- 1Department of Chemical and Petroleum Engineering, Khalifa University of Science and Technology (KUST), Abu Dhabi, United Arab Emirates
- 2Food Technology Research Institute (FTRI), Agricultural Research Center (ARC), Giza, Egypt
- 3Chemistry Department, Royal College of Surgeons in Ireland (RCSI), Dublin, Ireland
- 4Instituto de Ciencias Físicas, National Autonomous University of Mexico (UNAM), México City, Mexico
Editorial on the Research Topic
Bioimaging applications in biosensors and biomolecular electronics
This editorial summarizes the contributions to the Frontiers Research Topic “Bioimaging Applications in Biosensors and Biomolecular Electronics,” established under the Biosensors and Biomolecular Electronics and appearing under the Frontiers in Bioengineering and Biotechnology journals. After more than a decade of extensive investigation of bio-fabricated materials, they have evolved into one of our most valuable and intriguing nanomaterials. The current state of scientific studies on bionanomaterials, with a shift from standard procedures to multifunctional hybrids, is a testament to the field’s continuous advancement, published annually. These materials, with their potential applications in the environment, energy, and health, are a testament to the versatility of biomaterials. The interest in biomaterials is driven by various factors, including their ease and affordability to synthesize using eco-friendly or sustainable procedures, readily accessible surface functionalization, and their capacity to form complexes with other species. These unique physical properties, improved performance, and excellent stability make bionanomaterials stand out (Kaur et al., 2023). The evolution in developing and understanding biosensing and biomolecular systems with sustainable materials procedures has increased the scientific community’s interest in implementing such systems for all analytes (Dhall et al., 2024; Bhatia et al., 2024). However, the need for advanced biosensing technology is urgent, and further research and development in this area are crucial.
By 2023, the global biosensors market was projected to reach a staggering USD 30.1 billion, with a promising compound annual growth rate (CAGR) of 7.7%–9.6% from 2024 to 2034 (Figure 1). This rapid market growth, fueled by innovative and next-generation biosensor technology, presents a thrilling landscape for the future. The demand for point-of-care testing propels the development of tiny electrochemical and microfluidic immunosensors. Because advanced biosensors can give precise, real-time measurements of biological and chemical substances, they have a wide range of applications in many different sectors. For instance, medical diagnostics delivered disease detection kits for glucose sensors for diabetes, tumor markers for cancer, and pathogen detection for contagious diseases. Also, wearable health devices monitor heart rate, sweat composition, oxygen saturation, and body temperature. Increasing investments in wearable medical device R&D for female fertility (Ankit, 2025; Global Statistics Report – 2032, 2023) further underscore this market growth potential for researchers and industry professionals in bionanomaterials. Moreover, many startups and established companies contribute to developing advanced wearables for chronic disease diagnosis and real-time monitoring. Their innovative technologies make insulin-dependent diabetic testing devices easier, more portable, and more convenient, increasing acceptance and improving patients’ quality of life (Ankit, 2025).
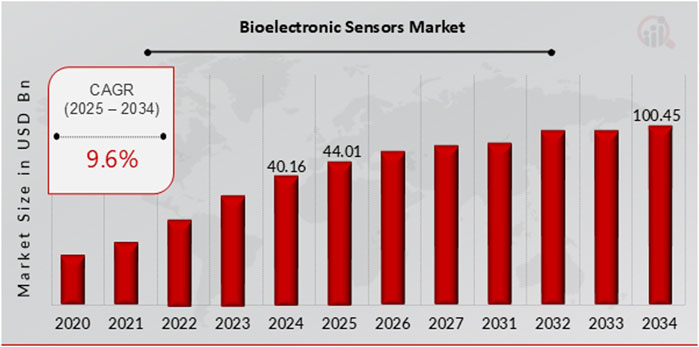
Figure 1. Secondary research, primary research, MRFR database and analyst review (Dhall et al., 2024).
So, there is vast potential for fabricating highly efficient biomaterials with broad spectral emissions, improved yield efficiencies, and easy-to-use, reusable probes. Beyond the current understanding of the formation of biomaterials, there is a pressing need to delve deeper into the source behind the atomic and molecular-level engineering-based selectivity of a specific analyte. This ongoing scientific exploration is crucial for unlocking the full potential of bionanomaterials (Sharma et al., 2025).
Opportunities exist to enhance the design of biomaterials for future applications at the field level. This involves addressing issues such as collateral contamination from biomaterial probes and improving efficiency in reproducibility, recovery, purification, and separation from the reaction solution. However, the true scientific breakthrough lies in understanding the formation and interaction mechanisms, a goal that can be achieved through the design and execution of well-organized experimental designs. The selectivity of the developed sensor for a particular target is always challenging, so researchers must explore novel biosensor architectures with extremely selective sensitivity, even in complex settings. Especially when dealing with complicated biological samples (such as blood or urine), biosensors may encounter difficulties because of interference with other components in the sample.
Also, to attain very low detection limits and advance the possibility for practical application in therapeutic domains, future research should also focus more on developing highly sensitive sensing devices on targeted cells and infections. Also, certain biosensors could take a long time to give precise readings, which could be a drawback for applications that must be completed quickly. Additionally, the restricted solubility of bionanomaterials in bodily fluids has hindered their use in biological sensing and imaging applications, presenting a significant challenge that requires further research and innovation to overcome. In addition to neural/cardiac recording, the creation of extremely biocompatible, electrochemically active, and flexible implantable tissue monitors and neural electrodes using sophisticated microfabrication and 3D printing methods for real-time monitoring of the cellular or tissue signals is crucial for the advancement of prosthetic devices, artificial intelligence, and robots in the future. However, producing and maintaining high-quality biosensors may be costly, which might prevent their widespread usage. Thus, developing biosensors at a limited and affordable price can demand the widespread production and utility of biosensors.
On the other hand, nanomaterials with their unique physical and chemical properties (e.g., high surface area, size-tunable optical properties, superparamagnetism, and ease of functionalization) have emerged in the field of bioimaging, offering unparalleled advantages in different aspects (sensitivity, resolution, and multifunctionality) from molecular level to whole-body imaging. For instance, nanoparticles with unique photophysical (e.g., photostability and tunable emission) properties and superparamagnetic nanoparticles have proven highly valuable in long-term tracking and multiplex bioimaging. Furthermore, by tailoring, surface modification, and functionalizing the nanoparticles with responsive coatings or biomolecules, researchers can develop targeted imaging probes that selectively accumulate at disease sites, improving diagnostic accuracy. Integrating nanomaterials of different functionalities (plasmonic, fluorescent, magnetic) into multimodal and/or multifunctional advanced materials further enhances their applications in bioimaging, treatment-targeted delivery, and controlled release (Gao et al., 2020; Encarnación et al., 2022; Han et al., 2019).
Author contributions
ST: Validation, Writing – original draft, Writing – review and editing, Visualization. AA: Writing – original draft, Writing – review and editing. NB: Validation, Writing – original draft, Writing – review and editing, Conceptualization, Methodology, Supervision.
Funding
The author(s) declare that financial support was received for the research and/or publication of this article. NR acknowledge the financial support given by DGAPA-UNAM (Dirección General de Asuntos del Personal Académico) under Project No. PAPIIT- IA202324.
Acknowledgments
We thank the authors of the papers published in this Research Topic for their valuable contributions and the referees for their rigorous review, which significantly improved the quality of this work. We also thank the Biosensors and Biomolecular Electronics section editorial board and the Frontiers specialists.
Conflict of interest
The authors declare that the research was conducted in the absence of any commercial or financial relationships that could be construed as a potential conflict of interest. The author(s) declared that they were an editorial board member of Frontiers, at the time of submission. This had no impact on the peer review process and the final decision.
Generative AI statement
The author(s) declare that no Generative AI was used in the creation of this manuscript.
Publisher’s note
All claims expressed in this article are solely those of the authors and do not necessarily represent those of their affiliated organizations, or those of the publisher, the editors and the reviewers. Any product that may be evaluated in this article, or claim that may be made by its manufacturer, is not guaranteed or endorsed by the publisher.
References
Bhatia, D., Paul, S., Acharjee, T., and Ramachairy, S. S. (2024). Biosensors and their widespread impact on human health. Sensors Int. 5, 100257. doi:10.1016/j.sintl.2023.100257
Ankit, G. (2025). Bioelectronic sensors market Report size, share and trends 2034. Report No.: MRFR/SEM/10826-HCR. Available online at: https://www.marketresearchfuture.com/reports/bioelectronic-sensors-market-12348 (Accessed January 28, 2025).
Dhall, S., Nigam, A., Harshavardhan, M., Mukherjee, A., and Srivastava, P. (2024). A comprehensive overview of methods involved in nanomaterial production and waste disposal from research labs and industries and existing regulatory guidelines for handling engineered nanomaterials. Environ. Chem. Ecotoxicol. 6, 269–282. doi:10.1016/j.enceco.2024.06.002
Encarnación, C., Jimenez de Aberasturi, D., and Liz-Marzán, L. M. (2022). Multifunctional plasmonic-magnetic nanoparticles for bioimaging and hyperthermia. Adv. Drug Deliv. Rev. 189, 114484. doi:10.1016/j.addr.2022.114484
Gao, Y., Wang, K., Zhang, J., Duan, X., Sun, Q., and Men, K. (2020). Multifunctional nanoparticle for cancer therapy, MedComm 4 (1), e187. doi:10.1002/mco2.187
Global Statistics Report – 2032 (2023). Biosensors market size analysis. Report No.: GMI747. Available online at: https://www.gminsights.com/industry-analysis/biosensors-market?gad_source=1&gclid=Cj0KCQiAy8K8BhCZARIsAKJ8sfR_cCnvhPcNtXcp4fp5wsias39vxSxJch9Lg-P1ZHpLvXxaRYMkdVIaAtrcEALw_wcB (Accessed January 28, 2025).
Han, X., Xu, K., Taratula, O., and Farsad, K. (2019). Applications of nanoparticles in biomedical imaging. Nanoscale 11, 799–819. doi:10.1039/c8nr07769j
Kaur, I., Batra, V., Kumar Reddy Bogireddy, N., Torres Landa, S. D., and Agarwal, V. (2023). Detection of organic pollutants, food additives and antibiotics using sustainable carbon dots. Food Chem. 406, 135029. doi:10.1016/J.FOODCHEM.2022.135029
Keywords: biosensors, electronics, biomaterials, bio-inspired, biomimetic, biorobotics, smart, stimuli-responsive
Citation: Tammina SK, Abdelmonem AM and Bogireddy NKR (2025) Editorial: Bioimaging applications in biosensors and biomolecular electronics. Front. Bioeng. Biotechnol. 13:1568124. doi: 10.3389/fbioe.2025.1568124
Received: 28 January 2025; Accepted: 14 April 2025;
Published: 25 April 2025.
Edited by:
Dan Du, Washington State University, United StatesReviewed by:
Gao-Chao Fan, Qingdao University of Science and Technology, ChinaXiaoli Cai, Wuhan University of Science and Technology, China
Copyright © 2025 Tammina, Abdelmonem and Bogireddy. This is an open-access article distributed under the terms of the Creative Commons Attribution License (CC BY). The use, distribution or reproduction in other forums is permitted, provided the original author(s) and the copyright owner(s) are credited and that the original publication in this journal is cited, in accordance with accepted academic practice. No use, distribution or reproduction is permitted which does not comply with these terms.
*Correspondence: Naveen Kumar Reddy Bogireddy, bmF2ZWVuQGljZi51bmFtLm14