- 1Shenzhen Hospital, Shanghai University of Traditional Chinese Medicine, Shenzhen, China
- 2Department of Urology, The Third Affiliated Hospital of Shenzhen University, Shenzhen University, Shenzhen, China
- 3Department of Urology, The Affiliated South China Hospital of Shenzhen University, Shenzhen University, Shenzhen, China
In the quest to tackle stress urinary incontinence (SUI), the synthesis of cutting-edge biomaterials and regenerative materials has emerged as a promising frontier. Briefly, animal models like vaginal distension and bilateral ovariectomy serve as crucial platforms for unraveling the intricacies of SUI, facilitating the evaluation of innovative treatments. The spotlight, however, shines on the development and application of novel biomaterials—ranging from urethral bulking agents to nano-gel composites—which aim to bolster urethral support and foster tissue regeneration. Furthermore, the exploration of stem cell therapies, particularly those derived from adipose tissues and urine, heralds a new era of regenerative medicine, offering potential for significant improvements in urinary function. This review encapsulates the progress in biomaterials and regenerative strategies, highlighting their pivotal role in advancing the treatment of SUI, thereby opening new avenues for effective and minimally invasive solutions.
1 Introduction
It is remarkable that SUI, a disorder impacting approximately one in three women and inducing distress on multiple fronts—physical, psychological, and social—does not garner more attention (Sims et al., 2022; Hu and Pierre, 2019). SUI significantly impacts normal lives, causing persistent anxiety and embarrassment, and imposes a significant economic burden on society (Hampel et al., 2004; Ford et al., 2017; Zhu et al., 2024). The incidence of urinary incontinence varies by age, as shown in Figure 1 (Gasquet et al., 2006). The occurrence of SUI is strongly associated with the bladder. Normally, urination is a voluntary process. When the bladder is full of urine, the pressure receptors on the bladder wall send signals to the brain, causing the sensation of the urge to urinate. The process of normal urination is depicted in Figure 2 (D'Ancona et al., 2019; Denisenko et al., 2021). SUI easily occurs when the anatomy of the pelvic nerves, urethra, and surrounding urethra area changes (Yang et al., 2022a; Ostrzenski, 2020). Research has confirmed that overweight and obesity are risk factors for SUI (Shang et al., 2023; Alsannan et al., 2024; Pang et al., 2023; Wang et al., 2023). The ideal treatment for SUI is not yet available. To enhance our understanding and treatment of urinary incontinence, this review aims to highlight the current advancements in the application of Urethral Bulking Agents (UBAs) and Tissue-engineered Repair Materials (TERMs) within the framework of disease models for SUI, underscoring the pivotal role of exploring biomaterials for therapeutic innovation.
2 Animal models in SUI
Animal models are essential in developing biomaterial therapies for SUI, enabling an understanding of the disease’s mechanisms and assessing therapies’ safety and effectiveness. These models replicate human SUI conditions, allowing researchers to test how biomaterials, such as UBAs and engineered scaffolds, repair urinary functions. They help optimize these materials for biocompatibility, degradation, and mechanical properties, ensuring suitability for human application. Additionally, animal studies are pivotal in devising minimally invasive techniques and guiding clinical trials, bridging the gap from laboratory research to clinical practice in SUI treatment. Disease models currently used for SUI are shown in Table 1.
Animal models often involving small animals such as rats, rabbits, and mice, are divided into two primary types: Induction and Spontaneity. Each type mirrors particular facets of SUI and exhibits distinct characteristics. These modeling techniques are primarily employed in small animals like rats, rabbits, and mice. In addition, large animals including goats (Burdzinska et al., 2018), sheep (Khalifa et al., 2022), pigs (Burdzinska et al., 2018; Burdzińska et al., 2012; Kelp et al., 2017; Boissier et al., 2016), dogs (Kato et al., 1999; Ali-El-Dein and Ghoneim, 2001; Eberli et al., 2009), and cynomolgus monkeys (Badra et al., 2013a; Williams et al., 2016a; Badra et al., 2013b) can also serve as SUI models. The evaluation of animal models is crucial to the successful establishment of the model. Pathological sections serve as the standard for determining the successful establishment of an SUI model, but other methods can also be utilized to ascertain the success of animal sampling, such as Sneeze Testing, Manual LPP Testing, Vertical Tilt Table LPP Testing, and Electrical Stimulation (ES) LPP Testing (Jiang and Damaser, 2011). The animal models commonly used in current research for SUI are shown in Figure 3.
2.1 Induced animal model
2.1.1 Vaginal distension
Vaginal distension (VD), or simulated birth trauma, is a method used to study the pathophysiology of SUI from childbirth and develop animal models. The procedure involves inserting a Foley catheter into the rat’s vagina, sealing the vaginal opening, and inflating the balloon with water or air. Within 4 h, edema is noticeable in the external urethral sphincter (EUS) and urethral/vaginal septum, with muscle disruption and fragmentation of the EUS occurring after 6 h. Inflammatory damage, indicated by polymorphonuclear leukocytes, peaks in the EUS after 4 h. Longer VD durations result in increased tissue edema, muscle damage, and changes in the urethra and vagina. After 2 weeks, the VD rats’ leak point pressure (LPP) significantly drops, with notable pathological differences persisting at 4 weeks in the urethra, pelvic ganglia, and levator muscle (Lin et al., 1998; Phull et al., 2011; Woo et al., 2009). Enhancing the model, a weight may be added to simulate traction injury (Wu et al., 2019). The specificity of EUS innervation and neurodegeneration suggests pudendal nerve vulnerability during VD (Damaser et al., 2003). While some believe model success may relate to the estrus cycle, studies indicate that the induction of reversible SUI in mice is not cycle-dependent (Huang et al., 2014).
2.1.2 Bilateral ovariectomy (OVX)
Under isoflurane anesthesia, rats underwent a bilateral ovariectomy (OVX) through dorsolateral incisions, 2 cm below the last rib, followed by sutured wound closure. Six weeks later, OVX rats showed a marked decrease in continence, especially during sneezing, increased body weight, and reduced uterine weight, indicating successful estrogen depletion (Kadekawa et al., 2020). Previous studies have linked estrogen levels with body and uterine weights (Yoshida et al., 2007). Aging, compared to OVX-induced estrogen deficiency, more significantly affects baseline urethral function over reflex activity of striated muscles, with estrogen deficiency further impairing continence reflexes. Thus, aging and estrogen deficiency synergistically impact urethral function and continence mechanisms, contributing to SUI development (Kitta et al., 2016).
2.1.3 Nerve injury
The pudendal nerve, located in the ischiorectal fossa, was thoroughly crushed twice, for a duration of 30 s on each side, near the obturator nerve’s branch point using a Castroviejo needle holder. The immediate visualization of the nerve injury was facilitated by the transparency of the nerve sheet at the crush location. This technique is termed pudendal nerve crush (PNC). Following LPP testing, it was observed that the control group’s LPP value was significantly higher than that of the pudendal nerve compression group (Damaser et al., 2003). Bilateral pudendal nerve resection at the level of the lumbosacral trunk anastomosis in rats constitutes the procedure known as pudendal nerve transection (PNT). HE and IHC staining revealed irreversible loss of striated muscle mass in the sphincter region and an increase in collagen deposition indicative of muscle atrophy. Significant decreases in LPP measurements were also observed following bilateral PNT (Khorramirouz et al., 2016). The underlying principle of pudendal nerve ligation (PNL) mirrors that of pudendal neurotomy. After nerve blockade, sensory loss in the innervated area and muscle loss of contractility occurs, leading to SUI (Furuta et al., 2008). Bilateral pelvic nerve injury (PNI) (Pinar et al., 2022; Lee et al., 2004), sciatic nerve transection (Lee et al., 2003), and impairment of the dorsal nerve of the clitoris (Cruz et al., 2016) can also induce SUI in rats.
2.1.4 Destruction of periurethral tissue
The content of smooth muscle in the cauterized group was significantly reduced, making vascular expression almost imperceptible due to the destruction of the tissue around the urethra through electrocautery (Hong et al., 2013). This model serves as a viable animal model for studying angiogenesis, neurogenic, and myogenic injuries. After the transection of the pubo-urethral ligament, the LPP value showed a significant decrease, mirroring the effects observed in the modeling method of nerve transection (Kefer et al., 2009).
2.1.5 Other methods
The nerve anesthetic botulinum-A toxin, a neuromuscular blocking agent, paralyzes muscle nerves and blocks the transmission of information between motor nerves and muscles. Following the administration of botulinum-A toxin around the urethra in rats, an increase in urinary output and muscle tissue atrophy were observed (Bandyopadhyay et al., 2014). Spinal cord injury (SCI) frequently results in neurogenic detrusor overactivity (NDO) as a result of the sprouting of sensory afferents on the lumbosacral spinal cord. NDO is characterized by a high frequency of voiding contractions and an increased intravesical pressure, potentially leading to SUI. Interestingly, injections of botulinum-toxin A into the bladder wall constitute an effective option to manage NDO (Coelho et al., 2016). To produce the retroflexed bladder, the bladder was surgically attached posteriorly to the psoas muscle. In rats with a retroflexed bladder, a marked reduction in both the urethral pressure reaction and sneeze-induced LPP was observed (Kawamorita et al., 2010). Inducing urinary bladder overactivity and urinary incontinence in mice through the diuretic Furosemide is another method (Saporito et al., 2016). SUI is prevalent in individuals with Parkinson’s disease (PD). Based on these clinical observations, a comorbid disease model of PD and SUI was developed. A rat model was induced through the injection of 6-hydroxydopamine. Depletion of dopamine significantly impairs the active urethral closure mechanism (Ouchi et al., 2019). To ensure the stability and suitability of the experimental animal model, combining multiple modeling methods is often advantageous. Examples include combining VD with OVX (Li et al., 2022; Li et al., 2016), PNT (Ni et al., 2018), and PNC (Balog et al., 2023).
2.2 Spontaneous animal models
The main reason for the popularity of spontaneous animal models lies in their ability to minimize artificial modeling factors, thereby more closely resembling naturally occurring human diseases. Among experimental animals exhibiting genetic obesity, Zucker fatty (ZF) rats represent the most widely utilized models. This condition is attributed to the recessive gene fa, resulting from a mutation in the Fa gene. The model includes two sublines: lean and obese types. Obese ZF rats exhibit characteristics remarkably similar to human obesity and the early stages of type II diabetes, including insulin resistance, an abnormal glucose tolerance test, and a normal fasting glucose. Furthermore, it presents a series of obesity-related complications, including high blood lipids and reduced reproductive capacity. Obesity impairs urethral sphincter function through IMCL deposition, leading to atrophy and distortion of urethral striated muscle. Therefore, ZF rats could serve as a consistent and reliable animal model for studying obesity-associated SUI (Wang et al., 2017). Interestingly, ZF rats exposed to single pulse electric field stimulation (EFS) during organ bathing experiments may exhibit SUI and compromised urethral sphincter contractility (Lee et al., 2017).
The double mutant GLI family zinc finger Gli2; Gli3+/−Δ699/+ murine model of SUI has recently been developed as a reliable model. Histological changes in the bladder neck and urethra can directly lead to SUI, with prostate hypoplasia being a possible additional factor. This may be related to premature termination of the spinal cord (Yadav et al., 2023).
Estrogen exerts its effects through two distinct nuclear receptors: estrogen receptor alpha (ERα) and estrogen receptor beta (ERβ). ERβ is believed to be associated with the onset of SUI. The primary site of ERβ expression is in the granulosa cells of the ovary. A significant decrease in LPP and maximum urethral closure pressure (MUCP) values in the ERβ−/− group, compared to the ERβ+ group, indicates that ERβ gene knockout plays a critical role in the development of SUI (Hamilton et al., 2017; Chen et al., 2014). The pathological mechanism of this approach is akin to that of OVX in rats, which influences estrogen levels.
In clinical practice, the risk of SUI escalates with age (Pearlman and Kreder, 2020). These clinical characteristics constitute the primary rationale for employing elderly rats in the SUI model. Compared to young (3 months) and middle-aged (12 months) rats, aged rats (24 months) exhibited a significant decrease in LPP value, an increase in the area of connective tissue in the EUS layer, a significant decrease in the number of EUS fibers, and an increase in the cross-sectional area of EUS fibers (Yanai-Inamura et al., 2019).
3 The current therapies
SUI is widely recognized as a global public health issue, with effective management significantly enhancing patients’ quality of life. Currently, a variety of therapeutic options are available. Clinicians with relevant experience should determine the appropriate intervention—conservative or surgical—based on the severity of the patient’s symptoms.
Non-surgical measures are generally recommended as the initial approach for managing SUI. Pharmacological treatments, including anti-muscarinic agents, antidepressants, estrogen, botulinum toxin, and α-adrenergic agonists, can increase urethral sphincter pressure or decrease bladder activity. However, when conservative measures fail to provide the desired therapeutic outcomes, surgical intervention becomes necessary.
In recent years, there have been significant advancements in surgical techniques for SUI. Options now include bladder neck injections, midurethral slings, vaginal suspensions, and autologous fascia slings (Nightingale, 2020). Table 2 summarizes the current methods used to treat SUI.
3.1 Conservative management
Although surgery can reduce leakage to some extent, it cannot fully resolve SUI (Nygaard and Norton, 2022). Other essential interventions include acupuncture, moxibustion, pelvic floor muscle training (PFMT), yoga, and lifestyle modifications.
Acupuncture and moxibustion, rooted in ancient Eastern traditions, treat diseases by stimulating acupoints and require consideration of individual differences for syndrome differentiation. While these methods have shown improvements in urine leakage (Liu et al., 2017), meta-analyses indicate that they are not superior to drug treatments (Wang et al., 2013). Improvements in research methodologies, including randomization, blinding, treatment interpretation, and acupuncturist certification, are needed (Yang et al., 2022b). Current statistical analysis methods in randomized controlled trials are also often inadequate (Liu et al., 2022).
PFMT for female SUI has received an A-level evidence rating based on numerous RCT meta-analyses (Dumoulin et al., 2011). Long-term follow-up can maintain the benefits of PFMT without additional training incentives. Significant differences exist in the quality of interventions between short-term and long-term PFMT studies, yet short-term results can be preserved during long-term follow-up (Bø and Hilde, 2013). The mechanisms include enhancing pelvic floor muscle strength, improving timing awareness, and strengthening core muscles (Sheng et al., 2022).
Yoga, a psychosomatic therapy, has been effective in improving urinary incontinence and can be combined with PFMT (Tenfelde and Janusek, 2014; Kim et al., 2015). Lifestyle modifications, such as weight reduction, can also alleviate urinary incontinence symptoms associated with obesity (Kerdraon and Denys, 2009). Massage treatment has shown significant reductions in urine leakage, with complete symptom alleviation after 1 month of discontinuing therapy in some cases (Kassolik et al., 2013).
Medical management is a crucial aspect of SUI treatment. Anti-muscarinic drugs, the first-line treatment for overactive bladder, include oxybutynin, propiverine, tolterodine, solifenacin, darifenacin, trospium, imidafenacin, and fesoterodine (Swinburn et al., 2011; Yamada et al., 2018). These drugs can cause side effects like dry mouth, blurred vision, and constipation, depending on their specificity for bladder muscarinic receptors. When anti-muscarinic drugs are unsuitable, β-adrenergic receptor agonists like mirabegron and solabegron are effective alternatives (Mostafaei et al., 2022; Ellsworth, 2012). Meta-analyses have shown their effectiveness comparable to anti-muscarinic drugs (Kelleher et al., 2018; Ohlstein et al., 2012).
Pharmacological research suggests lower urinary tract activity is centrally regulated by 5-HT and NE receptor agonists and antagonists. Duloxetine, a 5-hydroxytryptamine/norepinephrine reuptake inhibitor, treats SUI by inhibiting serotonin and norepinephrine reuptake at the presynaptic neuron in Onuf’s nucleus of the sacral spinal cord (Bitter et al., 2011; Jost and Marsalek, 2004). Research supports duloxetine as a viable treatment option for female SUI (Deepak et al., 2011; Mariappan et al., 2007; Basu and Duckett, 2009).
Hormone therapy is commonly used for lower urinary tract symptoms. Estrogen can increase urethral pressure, significantly improving or curing SUI in many cases (Elia and Bergman, 1993). However, evidence for estrogen supplementation’s effectiveness in improving urinary incontinence remains insufficient (Quinn and Domoney, 2009). In patients with bladder muscle overactivity and urinary incontinence due to nervous system disease, botulinum toxin injections have improved symptoms and bladder function without side effects (Kennelly et al., 2022).
3.2 Surgical management
When general management proves ineffective, surgical intervention becomes necessary. The advent of minimally invasive, retropubic, synthetic midurethral sling operations has revolutionized SUI surgery, surpassing traditional procedures like colposuspension and autologous fascial slings (Giarenis and Cardozo, 2014). The success of these surgeries depends on the surgeon’s experience, but risks include bladder, intestinal, or vascular damage via the obturator approach.
Surgical options for SUI include open abdominal retrovaginal suspension, anterior vaginal repair, suburethral suspension, bladder neck pin suspension, periurethral or transurethral injection of fillers, artificial urethral sphincters, and laparoscopic vaginal suspension (Kirchin et al., 2017). Among these, retrovaginal suspension, slings, and urethral dilation injections are the most commonly employed techniques. Urethral mid-suspension is a preferred minimally invasive procedure due to its low complication rate and favorable prognosis, while urethral dilation injections are safer for high-risk patients (Umoh and Arya, 2012).
The Uratape procedure, which involves tension-free tape insertion beneath the urethra, and transvaginal tape slings are commonly used techniques. Despite their effectiveness, complications such as bladder, intestinal, and vascular injuries can occur (Delorme et al., 2004; Reisenauer et al., 2006). The development of the midurethral sling (MUS) has been crucial, with various slings available that are inserted at the midurethral level either retropubically or transobturatorily (Rapp et al., 2009; Kane and Nager, 2008). MUS procedures typically involve minimal incisions, quick recovery, and high cure rates with minimal complications. Long-term outcomes are generally positive, with patients resuming normal activities shortly after surgery (Ford et al., 2017). Traditional slings are as effective as minimally invasive slings but have a higher incidence of adverse reactions. They exhibit a similar cure rate to open posterior pubic vaginal suspension, though long-term adverse events remain unclear (Rehman et al., 2017).
Urethral injections (periurethral/intraurethral) are a well-established minimally invasive treatment for uncomplicated SUI, particularly in patients unresponsive to conservative management or those contraindicated for drug treatment (Chapple et al., 2005). Various agents, such as autologous fat, carbon beads, calcium hydroxyapatite (CaHA), ethylene vinyl alcohol copolymer (EVOH), glutaraldehyde cross-linked bovine collagen, porcine dermal implants, polytetrafluoroethylene, and silicone particles, can be used as fillers. Despite insufficient evidence to fully guide clinical practice, urethral fillers may offer a cost-effective alternative to other surgical options (Kirchin et al., 2017).
4 The ideal material
The ideal biomaterial would be readily available, cost-effective, chemically and physically inert, sterile, non-carcinogenic, mechanically robust, resistant to alteration by bodily tissues, and carry a low risk of infection and rejection. After healing from incontinence surgery, the graft should restore the pelvis to its natural structure and function, mirroring the durability of autologous tissue. Furthermore, the material must remain in place long enough to be integrated by the host tissue. To minimize impact on the surrounding area during surgery, the biomaterial should be supple, able to withstand mechanical stress and shrinking, and flexible (Sangster and Morley, 2010).
Synthetic biomaterials have potential applications in treating urinary incontinence. Synthetic materials often address urinary incontinence problems through implants, injections, films, or tablets. They increase the ability of the urethra to close by strengthening the urethral sphincter or supporting the urethral tissue, thereby reducing urine leakage.
5 Urethral bulking agents (UBAs)
The primary mechanism by which UBAs work involves the injection of these materials around the urethra, leading to an increase in urethral volume. This augmentation helps to coapt the urethral lumen, enhancing the closure pressure at rest and improving the functional length of the urethra, which is crucial for maintaining continence.
5.1 Plasmid DNA-loaded injectable agent
The researcher prepared pDNA (encoding for bFGF) complex-loaded poly (dl-lactic-co-glycolic acid) (PLGA)/Pluronic F127 mixture solution dispersed with polycaprolactone (PCL) microspheres as an injectable bioactive bulking agent (Choi et al., 2013). The mixture solution was designed to solidify at the injured site upon contact with water or body fluid, thus enabling stable deposition of PCL microspheres in the solidified PLGA matrix to maintain its initial volume without migration. The researcher anticipated that the prepared bulking agent could provide a passive bulking effect (by PCL microspheres) and allow prolonged stimulation of the defect tissues around the urethra (through long-term production of bFGF from the cells transfected by continuously released pDNA) for the effective treatment of SUI.
5.2 Macro/nano-gel composite
Nanogels present several advantages for drug delivery, including high biocompatibility from their natural or synthetic polymer composition, which reduces immune reactions and toxicity. They enable controlled drug release, with their network structure allowing for modulated release rates, which improves efficacy and reduces side effects. Additionally, encapsulated drugs in nanogels are protected, increasing their stability. Surface modifications of nanogels enhance targeting to specific cells, making them an effective drug delivery system.
Researchers have discovered a specific macro/nanogel type useable as an injectable, bioactive bulking agent for treating incontinence. It comprises in situ generated gelatin-based macrogels and self-assembling heparin-based nanogels. These hybrid hydrogels are produced via an enzymatic process involving hydrogen peroxide and horseradish peroxidase. To create a hybrid gel matrix capable of continuously releasing growth factor (GF) for up to 28 days, a gelatin gel matrix was mixed with a GF-loaded heparin nanogel. Furthermore, these hydrogel composites promote the regeneration of urethral muscle tissue around the urethral wall, aiding in the restoration of biological function upon in vivo injection (Park et al., 2014). The method for the regeneration and creation of composites combining macro and nanogel is illustrated in Figure 4.
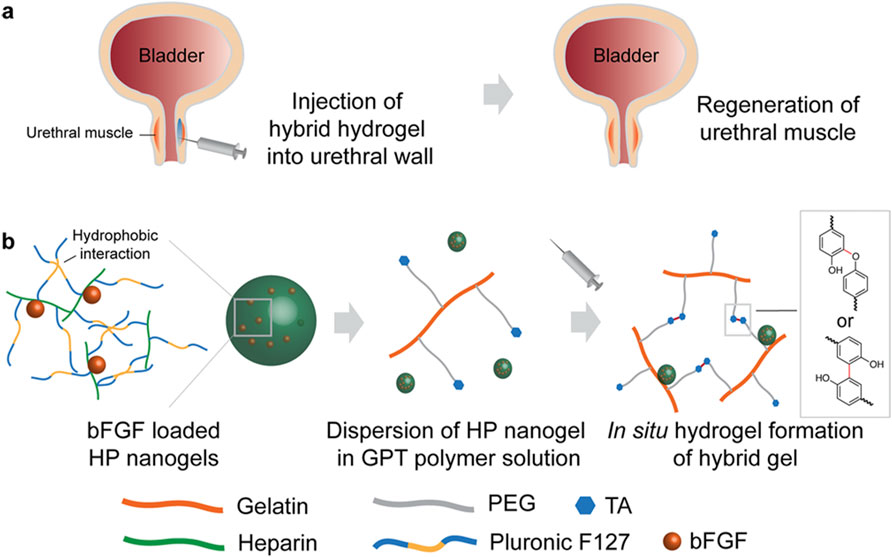
Figure 4. Regeneration approach and construction of hybrid macro/nanogel composites. (A) Diagram illustrating urethral muscle regeneration utilizing an injectable, bioactive bulking hydrogel. (B) Formation of bFGF-loaded HP nanogels via hydrophobic interactions. Reprinted with permission from ref. (Park et al., 2014). Copyright 2014, ACS.
5.3 Liquid crystal elastomer based dynamic device for urethral support
This is a novel device of liquid crystal elastomers (LCE) inspired by static slings that non-invasively adjust their tension by changing shape in response to temperature increases induced by transcutaneous infrared light, thereby reducing urethral resistance and improving urination, studies have shown that the LCE-CB device can treat SUI by increasing the urethral resistance to leakage under the application of abdominal pressure (Tasmim et al., 2023).
5.4 CAD/CAM collagen scaffolds for tissue engineering
The present research has established a method utilizing CAD/CAM technology for the creation of a scaffold composed entirely of collagen, characterized by its high porosity and patterned structure. The dense packing and alignment of the collagen molecules have endowed the scaffold with significant mechanical strength. It has been indicated that adjustments to the structure’s number of layers and its shape could allow for the tuning of its mechanical characteristics to suit various applications in tissue engineering, including SUI (Islam et al., 2015). The scaffold is shown in Figure 5.
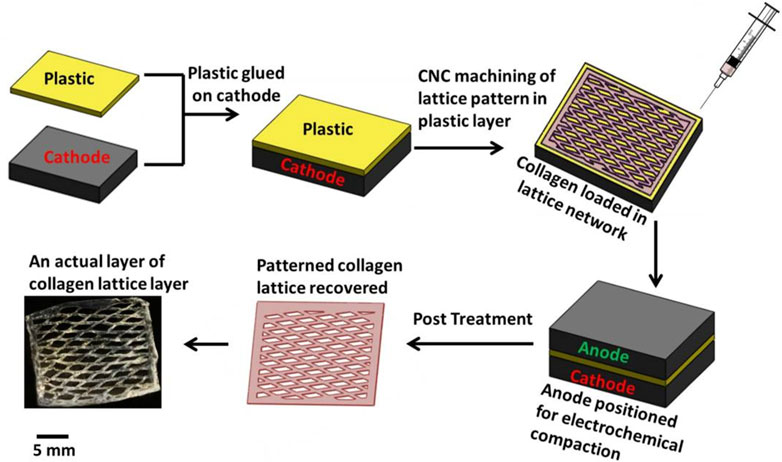
Figure 5. Process of fabricating individual patterned layer. Reprinted with permission from ref. (Islam et al., 2015). Copyright 2015, IOP Publishing.
5.5 Other commercial UBAs
5.5.1 Ethylene vinyl alcohol (Tegress™)
Ethylene vinyl alcohol (EVA) copolymer serves as a permanent filler. It exhibits good biocompatibility and a low propensity for allergic reactions (Kuhn et al., 2008). However, a follow-up study involving 20 patients revealed that a significant proportion experienced serious complications. These complications included surgical-related issues and urinary tract erosion (Hurtado et al., 2007). A subsequent study also reported similar complications (Hurtado et al., 2008). Due to these studies and growing reports of complications associated with Tegress™, the manufacturer voluntarily removed the bulking agent from the market in December 2007 (Mukkamala et al., 2013).
5.5.2 Dextranomer/hyaluronic acid copolymer (Deflux™, Zuidex™)
Dextranomer, a highly hydrophilic dextran polymer solubilized in a base of non-animal stabilized hyaluronic acid, has received approval as an injectable agent for childhood vesicoureteric reflux (Deflux™) and, in Europe, for women with SUI (Zuidex™) (Lightner et al., 2010). A study comparing Deflux™ and Teflon™ as bladder neck injections in children found that neither the number of injections nor the type of filling agent impacted the outcome: short-term improvements were observed, but long-term effects were lacking]. Researchers have concluded that bladder neck injections of filling agents are generally ineffective for treating incontinence (Dyer et al., 2007). Another dextranomer/hyaluronic acid copolymer, Zuidex™, used as a urinary filler, has shown through clinical evidence to significantly improve patients’ subjective quality of life and objective incontinence measurements post-treatment (van Kerrebroeck et al., 2004). However, reports of side effects have emerged, including the formation of uretrovaginal fibrosis associated with “sterile abscission” post-treatment (Hilton, 2009).
5.5.3 Glutaraldehyde rross-linked collagen (Contigen™)
Glutaraldehyde cross-linked (GAX) collagen, a sterile injection gel, is composed of highly purified bovine dermal collagen, cross-linked with 0.0075% glutaraldehyde, and suspended in physiological saline solution with 0.3% lidocaine. After receiving FDA approval in 1985, it has been primarily used as an intradermal therapy for correcting soft tissue contouring abnormalities. Subsequently, its applications expanded to include use as a urethral filler for urinary incontinence. While its efficacy is supported by clinical evidence (Stricker and Haylen, 1993; Shortliffe et al., 1989), some studies question its long-term effectiveness (Sundaram et al., 1997). Currently, reported adverse reactions include local injection calcification (Knudson et al., 2006), hematuria, urinary retention, urethral mucosal prolapse, among others (Stothers et al., 1998; Harris et al., 1998). In 2012, GAX was officially withdrawn from the European market (Lüttmann et al., 2019).
5.5.4 Calcium hydroxylapatite (Coaptite™)
Hydroxyapatite calcium (CaHA, Coapatite™) consists of spherical CaHA particles with diameters ranging from 75 to 125 microns and was approved by the US FDA in 2005 (Csuka et al., 2023). Evidence suggests that hydroxyapatite particles may serve as effective fillers for treating stress incontinence (Mayer et al., 2001; Griffin et al., 2016). A 12-month clinical study comparing CaHA (Coapatite) with Contigen™ found that both CaHA and collagen were well tolerated, with no systemic adverse events observed for either product (Mayer et al., 2007).
5.5.5 Carbon coated beads (Durasphere™)
Durasphere™, an injectable bulking agent featuring carbon-coated beads, has FDA approval for treating SUI. Its confirmed and potential benefits include non-immunogenicity, tissue non-responsiveness, efficacy with minimal injection volume, and durability (Pannek et al., 2001; Madjar et al., 2003). Unfortunately, similar to Contigen™, concerns exist regarding Durasphere’s long-term efficacy (Chrouser et al., 2004). Carbon-coated beads are not superior to current alternative filler biomaterials due to consistent particle migration and limited success (Pannek et al., 2001).
5.5.6 Polytetrafluoroethylene (Teflon™)
Polytetrafluoroethylene (PTFE) is a type of plastic fluoropolymer material (Becmeur et al., 1990). Previously, Teflon™ was utilized as a urethral filler for treating urinary incontinence (Kaufman et al., 1984). This method involves injecting granules into the tissues surrounding the urethra to increase closing pressure and alleviate urinary incontinence symptoms. However, research has identified potential problems and complications associated with this treatment method. One of the primary concerns involves potential organizational reactions and side effects. Long-term use of this filler material can result in complications, including tissue inflammation, infection, foreign body reactions, and urethral stricture. Additionally, the filler material may shift or disperse within the surrounding urethral tissues, resulting in unstable treatment outcomes (McKinney et al., 1995; Kiilholma et al., 1993; Martínez-Piñeiro et al., 1989; Kiilholma and Mäkinen, 1991).
5.5.7 Polydimethylsiloxane (Urolastic™) and cross-linked polydimethylsiloxane (Macroplastique™)
Polydimethylsiloxane (Urolastic™), an organic polymer containing silicon, is widely used in medical and biological fields. It has been approved by the Food and Drug Administration in 2006 as a treatment option for urinary incontinence (Serati et al., 2023). Injecting polydimethylsiloxane into the tissue surrounding the urethra increases its support and function. The treatment aims to enhance urethral sphincter function and reduce urinary incontinence symptoms. Three-year cohort research suggests Macroplastique™ as a viable SUI treatment alternative, offering long-lasting results and a low incidence of side effects (Serati et al., 2019). A multicenter, randomized, controlled, single-blind study shows cross-linked polydimethylsiloxane to be more effective than Contigen™ (Ghoniem et al., 2009).
5.5.8 Pig collagen protein (Permacol™)
A bioactive, injectable bulking agent comprising Permacol™ and recombinant insulin-like growth factor-1 conjugated to fibrin micro-beads (fib_rIGF-1), known for its bulk stability and ability to induce muscle regeneration. Research has confirmed its ability to promote angiogenesis at the injection site and to foster smooth muscle tissue formation (Vardar et al., 2019). A prospective randomized trial revealed Permacol’s superiority over Macroplastique™ in treatment efficacy (Bano et al., 2005). Additionally, it significantly enhances function and quality of life in cases of constipation, fecal incontinence, and prolapse, and also ameliorates the ecological symptoms of the urinary system (McLean et al., 2018).
5.5.9 Biomimetic synthetic materials (Regensling™)
The aim of Regensling™, a synthetic sling device featuring biomimetic construction, is to provide long-term mechanical support while minimizing the risk of complications. Regensling™ exhibited a milder inflammatory response compared to the control material and was enveloped in a thin layer of fibrous tissue, showing good compliance. Throughout the testing, Regensling™ demonstrated consistent strength, with a notable upward trend (Lai et al., 2017).
5.5.10 Polyacrylamide hydrogel (Bulkamid®, Aquamid™)
Polyacrylamide hydrogel (PAHG) includes Bulkamid® and Aquamid™. Research has confirmed that Bulkamid® is at least as effective as Contigen™. Bulkamid® has shown positive, long-lasting effects on stress incontinence with minimal risk of significant side effects. With Contigen™ no longer on the market, Bulkamid® emerges as a promising treatment option for women with stress incontinence due to its straightforward bulking method (Sokol et al., 2014; Brosche et al., 2021). Overall, 21% of women did not respond adequately to PAHG (Bulkamid®) injection therapy. The therapy had few moderate short-term adverse effects and no significant long-term adverse events (Hoe et al., 2022). For PAHG (Aquamid™), treatment outcomes are similar, albeit with some adverse events like urinary retention and urinary tract infections. Similar results were observed with PAHG (Aquamid™) therapy, though side effects such as bladder retention and urinary tract infections were reported (Lose et al., 2006). Commercial UBAs are shown in Table 3.
6 Tissue-engineered repair materials (TERMs)
The application of stem cells in tissue engineering and repair materials constitutes a significant advancement in the field of regenerative medicine, largely thanks to the unique properties of stem cells, such as their ability for self-renewal and potential to differentiate into various cell types. The primary sources of stem cells include embryos, fetuses, and adult tissues, as well as somatic cells that have been reprogrammed through genetic modification to differentiate. These are known as induced pluripotent stem cells (iPSCs) (Bacakova et al., 2018). In tissue engineering, stem cells can enhance tissue recovery by either directly integrating with and replacing damaged tissues (through differentiation) or by secreting factors that regulate the host’s response (via paracrine signaling) (Hakim et al., 2015). Table 4 summarizes the regenerative materials used to treat SUI.
6.1 Stem cell therapy
6.1.1 Urine-derived stem cells
Cells from voided urine of women were reprogrammed into iPSCs. These iPSCs line U1 and hESC line H9 were differentiated into fibroblasts expressing specific markers. Myogenic differentiation was induced by CHIR99021 and confirmed by the expression of myogenic factors. Human iPSC-derived fibroblasts and myocytes were engrafted into the periurethral region of RNU rats, with successful tracking using ferric nanoparticles. This method allows scalable derivation, culture, and in vivo tracing of patient-specific cells to regenerate urethral damage and restore continence in rat SUI models. The research schematic is in Figure 6.
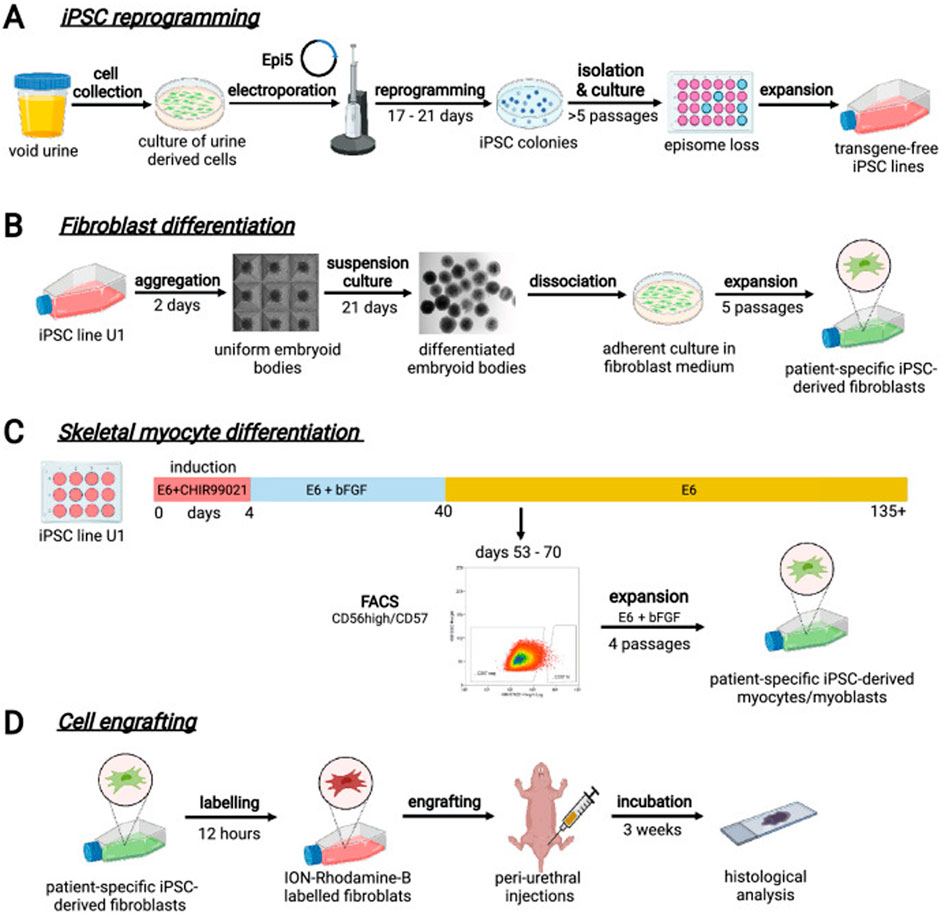
Figure 6. Schematic diagram of urine-derived stem cell therapy for SUI. (A) Reprogramming iPSCs from urine samples. (B) Fibroblast differentiation. (C) Skeletal myocyte differentiation. (D) Engraftment of cells into rat tissues followed by histological evaluation. Reprinted with permission from ref. (Kibschull et al., 2023). Copyright 2023, Springer Nature.
6.1.2 Adipose-derived stem cells
The great potential of murine ADSC for the regeneration of urinary sphincter function has been reported. Significant and complete recovery of urethral sphincter function was observed after injection of ADSC, triggering stronger CD45+ leukocyte infiltration compared with the control group (Knoll et al., 2024).
6.1.3 Bone marrow derived stem cells
This result has also been confirmed in human MSDC cells. Human mesenchymal stem cells can alter tissue response to acute injury and improve tissue function (Sadeghi et al., 2020). To achieve directed differentiation and sustained release of stem cells, the researchers transduced elastin into bone marrow mesenchymal stem cells (BMSCs) and used bFGF to differentiate the elastin-expressing BMSCs into fibroblasts, which produce collagen and elastin. Achieving sustained release of bFGF by formulating it in polylactic-glycolic acid (PLGA) nanoparticles resulted in significant improvements in urodynamics in disease models following treatment (Jin et al., 2016a).
6.1.4 Dental pulp-derived stem cells
Human dental pulp stem cells (DPSCs) exhibit promising therapeutic effects for treating SUI. These cells have shown the ability to differentiate into muscle-related cells in laboratory conditions. After being injected, within 4 weeks, DPSCs were effectively integrated into the external urethral sphincter, almost completely restoring its thickness. This differentiation towards muscle cells persisted in the body, promoting blood vessel formation and substantially aiding in the restoration of urinary control. Additionally, the detection of hDPSCs within nerve tissues indicates their potential role in the healing of cut nerves (Zordani et al., 2019).
6.1.5 Stem cell delivery system
The long-term efficacy of using stem cells directly to participate in the repair of urinary incontinence tissue is unsatisfactory, mainly due to the retention time and quantity of stem cells after injection. Thus, researchers have developed a new cell delivery system for injection therapy using superparamagnetic iron oxide (SPIO). Simply put, by constructing magnetized cells targeted and anchored at the target site, dispersed cells can be prevented from leaking into surrounding tissues after injection, thereby improving cell retention and enhancing treatment efficacy (Wang et al., 2020a).
6.2 Extracellular components
6.2.1 Extracellular matrix fragments
The researchers injected extracellular matrix fragments of adipose stem cell sheets (ADSC ECM) into the rat urethra, where they fully integrated with surrounding tissue within 1 week. Four weeks after transplantation, host cells regenerated within the ADSC ECM fragment-injected area. Furthermore, positive staining for myosin confirmed that new smooth muscle tissue had formed around ADSC ECM fragments. These results suggest that injection of ECM fragments may be a promising minimally invasive approach to treat SUI (Wang et al., 2020b).
6.2.2 Exosomes
Exosomes are nanosized membrane vesicles secreted by cells. They are a component of the intercellular communication system, carrying diverse payloads and mediating both pathogenetic and therapeutic actions, depending on the environment and cell sources (Floriano et al., 2020). Researchers extracted urine-derived stem cells exosomes (USCs-Exo) from urine and locally injected them into the pubococcygeus muscle and surrounding areas in a rat model of urinary incontinence, leading to significant improvements in urodynamic parameters. Molecular experiments revealed that USCs Exo promotes ERK phosphorylation in vitro and the activation, proliferation, and myotube differentiation of rat skeletal muscle SCs (Wu et al., 2019). Adipose-derived mesenchymal stem cell-secreted extracellular vesicles have been found to regulate type I collagen metabolism in fibroblasts, treating urinary incontinence (Liu et al., 2018). Human platelet-derived extracellular vesicles have shown to enhance myoblast proliferation and restore urethral sphincter function after sustained in vivo release. However, their very short half-life often limits significant benefits in clinical applications. A highly stable exocrine platform enables continuous release in the bioenhanced hydrogel. In a pig model of SUI, delivering PEP bioenhanced collagen one induced functional recovery of the EUS (Rolland et al., 2022). M2 macrophage-derived exosomes (M2-EXO) have been shown to reduce inflammatory cell infiltration in the SUI model and offer therapeutic effects on damaged pubococcygeal muscles (Zhou et al., 2021). ES enhances the expression of neurotrophic factors by Schwann cells, encouraging nerve cell regeneration. Meanwhile, Schwann cell-derived extracellular vesicles can stimulate neural activity, playing a role in providing therapeutic benefits for urinary incontinence (Hu et al., 2019; Zhou et al., 2018).
6.3 Other tissue-engineered repair materials
Platelet-rich plasma (PRP) is a multifunctional preparation widely used in regenerative medicine (Sánchez et al., 2017). It is rich in platelets as well as a large number of cytokines, chemokines and growth factors (Mussano et al., 2016). Researchers have demonstrated that injecting PRP into the urinary sphincter to repair damaged tissue and rejuvenate aged cells (Lee et al., 2022).
Intra-arterial injection of rat mesoangioblasts (MABs) and stable Vascular Endothelial Growth Factor (VEGF)-expressing MABs, improve recovery of urethral and vaginal function following simulated vaginal delivery (SVD) (Mori da Cunha et al., 2023). An important soluble GF secreted primarily from MABs is VEGF, which has myogenic and neuroprotective effects and induces nerve regeneration (Shimizu-Motohashi and Asakura, 2014; Borselli et al., 2010; Sun et al., 2003; Park et al., 2016).
Subcutaneous fat is a viable source of stromal vascular fraction (SVF) and is considered a source of autologous progenitor cells for tissue engineering and regenerative medicine applications. In a preclinical study using a large animal (pig) model of urethral injury, it was found that SVF obtained from autologous fat aids in the anatomical and functional recovery of the damaged urethra (Boissier et al., 2016). Similar results were also verified in a rat urinary incontinence model (Inoue et al., 2018).
The intraurethral administration of finely chopped autologous muscle tissue presents a straightforward surgical method that safe and fairly effective for women experiencing uncomplicated SUI. This technique is favorably comparable to a more complex regenerative approach that employs muscle-derived cells expanded in vitro (Gräs et al., 2014).
Inhibiting MicroRNA-29a-3p led to an increase in both the expression and secretion of elastin in BMSCs cultured in vitro. Furthermore, when BMSCs with MicroRNA-29a-3p inhibition were co-injected with PLGA encapsulating bFGF nanoparticles into rats with pelvic floor dysfunction (PFD) in vivo, there was a notable enhancement in the outcomes of urodynamic tests (Jin et al., 2016b).
Autologous skeletal muscle precursor cells (skMPC) therapy has been reported to treat urinary incontinence in a monkey disease model. Interestingly, researchers found that the effectiveness of skMPC therapy diminished in older monkeys, those with higher body weights, and monkeys experiencing psychosocial stress, which is linked to decreased production of endogenous estrogen (Williams et al., 2017; Williams et al., 2016b).
Inducing stem cell homing effect is also a good choice for the treatment of SUI. Researchers have developed a new injectable hydrogel based on beta-chitin (Yang et al., 2023). The rapid gel-forming properties of hydrogels with a 3% concentration can quickly stabilize SDF-1 and bFGF inside the hydrogel and delay their release in vivo. In the early stage, the hydrogel’s stiffness provides urethral support, similar to the TVT-O slings. In the later stage, released factors induce BMSCs to home and differentiate into fibroblasts, and the three-dimensional structure of hydrogels can stabilize BMSCs and form a cell microenvironment, which promotes fibroblast replenishment and collagen production, thereby repairing the support structure under the urethra and alleviating SUI symptoms. The system preparation and mechanism process are shown in Figure 7.
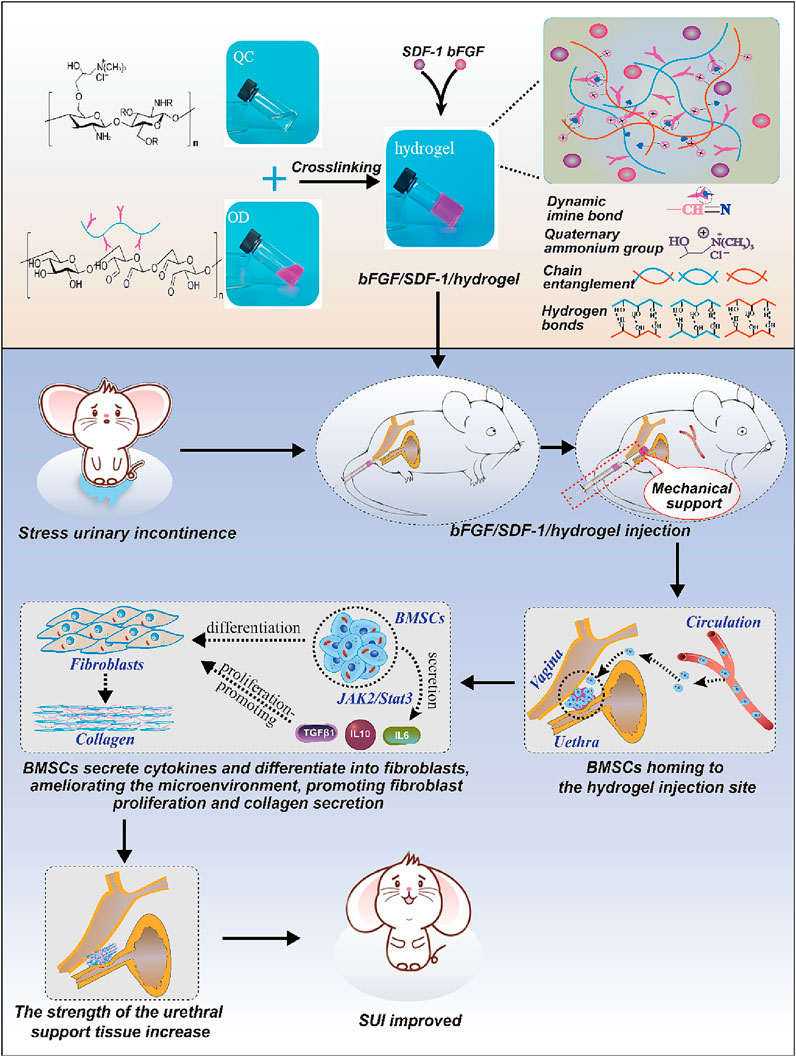
Figure 7. Schematic diagram of induced stem cell homing treatment for SUI. Reprinted with permission from ref. (Yang et al., 2023). Copyright 2023, Elsevier.
7 Conclusion and perspectives
This study highlights the role of both induced and spontaneous animal models in investigating SUI’s pathophysiology and the impact of innovative treatments. It delves into the potential of various biomaterials and therapeutic strategies, emphasizing the synergy between cutting-edge materials, cell therapy, and tissue engineering in addressing urinary incontinence. The findings indicate that biomaterials, such as UBAs and TERMs, along with stem cells from adipose tissue and urine, are pivotal in enhancing urethral support and regenerating sphincter function. Innovations like CAD/CAM collagen scaffolds and injectable hydrogels are making strides towards customizable, minimally invasive solutions tailored to patient needs. However, treatment variability and potential complications highlight the need for further research and therapeutic refinement. Future efforts will focus on precision in cell delivery, durability of biomaterials, and minimizing adverse effects, aiming to develop more effective and less invasive treatments for urinary incontinence, thereby enhancing patient quality of life. This outlook encourages a multidisciplinary strategy to advance urinary incontinence therapies, leveraging advancements in biomaterials, stem cell science, and tissue engineering.
Author contributions
XT: Investigation, Methodology, Project administration, Visualization, Writing–original draft, Validation. GL: Conceptualization, Funding acquisition, Supervision, Writing–review and editing. CL: Investigation, Writing–review and editing, Methodology. CK: Investigation, Writing–review and editing. HL: Conceptualization, Funding acquisition, Supervision, Writing–review and editing. SW: Conceptualization, Funding acquisition, Supervision, Writing–review and editing.
Funding
The author(s) declare that financial support was received for the research, authorship, and/or publication of this article. This work was partially supported by the National Natural Science Foundation of China (92359202), the Shenzhen Key Medical Discipline (No. SZXK021), and the Sanming Project of Medicine in Shenzhen (No. SASM202201024).
Conflict of interest
The authors declare that the research was conducted in the absence of any commercial or financial relationships that could be construed as a potential conflict of interest.
Publisher’s note
All claims expressed in this article are solely those of the authors and do not necessarily represent those of their affiliated organizations, or those of the publisher, the editors and the reviewers. Any product that may be evaluated in this article, or claim that may be made by its manufacturer, is not guaranteed or endorsed by the publisher.
References
Ali-El-Dein, B., and Ghoneim, M. A. (2001). Effects of selective autonomic and pudendal denervation on the urethral function and development of retention in female dogs. J. urology 166, 1549–1554. doi:10.1097/00005392-200110000-00089
Alsannan, B., Alharmi, J., Alrahal, F., Al Mansoor, S., and Tulandi, T. (2024). Prevalence and quality of life among overweight and obese women with different severity and types of urinary incontinence. Med. Princ. Pract. Int. J. Kuwait Univ. Health Sci. Centre 33, 47–55. doi:10.1159/000534651
Bacakova, L., Zarubova, J., Travnickova, M., Musilkova, J., Pajorova, J., Slepicka, P., et al. (2018). Stem cells: their source, potency and use in regenerative therapies with focus on adipose-derived stem cells - a review. Biotechnol. Adv. 36, 1111–1126. doi:10.1016/j.biotechadv.2018.03.011
Badra, S., Andersson, K. E., Dean, A., Mourad, S., and Williams, J. K. (2013a). A nonhuman primate model of stable urinary sphincter deficiency. J. urology 189, 1967–1974. doi:10.1016/j.juro.2012.09.103
Badra, S., Andersson, K. E., Dean, A., Mourad, S., and Williams, J. K. (2013b). Long-term structural and functional effects of autologous muscle precursor cell therapy in a nonhuman primate model of urinary sphincter deficiency. J. urology 190, 1938–1945. doi:10.1016/j.juro.2013.04.052
Balog, B. M., Deng, K., Askew, T., Hanzlicek, B., Kuang, M., and Damaser, M. S. (2023). Brain-derived neurotrophic factor is indispensable to continence recovery after a dual nerve and muscle childbirth injury model. Int. J. Mol. Sci. 24, 4998. doi:10.3390/ijms24054998
Bandyopadhyay, B., Thakur, A., Dave, V., Viswanathan, C., and Ghosh, D. (2014). A non-invasive method to evaluate the efficacy of human myoblast in botulinum-A toxin induced stress urinary incontinence model in rats. Urology J. 10, 1126–1134.
Bano, F., Barrington, J. W., and Dyer, R. (2005). Comparison between porcine dermal implant (Permacol) and silicone injection (Macroplastique) for urodynamic stress incontinence. Int. urogynecology J. pelvic floor Dysfunct. 16, 147–150. doi:10.1007/s00192-004-1216-y
Basu, M., and Duckett, J. R. (2009). Update on duloxetine for the management of stress urinary incontinence. Clin. interventions aging 4, 25–30. doi:10.2147/cia.s4062
Becmeur, F., Geiss, S., Laustriat, S., Bientz, J., Marcellin, L., and Sauvage, P. (1990). History of teflon. Eur. Urol. 17, 299–300. doi:10.1159/000464064
Bitter, I., Filipovits, D., and Czobor, P. (2011). Adverse reactions to duloxetine in depression. Expert Opin. drug Saf. 10, 839–850. doi:10.1517/14740338.2011.582037
Bø, K., and Hilde, G. (2013). Does it work in the long term? A systematic review on pelvic floor muscle training for female stress urinary incontinence. Neurourol. urodynamics 32, 215–223. doi:10.1002/nau.22292
Boissier, R., Magalon, J., Sabatier, F., Veran, J., Giraudo, L., Giusiano, S., et al. (2016). Histological and urodynamic effects of autologous stromal vascular fraction extracted from fat tissue with minimal ex vivo manipulation in a porcine model of intrinsic sphincter deficiency. J. urology 196, 934–942. doi:10.1016/j.juro.2016.04.099
Borselli, C., Storrie, H., Benesch-Lee, F., Shvartsman, D., Cezar, C., Lichtman, J. W., et al. (2010). Functional muscle regeneration with combined delivery of angiogenesis and myogenesis factors. Proc. Natl. Acad. Sci. U. S. A. 107, 3287–3292. doi:10.1073/pnas.0903875106
Brosche, T., Kuhn, A., Lobodasch, K., and Sokol, E. R. (2021). Seven-year efficacy and safety outcomes of Bulkamid for the treatment of stress urinary incontinence. Neurourol. urodynamics 40, 502–508. doi:10.1002/nau.24589
Burdzińska, A., Crayton, R., Dybowski, B., Koperski, Ł., Idziak, M., Fabisiak, M., et al. (2012). Urethral distension as a novel method to simulate sphincter insufficiency in the porcine animal model. Int. J. urology official J. Jpn. Urological Assoc. 19, 676–682. doi:10.1111/j.1442-2042.2012.02994.x
Burdzinska, A., Dybowski, B., Zarychta-Wiśniewska, W., Kulesza, A., Butrym, M., Zagozdzon, R., et al. (2018). Intraurethral co-transplantation of bone marrow mesenchymal stem cells and muscle-derived cells improves the urethral closure. Stem cell Res. and Ther. 9, 239. doi:10.1186/s13287-018-0990-2
Chapple, C. R., Wein, A. J., Brubaker, L., Dmochowski, R., Pons, M. E., Haab, F., et al. (2005). Stress incontinence injection therapy: what is best for our patients? Eur. Urol. 48, 552–565. doi:10.1016/j.eururo.2005.06.012
Chen, Y. H., Chen, C. J., Yeh, S., Lin, Y. N., Wu, Y. C., Hsieh, W. T., et al. (2014). Urethral dysfunction in female mice with estrogen receptor β deficiency. PloS one 9, e109058. doi:10.1371/journal.pone.0109058
Choi, S. J., Oh, S. H., Kim, I. G., Chun, S. Y., Lee, J. Y., and Lee, J. H. (2013). Functional recovery of urethra by plasmid DNA-loaded injectable agent for the treatment of urinary incontinence. Biomaterials 34, 4766–4776. doi:10.1016/j.biomaterials.2013.03.045
Chrouser, K. L., Fick, F., Goel, A., Itano, N., Sweat, S., and Lightner, D. (2004). Carbon coated zirconium beads in β-glucan gel and bovine glutaraldehyde cross-linked collagen injections for intrinsic sphincter deficiency: continence and satisfaction after extended followup. J. urology 171, 1152–1155. doi:10.1097/01.ju.0000103688.83606.06
Coelho, A., Oliveira, R., Cruz, F., and Cruz, C. D. (2016). Impairment of sensory afferents by intrathecal administration of botulinum toxin A improves neurogenic detrusor overactivity in chronic spinal cord injured rats. Exp. Neurol. 285, 159–166. doi:10.1016/j.expneurol.2016.05.029
Cruz, Y., Juárez, R., Medel, A., Corona-Quintanilla, D. L., Pacheco, P., and Juárez, M. (2016). Coital urinary incontinence induced by impairment of the dorsal nerve of the clitoris in rats. J. urology 195, 507–514. doi:10.1016/j.juro.2015.06.105
Csuka, D. A., Ha, J., Hanna, A. S., Kim, J., Phan, W., Ahmed, A. S., et al. (2023). Foreign body granuloma development after calcium hydroxylapatite injection for stress urinary incontinence: a literature review and case report. Arab J. urology 21, 118–125. doi:10.1080/2090598x.2022.2146859
Damaser, M. S., Broxton-King, C., Ferguson, C., Kim, F. J., and Kerns, J. M. (2003). Functional and neuroanatomical effects of vaginal distention and pudendal nerve crush in the female rat. J. urology 170, 1027–1031. doi:10.1097/01.ju.0000079492.09716.43
D'Ancona, C., Haylen, B., Oelke, M., Abranches-Monteiro, L., Arnold, E., Goldman, H., et al. (2019). The International Continence Society (ICS) report on the terminology for adult male lower urinary tract and pelvic floor symptoms and dysfunction. Neurourol. urodynamics 38, 433–477. doi:10.1002/nau.23897
Deepak, P., Kumar, T. N., and Sen, T. K. (2011). Evaluation of efficacy of duloxetine in stress urinary incontinence in women. Indian J. Pharmacol. 43, 176–179. doi:10.4103/0253-7613.77357
Delorme, E., Droupy, S., de Tayrac, R., and Delmas, V. (2004). Transobturator tape (Uratape®): a new minimally-invasive procedure to treat female urinary incontinence. Eur. Urol. 45, 203–207. doi:10.1016/j.eururo.2003.12.001
Denisenko, A. A., Clark, C. B., D'Amico, M., and Murphy, A. M. (2021). Evaluation and management of female urinary incontinence. Can. J. urology 28, 27–32.
Dumoulin, C., Glazener, C., and Jenkinson, D. (2011). Determining the optimal pelvic floor muscle training regimen for women with stress urinary incontinence. Neurourol. urodynamics 30, 746–753. doi:10.1002/nau.21104
Dyer, L., Franco, I., Firlit, C. F., Reda, E. F., Levitt, S. B., and Palmer, L. S. (2007). Endoscopic injection of bulking agents in children with incontinence: dextranomer/hyaluronic acid copolymer versus polytetrafluoroethylene. J. urology 178, 1628–1631. doi:10.1016/j.juro.2007.05.092
Eberli, D., Andersson, K. E., Yoo, J. J., and Atala, A. (2009). A canine model of irreversible urethral sphincter insufficiency. BJU Int. 103, 248–253. doi:10.1111/j.1464-410X.2008.08001.x
Elia, G., and Bergman, A. (1993). Estrogen effects on the urethra: beneficial effects in women with genuine stress incontinence. Obstetrical and Gynecol. Surv. 48, 509–517. doi:10.1097/00006254-199307000-00028
Ellsworth, P. (2012). Treatment of overactive bladder symptoms beyond antimuscarinics: current and future therapies. Postgrad. Med. 124, 16–27. doi:10.3810/pgm.2012.05.2544
Floriano, J. F., Willis, G., Catapano, F., de Lima, P. R., Reis, F. V. D. S., Barbosa, A. M. P., et al. (2020). Exosomes could offer new options to combat the long-term complications inflicted by gestational diabetes mellitus. Cells 9, 675. doi:10.3390/cells9030675
Ford, A. A., Rogerson, L., Cody, J. D., Aluko, P., and Ogah, J. A. (2017). Mid-urethral sling operations for stress urinary incontinence in women. Cochrane database Syst. Rev. 7, Cd006375. doi:10.1002/14651858.CD006375.pub4
Furuta, A., Kita, M., Suzuki, Y., Egawa, S., Chancellor, M. B., de Groat, W. C., et al. (2008). Association of overactive bladder and stress urinary incontinence in rats with pudendal nerve ligation injury. Am. J. physiology. Regul. Integr. Comp. physiology 294, R1510–R1516. doi:10.1152/ajpregu.00838.2007
Gasquet, I., Tcherny-Lessenot, S., Gaudebout, P., Bosio Le Goux, B., Klein, P., and Haab, F. (2006). Influence of the severity of stress urinary incontinence on quality of life, health care seeking, and treatment: a national cross-sectional survey. Eur. Urol. 50, 818–825. doi:10.1016/j.eururo.2006.03.052
Ghoniem, G., Corcos, J., Comiter, C., Bernhard, P., Westney, O. L., and Herschorn, S. (2009). Cross-linked polydimethylsiloxane injection for female stress urinary incontinence: results of a multicenter, randomized, controlled, single-blind study. J. urology 181, 204–210. doi:10.1016/j.juro.2008.09.032
Giarenis, I., and Cardozo, L. (2014). Managing urinary incontinence: what works? Climacteric J. Int. Menopause Soc. 17 (Suppl. 2), 26–33. doi:10.3109/13697137.2014.947256
Gräs, S., Klarskov, N., and Lose, G. (2014). Intraurethral injection of autologous minced skeletal muscle: a simple surgical treatment for stress urinary incontinence. J. urology 192, 850–855. doi:10.1016/j.juro.2014.04.005
Griffin, M. A., Janosek-Albright, K. J., Diaz-Insua, M., Elshatanoufy, S., and Atiemo, H. O. (2016). Quality of life outcomes in peri-urethral calcium hydroxylapatite injection. Int. urogynecology J. 27, 1879–1887. doi:10.1007/s00192-016-3053-1
Hakim, L., De Ridder, D., and Van der Aa, F. (2015). Slings for urinary incontinence and the application of cell-based therapy. Adv. drug Deliv. Rev. 82-83, 22–30. doi:10.1016/j.addr.2014.11.006
Hamilton, K. J., Hewitt, S. C., Arao, Y., and Korach, K. S. (2017). Estrogen hormone biology. Curr. Top. Dev. Biol. 125, 109–146. doi:10.1016/bs.ctdb.2016.12.005
Hampel, C., Artibani, W., Espuña Pons, M., Haab, F., Jackson, S., Romero, J., et al. (2004). Understanding the burden of stress urinary incontinence in Europe: a qualitative review of the literature. Eur. Urol. 46, 15–27. doi:10.1016/j.eururo.2004.02.003
Harris, R. L., Cundiff, G. W., Coates, K. W., Addison, W. A., and Bump, R. C. (1998). Urethral prolapse after collagen injection. Am. J. obstetrics Gynecol. 178, 614–615. doi:10.1016/s0002-9378(98)70450-8
Hilton, P. (2009). Urethrovaginal fistula associated with 'sterile abscess' formation following periurethral injection of dextranomer/hyaluronic acid co-polymer (Zuidex) for the treatment of stress urinary incontinence--a case report. BJOG Int. J. obstetrics Gynaecol. 116, 1527–1530. doi:10.1111/j.1471-0528.2009.02306.x
Hoe, V., Yao, H. H., Gough, K., and O'Connell, H. E. (2022). Long-term outcomes of polyacrylamide hydrogel treatment in women with stress urinary incontinence. BJU Int. 130 (Suppl. 1), 22–24. doi:10.1111/bju.15717
Hong, S. H., Piao, S., Kim, I. G., Lee, J. Y., Cho, H. J., Kim, S. W., et al. (2013). Comparison of three types of stress urinary incontinence rat models: electrocauterization, pudendal denervation, and vaginal distension. Urology 81 (465), 465.e1–465.e6. doi:10.1016/j.urology.2012.10.029
Hu, J. S., and Pierre, E. F. (2019). Urinary incontinence in women: evaluation and management. Am. Fam. Physician 100, 339–348.
Hu, M., Hong, L., Liu, C., Hong, S., He, S., Zhou, M., et al. (2019). Electrical stimulation enhances neuronal cell activity mediated by Schwann cell derived exosomes. Sci. Rep. 9, 4206. doi:10.1038/s41598-019-41007-5
Huang, Y., Daneshgari, F., and Liu, G. (2014). Successful induction of stress urinary incontinence in mice by vaginal distension does not depend on the estrous cycle. Urology 83, 958.e1–958.e6. doi:10.1016/j.urology.2013.12.028
Hurtado, E., McCrery, R., and Appell, R. (2007). The safety and efficacy of ethylene vinyl alcohol copolymer as an intra-urethral bulking agent in women with intrinsic urethral deficiency. Int. urogynecology J. pelvic floor Dysfunct. 18, 869–873. doi:10.1007/s00192-006-0251-2
Hurtado, E. A., McCrery, R. J., and Appell, R. A. (2008). Complications of ethylene vinyl alcohol copolymer as an intraurethral bulking agent in men with stress urinary incontinence. Urology 71, 662–665. doi:10.1016/j.urology.2007.10.016
Inoue, K. I., Kishimoto, S., Kaga, K., Fuse, M., Furuta, A., and Yamanishi, T. (2018). Autologous and heterotopic transplantation of adipose stromal vascular fraction ameliorates stress urinary incontinence in rats with simulated childbirth trauma. Regen. Ther. 8, 9–14. doi:10.1016/j.reth.2017.11.003
Islam, A., Chapin, K., Younesi, M., and Akkus, O. (2015). Computer aided biomanufacturing of mechanically robust pure collagen meshes with controlled macroporosity. Biofabrication 7, 035005. doi:10.1088/1758-5090/7/3/035005
Jiang, H. H., and Damaser, M. S. (2011). Animal models of stress urinary incontinence. Handb. Exp. Pharmacol., 45–67. doi:10.1007/978-3-642-16499-6_3
Jin, M., Chen, Y., Zhou, Y., Mei, Y., Liu, W., Pan, C., et al. (2016a). Transplantation of bone marrow-derived mesenchymal stem cells expressing elastin alleviates pelvic floor dysfunction. Stem cell Res. and Ther. 7, 51. doi:10.1186/s13287-016-0308-1
Jin, M., Wu, Y., Wang, J., Ye, W., Wang, L., Yin, P., et al. (2016b). MicroRNA-29 facilitates transplantation of bone marrow-derived mesenchymal stem cells to alleviate pelvic floor dysfunction by repressing elastin. Stem cell Res. and Ther. 7, 167. doi:10.1186/s13287-016-0428-7
Jost, W., and Marsalek, P. (2004). Duloxetine: mechanism of action at the lower urinary tract and Onuf's nucleus. Clin. Aut. Res. official J. Clin. Aut. Res. Soc. 14, 220–227. doi:10.1007/s10286-004-0197-8
Kadekawa, K., Kawamorita, N., Shimizu, T., Kurobe, M., Turnbull, P. S., Chandra, S., et al. (2020). Effects of a selective androgen receptor modulator (SARM), GSK2849466A, on stress urinary incontinence and bladder activity in rats with ovariectomy-induced oestrogen deficiency. BJU Int. 125, 911–919. doi:10.1111/bju.15022
Kane, A. R., and Nager, C. W. (2008). Midurethral slings for stress urinary incontinence. Clin. obstetrics Gynecol. 51, 124–135. doi:10.1097/GRF.0b013e318161e687
Kassolik, K., Kurpas, D., Andrzejewski, W., Wilk, I., and Swiatek, M. (2013). The effectiveness of massage in stress urinary incontinence-case study. Rehabilitation Nurs. official J. Assoc. Rehabilitation Nurses 38, 306–314. doi:10.1002/rnj.91
Kato, H., Igawa, Y., Khaleque, M. A., and Nishizawa, O. (1999). Bladder dysfunction after proximal urethrolysis in female dogs. Int. J. urology official J. Jpn. Urological Assoc. 6, 33–37. doi:10.1046/j.1442-2042.1999.06126.x
Kaufman, M., Lockhart, J. L., Silverstein, M. J., and Politano, V. A. (1984). Transurethral polytetrafluoroethylene injection for post-prostatectomy urinary incontinence. J. urology 132, 463–464. doi:10.1016/s0022-5347(17)49692-3
Kawamorita, N., Kaiho, Y., Nakagawa, H., Miyazato, M., Matsushita, M., and Arai, Y. (2010). Novel rat model of stress urinary incontinence with a retroflexed bladder. Int. urogynecology J. 21, 595–601. doi:10.1007/s00192-009-1070-z
Kefer, J. C., Liu, G., and Daneshgari, F. (2009). Pubo-urethral ligament injury causes long-term stress urinary incontinence in female rats: an animal model of the integral theory. J. urology 181, 397–400. doi:10.1016/j.juro.2008.09.002
Kelleher, C., Hakimi, Z., Zur, R., Siddiqui, E., Maman, K., Aballéa, S., et al. (2018). Efficacy and tolerability of mirabegron compared with antimuscarinic monotherapy or combination therapies for overactive bladder: a systematic review and network meta-analysis. Eur. Urol. 74, 324–333. doi:10.1016/j.eururo.2018.03.020
Kelp, A., Albrecht, A., Amend, B., Klünder, M., Rapp, P., Sawodny, O., et al. (2017). Establishing and monitoring of urethral sphincter deficiency in a large animal model. World J. urology 35, 1977–1986. doi:10.1007/s00345-017-2088-3
Kennelly, M., Cruz, F., Herschorn, S., Abrams, P., Onem, K., Solomonov, V. K., et al. (2022). Efficacy and safety of AbobotulinumtoxinA in patients with neurogenic detrusor overactivity incontinence performing regular clean intermittent catheterization: pooled results from two phase 3 randomized studies (CONTENT1 and CONTENT2). Eur. Urol. 82, 223–232. doi:10.1016/j.eururo.2022.03.010
Kerdraon, J., and Denys, P. (2009). Conservative treatment of female stress urinary incontinence. J. de Gynecol. obstetrique Biol. de reproduction 38, S174–S181. doi:10.1016/s0368-2315(09)73577-x
Khalifa, A. O., Isali, I., Celik, H., Mastran, M., McClellan, P., Gillespie, C., et al. (2022). A preliminary evaluation of in vivo response to a filament-wound macroporous collagen midurethral sling in an ovine model. J. Biomed. Mater. Res. Part B, Appl. biomaterials 110, 2676–2685. doi:10.1002/jbm.b.35120
Khorramirouz, R., Mozafarpour, S., Kameli, S. M., Ladi Seyedian, S. S., Oveisi, N., Rahimi, Z., et al. (2016). A novel method of urinary sphincter deficiency: serial histopathology evaluation in a rat model of urinary incontinence. Anat. Rec. Hob. N.J. 299, 173–180. doi:10.1002/ar.23291
Kibschull, M., Nguyen, T. T. N., Chow, T., Alarab, M., Lye, S. J., Rogers, I., et al. (2023). Differentiation of patient-specific void urine-derived human induced pluripotent stem cells to fibroblasts and skeletal muscle myocytes. Sci. Rep. 13, 4746. doi:10.1038/s41598-023-31780-9
Kiilholma, P., and Mäkinen, J. (1991). Disappointing effect of endoscopic Teflon injection for female stress incontinence. Eur. Urol. 20, 197–199. doi:10.1159/000471699
Kiilholma, P. J., Chancellor, M. B., Makinen, J., Hirsch, I. H., and Klemi, P. J. (1993). Complications of Teflon injection for stress urinary incontinence. Neurourol. urodynamics 12, 131–137. doi:10.1002/nau.1930120206
Kim, G. S., Kim, E. G., Shin, K. Y., Choo, H. J., and Kim, M. J. (2015). Combined pelvic muscle exercise and yoga program for urinary incontinence in middle-aged women. Jpn. J. Nurs. Sci. JJNS 12, 330–339. doi:10.1111/jjns.12072
Kirchin, V., Page, T., Keegan, P. E., Atiemo, K. O., Cody, J. D., McClinton, S., et al. (2017). Urethral injection therapy for urinary incontinence in women. Cochrane database Syst. Rev. 7, Cd003881. doi:10.1002/14651858.CD003881.pub4
Kitta, T., Yoshikawa, S., Kawamorita, N., de Groat, W. C., Nonomura, K., and Yoshimura, N. (2016). The effect of ovariectomy on urethral continence mechanisms during sneeze reflex in middle-aged versus young adult rats. Neurourol. urodynamics 35, 122–127. doi:10.1002/nau.22690
Knoll, J., Amend, B., Harland, N., Isser, S., Bézière, N., Kraushaar, U., et al. (2024). Cell therapy by mesenchymal stromal cells versus myoblasts in a pig model of urinary incontinence. Tissue Eng. Part A 30, 14–30. doi:10.1089/ten.TEA.2023.0103
Knudson, M. J., Cooper, C. S., Block, C. A., Hawtrey, C. E., and Austin, J. C. (2006). Calcification of glutaraldehyde cross-linked collagen in bladder neck injections in children with incontinence: a long-term complication. J. urology 176, 1143–1146. doi:10.1016/j.juro.2006.04.059
Kuhn, A., Stadlmayr, W., Sohail, A., and Monga, A. (2008). Long-term results and patients’ satisfaction after transurethral ethylene vinyl alcohol (Tegress®) injections: a two-centre study. Int. urogynecology J. pelvic floor Dysfunct. 19, 503–507. doi:10.1007/s00192-007-0479-5
Lai, K., Zhang, J., Wang, G., Luo, X., Liu, M., Zhang, X., et al. (2017). A biomimetic mesh for treating female stress urinary incontinence. Biofabrication 9, 015008. doi:10.1088/1758-5090/aa515e
Lee, J. Y., Cannon, T. W., Pruchnic, R., Fraser, M. O., Huard, J., and Chancellor, M. B. (2003). The effects of periurethral muscle-derived stem cell injection on leak point pressure in a rat model of stress urinary incontinence. Int. urogynecology J. pelvic floor Dysfunct. 14, 31–37. ; discussion 37. doi:10.1007/s00192-002-1004-5
Lee, J. Y., Paik, S. Y., Yuk, S. H., Ghil, S. H., and Lee, S. S. (2004). Long term effects of muscle-derived stem cells on leak point pressure and closing pressure in rats with transected pudendal nerves. Mol. cells 18, 309–313. doi:10.1016/s1016-8478(23)13117-7
Lee, P. J., Jiang, Y. H., and Kuo, H. C. (2022). Therapeutic efficacy and mid-term durability of urethral sphincter platelet-rich plasma injections to treat postprostatectomy stress urinary incontinence. Biomedicines 10, 2235. doi:10.3390/biomedicines10092235
Lee, Y. C., Lin, G., Wang, G., Reed-Maldonado, A., Lu, Z., Wang, L., et al. (2017). Impaired contractility of the circular striated urethral sphincter muscle may contribute to stress urinary incontinence in female zucker fatty rats. Neurourol. urodynamics 36, 1503–1510. doi:10.1002/nau.23165
Li, G., Yu, C., Yu, P., Peng, Q., Wang, Q., Ren, S., et al. (2022). Periurethral and intravenous injections of adipose-derived stem cells to promote local tissue recovery in a rat model of stress urinary incontinence. Urology 167, 82–89. doi:10.1016/j.urology.2022.05.018
Li, M., Li, G., Lei, H., Guan, R., Yang, B., Gao, Z., et al. (2016). Therapeutic potential of adipose-derived stem cell-based microtissues in a rat model of stress urinary incontinence. Urology 97, 277.e1–277.e7. doi:10.1016/j.urology.2016.08.009
Lightner, D. J., Fox, J., and Klingele, C. (2010). Cystoscopic injections of dextranomer hyaluronic acid into proximal urethra for urethral incompetence: efficacy and adverse outcomes. Urology 75, 1310–1314. doi:10.1016/j.urology.2009.12.061
Lin, A. S., Carrier, S., Morgan, D. M., and Lue, T. F. (1998). Effect of simulated birth trauma on the urinary continence mechanism in the rat. Urology 52, 143–151. doi:10.1016/s0090-4295(98)00136-8
Liu, J., Luo, X., Yao, M., Zhao, L., Zhou, X., Liu, Y., et al. (2022). Use of statistical methods among acupuncture randomized controlled trials was far from satisfactory. J. Clin. Epidemiol. 152, 1–12. doi:10.1016/j.jclinepi.2022.09.008
Liu, X., Wang, S., Wu, S., Hao, Q., Li, Y., Guo, Z., et al. (2018). Exosomes secreted by adipose-derived mesenchymal stem cells regulate type I collagen metabolism in fibroblasts from women with stress urinary incontinence. Stem cell Res. and Ther. 9, 159. doi:10.1186/s13287-018-0899-9
Liu, Z., Liu, Y., Xu, H., He, L., Chen, Y., Fu, L., et al. (2017). Effect of electroacupuncture on urinary leakage among women with stress urinary incontinence: a randomized clinical trial. Jama 317, 2493–2501. doi:10.1001/jama.2017.7220
Lose, G., Mouritsen, L., and Nielsen, J. B. (2006). A new bulking agent (polyacrylamide hydrogel) for treating stress urinary incontinence in women. BJU Int. 98, 100–104. doi:10.1111/j.1464-410X.2006.06205.x
Lüttmann, K., Merle, R., and Nickel, R. (2019). Retrospective analysis after endoscopic urethral injections of glutaraldehyde-cross-linked-collagen or dextranomer/hyaluronic acid copolymer in bitches with urinary incontinence. J. small animal Pract. 60, 96–101. doi:10.1111/jsap.12949
Madjar, S., Covington-Nichols, C., and Secrest, C. L. (2003). New periurethral bulking agent for stress urinary incontinence: modified technique and early results. J. urology 170, 2327–2329. doi:10.1097/01.ju.0000090968.85279.84
Mariappan, P., Alhasso, A., Ballantyne, Z., Grant, A., and N'Dow, J. (2007). Duloxetine, a serotonin and noradrenaline reuptake inhibitor (SNRI) for the treatment of stress urinary incontinence: a systematic review. Eur. Urol. 51, 67–74. doi:10.1016/j.eururo.2006.08.041
Martínez-Piñeiro, L., Jiménez de León, J., Cózar, J. M., Navarro, J., Cuervo Blanco, E., and Martínez-Piñeiro, J. A. (1989). Obstructive uropathy secondary to the periurethral injection of teflon paste. Arch. espanoles Urol. 42, 571–573.
Mayer, R., Lightfoot, M., and Jung, I. (2001). Preliminary evaluation of calcium hydroxylapatite as a transurethral bulking agent for stress urinary incontinence. Urology 57, 434–438. doi:10.1016/s0090-4295(00)01098-0
Mayer, R. D., Dmochowski, R., Appell, R., Sand, P., Klimberg, I., Jacoby, K., et al. (2007). Multicenter prospective randomized 52-week trial of calcium hydroxylapatite versus bovine dermal collagen for treatment of stress urinary incontinence. Urology 69, 876–880. doi:10.1016/j.urology.2007.01.050
McKinney, C. D., Gaffey, M. J., and Gillenwater, J. Y. (1995). Bladder outlet obstruction after multiple periurethral polytetrafluoroethylene injections. J. urology 153, 149–151. doi:10.1097/00005392-199501000-00053
McLean, R., Kipling, M., Musgrave, E., and Mercer-Jones, M. (2018). Short- and long-term clinical and patient-reported outcomes following laparoscopic ventral mesh rectopexy using biological mesh for pelvic organ prolapse: a prospective cohort study of 224 consecutive patients. Colorectal Dis. official J. Assoc. Coloproctology G. B. Irel. 20, 424–436. doi:10.1111/codi.13996
Mori da Cunha, M., van der Veer, B. K., Giacomazzi, G., Mackova, K., Cattani, L., Koh, K. P., et al. (2023). VEGF overexpressed mesoangioblasts enhance urethral and vaginal recovery following simulated vaginal birth in rats. Sci. Rep. 13, 8622. doi:10.1038/s41598-023-35809-x
Mostafaei, H., Salehi-Pourmehr, H., Jilch, S., Carlin, G. L., Mori, K., Quhal, F., et al. (2022). Choosing the most efficacious and safe oral treatment for idiopathic overactive bladder: a systematic review and network meta-analysis. Eur. Urol. focus 8, 1072–1089. doi:10.1016/j.euf.2021.08.011
Mukkamala, A., Latini, J. M., and Cameron, A. P. (2013). Urethrocutaneous fistula after use of Tegress bulking agent: case report and review of the literature. Can. Urological Assoc. J. = J. de l'Association des urologues du Can. 7, E833–E836. doi:10.5489/cuaj.481
Mussano, F., Genova, T., Munaron, L., Petrillo, S., Erovigni, F., and Carossa, S. (2016). Cytokine, chemokine, and growth factor profile of platelet-rich plasma. Platelets 27, 467–471. doi:10.3109/09537104.2016.1143922
Ni, J., Li, H., Zhou, Y., Gu, B., Xu, Y., Fu, Q., et al. (2018). Therapeutic potential of human adipose-derived stem cell exosomes in stress urinary incontinence - an in vitro and in vivo study. Cell. physiology Biochem. Int. J. Exp. Cell. physiology, Biochem. Pharmacol. 48, 1710–1722. doi:10.1159/000492298
Nightingale, G. (2020). Management of urinary incontinence. Post. Reprod. health 26, 63–70. doi:10.1177/2053369120927112
Nygaard, I., and Norton, P. (2022). Surgery for stress urinary incontinence in women - improvement but not a cure. N. Engl. J. Med. 386, 1280–1281. doi:10.1056/NEJMe2201166
Ohlstein, E. H., von Keitz, A., and Michel, M. C. (2012). A multicenter, double-blind, randomized, placebo-controlled trial of the β3-adrenoceptor agonist solabegron for overactive bladder. Eur. Urol. 62, 834–840. doi:10.1016/j.eururo.2012.05.053
Ostrzenski, A. (2020). The new etiology and surgical therapy of stress urinary incontinence in women. Eur. J. obstetrics, Gynecol. reproductive Biol. 245, 26–34. doi:10.1016/j.ejogrb.2019.11.023
Ouchi, M., Kitta, T., Kanno, Y., Higuchi, M., Togo, M., Takahashi, Y., et al. (2019). Dopaminergic urethral closure mechanisms in a rat model of Parkinson's disease. Neurourol. urodynamics 38, 1203–1211. doi:10.1002/nau.23989
Pang, H., Yin, Y., Xue, J., Chen, X., Pang, J., Zhang, J., et al. (2023). Associations between visceral adipose index and stress urinary incontinence among US adult women: a cross-sectional study. World J. urology 41, 3671–3678. doi:10.1007/s00345-023-04667-7
Pannek, J., Brands, F. H., and Senge, T. (2001). Particle migration after transurethral injection of carbon coated beads for stress urinary incontinence. J. urology 166, 1350–1353. doi:10.1097/00005392-200110000-00028
Park, H. W., Jeon, H. J., and Chang, M. S. (2016). Vascular endothelial growth factor enhances axonal outgrowth in organotypic spinal cord slices via vascular endothelial growth factor receptor 1 and 2. Tissue Eng. Regen. Med. 13, 601–609. doi:10.1007/s13770-016-0051-9
Park, K. M., Son, J. Y., Choi, J. H., Kim, I. G., Lee, Y., Lee, J. Y., et al. (2014). Macro/Nano-gel composite as an injectable and bioactive bulking material for the treatment of urinary incontinence. Biomacromolecules 15, 1979–1984. doi:10.1021/bm401787u
Pearlman, A., and Kreder, K. (2020). Evaluation and treatment of urinary incontinence in the aging male. Postgrad. Med. 132, 9–17. doi:10.1080/00325481.2020.1831790
Phull, H. S., Pan, H. Q., Butler, R. S., Hansel, D. E., and Damaser, M. S. (2011). Vulnerability of continence structures to injury by simulated childbirth. Am. J. physiology. Ren. physiology 301, F641–F649. doi:10.1152/ajprenal.00120.2011
Pinar, U., Grossetete, F., Barnouin, L., and Chartier-Kastler, E. (2022). Exploratory safety study of an umbilical cord derived urethral sling in bilateral pudendal nerves injury-induced urinary incontinence in female rats. Neurourol. urodynamics 41, 777–786. doi:10.1002/nau.24895
Quinn, S. D., and Domoney, C. (2009). The effects of hormones on urinary incontinence in postmenopausal women. Climacteric J. Int. Menopause Soc. 12, 106–113. doi:10.1080/13697130802630083
Rapp, D. E., Kobashi, K. C., and Govier, F. E. (2009). Placement of a transvaginal bone-anchored sling A new minimally invasive technique is useful in stress urinary incontinence. Am. J. Obstet. Gynecol. 200, 345.e341–343. doi:10.1016/j.ajog.2008.10.007
Rehman, H., Bezerra, C. A., Bruschini, H., Cody, J. D., and Aluko, P. (2017). Traditional suburethral sling operations for urinary incontinence in women. Cochrane database Syst. Rev. 7, Cd001754. doi:10.1002/14651858.CD001754.pub4
Reisenauer, C., Kirschniak, A., Drews, U., and Wallwiener, D. (2006). Transobturator vaginal tape inside-out. A minimally invasive treatment of stress urinary incontinence: surgical procedure and anatomical conditions. Eur. J. obstetrics, Gynecol. reproductive Biol. 127, 123–129. doi:10.1016/j.ejogrb.2005.11.029
Rolland, T. J., Peterson, T. E., Singh, R. D., Rizzo, S. A., Boroumand, S., Shi, A., et al. (2022). Exosome biopotentiated hydrogel restores damaged skeletal muscle in a porcine model of stress urinary incontinence. NPJ Regen. Med. 7, 58. doi:10.1038/s41536-022-00240-9
Sadeghi, Z., Kenyon, J. D., Richardson, B., Khalifa, A. O., Cartwright, M., Conroy, B., et al. (2020). Transcriptomic analysis of human mesenchymal stem cell therapy in incontinent rat injured urethra. Tissue Eng. Part A 26, 792–810. doi:10.1089/ten.tea.2020.0033
Sánchez, M., Anitua, E., Delgado, D., Sanchez, P., Prado, R., Orive, G., et al. (2017). Platelet-rich plasma, a source of autologous growth factors and biomimetic scaffold for peripheral nerve regeneration. Expert Opin. Biol. Ther. 17, 197–212. doi:10.1080/14712598.2017.1259409
Sangster, P., and Morley, R. (2010). Biomaterials in urinary incontinence and treatment of their complications. Indian J. urology IJU J. Urological Soc. India 26, 221–229. doi:10.4103/0970-1591.65394
Saporito, M. S., Zuvich, E., and DiCamillo, A. (2016). A mouse model of furosemide-induced overactive bladder. Curr. Protoc. Pharmacol. 74, 5.68.61–5. doi:10.1002/cpph.10
Serati, M., Braga, A., Scancarello, C., De Rosa, A., Frigerio, M., Baruch, Y., et al. (2023). Does the polydimethylsiloxane urethral injection (Macroplastique(®)) improve sexual function in women, in fertile age, affected by stress urinary incontinence? Med. Kaunas. Lith. 59, 580. doi:10.3390/medicina59030580
Serati, M., Soligo, M., Braga, A., Cantaluppi, S., Coluccia, A. C., Di Dedda, M. C., et al. (2019). Efficacy and safety of polydimethylsiloxane injection (Macroplastique®) for the treatment of female stress urinary incontinence: results of a series of 85 patients with ≥3 years of follow-up. BJU Int. 123, 353–359. doi:10.1111/bju.14550
Shang, X., Fu, Y., Jin, X., Wang, C., Wang, P., Guo, P., et al. (2023). Association of overweight, obesity and risk of urinary incontinence in middle-aged and older women: a meta epidemiology study. Front. Endocrinol. 14, 1220551. doi:10.3389/fendo.2023.1220551
Sheng, Y., Carpenter, J. S., Ashton-Miller, J. A., and Miller, J. M. (2022). Mechanisms of pelvic floor muscle training for managing urinary incontinence in women: a scoping review. BMC women's health 22, 161. doi:10.1186/s12905-022-01742-w
Shimizu-Motohashi, Y., and Asakura, A. (2014). Angiogenesis as a novel therapeutic strategy for Duchenne muscular dystrophy through decreased ischemia and increased satellite cells. Front. physiology 5, 50. doi:10.3389/fphys.2014.00050
Shortliffe, L. M., Freiha, F. S., Kessler, R., Stamey, T. A., and Constantinou, C. E. (1989). Treatment of urinary incontinence by the periurethral implantation of glutaraldehyde cross-linked collagen. J. urology 141, 538–541. doi:10.1016/s0022-5347(17)40885-8
Sims, L., Hay-Smith, J., and Dean, S. (2022). Pelvic floor exercises and female stress urinary incontinence. Br. J. general Pract. J. R. Coll. General Pract. 72, 185–187. doi:10.3399/bjgp22X719033
Sokol, E. R., Karram, M. M., and Dmochowski, R. (2014). Efficacy and safety of polyacrylamide hydrogel for the treatment of female stress incontinence: a randomized, prospective, multicenter North American study. J. urology 192, 843–849. doi:10.1016/j.juro.2014.03.109
Stothers, L., Goldenberg, S. L., and Leone, E. F. (1998). Complications of periurethral collagen injection for stress urinary incontinence. J. urology 159, 806–807. doi:10.1097/00005392-199803000-00049
Stricker, P., and Haylen, B. (1993). Injectable collagen for type 3 female stress incontinence: the first 50 Australian patients. Med. J. Aust. 158, 89–91. doi:10.5694/j.1326-5377.1993.tb137530.x
Sun, Y., Jin, K., Xie, L., Childs, J., Mao, X. O., Logvinova, A., et al. (2003). VEGF-induced neuroprotection, neurogenesis, and angiogenesis after focal cerebral ischemia. J. Clin. investigation 111, 1843–1851. doi:10.1172/jci17977
Sundaram, C. P., Reinberg, Y., and Aliabadi, H. A. (1997). Failure to obtain durable results with collagen implantation in children with urinary incontinence. J. urology 157, 2306–2307. doi:10.1016/s0022-5347(01)64770-0
Swinburn, P., Lloyd, A., Ali, S., Hashmi, N., Newal, D., and Najib, H. (2011). Preferences for antimuscarinic therapy for overactive bladder. BJU Int. 108, 868–873. doi:10.1111/j.1464-410X.2010.09882.x
Tasmim, S., Yousuf, Z., Rahman, F. S., Seelig, E., Clevenger, A. J., VandenHeuvel, S. N., et al. (2023). Liquid crystal elastomer based dynamic device for urethral support: potential treatment for stress urinary incontinence. Biomaterials 292, 121912. doi:10.1016/j.biomaterials.2022.121912
Tenfelde, S., and Janusek, L. W. (2014). Yoga: a biobehavioral approach to reduce symptom distress in women with urge urinary incontinence. J. Altern. complementary Med. (New York, N.Y.) 20, 737–742. doi:10.1089/acm.2013.0308
van Kerrebroeck, P., Ter Meulen, F., Larsson, G., Farrelly, E., Edwall, L., and Fianu-Jonasson, A. (2004). Treatment of stress urinary incontinence using a copolymer system: impact on quality of life. BJU Int. 94, 1040–1043. doi:10.1111/j.1464-410X.2004.05101.x
Vardar, E., Vythilingam, G., Pinnagoda, K., Engelhardt, E., Zambelli, P., Hubbell, J., et al. (2019). A bioactive injectable bulking material; a potential therapeutic approach for stress urinary incontinence. Biomaterials 206, 41–48. doi:10.1016/j.biomaterials.2019.03.030
Wang, L., Lin, G., Lee, Y., Reed-Maldonado, A. B., Sanford, M. T., Wang, G., et al. (2017). Transgenic animal model for studying the mechanism of obesity-associated stress urinary incontinence. BJU Int. 119, 317–324. doi:10.1111/bju.13661
Wang, Q., Que, Y. Z., Wan, X. Y., and Lin, C. Q. (2023). Prevalence, risk factors, and impact on life of female urinary incontinence: an epidemiological survey of 9584 women in a region of southeastern China. Risk Manag. Healthc. policy 16, 1477–1487. doi:10.2147/rmhp.S421488
Wang, Y., Duan, M., Rahman, M., Yang, M., Zhao, W., Zhou, S., et al. (2020b). Use of bioactive extracellular matrix fragments as a urethral bulking agent to treat stress urinary incontinence. Acta biomater. 117, 156–166. doi:10.1016/j.actbio.2020.09.049
Wang, Y., Zhishun, L., Peng, W., Zhao, J., and Liu, B. (2013). Acupuncture for stress urinary incontinence in adults. Cochrane database Syst. Rev. 2013, Cd009408. doi:10.1002/14651858.CD009408.pub2
Wang, Y., Zhou, S., Yang, R., Rahman, M., Sequeira, R. C., Cao, N., et al. (2020a). Magnetic targeting of super-paramagnetic iron oxide nanoparticle labeled myogenic-induced adipose-derived stem cells in a rat model of stress urinary incontinence. Nanomedicine Nanotechnol. Biol. Med. 30, 102281. doi:10.1016/j.nano.2020.102281
Williams, J. K., Badlani, G., Dean, A., Lankford, S., Poppante, K., Criswell, T., et al. (2016b). Local versus intravenous injections of skeletal muscle precursor cells in nonhuman primates with acute or chronic intrinsic urinary sphincter deficiency. Stem cell Res. and Ther. 7, 147. doi:10.1186/s13287-016-0411-3
Williams, J. K., Dean, A., Badra, S., Lankford, S., Poppante, K., Badlani, G., et al. (2016a). Cell versus chemokine therapy in a nonhuman primate model of chronic intrinsic urinary sphincter deficiency. J. urology 196, 1809–1815. doi:10.1016/j.juro.2016.05.106
Williams, J. K., Dean, A., Lankford, S., Criswell, T., Badlani, G., and Andersson, K. E. (2017). Determinates of muscle precursor cell therapy efficacy in a nonhuman primate model of intrinsic urinary sphincter deficiency. Stem cell Res. and Ther. 8 (1), 1. doi:10.1186/s13287-016-0461-6
Woo, L. L., Hijaz, A., Pan, H. Q., Kuang, M., Rackley, R. R., and Damaser, M. S. (2009). Simulated childbirth injuries in an inbred rat strain. Neurourol. urodynamics 28, 356–361. doi:10.1002/nau.20644
Wu, R., Huang, C., Wu, Q., Jia, X., Liu, M., Xue, Z., et al. (2019). Exosomes secreted by urine-derived stem cells improve stress urinary incontinence by promoting repair of pubococcygeus muscle injury in rats. Stem cell Res. and Ther. 10, 80. doi:10.1186/s13287-019-1182-4
Yadav, P., Farhat, W. A., Hijaz, A., Seo, J., Hui, C. C., Tuba-Ang, K., et al. (2023). A study on etiology of incontinence in double knockout mouse model. J. Pediatr. urology 19, 23.e1–23.e9. doi:10.1016/j.jpurol.2022.10.002
Yamada, S., Ito, Y., Nishijima, S., Kadekawa, K., and Sugaya, K. (2018). Basic and clinical aspects of antimuscarinic agents used to treat overactive bladder. Pharmacol. and Ther. 189, 130–148. doi:10.1016/j.pharmthera.2018.04.010
Yanai-Inamura, H., Ohashi, R., Ishigami, T., Hirata, T., Kumakura, F., Kono, G., et al. (2019). Urethral function and histopathology in aged female rats as a stress urinary incontinence model. Low. Urin. Tract. symptoms 11, O186–o192. doi:10.1111/luts.12209
Yang, L., Xie, F., Li, Y., Lu, Y., Li, B., Hong, S., et al. (2023). Chitin-based hydrogel loaded with bFGF and SDF-1 for inducing endogenous mesenchymal stem cells homing to improve stress urinary incontinence. Carbohydr. Polym. 319, 121144. doi:10.1016/j.carbpol.2023.121144
Yang, X., Qin, C., Li, M., and Shi, L. (2022b). How about the RCTs' quality of acupuncture treatment for female urinary incontinence in recent 20 years? A report quality assessment. World J. urology 41, 197–204. doi:10.1007/s00345-022-04213-x
Yang, X., Wang, X., Gao, Z., Li, L., Lin, H., Wang, H., et al. (2022a). The anatomical pathogenesis of stress urinary incontinence in women. Med. Kaunas. Lith. 59, 5. doi:10.3390/medicina59010005
Yoshida, J., Aikawa, K., Yoshimura, Y., Shishido, K., Yanagida, T., and Yamaguchi, O. (2007). The effects of ovariectomy and estrogen replacement on acetylcholine release from nerve fibres and passive stretch-induced acetylcholine release in female rat bladder. Neurourol. urodynamics 26, 1050–1055. doi:10.1002/nau.20438
Zhou, M., Hu, M., He, S., Li, B., Liu, C., Min, J., et al. (2018). Effects of RSC96 Schwann cell-derived exosomes on proliferation, senescence, and apoptosis of dorsal root ganglion cells in vitro. Med. Sci. Monit. Int. Med. J. Exp. Clin. Res. 24, 7841–7849. doi:10.12659/msm.909509
Zhou, M., Li, B., Liu, C., Hu, M., Tang, J., Min, J., et al. (2021). M2 Macrophage-derived exosomal miR-501 contributes to pubococcygeal muscle regeneration. Int. Immunopharmacol. 101, 108223. doi:10.1016/j.intimp.2021.108223
Zhu, F., Chen, M., Xiao, Y., Huang, X., Chen, L., and Hong, L. (2024). Synergistic interaction between hyperlipidemia and obesity as a risk factor for stress urinary incontinence in Americans. Sci. Rep. 14, 7312. doi:10.1038/s41598-024-56744-5
Zordani, A., Pisciotta, A., Bertoni, L., Bertani, G., Vallarola, A., Giuliani, D., et al. (2019). Regenerative potential of human dental pulp stem cells in the treatment of stress urinary incontinence: in vitro and in vivo study. Cell Prolif. 52, e12675. doi:10.1111/cpr.12675
Glossary
Keywords: stress urinary incontinence, biomaterials, urethral bulking agents, tissueengineered repair materials, animal models
Citation: Tan X, Li G, Li C, Kong C, Li H and Wu S (2024) Animal models, treatment options, and biomaterials for female stress urinary incontinence. Front. Bioeng. Biotechnol. 12:1414323. doi: 10.3389/fbioe.2024.1414323
Received: 08 April 2024; Accepted: 20 August 2024;
Published: 29 August 2024.
Edited by:
Jianxun Ding, Chinese Academy of Sciences (CAS), ChinaReviewed by:
Nailong Cao, Shanghai Jiao Tong University, ChinaSita Somara, RTI International, United States
Copyright © 2024 Tan, Li, Li, Kong, Li and Wu. This is an open-access article distributed under the terms of the Creative Commons Attribution License (CC BY). The use, distribution or reproduction in other forums is permitted, provided the original author(s) and the copyright owner(s) are credited and that the original publication in this journal is cited, in accordance with accepted academic practice. No use, distribution or reproduction is permitted which does not comply with these terms.
*Correspondence: Huizhen Li, NDQ1ODgzNThAcXEuY29t; Song Wu, ZG9jdG9yX3d1c29uZ0AxMjYuY29t