- 1Department of Diagnostic and Interventional Radiology, University Hospital Bonn, Bonn, Germany
- 2Quantitative Imaging Lab Bonn, University Hospital Bonn, Bonn, Germany
- 3Department of Internal Medicine II–Cardiology, University Hospital Bonn, Bonn, Germany
- 4Department of Pediatric Cardiology, University Hospital Bonn, Bonn, Germany
Background: Mass COVID-19 vaccination campaigns have helped impede the COVID-19 pandemic. In rare cases, some vaccines have led to vaccine associated myocarditis in a specific subset of the population, usually young males. Cardiac magnetic resonance (CMR) can reliably diagnose vaccine associated myocarditis, but follow-up data of CMR proven acute myocarditis is scarce.
Materials and methods: Nine patients with acute vaccine associated myocarditis underwent baseline and follow-up CMR examinations and were compared to baseline parameters at initial presentation and to a group of 20 healthy controls. CMR protocol included functional assessment, T1 and T2 mapping, T2 signal intensity ratio, strain feature tracking, and late gadolinium enhancement (LGE).
Results: Myocarditis patients (n = 9, aged 24 ± 6 years, 8 males) underwent CMR follow-up after an average of 5.8 ± 4.3 months. All patients showed a complete resolution of visual myocardial edema while also demonstrating a reduction in overall LGE extent from baseline to follow-up (4.2 ± 2.1 vs. 0.9 ± 0.8%, p < 0.001), although visual LGE was still noted in all patients. Left ventricular ejection fraction was normal at baseline and at follow-up (58 ± 6 vs. 62 ± 4%, p = 0.10) as well as compared to a healthy control group (60 ± 4%, p = 0.24). T1 (1024 ± 77 vs. 971 ± 34 ms, p = 0.05) and T2 relaxations times (57 ± 6 vs. 51 ± 3 ms, p = 0.03) normalized at follow-up. Most patients reported a resolution of clinical symptoms, while two (22%) reported new onset of exertional dyspnea.
Conclusion: Patients with COVID-19 vaccine associated acute myocarditis showed a complete, uncomplicated resolution of myocardial inflammation on follow-up CMR, which was associated with a near complete resolution of symptoms. Minor, residual myocardial scarring was present on follow-up LGE imaging. The long-term implications of the remaining myocardial scar-tissue after vaccine associated myocarditis remain unknown warranting further studies.
Introduction
The start of the coronavirus disease-19 (COVID-19) outbreak caused by the severe acute respiratory syndrome coronavirus 2 (SARS-CoV-2) at the end of 2019 prompted a world-wide effort to curtail the spread of the pandemic. One possible way to achieve this was the development of safe and effective vaccines. This effort cumulated in a rapid deployment of a few COVID-19 vaccines, most commonly and notably the Ad26.COV2-S [recombinant] (Janssen) vaccine, the mRNA-1273 (Moderna) vaccine, the BNT162b2 (Pfizer/BioNTech) vaccine, and the ChAdOx1-S [recombinant] (AstraZeneca) vaccine. Shortly after mass immunization programs began, reports of vaccine associated adverse reactions, such as fever, deep venous thrombosis, and myocarditis, started to emerge. Myocarditis and pericarditis are rare cardiovascular adverse vaccine reactions with an estimated incidence of approximately 0.48 cases per 100,000 administered vaccines (1). The Vaccine Adverse Event Reporting System (VAERS), set up by the Disease Control and Prevention (CDC) and the U.S. Food and Drug Administration (FDA), currently record 94 cases of reported vaccine associated myocarditis for the Janssen vaccine, 980 for the Moderna vaccine and 1897 for the BioNTech vaccine (2). Typical clinical presentation includes new exertional dyspnea and/or acute chest pain, accompanied by elevated troponin T, hours to days after COVID-19 vaccination. Cardiac magnetic resonance (CMR) studies have shown that the pattern of myocardial involvement in vaccine associated myocarditis was similar to acute viral induced myocarditis (3–5). In accordance with the 2018 modified Lake Louise Criteria for the diagnosis of acute myocarditis, typical findings include generalized or focal edema, prolonged T1 or T2 relaxation times, increased extracellular volume (ECV), and focal necrosis on late gadolinium enhancement (LGE) imaging (6). Initial clinical studies suggest a mild clinical course of disease with rapid resolution of symptoms (7). However, there is currently a scarcity of follow-up studies, making it difficult to determine the possible risks associated with vaccine associated myocarditis. This study reports follow-up CMR findings in patients after initial acute vaccine associated myocarditis.
Materials and methods
This retrospective study was approved by the appropriate institutional ethics committee and performed in concordance with the Declaration of Helsinki and International Conference on Harmonization of Good Clinical Practice. The requirement for written informed consent was waived. Patients who underwent initial CMR with diagnosis of acute vaccine-associated myocarditis and subsequent follow-up CMR at the Department of Diagnostic and Interventional Radiology from September 2021 to August 2022 were retrospectively identified.
Initial symptoms for referral for CMR included exertional dyspnea, chest pain, fever, or palpitations with associated elevated troponin T, within hours or days of receiving a COVID-19 vaccine. All patients received at least one dose of a COVID-19 vaccine approved for use in the European Union. Initial CMR results were positive for myocarditis as defined by the 2018 Lake Louise criteria (6). Reasons for follow-up referrals were standardized CMR follow-up of acute myocarditis according to local guidelines, examination before return to physical activity, or persistent cardiac symptoms under exertion. Clinical patient information was gathered through the local hospital information system.
The control group consisted of healthy subjects without previous myocarditis and no cardiovascular disease history who underwent CMR for study control reasons. Controls were age-matched to the myocarditis cohort and had normal CMR results without structural abnormalities.
Cardiac magnetic resonance protocol
All CMR examinations were performed using clinical whole-body MRI systems (Ingenia 1.5T or 3.0T; Philips Healthcare, Best, The Netherlands). Signal reception was achieved by a 16-channel torso coil using a digital interface. A signal intensity correction algorithm (CLEAR: Constant LEvel AppeaRance; Philips Medical Systems) was utilized to correct for torso-coil related signal inhomogeneities. Short-axis, 2-chamber, 3-chamber, and 4-chamber cine views were acquired using electrocardiogram gated, breath-hold steady state free precession sequences for functional analysis. A transversal respiratory-gated fat-suppressed T2-weighted fast spin echo sequence (Philips MultiVane XD, Philips Healthcare, Best, The Netherlands) was acquired for the assessment of axillary and mediastinal lymphadenopathy, which was also included in the follow-up protocol. Myocardial edema was visualized using T2-weighted short-tau inversion-recovery sequences in short axis and transversal views. T2 STIR images were also used to calculate T2 signal intensity ratio. Myocardial T1 and T2 mapping was performed in end-diastolic short axis views with acquisition of apical, midventricular, and basal sections. A six-echo gradient spin-echo sequence (GraSE) was applied for myocardial T2 mapping (8). Myocardial T1 mapping was achieved using a standard 3(3)3(3)5 modified Look-Locker inversion recovery (MOLLI) acquisition scheme, with post-contrast T1 maps acquired 10 min after the administration of contrast medium (9). For contrast enhancement, a 0.2 mmol/kg of body weight bolus of gadoterate meglumine (Clariscan; GE Healthcare, Chicago, IL, USA) was used. Segmented inversion-recovery gradient-echo sequences for LGE imaging were obtained in short axis, 2-chamber, 4-chamber, and transversal views. The Look-Locker method was utilized to determine the optimal inversion time for LGE image acquisition as previously described (10). Sequence parameters for 1.5 Tesla are summarized in Supplementary Table 1.
Image analysis
Image analysis was performed by a board-certified cardiovascular radiologist (J.A.L with 10 years of experience in CMR) and a radiology resident (D.K. with 4 years of experience in CMR) using dedicated software (IntelliSpace Portal, version 12.1.4.; Philips Medical Systems). Papillary muscles were included for the volumetric quantification of the left ventricle. Global systolic radial, longitudinal, and circumferential strain were calculated by using feature tracking strain analysis software (CAAS MR Solutions, version 5.2.1.; Philips Medical Systems) using short-axis, 2-chamber, and 4-chamber balanced steady state free precession cine imaging.
Focal areas of regional high signal intensities in a non-ischemic distribution pattern on T2 short-tau inversion-recovery and on LGE images were visually assessed by consensus agreement of the two readers. Quantitative markers of myocardial edema (T2 signal intensity ratio) and myocardial injury and fibrosis (enhanced areas were defined as those with a signal intensity ≥ 3.0 standard deviations above the mean signal intensity of normal myocardium) were calculated as previously reported (11–13). Motion correction was achieved using a software-implemented algorithm (fast elastic image registration, IntelliSpace Portal) for myocardial T1 and T2 relaxation maps, deriving global T1 and T2 relaxation times. Hematocrit-corrected global ECV values were calculated as previously described (12, 14, 15). For scans at 1.5 Tesla, institution specific cutoffs (≥1000 ms for myocardial T1 relaxation times and ≥ 55.9 ms for myocardial T2 relaxation times) for the assessment of the 2018 Lake Louise criteria were used as previously described (16). LGE distribution was classified according to the American Heart Association 17 segment heart model (17). LGE localization was classified according to wall involvement (subepicardial, midmyocardial, subendocardial, transmural, or patchy). Axial T2 weighted images were assessed for axillary lymph node enlargement and compared to previous imaging in the injection arm of the vaccine. For the control group, the largest axillary lymph node of either side was used. The largest short axis diameter measured in millimeter was recorded.
Statistical analysis
Prism (version 8.4.1; GraphPad Software) and Jamovi (version 2.2; The Jamovi Project) were used for statistical analysis. Data are given as means ± standard deviation or as percent to absolute frequency. Continuous variables were summarized as median with interquartile range (IQR) or as mean ± standard deviation, as appropriate. Normal distribution was checked using the Shapiro–Wilk test. For comparison of continuous variables and inter-individual variables the Student’s T-test was used. A paired T-Test was used for the comparison of means in variables recorded at baseline and at follow-up. Mann–Whitney-U test was used for non-normal distributed data. Dichotomous variables were compared by using the χ2 test. One-way analysis of variance (ANOVA) followed by Tukey post-hoc multiple comparison tests was performed to compare variables in three groups. The level of statistical significance was set to P < 0.05.
Results
Patient characteristics
In total, nine patient datasets with baseline and follow-up CMR (8 male [89%], aged 24 ± 6 years) were available for retrospective analysis. A detailed comparison with 20 age-/and gender-matched controls (18 males [89%], aged 26 ± 7 years) is given in Table 1. Patients with vaccine associated myocarditis received the following vaccines: Pfizer/BioNTech (n = 7, 78%) of which one was a first dose, four were the second dose, and two were a booster dose (third vaccination); Moderna (n = 1, 11%) of which it was a second dose; Janssen (n = 1, 11%) first dose (only one dose required). Highly sensitive troponin T levels were elevated in all nine patients (median 644 ng/l [IQR: 159–930 ng/l]). All patients were treated with either cardioprotective or anticoagulative medication: 6 out of 9 (67%) were treated with beta-blockers, 4 (44%) with ACE-inhibitors, 2 (22%) with low molecular weight heparin, and one (11%) with a diuretic.
Clinical symptoms
All nine had clinical symptoms of acute myocarditis at initial scan: 8 out of 9 (89%) presented with chest pain, 3 (33%) with exertional dyspnea, and 2 (22%) with occasional fever. At follow up, only 1 out of 9 (11%) patients reported persistent chest pain, 2 patients reported new onset of exertional dyspnea (22%), and none reported fever. Patients did not report signs of infection prior to vaccination. Median number of days to symptom onset after vaccination was 0 days (IQR: 0–1 days; mean 0.6 ± 1.0 days), median time to initial CMR was 6.5 days (IQR: 5.3–12.8 days; mean 8.1 ± 3.9 days), and median time to follow-up was 3.0 months (IQR: 2.0–10.5; mean 5.8 ± 4.3 months).
Cardiac magnetic resonance results
T1 and T2 relaxation times from the same scanner at the same field strength (1.5T) were available for 8 patients while one patient received both, baseline and follow-up scans using a 3.0T MRI scanner. T1 and T2 maps from this patient were excluded from consecutive analysis. All patients demonstrated positive LGE findings typical for acute myocarditis. The most common LGE distribution pattern was subepicardial (n = 9, 100%), followed by midwall (n = 3, 33%) or diffuse transmural involvement (n = 2, 22%). LGE distribution according to the American Heart Association 17-segment heart model at baseline and at follow-up is depicted in Figure 1. Visual myocardial edema was noted in 7 out of 9 patients at baseline (78%) with complete resolution at follow-up in all patients. Direct baseline to follow-up imaging comparisons are shown in Figures 2–7. All p-values within the text are derived from a paired t-test analysis between baseline and follow-up data unless otherwise noted. All patients demonstrated a significant reduction of overall LGE extent from baseline to follow-up (4.2 ± 2.1 vs. 0.9 ± 0.8%, p = 0.001), although persistent LGE was noted in all patients at follow-up, consistent with post-inflammatory scar tissue. In two cases (22%) visual LGE on follow-up was barely discernable, and in all cases visual LGE demonstrated a reduction in signal intensity. Septal LGE sparring was noted in most patients and only 2 patients demonstrated septal LGE. T2 relaxation times were noted to be significantly higher at baseline compared to follow-up (57 ± 6 vs. 51 ± 3 ms, p = 0.03). T1 relaxation times were also higher at baseline, although not statistically significant (1024 ± 77 vs. 971 ± 34 ms, p = 0.05).
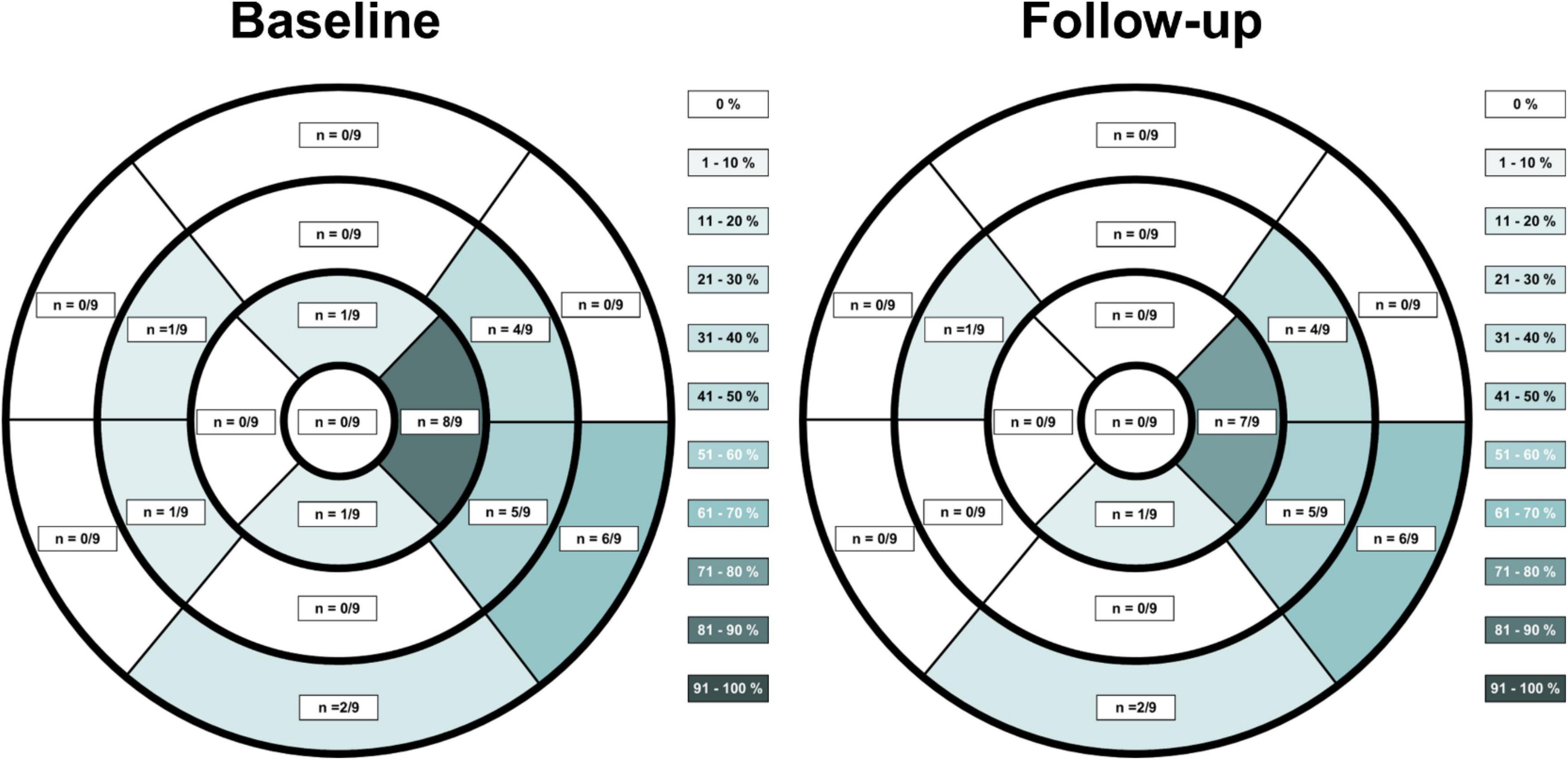
Figure 1. Late gadolinium enhancement (LGE) distribution on CMR in patients suffering from vaccine associated myocarditis at acute baseline imaging and at follow-up according to the American Heart Association 17-segment heart model. LGE extent was reduced on follow-up imaging, although LGE findings were still discernable at most previous locations.
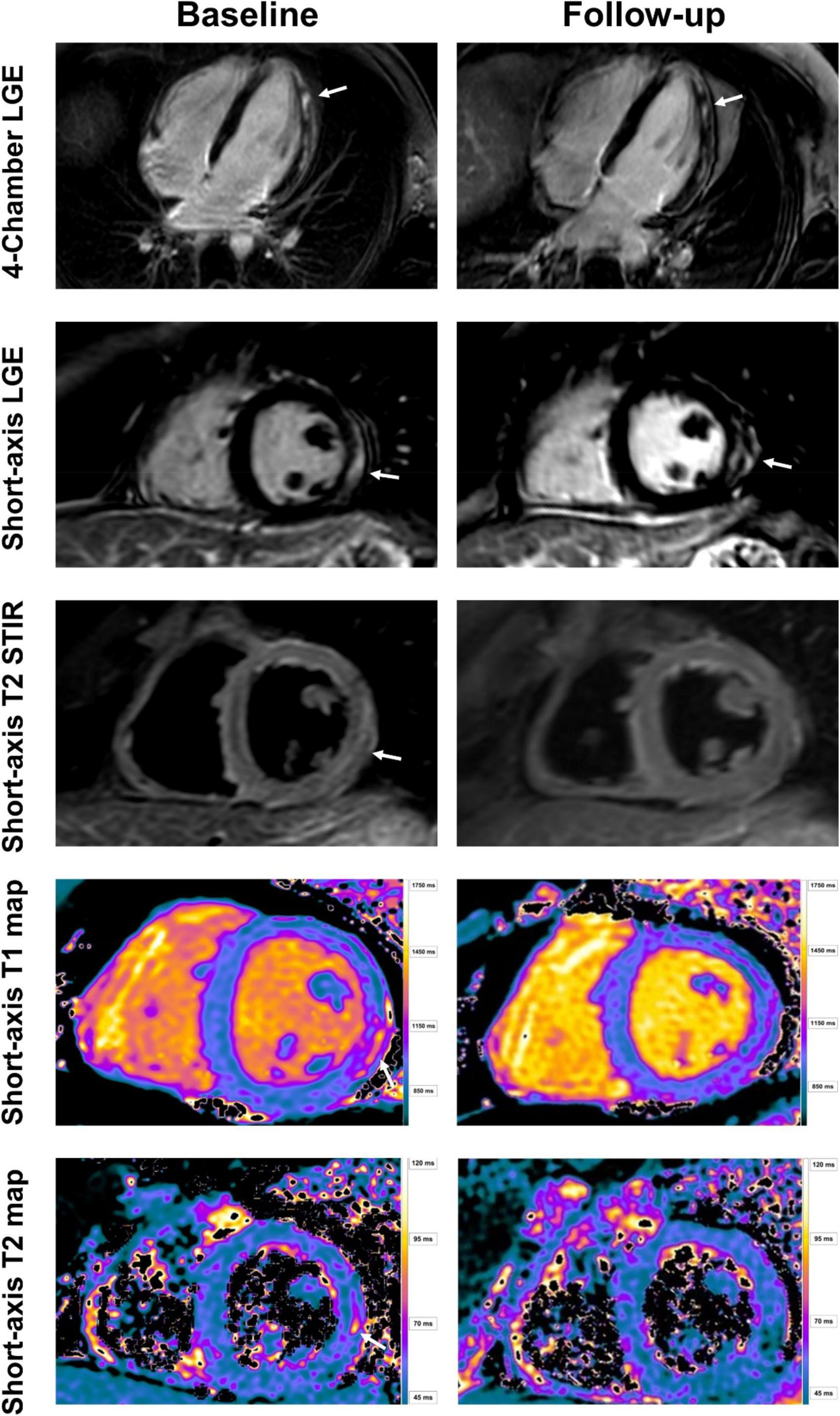
Figure 2. Baseline and follow-up cardiac imaging in a 25-year-old male with acute vaccine associated myocarditis after receiving his second vaccine with Pfizer/BioNTech. Short-axis and 4-chamber late gadolinium enhancement (LGE) views demonstrate subepicardial enhancement along the midventricular and apical inferolateral wall (arrows). T2 short-axis short tau inversion recovery (STIR) imaging corresponding to the LGE findings shows a resolution of edema from baseline to follow-up (arrow). Normalization of T1 and T2 relaxation times are also demonstrated over time (arrow). Note, however, the persistent LGE along the inferolateral wall even at follow-up, consistent with scar tissue.
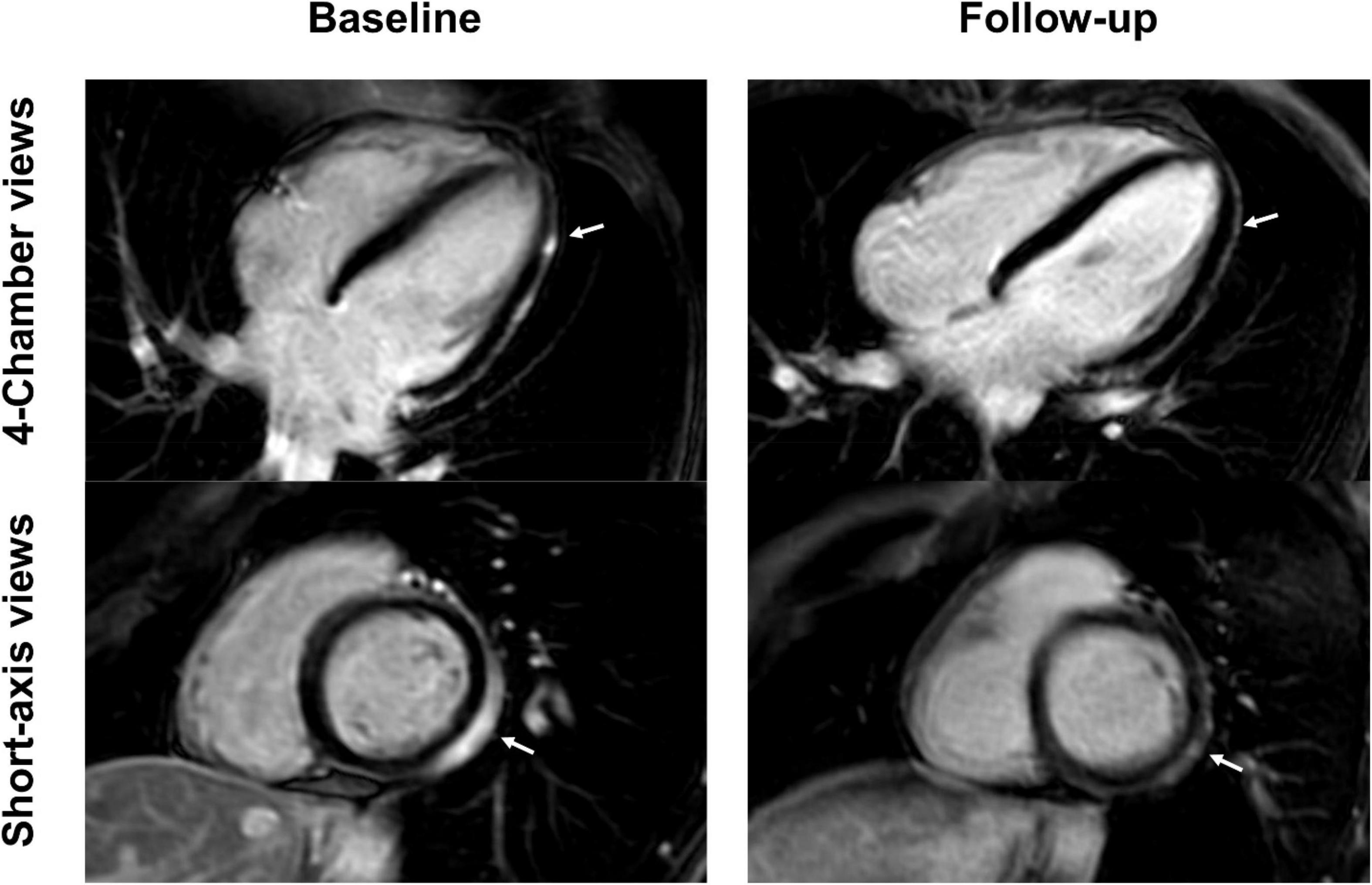
Figure 3. Short-axis and 4-chamber late gadolinium enhancement (LGE) views in a 28-year-old, previously healthy male after receiving his second Moderna vaccine. Reduction of subepicardial enhancement along the lateral wall from baseline (arrows) to follow-up 11-months later consistent with myocardial scarring. This patient reported new onset of occasional exertional dyspnea after initial acute vaccine associated myocarditis.
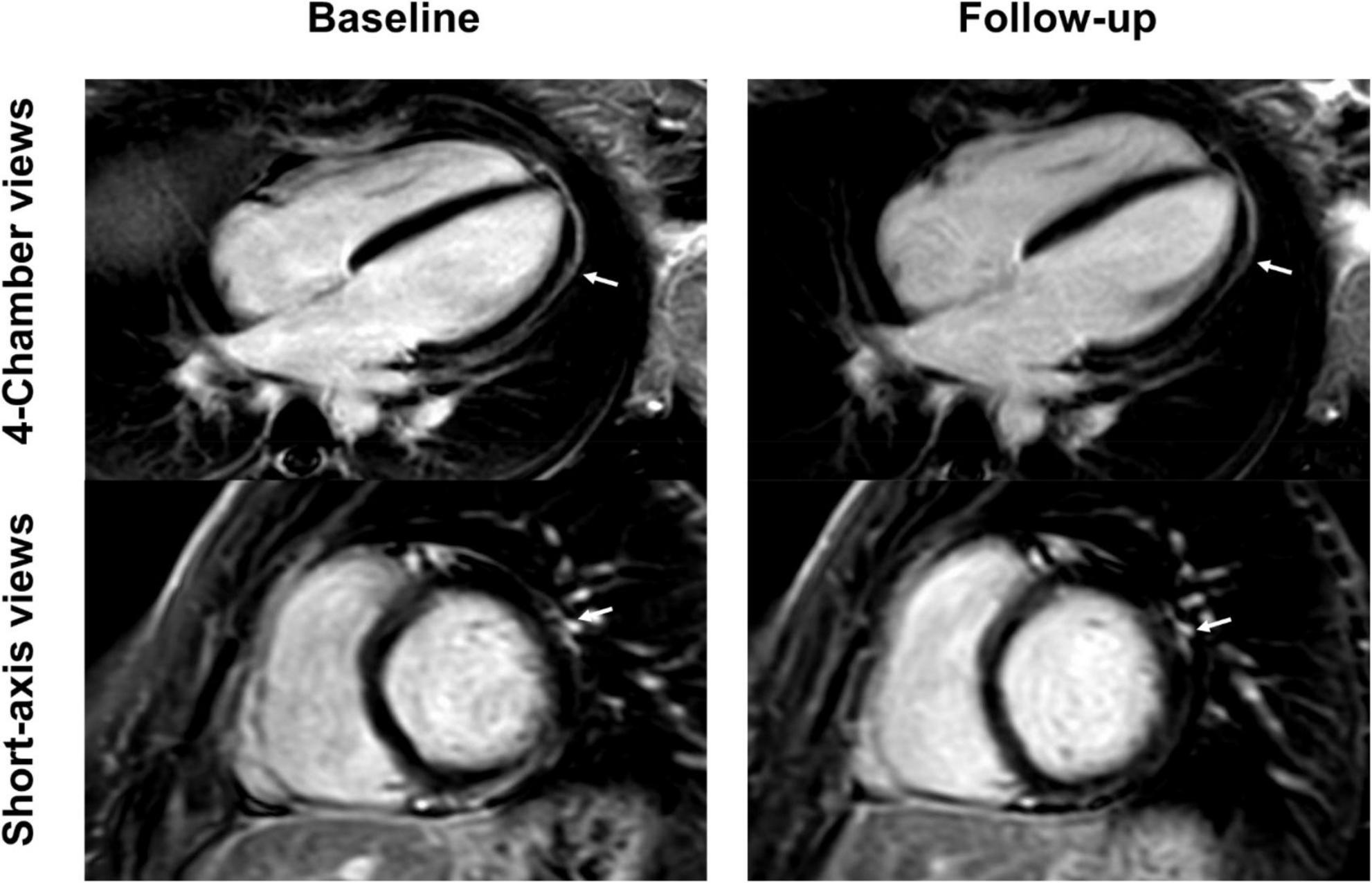
Figure 4. Short-axis and 4-chamber late gadolinium enhancement (LGE) views in a 26-year-old, previously healthy female after receiving the first dose of the Pfizer/BioNTech vaccine. Nearly complete resolution of LGE (arrows) at follow-up 2 months later with minor enhancement discernable at the apical lateral segment. Clinical correlation showed a complete resolution of previous symptoms which included chest pain and exertional dyspnea.
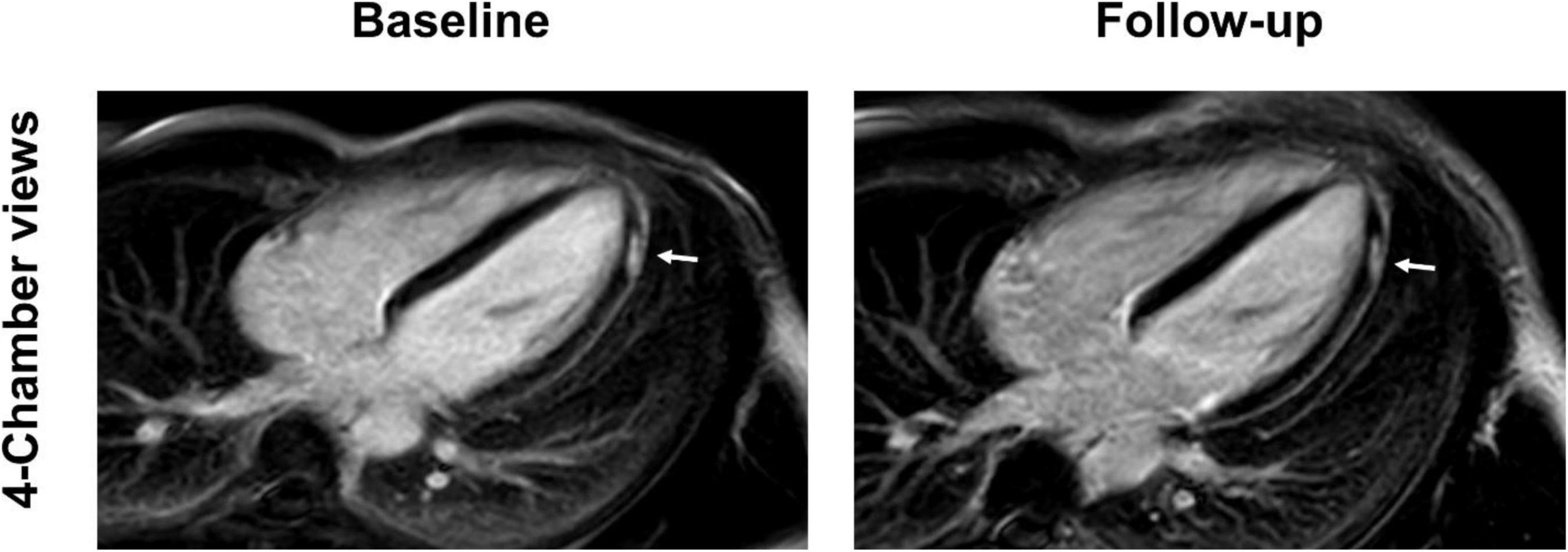
Figure 5. 4-chamber late gadolinium enhancement (LGE) views of a 36-year-old, previously healthy male after receiving the third dose of the Pfizer/BioNTech vaccine. The LGE lesion at the apical lateral wall (arrow) reduced at follow-up 3 months later, but was still visible, a finding which is consistent with scar tissue. Patient reported complete resolution of clinical symptoms which included chest pain and palpitations.
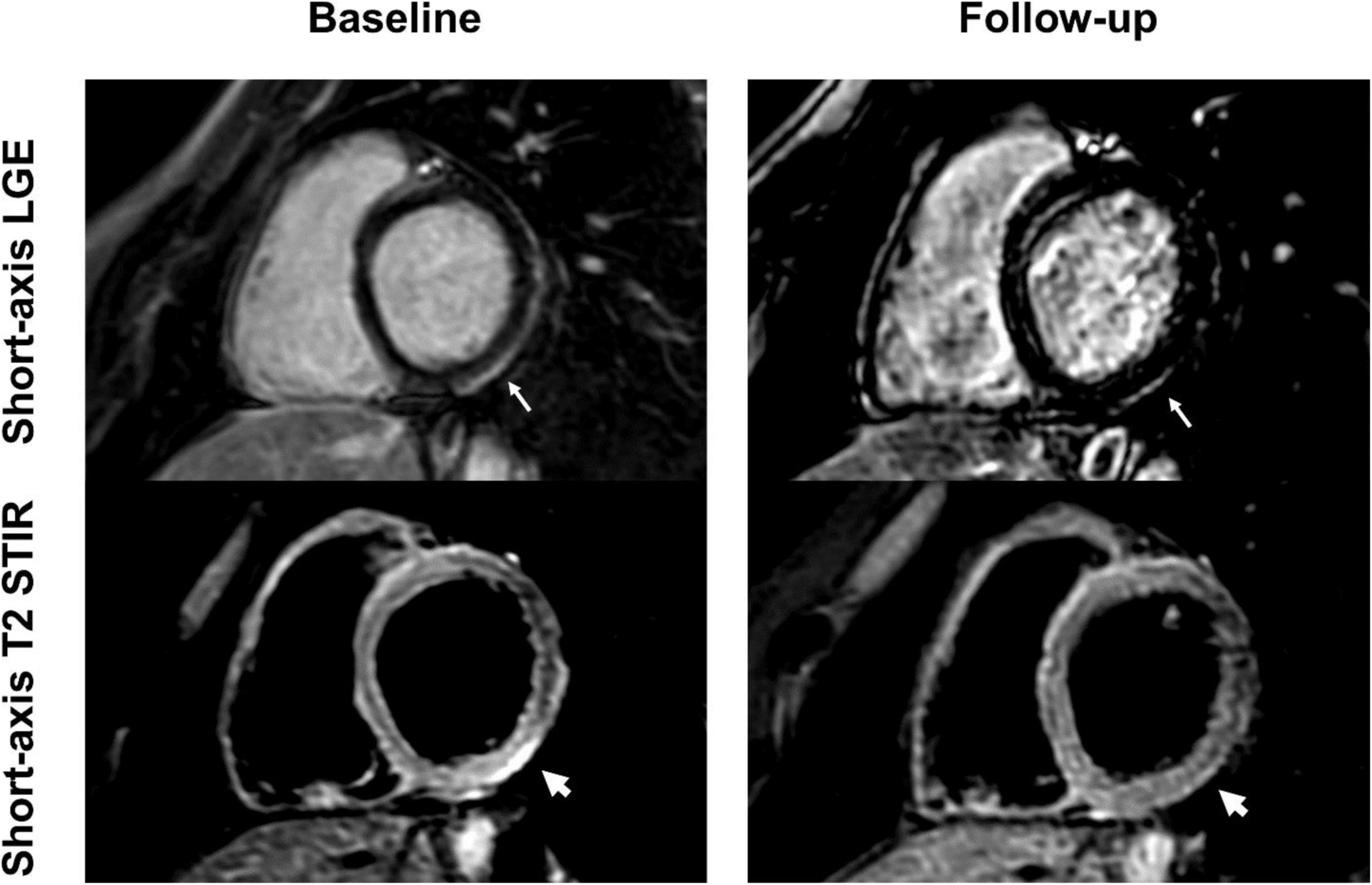
Figure 6. Short-axis late gadolinium enhancement (LGE) and short-axis T2 short tau inversion recovery (STIR) views of a 24-year-old, previously healthy male after receiving the second dose of the Pfizer/BioNTech vaccine. LGE at the basal inferolateral wall (thin arrows) shows a marked decrease at 6-month follow-up, with only minimal remaining findings. T2 STIR imaging shows focal myocardial edema corresponding to the location of the LGE (thick arrows) with complete resolution at follow-up. The patient reported complete resolution of previous clinical symptoms but complained of new onset exertional dyspnea.
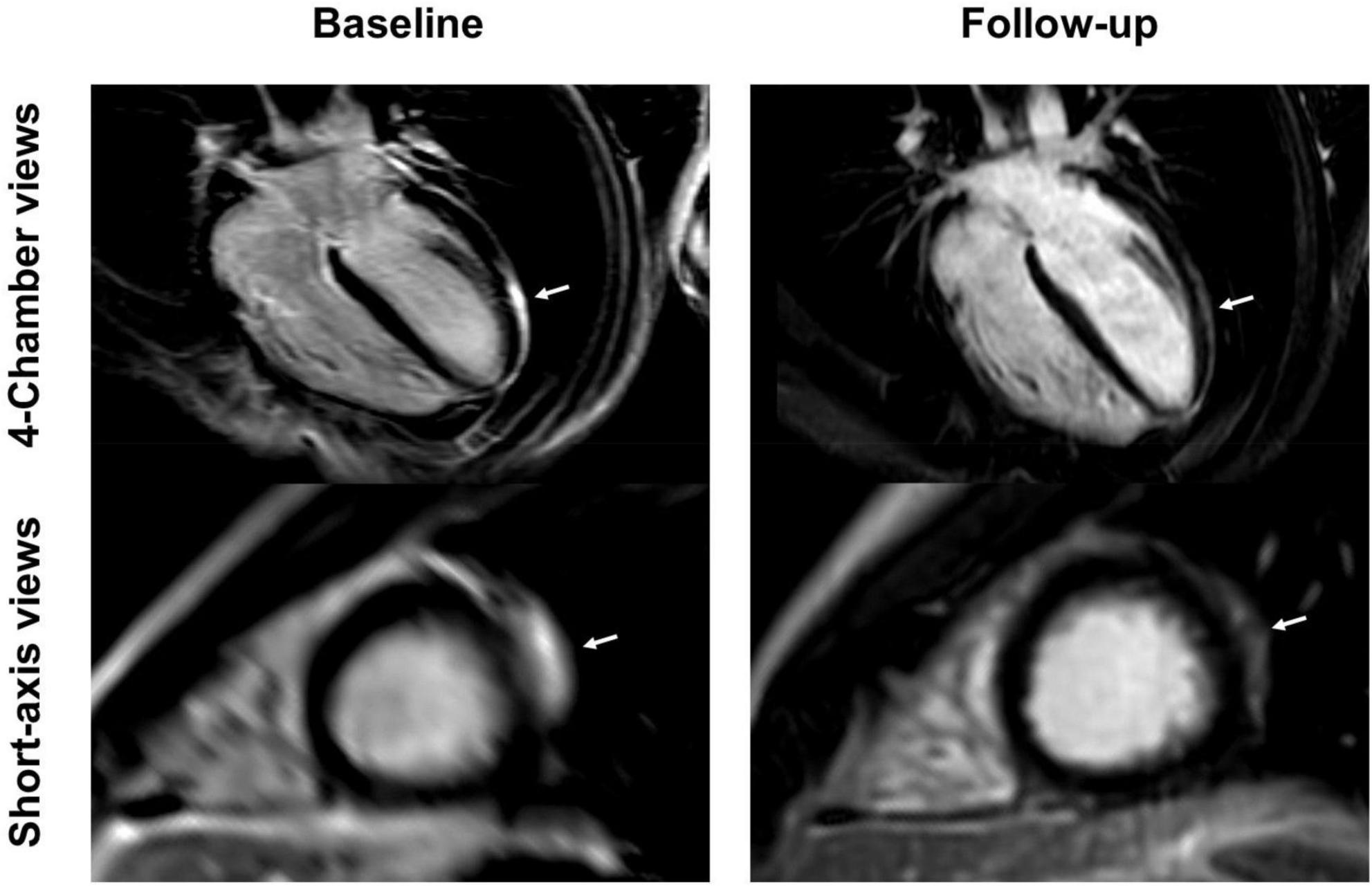
Figure 7. 4-chamber and short-axis late gadolinium enhancement (LGE) views of a 15-year-old male after receiving the second dose of the Pfizer/BioNTech vaccine. Subepicardial and pericardial LGE at the apical lateral wall (arrows) shows a marked decrease at the 9-month follow-up with residual findings. Resolution of chest pain at follow-up was accompanied by new onset of occasional exertional dyspnea.
No significant differences between baseline and follow-up investigation were noted for left ventricular ejection fraction (58 ± 6 vs. 62 ± 4%; p = 0.10), left ventricular end diastolic volume (158 ± 32 vs. 161 ± 28 ml; p = 0.62), or left ventricular end diastolic volume index (83 ± 12 vs. 83 ± 9 ml/m2; p = 0.87). A significant reduction in axillary lymph node size was noted between baseline and follow-up (11.8 ± 2.3 vs. 7.8 ± 2.3 mm; p = 0.003). A slightly significant improvement in systolic global circumferential strain (GCS) was noted from baseline to follow-up (−12.7 ± 1.9 vs. −14.9 ± 2.7%, p = 0.04). No such statistically significant difference was noted for systolic global radial strain (GRS, 25.6 ± 6.4 vs. 30.2 ± 10.2%, p = 0.12) or global longitudinal strain (GLS, −17.0 ± 2.2 vs. −16.4 ± 1.9%, p = 0.08) between baseline and follow-up. Table 2 provides an overview of some currently available data regarding vaccine associated myocarditis.
Discussion
This retrospective study analyzed clinical and CMR data from nine, non-hospitalized patients with vaccine associated myocarditis regarding parameters such as left ventricular function, LGE extent, and myocardial T1 and T2, at initial imaging and at follow-up as well as to an age and sex matched control group. While vaccine associated myocarditis is a rare possible complication of currently available COVID-19 vaccines, its clinical presentation and CMR characteristics at the acute stage and follow-up should be known to cardiovascular imaging physicians.
Vaccine associated myocarditis is more likely to occur after vaccination with mRNA-based vaccines, although we report of one case after vaccination with a vector vaccine (Janssen). The number of received doses also seems to play a role as vaccine associated myocarditis is less likely to be noted after the first dose, usually causing symptoms after the second or third booster dose, suggesting that prior exposure is necessary for the development of vaccine associated myocarditis. However, the exact pathomechanism of vaccine associated myocarditis remains unknown. Current theories focus on a hypersensitivity reaction or cross reactions of spike proteins with myocardial contractile proteins (33, 34).
Collaborating previously published findings, we found vaccine associated myocarditis to predominantly affect younger males (1, 3, 35). Typically, clinical course of disease is mild with rapid resolution of symptoms within a few months (17, 19, 25, 28, 33), although persistent LGE on CMR indicative of fibrous scar tissue was noted in all our patients. Resolution of other CMR findings (elevated T1 and T2 times, focal or diffuse edema) are to be expected as the extent of the inflammatory process diminishes over time. Other studies have noted a relatively normal left ventricular ejection fraction in vaccine associated myocarditis compared to patients with other causes of myocarditis (4). We found similar findings in our study, as parameters of myocardial function were not noticeably impaired. Cardiac strain has been shown to improve the diagnostic performance of the 2018 revised Lake Louise Criteria and provide prognostic value regarding major adverse cardiovascular events for acute myocarditis (36–39). Strain analysis might be able to detect subtle changes in myocardial tissue earlier when other traditional prognostic markers such as left ventricular ejection fraction (LVEF) or LGE are normal. Strain encoded MRI has been reported to be able to identify patients with subclinical LVEF dysfunction, potentially at risk for heart failure (40). In ischemic heart disease, strain encoded MRI was able to differentiate between reversible and irreversible myocardial injury (41). Reduced GLS and GCS have also been associated with edema in suspected acute myocarditis (42). Feature tracking strain analysis did not show any statistically significant difference in systolic GRS or GLS between the three groups. A decrease in GLS has been previously described to be a negative prognostic marker for major adverse cardiovascular events (36). A small, but discernable improvement was noted for GCS going from baseline to follow-up, as well as an insignificant improvement in GRS and worsening in GLS. The difference in these values might be attributed to the small patient population of this study. Resolution of symptoms was noted in most patients, although two patients reported new onset of occasional exertional dyspnea. This may be in part due to residual LGE findings on CMR, which has been previously described as a marker of unfavorable prognosis when paired with a resolution of associated edema (43). The full implications of remaining myocardial scar tissue are unknown in such a young patient group. The risk of developing ventricular arrhythmias for such young patients after vaccine-induced acute myocarditis is currently unknown. A preference for lateral ventricular wall involvement for LGE with septal sparring has been observed in the majority of patients, indicating a more favorable prognosis regarding the development of arrhythmias (44–47).
Our study suffers from limitations, including the small patient cohort and variable follow-up times. Most statistical comparisons were not corrected for multiple testing due to the small data set. As of date, there are no published consensus criteria for the diagnosis of vaccine associated myocarditis after COVID-19 vaccination. We therefore defined it as myocarditis like symptoms after COVID-19 vaccination with abnormal CMR findings in previously healthy people accompanied with elevated troponin T levels within a reasonable number of days of COVID-19 vaccination. Not all CMR examinations were performed on the same machine or at the same field strength, diminishing data sets available for statistical analysis. Furthermore, the reference standard for diagnosis of myocarditis, endomyocardial biopsy, was not performed as it is not part of the best standard of care practice at our institution. Further studies comparing CMR findings in vaccine associated myocarditis with other causes of myocarditis akin to data published by Fronza et al. offer an outlook for potential future research (35).
Conclusion
Vaccine associated myocarditis tends to affect younger, predominantly male patients and shows abnormal CMR findings such as focal or diffuse edema, elevated T1 and T2 relaxation times, and LGE. While the overall prognosis seems to be favorable and a rapid resolution of symptoms is observed, reduced, yet persistent LGE findings indicative of myocardial fibrosis in light of complete resolution of edema have been noted. Further studies are needed to examine the long-term effects of the remaining scar-tissue and develop recommendations for patients with a history of vaccine associated myocarditis regarding booster doses.
Data availability statement
The raw data supporting the conclusions of this article can be provided upon reasonable request by the authors, without reservation.
Ethics statement
The studies involving human participants were reviewed and approved by Ethikkommission der Medizinischen Fakultät Bonn, University Hospital Bonn, Venusberg-Campus 1, 53127, Bonn. Written informed consent from the participants or their legal guardian/next of kin was not required to participate in this study in accordance with the national legislation and the institutional requirements.
Author contributions
JL and DKr contributed to the conception and design of the study and performed the statistical analysis. DKr organized the database and wrote the first draft of the manuscript. JL, AI, DKu, DKr, CP, and SZ wrote sections of the manuscript. All authors contributed to the manuscript revision, read, and approved the submitted version.
Funding
This work was supported by German Foundation of Heart Research (F/28/2).
Conflict of interest
The authors declare that the research was conducted in the absence of any commercial or financial relationships that could be construed as a potential conflict of interest.
Publisher’s note
All claims expressed in this article are solely those of the authors and do not necessarily represent those of their affiliated organizations, or those of the publisher, the editors and the reviewers. Any product that may be evaluated in this article, or claim that may be made by its manufacturer, is not guaranteed or endorsed by the publisher.
Supplementary material
The Supplementary Material for this article can be found online at: https://www.frontiersin.org/articles/10.3389/fcvm.2022.1049256/full#supplementary-material
References
1. Witberg G, Barda N, Hoss S, Richter I, Wiessman M, Aviv Y, et al. Myocarditis after covid-19 vaccination in a large health care organization. N Engl J Med. (2021) 385:2132–9. doi: 10.1056/NEJMoa2110737
2. United States Department of Health and Human Services [DHHS], Public Health Service [PHS], Centers for Disease Control [CDC]/Food and Drug Administration [FDA].Vaccine Adverse Event Reporting System (VAERS) 1990 - 07/22/2022, CDC WONDER On-line Database. Washington, D.C: United States Department of Health and Human Services (accessed October 2022).
3. Kravchenko D, Isaak A, Mesropyan N, Endler C, Bischoff L, Vollbrecht T, et al. Cardiac MRI in suspected acute myocarditis after covid-19 mrna vaccination. Rofo. (2022) 194:1003–11. doi: 10.1055/a-1752-0951
4. Fronza M, Thavendiranathan P, Chan V, Karur GR, Udell JA, Wald RM, et al. Myocardial injury pattern at mri in covid-19 vaccine-associated myocarditis. Radiology. (2022) 304:553–62. doi: 10.1148/radiol.212559
5. Luetkens JA, Isaak A, Öztürk C, Mesropyan N, Monin M, Schlabe S, et al. Cardiac MRI in suspected acute covid-19 myocarditis. Radiology. (2021) 3:e200628. doi: 10.1148/ryct.2021200628
6. Luetkens JA, Faron A, Isaak A, Dabir D, Kuetting D, Feisst A, et al. Comparison of original and 2018 lake louise criteria for diagnosis of acute myocarditis: results of a validation cohort. Radiol Cardiothorac Imaging. (2019) 1:e190010. doi: 10.1148/ryct.2019190010
7. Truong DT, Dionne A, Muniz JC, McHugh KE, Portman MA, Lambert LM, et al. Clinically suspected myocarditis temporally related to covid-19 vaccination in adolescents and young adults: suspected myocarditis after covid-19 vaccination. Circulation. (2022) 145:345–56.
8. Sprinkart AM, Luetkens JA, Träber F, Doerner J, Gieseke J, Schnackenburg B, et al. Gradient spin echo (GraSE) imaging for fast myocardial T2 mapping. J Cardiovasc Magn Reson. (2015) 17:12. doi: 10.1186/s12968-015-0127-z
9. Messroghli DR, Radjenovic A, Kozerke S, Higgins DM, Sivananthan MU, Ridgway JP. Modified look-locker inversion recovery (MOLLI) for high-resolution T1 mapping of the heart. Magn Reson Med. (2004) 52:141–6. doi: 10.1002/mrm.20110
10. Look DC, Locker DR. Time saving in measurement of NMR and EPR relaxation times. Review Sci Instruments. (1970) 41:250–1. doi: 10.1063/1.1684482
11. Kramer CM, Barkhausen J, Flamm SD, Kim RJ, Nagel E. Standardized cardiovascular magnetic resonance (CMR) protocols 2013 update. J Cardiovasc Magn Reson. (2013) 15:91. doi: 10.1186/1532-429X-15-91
12. Luetkens JA, Doerner J, Thomas DK, Dabir D, Gieseke J, Sprinkart AM, et al. Acute myocarditis: multiparametric cardiac MR imaging. Radiology. (2014) 273:383–92. doi: 10.1148/radiol.14132540
13. Flett AS, Hasleton JM, Quarta G, Hausenloy D, Muthurangu V, Moon JC. The full width half maximum technique is superior for LGE quantification regardless of its aetiology. J Cardiovasc Magn Reson. (2010) 12(Suppl 1.):O41. doi: 10.1186/1532-429X-12-S1-O41
14. Luetkens JA, Doerner J, Schwarze-Zander C, Wasmuth J-C, Boesecke C, Sprinkart AM, et al. Cardiac magnetic resonance reveals signs of subclinical myocardial inflammation in asymptomatic HIV-infected patients. Circ Cardiovasc Imaging. (2016) 9:e004091. doi: 10.1161/CIRCIMAGING.115.004091
15. Kravchenko D, Isaak A, Zimmer S, Mesropyan N, Reinert M, Faron A, et al. Cardiac MRI in patients with prolonged cardiorespiratory symptoms after mild to moderate covid-19. Radiology. (2021) 301:E419–25. doi: 10.1148/radiol.2021211162
16. Luetkens JA, Homsi R, Sprinkart AM, Doerner J, Dabir D, Kuetting DL, et al. Incremental value of quantitative CMR including parametric mapping for the diagnosis of acute myocarditis. Eur Heart J Cardiovasc Imaging. (2016) 17:154–61. doi: 10.1093/ehjci/jev246
17. Cerqueira MD, Weissman NJ, Dilsizian V, Jacobs AK, Kaul S, Laskey WK, et al. Standardized myocardial segmentation and nomenclature for tomographic imaging of the heart. A statement for healthcare professionals from the cardiac imaging committee of the council on clinical cardiology of the American heart association. Circulation. (2002) 105:539–42. doi: 10.1161/hc0402.102975
18. Ahmed SK. Myocarditis after BNT162b2 and mRNA-1273 covid-19 vaccination: a report of 7 cases. Ann Med Surg. (2022) 77:103657. doi: 10.1016/j.amsu.2022.103657
19. Evertz R, Schulz A, Lange T, Backhaus SJ, Vollmann D, Kowallick JT, et al. Cardiovascular magnetic resonance imaging patterns of acute covid-19 mRNA vaccine-associated myocarditis in young male patients: a first single-center experience. Front Cardiovasc Med. (2022) 9:965512. doi: 10.3389/fcvm.2022.965512
20. Abellan C, Vivekanantham H, Mahmoudi A, Cook S, Doll S, Arroyo D. A case series of acute myocarditis associated with SARS-CoV-2 mRNA vaccination. Cardiovasc Med. (2021) 24:w10103.
21. Diaz GA, Parsons GT, Gering SK, Meier AR, Hutchinson IV, Robicsek A. Myocarditis and pericarditis after vaccination for covid-19. JAMA. (2021) 326:1210–2. doi: 10.1001/jama.2021.13443
22. Bautista García J, Peña Ortega P, Bonilla Fernández JA, Cárdenes León A, Ramírez Burgos L, Caballero Dorta E. Acute myocarditis after administration of the BNT162b2 vaccine against covid-19. Rev Esp Cardiol. (2021) 74:812–4. doi: 10.1016/j.rec.2021.04.005
23. Isaak A, Feisst A, Luetkens JA. Myocarditis following covid-19 vaccination. Radiology. (2021) 301:E378–9. doi: 10.1148/radiol.2021211766
24. Jain SS, Steele JM, Fonseca B, Huang S, Shah S, Maskatia SA, et al. Covid-19 vaccination-associated myocarditis in adolescents. Pediatrics. (2021) 148:e2021053427. doi: 10.1542/peds.2021-053427
25. Kim HW, Jenista ER, Wendell DC, Azevedo CF, Campbell MJ, Darty SN, et al. Patients with acute myocarditis following mRNA covid-19 vaccination. JAMA Cardiol. (2021) 6:1196–201. doi: 10.1001/jamacardio.2021.2828
26. Larson KF, Ammirati E, Adler ED, Cooper LT Jr, Hong KN, Saponara G, et al. Myocarditis after BNT162b2 and mRNA-1273 vaccination. Circulation. (2021) 144:506–8. doi: 10.1161/CIRCULATIONAHA.121.055913
27. Marshall M, Ferguson ID, Lewis P, Jaggi P, Gagliardo C, Collins JS, et al. Symptomatic acute myocarditis in 7 adolescents after Pfizer-BioNTech covid-19 vaccination. Pediatrics. (2021) 148:e2021052478. doi: 10.1542/peds.2021-052478
28. Montgomery J, Ryan M, Engler R, Hoffman D, McClenathan B, Collins L, et al. Myocarditis following immunization with mRNA covid-19 vaccines in members of the US Military. JAMA Cardiol. (2021) 6:1202–6. doi: 10.1001/jamacardio.2021.2833
29. Abu Mouch S, Roguin A, Hellou E, Ishai A, Shoshan U, Mahamid L, et al. Myocarditis following covid-19 mRNA vaccination. Vaccine. (2021) 39:3790–3. doi: 10.1016/j.vaccine.2021.05.087
30. Perez Y, Levy ER, Joshi AY, Virk A, Rodriguez-Porcel M, Johnson M, et al. Myocarditis following coronavirus disease 2019 mRNA vaccine: a case series and incidence rate determination. Clin Infect Dis. (2022) 75:e749–54. doi: 10.1093/cid/ciab926
31. Rosner CM, Genovese L, Tehrani BN, Atkins M, Bakhshi H, Chaudhri S, et al. Myocarditis temporally associated with covid-19 vaccination. Circulation. (2021) 144:502–5. doi: 10.1161/CIRCULATIONAHA.121.055891
32. Shaw KE, Cavalcante JL, Han BK, Gössl M. Possible association between covid-19 vaccine and myocarditis. JACC. (2021) 14:1856–61. doi: 10.1016/j.jcmg.2021.06.002
33. Bozkurt B, Kamat I, Hotez PJ. Myocarditis with covid-19 mRNA vaccines. Circulation. (2021) 144:471–84. doi: 10.1161/CIRCULATIONAHA.121.056135
34. Heymans S, Cooper LT. Myocarditis after covid-19 mRNA vaccination: clinical observations and potential mechanisms. Nat Rev Cardiol. (2022) 19:75–7. doi: 10.1038/s41569-021-00662-w
35. Fronza M, Thavendiranathan P, Karur GR, Abdel-Qadir H, Udell JA, Wald RM, et al. Cardiac MRI and clinical follow-up in covid-19 vaccine-associated myocarditis. Radiology. (2022) 304:E48–9. doi: 10.1148/radiol.220802
36. Fischer K, Obrist SJ, Erne SA, Stark AW, Marggraf M, Kaneko K, et al. Feature tracking myocardial strain incrementally improves prognostication in myocarditis beyond traditional CMR imaging features. JACC Cardiovasc Imaging. (2020) 13:1891–901. doi: 10.1016/j.jcmg.2020.04.025
37. Isaak A, Kravchenko D, Mesropyan N, Endler C, Bischoff LM, Vollbrecht T, et al. Layer-specific strain analysis with cardiac MRI feature tracking in acute myocarditis. Radiol Cardiothorac Imaging. (2022) 4:e210318. doi: 10.1148/ryct.210318
38. Uziȩbło-Życzkowska B, Mielniczuk M, Ryczek R, Krzesiński P. Myocarditis successfully diagnosed and controlled with speckle tracking echocardiography. Cardiovasc Ultrasound. (2020) 18:19. doi: 10.1186/s12947-020-00203-4
39. Korosoglou G, Giusca S, Hofmann NP, Patel AR, Lapinskas T, Pieske B, et al. Strain-encoded magnetic resonance: a method for the assessment of myocardial deformation. ESC Heart Fail. (2019) 6:584–602. doi: 10.1002/ehf2.12442
40. Korosoglou G, Giusca S, Montenbruck M, Patel AR, Lapinskas T, Götze C, et al. Fast strain-encoded cardiac magnetic resonance for diagnostic classification and risk stratification of heart failure patients. JACC Cardiovasc Imaging. (2021) 14:1177–88. doi: 10.1016/j.jcmg.2020.10.024
41. Neizel M, Lossnitzer D, Korosoglou G, Schäufele T, Peykarjou H, Steen H, et al. Strain-encoded MRI for evaluation of left ventricular function and transmurality in acute myocardial infarction. Circ Cardiovasc Imaging. (2009) 2:116–22. doi: 10.1161/CIRCIMAGING.108.789032
42. Luetkens JA, Schlesinger-Irsch U, Kuetting DL, Dabir D, Homsi R, Doerner J, et al. Feature-tracking myocardial strain analysis in acute myocarditis: diagnostic value and association with myocardial oedema. Eur Radiol. (2017) 27:4661–71. doi: 10.1007/s00330-017-4854-4
43. Ammirati E, Frigerio M, Adler ED, Basso C, Birnie DH, Brambatti M, et al. Management of acute myocarditis and chronic inflammatory cardiomyopathy: an expert consensus document. Circ Heart Fail. (2020) 13:e007405. doi: 10.1161/CIRCHEARTFAILURE.120.007405
44. Casella M, Bergonti M, Narducci ML, Persampieri S, Gasperetti A, Conte E, et al. Prior myocarditis and ventricular arrhythmias: the importance of scar pattern. Heart Rhythm. (2021) 18:589–96. doi: 10.1016/j.hrthm.2020.12.016
45. Pandozi C, Mariani MV, Chimenti C, Maestrini V, Filomena D, Magnocavallo M, et al. The scar: the wind in the perfect storm—insights into the mysterious living tissue originating ventricular arrhythmias. J Interv Card Electrophysiol. (2022) 1–12. [Epub ahead of print]. doi: 10.1007/s10840-021-01104-w
46. Gräni C, Eichhorn C, Bière L, Murthy VL, Agarwal V, Kaneko K, et al. Prognostic value of cardiac magnetic resonance tissue characterization in risk stratifying patients with suspected myocarditis. J Am Coll Cardiol. (2017) 70:1964–76. doi: 10.1016/j.jacc.2017.08.050
Keywords: cardiac magnetic resonance, myocarditis, COVID-19, vaccination, follow-up
Citation: Kravchenko D, Isaak A, Mesropyan N, Bischoff LM, Pieper CC, Attenberger U, Kuetting D, Zimmer S, Hart C and Luetkens JA (2022) Cardiac magnetic resonance follow-up of COVID-19 vaccine associated acute myocarditis. Front. Cardiovasc. Med. 9:1049256. doi: 10.3389/fcvm.2022.1049256
Received: 20 September 2022; Accepted: 24 October 2022;
Published: 09 November 2022.
Edited by:
Grigorios Korosoglou, GRN-Klinik Weinheim, GermanyReviewed by:
Dominik Buckert, Ulm University Medical Center, GermanyChristoph Gräni, Universitätsspital Bern, Switzerland
Moritz Montenbruck, Katholisches Marienkrankenhaus GmbH, Germany
Copyright © 2022 Kravchenko, Isaak, Mesropyan, Bischoff, Pieper, Attenberger, Kuetting, Zimmer, Hart and Luetkens. This is an open-access article distributed under the terms of the Creative Commons Attribution License (CC BY). The use, distribution or reproduction in other forums is permitted, provided the original author(s) and the copyright owner(s) are credited and that the original publication in this journal is cited, in accordance with accepted academic practice. No use, distribution or reproduction is permitted which does not comply with these terms.
*Correspondence: Julian A. Luetkens, anVsaWFuLmx1ZXRrZW5zQHVrYm9ubi5kZQ==
†ORCID: Dmitrij Kravchenko, orcid/0000-0003-0331-2045