Enhancing patient survival rates hinges significantly on the early detection and intervention of liver disease. Among the myriad of detection methods, fluorescence probe technology has garnered attention for its in situ applicability, multi-level analysis, and high sensitivity. Scientists have diligently developed various fluorescent probes tailored for different liver disease enzymes. Extensive clinical trials and practical applications have underscored the effectiveness of monitoring enzymes. This approach proves instrumental in disease screening and prognostic assessment. The diverse applications of fluorescence probe technology have markedly elevated imaging resolution, equipping healthcare professionals with more precise tools for disease diagnosis. This, in turn, facilitates real-time and accurate detection, contributing significantly to the meaningful early diagnosis of liver disease.
We categorically assert that enzyme biosensors designed for the diagnosis of liver disease hold considerable promise for clinical application (Figure 7). Yet, there remains a substantive gap between their current state and practical deployment in a clinical setting. From a clinical vantage point, it is imperative, firstly, to broaden the scope of toxicological investigations to ascertain that such biosensors do not adversely affect patient health—a fundamental criterion for their use. This includes ensuring that the biosensors do not detrimentally impact not only the target organ but also other physiological systems. Secondly, there is a pressing need to incorporate more sophisticated medical indicators into biosensor research, a measure that would facilitate the seamless integration of these devices within the medical field and catalyze their advancement. Such an approach would not only enhance the relevance of biosensors to clinical diagnostics but also ensure that their development aligns with the overarching objectives of medical science, thereby fostering a more holistic approach to patient care and treatment. Besides these challenges above, to further propel the development of high-performance enzyme biosensors and facilitate their commercialization, several crucial challenges pertaining to biomarker fluorescent probes need to be addressed: i) Evaluating the potential impact of sensors on the physiological activities of organisms is paramount. ii) Overcoming the limitation posed by the water solubility of biomarker fluorescent probes is crucial for expanding their applications. iii) Ensuring the metabolism period of biomarkers aligns with the intended tracking period is essential. iv) Designing high-performance biomarker biosensors necessitates the integration of multiple advantages into a single molecule, such as strong fluorescence in the NIR or NIR-II region, excellent stability, and biocompatibility without toxicity. v) Exploring and developing more applications for biomarker fluorescent probes will further enhance their versatility and utility. Addressing these challenges will undoubtedly contribute to the maturation of enzyme probe technology and its broader adoption in various biomedical applications.
Author contributions
SfL: Conceptualization, Funding acquisition, Resources, Writing–original draft. YY: Investigation, Project administration, Supervision, Writing–review and editing. ShL: Conceptualization, Methodology, Writing–review and editing. CW: Investigation, Project administration, Supervision, Writing–review and editing. WS: Conceptualization, Funding acquisition, Investigation, Supervision, Writing–review and editing. XH: Investigation, Project administration, Visualization, Writing–review and editing.
Funding
The author(s) declare that financial support was received for the research, authorship, and/or publication of this article. This work was financially supported by the Shandong Provincial Natural Science Foundation General Project, China (ZR2021MH052).
Conflict of interest
The authors declare that the research was conducted in the absence of any commercial or financial relationships that could be construed as a potential conflict of interest.
Publisher’s note
All claims expressed in this article are solely those of the authors and do not necessarily represent those of their affiliated organizations, or those of the publisher, the editors and the reviewers. Any product that may be evaluated in this article, or claim that may be made by its manufacturer, is not guaranteed or endorsed by the publisher.
References
Aithal, G. P., Watkins, P. B., Andrade, R. J., Larrey, D., Molokhia, M., Takikawa, H., et al. (2011). Case definition and phenotype standardization in drug-induced liver injury. Clin. Pharmacol. Ther. 89, 806–815. doi:10.1038/clpt.2011.58
Alifu, N., Zebibula, A., Qi, J., Zhang, H., Sun, C., Yu, X., et al. (2018). Single-molecular near-infrared-II theranostic systems: ultrastable aggregation-induced emission nanoparticles for long-term tracing and efficient photothermal therapy. ACS Nano 12, 11282–11293. doi:10.1021/acsnano.8b05937
Amacher, D. E., Schomaker, S. J., and Aubrecht, J. (2013). Development of blood biomarkers for drug-induced liver injury: an evaluation of their potential for risk assessment and diagnostics. Mol. Diagn Ther. 17, 343–354. doi:10.1007/s40291-013-0049-0
Asanuma, D., Sakabe, M., Kamiya, M., Yamamoto, K., Hiratake, J., Ogawa, M., et al. (2015). Sensitive β-galactosidase-targeting fluorescence probe for visualizing small peritoneal metastatic tumours in vivo. Nat. Commun. 6, 6463. doi:10.1038/ncomms7463
Asrani, S. K., Devarbhavi, H., Eaton, J., and Kamath, P. S. (2019). Burden of liver diseases in the world. J. Hepatology 70, 151–171. doi:10.1016/j.jhep.2018.09.014
Atallah, E., Freixo, C., Alvarez-Alvarez, I., Cubero, F. J., Gerbes, A. L., Kullak-Ublick, G. A., et al. (2021). Biomarkers of idiosyncratic drug-induced liver injury (DILI) - a systematic review. Expert Opin. Drug Metabolism Toxicol. 17, 1327–1343. doi:10.1080/17425255.2021.1999410
Author Anonymous (2019). Drug-induced liver injury. Nat. Rev. Dis. Prim. 5, 59. doi:10.1038/s41572-019-0117-9
Bertolini, A., Ferrari, A., Ottani, A., Guerzoni, S., Tacchi, R., and Leone, S. (2006). Paracetamol: new vistas of an old drug. CNS Drug Rev. 12, 250–275. doi:10.1111/j.1527-3458.2006.00250.x
Bhaskar, S., Srinivasan, V., and Ramamurthy, S. S. (2023). Nd2O3-Ag nanostructures for plasmonic biosensing, antimicrobial, and anticancer applications. ACS Appl. Nano Mat. 6, 1129–1145. doi:10.1021/acsanm.2c04643
Board, P. G., and Anders, M. W. (2007). Glutathione transferase omega 1 catalyzes the reduction of S-(Phenacyl)glutathiones to acetophenones. Chem. Res. Toxicol. 20, 149–154. doi:10.1021/tx600305y
Board, P. G., and Menon, D. (2013). Glutathione transferases, regulators of cellular metabolism and physiology. Biochimica Biophysica Acta (BBA) - General Subj. 1830, 3267–3288. doi:10.1016/j.bbagen.2012.11.019
Boursier, J., Roux, M., Costentin, C., Chaigneau, J., Fournier-Poizat, C., Trylesinski, A., et al. (2023). Practical diagnosis of cirrhosis in non-alcoholic fatty liver disease using currently available non-invasive fibrosis tests. Nat. Commun. 14, 5219. doi:10.1038/s41467-023-40328-4
Chen, J., Chen, L., Wu, Y., Fang, Y., Zeng, F., Wu, S., et al. (2021). A H2O2-activatable nanoprobe for diagnosing interstitial cystitis and liver ischemia-reperfusion injury via multispectral optoacoustic tomography and NIR-II fluorescent imaging. Nat. Commun. 12, 6870. doi:10.1038/s41467-021-27233-4
Chen, J., Fang, Y., Sun, L., Zeng, F., and Wu, S. (2020). An activatable probe for detecting alcoholic liver injury via multispectral optoacoustic tomography and fluorescence imaging. Chem. Commun. 56, 11102–11105. doi:10.1039/D0CC04635C
Cheng, D., Peng, J., Lv, Y., Su, D., Liu, D., Chen, M., et al. (2019a). De novo design of chemical stability near-infrared molecular probes for high-fidelity hepatotoxicity evaluation in vivo. J. Am. Chem. Soc. 141, 6352–6361. doi:10.1021/jacs.9b01374
Cheng, D., Xu, W., Yuan, L., and Zhang, X. (2017). Investigation of drug-induced hepatotoxicity and its remediation pathway with reaction-based fluorescent probes. Anal. Chem. 89, 7693–7700. doi:10.1021/acs.analchem.7b01671
Cheng, P., Miao, Q., Li, J., Huang, J., Xie, C., and Pu, K. (2019b). Unimolecular chemo-fluoro-luminescent reporter for crosstalk-free duplex imaging of hepatotoxicity. J. Am. Chem. Soc. 141, 10581–10584. doi:10.1021/jacs.9b02580
Denney, R. M., Fritz, R. R., Patel, N. T., and Abell, C. W. (1982). Human liver MAO-A and MAO-B separated by immunoaffinity chromatography with MAO-B-specific monoclonal antibody. Science 215, 1400–1403. doi:10.1126/science.7063850
DiMasi, J. A. (2001). Risks in new drug development: approval success rates for investigational drugs. Clin Pharma Ther. 69, 297–307. doi:10.1067/mcp.2001.115446
Enbanathan, S., and Iyer, S. K. (2022). A novel phenanthridine and terpyridine based D-π-A fluorescent probe for the ratiometric detection of Cd2+ in environmental water samples and living cells. Ecotoxicol. Environ. Saf. 247, 114272. doi:10.1016/j.ecoenv.2022.114272
Fabrellas, N., Carol, M., Palacio, E., Aban, M., Lanzillotti, T., Nicolao, G., et al. (2020). Nursing care of patients with cirrhosis: the LiverHope nursing Project. Hepatology 71, 1106–1116. doi:10.1002/hep.31117
Fallowfield, J. A., and Ramachandran, P. (2021). A relaxin-based nanotherapy for liver fibrosis. Nat. Nanotechnol. 16, 365–366. doi:10.1038/s41565-020-00832-w
Fan, L., Pan, Y., Li, W., Xu, Y., Duan, Y., Li, R., et al. (2021). A near-infrared fluorescent probe with large Stokes shift for visualizing and monitoring mitochondrial viscosity in live cells and inflammatory tissues. Anal. Chim. Acta 1149, 338203. doi:10.1016/j.aca.2021.338203
Gao, H., Kam, C., Chou, T. Y., Wu, M.-Y., Zhao, X., and Chen, S. (2020). A simple yet effective AIE-based fluorescent nano-thermometer for temperature mapping in living cells using fluorescence lifetime imaging microscopy. Nanoscale Horiz. 5, 488–494. doi:10.1039/C9NH00693A
Gao, Y., He, Z., He, X., Zhang, H., Weng, J., Yang, X., et al. (2019). Dual-color emissive AIEgen for specific and label-free double-stranded DNA recognition and single-nucleotide polymorphisms detection. J. Am. Chem. Soc. 141, 20097–20106. doi:10.1021/jacs.9b09239
Geng, X., Gao, D., Hu, D., Liu, Q., Liu, C., Yuan, Z., et al. (2020). Active-targeting NIR-II phototheranostics in multiple tumor models using platelet-camouflaged nanoprobes. ACS Appl. Mat. Interfaces 12, 55624–55637. doi:10.1021/acsami.0c16872
Gonçalves, D. C., Lira, F. S., Yamashita, A. S., Carnevali Junior, L. C., Eder, R., Laviano, A., et al. (2019). Liver lipid metabolism disruption in cancer cachexia is aggravated by cla supplementation -induced inflammation. Clin. Nutr. 38, 2219–2230. doi:10.1016/j.clnu.2018.09.023
Guo, F.-F., Wu, W.-N., Zhao, X.-L., Wang, Y., Fan, Y.-C., Zhang, C.-X., et al. (2022). A water-soluble lysosome-targetable fluorescent probe for carboxylesterase detection and its application in biological imaging. Dyes Pigments 199, 110079. doi:10.1016/j.dyepig.2022.110079
Guo, X., Mu, S., Li, J., Zhang, Y., Liu, X., Zhang, H., et al. (2020). Fabrication of a water-soluble near-infrared fluorescent probe for selective detection and imaging of dipeptidyl peptidase IV in biological systems. J. Mat. Chem. B 8, 767–775. doi:10.1039/C9TB02301A
Han, H.-H., Tian, H., Zang, Y., Sedgwick, A. C., Li, J., Sessler, J. L., et al. (2021). Small-molecule fluorescence-based probes for interrogating major organ diseases. Chem. Soc. Rev. 50, 9391–9429. doi:10.1039/D0CS01183E
He, X., Wang, X., Zhang, L., Fang, G., Liu, J., and Wang, S. (2018). Sensing and intracellular imaging of Zn2+ based on affinity peptide using an aggregation induced emission fluorescence “switch-on” probe. Sensors Actuators B Chem. 271, 289–299. doi:10.1016/j.snb.2018.05.082
Hou, X., Peng, J., Zeng, F., Yu, C., and Wu, S. (2017). An anthracenecarboximide fluorescent probe for in vitro and in vivo ratiometric imaging of endogenous alpha-l-fucosidase for hepatocellular carcinoma diagnosis. Mat. Chem. Front. 1, 660–667. doi:10.1039/C6QM00112B
Howell, L. S., Ireland, L., Park, B. K., and Goldring, C. E. (2018). MiR-122 and other microRNAs as potential circulating biomarkers of drug-induced liver injury. Expert Rev. Mol. Diagnostics 18, 47–54. doi:10.1080/14737159.2018.1415145
Jia, Y., Wang, J., Li, P., Ma, X., and Han, K. (2021). Directionally modified fluorophores for super-resolution imaging of target enzymes: a case study with carboxylesterases. J. Med. Chem. 64, 16177–16186. doi:10.1021/acs.jmedchem.1c01469
Jiang, A., Chen, G., Xu, J., Liu, Y., Zhao, G., Liu, Z., et al. (2019). Ratiometric two-photon fluorescent probe for in situ imaging of carboxylesterase (CE)-mediated mitochondrial acidification during medication. Chem. Commun. 55, 11358–11361. doi:10.1039/C9CC05759E
Kim, D., Beck, S., and Jung, J. (2018). Monitoring of monoamine oxidases as biomarkers of disease and disorder. Bull. Korean Chem. Soc. 39, 277–278. doi:10.1002/bkcs.11397
Lee, A. S. (2007). GRP78 induction in cancer: therapeutic and prognostic implications. Cancer Res. 67, 3496–3499. doi:10.1158/0008-5472.CAN-07-0325
Li, H., Kim, D., Yao, Q., Ge, H., Chung, J., Fan, J., et al. (2021). Activity-based NIR enzyme fluorescent probes for the diagnosis of tumors and image-guided surgery. Angew. Chem. Int. Ed. 60, 17268–17289. doi:10.1002/anie.202009796
Li, H., Kim, H., Xu, F., Han, J., Yao, Q., Wang, J., et al. (2022). Activity-based NIR fluorescent probes based on the versatile hemicyanine scaffold: design strategy, biomedical applications, and outlook. Chem. Soc. Rev. 51, 1795–1835. doi:10.1039/D1CS00307K
Li, H., Li, Y., Yao, Q., Fan, J., Sun, W., Long, S., et al. (2019a). In situ imaging of aminopeptidase N activity in hepatocellular carcinoma: a migration model for tumour using an activatable two-photon NIR fluorescent probe. Chem. Sci. 10, 1619–1625. doi:10.1039/C8SC04685A
Li, L., Zhang, C., Ge, J., Qian, L., Chai, B., Zhu, Q., et al. (2015). A small-molecule probe for selective profiling and imaging of monoamine oxidase B activities in models of Parkinson’s disease. Angew. Chem. Int. Ed. 54, 10821–10825. doi:10.1002/anie.201504441
Li, M., Zhai, C., Wang, S., Huang, W., Liu, Y., and Li, Z. (2019b). Detection of carboxylesterase by a novel hydrosoluble near-infrared fluorescence probe. RSC Adv. 9, 40689–40693. doi:10.1039/C9RA08150J
Lim, S., Yoon, H. Y., Park, S.-J., Song, S., Shim, M. K., Yang, S., et al. (2021). Predicting in vivo therapeutic efficacy of bioorthogonally labeled endothelial progenitor cells in hind limb ischemia models via non-invasive fluorescence molecular tomography. Biomaterials 266, 120472. doi:10.1016/j.biomaterials.2020.120472
Liu, C., Lu, S., Yang, L., Chen, P., Bai, P., and Wang, Q. (2017a). Near-infrared neodymium tag for quantifying targeted biomarker and counting its host circulating tumor cells. Anal. Chem. 89, 9239–9246. doi:10.1021/acs.analchem.7b02016
Liu, F., Wang, Z., Wang, W., Luo, J.-G., and Kong, L. (2018a). Red-emitting fluorescent probe for detection of γ-glutamyltranspeptidase and its application of real-time imaging under oxidative stress in cells and in vivo. Anal. Chem. 90, 7467–7473. doi:10.1021/acs.analchem.8b00994
Liu, H., Li, K., Hu, X., Zhu, L., Rong, Q., Liu, Y., et al. (2017b). In situ localization of enzyme activity in live cells by a molecular probe releasing a precipitating fluorochrome. Angew. Chem. Int. Ed. 56, 11788–11792. doi:10.1002/anie.201705747
Liu, H.-W., Chen, L., Xu, C., Li, Z., Zhang, H., Zhang, X.-B., et al. (2018b). Recent progresses in small-molecule enzymatic fluorescent probes for cancer imaging. Chem. Soc. Rev. 47, 7140–7180. doi:10.1039/C7CS00862G
Liu, X., Fan, N., Wu, L., Wu, C., Zhou, Y., Li, P., et al. (2018c). Lighting up alkaline phosphatase in drug-induced liver injury using a new chemiluminescence resonance energy transfer nanoprobe. Chem. Commun. 54, 12479–12482. doi:10.1039/C8CC07211F
Liu, Y., Teng, L., Xu, C., Liu, H.-W., Xu, S., Guo, H., et al. (2019). A “Double-Locked” and enzyme-activated molecular probe for accurate bioimaging and hepatopathy differentiation. Chem. Sci. 10, 10931–10936. doi:10.1039/C9SC03628H
Llovet, J. M., Ricci, S., Mazzaferro, V., Hilgard, P., Gane, E., Blanc, J.-F., et al. (2008). Sorafenib in advanced hepatocellular carcinoma. N. Engl. J. Med. 359, 378–390. doi:10.1056/NEJMoa0708857
Long, S., Chen, L., Xiang, Y., Song, M., Zheng, Y., and Zhu, Q. (2012). An activity-based fluorogenic probe for sensitive and selective monoamine oxidase-B detection. Chem. Commun. 48, 7164. doi:10.1039/c2cc33089j
Louvet, A., and Mathurin, P. (2015). Alcoholic liver disease: mechanisms of injury and targeted treatment. Nat. Rev. Gastroenterol. Hepatol. 12, 231–242. doi:10.1038/nrgastro.2015.35
Lu, H., Zhao, X., Tian, W., Wang, Q., and Shi, J. (2014). Pluronic F127–folic acid encapsulated nanoparticles with aggregation-induced emission characteristics for targeted cellular imaging. RSC Adv. 4, 18460. doi:10.1039/c4ra01355g
Ma, K., Wang, H., Li, X., Xu, B., and Tian, W. (2015). Turn-on sensing for Ag+ based on AIE-active fluorescent probe and cytosine-rich DNA. Anal. Bioanal. Chem. 407, 2625–2630. doi:10.1007/s00216-015-8467-y
McDonald, A. G., and Tipton, K. F. (2022). Enzyme nomenclature and classification: the state of the art. FEBS J. febs 16274, 2214–2231. doi:10.1111/febs.16274
Mitchell, C., Mahrouf-Yorgov, M., Mayeuf, A., Robin, M.-A., Mansouri, A., Fromenty, B., et al. (2011). Overexpression of Bcl-2 in hepatocytes protects against injury but does not attenuate fibrosis in a mouse model of chronic cholestatic liver disease. Lab. Investig. 91, 273–282. doi:10.1038/labinvest.2010.163
Nath, B., and Szabo, G. (2012). Hypoxia and hypoxia inducible factors: diverse roles in liver diseases. Hepatology 55, 622–633. doi:10.1002/hep.25497
Nguyen, T. H., Law, D. T., and Williams, D. B. (1991). Binding protein BiP is required for translocation of secretory proteins into the endoplasmic reticulum in Saccharomyces cerevisiae. Proc. Natl. Acad. Sci. U.S.A. 88, 1565–1569. doi:10.1073/pnas.88.4.1565
Okamura, K., Kimata, Y., Higashio, H., Tsuru, A., and Kohno, K. (2000). Dissociation of Kar2p/BiP from an ER sensory molecule, Ire1p, triggers the unfolded protein response in yeast. Biochem. Biophysical Res. Commun. 279, 445–450. doi:10.1006/bbrc.2000.3987
Pacher, P., Beckman, J. S., and Liaudet, L. (2007). Nitric oxide and peroxynitrite in health and disease. Physiol. Rev. 87, 315–424. doi:10.1152/physrev.00029.2006
Park, J.-H., Ju, Y. H., Choi, J. W., Song, H. J., Jang, B. K., Woo, J., et al. (2019). Newly developed reversible MAO-B inhibitor circumvents the shortcomings of irreversible inhibitors in Alzheimer’s disease. Sci. Adv. 5, eaav0316. doi:10.1126/sciadv.aav0316
Qi, Y.-L., Wang, H.-R., Chen, L.-L., Yang, B., Yang, Y.-S., He, Z.-X., et al. (2022). Multifunctional fluorescent probe for simultaneously detecting microviscosity, micropolarity, and carboxylesterases and its application in bioimaging. Anal. Chem. 94, 4594–4601. doi:10.1021/acs.analchem.1c04286
Qin, H., Li, L., Li, K., and Xiaoqi, Y. (2019). Novel strategy of constructing fluorescent probe for MAO-B via cascade reaction and its application in imaging MAO-B in human astrocyte. Chin. Chem. Lett. 30, 71–74. doi:10.1016/j.cclet.2018.05.018
Qu, C., Xiao, Y., Zhou, H., Ding, B., Li, A., Lin, J., et al. (2019). Quaternary ammonium salt based NIR-II probes for in vivo imaging. Adv. Opt. Mat. 7, 1900229. doi:10.1002/adom.201900229
Rodriguez-Rios, M., Megia-Fernandez, A., Norman, D. J., and Bradley, M. (2022). Peptide probes for proteases – innovations and applications for monitoring proteolytic activity. Chem. Soc. Rev. 51, 2081–2120. doi:10.1039/D1CS00798J
Schuppan, D., Ashfaq-Khan, M., Yang, A. T., and Kim, Y. O. (2018). Liver fibrosis: direct antifibrotic agents and targeted therapies. Matrix Biol. 68 (69), 435–451. doi:10.1016/j.matbio.2018.04.006
Sohrabpour, A. A., Mohamadnejad, M., and Malekzadeh, R. (2012). Review article: the reversibility of cirrhosis. Aliment. Pharmacol. Ther. 36, 824–832. doi:10.1111/apt.12044
Sun, F., Liu, J., Huang, Y., Zhu, X., Liu, Y., Zhang, L., et al. (2021). A quinoline derived D-A-D type fluorescent probe for sensing tetrameric transthyretin. Bioorg. Med. Chem. Lett. 52, 128408. doi:10.1016/j.bmcl.2021.128408
Sun, J., Chen, N., Chen, X., Zhang, Q., and Gao, F. (2019). Two-photon fluorescent nanoprobe for glutathione sensing and imaging in living cells and zebrafish using a semiconducting polymer dots hybrid with dopamine and β-cyclodextrin. Anal. Chem. 91, 12414–12421. doi:10.1021/acs.analchem.9b03010
Sung, H., Ferlay, J., Siegel, R. L., Laversanne, M., Soerjomataram, I., Jemal, A., et al. (2021). Global cancer statistics 2020: GLOBOCAN estimates of incidence and mortality worldwide for 36 cancers in 185 countries. CA A Cancer J. Clin. 71, 209–249. doi:10.3322/caac.21660
Tang, C., Du, Y., Liang, Q., Cheng, Z., and Tian, J. (2020). Development of a novel histone deacetylase-targeted near-infrared probe for hepatocellular carcinoma imaging and fluorescence image-guided surgery. Mol. Imaging Biol. 22, 476–485. doi:10.1007/s11307-019-01389-4
Uetrecht, J. (2010). Adverse drug reactions. Berlin, Heidelberg: Springer Berlin Heidelberg. doi:10.1007/978-3-642-00663-0
Walker, M. C., and Edmondson, D. E. (1994). Structure-activity relationships in the oxidation of benzylamine analogs by bovine liver mitochondrial monoamine oxidase B. Biochemistry 33, 7088–7098. doi:10.1021/bi00189a011
Wang, D.-D., Zou, L.-W., Jin, Q., Guan, X.-Q., Yu, Y., Zhu, Y.-D., et al. (2020a). Bioluminescent sensor reveals that carboxylesterase 1A is a novel endoplasmic reticulum-derived serologic indicator for hepatocyte injury. ACS Sens. 5, 1987–1995. doi:10.1021/acssensors.0c00384
Wang, F., Yu, S., Xu, Z., Li, L., Dang, Y., Xu, X., et al. (2018a). Acid-promoted D-A-D type far-red fluorescent probe with high photostability for lysosomal nitric oxide imaging. Anal. Chem. 90, 7953–7962. doi:10.1021/acs.analchem.8b00612
Wang, H., Ma, K., Xu, B., and Tian, W. (2016). Tunable supramolecular interactions of aggregation-induced emission probe and graphene oxide with biomolecules: an approach toward ultrasensitive label-free and “turn-on” DNA sensing. Small 12, 6613–6622. doi:10.1002/smll.201601544
Wang, K., and Zhou, Q. (2013). Biomarkers of drug-induced liver injury. CBF 1, 1. doi:10.2147/CBF.S27900
Wang, N., Chen, L., Chen, W., and Ju, H. (2021). Potential- and color-resolved electrochemiluminescence of polymer dots for array imaging of multiplex MicroRNAs. Anal. Chem. 93, 5327–5333. doi:10.1021/acs.analchem.1c00620
Wang, Q., Xu, J., Geng, R., Cai, J., Li, J., Xie, C., et al. (2020b). High performance one-for-all phototheranostics: NIR-II fluorescence imaging guided mitochondria-targeting phototherapy with a single-dose injection and 808 nm laser irradiation. Biomaterials 231, 119671. doi:10.1016/j.biomaterials.2019.119671
Wang, R., Han, X., You, J., Yu, F., and Chen, L. (2018b). Ratiometric near-infrared fluorescent probe for synergistic detection of monoamine oxidase B and its contribution to oxidative stress in cell and mice aging models. Anal. Chem. 90, 4054–4061. doi:10.1021/acs.analchem.7b05297
Wang, Z., Han, D., Wang, H., Zheng, M., Xu, Y., and Zhang, H. (2023a). Organic semiconducting nanoparticles for biosensor: a review. Biosensors 13, 494. doi:10.3390/bios13040494
Wang, Z., Ma, J., Li, C., and Zhang, H. (2023b). Conjugated aggregation-induced fluorescent materials for biofluorescent probes: a review. Biosensors 13, 159. doi:10.3390/bios13020159
Westlund, K. N., Denney, R. M., Kochersperger, L. M., Rose, R. M., and Abell, C. W. (1985). Distinct monoamine oxidase A and B populations in primate brain. Science 230, 181–183. doi:10.1126/science.3875898
Wu, J., Liu, W., Ge, J., Zhang, H., and Wang, P. (2011). New sensing mechanisms for design of fluorescent chemosensors emerging in recent years. Chem. Soc. Rev. 40, 3483. doi:10.1039/c0cs00224k
Wu, X., Wang, R., Kwon, N., Ma, H., and Yoon, J. (2022). Activatable fluorescent probes for in situ imaging of enzymes. Chem. Soc. Rev. 51, 450–463. doi:10.1039/D1CS00543J
Wu, X., Wang, R., Qi, S., Kwon, N., Han, J., Kim, H., et al. (2021). Rational design of a highly selective near-infrared two-photon fluorogenic probe for imaging orthotopic hepatocellular carcinoma chemotherapy. Angew. Chem. Int. Ed. 60, 15418–15425. doi:10.1002/anie.202101190
Xiong, H., Xiao, H., Luo, C., Chen, L., Liu, X., Hu, Z., et al. (2019). GRP78 activates the Wnt/HOXB9 pathway to promote invasion and metastasis of hepatocellular carcinoma by chaperoning LRP6. Exp. Cell Res. 383, 111493. doi:10.1016/j.yexcr.2019.07.006
Yang, Z.-M., Mo, Q.-Y., He, J.-M., Mo, D.-L., Li, J., Chen, H., et al. (2020). Mitochondrial-targeted and near-infrared fluorescence probe for bioimaging and evaluating monoamine oxidase A activity in hepatic fibrosis. ACS Sens. 5, 943–951. doi:10.1021/acssensors.9b02116
Zhang, S., Liu, H., Yin, M., Pei, X., Hausser, A., Ishikawa, E., et al. (2020). Deletion of protein kinase D3 promotes liver fibrosis in mice. Hepatology 72, 1717–1734. doi:10.1002/hep.31176
Zhang, W., Huang, T., Li, J., Sun, P., Wang, Y., Shi, W., et al. (2019). Facial control intramolecular charge transfer of quinoid conjugated polymers for efficient in vivo NIR-II imaging. ACS Appl. Mat. Interfaces 11, 16311–16319. doi:10.1021/acsami.9b02597
Zhang, W., Luo, Y., Zhao, J., Lin, W.-H., Ni, X.-L., Tao, Z., et al. (2022). tQ[14]-based AIE supramolecular network polymers as potential bioimaging agents for the detection of Fe3+ in live HeLa cells. Sensors Actuators B Chem. 354, 131189. doi:10.1016/j.snb.2021.131189
Zhang, Y., Huang, X., Liu, W., Zhang, G., Zhang, D., and Jiang, X. (2016). Organic nanoparticles formed by aggregation-induced fluorescent molecules for detection of hydrogen sulfide in living cells. Sci. China Chem. 59, 106–113. doi:10.1007/s11426-015-5543-2
Zhang, Y., Zhuang, W., Chen, J., Li, C., Li, S., and Chen, M. (2023). Aggregation-induced emission fluorescent probes for lipid droplets-specific bioimaging of cells and atherosclerosis plaques. Spectrochimica Acta Part A Mol. Biomol. Spectrosc. 286, 122017. doi:10.1016/j.saa.2022.122017
Zheng, Y., Lu, H., Jiang, Z., Guan, Y., Zou, J., Wang, X., et al. (2017). Low-power white light triggered AIE polymer nanoparticles with high ROS quantum yield for mitochondria-targeted and image-guided photodynamic therapy. J. Mat. Chem. B 5, 6277–6281. doi:10.1039/C7TB01443K
Zhou, H., Tang, J., Zhang, J., Chen, B., Kan, J., Zhang, W., et al. (2019). A red lysosome-targeted fluorescent probe for carboxylesterase detection and bioimaging. J. Mat. Chem. B 7, 2989–2996. doi:10.1039/C9TB00310J
Zhou, J., and Ma, H. (2016). Design principles of spectroscopic probes for biological applications. Chem. Sci. 7, 6309–6315. doi:10.1039/C6SC02500E
Zhou, J., Yuan, Y.-F., Zhuo, J.-B., and Lin, C.-X. (2018). Synthesis and characterization of cyclophane: the highly selective recognition of Fe3+ in aqueous solution and H2PO4− in acetonitrile solution. Tetrahedron Lett. 59, 1059–1064. doi:10.1016/j.tetlet.2018.02.005
Zhu, X., Hu, J., Zhao, Z., Sun, M., Chi, X., Wang, X., et al. (2015). Kinetic and sensitive analysis of tyrosinase activity using electron transfer complexes: in vitro and intracellular study. Small 11, 862–870. doi:10.1002/smll.201401595
Keywords: liver disease, enzymes, biosensors, early diagnosis, liver cancer
Citation: Liu S, Yin Y, Liu S, Wang C, Sun W and Hu X (2024) Shining a light on liver health: advancements in fluorescence-enhanced enzyme biosensors for early disease detection. Front. Bioeng. Biotechnol. 12:1392857. doi: 10.3389/fbioe.2024.1392857
Received: 28 February 2024; Accepted: 05 April 2024;
Published: 19 April 2024.
Edited by:
Abuelmagd Mahmoud Abdelmonem, Royal College of Surgeons in Ireland, IrelandReviewed by:
Lin Huang, Shanghai Jiao Tong University, ChinaXiaoming Hu, East China Jiaotong University, China
Copyright © 2024 Liu, Yin, Liu, Wang, Sun and Hu. This is an open-access article distributed under the terms of the Creative Commons Attribution License (CC BY). The use, distribution or reproduction in other forums is permitted, provided the original author(s) and the copyright owner(s) are credited and that the original publication in this journal is cited, in accordance with accepted academic practice. No use, distribution or reproduction is permitted which does not comply with these terms.
*Correspondence: Shifeng Liu, bGl1c2hpZmVuZzA5MDFAMTI2LmNvbQ==; Xiaokun Hu, aHV4aWFva3VuNzcwQDE2My5jb20=