- 1The Department of Ophthalmology, Sichuan Provincial People’s Hospital, University of Electronic Science and Technology of China, Chengdu, China
- 2Department of Laboratory Medicine, Sichuan Academy of Medical Sciences and Sichuan Provincial People's Hospital, University of Electronic Science and Technology, Chengdu, China
- 3Department of Stomatology, The Institute of Oral Science, Longgang Otorhinolaryngology Hospital of Shenzhen, Shenzhen, China
- 4Key Laboratory for Advanced Technologies of Materials, Institute of Biomaterials and Surface Engineering, Ministry of Education, Southwest Jiaotong University, Chengdu, China
- 5Department of Cardiology, Sichuan Provincial People’s Hospital, University of Electronic Science and Technology of China, Chengdu, China
- 6Office of Scientific Research, Chongqing Industry Polytechnic College, Chongqing, China
- 7Clinical Immunology Translational Medicine Key Laboratory of Sichuan Province, Sichuan Provincial People’s Hospital, University of Electronic Science and Technology ofChina, Chengdu, China
Introduction: Titanium dioxide (TiO2) films have been widely studied as blood-contacting materials, but their positively charged surface and low density of surface hydroxyl (-OH) groups result in poor intrinsic anticoagulant properties. Furthermore, TiO2 surfaces readily adsorb carbon-containing contaminants from the environment, causing a rapid decline in anticoagulant performance during storage. Thus, improving TiO2's intrinsic anticoagulant properties and extending its shelf-life remain challenging.
Methods: We fabricated a bilayer film by depositing a ∼40 nm silica (SiO2) overlayer onto TiO2 using unbalanced magnetron sputtering. Surface properties (hydrophilicity, surface charge, and contaminant adsorption) and anticoagulant performance (platelet adhesion after storage) of the resulting SiO2/TiO2 bilayer were characterized.
Results: The SiO2/TiO2 bilayer exhibited long-lasting hydrophilicity, a net negative surface charge, minimal adsorption of carbonaceous contaminants, and a high surface -OH group content. These characteristics are attributed to the formation of interfacial Si–O–Ti bonds, which in turn led to significantly enhanced anticoagulant properties. Notably, after 15 weeks of storage, platelet surface coverage on the bilayer was less than 30% of that on a TiO2-only film, indicating greatly improved long-term hemocompatibility.
Discussion: By maintaining a hydrophilic, clean surface with abundant surface -OH groups, the SiO2/TiO2 bilayer achieved superior intrinsic anticoagulant performance that was preserved over long-term storage. This bilayer approach addresses key limitations of TiO2, suggesting that SiO2/TiO2 coatings are a promising alternative to pure TiO2 films for blood-contacting devices.
1 Introduction
Titanium dioxide (TiO2) films have been widely used as surface modification materials for blood-contacting devices (Nan et al., 1998), such as vascular stents (Yang et al., 2019; Cui et al., 2021), due to their relatively good hemocompatibility (Shao et al., 2010), corrosion resistance (Shan et al., 2008), and biosafety (Zhang et al., 2020). However, the anticoagulant properties of TiO2 films still need to be further enhanced. Since the 1990s, many modification methods for enhancing the anticoagulant properties of TiO2 films have been reported. One of the typical strategies is to modify anticoagulant biomolecules or load drugs on the TiO2 surface (Li et al., 2018; Huang et al., 2014; Fan et al., 2016). However, introducing biomolecules or drugs brings new problems, such as the complex and poorly reproducible preparation processes and the long-term stability of modified biomolecules in vivo (Weng et al., 2011; Yang et al., 2017). On the other hand, changing the structures or chemical features of the TiO2 films to enhance its anticoagulant properties has not stopped. The mechanism of those strategies can be attributed to: (1) increasing hydrophilicity (Xu et al., 2017). The hydrophilic surface can effectively reduce blood protein and platelet adhesion. (2) Loading negative charge (Zhao et al., 2014). It can reduce the adhesion of negatively charged proteins in the blood through electrostatic repulsion. (3) Increasing −OH content (Yi et al., 2018). −OH can effectively reduce the degree of protein denaturation during the adsorption process. However, the long-term preservation of the anticoagulant properties of TiO2 films under routine storage has received less attention.
Since 2008, the aging issue of the bioactivity of TiO2 has started to attract attention (Att et al., 2009a; Att et al., 2009b). Clean TiO2, which is superhydrophilic and significantly positively charged, can promote protein and cell adhesion and growth. However, with the increase of storage time, TiO2 continuously adsorbs carbon-containing adsorbates from the air, leading to the loss of hydrophilicity and the change of surface charge from positive to negative, leading to the decline of protein and cell adhesion (Hori et al., 2010; Iwasa et al., 2010).
The gradual transformation of the naturally bioactive surface of TiO2 into a biologically inert surface when stored in the air became an essential insight for using Ti materials in dental and orthopedic applications (Ueno et al., 2010; Hirota et al., 2020). At the same time, this insight enlightens us that: (1) when TiO2 is used as a blood contact material, its anticoagulant ability is necessarily affected by the increase of carbon-containing adsorbates during the storage. (2) The new TiO2, with the hydrophilic but positively charged surface, which promotes the negative charged plasma protein adhesion (Barbir et al., 2021), may be detrimental to inhibiting thrombus generation. (3) After the storage, the negatively charged TiO2 surface, which is hydrophobic and contains procoagulant -CH3 groups from the carbon-containing adsorbates (Sivaraman and Latour, 2010a; Sivaraman and Latour, 2010b), may also be detrimental to inhibiting thrombus generation.
Starting in 2014, we have reported a series on the photo-induced anticoagulant properties of TiO2: after UV irradiation, by enhancing the hydrophilicity and oxidation of surface carbon-containing adsorbates, TiO2 films obtain excellent anticoagulant properties (Chen et al., 2014; Chen et al., 2015). However, this photo-induced anticoagulant property still diminishes with the increase in storage time (Liao et al., 2017). Therefore, long-term storage may be a common challenge for all types of Ti/TiO2-based blood contact devices since medical devices are subject to lengthy storage, transportation, and distribution processes before use.
We noticed that the anticoagulant properties of UV-treated TiO2 (TiO2-UV) are lost simultaneously with its photo-induced hydrophilic properties. Therefore, can the method to extend the storage time of TiO2 photophilic similarly extend the preservation time of its anticoagulant properties?
It has been reported that the construction of a surface consisting of a mixture of SiO2 and TiO2 by sol-gel method is an effective method for obtaining long-term superhydrophilicity (Guan and Yin, 2005). Meanwhile, the SiO2 and TiO2 mixed surface may be rich in -OH groups (Miyashita et al., 2001). However, the interface between SiO2 and TiO2 could form the Si-O-Ti bond, which has a positive electrostatic charge (Guan et al., 2003). The exposure of Si-O-Ti bond to the surface will be detrimental to the material’s anticoagulation. In this context, we envision the construction of bilayer films with SiO2 in the upper layer and TiO2 in the lower layer. This bilayer film could embed the positive Si-O-Ti bond at the interface, and the SiO2 surface is then likely to be negatively charged due to charge balance (Kim et al., 2011). This bilayer strategy requires the preparation of sufficiently thin and dense SiO2 films. Unbalance magnetron sputtering can produce dense TiO2 and SiO2 films with excellent mechanical properties on devices with complex surface topography, and the film thickness can be precisely controlled by the sputtering time and other process modulations (Buranawong et al., 2012; Seifarth et al., 1998).
In this study, we prepared TiO2 and SiO2 films by the unbalance magnetron sputtering. We prepared SiO2 films with different thicknesses on TiO2 films to obtain the SiO2 and TiO2 bilayer films. The bilayer films with SiO2 layer thicknesses of about 40 nm showed the best hydrophilic and anticoagulant properties. Then this optimal SiO2 and TiO2 bilayer film was evaluated in detail on the surface physical and chemical properties. Furthermore, the bilayer films’ natural and long-term hydrophilicity and long-term anticoagulant ability were systematically evaluated. Our results showed that the SiO2&TiO2 bilayer film had desirable natural and long-term superhydrophilicity and anticoagulant properties, which might be closely related to the inhibition of adsorption of carbon-containing adsorbates by the unique surfaces properties.
2 Materials and methods
2.1 Preparation of the SiO2 and TiO2 bilayer films
As shown in Figure 1, the TiO2 film was first prepared on a silicon substrate using the unbalance magnetron sputtering deposition system (UBMS450,Chinese Academy of Sciences, China), and then, in the same vacuum chamber, it was further covered with SiO2 film to obtain the SiO2 and TiO2 bilayer film. The detailed preparation parameters are shown in the Table 1.
2.2 UV irradiation treatment
The samples were UV irradiated for 1 h by a lithography machine (URE-2000, Chinese Academy of Sciences, China). The UV light intensity was 16 mW/cm2, and the UV wavelength was 365 nm.
2.3 Material characterization
A surface profiler (Ambios XP-2, Ambios, Santa Cruz, CA) was employed to determine the thickness of the films. A drop shape analysis system (DSA 100, Krüss, Germany) was used to examine the hydrophilicity of the films by the sessile drop method (5-µL droplet).
The structures of TiO2 films were determined by X-ray diffraction (XRD) (X’Pert Pro MPD, Philips, Holland) using a copper target at a glancing angle of 0.5°. An atomic force microscope (AFM) (SPI 3800; NSK, Japan) was used in tapping mode to detect the roughness of the samples.
X-ray photoelectron spectroscopy (XPS, XSAM800, Kratos Ltd., United KIngdom) was used to detect the surface chemical features of the films. The instrument was equipped with a monochromatic Al Kα (1,486.6 eV) X-ray source operated at 12 kV × 15 mA at a pressure of 2 × 10−10 mbar. The C 1s peak at 284.8 eV was used as a reference for charge correction. The surface information of the samples were measured directly using XPS without any cleaning. The subsurface Information of the samples were measured using XPS after ion-beam sputtering.
EST111 Static Charge Meter (EST Electro-Static Test, Co. Ltd., China) was used to detect the surface charge of samples (18 × 18 mm2).
2.4 Storage of samples
Samples were stored in the air for 1 day to 15 weeks to study the long-term preservation of surface properties and biological properties of the samples. The samples were placed in 24-well plates, covered by cling film to avoid adhesion of dust in the surface air, and stored in a class 10,000 clean room.
2.5 Platelet adhesion assay and P-selectin staining assay
The platelets adhesion test was detailly described elsewhere (Yang et al., 2020). Scanning electron microscope (SEM; Quanta 200, FEI, Holland) was used to observe the shape of adhered platelet. The optical microscope (DM4000M, Leica, Germany) was used to calculate the platelet surface coverage (PSC).
P-selectin was determined by indirect immunochemistry to examine the activation level of adhered platelets. Firstly, added 50 mL PRP on the samples and incubated at 37°C for 60 min. Then, washed the samples for 3 times with PBS. After that, each sample was covered with 20 µL of FITC-labeled antieCD62P (1:100) (MCA796GA, Serotec Co.). After incubating at 37°C for 60 min, washing samples for thress times, drying by N2 flow. Then the stained samples were observed under an inverted fluorescence microscope (IX51, Olympus, Japan).
2.6 Fibrinogen adsorption and its conformational change
Fresh platelet-poor plasma (PPP) was obtained by centrifuging venous blood at 3000 rpm for 15 min. Then, 40 µL of PPP was dropped on the TiO2 samples (7 × 7 mm2). Afterward, samples were incubated at 37°C for 1 h. Then, we use the immunochemistry method to determine the relative quantification of fibrinogen adsorption and conformational change.
2.6.1 Fibrinogen adsorption test
Firstly, the samples were incubated with PPP and were rinsed thoroughly with PBS. Then the samples were blocked with 1 wt% bovine serum albumin (BSA) at 37°C for 1 h. Subsequently, rewashed the samples and added 20 µL of HRP(Horseradish Peroxidase)-labeled mouse-anti-human fibrinogen monoclonal antibody (first antibody, diluted 1:200 in PBS). Then, the samples were incubated at 37°C for 1 h before adding 70 µL of chromogenic substrate TMB solution (diluted 1:4 in PBS) to the samples’ surface for color reaction. After reacting for 15 min, 50 µL of 1 M H2SO4 was added to stop the color reaction. Afterward, 70 µL of the solutions on the sample’s surface was removed to a 96-well plate to be examined by a microplate reader at 450 nm. The ratio of adsorbed fibrinogen was calculated according to the calibration curve.
2.6.2 Fibrinogen conformational change test
The detection of fibrinogen conformational changes is broadly similar to the fibrinogen adsorption assay. The differences are: (1) the first antibody used is a mouse-anti-human γ-fibrinogen monoclonal antibody; (2) after the introduction of the first antibody and sufficient washing, a further second antibody was introduced: HRP-labeled goat-anti-mouse polyclonal antibody (second antibody, diluted 1:200 in PBS). The relative quantification of the exposure of fibrinogen’s γ chain was calculated according to the calibration curve.
2.7 Data analysis and statistics
Three parallel samples (n = 3) were used for all experiments. Statistical significance between sample groups was assessed using SPSS 11.5 software with one-way ANOVA and LSD post hoc test. Differences between samples were considered statistically different when P < 0.05.
3 Results and discussion
3.1 Effect of SiO2 film thickness on hydrophilicity and anticoagulant properties of SiO2 and TiO2 bilayer films
As shown in Figure 2A, the TiO2 film was obtained by magnetron sputtering with a thickness of about 220 nm. Then the SiO2 films were deposited on the TiO2 film to obtain the SiO2&TiO2 bilayer films. By altering the film deposition time, SiO2&TiO2 bilayer films with SiO2 films with thicknesses of about 17 nm, 42 nm, and 83 nm were prepared, which were named 1#, 2#, and 3#, respectively. In addition, SiO2 films were deposited on Si as control samples.
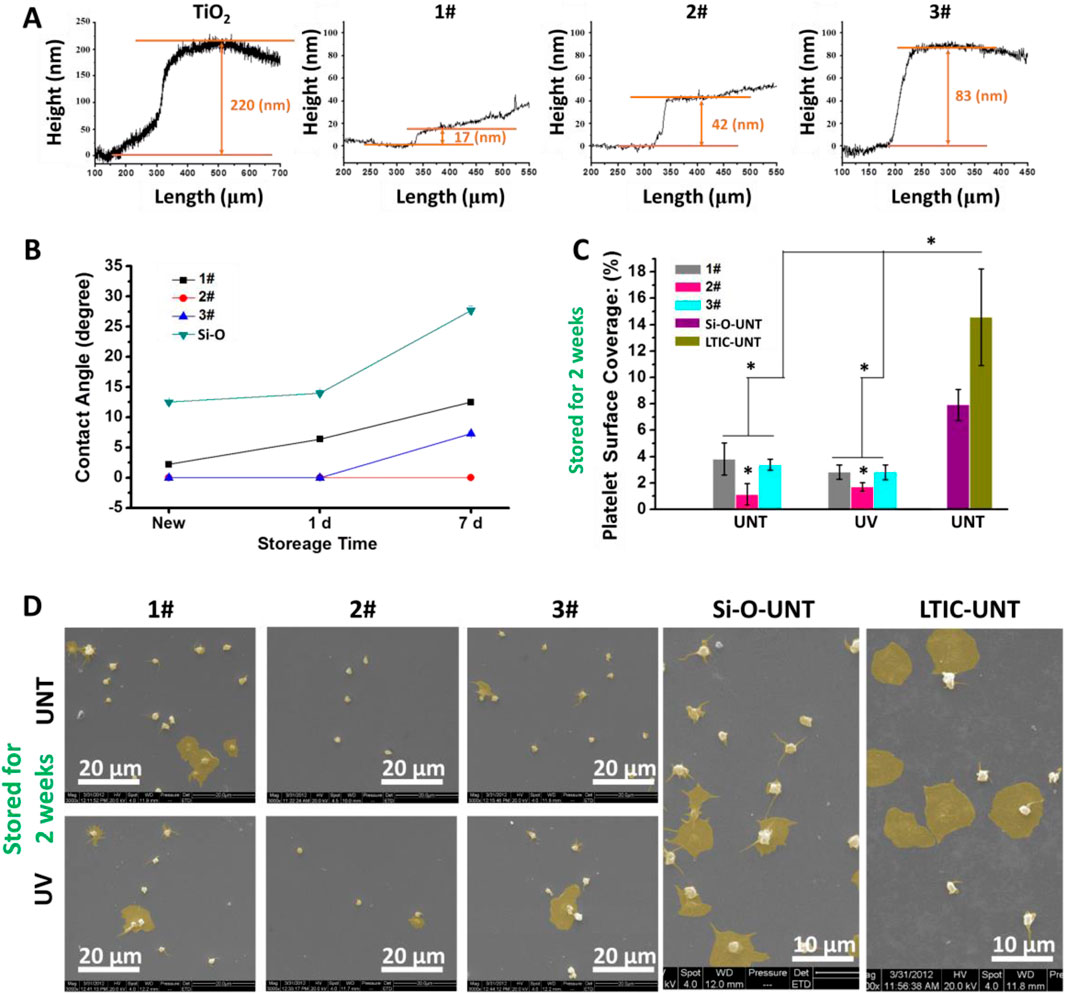
Figure 2. (A) Characterization of film thickness, (B) hydrophilicity of film after 7 days of storage in air, (C) statistics of platelet adhesion area ratio on film surface after 7 days of storage in air. (Data expressed as mean ± optical microscope, and analyzed using the one-way ANOVA and LSD post hoc test, *p < 0.05). (D) SEM photographs of adhered platelets.
As shown in Figure 2B, the newly fabricated 1#, 2#, and 3# were superhydrophilic surfaces (water contact angle <5°) (Padilla and Carey, 2014), among which the water contact angle of 2# and 3# were even 0°. At the same time, the SiO2 films directly deposited on Si had a water contact angle of about 12.5° and did not exhibit superhydrophilicity. After 1 day of storage, the water contact angle of 1# rose to 6.4° and lost superhydrophilicity, while 2# and 3# still maintained superhydrophilicity. When stored for 7 days, the water contact angles of SiO2, 1#, and 3# were about 7.3°, 12.5°, and 27.7° respectively, while the water contact angle of 2# remained at 0° and showed a superhydrophilicity.
Figure 2C showed the results of the platelet surface coverage (PSC, calculated from optical microscope photos) of samples. In the absence of UV treatment, the PSC values on 1#, 2#, and 3# were 3.8%, 1.1% and 3.3%, respectively, significantly lower than those on the positive control LTIC(7.9%) and SiO2 films (14.6%). The PSC value of 2# was lower than that of 1# and 3#. Meanwhile, the UV treatment showed no significant effect on the anticoagulant properties of the bilayer films (1#, 2#, 3#).
Figure 2D showed that many activated and spreading platelets were observed on the surfaces of the positive controls (SiO2 and LTIC). In 1# and 3#, most platelets adhere to the sample’s surface in a spherical shape, and only a few platelets undergo spreading, indicating the activation of platelets was inhibited (Chesnutt and Han, 2013). Moreover, in 2#, only a tiny amount of platelets were found to undergo spreading. Combining the hydrophilicity, platelet adhesion, and SEM results, we elected 2# for further study.
3.2 Structural and surface physical-chemical state analysis of SiO2&TiO2 bilayer films
As shown in Figure 3A, the XRD results indicated that the TiO2 obtained by magnetron sputtering is anatase crystalline type (Tekdir et al., 2021). After being covered by the SiO2 film, the XRD results of sample 2# showed a substantial decrease in the intensity of the TiO2 signal. Meanwhile, SiO2 signal was not observed, which could be related to the SiO2 layer being thin or not highly crystallized. As shown in Figure 3B, the roughness of the TiO2 film was 2.2 nm, while the roughness of sample 2# slightly increased to 3.4 nm.
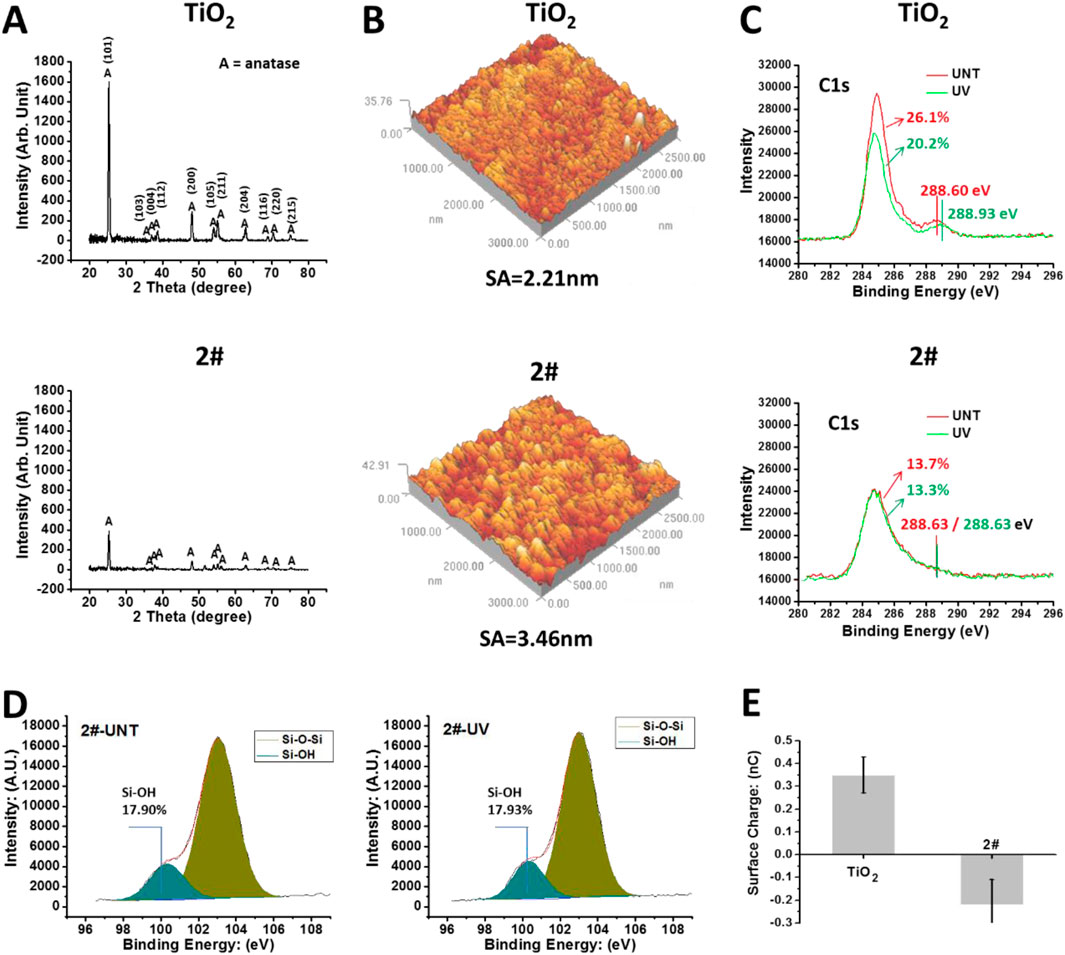
Figure 3. (A) XRD results of TiO2 with SiO2 and TiO2 bilayer films. (B) AFM results of the samples. (C) After 4 weeks of storage, XPS results of the sample surface: C1s high-resolution spectrum. (D) After 4 weeks of storage, the Si2p high-resolution spectrum on the surface of sample 2#. (E) The surface charge of the samples’ surface after 4 weeks of storage.
Then, we tested the chemical state of the samples’ surface using XPS without any cleaning. As shown in Figure 3C, after 4 weeks of storage, the shoulder peak around 288.6 eV, a characteristic peak of oxygen-containing hydrocarbon adsorbate (Ti-O-C) (Baek et al., 2013), was observed on the TiO2-UNT surface. This peak shifted toward 288.93 eV after UV irradiation, implying the oxidation of oxygen-containing hydrocarbon adsorbates due to the photocatalytic oxidation effect (Mattsson and Österlund, 2010), resulting in the generation of Ti-O-C=O residues (Xing et al., 2014). In contrast, on the surface of sample 2#, UV treatment did not affect the binding energy of the shoulder peak around 288.6 eV, which implied that 2# does not have the photocatalytic oxidation ability. In addition, the percentage of C element in the TiO2 surface was 26.1%, which decreased to 20.2% after UV irradiation. The decrease of C element on the surface of TiO2 after UV treatment was closely related to the photocatalytic self-cleaning effect of the TiO2 (Guan, 2005).
In comparison, the percentage of C elements on the surface of 2# was 13.7% and 13.3% before/after UV irradiation, indicating the 2# sample did not own the photocatalytic self-cleaning effect. However, the total amount of C elements on the 2# sample surface, about 13%, was significantly lower than that on the TiO2 surface (26.1%), even after UV self-cleaning (20.2%), indicating a significantly low carbon-containing adsorbate on the bilayer surface after 4 weeks of storage (Liao et al., 2021). This low amount of carbon-containing adsorbates on the surface of 2# might be beneficial and preserve the material surface’s inherent properties, such as hydrophilic properties (Antonello et al., 2014).
As shown in Figure 3D, the Si2p high-resolution spectrum of XPS showed that the surface of 2#, after 4 weeks of storage, contained a large amount of Si-OH (Paparazzo et al., 1992), and the UV treatment did not affect its Si-OH content. The large amount of -OH might bring high hydrophilicity and a negative charge to the surface of 2# (Houmard et al., 2011). As shown in Figure 3E, after 4 weeks of storage, the TiO2 surface had a positive charge (about 0.35 nC), while the 2# surface had a negative charge (about −0.22 nC).
In summary, SiO2 and TiO2 bilayer films had a low contamination rate, abundant hydroxyl groups, and negative charge, all of which might be beneficial to reduce coagulation reactions of the bilayer films in blood and ensure their anticoagulant properties for long-term storage in the air.
3.3 Analysis of the chemical state at the interface between SiO2 and TiO2 for SiO2 and TiO2 bilayer films
As shown in Figure 4A, the characteristic elemental signals of the 2# surface and the subsurface were detected using XPS at argon plasma etching (etching times (TE) = 0 s, 5 s, and 60 s). The results showed a high Si content on the surface and subsurface (TE = 0–60 s) of the 2# sample, and no Ti element was found. It indicated that SiO2 layer achieved a continuous and complete coverage of the TiO2 layer. When the TE extended to 840 s, the signal of the Si element started to fall, while the signal of the Ti element started to rise. When the TE = 1080 s, the Si element signal drops to within 5%, while the Ti element percentage rises to more than 25%. Obviously, when TE = 840–1080 s, the signal from the interface between SiO2 and TiO2 was collected.
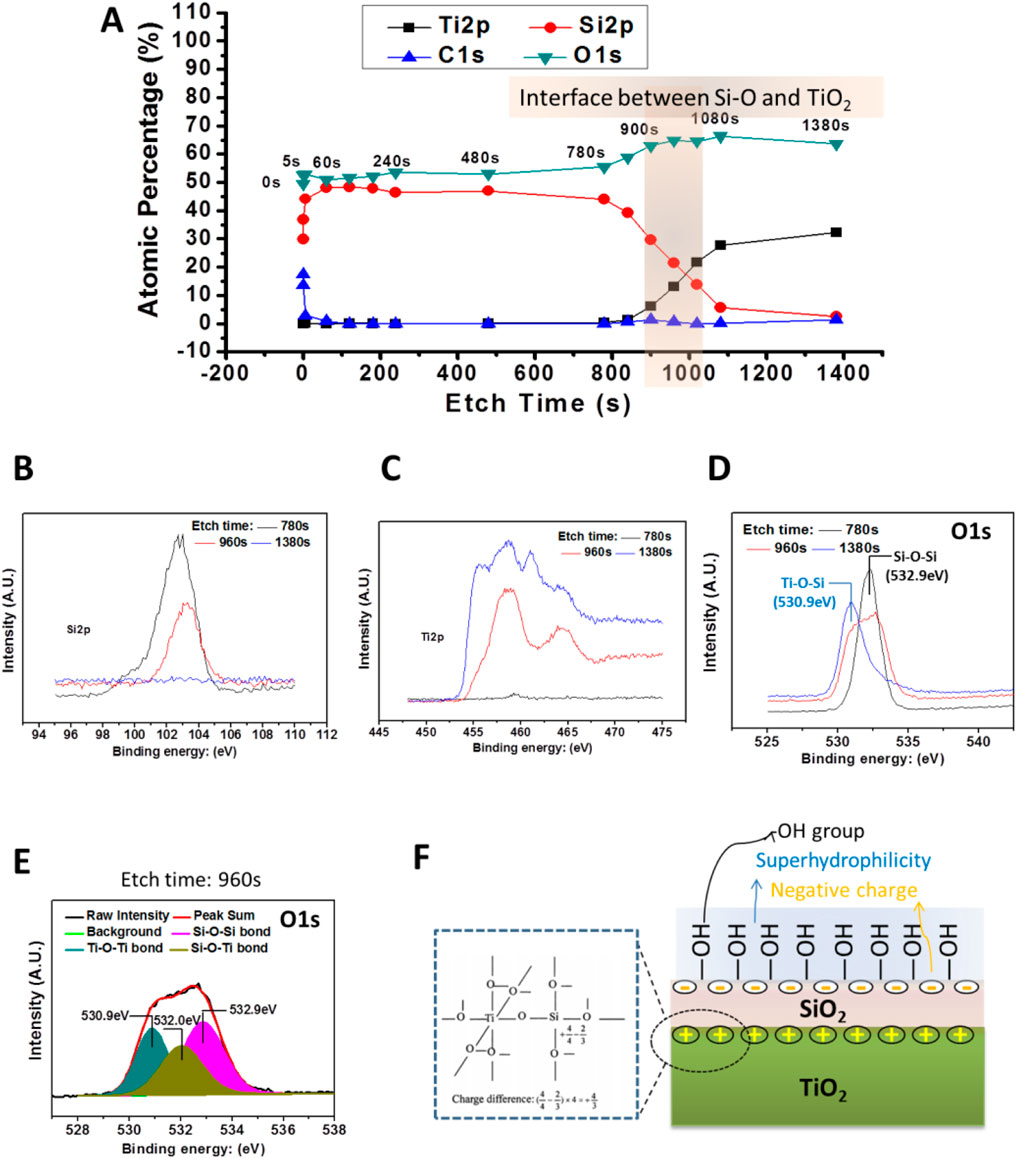
Figure 4. (A) XPS results of the bilayer film (2#) etching. (B,C) High-resolution patterns of Si2p and Ti2p peaks of the bilayer film were detected by XPS after different, etch times. (D) The high-resolution spectrum of O1s peaks of the bilayer films were detected by XPS after different, etch times. (E) O1s high-resolution spectrum of 2# after etching for 960 s. (F) Schematic diagram of 2# samples at the interface between TiO2 and SiO2 films and the possible physiochemical characteristics of the bilayer film’s surface.
Furthermore, by analyzing Figure 4A, it could be seen that TE = 960 s, the signal was from the SiO2 and TiO2 interface, while TE = 780 s and TE = 1380 s, the signal could be come from the SiO2 film and TiO2 film near the interface, respectively. Therefore, we analyze the high-resolution spectra of Si2p, Ti2p, and O1s when TE = 780 s, 960 s, and 1380 s. As shown in Figures 4B,C, for TE = 780 s, a strong Si peak is detected, while the Ti peak signal is very weak, and the opposite was true for the TE = 1380 s surface, where only the Ti signal was detected, while without the Si peak. In contrast, when TE = 960 s, both Si and Ti peaks were detected, and these peaks were lower than the Si peak at TE = 780 s and the Ti peak at TE = 960 s, respectively. These results verify that when TE = 780 s, 960 s, and 1380 s, signals were from SiO2, SiO2/TiO2 interface, and TiO2, respectively.
As shown in Figure 4D, the O1s high-resolution spectrum showed that when TE = 780 s, the peak at 532.9 eV could be attributed to the Si-O-Si bond, while the TE = 1380 s, the peak at 530.9 eV might come from the Ti-O-Ti bond (Gao and Wachs, 1999). However, it was interesting that when TE = 960 s, a broad peak appeared at 530.9–533.0 eV. Further analysis of this peak revealed (Figure 4E) that the O1s peak at TE = 780 s could be divided into three peaks. Besides the Si-O-Si bond and Ti-O-Ti bond mentioned earlier, an additional peak at 520.0 eV appeared, which could be attributed to the Si-O-Ti bond (Zhang et al., 2004). This result indicated that during the preparation of SiO2 films, Si atoms enter into the TiO2 film, forming a Si-O-Ti bond. As illustrated in Figure 4F, the Si-O-Ti bond formation leads to the charge imbalance so that a positive-rich charge might be formed at the interface between SiO2 and TiO2 (Guan et al., 2003; Kim et al., 2011), while a negative charge was formed on the outer surface of the SiO2 layer according to the charge balance principle (Permpoon et al., 2008). At the same time, the charge imbalance at the interface induced the formation of a large number of -OH groups. This might be why the SiO2 and TiO2 bilayer film’s surface was negatively charged and rich in -OH groups (Zhang et al., 2019).
3.4 Hydrophilicity testing of SiO2 and TiO2 bilayer films after long-term of storage
As shown in Figures 5A,B, the water contact angle of the new TiO2 film was 2.1°, which exhibited superhydrophilicity. While the water contact angle of the newly made 2# sample is 0, indicating that its hydrophilicity was more potent than that of the new TiO2 film. After 2 weeks of storage in air, the water contact angle of TiO2 increased to 22.4°, while the water contact angle of 2# was still 0°. After 4 weeks of storage, the water contact angle of TiO2 continued to rise to 42.5°, while the water contact angle of 2# was only 2.6°, still maintaining superhydrophilicity. After 7 weeks of storage, the water contact angle of TiO2 was 58.5°, while that of 2# was only 9.6°. Therefore, the natural superhydrophilicity of 2# seemed could maintain in the air for more than 4 weeks. Finally, when the storage time came to 15 weeks, the TiO2 water contact angle increased to 71°, while the 2# water contact angle was only 38.6°. The above results show that the bilayer film had better hydrophilic properties than TiO2, and its hydrophilic properties could be preserved in the air longer than TiO2. Further, after UV irradiation treatment of the 15-week-stored TiO2, its water contact angle decreased to 3.2°, exhibiting photo-induced superhydrophilicity, which could be related to the abundant of–OH in its surface (Houmard et al., 2011). In contrast, the hydrophilicity of sample 2# did not change after UV irradiation. This result indicated that it did not possess photo-induced hydrophilic properties, which could be related to the absence of the photocatalytic activity of the bilayer films (Wang et al., 1997).
3.5 Anticoagulant property analysis of SiO2 and TiO2 bilayer films after long-term of storage
As shown in Figures 6A,C, many activated and spreading platelets were found on the freshly prepared, stored for 4 weeks, and stored for 15 weeks TiO2 surfaces. The platelet surface coverage (PSC, calculated from optical microscope photos) of the above samples was 8.2%, 17.4%, and 15.2%, respectively. After UV treatment, the PSC of TiO2 stored for 4 and 15 weeks significantly decreased to 1.8% and 1.4% due to the photo-induced anticoagulant effect of TiO2. Moreover, the platelet morphology on the TiO2-UV surface was predominantly spherical, indicating the platelets’ activiation was suppresed (Chesnutt and Han, 2013).
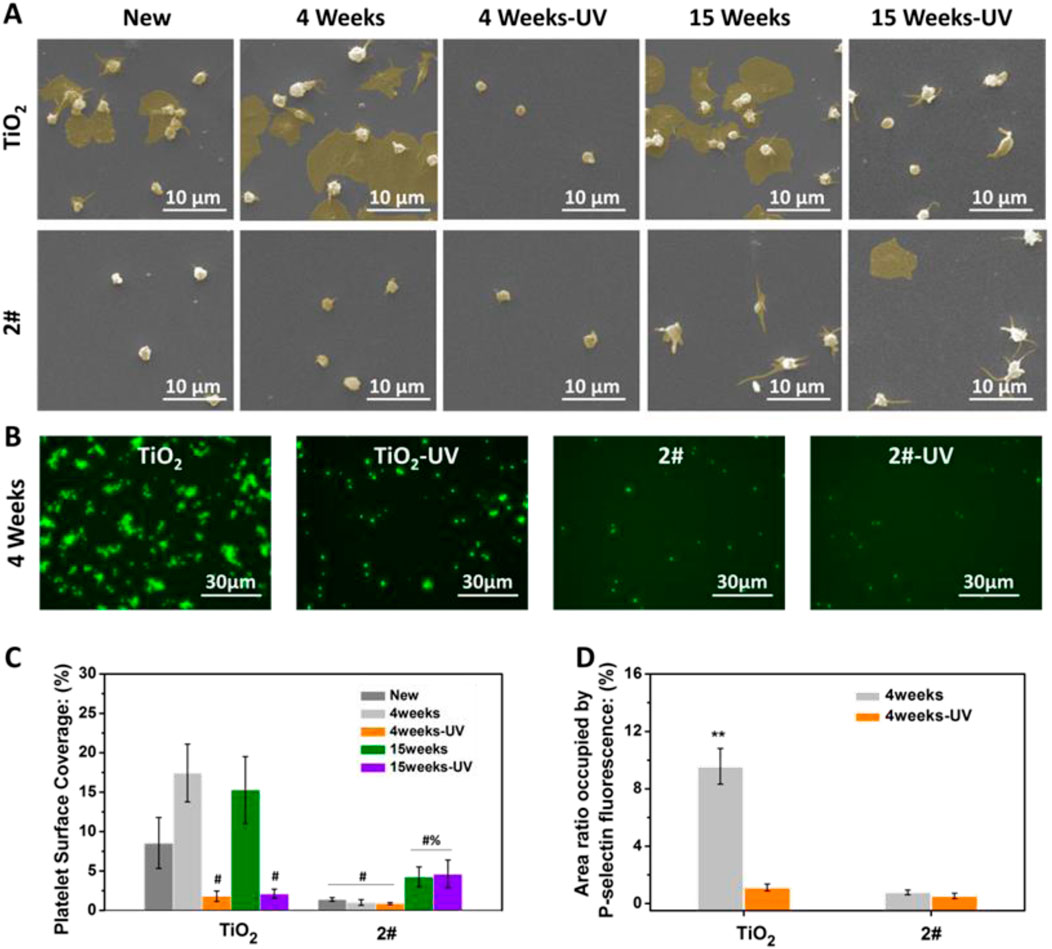
Figure 6. (A) SEM photographs of platelets, (B) platelet surface coverage (PSC) (# indicating the significant difference (p < 0.05) between samples and the positive controls (untreated TiO2 films), % indicating the significant difference (p < 0.05) between samples and the negative controls (UV-treated TiO2 films)), (C) P-selectin staining photographs, (D) P-selectin statistical results. (**p < 0.01) (Data expressed as mean ± optical microscope or fluorescence microscope, and analyzed using the one-way ANOVA and LSD post hoc test).
For sample 2#, only a small amount of spherical platelet adhesion was found on the surface of the freshly prepared sample, and its PSC was about 1.0%. Only a small amount of spherical platelet adhesion was found for 4 weeks of stored 2#, no matter with or without UV irradiation. The PSCs of 2#-UNT and 2#-UV were about 0.8% and 0.7%, showing an excellent anticoagulant property. On the surface of samples stored for 15 weeks, no matter with or without UV irradiation, most platelets on 2# extended pseudopods and individual platelets underwent spreading. The PSCs of 2#-UNT and 2#-UV were about 4.2% and 4.6%.
The above results indicated that the new and the 4 weeks stored 2# bilayer films possess anticoagulant properties comparable to, or even better than, UV-irradiated TiO2 films. After 15 weeks of storage, the anticoagulant properties of 2# decreased but were still remarkable. This result fully illustrated that the bilayer film strategy could be expected to provide long-term, excellent anticoagulant properties.
As shown in Figures 6B,D, P-selectin staining was performed on the platelets adhered to the samples after 4 weeks of storage. Many P-selectin-positive platelets were found on the TiO2 surface, accounting for a surface area ratio of 9.5%, indicating a large number of platelet activation (Blann and Lip, 1997). On the TiO2-UV surface, P-selectin-positive cells were substantially reduced, only in 1.1% of the surface area. In contrast, on the 2# and 2#-UV surfaces, the area ratio of P-selectin positive cells was 0.8% and 0.5%, which is less than the value of TiO2-UV. This result indicated that 2# could inhibit platelet activation better than TiO2-UV. This result further illustrated the excellent natural anticoagulant property of SiO2 and TiO2 bilayer films and the long-term preservation of the anticoagulant properties.
3.6 Possible mechanism of the anticoagulant property of SiO2 and TiO2 bilayer films
Fibrinogen is a negatively charged plasma protein with a molecular weight of 330 kD (Fujikawa et al., 2001; Price et al., 2001), and it is the coagulation factor I (Sørensen et al., 2012). Adsorption of fibrinogen to the material’s surface is a fundamental cause of coagulation. Therefore, we further investigated the adhesion behavior of fibrinogen in order to study the anticoagulation mechanism of SiO2 and TiO2 films. The samples were stored for 4 weeks before the test. As shown in Figure 7A, the fibrinogen adsorption on TiO2 without and with UV treatment was about 18.6% and 8.7%, respectively. As for 2# without and with UV irradiation, the fibrinogen adsorption values were 8.8% and 9.1%, respectively. This result indicated that 2# bilayer films had the same strong ability to inhibit fibrinogen adsorption as TiO2-UV. In particular, the ability of the 2# sample to inhibit fibrinogen adsorption was abolished when it was pretreated with Ca2+ for 12 h. This indicated that the electrostatic repulsion worked during the negatively charged 2# surface inhibited the adsorption of negatively charged fibrinogen in the blood (Iwasa et al., 2010).
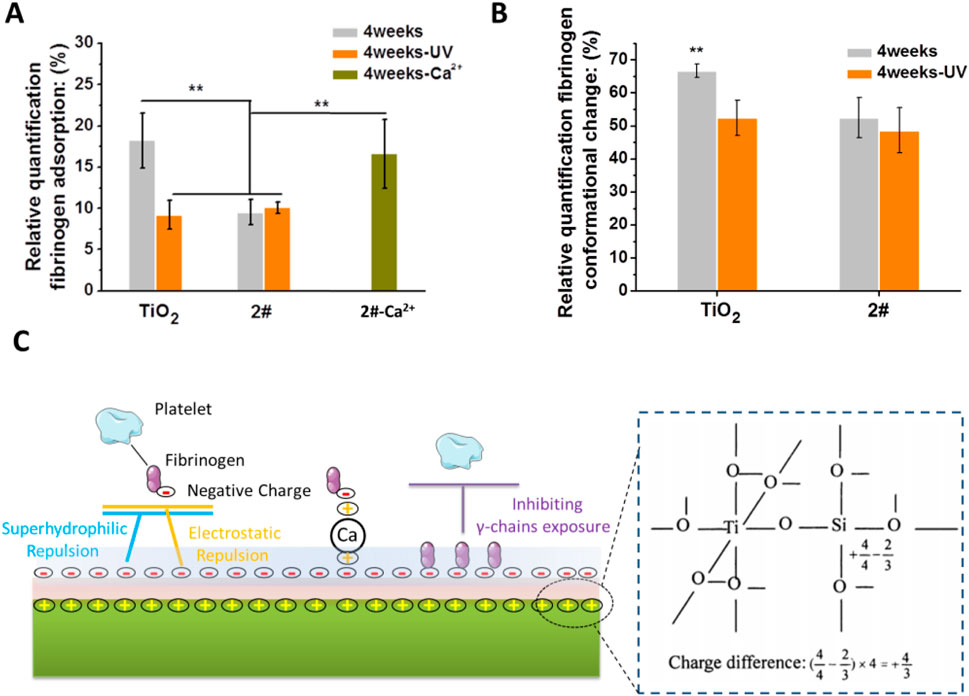
Figure 7. (A) Fibrinogen adsorption. (B) Fibrinogen conformational changes. (C) Possible anticoagulation mechanism of SiO2 and TiO2 bilayer films.
Fibrinogen denaturation is a more direct cause of blood coagulation than fibrinogen adhesion (Sivaraman and Latour, 2010a). Therefore, we performed the fibrinogen conformational change experiments. As shown in Figure 7B, the 2# sample had a natural ability to inhibit fibrinogen denaturation close to that of TiO2-UV. The superhydrophilic (Li et al., 2020), low carbon-containing adsorbates (Liao et al., 2021) adsorption and -OH group-rich nature (Rodrigues et al., 2006) of 2# played a crucial role in its anticoagulant property.
As shown in Figure 7C, we briefly summarized the possible mechanisms of the long-term anticoagulant properties of SiO2 and TiO2 bilayer films. The formation of Ti-O-Si bond at the interface between SiO2 and TiO2 induced the loading of positive charge at the interface (Guan et al., 2003; Kim et al., 2011), thus resulting in the surface of the bilayer film becoming negative charging (Permpoon et al., 2008), superhydrophilic (Houmard et al., 2011), rich in -OH groups (Zhang et al., 2019), and low in carbon-containing adsorbates adsorption (Houmard et al., 2011). These unique physical-chemical properties reduce fibrinogen adsorption on 2#, most likely through hydrophilic and electrostatic repulsion. In addition, the bilayer films inhibited the denaturation of fibrinogen, which might be achieved by the large amount of -OH group that could maintain the natural conformational of fibrinogen (Rodrigues et al., 2006). More importantly, the anticoagulant properties of this SiO2 and TiO2 bilayer film lasted up to 15 weeks when stored in air, probably due to its -OH group-rich and long-term hydrophilic property, which resulted in slow contamination of its surface with carbon-containing adsorbates (Houmard et al., 2011), and thus prolonged preservation of its anticoagulant properties.
Recently, Zhao et al. from Sichuan University reported an anticoagulant coating with hidden positive charges, which concealed the positive charges through PEG and prevented the adsorbed factor XII from initiating subsequent coagulation by the fixation effect of positive charges on factor XII and the hydrophilic effect of PEG (Ji et al., 2024). Our research has significant similarities with theirs, but we are the first to achieve the above design through inorganic materials. We believe that this study has theoretical reference significance for the subsequent design of fixed-hydrophilic anticoagulant coatings.
4 Conclusion
The SiO2 and TiO2 bilayer films were prepared by the unbalance magnetron sputtering technique. The surface of this film exhibited the following features: (1) long-lasting superhydrophilicity for more than 4 weeks, (2) high content of -OH, (3) negative charging, and (4) low adsorption rate of carbon-containing absorbates. The above features might be closely related to forming a positively charged Si-O-Ti bond at the interface between the SiO2 and TiO2 layers. Due to their unique surface physicochemical properties, SiO2 and TiO2 bilayer films could effectively inhibit the adsorption and activation of fibrinogen in the blood, thus exhibiting excellent anticoagulant properties and could be stored in the air for a long time. This study provided a new idea to solve the difficulty of long-term preservation of the anticoagulant properties of TiO2 films. This strategy of bilayer films was expected to provide a theoretical reference for developing new multifunctional TiO2-based films.
Data availability statement
The original contributions presented in the study are included in the article/supplementary material, further inquiries can be directed to the corresponding authors.
Ethics statement
Ethical approval was not required for the studies on humans in accordance with the local legislation and institutional requirements because only commercially available established cell lines were used.
Author contributions
XL: Methodology, Writing – original draft. XC: Writing – original draft. HJ: Writing – original draft. ZH: Writing – review and editing. HS: Writing – original draft, Data curation, Investigation. QH: Writing – original draft, Methodology. AZ: Writing – original draft, Data curation, Investigation. NH: Writing – original draft, Investigation, Validation. PY: Writing – review and editing, Data curation, Investigation. JC: Conceptualization, Funding acquisition, Project administration, Writing – review and editing.
Funding
The author(s) declare that financial support was received for the research and/or publication of this article. This work was supported by the Sichuan Science and Technology Program No. 2023YFS0308 (Xiangqin Liu) and 2023YFS0043 (Jiang Chen), the Science and Technology Research Program of Chongqing Education Commission of China.(No. KJQN202103212) (Qiongjian Huang), and the National Natural Science Foundation of China No. 82070930 (Xiaoqi Liu).
Conflict of interest
The authors declare that the research was conducted in the absence of any commercial or financial relationships that could be construed as a potential conflict of interest.
Generative AI statement
The authors declare that no Generative AI was used in the creation of this manuscript.
Publisher’s note
All claims expressed in this article are solely those of the authors and do not necessarily represent those of their affiliated organizations, or those of the publisher, the editors and the reviewers. Any product that may be evaluated in this article, or claim that may be made by its manufacturer, is not guaranteed or endorsed by the publisher.
References
Antonello, A., Soliveri, G., Meroni, D., Cappelletti, G., and Ardizzone, S. (2014). Photocatalytic remediation of indoor pollution by transparent TiO2 films. Catal. Today 230, 35–40. doi:10.1016/j.cattod.2013.12.033
Att, W., Hori, N., Iwasa, F., Yamada, M., Ueno, T., and Ogawa, T. (2009b). The effect of UV-photofunctionalization on the time-related bioactivity of titanium and chromium–cobalt alloys. Biomaterials 30 (26), 4268–4276. doi:10.1016/j.biomaterials.2009.04.048
Att, W., Hori, N., Takeuchi, M., Ouyang, J., Yang, Y., Anpo, M., et al. (2009a). Time-dependent degradation of titanium osteoconductivity: an implication of biological aging of implant materials. Biomaterials 30 (29), 5352–5363. doi:10.1016/j.biomaterials.2009.06.040
Baek, M.-H., Jung, W.-C., Yoon, J.-W., Hong, J.-S., Lee, Y.-S., and Suh, J.-K. (2013). Preparation, characterization and photocatalytic activity evaluation of micro- and mesoporous TiO2/spherical activated carbon. J. Ind. Eng. Chem. 19 (2), 469–477. doi:10.1016/j.jiec.2012.08.026
Barbir, R., Capjak, I., Crnković, T., Debeljak, Ž., Jurašin, D. D., Ćurlin, M., et al. (2021). Interaction of silver nanoparticles with plasma transport proteins: a systematic study on impacts of particle size, shape and surface functionalization. Chem.-Biol. Interact. 335, 109364. doi:10.1016/j.cbi.2020.109364
Blann, A. D., and Lip, G. Y. (1997). Hypothesis: is soluble P-selectin a new marker of platelet activation? Atherosclerosis 128 (2), 135–138. doi:10.1016/s0021-9150(96)05980-1
Buranawong, A., Witit-anun, N., and Chaiyakun, S. (2012). Total pressure and annealing temperature effects on structure and photo-induce hydrophilicity of reactive DC sputtered TiO 2 thin films. Eng. J. 16 (3), 79–90. doi:10.4186/ej.2012.16.3.79
Chen, J., Yang, P., Liao, Y., Wang, J., Chen, H., Sun, H., et al. (2015). Effect of the duration of UV irradiation on the anticoagulant properties of titanium dioxide films. Acs Appl. Mater Inter 7 (7), 4423–4432. doi:10.1021/am509006y
Chen, J., Zhao, A., Chen, H., Liao, Y., Yang, P., Sun, H., et al. (2014). The effect of full/partial UV-irradiation of TiO2 films on altering the behavior of fibrinogen and platelets. Colloids Surf. B. Biointerfaces 122 (0), 709–718. doi:10.1016/j.colsurfb.2014.08.004
Chesnutt, J. K., and Han, H.-C. (2013). Platelet size and density affect shear-induced thrombus formation in tortuous arterioles. Phys. Biol. 10 (5), 056003. doi:10.1088/1478-3975/10/5/056003
Cui, J., He, S., Dai, S., Liu, L., Zhao, A., Lu, L., et al. (2021). Stepwise assembly of functional proteins on Photo-activated TiO2 surfaces confers anti-oxidative stress ability and stealth effect to vascular stents. Chem. Eng. J. 424, 130392. doi:10.1016/j.cej.2021.130392
Fan, Y., Pan, X., Wang, K., Wu, S., Han, H., Yang, P., et al. (2016). Influence of chirality on catalytic generation of nitric oxide and platelet behavior on selenocystine immobilized TiO2 films. Colloids Surf. B. Biointerfaces 145, 122–129. doi:10.1016/j.colsurfb.2016.04.043
Fujikawa, K., Suzuki, H., McMullen, B., and Chung, D. (2001). Purification of human von Willebrand factor–cleaving protease and its identification as a new member of the metalloproteinase family. Blood, J. Am. Soc. Hematol. 98 (6), 1662–1666. doi:10.1182/blood.v98.6.1662
Gao, X., and Wachs, I. E. (1999). Titania–silica as catalysts: molecular structural characteristics and physico-chemical properties. Catal. Today 51 (2), 233–254. doi:10.1016/s0920-5861(99)00048-6
Guan, K. (2005). Relationship between photocatalytic activity, hydrophilicity and self-cleaning effect of TiO2/SiO2 films. Surf. Coat. Technol. 191 (2-3), 155–160. doi:10.1016/j.surfcoat.2004.02.022
Guan, K., Lu, B., and Yin, Y. (2003). Enhanced effect and mechanism of SiO2 addition in super-hydrophilic property of TiO2 films. Surf. Coat. Technol. 173 (2-3), 219–223. doi:10.1016/s0257-8972(03)00521-8
Guan, K.-s., and Yin, Y.-s. (2005). Effect of rare earth addition on super-hydrophilic property of TiO2/SiO2 composite film. Mater Chem. Phys. 92 (1), 10–15. doi:10.1016/j.matchemphys.2004.01.044
Hirota, M., Ozawa, T., Iwai, T., Mitsudo, K., and Ogawa, T. (2020). UV-mediated photofunctionalization of dental implant: a seven-year results of a prospective study. J. Clin. Med. 9 (9), 2733. doi:10.3390/jcm9092733
Hori, N., Ueno, T., Minamikawa, H., Iwasa, F., Yoshino, F., Kimoto, K., et al. (2010). Electrostatic control of protein adsorption on UV-photofunctionalized titanium. Acta Biomater. 6 (10), 4175–4180. doi:10.1016/j.actbio.2010.05.006
Houmard, M., Berthomé, G., Joud, J., and Langlet, M. (2011). Enhanced cleanability of super-hydrophilic TiO2–SiO2 composite surfaces prepared via a sol–gel route. Surf. Sci. 605 (3-4), 456–462. doi:10.1016/j.susc.2010.11.017
Huang, J., Dong, P., Hao, W., Wang, T., Xia, Y., Da, G., et al. (2014). Biocompatibility of TiO2 and TiO2/heparin coatings on NiTi alloy. Appl. Surf. Sci. 313, 172–182. doi:10.1016/j.apsusc.2014.05.182
Iwasa, F., Hori, N., Ueno, T., Minamikawa, H., Yamada, M., and Ogawa, T. (2010). Enhancement of osteoblast adhesion to UV-photofunctionalized titanium via an electrostatic mechanism. Biomaterials 31 (10), 2717–2727. doi:10.1016/j.biomaterials.2009.12.024
Ji, H., Yu, K., Abbina, S., Xu, L., Xu, T., Cheng, S., et al. (2024). Antithrombotic coating with sheltered positive charges prevents contact activation by controlling factor XII–biointerface binding. Nat. Mater 24, 626–636. doi:10.1038/s41563-024-02046-0
Kim, H. J., Kang, I. G., Kim, D. H., and Choi, B. H. (2011). Dispersion characteristics of TiO2 particles coated with the SiO2 nano-film by atomic layer deposition. J. Nanosci. Nanotechno 11 (12), 10344–10348. doi:10.1166/jnn.2011.5011
Li, L., Yang, L., Liao, Y., Yu, H., Liang, Z., Zhang, B., et al. (2020). Superhydrophilic versus normal polydopamine coating: a superior and robust platform for synergistic antibacterial and antithrombotic properties. Chem. Eng. J. 402, 126196. doi:10.1016/j.cej.2020.126196
Li, P., Zheng, W., Ma, W., Li, X., Li, S., Zhao, Y., et al. (2018). In-situ preparation of amino-terminated dendrimers on TiO2 films by generational growth for potential and efficient surface functionalization. Appl. Surf. Sci. 459, 438–445. doi:10.1016/j.apsusc.2018.08.044
Liao, Y., Li, L., Chen, J., Yang, P., Zhao, A., Sun, H., et al. (2017). Tailoring of TiO2 films by H2SO4 treatment and UV irradiation to improve anticoagulant ability and endothelial cell compatibility. Colloids Surf. B. Biointerfaces 155 (Suppl. C), 314–322. doi:10.1016/j.colsurfb.2017.04.021
Liao, Y., Zhou, Z., Dai, S., Jiang, L., Yang, P., Zhao, A., et al. (2021). Cell-friendly photo-functionalized TiO2 nano-micro-honeycombs for selectively preventing bacteria and platelet adhesion. Mater. Sci. Eng. C 123, 111996. doi:10.1016/j.msec.2021.111996
Mattsson, A., and Österlund, L. (2010). Adsorption and photoinduced decomposition of acetone and acetic acid on anatase, brookite, and rutile TiO2 nanoparticles. J. Phys. Chem. C 114 (33), 14121–14132. doi:10.1021/jp103263n
Miyashita, K., Kuroda, S., Ubukata, T., Ozawa, T., and Kubota, H. (2001). Enhanced effect of vacuum-deposited SiO2 overlayer on photo-induced hydrophilicity of TiO2 film. J. Mater Sci. 36 (16), 3877–3884. doi:10.1023/a:1017901717571
Nan, H., Ping, Y., Xuan, C., Yongxang, L., Xiaolan, Z., Guangjun, C., et al. (1998). Blood compatibility of amorphous titanium oxide films synthesized by ion beam enhanced deposition. Biomaterials 19 (7), 771–776. doi:10.1016/s0142-9612(98)00212-9
Padilla, J., and Carey, V. P. (2014). Water droplet vaporization on superhydrophilic nanostructured surfaces at high and low superheat, ASME International Mechanical Engineering Congress and Exposition. American Society of Mechanical Engineers, V08BT10A070.
Paparazzo, E., Fanfoni, M., Severini, E., and Priori, S. (1992). Evidence of Si–OH species at the surface of aged silica. J. Vac. Sci. and Technol. A Vac. Surfaces, Films 10 (4), 2892–2896. doi:10.1116/1.577726
Permpoon, S., Houmard, M., Riassetto, D., Rapenne, L., Berthomé, G., Baroux, B., et al. (2008). Natural and persistent superhydrophilicity of SiO 2/TiO 2 and TiO 2/SiO 2 bi-layer films. Thin Solid Films 516 (6), 957–966. doi:10.1016/j.tsf.2007.06.005
Price, M., Cornelius, R., and Brash, J. (2001). Protein adsorption to polyethylene glycol modified liposomes from fibrinogen solution and from plasma. Biochimica Biophysica Acta (BBA)-Biomembranes 1512 (2), 191–205. doi:10.1016/s0005-2736(01)00330-3
Rodrigues, S. N., Gonçalves, I. C., Martins, M., Barbosa, M. A., and Ratner, B. D. (2006). Fibrinogen adsorption, platelet adhesion and activation on mixed hydroxyl-/methyl-terminated self-assembled monolayers. Biomaterials 27 (31), 5357–5367. doi:10.1016/j.biomaterials.2006.06.010
Seifarth, H., Grötzschel, R., Markwitz, A., Matz, W., Nitzsche, P., and Rebohle, L. (1998). Preparation of SiO2 films with embedded Si nanocrystals by reactive rf magnetron sputtering. Thin Solid Films 330 (2), 202–205. doi:10.1016/s0040-6090(98)00609-9
Shan, C., Hou, X., and Choy, K.-L. (2008). Corrosion resistance of TiO2 films grown on stainless steel by atomic layer deposition. Surf. Coat. Technol. 202 (11), 2399–2402. doi:10.1016/j.surfcoat.2007.08.066
Shao, H., Yu, C., Xu, X., Wang, J., Zhai, R., and Wang, X. (2010). Influence of Ti nanocrystallization on microstructure, interface bonding, surface energy and blood compatibility of surface TiO2 films. Appl. Surf. Sci. 257 (5), 1649–1654. doi:10.1016/j.apsusc.2010.08.116
Sivaraman, B., and Latour, R. A. (2010a). The relationship between platelet adhesion on surfaces and the structure versus the amount of adsorbed fibrinogen. Biomaterials 31 (5), 832–839. doi:10.1016/j.biomaterials.2009.10.008
Sivaraman, B., and Latour, R. A. (2010b). The Adherence of platelets to adsorbed albumin by receptor-mediated recognition of binding sites exposed by adsorption-induced unfolding. Biomaterials 31 (6), 1036–1044. doi:10.1016/j.biomaterials.2009.10.017
Sørensen, B., Larsen, O. H., Rea, C. J., Tang, M., Foley, J. H., and Fenger-Eriksen, C. (2012). “Fibrinogen as a hemostatic agent,” in Semin thromb hemost. Thieme Medical Publishers, 268–273.
Tekdir, H., Yetim, T., and Yetim, A. F. (2021). Corrosion properties of ceramic-based TiO2 films on plasma oxidized Ti6Al4V/316L layered implant structured manufactured by selective laser melting. J. Bionic Eng. 18 (4), 944–957. doi:10.1007/s42235-021-0055-6
Ueno, T., Yamada, M., Suzuki, T., Minamikawa, H., Sato, N., Hori, N., et al. (2010). Enhancement of bone–titanium integration profile with UV-photofunctionalized titanium in a gap healing model. Biomaterials 31 (7), 1546–1557. doi:10.1016/j.biomaterials.2009.11.018
Wang, R., Hashimoto, K., Fujishima, A., Chikuni, M., Kojima, E., Kitamura, A., et al. (1997). Light-induced amphiphilic surfaces. Nature 388 (6641), 431–432. doi:10.1038/41233
Weng, Y., Song, Q., Zhou, Y., Zhang, L., Wang, J., Chen, J., et al. (2011). Immobilization of selenocystamine on TiO2 surfaces for in situ catalytic generation of nitric oxide and potential application in intravascular stents. Biomaterials 32 (5), 1253–1263. doi:10.1016/j.biomaterials.2010.10.039
Xing, M., Shen, F., Qiu, B., and Zhang, J. (2014). Highly-dispersed boron-doped graphene nanosheets loaded with TiO2 nanoparticles for enhancing CO2 photoreduction. Sci. Rep. 4, 6341. doi:10.1038/srep06341
Xu, L., Zhang, K., Wu, C., Lei, X., Ding, J., Shi, X., et al. (2017). Micro-arc oxidation enhances the blood compatibility of ultrafine-grained pure titanium. Materials 10 (12), 1446. doi:10.3390/ma10121446
Yang, F., Chang, R., and Webster, T. J. (2019). 2. Int. J. Nanomed 14, 9955–9970. doi:10.2147/ijn.s199093
Yang, Y., Gao, P., Wang, J., Tu, Q., Bai, L., Xiong, K., et al. (2020). Endothelium-mimicking multifunctional coating modified cardiovascular stents via a stepwise metal-catechol-(amine) surface engineering strategy. Res. (Wash D C) 2020, 9203906. doi:10.34133/2020/9203906
Yang, Y., Li, X., Qiu, H., Li, P., Qi, P., Maitz, M. F., et al. (2017). Polydopamine modified TiO2 nanotube arrays for long-term controlled elution of bivalirudin and improved hemocompatibility. Acs Appl. Mater Inter 10 (9), 7649–7660. doi:10.1021/acsami.7b06108
Yi, X., Qiongjian, H., Jiang, C., and Nan, H. (2018). Effect of annealing treatment in different atmospheres on the anticoagulant ability of Ti–O films. J. Biomater. Tiss. Eng. 8 (2), 155–164. doi:10.1166/jbt.2018.1739
Zhang, G., Zhang, X., Yang, Y., Zhang, H., Shi, J., Yao, X., et al. (2020). Near-infrared light-triggered therapy to combat bacterial biofilm infections by MoSe2/TiO2 nanorod arrays on bone implants. Adv. Mater Interfaces 7 (1), 1901706. doi:10.1002/admi.201901706
Zhang, H., Luo, X., Xu, J., Xiang, B., and Yu, D. (2004). Synthesis of TiO2/SiO2 core/shell nanocable arrays. J. Phys. Chem. B 108 (39), 14866–14869. doi:10.1021/jp049770d
Zhang, M., Lei, E., Zhang, R., and Liu, Z. (2019). The effect of SiO2 on TiO2-SiO2 composite film for self-cleaning application. Surfaces Interfaces 16, 194–198. doi:10.1016/j.surfin.2018.10.005
Keywords: SiO2 and TiO2 bilayer films, long-term anticogulant property, long-term superhydrophilicity, platelet, fibrinogen
Citation: Liu X, Chen X, Jiang H, He Z, Sun H, Huang Q, Zhao A, Huang N, Yang P and Chen J (2025) Natural and long-term preservable anticoagulant property of SiO2 and TiO2 bilayer films. Front. Bioeng. Biotechnol. 13:1578099. doi: 10.3389/fbioe.2025.1578099
Received: 17 February 2025; Accepted: 29 April 2025;
Published: 19 May 2025.
Edited by:
Cuiqi Zhou, Cedars Sinai Medical Center, United StatesReviewed by:
Zhuoyue Chen, Northwest University, ChinaGuangsheng Du, Sichuan University, China
Xiaohui Mou, The Tenth Affiliated Hospital of Southern Medical University, China
Yuanyuan Cui, Henan University, China
Copyright © 2025 Liu, Chen, Jiang, He, Sun, Huang, Zhao, Huang, Yang and Chen. This is an open-access article distributed under the terms of the Creative Commons Attribution License (CC BY). The use, distribution or reproduction in other forums is permitted, provided the original author(s) and the copyright owner(s) are credited and that the original publication in this journal is cited, in accordance with accepted academic practice. No use, distribution or reproduction is permitted which does not comply with these terms.
*Correspondence: Ping Yang, eWFuZ3BpbmdAc3dqdHUuZWR1LmNu; Jiang Chen, MjgzODc2NTMzQHFxLmNvbQ==
†These authors have contributed equally to this work